1. Introduction
Glioblastoma multiforme (GBM) is the most devastating malignant brain tumor with median survival of three months in untreated patients (Malmström et al. Citation2012). Under therapy, even with most aggressive regimen, the prognosis of GBM patients remains poor, with a median survival of 15 months, and 5-year survival < 10% (Davis Citation2016).
Although MRI is the gold standard for GBM diagnosis and treatment follow-up, it is not sensitive to tumor infiltration and cannot properly discriminate treatment response and immune activities from tumor progression, leading to clinical uncertainties like pseudo-response and pseudo-relapse.
Magnetic Resonance Elastography (MRE) is a non- invasive technique that remotely quantifies the complex shear modulus G* = Gd + i Gl of the tissues, e.g., in the brain (Simon et al. Citation2013). To obtain such information, a continuous periodic vibration in the organ of interest is induced by means of an external transducer placed in contact with the patient. During the vibration, a modified MR sequence encodes the displacement in each voxel (Muthupillai et al. Citation1995), and this data is then used to locally invert the complex wave equation (Garteiser et al. Citation2013). Therefore, many different parameters may affect the quality and accuracy of the resulting mechanical information. For instance, it was previously shown that inadequate ratios between the vibration wavelength and the voxel size may lead to inaccurate estimation of stiffness even in homogeneous regions (Honarvar et al. Citation2016).
The main goal of this preliminary study is to define and optimize an MRE protocol for GBM patients that provides meaningful and robust data while preserving patient’s comfort. The performance and possible limitations of the protocol will set the stage for our clinical study.
2. Methods
Numerical simulations were performed with COMSOL Multiphysics© (COMSOL AB, Stockholm, Sweden) to produce displacement fields in ideal conditions (i.e., without noise and reflections) in objects with ground-truth G* distributions (). The latter synthetic MRE data was used to investigate the accuracy of the G* maps with the same processing pipeline used for experimental data reconstruction (Garteiser et al. Citation2013). Error on the estimated object sizes was obtained from the mean ± SD FWHM calculated from 8 line-profiles drawn across each object.
Figure 1. (A) Simulation: ideal Gd distribution at 1 mm resolution (a) & the reconstructed Gd maps, for an isotropic resolution of 3 mm (b), 2 mm (c) & 1 mm (d). The diameter of the inclusions varies from 30 mm to 6 mm, a single frequency of 50 Hz was simulated. (B) Simulation results: error on the estimated object size calculated from the mean ± SD FWMH obtained along 8 line profiles crossing each object.
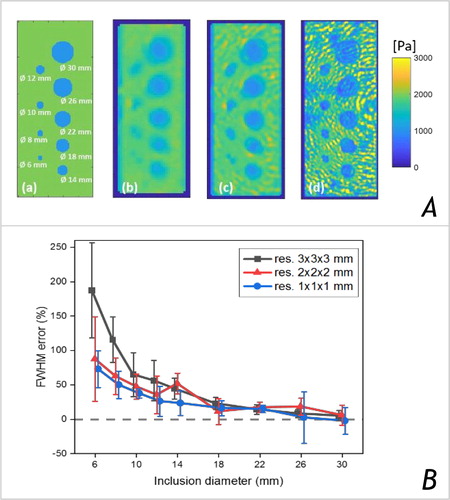
MRE was performed on a clinical 3T scanner using a fractional encoding gradient echo-based sequence (Garteiser et al. Citation2013). The vibration was produced by a rotating-mass transducer positioned on one side of the head, and connected to a mechanical driver via a rotating axis (Runge et al. Citation2019).
Protocol optimization was performed on volunteers with the aim to address trade-offs between the acquisition time, patient’s comfort, and robustness.
Comfort was evaluated using different rotating mass sizes and a 3D-printed holder for improved coupling/positioning between the head and the transducer inside the MRI detector. The optimum transducer positioning and the induced wave quality were assessed from the waves’ amplitude in the brain, the absence of contribution from frequencies different from the induced vibration, as well as the degree of induced shear waves.
In the optimized conditions and considering our experimental constraints, the MRE protocol was set as follows: 50 Hz vibration, 60 mT/m Motion Encoding Gradient (MEG) intensity, 3 mm isotropic voxel size, 192x192 mm field-of-view, 9 slices, for a total acquisition time of 200 s (3 encoded directions and a reference scan with MEG set to zero).
3. Results and discussion
Our simulations showed that Gd values are correctly reconstructed within a uniform object (, b-d). However, the assumption of mechanical homogeneity along with 3rd derivatives calculations produced blurred contours at interfaces, which was more prominent in the case of smaller objects or larger voxel size, leading to errors >50% in the estimated sizes for objects ≤14mm (see ).
The 3D-printed holder improved the coupling between the head and transducer, wave quality, and comfort as reported by our volunteers (results not shown). The current protocol provides good wave amplitude inside the entire brain that allows for successful image reconstruction while maintaining comfort and short acquisition times. shows typical data (often called ‘‘elastograms’’) obtained with our MRE protocol.
4. Conclusions
Our protocol provides good wave amplitude inside the brain allowing for successful image reconstruction while maintaining short acquisition times and comfort. As highlighted by our simulations, our protocol enables mapping Gd with confidence in objects larger than 14 mm. This, however, needs to be fully characterized in a larger cohort of volunteers so that clinicians can read the elastograms with certainty. Once this step is validated, the diagnostic performances of MRE in discriminating between tumors’ progression or response to treatments will be assessed.
Additional information
Funding
References
- Davis ME. 2016. Glioblastoma: overview of disease and treatment. Clin J Oncol Nurs. 20:1–14.
- Garteiser P, Sahebjavaher RS, Ter Beek LC, Salcudean S, Vilgrain V, Van Beers BE, Sinkus R. 2013. Rapid acquisition of multifrequency, multislice and multidirectional MR elastography data with a fractionally encoded gradient echo sequence. NMR Biomed. 26(10):1326–1335.
- Honarvar M, Rohling R, Salcudean SE. 2016. A comparison of direct and iterative finite element inversion techniques in dynamic elastography. Phys Med Biol. 61(8):3026–3048.
- Malmström A, Grønberg BH, Marosi C, Stupp R, Frappaz D, Schultz H, Abacioglu U, Tavelin B, Lhermitte B, Hegi ME, et al. 2012. Temozolomide versus standard 6-week radiotherapy versus hypofractionated radiotherapy in patients older than 60 years with glioblastoma: The Nordic randomised, phase 3 trial. Lancet Oncol. 13(9):916–926.
- Muthupillai R, Lomas D, Rossman P, Greenleaf J, Manduca A, Ehman R. 1995. Magnetic resonance elastography by direct visualization of propagating acoustic strain waves. Science (80-). 269(5232):1854–1857.
- Runge JH, Hoelzl SH, Sudakova J, Dokumaci AS, Nelissen JL, Guenthner C, Lee J, Troelstra M, Fovargue D, Stoker J, et al. 2019. A novel magnetic resonance elastography transducer concept based on a rotational eccentric mass: preliminary experiences with the gravitational transducer. Phys Med Biol. 64(4):045007.
- Simon M, Guo J, Papazoglou S, Scholand-Engler H, Erdmann C, Melchert U, Bonsanto M, Braun J, Petersen D, Sack I, et al. 2013. Non-invasive characterization of intracranial tumors by magnetic resonance elastography. New J Phys. 15.