ABSTRACT
Introduction: Oligosaccharides found in mammalian milk have shown the potential to alter brain development across multiple species. The diversity and concentration of these oligosaccharides is species-specific and varies greatly between individuals, thus understanding their role in cognitive development is warranted. We investigated the impact of early life dietary fucosylated/neutral or sialylated human milk oligosaccharides (HMO) on behaviours in tasks assessing anxiety, motivation, appetite, learning, and memory.
Methods: Sixty-four female Göttingen minipigs were artificially reared from 2 weeks postnatal and provided milk replacers. The study used four groups: no additional oligosaccharides (Con), fucosylated and neutral oligosaccharides (FN, 4 g/L), sialylated oligosaccharides (SL, 0.68 g/L), or both FN and SL (FN + SL, 4 g/L) from 2 to 11 weeks postnatal. One reference group was sow-reared. Weaning occurred between 10 and 11 weeks postnatal, and thereafter an obesogenic diet was provided. Behavioral tasks were conducted over three periods: 1) 0–11 weeks; 2) 16–29 weeks; 3) 39–45 weeks. Tasks included a spatial holeboard task, open field task, exposure to a novel object, runway task, single-feed task, and home pen behaviour observation.
Results: In the holeboard, the SL group demonstrated improved reference memory during reversal trials between 16-29 weeks. All groups demonstrated equivalent behavior in open field, novel object, runway, and single-feed tasks, as well as in their home pens (Ps > 0.05).
Discussion: These results suggest that early life dietary intake of sialylated oligosaccharides may provide an improvement to cognition during the equivalent developmental stage of adolescence.
Introduction
Human milk oligosaccharides (HMO) are nondigestible carbohydrates found in human milk that have a significant impact on brain development, the microbiome, and intestinal development of the infant [Citation1–3]. Human milk is unique in that it contains oligosaccharides at a greater concentration and diversity than most animal species [Citation4]. The typical structure of milk oligosaccharides follows repeated glucose-galactose backbones with a variety of linear or branched chains that may be modified (e.g. fucosylated or sialylated, see [Citation1] for in-depth review). Emerging evidence suggests these components have a variety of functions, including prebiotic-like activity, with the capacity to alter brain development [Citation5–14].
While there is a growing interest and recognition in the neurodevelopmental role of HMO, there are very few studies published and most are in preclinical models [Citation10]. Of those found in human milk, 2′-fucosyllactose (2′-FL), 3′-sialyllactose (3'SL) and 6′sialyllactose (6′-SL) have been studied in animal models with neurodevelopmental outcomes. Adult and pup rodents supplemented with 2′-FL demonstrated improved learning and memory, hypothesized to be due to vagally mediated improved hippocampal long-term potentiation [Citation7,Citation8,Citation11]. Mice supplemented with sialyllactose demonstrated improved spatial navigation [Citation5] or reduced anxiety-like behavior in response to stress [Citation6]. Absence of 6'SL in maternal milk was associated with deficits in learning, memory and attention through short term regulation of genes modulating patterning and myelination of the prefrontal cortex [Citation15]. Furthermore, pigs fed sialyllactose have been found to have enriched sialic acid in some areas of the brain [Citation9,Citation12]. In a recent study investigating the potential impact of 6′-SL on preterm pig development, Obelitz-Ryom et al. have reported improved performance in a working memory task and upregulation of myelin-related gene expression in the hippocampus [Citation13]. There is only limited clinical data reported on HMO and brain development so far. A study in an obese Hispanic population in the US, where breast-milk levels of 2′-FL at 1 month of age were positively associated with the Bayley-III cognitive sub-scale at 24 months of age [Citation14].
Still, it is unknown which HMO at which concentrations are necessary or sufficient to induce some alteration to brain development. Confounding this issue is the finding that the concentration and diversity of HMO vary wildly between mothers and with geographic location [Citation16]. Thus, the identification of a single ‘factor’ is likely unrealistic. Yet, as not all infants can be breastfed, understanding which of the HMO are important for brain development is key to improving global infant health.
The need to address early nutrition as a strategy to increase health and to decrease non-communicable diseases in future generations is well recognized and has been included among WHO’s recommendations for fighting childhood obesity [Citation17]. A meta-analysis has found that breastfeeding may provide a reduction of 10% in the prevalence of overweight or obesity in children breastfed for relatively longer durations. Numerous components in human milk may contribute to this effect [Citation18], and some HMO have been linked to infant weight and fat mass [Citation19]. Beyond its metabolic effects, obesity is also known to impair cognitive function [Citation20–23]. Though evidence is limited, HMO may be novel components of milk capable of preventing obesity-related cognitive deficits during development.
The objective of the present study was to assess the potential lasting impacts of HMO intake during early development on cognition and behavior in the context of obesity. We chose to investigate these effects using the Göttingen minipig due to the broad developmental, anatomical and physiological similarities of both the gastrointestinal tract [Citation24,Citation25] and the nervous system of the pig [Citation26–30] compared to the human. We previously demonstrated that combinations of human, bovine, or non-milk sources of oligosaccharides were capable of influencing cognition and brain development in a piglet model during neonatal development [Citation31,Citation32]. Here, we investigated the impact of milk formulas supplemented with various blends of HMO containing fucosylated, neutral, and/or sialylated HMO on behavioral and cognitive development. Oligosaccharides included: 2′-fucosyllactose, di-fucosyllactose, lacto-N-tetraose, lacto-N-neotetraose, 3′-sialyllactose, 6′-sialyllactose, and their combination. Pigs were tested for emotional reactivity, motivation, and learning and memory from pre-weaning development through sexual maturity. Furthermore, we assessed if these HMO impacted development in the context of a post-weaning obesogenic diet.
Methods
The protocol of the experiment (sows: AVD104002015325, pigs: AVD104002016515) was approved by the Animal Care and Use committee of Wageningen University & Research (Wageningen, The Netherlands) on June 13, 2017, and in accordance with the Dutch law on animal experimentation, which complies with the European Directive 2010/63/EU on the protection of animals used for scientific purposes.
Animals and housing
In total, 64 female pigs (Göttingen minipigs, Ellegaard, Denmark) born from 20 sows were subjected to a series of behavioral tests during 3 consecutive periods (See ): Period 1 between birth and 11 weeks of age, i.e. during the period of early development, Period 2 between 16 and 21 weeks of age, i.e. shortly before sexual maturity, and Period 3 between 39 and 45 weeks of age, i.e. sexual maturity [Citation33]. The study was conducted over 3 cohorts, with 2-week intervals between cohorts.
