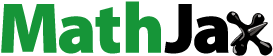
1. Introduction
Due to the growth of Work-related Musculoskeletal Disorders especially during manual handling tasks, researches are now focusing on the development of new solution such as exoskeleton to enhance and/or assist human motion (Looze et al. 2015).
Exoskeletons are considered as collaborative robots even if they are passive (using non-powered option).
In the medical field, active exoskeletons are well studied to assist patients during rehabilitation and medical surgery (Jarrassé et al. Citation2014; Maciejasz et al. Citation2014). Due to their low prices and attractiveness, the use of passive exoskeleton in the field of manual handling shows an important growth during the last few years. Several studies focused on perceived discomfort and electromyography (EMG) (Huysamen et al. Citation2018; Saad et al. 2019) show a significant decrease on EMG activity (Bosch et al. Citation2016; Theurel et al. Citation2018). However, these studies did not consider the kinematic aspect and have somehow ignored the detrimental impact that could have an exoskeleton on the user’s motion.
As a result, the design and the use of exoskeletonsmust comply with the new international standard ISO-10218 (International Standard 2011). However, researchers have not been able to achieve a common consensus to evaluate the contribution of these new solutions.
The purpose of the present work is then to establish an experimental protocol to assess and analyse the level of transparency assistance during the use of a passive exoskeleton.
2. Methods
2.1 Experimental tasks
One healthy subject (Age: 28 years, Mass: 90 kg, Height: 1,84 m) participated in this preliminary study.
He performed three different tasks with and without the assistance of a passive exoskeleton.1) Up/Down/up task: consists on lifting a load from a platform (h = 80 cm) to the ground, and from the ground to the platform. 2) Walking task: The participant had to walk over a distance of 7 m at a free chosen speed, carrying a two -handled box. 3) Static task: The subject was instructed to stand still while holding a box. Four boxes were carried during the tasks: two “Heavy” (Mass = 20 kg, 35 x 35 x 24 cm) and two “Light” (Mass = 10 kg, 30 x 24 x 24 cm). The subject was free to work at his own pace.
2.2 Data recording
2.2.1 Kinematics
50 reflective markers (2.5 cm ∅) were fixed on the subject to identify 15 segments. The 3-D kinematics of the upper limb, lower limb, and the trunk was then recorded by 38 cameras Vicon Motion Systems Ltd at the frequency of 100 Hz.
Based on 3 D markers positions we define segment’s reference frames to estimate 3 D joints angles.
2.2.2 EMG
Due to their contribution during manual handling of loads (Nielsen et al. Citation1998), the electromyographic activity of the biceps brachii, the lateral deltoid, the middle fibers of the trapezius and the erector spinae longissimus were recorded using a MyoResearch XP Master Ed. Software (Noraxon USA 2018).
Before placing the electrodes, the skin was shaved, abraded and cleaned following the SENIAM recommendations. For each muscle, a pair of bipolar Ag/Ag-Cl electrodes; with a 20 mm inter-electrode distance; were placed on the skin in order to reduce the impedance.
EMG signals were monitored at 1500 Hz, filtered with at 20-500 Hz Bandpass, rectified the signal and obtained the RMS envelope. Then the signal was smoothed with the Savitsky-Golay filter.
2.2.3 Postural balance
One force plate (AMTI) was used to asses postural balance criteria. Anteroposterior (Xi) and sagittal (Yi) displacement are used to compute the length of the displacement (D) and the surface (S) of the ellipse which covers 90% of the data as follow:
3. Results and discussion
3.1 Kinematics
The below shows an example of the kinematic modification during an Up/Down/Up task for four joints. RULA assessment tool was used to evaluate the exposure of the subject to ergonomic risk factors and the effect of the exoskeleton on its motion.
It seems that the use of the exoskeleton does not affect the motion of the user during the Up/Down/Up task. In the same way, we found that this exoskeleton does not interfere with the walking task. We can than hypotheses that this exoskeleton is transparent.
3.2 EMG
below represents the EMG activity of the equivalent muscle (the sum of the 8 muscles) during the Up/Down/Up task with and without the use of the exoskeleton. It is clearly shown that the use of this exoskeleton reduces EMG activity during the handling of the four boxes. Furthermore, this funding is supported by the results of the walking task and the postural balance task.
Based on the decrease of EMG activities, we can hypothesize that the use of this exoskeleton allows assistance to the worker.
3.3 Postural balance
Based on the process of the force platform data, the results show that the use of this exoskeleton does not modify the postural balance. It was hypothesized that a transparent device should not increase postural strains and must maintain the same postural balance all over the task.
4. Conclusions
The main purpose of this study was to establish a reproducible and accurate protocol to estimate the level of the transparency assistance of a passive exoskeleton. Based on the kinematics, the EMG and the postural balance this protocol allow a biomechanical feedback of the exoskeleton use effect. However, the user’s level of knowledge and experience could alter the results. In order to verify these hypotheses (transparency, assistance, experience), this study will be extended to a larger population.
Acknowledgments
The authors report no conflicts of interest in this work.
References
- Bosch T, van Eck J, Knitel K, de Looze M. 2016. The effects of a passive exoskeleton on muscle activity, discomfort and endurance time in forward bending work. Appl Ergon. 54:212–217.
- Huysamen K, Bosch T, de Looze M, Stadler K S, Graf E, O'Sullivan L W. 2018. Evaluation of a passive exoskeleton for static upper limb activities. Appl Ergon. 70:148–155.
- International Standard. 2011. Robots ans robotics devices-Safety requirements for industrial robots. ISO 10218-1:2011(E).
- Looze MPD, Bosch T, Krause F, Stadler KS, O’Sullivan LW. 2015. Exoskeletons for industrial application and their potential effects on physical workload. Ergonomics. 59(5):671–681.
- Jarrassé N, Proietti T, Crocher v, Robertson J, Sahbani A, Morel G, Roby-Brami A. 2014. Robotic exoskeletons: a perspective for the rehabilitation of arm coordination in stroke patient. Front Hum Neurosci.
- Maciejasz P, Eschweiler J, Gerlach-Hahn K, Jansen-Troy A, Leonhardt S. 2014. A survey on robotic devices for upper limb rehabilitation. J NeuroEng Rehabil. 11(1):3.
- Nielsen PK, Andersen L, Jørgensen K. 1998. The muscular load on the lower back and shoulders due to lifting at different lifting heights and frequencies. Appl Ergon. 29(6):445–450.
- Theurel J, Desbrosses K, Roux T, Savescu A. 2018. Physiological consequences of using an upper limb exoskeleton during manual handling tasks. Appl Ergon. 67:211–217.