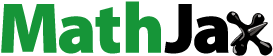
1. Introduction
High level endurance sports, like biking, may lead to a vascular pathology called endofibrosis. Its symptoms are an arterial wall thickening and a reduction of the artery lumen calibre. Cyclists are impacted in the iliac arteries due to a pinching and kinking mechanism, but other athletes may be vulnerable like rowers, triathletes or soccer players (Feugier and Chevalier Citation2004). This pathology was addressed increasingly over the years in the vascular surgery literature (INSITE Collaborators Citation2016). Arterial endofibrosis is a hypertrophic arterial remodelling pathology that affects a young and healthy population. According to Pr Feugier, specialist of this pathology in Lyon’s South Hospital (Feugier and Chevalier Citation2004), endofibrosis is close to another vascular pathology, called myointimal hyperplasia.
The development mechanisms of endofibrosis involves several factors and phenomena, such as hemodynamics, biochemistry, cells evolution, etc. We focus here on the biomechanical modelling of the endofibrosis in the iliac artery. Our goal is to bring a better understanding of this disease by a model that couples cell population evolution, hemodynamics, biochemical evolution of growth factors. The model is expected to permit a parametric analysis, and to provide prediction data about the most important mechanisms at play, which we illustrate here on a case of initially wounded artery.
2. Methods
To bring a better understanding of the endofibrosis onset and growth during the practice of sport, we propose a growth and remodeling numerical model by coupling hemodynamics (§2.3) with relevant models of endofibrotic tissue growth (§2.1) and of biochemical kinetics (§2.2).
2.1. Arterial cells population modeling
Based on a previous numerical study (Donadoni et al. Citation2017), we propose an improved model for arterial cells populations evolution, using a set of seven ordinary differential equations and two delay differential equations. They model the dynamical evolution of the main populations involved in endofibrosis in two of the artery’s layers, the intima and the media: vascular smooth muscle cells (vSMCs) either contractile (cSMCs) or secretory (sSMCs), endothelial cells that line the inner wall of the artery (ECs) and extra-cellular matrix (ECM), mostly collagen fiber.
2.2. Biochemical modelling
Populations functional properties, such as proliferation or apoptosis, appear in the kinetic populations’ balance equations. These parameters are strongly related to growth factors (GFs) bio-availability. We consider GFs in an extensive understanding, including all biochemical messengers and molecules signaling for functional properties modifications in populations equations. We thus develop a phenomenological biochemical model of GFs dynamics within intima and media layers. The GFs that we base our model on in first approximation, are listed in , illustrating the coupling between cells populations that produce or receive these biochemical molecules.
Table 1. Growth factors & producers/targets cells.
2.3. Hemodynamics modelling
Arterial hemodynamics is assumed to be a laminar steady Poiseuille flow, and blood is assimilated to an incompressible Newtonian fluid. Therefore, the hemodynamics are modelled by Navier–Stokes equations
where
is the velocity,
the pressure,
the blood density,
the kinematic viscosity. The wall shear stress (WSS)
is the friction stress exercised by blood on the arterial walls of normal vector
2.4. Loose coupling between the model equations
Through hemodynamics stimuli, ECs modulate the production rate of GFs and apparent permeability of the ECs layer. We develop relations to correlate the WSS sensed by ECs and the functional properties of cells (Andrews et al. Citation2010). Bio-availability is therefore related to WSS, and consequently hemodynamics influences largely the populations’ functional properties. The parameters of differential equations are thus coupled with GFs bio-availability.
The hemodynamics timescale is the cardiac cycle period and is of the order of 1 second. Arterial remodelling timescales are known to be within days to months. Based on this scale-separation assumption, we propose a loose coupling relation between arterial tissues growth and hemodynamics. We thus develop ad’hoc remodelling criteria whereby the blood flow is modified by tissues growth, assuming inward remodelling as proposed by (van Varik et al. Citation2012).
3. Results and discussion
We experiment our model by simulating the evolution of an initially damaged, by stripping of the EC layer, idealized axisymmetric artery. This numerical experiment is a first test-case, and we compare our model’s results to experimental animal model of angioplasty from the literature (Clowes and Schwartz Citation1985; Clowes Citation1993) at short and long times.
At short time, we compare the de-differentiation in cell phenotype, from cSMCs to sSMCs, and migration of vSMCs from the media tunic toward the intima tunic. These phenomena are essential in the early stages of the development of myointimal hyperplasia.
The cSMCs population evolution is reported in with the ‘unlabeled cell index’ proposed by (Clowes and Schwartz Citation1985). The figure shows that our model compares well with experimental data. During the comparison, we also calibrated the constants of our model related to de-differentiation and migration phenomena.
At long time (several months to one year), a steady state regime is expected with a intimal composition of 60–80% of ECM and 20–40% of vSMCs (Clowes Citation1993). As shown in , our model predicts a composition of the intima layer of 60% of ECM and 30% of vSMCs after twelve months. The behavior of our model at long timescale is thus qualitatively and quantitatively consistent with data from the literature. This is not the case of some previous models, which were designed for short-term predictions only (Donadoni et al. Citation2017). In addition, multiple phases in the development of the pathology are visible in , that illustrates the possible complex dynamics of the pathology, which we plan to investigate further.
4. Conclusions
With respect to existing models, our model is enriched with additional phenomena, such as the irreversible deposition of aged collagen fiber within ECM (Feugier and Chevalier Citation2004). The main contribution of this model compared to (Donadoni et al. Citation2017) is that under physiological initial conditions and wall shear stress stimuli, our model remains in a vascular homeostatic equilibrium. In addition, during the perturbation of this equilibrium, as with an initial damage of ECs layer, the arterial wall finds a new equilibrium in accordance with WSS stimuli and new GFs bio-availabilities. In a coming study, we shall use the model in various conditions, and assess its predictive capabilities against various cardiac conditions, with possible improvement in the coupling method.
Disclosure statement
No potential conflict of interest was reported by the author(s).
References
- Andrews AM, Jaron J, Buerk DG, Kirby PL, Barbee KA. 2010. Direct, real-time measurement of shear stress-induced nitric oxide produced from endothelial cells in vitro. Nitric Oxide. 23(4):335–342.
- Clowes AW, Schwartz SM. 1985. Significance of quiescent smooth muscle migration in the injured rat carotid artery. Circ Res. 56(1):139–145.
- Clowes AW. 1993. Intimal hyperplasia and graft failure. Cardiovasc Pathol. 2(3):179–186.
- Donadoni F, Pichardo-Almarza C, Bartlett M, Dardik A, Homer-Vanniasinkam S, Díaz-Zuccarini V. 2017. Patient-specific, multi-scale modeling of neointimal hyperplasia in vein grafts. Front Physiol. 8:226.
- Feugier P, Chevalier JM. 2004. Endofibrosis of the iliac arteries: an underestimated problem. Acta Chir Belg. 104 (6):635–640.
- INt’al Study group for Ident. & Treatment of Endofibrosis (INSITE Collaborators). 2016. Diagnosis & management of iliac artery endofibrosis: results of a delphi consensus study. Eur J Vasc & Endovasc Surg. 52(1):90–98.
- van Varik B, Rennenberg RJMW, Reutelingsperger C, Kroon A, de Leeuw P, Schurgers L. 2012. Mechanisms of arterial remodeling: Lessons from genetic diseases. Front Genet. 3:290.