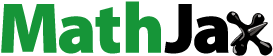
1. Introduction
Insufficient residual alveolar bone height is a common obstacle for the placement of dental implants in the posterior maxilla. Sinus lifting procedure involves the creation of a sub-sinus membrane pocket and the grafting with granular bone substitutes. Perforation of the sinus membrane is the most common complication with a large incidence rate (Testori et al. Citation2019). Graft material leak, through the perforation, results in a dental implant failure and/or postoperative sinusitis, requiring a complete reviewing of the maxillary sinus cavity and the removal of the remaining bone graft. The high perforation rate has led to develop new options involving 3 D biodegradable scaffolds instead of using granular bone substitutes. Case reports and case series have pointed out successful new bone formation after sinus membrane elevation using reoxidised cellulose (Gray et al. Citation2001), venous blood clot (Moon et al. Citation2011) and gelatin sponge (Sohn et al. Citation2010) as space maintainers. The main limitation of this promising technique was that the sub membrane space volume was limited and hardly predictable. The aim of this study was to evaluate the mechanical behaviour and volume kinematics of several haemostatic sponges to find out the best haemostatic medical device as space maintainers for new bone formation in sinus lift surgery. This study has been focused only on two devices: Gelita-spon® and Hemocollagene®. It aims to compare substitutes respectively made of porcine gelatine and bovine collagen.
2. Methods
2.1. Samples
The devices were provided in a cubic shape (∼10 mm edge) by the manufacturers and were not recut. This decision was supported by the fact that the aim is to mimic their use in surgical condition. In total 5 samples per device type have been studied: 3 in dry condition, 1 in saline buffer (PBS) saturated condition and 1 for volume analysis. The dry dimensions were measured thanks to a calliper and image analysis for the volume tracking. The samples were weighed with an accurate Sartorius CPA225D scale. The device’s microstructure has been assessed through scanning electron microscopy (SEM) through two conditions: native and after 50% of applied strain. This latter load refers to the manipulation of sponges while sinus lift surgery.
2.2. Mechanical test
The mechanical tests were performed on dry samples at different velocities with an applied strain around 50%. One sample was also tested in the same condition but saturated with PBS. A Universal Testing Machine Zwicky 0.5 equipped with a 100 N loadcell was used to measure samples’ behaviour through engineering stress strain curves.
2.3. Volume tracking
Finally, the fifth sample was immersed in a tank equipped with three perpendicularly oriented cameras to capture pictures during up to five days. The workbench has been custom made using Raspberry Pi 3 plus three 5 M Pixel cameras. The volume has been computed considering the sample as hexahedral and the coordinates of the apexes have been tracked manually through image analysis. The samples were glued on the bottom hence only the coordinates of the four upper points where collected. The characteristic time of volume
contraction has been assessed through the exponential expression:
where is the initial sponge volume,
the variation of volume in between the initial and final captures and
the current time. Data were post-processed thanks to Python libraries.
3. Results and discussion
Hemocollagene® sponges are denser than Gelita-spon® ones as presented in . This first observation plays a major role regarding the effective volume of sponge required to lift the sinus membrane. SEM analysis highlighted difference in microstructure in terms of pore radius and wall thickness (). These results are consistent with the literature (Aubert et al. Citation2017) and have to be considered regarding the cell migration within the tissue (i.e., required for achieve sponge integration to the tissue host) and for the mechanical behaviour modelling. Actually, once inside the body the cells will interact and colonize the sponge through mechanisms such as hydro-chemo-mechanics that require geometries and modelling parameters. From the mechanical point of view, elastic linear moduli highlight an advantage for the stiffer Gelita-spon® that might carry loads more than twice higher than the Hemocollagene® in dry condition.
Table 1. Sponges’ properties (mean SD).
The same trend has been observed once PBS saturated, but one sample is not enough to conclude. This hydrated test pointed out the difficulty to wet the Gelita-spon® sponge compared to the other studied that absorbed a volume five times higher. This observation has been confirmed during the volume tracking for five days.
The results presented in show the shrinkage of both sponges as function of time. The quality of the procedure has been validated by a preliminary study with known volumes and the first curves' points are consistent with the range provided in for the initial dry volume. It clearly appears that four days are enough for the Hemocollagene® to reach an asymptotic volume while the other one is far from it. The characteristic time provided are preliminary results that qualitatively confirms the previous conclusions. From a clinical point of view, this information combined to the classical monitoring through cone beam scans is crucial to define the best period to insert the implants. Although, being able to predict the tissue behaviour will decrease the quantity of X-ray scans for monitoring.
4. Conclusions
This preliminary study highlights the various responses that can be obtained with haemostatic sponges. The perspectives are to characterise their poro hyperelastic behaviour as well as their solubility to be able to simulate them within physiological conditions leading to mineralization. The cross linking will be also considered to improve their mechanical response.
Acknowledgements
The authors thank PICT-URCA platform for imaging core facilities and the “Gueules Cassées” 2017 grant.
No potential conflict of interest was reported by the author(s).
Disclosure statement
References
- Aubert L, Dubus M, Rammal H, Bour C, Mongaret C, Boulagnon C, Garnotel R, Schneider C, Rahouadj R, Laurent C, et al. 2017. Collagen-based medical device as a stem cell carrier for regenerative medicine. IJMS. 18(10):2210.
- Gray CF, Redpath TW, Bainton R, Smith FW. 2001. Magnetic resonance imaging assessment of a sinus lift operation using reoxidised cellulose (Surgicel) as graft material. Clin Oral Implants Res. 12(5):526–530.
- Moon JW, Sohn DS, Heo JU, Shin HI, Jung JK. 2011. New bone formation in the maxillary sinus using peripheral venous blood alone. J Oral Maxillofac Surg. 69(9):2357–2367.
- Sohn DS, Moon JW, Moon KN, Cho SC, Kang PS. 2010. New bone formation in the maxillary sinus using only absorbable gelatin sponge. J Oral Maxillofac Surg. 68(6):1327–1333.
- Testori T, Weinstein T, Taschieri S, Wallace SS. 2019. Risk factors in lateral window sinus elevation surgery. Periodontol 2000. 81(1):91–123.