Figure 1. Schematic depicting the study design. In total, 64 female pigs born from 20 sows were subjected to a series of behavioral tests during a period of early development, shortly before sexual maturity, and at sexual maturity [Citation33]. The study was conducted over 3 cohorts, with 2-week intervals between cohorts. During the first week of age, pigs were kept with the sows and housed in individual farrowing pens. At 2 weeks of age, formula-fed pigs were separated from sows and housed in mixed groups of 2 pigs per pen. In addition, 8 pigs were cross-fostered and kept with 3 sows until the end of the milk intervention at 10–11 weeks of age (termed the Sow-Reared (SR) group). After being weaned from maternal milk at 10–11 weeks of age, SR pigs were housed in the same conditions as the formula-fed pigs. At 2 weeks of age, formula-fed pigs were randomly allocated to 1 of 4 milk formulas, enriched with a different oligosaccharide mixture, up to 11 weeks postnatal. After weaning at 10–11 weeks of age, formula-fed and SR pigs were fed a high-energy, obesogenic diet. Generally, the sample size was n = 12 pigs for each group, with n = 8 in the SR group. Pigs were tested on a variety of behavioral tasks designed to assess anxiety, motivation, appetite, learning, and memory.
![Figure 1. Schematic depicting the study design. In total, 64 female pigs born from 20 sows were subjected to a series of behavioral tests during a period of early development, shortly before sexual maturity, and at sexual maturity [Citation33]. The study was conducted over 3 cohorts, with 2-week intervals between cohorts. During the first week of age, pigs were kept with the sows and housed in individual farrowing pens. At 2 weeks of age, formula-fed pigs were separated from sows and housed in mixed groups of 2 pigs per pen. In addition, 8 pigs were cross-fostered and kept with 3 sows until the end of the milk intervention at 10–11 weeks of age (termed the Sow-Reared (SR) group). After being weaned from maternal milk at 10–11 weeks of age, SR pigs were housed in the same conditions as the formula-fed pigs. At 2 weeks of age, formula-fed pigs were randomly allocated to 1 of 4 milk formulas, enriched with a different oligosaccharide mixture, up to 11 weeks postnatal. After weaning at 10–11 weeks of age, formula-fed and SR pigs were fed a high-energy, obesogenic diet. Generally, the sample size was n = 12 pigs for each group, with n = 8 in the SR group. Pigs were tested on a variety of behavioral tasks designed to assess anxiety, motivation, appetite, learning, and memory.](/cms/asset/81684cfb-beb8-41e0-ae3b-f31aa5f593fb/ynns_a_1975877_f0001_ob.jpg)
During the first 2 weeks of age, pigs were kept with the sows and housed in individual farrowing pens (1.8 × 1.5 m). Pens were equipped with a heated nest only accessible to the pigs and had shredded straw as bedding. At 2 weeks of age, formula-fed pigs were separated from sows and housed in mixed groups of 2 pigs per pen (2.5 × 1 m) enriched with shredded straw as bedding and equipped with a squeeze ball and a dog bed. In addition, 8 pigs were cross-fostered and kept with 3 sows until the end of the milk intervention at 10–11 weeks of age (termed the Sow-Reared (SR) group). After being weaned from maternal milk at 10–11 weeks of age, SR pigs were housed in the same conditions as the formula-fed pigs. During the period of milk intervention, pens were equipped with a water dispenser and a milk dispenser with 2 drinking cups. After weaning from milk, the milk dispensers were removed from the pens, and pens were equipped with 2 feeders and 2 water dispensers. Two metallic chains were also added to the pens as additional enrichment material.
Dietary treatments
The milk intervention lasted from 2 to 11 weeks of age (pigs were weaned on postnatal days 14 and 12 for cohorts 1 and 2-3, respectively). At 2 weeks of age, formula-fed pigs were randomly allocated to 1 of 4 milk formulas, enriched with a different oligosaccharide mixture. Pigs were fed milk replacer with either no additional oligosaccharides (Con), a mixture of fucosylated (2′-fucosyllactose and di-fucosyllactose) and neutral (lacto-N-tetraose and lacto-N-neotetraose) oligosaccharides (FN, 4 g/L), sialylated oligosaccharides (3′- and 6′-sialyllactose) (SL, 0.68 g/L), or both FN and SL (FN + SL, 4 g/L). See and for a description of the ingredient and nutrient composition. Milk replacer was fed three times per day according to body weight to provide approximately 65 g milk replacer powder (325 ml milk) per kg body weight per day. Sow-reared pigs were allowed to suckle, thus receiving porcine milk up until weaning. After weaning at 10–11 weeks of age, formula-fed and SR pigs were fed a high-energy, pelleted, obesogenic diet twice per day (Supplemental Table 1 for ingredient composition). Generally, the sample size was n = 12 pigs for each group, with n = 8 in the SR group. Eight extra pigs were included in the control group during Period 1 for additional analyses, but these were not included in Period 2 or 3. Final sample sizes of each group for each behavioral test are described in Supplemental Table 2.
Table 1. Ingredient composition of milk replacers.
Table 2. Macronutrient composition of the base diets.
Behavior
For all behavioral procedures, order of testing within and between days were counter-balanced for treatment, to mitigate the potential effects of testing in the morning vs. the evening.
Experimenter socialization
Before the start of the behavioral tests, all pigs were habituated to the experimenters in their home pens. Starting 1 week after the expected birth date, the experimenters entered each pen daily in random order for approximately 5 min, sat in the pen, and pigs were allowed to approach and touch the experimenters spontaneously. In turn, the experimenters tested whether they were allowed to touch the pigs’ nose, head, and body, and when the pigs showed no sign of fear, to hold the pigs in their hands.
Furthermore, because the pigs were not yet familiarized with solid food in Period 1, pigs were habituated to the food rewards that would be used in the behavioral tests (runway and holeboard tests). Daily, experimenters gave small pieces of apple by hand to the pigs. When pigs learned to accept the rewards, a few pieces of apple were offered in a plastic plate (the same as the one used in the runway), and later, into a bucket (the same as the ones used in the holeboard). After daily sessions of habituation, the experimenters spread a few pieces of apple on the floor of the pens so that the pigs could freely forage for apple. After 8 days, all pigs were fully habituated to the experimenters and food rewards.
