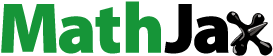
1. Introduction
Subcutaneous biodegradable sutures (e.g., Silhouette®; Sinclair Pharmaceuticals) are used to produce lifting effects on face and neck (Few et al. Citation2020). The benefit of these sutures lies in their minimally invasive insertion by a clinician. The presence of the suture stimulates the collagen fibre generation around the suture path. These collagen fibres will build up during the first few months after implantation and help maintain the mechanical lifting effect on the tissues during the degradation period of the suture material. One of the challenging aspects of suture insertion is suture positioning: for an optimal collagen fibre generation, the suture should move as little as possible. One of the main sources of suture movement is facial deformations induced by muscle contractions. In order to investigate these deformations, an “atlas” face model (Nazari et al. Citation2010) has been morphed to match the face of an elderly person. Using the three-dimensional muscle modelling developed by Nazari et al. (Citation2013) the effect of different muscle activations (single or multiple) on the face deformations can be simulated. We have used this approach to identify the locations that are appropriate for suture insertions with respect to minimising the potential stresses on the sutures due muscle activations.
2. Methods
2.1. FE model
The finite element of the face model, developped in the ANSYS software environment, consists of three layers totalling of 6342 hexahedral linear elements (with 8720 nodes). The outer layer corresponds to the dermis layer and the two other sublayers up to bony structure represent the hypodermis tissue of the face (). On the outer surface a thin membrane layer (0.1 mm thick) has been added to account for the epidermis layer. The material properties of the dermis and hypodermis layer are modelled with a Yeoh 2nd order model. Since skin stiffens exponentially when the stretch ratio exceeds a limiting value, a Gent material, which well accounts for this behaviour, has been used. The material parameters of these layers are given in . The inner surface of the deepest face layer is in contact with mandible and maxilla. Contacts between upper and lower lip have also been modelled. Global muscle fibre directions have been defined () in order to generate muscle elements around these directions. These muscle elements also include an active material part based on a Hill-Type model to generate forces.
Figure 1. (a) Cross section of the face model (b) the muscle directions (shown on half of mandible and maxilla).
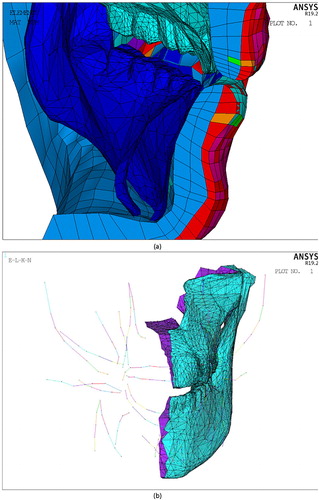
Table 1. Coefficients of the hyperelastic models.
3. Results and discussion
As shown by the EMG study of Root and Stephens (Citation2003) the natural use of facial muscles in daily life shows co-activation patterns, sometimes due to common synaptic drives. displays the face deformation in the lip corner region, which is very frequently the object of cosmetic treatments, due to the co-activation of muscles. In this work though, which focuses on muscles’ influences on facial deformation, it is also interesting to study single muscle activation, since it can induce larger and/or localized deformations with stronger possible impacts on inserted sutures. displays such a strong effect of a single muscle activation in the lip corner region. It provides a good estimation of the pattern of maximal face deformations in this region. Such a pattern can be used by clinicians to determine the appropriate place for suture implantation, which will efficiently reshape the face while reducing the impact of muscle activations on suture mobility. The best strategy should be to position the suture path just at the boundaries of the maximum displacement area (red arrows on ) even if the suture anchor point resides in maximum displacement area (tip of the arrow).
4. Conclusions
Suspension sutures are used to compensate for tissue structural changes due to ageing: This purpose is accomplished through collagen fiber generation along suture paths. In these sutures there are anchor points. The anchor point is located at the target lifting points. Even if the target points lie in the domain of maximum displacement or maximum stress region produced by muscle activation the suture paths should avoid the deformations induced by recurrent and strong muscle activation, as for example in the lip corner region affected by smiling, wincing and speaking. Our modelling approach enables to determine in a subject-specific manner the distribution of the deformations in the regions of interest, in order to find a suitable path for the insertion of the suture which preserves the efficiency of the lifting. Our results suggest that it is thus possible to avoid the region of the maximum displacement in the face by positioning the suture path just at the boundaries of this region. This action boosts the final purpose of these sutures which relies on the best possible collagen fibre generation. Doing so minimizes the suture mobility during daily facial movements and should provide the best possible effect. The process of verification and validation of the proposed suture placement strategy should be taken into account by following a patient’s lifting process through the period of collagen fibre generation.
Acknowledgments
Research supported by Sinclair Pharmaceuticals Ltd.
References
- Few JW, Vachon G, Pintas S, Smith JR. 2020. Nonsurgical tissue repositioning: analysis of long-term results and patient satisfaction from 100 absorbable suture suspension cases. Aesthetic Surg J Open Forum. 2(1):1–13.
- Nazari MA, Perrier P, Chabanas M, Payan Y. 2010. Simulation of dynamic orofacial movements using a constitutive law varying with muscle activation. Comput Methods Biomech Biomed Eng. 13(4):469–482.
- Nazari MA, Perrier P, Payan Y. 2013. The distributed lambda (λ) model (DLM): a 3-D, finite-element muscle model based on Feldman's λ model; assessment of orofacial gestures. J Speech Lang Hear Res. 56(6):S1909–1023.
- Root AA, Stephens JA. 2003. Organization of the central control of muscles of facial expression in man. J Physiol. 549(1):289–298.