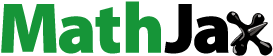
1. Introduction
A sustained exposure to Hand-Arm transmitted-Vibration may cause various vascular pathologies such as the vibration-induced Raynaud’s Syndrome. This disorder is characterized at the tissue level by an arterial growth and remodelling potentially induced by an intimal hyperplasia phenomenon. The proliferation and migration of the Vascular Smooth Muscle Cells (VSMCs) are the keystone of this intimal hyperplasia phenomenon. Wall Shear Stress (WSS) alterations play a key role in inducing such mechanisms. Moreover, finger-transmitted-vibration is likely to be responsible for a WSS decrease (Noel and Settembre Citation2017). So, the aim of our work is to develop an Agent-Based Model (ABM) of the WSS-modulated arterial growth induced by finger-transmitted vibration. This study is intended for the agent-based migration model.
2. Methods
2.1. Smooth muscle cells migration
The VSMCs are originally present in the media layer of the human muscular arteries. These cells can migrate to the intima layer in response to vascular disturbances. In the frame of our study, the low WSS resulting from vibration exposure induces the secretion of Platelet-Derived Growth Factors (PDGF) by the endothelial cells (Palumbo et al. Citation2002). Several studies have demonstrated that the PDGF can act as a chemoattractant and so have an effect on some cellular functions including migration. Indeed, when the VSMCs are exposed to PDGF, signalling pathways are activated and the migration process is induced (Jawien et al. Citation1992).
2.2. ABM design
Our ABM consists of a monolayer of endothelial cells (EC) and 5 to 10 layers of VSMCs. We neglected the adventitia as we are focusing on the VSMCs movement from the media to the intima only. Each cell is represented as an autonomous agent that interacts with its environment and other agents and can perform a number of biological behaviours such as proliferation, apoptosis, growth factor production and migration (only migration is covered here). The ABM was implemented in NetLogo© linked to Python© scripts for post processing analysis ().
A regular square lattice with Moore neighbourhood was used for cells positioning. Each lattice site can be occupied by only one cell. Multiple studies have shown that emerging cell growth patterns of lattice based ABM reflect the lattice symmetry (Drasdo Citation2005). In order to overcome this disadvantage, we developed an improved migration model that ensures a uniform final pattern of intimal thickening. This technique consists in weighting the cell’s displacement by a random periodic function depending on the angular position of each cell. The probability values are a function of the angular position of each cell and are calculated using the following equation:
with
and
a periodic function.
3. Results and discussion
For both the classical method and our new proposal, we calculated the final number of VSMCs present in each 22.5° angle opening normalized to the initial number. Hence, a uniform pattern should generate a normalized number equals to 1 on all angles.
With the classical method, the migration ABM describing the intimal thickening was affected by the lattice symmetry and did not preserve the rotational initial symmetry. During migration, more VSMCs were tending to move more often towards 0°, 90°, 180° and 270° orientations leading to cells accumulations in these zones ().
Figure 2. Polar curve of the final pattern of SMC migration (a) reflecting the lattice symmetry and (b) using our developed model. In the left graph, the radial coordinate represents the normalized number of the SMCs present in each 22.5° angle opening (i.e. number of cells in each angle opening divided by the initial number of the cells in the same angle opening). The right figures correspond to the ABM raw results. Bullets are the cells, red artery layer is the media and yellow the intima.
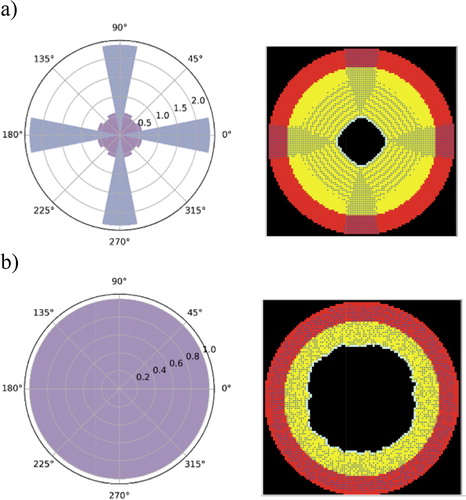
We conducted a parametrical study in order to find the mathematical form of and the corresponding
value that generate the least errors suitable for our model. We tested two periodic functions, a sinusoidal and a triangular function, with periods equal to
A parametrical study was performed on each function to find the corresponding value of
that generates the most uniform pattern with a normalized number of cells close to 1 on all angles. The study yielded a value of
for the sinusoidal and
for the triangular function. Furthermore, we observed that the triangular function with
provided more uniformity than the sinusoidal one with
Further analysis showed that the values of each function were independent of the initial number of cells, the diameter and thickness of the artery. Using this model, we were able to obtain a uniform intimal thickening that preserves a near circular form of the arterial geometry (). However, the study was limited to two choices of the weight periodic functions. If another form has to be chosen, a new parametrical study has to be performed to find the corresponding value of
4. Conclusions
In our present study, we developed a VSMCs migration model that ensured the uniformity of the emerging pattern of intimal thickening. The model consisted of controlling SMC movement towards the centre by using a periodic function depending on the angular position of each cell. However, we know that SMC migration is governed by PDGF secretion and diffusion into the arterial layers. In our future work, we will develop a diffusion model coupled with the ABM. Other cellular mechanisms such as proliferation, apoptosis will be also included. Our long-term goal is to couple the ABM with a finite element model that describes the mechanical behaviour of the artery. For each computational time step, the structural and geometrical changes induced by the arterial growth allow to compute the new mechanical equilibrium in the previous time step. This final mechanobiological model will be used as a tool in predicting arterial growth and remodelling induced by the Hand-Arm transmitted-Vibration.
References
- Jawien A, Bowen-Pope DF, Lindner V, Schwartz SM, Clowes AW. 1992. Platelet-derived growth factor promotes smooth muscle migration and intimal thickening in a rat model of balloon angioplasty. J Clin Invest. 89(2):507–511.
- Drasdo D. 2005. Coarse graining in simulated cell populations. Adv Complex Syst. 08(02n03):319–363.
- Noel C, Settembre N. 2017. A test setup for measuring the vibration effects on digital arteries vasoconstriction: a preliminary study. 52nd Human Response to Vibration Conference & Workshop; Sep 5-6, Cranfield Forensic Institute, Shrivenham SN6 8LA, UK, pp. 202–209.
- Palumbo R, Gaetano C, Antonini A, Pompilio G, Bracco E, RöNnstrand L, Heldin C-H, Capogrossi MC. 2002. Different effects of high and low shear stress on platelet-derived growth factor isoform release by endothelial cells consequences for smooth muscle cell migration. Arterioscler Thromb Vasc Biol. 22(3):405–411.