1. Introduction
Atomic Force Microscopy (AFM) is an interesting tool to investigate live cells, and provides accurate small force measurements (F) combined with piezo nanometric displacements that can be converted into indentation values (d). This provides classical curves F(d) allowing to measure the cell elastic (or Young) modulus E using Hertz model, this being done routinely on a flat – or not too curved – surface to obtain elastic mappings. This technique has proved valuable for the determination of elasticity change with cell type, or invasiveness (Lekka et al. Citation1999; Cross et al. Citation2008). But AFM can be used in different modes for example force modulation data allows to obtain viscoelasticity. Earlier works showed how to construct mappings (Radmacher et al. Citation1993) and more refined AFM viscoelasticity data was obtained as a function of frequency (Alcaraz et al. Citation2003; Abidine et al. Citation2015a, Citation2015b). This frequency dependence is important as it can outline different cell responses corresponding to the measurement location, and underlying cytoskeleton. Cells indeed have rich spatio-temporal rheological responses, depending on the environment. This is what will be considered in this work, by studying cells spread on various elastic substrates. We will show that, after correct interpretation of the data (Abidine et al. Citation2015b, Abidine et al. Citation2018), we can exhibit interesting cell viscoelastic behaviors depending on the substrate (gel or cell monolayer). This will be applied to bladder cancer cells of different invasiveness.
2. Methods
2.1. Microrheology: principle
The AFM principle is briefly explained below and the systems studied range from classical materials to biological samples (cells and tissues). When indenting a sample, the force (F0) – measured using the laser reflection on the back of the cantilever – is related to the indentation (d0) by F0 = 3E tanq d02/4/(1-n2) when using a pyramidal cantilever (q half-angle of the pyramid). E and n are the soft material parameters, respectively
Young modulus and Poisson coefficient. This relation is linearized as oscillations (of small amplitude d) at frequency f are superposed to this initial indentation d0. The result gives a modified relation for the shear modulus G*(w)=G’(w)+iG’’(w)=(1-n)/3/d0/tanq{F*/d*}, where F* is the complex force and d* the complex displacement. Thus one obtains (Alcaraz et al. Citation2003, Abidine et al. Citation2015a) the elastic (G’) and viscous components (G’’) as functions of the angular frequency w = 2pf (where f is the frequency in Hz).
2.2. Corrections
A first correction is to be made due to the fact that oscillations in a liquid – cells are within a culture medium – create a drag than needs to be determined. This involves calibrating at different distances which are extrapolated when the cantilever gets close to the cell (Alcaraz et al. Citation2003).
Another difficulty is often encountered as cells or gels are thin layers and this effect is to be accounted for when the substrate is hard, as done previously (Dimitriadis et al. Citation2002). But this problem also appears when cells are lying on soft substrates, as it is often the case in real biology. On soft substrates, moduli are underestimated, thus specific methods need to be used to account for such effects (Abidine et al. Citation2018).
3. Results and discussion
3.1. Changing the environment
The epithelial bladder cancer cells studied here have different invasiveness ranging from low grade to higher grade (RT112 < T24 < J82). They were plated on functionalized polyacrylamide (PA) gels of different stiffnesses, and exhibited different surface areas, also an endothelial substrate was used (). Cells were tested in the AFM force modulation mode with frequencies ranging from 1 Hz to 500 Hz and exhibited different behaviors. Note that measurements at different locations (N, P or E) also emphasized the variability of the viscoelastic parameters due to cytoskeleton organization as previously reported (Abidine et al. Citation2015b).
Figure 1. T24 cancer cell on a 28 kPa gel (measurement locations are N, P and E) and on HUVEC monolayer. Scale bar = 10µm. (Abidine et al. Citation2018).
