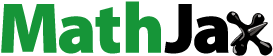
1. Introduction
Patient-specific models of blood flow are used for diagnosing Coronary Artery Disease (CAD). A remaining challenge is bridging scales from flow in arteries to the micro-circulation supplying the myocardium. Previous models developed for that purpose (Hyde et al. Citation2014) are descriptive rather than predictive. We propose a multiscale patient-specific model enabling blood flow simulation from the large coronary arteries to myocardial tissue.
2. Methods
The aorta, coronary arteries and left ventricle (LV) are segmented from Coronary CT Angiography (CCTA). The segmented arteries are coupled to a synthetic arterial network (Jaquet et al. Citation2019). An aortic root pressure of 93 mmHg drives flow into the model, which is terminated either by terminal resistances (coronary model) or is connected to a myocardium model (coupled model). For parameter tuning, the target flowrate is based on the patient LV mass, except for the obstructive CAD patient for which it comes from PET data as LV mass-based flow is too high.
2.1. Coronary model
Blood flow is modelled in the coronary network using the 1D approximation of the incompressible Navier–Stokes equations (Formaggia et al. Citation2003). The system of equations describing steady blood flow can be written as:
with
the coordinate of the centerline axis,
and
the flow rate and pressure,
the cross-sectional area of the vessel,
a geometry-related parameter tuned based on 3D simulations,
the blood density and
the kinematic viscosity.
2.2. Myocardium model
Blood flow in the myocardium is modelled by a single compartment Darcy model (Chapelle et al. Citation2010):
with
the Darcy velocity,
the capillary pressure,
the coupling pressure with the coronary model outlets and
the left atrium pressure taken null here. The parameters are:
a permeability of 0.002
,
and
conductances of flow entering and exiting the myocardium, assumed constant over the LV myocardium of volume
and initialized as:
where
is the average pressure of all coronary outlets, and
=15 mmHg the target average capillary pressure.
2.3. Coupling and validation
The coronary and the myocardium models are strongly yet iteratively coupled in both resting and hyperemic conditions. The interaction between the two models takes place at the coronary outlets, where pressures and flows are exchanged iteratively until the flow rate of each terminal segment between coupling iteration
and
does not change by more than 1%.
During postprocessing, the myocardium is divided into 17 segments as proposed by the American Heart Association (AHA). Myocardial Blood Flow (MBF) is calculated for each AHA segment with volume and associated flow rate
:
.
The spatial distribution of MBF is evaluated by computing the corresponding perfusion polar map, a 2D representation of the 3D myocardial volume. The complete method is summarized in .
Figure 1. Illustration of method for a patient with disease in the left anterior descending coronary artery. (A) CT imaging data. (B) Segmented geometry and CT-derived fractional flow reserve analysis (, clinical metric for CAD, 0.8 threshold between positive and negative results). (C)
results in segmented and synthetic vasculature. (D) MBF for coupled model. (E) Comparison of simulated (right) and
PET (left) perfusion maps.
![Figure 1. Illustration of method for a patient with disease in the left anterior descending coronary artery. (A) CT imaging data. (B) Segmented geometry and CT-derived fractional flow reserve analysis (FFRCT, clinical metric for CAD, 0.8 threshold between positive and negative results). (C) FFRCT results in segmented and synthetic vasculature. (D) MBF for coupled model. (E) Comparison of simulated (right) and [15O]H2O PET (left) perfusion maps.](/cms/asset/7c07064a-6fef-4169-80e8-85c11023c243/gcmb_a_1813433_f0001_c.jpg)
3. Results and discussion
We applied this method to six patients with suspected CAD who underwent CCTA and PET prior to invasive coronary angiography, which demonstrated non-obstructive CAD in five patients and obstructive CAD of the left anterior descending artery in one patient. MBF results for the coronary and coronary-myocardium coupled model were compared with PET data for five patients with non-obstructive CAD ().
Figure 2. Comparison of MBF results with PET exam data for five patients with mild CAD for (A) resting and (B) hyperemic conditions. Mean MBF values are depicted with grey squares. All AHA segments are taken into account.
![Figure 2. Comparison of MBF results with [15O]H2O PET exam data for five patients with mild CAD for (A) resting and (B) hyperemic conditions. Mean MBF values are depicted with grey squares. All AHA segments are taken into account.](/cms/asset/b888c8f8-6a46-4cfb-9d5c-6ac8a2aec1d6/gcmb_a_1813433_f0002_c.jpg)
Mean MBF matched measured data regardless of the model used. The coronary model exhibits a heterogeneous flow distribution not seen in the PET data as demonstrated by a larger variance of MBF whereas MBF is within the measured range for rest and hyperemia for the coupled model. Preliminary coupled model results in hyperemic conditions for a patient with obstructive CAD are seen in . Although, for this patient, hyperemic is tuned to match PET data (mean MBF = 1.82
), the exact region of the MBF perfusion deficit in the myocardium (in green) is predicted.
4. Conclusions
We described a novel multiscale method for simulation of coronary and myocardial blood flow. Results for 5 patients with non-obstructive CAD match PET data. Results for a patient with severe disease directly link coronary artery narrowing with impaired myocardial blood flow. Various CAD severities will next be tested.
References
- Chapelle D, Gerbeau JF, Sainte-Marie J, Vignon-Clementel IE. 2010. A poroelastic model valid in large strains with applications to perfusion in cardiac modeling. Comput Mech. 46(1):91–101.
- Formaggia L, Lamponi D, Quarteroni A. 2003. One-dimensional models for blood flow in arteries. J Eng Math. 47(3/4):251–276.
- Hyde ER, Cookson AN, Lee J, Michler C, Goyal A, Sochi T, Chabiniok R, Sinclair M, Nordsletten DA, Spaan J, et al. 2014. Multi-scale parameterisation of a myocardial perfusion model using whole-organ arterial networks. Ann Biomed Eng. 42(4):797–811.
- Jaquet C, Najman L, Talbot H, Grady L, Schaap M, Spain B, Kim HJ, Vignon-Clementel I, Taylor CA. 2019. Generation of patient-specific cardiac vascular networks: a hybrid image-based and synthetic geometric model. IEEE Trans Biomed Eng. 66(4):946–955.