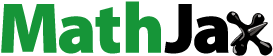
1. Introduction
The numerical approach of topological optimization allows determining the optimal distribution of the material in a given volume in the function of an objective. This approach has recently regained interest thanks to the development of additive manufacturing, which makes it possible to manufacture its resulting parts with complex geometries. In the biomedical field, this numerical approach can also improve the performance of medical devices. Most of the time, the objective of topological optimization is to maximize the stiffness of the considered part while reducing its mass or volume. For example, this criterion has been applied for the optmisation of craniofacial reconstruction prosthesis (Al-tamimi et al. Citation2017). Some studies have integrated biomechanical considerations in the form of geometrical constraints in taking into account anatomical areas to be avoided (Sutradhar et al. Citation2016).
To our knowledge, no study considers the phenomenon of “stress-shielding” between the bone and the implant in its optimization process. In this work, the originality is the optimization approach which does not only consider the mechanical characteristics of the optimized part (such as its stiffness) but also the mechanical characteristics of the surrounding bone. Thus, it involves specifically taking into account the mechanical behavior of the surrounding bone to topologically optimize the geometry of the medical device and improve the load transfer between the bone and the implant. For that, a numerical simplified Finite Element (FE) model is developed in this paper with a simplified geometry to highlight the possibility of controlling the load transfer bone and implant.
2. Methods
2.1. Topology optimization numerical strategy
The most used topological optimization method was adopted in this study, which defines a pseudo-density of the material related to its stiffness by the SIMP (Solid Isotropic Material with Penalization) extrapolation method (Bendsøe and Sigmund Citation2003). The sensitivity-based algorithm is applied, based on the MMA (Moving Asymptom Method), to solve the optimization problem.
Consideration of the bone and the stress-shielding phenomenon leads to reconsider the use of the topological optimization method. The idea presented in this paper is to dissociate the design domain, that is to say, the optimized part volume, and the domain where the mechanical variables that will build the objective function are considered. The topology of the implant is optimized by considering one or more variables associated with the bone, to control the transfer of load at the bone-implant interface and the loading applied to the bone.
It is chosen to use local variables of internal nodal force in the bone. This variable corresponds to the reaction force of one (or more) element on a node of the mesh. It is thus calculated starting from nodes and elements of the mesh, see .
Figure 1. (a) The use of internal nodal force in the bone mesh. (b) Presentation of the FE model composed of an implant part on a bone part.
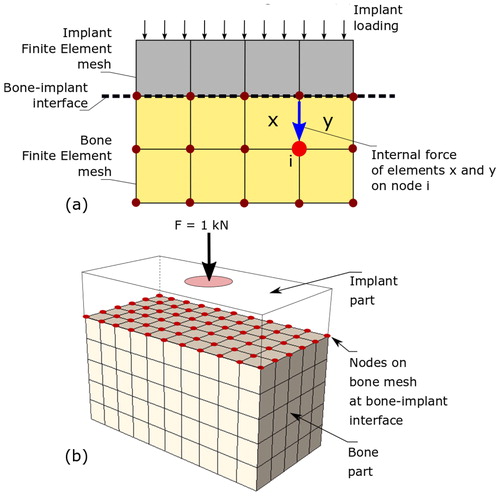
Thus, the objective is to control the mechanical state (stress or deformation field) of the surrounding peri-implanted bone through internal nodal forces. In this study, only the area of the bone at the interface with the implant is taken into account (first layer of elements).
2.2. Presentation of the 3D model
A simplified FE model is considered to show the interest of the developed approach. This model is composed of an implant part on cortical bone, both simplified by a rectangular parallelepiped. The geometry of the model is presented in . The internal force variables at the nodes at the bone-implant interface are considered. A compromise of the mesh size must, therefore, be made to avoid convergence problems and to optimize the computation time since all the nodes of the interface must be taken into account in the objective function. A mesh size of 1 mm defines the hexahedral mesh of the bone part. The implant part has meshed with hexahedral elements of 0.2 mm, defined by a convergence test. A load of 1000 N is applied to the implant, located in the center of its upper surface. The chosen objective is to homogenize the load transfer to distribute the load applied to the bone. The internal nodal force in each of the 66 nodes of the bone top surface is considered in the objective function. Since the initial load applied to the implant is 1000 N, the objective is, therefore, to apply 15.15 N to each node to distribute the load applied over the entire surface of the bone. The objective function can thus be defined:
(1)
(1)
The optimization calculation consists of minimizing the objective function φ, composed of the sum of the differences between the internal force of the node i Fii and the internal force to reach Firef. In the present case, i is between 1 and 66 and the internal reference force is 15.15 N.
3. Results and discussion
There is a convergence of the optimization calculation after approximately 40 iterations, with the minimization of the objective function, where the internal force in each node reaches the set point of 15.15 N.
The Von Mises stresses on the bone surface are presented in , where the final situation is compared with the initial situation. Initially, a localization of the stresses in the center of the bone surface is observed, due to the localized loading on the implant, see . The final situation shows a much more distributed and homogeneous stress field on the surface of the bone, see . Optimizing the geometry of the plate, therefore, made it possible to control the load applied to the bone, and in this case to homogenize it. The final design of the plate is presented in .
Figure 2. Comparison of the Von Mises stress fields on the top surface of the bone part at the (a) initial and (b) final situations. (c) Final optimized geometry of the implant part.
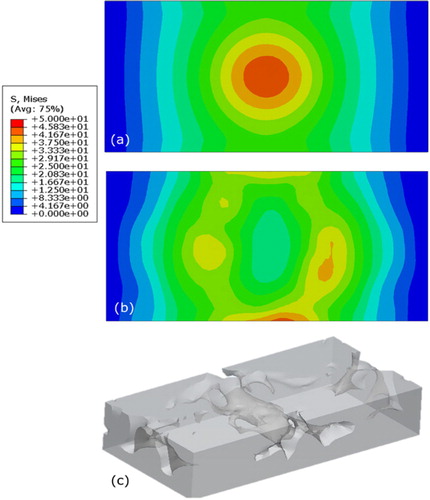
The final geometry has open and closed cavities. The pore size is dependent on the initial mesh size of the implant, defined by a convergence test of the mechanical problem. The manufacturability of the porosities was not considered in this study but constraints could be added, such as a minimum manufacturable pore size, the orientation with regard to the building direction or open cavities. However, this simplified model makes it possible to validate the use of nodal forces as a criterion for optimization, and more generally to validate this topological optimization approach for controlling load transfer at the bone-implant interface. The approach can be applied to concrete cases. It is suitable for devices with sufficient optimizable volume that can include manufacturable porosities and subject to high mechanical loading, from dental endo-osseous implant to hip prosthesis.
4. Conclusions
In this study, an original topological optimization approach has been developed, taking into account the surrounding bone and the load transfer between the implant and the bone. Through a simplified model, this approach proved to be functional. The approach can therefore be applied to loaded implantable medical devices with consideration of manufacturability constraints.
References
- Al-Tamimi AA, Rui P, Fernandes A, Peach C, Diver C, Bartolo PJ, Rui P, Fernandes A, Peach C. 2017. Metallic bone fixation implants: a novel design approach for reducing the stress shielding phenomenon. Virtual and Physical Protoyping. 12:141–151.
- Bendsøe MP, Sigmund O. 2003. Topology optimization: theory, methods, and applications. 2nd ed. Berlin: Springer.
- Sutradhar A, Park J, Carrau D, Nguyen TH, Miller MJ, Paulino GH. 2016. Designing patient-specific 3D printed craniofacial implants using a novel topology optimization method. Med Biol Eng Comput. 54(7):1123–1135.