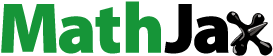
1. Introduction
Lesions of the Musculotendinous Unit (MTU) are a common pathology in musculoskeletal disorders and sports injuries which are a leading cause of functional impairment, long-term pain, and/or physical disability world-wide.
Though a large effort has been devoted to macroscopic failure evaluation, risk detection, and clinical observation, the management of these injuries suffer from a lack of knowledge of the underlying tissue-scale mechanical causes of such lesions. Indeed, there is a strong need of experimental results quantifying and describing MTU failure mechanisms and locations.
To this aim, rupture tests in mode I were performed through tensile tests (in the fibre and transverse directions) as well as peeling tests (to better investigate the transverse cohesion between muscle fibres), and mode II through shear lap tests (either at the muscle/muscle or muscle/tendon interface) under passive conditions on rabbit hamstring muscles. Statistical comparisons were made to assess the influence of the type of muscle (the Biceps Femoris (BF), the Semi-Tendinosus (ST) and the Semi-Membranosus (SM)), the traction direction and the type of loading.
2. Methods
2.1. Samples preparation
13 rabbit legs were obtained from crossbreeds New-Zealand rabbits of 75-105 days. Legs were dissected in order to separate each muscle, namely BF, ST and SM. Samples were prepared as depicted in . A dog-bone shaped punch was used to properly prepare tensile testing samples and reduce the size variability. All samples were measured with a caliper.
2.2. Mechanical testing
Displacement-controlled tensile tests were then performed at a calculated velocity matching a strain rate of 0.06%/s until rupture. Force values were recorded with a 4 Hz acquisition rate and a 20 N load cell.
For tensile and shear lap tests, one upper and one lower marks on the gage area were made, as seen in and a camera was synchronised with the force acquisition program in order to measure the initial and current length of the gage area of the sample with an optical marker tracking method. Images were acquired at a rate of 0.5 Hz with a 5 Mpx camera.
For peeling tests, several peeling cycles were performed in order to follow variation of energy release rate along the sample. The crack front was marked with print toner at the beginning of the test and at the end of each loading cycle to measure delamination areas at the end of the test.
2.3. Data analysis
For tensile and shear lap tests (assuming pure shear in the overlap area), the 1st Piola-Kirchoff (PK1) stress and stretch
in the tensile direction were calculated. Experimental data were then smoothed by a zero-phase Butterworth filter and were used to fit (i) a power law convex function for the toe region, (ii) followed by a linear function, and (iii) a non-linear concave function for the damage part. The two stretch values corresponding to the transitions between these three functions were also part of the fitting algorithm. As a result of this procedure, the damage initiation stretch was known (as the second transition stretch value identified), and hence used to separate elastic and damage parts of the experimental curve.
The energy release rate
and
were calculated, representing the energy required per unit surface to open the tissue in the fibre direction, the cross-fibre direction and shear respectively. For peeling tests, the energy release rate
(characterising crack opening of the tissue in cross-fibre direction in the
cycle) was calculated with
the fracture energy released and
the exposed area during the
cycle.
Statistical analyses were performed with an II-type ANOVA (2-way, N-way). A p-value less than 0.05 was assumed as significance threshold with a Bonferroni correction.
3. Results and discussion
Tensile experiment results showed that BF rupture initiated at a significantly (p
) higher stress but at a lower stretch, compared to other muscles (see ). They also confirmed the anisotropy of the elastic response with higher stress in the fibre direction than in transverse (p
) as well as the energy release rate (p
).
Figure 2. Tensile tests in longitudinal direction. Arrows show the 95% confidence interval of the damage initiation stretch/stress values and each line represent the average curve.
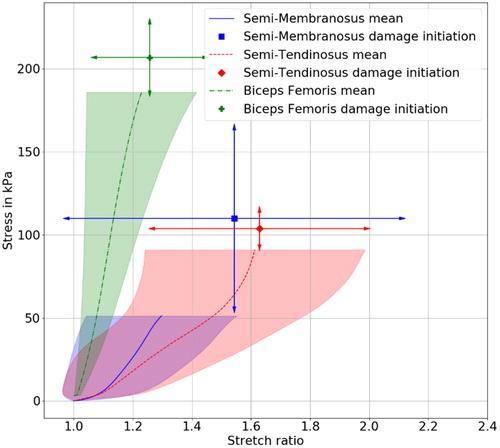
For peeling tests the energy release rate was much lower for BF than for other muscles (significantly between BF and SM: p of 0.03 and values of 0.23 ± 0.16 N/mm and 0.53 ± 0.08 N/mm), signifying an increased susceptibility to fibre decohesion in BF compared to others.
Also, a comparison between delamination on tendon and muscle showed that tendon required more energy to delaminate than muscle (p
). In addition, on mixed muscle/tendon samples, the rupture was always observed on the muscle close to the tendon. This observation of the musculo-tendinous junction weakness has been reported in the literature (Nikolaou et al. Citation1987). Indeed, the discontinuity between the tendon and the muscle may promote high strain gradients, leading to stress concentration in this region.
Comparison between tensile tests in the fibre direction and shear lap tests with muscle/muscle interface revealed lower strength in mode II than in mode I (p
with values of 181.38 ± 18.29 kPa and 24.84 ± 13.89 kPa).
The points made above in the discussion indicate that BF muscle could be the weakest link in the hamstring group (in the context of the experimental model of rabbits) in agreement with clinical studies, which reported the BF as the most involved in hamstring injuries (Schuermans et al. Citation2014). Our result suggest that, because of its weaker strength in shear compared to traction, a primary failure mode of the BF muscle may be mode II (decohesion due to shear). This hypothesis is also supported by the BF pennation, because of which a global tensile load on the muscle will cause local shear within the tissue. As a consequence, the BF would be more at risk of lesions than parallel muscles, as previously hypothesised by Fouré et al. (Fouré et al. Citation2019). Furthermore, our tests showed that this muscle is the weakest under cross-fibre loading so that any local damage in the BF (possibly near the musculo-tendinous junction) would more easily propagate.
This study was conducted on animal tissue. A similar work on human tissue is necessary to confirm or update the present results for further use in the context of human hamstring muscle mechanics.
4. Conclusion
Our study on hamstring muscles from rabbits suggests that the Biceps Femoris could be the weakest point of the hamstring group, with its musculotendinous junctions being possible damage initiation sites. These advances could be used as a basis for further experimental investigations (microstructural observations for instance) or to develop models to improve understanding of hamstring lesions.
Disclosure statement
No potential conflict of interest was reported by the authors.
References
- Fouré A, Le Troter A, Ogier AC, Guye M, Gondin J, Bendahan D. 2019. Spatial difference can occur between activated and damaged muscle areas following electrically-induced isometric contractions. J Physiol (Lond).). 597(16):4227–4236.
- Nikolaou PK, Macdonald BL, Glisson RR, Seaber AV, Garrett WE. 1987. Biomechanical and histological evaluation of muscle after controlled strain injury. Am J Sports Med. 15(1):9–14.
- Schuermans J, Van Tiggelen D, Danneels L, Witvrouw E. 2014. Biceps femoris and semitendinosus - teammates or competitors? New insights into hamstring injury mechanisms in male football players: A muscle functional MRI study. Br J Sports Med. 48(22):1599–1606.