Cognition
Apparatus
The holeboard arena (3 × 3 m) had black, wooden, 80-cm-high walls and 4 entrances with guillotine doors that could be operated from the south-west corner of the arena with a system of ropes and pulleys. The arena was surrounded by a corridor for the pig to access the 4 entrances, a waiting area containing a jute bag and toys in the south-east corner of the room, and an observation area for experimenters in the south-west corner of the room. In the arena, 16 gray metallic buckets (Period 1: ⌀ 10.5 cm – H10 cm, Period 2 and 3: ⌀12 cm – H12 cm) were screwed to the floor in a 4 × 4 matrix. During the test, 4 of the buckets were baited with a small piece of apple (Period 1 ∼ 12 × 12 × 12 mm; Period 2 ∼ 12 × 12 × 20 mm; Period 3 ∼ 12 × 12 × 30 mm) according to 1 of 4 configurations of baited buckets. To prevent the use of visual cues to find the rewards, the rewards were hidden under a thin layer of shredded straw. All buckets had a perforated false bottom under which fresh pieces of apple were placed at the start of the day to prevent the use of odor cues to locate the baited buckets. Pigs inside the arena were, however, able to see the walls and ceiling of the room, as well as the 2 experimenters who were standing in the south-west corner of the arena, thus providing visual clues for navigation. During the tests, the pen mate was kept in the waiting area, which may have provided auditory and olfactory clues for navigation as well. Pigs were deprived from milk (Period 1) or feed (Periods 2 and 3) overnight (approximately 12-8 h) during the whole duration of holeboard testing.
Period 1
Habituation
For 8 working days (i.e. excluding weekends) before the start of the tests, pigs were gradually habituated to the experimenters, buckets, rewards, the corridor leading to the test room, the test room, and the task in sessions of 10–15 min per day. On day 1, pigs were habituated to finding the food rewards (small pieces of apple) in buckets in their home pens. From days 2 to 6, pigs from the same pen were transported to the holeboard room in a transport box (59 × 40 × 30 cm) with shredded straw and were allowed to explore the holeboard arena, in which the 16 buckets were baited. Pigs were habituated to the holeboard arena together with their pen mate on days 2–4, and individually from days 4 to 6. While being tested individually, their pen mate was kept in the waiting area with toys. On days 7 and 8, pigs were subjected to individual habituation trials in which only 8 buckets were baited, with at least 2 trials of maximum 3 min per day. At the end of the habituation period all pigs consumed food rewards, showed no extreme stress responses (e.g. high-pitched vocalizations, standing alert, escape attempts) when alone in the holeboard arena, and performed the task (i.e. looked into the buckets).
Acquisition trials
From approximately 7–9 weeks of age, pigs were individually subjected to 2 massed trials (two trials were performed a few min apart) per day on 14 consecutive working days, totaling to 28 acquisition trials.
Each day of the test, both pigs from the pen were transported to the testing arena using a cart (60 × 40 × 42 cm) with shredded straw as bedding. While a pig was tested, its pen mate was kept in the waiting area. The test pig was led to the start box (at the south door) or released into the corridor where it could walk freely to the correct entrance door, which was then opened. Different entrances were used daily, with two different entrances per day of test (i.e. 1 entrance per trial), to prevent pigs from developing a fixed pattern of visits that would reduce the working memory (WM) load. The trial started when the pig had four legs in the holeboard arena and ended when the pig found all four rewards or after 180 s. Every time the pig visited a baited bucket for the first time, a clicker sound was produced to facilitate learning. Visits were counted when a pig inserted its snout into a bucket. If the pig completed the task (i.e. found the four rewards in fewer than 180 ), the exit (south) guillotine door was opened, the pig received verbal praise (e.g. ‘good job!’, ‘well done!’), and received half of a white grape. If the pig did not complete the task within 180 s, a police siren sound was produced for 1–2 s; the pig was not praised and did not receive a reward. After each trial, the pig was led back into the waiting area and the other pig was tested. After the two pigs per pen had been tested twice, they were transported back to their home pen and their morning milk ration was distributed.
In total three different configurations (chosen from a total of eight used across all pigs over the course of the study) of baited buckets were used for each pig. Each pig was tested on a fixed configuration throughout the acquisition phase, with the configuration of baited buckets differing between the two pigs within each pen and balanced between formulas and cohorts. Testing order within and between pens was alternated within and between days to balance between experimental groups.
Period 2
Re-acquisition and reversal trials
From approximately 16–19 weeks of age, pigs were individually subjected to 2 massed trials per day on 12 consecutive working days for a total of 24 re-acquisition trials. Pigs were tested with the same configuration as in Period 1, and the procedure was the same as in Period 1. Pigs, however, were not transported in a cart but could walk freely from their home pen to the testing room. After two pigs per pen had been tested twice, they walked back to their home pen and their morning feed ration was distributed.
After the re-acquisition phase was completed, pigs were individually subjected to 16 reversal trials, with 2 massed trials per day on 8 consecutive working days. The procedure was the same procedure as in the re-acquisition phase of Period 2, but pigs were assigned to a different configuration of baited buckets (e.g. A → C, C → A, B → D, D → B).
Period 3
Re-acquisition and reversal trials
From approximately 41–43 weeks of age, pigs were individually subjected to 2 massed trials per day on 12 consecutive working days for a total of 24 re-acquisition trials. Pigs were tested according to the same procedures as for Period 2, but only with the configuration of the reversal trials of Period 2. After the re-acquisition phase was completed, pigs were individually subjected to 16 reversal trials, with 2 massed trials per day on 8 consecutive working days. The procedure was the same procedure as in the re-acquisition phase of Period 3, but pigs were assigned to a different configuration of baited buckets (e.g. A → G, B → H, C → E, D → F).
Behavioral analysis
The following parameters were scored live using The Ethovision XT 10 software (Noldus Information Technology, Wageningen, The Netherlands): all visits and revisits to all buckets, latencies to all bucket visits, and trial duration, total number of defecations, urinations, high-pitched vocalizations and escape attempts during the trial. From the parameters recorded during the test, variables (described in Supplemental Table 3) were calculated a posteriori according to van der Staay et al [Citation34].
Supplementary behavioral testing
A full description of methods used for testing emotional reactivity, motivation, appetite, and home pen behavior can be found in the Supplemental Methods. Briefly, pigs were tested on multiple tasks to assess their response to novel environments/objects, physiological response to stress, motivation for food rewards, appetite, and typical home pen behaviors.
