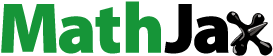
Keywords:
1. Introduction
Biomechanical modelling of human breast tissues has been widely studied for various medical applications such as surgical procedure training, pre-operative planning, diagnosis and clinical biopsy or image-guided surgery. Most research teams have designed subject-specific Finite Element (FE) models that are, for example, used to simulate compressions between plaques during mammography or tissue deformations due to needle biopsies. Such FE models usually include various breast tissues (skin, mammary gland, adipose tissue and pectoral muscles). Mira and colleagues showed the importance of also including some suspensory ligaments, the superficial and deep fascia in these FE models (Mira et al. Citation2018). Among the suspensory ligaments, the net of Cooper's ligaments (Gaskin et al. Citation2019) probably plays a role in the global deformation of the breast. However, to the better of our knowledge, these ligaments have never been included in any biomechanical model. This is probably due to the fact that the constitutive behaviour of such ligaments has never been investigated, because (1) of the difficulty to extract them from a specimen and (2) of their extreme fragility once extracted, which makes any experimental characterisation complicated. This paper addresses this issue with samples of Cooper’s ligament that were extracted and tested ex vivo in uniaxial tension. The results of the tests were fitted with a hyperelastic constitutive equation. The variations of the parameters of that equation are highlighted.
2. Methods
2.1. Cooper’s ligaments specimens
Cooper's ligaments are described as a three-dimensional net containing the fatty tissue and the mammary gland. They are attached to the dermis and pectoral muscle and merge on fascia layers as oppose to other ligaments present in joints attached between two muscles. To sample Cooper's ligaments on a cadaver, the technique consists in making a lateral incision at the level of the ribs until reaching the pectoral muscles and thus being able to access the breast from below going up toward the dermis. Our sampling of Cooper's ligaments was distributed over the entire breast volume. Sizes of samples varied between 13.9 mm and 15.1 mm long, 1.4 mm and 4.9 mm wide and 0.04 mm and 0.3 mm thick. Each sample was cut in the direction of the main fibres visible to the naked eye. The sample should be cut in such a way to respect the dimensions of the uniaxial tensile specimens as well as possible, by respecting a ratio of approximately four between the length and the width of the sample. The uniaxial tensile tests were performed on an MTS model C42.503 machine with 25 N sensors (see panel on ) with grips made to prevent the sample from sliding. To reproduce physiological conditions, each sample was placed on the tensile machine with a bath filled with saline heated to a temperature of 37 °C (Masri et al. Citation2018). A tensile fracture test was performed on each sample.
3. Results and discussion
shows the results of the uniaxial tensile tests performed on 28 Cooper’s ligament samples. The amplitudes of rupture stress and rupture strain during these tests are plotted on that figure. A relatively strong dispersion can be observed. The rupture stresses range from 250 kPa to 13 MPa with an average of 2.0 MPa. Rupture strains range from 1% to 16% with an average of 8.6%.
The assumption of a homogenous strain of the sample was made. The experimental curves present a horizontal step before a strain hardening. We propose to use a Neo-Hookean model to describe the horizontal step and to use a power function to describe the hardening (Jemiolo et al. Citation2001), thus assuming the strain-energy density function W with:
(1)
(1)
where I1 is the first invariant of the left Cauchy–Green tensor. The model has three parameters, C1 describing the initial slope and C2 and C3 describing the hardening. For each curve, an optimization process estimates the values of these three parameters to fit the experimental data.
provides the range of variation of the three parameters estimated from experimental data on the 28 samples. The C1 parameter varies between 230 kPa and 2.5 MPa, with a median of 710 kPa, 50% of the population being between 480 kPa and 1.2 MPa. The C2 parameter varies between 1.3 MPa and 110 MPa, with a median of 16 MPa, 50% of the population being between 7.6 MPa and 33 MPa. The C3 parameter varies between 1.4 and 2.2, with a median of 1,7, 50% of the population being between 1.5 and 1.7.
gathers the average, maximal, minimal and standard deviation values for the rupture strain, rupture stress and Young modulus estimated from all the tests. The Young modulus was computed as E = 6×C1 (assuming ligaments as quasi-incompressible).
Table 1. Summary of the mechanical values estimated from the 28 experimental tests.
The first point () is the high values for the equivalent Young modulus of Cooper’s ligaments (2–3 magnitude order stiffer than most breast tissue) but still less stiff than other ligaments such as knee ligament. The other point is the data dispersion (high standard deviations) observed on the experimental curves. This can be explained in several ways: the freshness of the tissue (the cadaver conserved in formalin), errors in section measurements (samples sectioned by hand) or the various location of the tissue.
However, this is to our knowledge the first time that many samples of Cooper’s ligaments were extracted and experimentally characterised by a tensile test.
4. Conclusions
Uniaxial tensile tests were performed on 28 Cooper’s ligaments of a single cadaver. A wide dispersion of the results was observed for the rupture stress, the rupture strain and the Young modulus. Surprisingly, the stiffness of these Cooper’s ligament is two to three orders of magnitude higher than the stiffness of most breast soft tissue.
An original constitutive law was proposed to model the hyperelastic behaviour of the ligaments with an accurate approximation of the stress-strain curve during the uniaxial traction.
As a perspective, other mechanical tests such as plane traction or channel compression could be used to check the behaviour of the model in these different cases of stress. Especially the anisotropy of Cooper’s ligaments will have to be investigated.
Disclosure statement
No potential conflict of interest was reported by the authors.
References
- Gaskin K, Peoples G, McGhee D. 2019. The fibro-adipose structure of the female breast: a dissection study. Wollongong, New South Wales, Australia: Anatomy Laboratory, University of Wollongong.
- Jemiolo S, Telega J. 2001. Transversely isotropic materials undergoing large deformations and application to modelling soft tissues. Mech Res Commun. 28:397–404.
- Masri C, Chagnon G, Favier D, Sartelet H, Girard E. 2018. Experimental characterization and constitutive modeling of the biomechanical behavior of male human urethral tissues validated by histological observations. Grenoble, France. Biomech Model Mechanobiol. 17(4):939–950.
- Mira A. 2018. Modélisation biomécanique du sein pour l'évolution de la compression et de la perception d'inconfort en mammographie. Grenoble: Université Grenoble Alpes.