1. Introduction
Cardiac tissue is made of cardiomyocytes, extra-cellular collagenous matrix, vasculature, etc., arranged in a highly hierarchical microstructure (Kanzaki et al. Citation2010). Despite the advances made in describing the myocardial microstructure (Streeter et al. Citation1969; Hasaballah et al. Citation2017), it is still debated, and its relationship to the myocardial macroscopic mechanical properties is still not fully understood. In most models of the literature, the passive behavior of the cardiac tissue is described by purely macroscopic models, which average the deformation mechanisms taking place at the smaller scales. Accordingly, one of the major challenges remains in understanding and quantifying the way the transmission between the scales, mainly the fiber scale and the tissue scale, operates in fibrous tissues.
Most constitutive models of cardiac tissue are based on the affine transformation assumption, which states that local kinematics of each constituent, follows the local macroscopic deformation. Although this assumption is ubiquitous, its validity has not yet been quantified. However, verifying this hypothesis experimentally raises technical challenges, since it requires a simultaneous observation of the macroscopic deformation and the microstructure orientation (Jayyosi et al. Citation2017).
2. Methods
In this study, we experimentally investigate the affine transformation assumption by combining a full-field Mueller Polarimetric Imaging (MPI) system with an in-situ traction device.
MPI has emerged as a successful technique to explore biological tissues (Pierangelo et al. Citation2013). It exploits the polarization of the light, which is very sensitive to detect modifications in the structure of tissues on a microscopic scale. This technique enables the complete polarimetric characterization of a tissue by the simultaneous measurement of various biophysical quantities related to its scattering (depolarization) or anisotropy (phase retardance, azimuth of the birefringence, etc.) properties.
This optical setup was combined with a mechanical traction device, in order to measure the evolutions of the different parameters during sample stretch. The tensile force was measured using 2 sensors attached to the device grips.
To quantify the local stretch, a high-resolution camera was placed on the opposite side of the sample with respect to the polarimetric setup. Digital Image Correlation was then used to measure the local stretches simultaneously and on the same sample.
3. Results and discussion
Myocardial tissue specimen from porcine left ventricles with average dimensions of 30*10*2 mm and various cardiac fibers orientations were stretched with our setup. The heterogeneity of the observed stretches on each sample confirmed the multiscale structure of the cardiac tissue.
Simultaneously, the evolution of the orientations was observed by polarimetry, and showed a tendency to align with the stretching direction.
Under the affine assumption, we were able to predict the evolution of the local orientation, knowing the local kinematic and the initial orientation. This computed orientation was compared to the MPI measured one.
Note that our resolution is not sufficient to observe a single fiber, but only bundles of dozens of fibers.
For the samples where the stretch was aligned with the fiber orientation, differences between predicted and measured orientations were centered on 0, as shown in , supporting the affine transformation assumption.
Figure 1. Angular difference between measured and predicted azimuth distributions for a sample around 30° (left) and 90° (right) at different stretch levels.
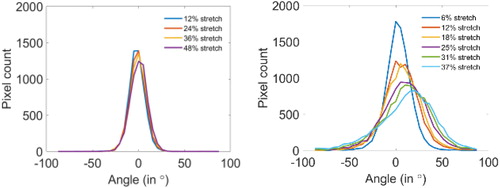
However, for the samples with an initial orientation perpendicular to the traction direction, a non-centered distribution of the difference was observed. This difference is explained by separation lines in the tissue, which open and create discontinuities in the tissue. The stretch measured on these lines is therefore not only the one of the cardiomyocytes, but includes the gap, leading to an overestimation of the microstructure reorientation.
The separation lines indicate the existence of deformation mechanisms at microscopic or mesoscopic spatial scales in the tissue, which should be further studied and included in structural constitutive modeling approaches.
Moreover, the macroscopic mechanical properties of the cardiac tissue have been experimentally investigated, and have revealed an anisotropic mechanical behavior of the tissue, in response to shear stress (Dokos et al. Citation2002).
The link between the microstructure and the macroscopic mechanical properties is still not fully understood. More specifically, it is still not clear how the anisotropy is induced at the macroscopic scale.
In order to shed light on this question, we decided to adopt a modeling approach, and numerically analyze the macroscopic mechanical behavior of cardiac tissue composed of different microstructure configurations consisting in altered organizations of cardiomyocytes and collagen planes, as shown in .
Figure 2. Complete (top) and section (bottom) views of the 3 adopted microstructural configurations with the cardiomyocytes in red and the collagen planes in blue.
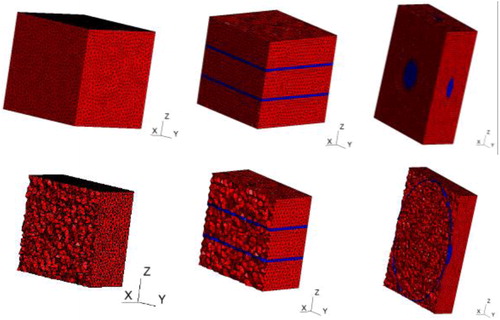
The orthotropic material behavior induced by these microstructures was compared to available experimental data. This study confirmed the importance of collagen planes in assuring the tissue’s orthotropic response and their organization was proven significant in reproducing the experimental results.
4. Conclusions
We were able to experimentally test the affine transformation assumption in the cardiac tissue by coupling Mueller Polarimetric Imaging with a traction device, and Digital Image Correlation. This experiment revealed defect lines which reflect weaknesses in the tissue, unveiling existing microstructural mechanisms which are studied in a modeling approach.
Acknowledgements
We thank V. de Greef for his technical support.
Disclosure statement
No potential conflict of interest was reported by the authors.
Additional information
Funding
References
- Dokos S, Smaill B, Young A, LeGrice I. 2002. Shear properties of passive ventricular myocardium. Am J Physiol Heart Circ Physiol.
- Hasaballah A, Sands G, Wilson A, Young A, Wang V, LeGrice I, Nash M. 2017. Three-Dimensional Quantification of Myocardial Collagen Morphology from Confocal Images. Springer International Publishing AG.
- Jayyosi C, Affagard J-S, Ducourthial G, Bonod-Bidaud C, Lynch B, Bancelin S, Ruggiero F, Schanne-Klein M-C, Allain J-M, Bruyère-Garnier K, et al. 2017. Affine kinematics in planar fibrous connective tissues: an experimental investigation. Biomech Model Mechanobiol. 16(4):1459–1473.
- Kanzaki Y, Terasaki F, Okabe M, Otsuka K, Katashima T, Fujita S, Ito T, Kitaura Y. 2010. Giant mitochondria in the myocardium of a patient with mitochondrial cardiomyopathy. Circulation. 121(6):831–832.
- Pierangelo A, Manhas S, Benalis A, Fallet C, Totobenazara J-L, Antonelli M-R, Novikova T, Gayet B, De Martino A, Validire P. 2013. Multispectral Mueller polarimetric imaging detecting residual cancer and cancer regression after neoadjuvant treatment for colorectal carcinomas. J Biomed Opt.
- Streeter D, Spotnitz H, Patel D, Ross J, Sonnenblick E. 1969. Fiber orientation in the canine left ventricle during diastole and systole. Circ Res. 24(3):339–347.