For all Periods 1–3, pigs were subjected to an open field task wherein they were allowed to explore an unfamiliar empty arena (3 × 3×1.2 m) for five min and their exploratory behaviors recorded (i.e. posture, interest in the floor and walls, vocalizations and excretions, etc. See Supplemental Table 4). In Periods 2–3, pigs were exposed to a novel object dropped in the middle of the arena for five min after exploration of the Open Field. In both Periods 2 and 3, heart rate and salivary cortisol were collected. Pigs were fitted with heart rate monitors (BioHarness 3.0, Zephyr Technology, Annapolis, MD, USA) for measurement of heart rate before (‘Pre-test’), during Open Field testing (OF), during the Novel Object test (‘NO’), after (‘Post-test’) testing, and during transport to and from testing (Transport 1 and 2, respectively). For salivary cortisol, saliva samples were collected from each pig in the home pen at 60 (t-60), 5–10 min before (t-5), and 15, 30, and 60 min after the pig entered the test arena (t15, t30, and t60, respectively). Salivary cortisol was measured in duplicate using a commercially available enzyme immunoassay kit (ELISA, IBL International GmbH, Hamburg, Germany) according to the procedures of the manufacturer. Behavioral observations were scored live by two trained observers using The Ethovision XT 10 software (Noldus Information Technology, Wageningen, The Netherlands). One observer recorded state behaviors: locomotion (e.g. walking, running, standing), exploratory (e.g. nosing, rooting, approaching), and ‘other behaviors’ (i.e. not performing exploratory behaviors); and one observer recorded event behaviors: vocalization (e.g. grunts, squeals, barks), defecation, urination, escape attempts and grooming.
To measure motivation, pigs were trained on a runway task to run down a narrow corridor (4–8 m in length) for a reward (2 apple pieces of up to 12 × 12 × 20 mm). Pigs were feed restricted overnight to ensure motivation. Pigs were provided 4 trials at each Period, with a maximum of 120 s for completion of each trial. If this limit was reached in two consecutive trials, testing was ended for that animal. Time to leave the start box, latency to complete task, and run time (latency to completion – leave time) were recorded.
To measure appetite, at 29 weeks-of-age, all pigs were subjected to 4 single-feed tests in which they had ad libitum access to their regular diet for 1-h meals in 2 consecutive days (2 meals/day). Feed refusals were weighed at the end of the 1-h meals to assess the pigs’ voluntary feed intake as a proxy of their appetite. The day before the testing, all pigs were weighed before their morning meal to correct feed intake for body weight.
Behavioral activity of pigs in their home pens was recorded at approximately 3 (Period 1, the SR group was omitted from this analysis), 21 (Period 2) and 40 (Period 3) weeks of age. Behaviors were scored live using a 1.5-min instantaneous scan sampling schedule for 6 h/day during 6 sessions of 1 h (08:15–09:15, 09:30–10:30, 10:45–11:45, 14:00–15:00, 15:15–16:15, 16:30–17:30 h). The behaviors, described in Supplemental Table 5, were scored using The Observer® 5.0 software (Noldus Information Technology, Wageningen, The Netherlands) in Periods 1 and 2, and a paper score sheet in Period 3. Per period, the same observer recorded the behaviors for the 3 cohorts of animals to limit inter-observer observation bias.
Statistical analysis
Statistical analyses were conducted with SAS 9.3 and 9.4 (SAS Institute, Cary, NC, USA). Exact models used are described in their respective tables, however, the general approach used is as described. Data were analyzed with the MIXED procedure (analysis of variance), and significant main effects were further analyzed using the differences of the least square means with a Tukey adjustment for multiple comparisons. Data were analyzed by period, with diet and cohort as main effects. For holeboard data, performance across each of two days were considered 1 ‘block’ (i.e. 2 trials per day over 2 days was averaged to create a single ‘block’ containing 4 total trials). Ultimately, cohort, block, and diet were included as fixed effects. Block by diet interactions were found insignificant in the model for almost all variables, and thus removed. The distribution of model residuals was checked with the UNIVARIATE procedure. If model residuals were not normally distributed, analyses were performed on (arc sin) square root or logarithmically transformed data such that the data met the normality assumption or analyses were performed using non-parametric tests. For variables where no normal distribution of the model residuals could be obtained, data were analyzed with the non-parametric Kruskal–Wallis test and/or as binary data using the GLIMMIX procedure. However, in each case model fit was similar between raw/transformed and non-/parametric tests. Thus, all data are presented as (untransformed) means ± SEMs and analyzed via mixed model ANOVA. The number of experimental units used in the analyses for each test are presented in Supplemental Table 2. Given the rearing environment of the SR group was largely different from the formula-fed groups, the SR group was not included in statistical analyses and are meant to serve as a reference group only. Performance on behavioral tasks from this group are in the Supplemental Material.
Results
As noted in the methods, the SR group was only included as a reference. No statistical comparisons were made between the SR group and any other group.
Growth
No differences were found in growth between any groups (Diet effect, P > 0.050, ). Milk and feed intake were similar between groups, with some variation in feed intake during Period 2.
Figure 2. Growth and intake data. Across the trial, there were no differences in bodyweight growth between groups (P < 0.05). A lean reference using Göttingen minipigs from the vendor (Ellegaard) is shown to verify the weight-gain inducing capacity of the diets used. Milk and feed intake were measured per pen (2 animals per pen). Milk replacer was provided at 325 mL/kg BW per day and pelleted feed was fed twice per day. Variation in milk intake was observed during Periods 1 and 2, however by Period 3 feed intake was equivalent between groups as all pigs consumed the pelleted feed provided.
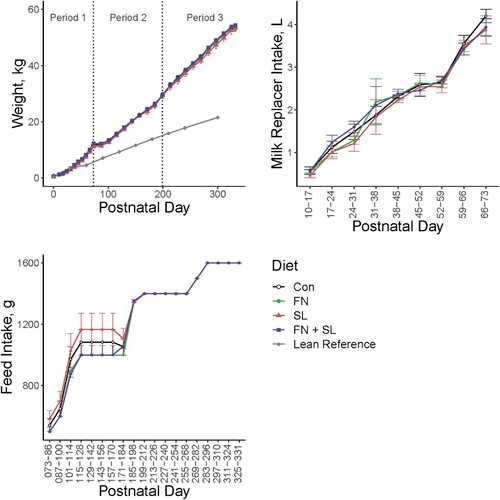
Cognition
Period 1
Acquisition
Although working and reference memory scores increased while errors decreased over time (Block effect, P < 0.001), this period was considered training as almost all groups did not reach 80% or better working memory scores and 9 of 62 pigs did not find the 4 rewards for more than half of the trials (N: Con, 3; FN, 3; SL, 2; FN + SL, 1). All variables measured showed no difference between groups (Diet effect, all P > 0.15, data not shown).