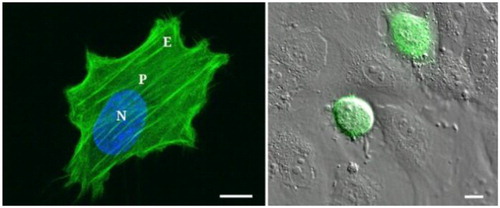
3.2. Role of cell invasiveness
AFM measurements were made on the previously described bladder cancer cells and show the obvious role of invasiveness (as in Cross et al. Citation2008) that decreases both elastic and viscous moduli in a dramatic way on gels. On the other hand, we find an increase of the complex modulus |G*| with invasiveness when cells are lying on a HUVEC monolayer (). The data of G’(f) and G”(f) in shows an almost power law dependence in terms of f. Fitting of the data can be made using a simple viscoelastic model depending on a few parameters, in particular the elastic plateau modulus GN0 and the transition frequency fT (Abidine et al. Citation2015b). fT is a important parameter giving information on the transition from the elastic and the glassy state and low values are typical of a less organized cytoskeleton, notable for invasive cancer cells.
Figure 2. G’(f), G”(f) for J82 cell on a HUVEC monolayer (position P). Corresponding |G*|(1.4 Hz) for 3 cancer cells. The raw data is in black. The data corrected for soft substrate effects is in red (Abidine et al. Citation2018).
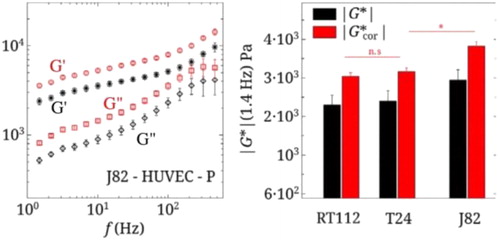
4. Conclusions
By developing this AFM-based microrheology technique, information on the viscoelastic behavior can be reported and compared for cells of various invasiveness on different substrates and at different cell locations. This information can be coupled with viscoelastic models to extract parameters related to cell viscoelasticity. These parameters have been tested further in more biological situations such as cell transmigration across the endothelium (Abidine et al. Citation2018) and reveal possible cytoskeletal reorganization processes, in agreement with cell viscoelastic properties.
This opens up a new methodology to explain physiological or pathological mechanisms occuring in other biological processes.
Additional information
Funding
References
- Abidine Y, Constantinescu A, Laurent VM, Rajan VS, Michel R, Laplaud V, Duperray A, Verdier C. 2018. Mechanosensitivity of cancer cells in contact with soft substrates using AFM. Biophys J. 114(5):1165–1175.
- Abidine Y, Laurent V, Michel R, Duperray A, Verdier C. 2015b. Local mechanical properties of bladder cancer cells measured by AFM as a signature of metastatic potential. Eur Phys J Plus. 130:202.
- Abidine Y, Laurent V, Michel R, Duperray A, Palade L, Verdier C. 2015a. Physical properties of polyacrylamide gels probed by AFM and rheology. EPL. 109(3):38003.
- Alcaraz J, Buscemi L, Grabulosa M, Trepat X, Fabry B, Farré R, Navajas D. 2003. Microrheology of human lung epithelial cells measured by atomic force microscopy. Biophys J. 84(3):2071–2079.
- Cross SE, Jin YS, Tondre J, Wong R, Rao J, Gimzewski JK. 2008. AFM-based analysis of human metastatic cancer cells. Nanotechnology. 19(38):384003.
- Dimitriadis EK, Horkay F, Maresca J, Kachar B, Chadwick RS. 2002. Determination of elastic moduli of thin layers of soft material using the atomic force microscope. Biophys J. 82(5):2798–2810.
- Lekka M, Laidler P, Gil D, Lekki J, Stachura Z, Hrynkiewicz AZ. 1999. Elasticity of normal and cancerous human bladder cells studied by scanning force microscopy. Eur Biophys J. 28(4):312–316.
- Radmacher M, Tillmann RW, Gaub HE. 1993. Imaging viscoelasticity by force modulation with the atomic force microscope. Biophys J. 64(3):735–742.