Period 2
Re-acquisition
During acquisition, all groups showed improved performance with time (Block effect, P < 0.001) for working and reference memory scores. Diet effects included the percentage of working memory errors, total trial duration, latency to first baited visit, and inter-visit intervals (Diet effect, all P < 0.05). The SL group displayed the lowest percentage working memory errors (this was significant only when compared to the FN group post-hoc, P = 0.018). Test groups demonstrated longer trial durations, latency to first baited visit, and greater inter-visit intervals than the control group (Diet effect, all P < 0.05, Supplemental Table 6, and ).
Figure 3. Average performance by training block on select variables in the holeboard task. Data represent an average of 4 trials within each block. By block, animals in all groups improved both their working memory (WM) and reference memory (RM) scores, while errors decreased. As expected, reversal trials lowered performance which quickly recovered in subsequent blocks. Performance during Period 1 was considered ‘training’ as most groups demonstrated poor behavior, thus is not shown. Data were analyzed via one-way ANOVA sliced by time with diet, cohort, and blocks as main effects (shown in ). Abbreviations: Con, control group; FN, group fed fucosylated and neutral oligosaccharides; SL, group fed sialylated oligosaccharides; FN + SL, group fed both FN and SL.
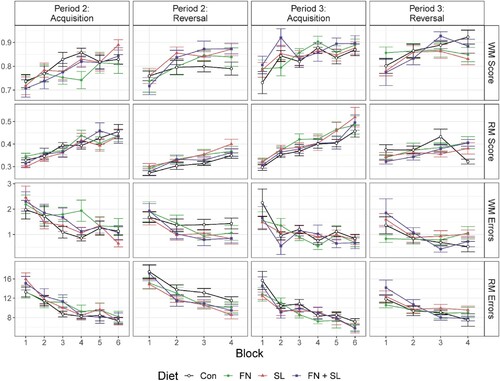
Figure 4. Average performance on select variables in the holeboard task. Averaged over blocks, the SL group demonstrated greater reference memory (RM) scores and fewer errors (P < 0.05) on the reversal phase at Period 2. The Control group demonstrated the shortest inter-visit interval (IVI) at all times measured, and also completed the trial faster during acquisition and reversal trials of Periods 2 and 3, respectively (P < 0.05). Data were analyzed via one-way ANOVA sliced by time with diet and cohort as main effects with a post-hoc Tukey adjustment for multiple pairwise comparisons. Abbreviations: Con, control group; FN, group fed fucosylated and neutral oligosaccharides; SL, group fed sialylated oligosaccharides; FN + SL, group fed both FN and SL.
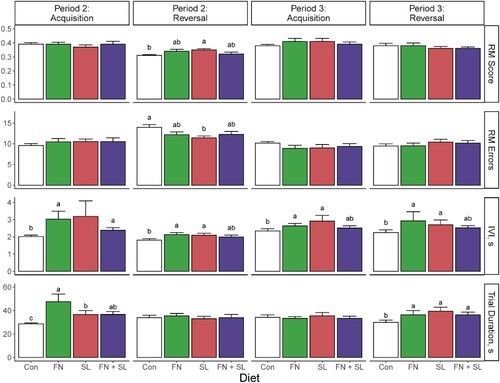
Reversal
All groups were able to learn a new configuration as evidenced by increased working and reference memory scores over time (Block effect, P < 0.001). Overall, we observed a significant effect of diet on reference memory score, percentage of reference memory errors, and total errors (Diet effect, all P ≤ 0.010). These effects were driven by the improved performance of the SL group, which exhibited significant improvement for these variables compared to the control group, specifically an increase of reference memory score and a reduction of percentage of reference memory errors and total errors (Supplemental Table 7, and ).
Period 3
Re-acquisition
All groups demonstrated improved working and reference memory scores over time (Block effect, P < 0.001), and although controls demonstrated the lowest memory scores and a greater number of errors, there were no differences between groups in memory scores (Diet effect, all P > 0.050). FN and SL groups displayed longer inter-visit intervals compared to controls (pairwise mean comparison, all P = 0.001, Supplemental Table 8, and ).
Reversal
All groups showed increased working and reference memory scores with time (Block effect, P ≤ 0.001), however, there were no differences in memory scores between groups (Diet effect, all P ≥ 0.308). Similar to performance during the acquisition task during Period 2, test groups had longer trial durations on average than the control group (Diet effect, P < 0.001), which was reflected in longer inter-visit intervals (Diet effect, P = 0.013, Supplemental Table 9, and ).
Emotional reactivity
Overall, few differences were found between groups for any variable related to emotional reactivity during the Open Field, or Novel Object tasks.
Period 1
Open field
Each group performed similarly, with the test groups spending less time performing ‘Other’ behaviors as opposed to exploratory related behavior compared to the Control group (Diet effect, P = 0.090, Supplemental Table 10, ).
Figure 5. Observed behavior during the Open Field and Novel Object tasks. Behaviors were characterized by % time spent in various postures, exploratory behaviors, and frequency of discrete events. See Supplemental Table 4 for a detailed description. Due to great variability, at no point did any group demonstrate behavior different from another. Data were analyzed via one-way ANOVA sliced by time with diet and cohort as main effects. Abbreviations: Con, control group; FN, group fed fucosylated and neutral oligosaccharides; SL, group fed sialylated oligosaccharides; FN + SL, group fed both FN and SL.
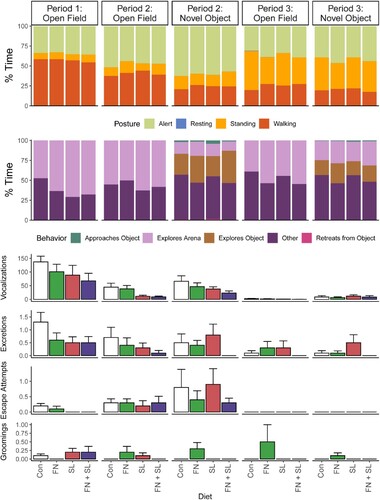
Period 2
Open field and novel object tasks
Groups SL and FN + SL exhibited the fewest vocalizations as compared to other groups (Diet effect, P = 0.088) during the OF task. Otherwise, all groups behaved similarly with regard to posture and activity in the OF and NO tasks (Supplemental Tables 11 and 12, ). At no time prior to, during, or after the behavioral tasks were heart rate or salivary cortisol different between groups (Diet effect, all P ≥ 0.144, Supplemental Table 16, ).
Figure 6. Salivary cortisol before and after Open Field testing. Saliva samples were collected from each pig in the home pen at 60 before (t-60), 5–10 min before (t-5), and 15, 30, and 60 min after the pig entered the test arena (t15, t30, and t60, respectively). In general, salivary cortisol exhibited high variability between and within-groups. At Period 2, there were no differences between groups. During Period 3, the FN + SL group displayed the lowest salivary cortisol at all times; this was only significantly different from other groups at t15 and t60. Data were analyzed via one-way ANOVA sliced by time with diet and cohort as main effects with a post-hoc Tukey adjustment for multiple pairwise comparisons. Abbreviations: Con, control group; FN, group fed fucosylated and neutral oligosaccharides; SL, group fed sialylated oligosaccharides; FN + SL, group fed both FN and SL.
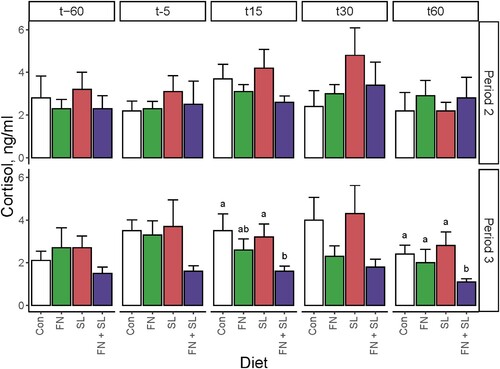
Period 3
Open field and novel object tasks
Behavioral performance was similar across all formula-fed groups during the Open Field task. During the Novel Object task, groups SL and FN + SL tended to spend a greater proportion of time approaching the novel object than other groups (Diet effect, P = 0.062). All other behaviors were similar between groups (Diet effect, all P ≥ 0.535, Supplemental Tables 14 and 15, ). There were no differences in average heart rate between groups at any time before, during, or after behavioral testing between groups (Diet effect, all P ≥ 0.178). Cortisol was overall low in group FN + SL and was different from the other groups at 15 and 60 min after the start of the emotional reactivity tests (Diet effect, P < 0.050, Supplemental Table 16, ).
Home pen observations
During Period 1 the FN group spent the most time displaying ingestive behaviors compared to all other groups (pairwise mean comparison P < 0.05). However, all other behaviors at each time observed were equivalent between groups (Diet effect, P ≥ 0.05, Supplemental Table 17).
Motivation and appetite
Performance on the runway and single-feed tasks was equivalent between groups at all times measured (Diet effect, P > 0.100 for all variables, Supplemental Tables 18 and 19).
Discussion
In the present experiment, the goal was to assess if oligosaccharides that are present in human milk have the capacity to promote cognitive development in the context of an obesogenic diet. Thus, Göttingen minipigs were artificially reared at an early age and exclusively fed a milk replacer supplemented with fucosylated+neutral and/or sialylated oligosaccharides, and their performance in multiple cognitive domains was assessed. A group of sow-reared pigs were included in the study as a reference group but were not included in statistical analyses.
Porcine milk contains primarily neutral oligosaccharides (65–85% neutral), with < 30% acidic and <10% neutral fucosylated. The concentration of oligosaccharides in porcine milk is greatest in colostrum (∼250 mg/L) and lowers to less than 100 mg/L after 18 days of lactation [Citation35]. Human milk, though exhibiting great geographical diversity [Citation16], is largely neutral fucosylated, with a lesser proportion of acidic and neutral nonfucosylated HMO [Citation3]. Total concentrations of OS can range from 5-15 g/L, much higher than either bovine or porcine milk [Citation1]. Thus, the doses used in the current study approximate concentrations seen in mature porcine milk (in the Control group), or those much higher than porcine milk and closer to the lower end of that seen in human milk (all other test groups).
Ultimately, we found that oligosaccharide intake had minimal or no effects on growth or behavioral indices of emotional reactivity, stress, motivation, and appetite. On a spatial task testing working and reference memory, pigs fed sialylated oligosaccharides demonstrated a moderate improvement in performance between weaning and sexual maturity (i.e. the equivalent of adolescence). Such findings suggest oligosaccharides may play a specific role in cognitive development, particularly during the adolescent time period.
Sialylated oligosaccharides promote cognitive flexibility in a spatial memory task
The holeboard task was chosen for its ability to separate the subject’s performance into domain-specific memories: working and reference memory. As reviewed in depth by Nadel and Hardt [Citation36], reference and working memory are distinct concepts. Reference memory represents a continuous process requiring sensation, perception, encoding, storage, and retrieval of information. A process occurring over time that requires protein synthesis and structural synaptic change. Consequently, alterations to reference memory can act at one or multiple points throughout this process. In contrast, working memory is responsible for storing and manipulating information for an acute length of time. In the holeboard task, reference memory can be measured by the ability of a subject to remember the spatial locations of the baited buckets across trials. Working memory is the ability of the subject to remember (and avoid) the spatial arrangements of buckets already visited within a trial. In our study, all pigs, independently of the treatments, exhibited improvement of both reference and working spatial memory with training in all three periods, confirming the ability of (young) pigs to learn during the task [Citation37,Citation38]. Interestingly, we found that early life dietary supplementation with sialylated HMO improved the reference memory of piglets during reversal learning, but only when assessed in period 2 (between weaning and sexual maturity, i.e. early adolescence) and not in period 3 (adulthood). That the diet effects were specific to reversal learning and were only found during early adolescence, and not during adulthood, might be due to differences in the ontogeny of different memory function in pigs.
There are limited studies of the early ontogeny of spatial learning in pigs. Elmore et al [Citation39]. reported that pigs are able to learn a spatial T-maze task as early as 2 weeks of age. When the task was more complicated, such as the radial 8-arm maze, 2-week-old piglets needed a non-spatial version of the task to be able to perform [Citation40]. There are limited studies investigating the ontogeny of reversal learning in any animal model. As reviewed by Izquierdo and colleagues [Citation41], reversal training is a method to study cognitive flexibility, hallmarked by the ability to rapidly alter behavior in response to changes to a learned association (or set of rules). In this case, pigs have learned to associate the spatial location of baited buckets with a reward. During reversal, the locations of these buckets change, and the pigs must inhibit their previous associations to learn the new spatial locations. Here, the use of working and reference memory are still required, recruiting multiple brain regions within the medial temporal lobe.
In rats, the capacity to exhibit reversal learning has been reported in the T-maze as early as PND 19–21 [Citation42,Citation43], suggesting that rats are capable of exhibiting cognitive flexibility around weaning age. Saperstein et al. [Citation44] reported that reversal learning in a non-spatial olfactory discrimination learning task was visible as early as PND 12. Willing et al. [Citation45] demonstrated that rats’ cognitive flexibility, measured as the capacity to learn a new platform in the water maze, increased from pre-pubertal to post-pubertal periods. It is possible that early adolescence is a period where reversal learning capacity is at its lowest in pigs and would, therefore, benefit the most from supplementation of sialylated HMO in early life. Consequently, a harder task in adulthood could have facilitated the detection of a beneficial effect of the early life HMO supplementation in this period as well. In line with such a hypothesis, Wang et al. [Citation46] reported that early life sialic acid supplementation only benefited learning in adult pigs in a hard task but not in an easy one.
Here, we demonstrated that early-life supplementation with sialyllactose moderately improved cognitive flexibility. Early life sialyllactose supplementation has been shown to attenuate stress-related behavior [Citation6], alter the sialic acid composition in the brain [Citation9Citation12], improve performance in spatial tasks in preterm pigs [Citation13], and improve learning and memory in cross-fostered rats [Citation11]. On the other hand, sialyllactose has been shown to have no additional benefit when added to a diet already containing oligosaccharides [Citation47], or minimally impact spatial navigation [Citation5]. Studies on ingredients containing sialic acid such as gangliosides [Citation48], complex milk lipids [Citation49], lactoferrin [Citation50], and casein glycomacropeptide [Citation46] have been shown to have some positive impact on cognition.
The importance of sialic acid in brain development has been well reviewed. Briefly, 75% of sialic acid is present in the brain in gangliosides, which themselves have critical roles during neurodevelopment for the synthesis and regulation of neurons, synapses, axons, and myelin [Citation48,Citation51]. Though sialic acid can be synthesized de novo, it is believed that exogenous sources may also be required for optimal brain development [Citation51]. In early life, this exogenous source is human milk, in which about ¾ of sialic acid is present in oligosaccharide bound form (i.e. as sialylated milk oligosaccharides), or infant formula, in which sialic acid level is significantly lower [Citation51]. Here, we observed a cognitive promoting effect of early life sialyllactose supplementation specific to a period of sexual development. Adolescence is a known time of great cognitive change and plasticity in the brain [Citation52]. For example, pruning of dendritic spines and loss of synaptic density are well documented in the rodent prefrontal cortex [Citation53,Citation54]. Such changes coincide with improvements in reversal learning during adolescence [Citation45]. Thus, it is tempting to speculate that the effects observed in this study are of a programing effect wherein increased sialyllactose in early life better primed the brain for the synaptic reorganization that occurred during adolescence.
Independent of sialyllactose, all groups fed oligosaccharides in this study spent more time between visits to buckets in the holeboard task as compared to the control group during both Periods 2 and 3. Speculatively, a combination of shorter inter-visit intervals, shorter trial duration, lower memory scores, and greater errors in the control group suggest that pigs fed oligosaccharides may have had a greater executive function and inhibited the impulse to quickly visit buckets and exhaustively find the rewards. Despite these promising results, only sialyllactose, but not FN or a mixture of FN and SL, showed a promoting effect on cognition in our study. Yet, a growing body of literature across many different oligosaccharides suggests a link to cognition. For example, chitosan oligosaccharides [Citation55], fructooligosaccharides [Citation56–59], galactooligosaccharides [Citation56,Citation58,Citation60–64], 2′-fucosyllactose [Citation8,Citation7,Citation11] or mixes of these oligosaccharides [Citation31,Citation32] have all been shown to impact cognition. Few of these have been measured in human trials.
2′-Fucosyllactose in milk at 1 month of age was recently found to mediate the relationship between breast feeding frequency at 1 month of age and performance on the Bayley-III at 24 months of age [Citation14] in human infants. However, this relationship did not hold at 6 months of age. The authors suggest this may be evidence of a critical window during early development. Of note, however, is that disialyllacto-N-tetraose at 1 month of age was inversely related to cognitive performance, and at 6 months of age lacto-N-hexaose, fucosyl-lacto-N-hexaose, and sialyl-lacto-N-tetraose b were all related to cognitive performance [Citation14]. In an observational study of Malawian mother-infant dyads, Jorgensen et al. [Citation65] identified relationships between infant cognition and maternal bioactives that were dependent on the secretor status of the mother. In mothers that secrete 2′FL, the relative abundance of fucosylated and sialyllated HMO were positively associated with language at 18 months of age. Multiple HMO were also positively associated with motor skills in dyads from mothers that do not secrete 2′FL. Ultimately, many HMO likely play a role in cognition.
In light of significant evidence implicating a link between multiple oligosaccharides of various compositions and cognition, it is unclear why the present study suggests this only occurred for sialyllactose alone. A variety of factors may be at play: synergistic effects between oligosaccharides, different animal models used, various methods employed to test cognition, doses, etc. Beyond methodological and dietary differences, the reason behind the discrepancy may not surface until further mechanistic research is performed. Even for studies of single, discrete compounds (i.e. the structure of 2′-FL or 3′-SL is consistent, whereas galactooligosaccharide may have various degrees of polymerization and branching) the true mechanism is not known. Hypotheses surrounding the gut–brain-axis are largely divided into direct effects (via the vagus nerve) or indirect effects (absorption of metabolites/bioactives or modulation of the immune system) [Citation66].
HMO minimally impacted emotional reactivity, motivation to obtain food rewards, or appetite
Ultimately, the effects of the diet on behavioral measures of emotional reactivity and exploratory behavior were minimal. At no time was exploratory behavior during the Open Field or Novel Object tasks different between formula-fed groups. During Period 1, formula-fed groups tended to spend more time exhibiting explorative vs. non-explorative behaviors as compared to the Control group, however this was variable and did not reach significance. During Period 2, the Control and FN groups tended to vocalize more during the Open Field task, but again such differences were minimal. During the Novel Object task, the SL and FN+ SL tended to spend a greater percentage of their time approaching the novel object than other groups, but not actively exploring the novel object.
During home pen observations, the behavior was equivalent at nearly all times measured. During Period 1, the FN group displayed greater ingestive behaviors, but these did not translate to differences in body weight gain. There was no difference in performance between any of the groups on either the runway task at any age or the single-feed test. Such results suggest that the effects on cognition arose not out of alterations to the hedonic sensation or perception of the rewards used. Together with the lack of effect on exploratory behavior and general reactivity these results suggest that across groups pigs were in similar motivational states. Thus, the effects on cognition observed in the holeboard task may reasonably be inferred to arise out of some alteration to learning and memory processes and not sensation, perception, stress, or emotional processing.
Biological indices of emotional excitation measured such as heart rate activity and cortisol also revealed few-to-no differences between groups. All groups tended to have lower heart rates when not in transport to and from behavioral testing. Salivary cortisol was variable between and within-groups, however, the FN + SL group had, on average, lower salivary cortisol than all other groups regardless of time point at Period 3. Specifically, pigs fed FN + SL showed lower salivary cortisol 15 and 60 min after the start of testing. This effect is similar to that found by Schmidt et al. [Citation67], who demonstrated adult humans provided galactooligosaccharide had a smaller change in waking cortisol than controls.
Previous studies have shown that various prebiotics elicited an anxiolytic effect on animals exposed to social stressors [Citation6,Citation68], lipopolysaccharide injection [Citation60], and chronic unpredictable mild stress [Citation69,Citation70]. While salivary cortisol was reduced in the FN + SL group, behavior appeared to be unaffected. The results herein are largely discordant with previous data on prebiotics and emotion-related outcomes. It may be likely that the magnitude of stress measured in this study was too low and oligosaccharides would have provided a protective effect in the face of a greater stressor. The stressors used in previous studies were arguably more disruptive than the present use of an open field and novel object.
All together our results demonstrate minimal effects on behaviors related to emotional reactivity in response to a modest stressor; however, the majority of data from previous studies largely support the hypothesis that prebiotics confer a protective effect against many stressors.
Conclusions
We conducted a controlled feeding trial in Göttingen minipigs to address the potential effects of early-life dietary intake of human milk oligosaccharides on cognitive development. During sexual development, sialyllactose improved reference memory during a reversal task, indicative of improved cognitive flexibility. No impact of any tested oligosaccharide mixture was found on emotional reactivity, motivation, or appetite. The mechanisms underlining this cognitive improvement are unknown but suggest discrete improvements to cognitive domains related to learning, memory, and inhibitory control.
Declaration of interest
JH is an employee of Société des Produits Nestlé (Nestlé) and SF is an owner and employee of Traverse Science. The study was funded by Nestlé. SJK and TS received funding from Nestlé to execute the study. JH had a role in the study design, interpretation and manuscript writing but did not execute the study or perform any statistical analyses. SF received funding from Nestlé to perform manuscript writing services. No funding was provided by Traverse Science. CC, IR, SJK, TS performed the study, statistical analyses and manuscript writing. The remaining authors declare no other conflicts of interest, financial or otherwise.
Supplemental Material
Download Zip (381.8 KB)Disclosure statement
No potential conflict of interest was reported by the author(s).
Data availability statement
Data may be made available upon request.
Additional information
Notes on contributors
Caroline Clouard
Caroline Clouard obtained her Ph.D. on food preferences and aversions in pigs at the French National Institute for Agricultural Research (INRA), France, in 2012. She then worked as a postdoctoral researcher at Wageningen University and Research, The Netherlands, for 4 years. Since 2019, she is working as a researcher at the French National Institute for Agriculture, Food and Environment (INRAE), France. Her research interests are pig behavior and welfare, with a focus on social behavior, cognition and positive emotions.
Inonge Reimert
Inonge Reimert obtained her Ph.D. at Wageningen University and Research in 2014. Thereafter she stayed at this university first as a postdoctoral research and since 2021 as a senior researcher. Her work is about pig behaviour and welfare by studying, for example, the effect of enrichment on pig behaviour as well as how pigs express positive and negative affective states.
Sietse-Jan Koopmans
Sietse Jan Koopmans obtained his Ph.D. at Leiden University Medical Center (LUMC), The Netherlands in 1991. He then worked as a postdoctoral researcher at the University of Texas Health Science Center at San Antonio, USA. After his return to LUMC, he moved to Wageningen University and Research, The Netherlands as a researcher on nutrition, metabolism and physiology. His research topics include the use of various pig models for metabolic and cardiovascular diseases and to study the mode of action of diet and functional foods.
Teun Schuurman
Teun Schuurman obtained his Ph.D. at Groningen University, the Netherlands, in 1981. He then worked as a postdoctoral researcher and later on as department head at Bayer CNS research in Cologne, Germany. In 1994 he was appointed as professor of behavioral- and neurobiology at Wageningen University, the Netherlands. His research interests included the preclinical development of drugs for neuropsychiatric diseases and the development of pig models for food and pharma research. He retired recently.
Jonas Hauser
Jonas Hauser obtained his Ph.D. at the Swiss Institute of Technology - Zurich in 2007. He then worked as a postdoctoral researcher at the Swiss Institute of Technology - Zurich and University College London prior to joining the Nestlé Institute for Health Sciences as a senior specialist in cognition. His topics include nutrition to support neurodevelopment, nutrition to help mitigate neurodevelomental disease symptoms and the cellular and molecular mechanisms mediating these nutritional effects.
References
- Bode L. Human milk oligosaccharides: Every baby needs a sugar mama. Glycobiology. 2012;22:1147–62. doi:10.1093/glycob/cws074.
- Donovan SM, Comstock SS. Human milk oligosaccharides Influence neonatal mucosal and systemic immunity. Ann Nutr Metab. 2016;69(Suppl 2):42–51. doi:10.1159/000452818.
- Kirmiz N, Robinson RC, Shah IM, Barile D, Mills DA. Milk glycans and their interaction with the infant-Gut microbiota. Annu Rev Food Sci Technol. 2018;9:429–50. doi:10.1146/annurev-food-030216-030207.
- Urashima T, Saito T, Nakamura T, Messer M. Oligosaccharides of milk and colostrum in non-human mammals. Glycoconj J. 2001;18:357–71. doi:10.1023/A:1014881913541.
- Sakai F, Ikeuchi Y, Urashima T, Fujihara M, Ohtsuki K, Yanahira S. Effects of feeding sialyllactose and galactosylated N-acetylneuraminic acid on swimming learning ability and brain lipid composition in adult rats. J Appl Glycosci. 2006;53:249–54. doi:10.5458/jag.53.249.
- Tarr AJ, Galley JD, Fisher SE, Chichlowski M, Berg BM, Bailey MT. The prebiotics 3′sialyllactose and 6′sialyllactose diminish stressor-induced anxiety-like behavior and colonic microbiota alterations: evidence for effects on the gut–brain axis. Brain Behav Immun. 2015;50:166–77. doi:10.1016/j.bbi.2015.06.025.
- Vázquez E, Barranco A, Ramírez M, Gruart A, Delgado-García JM, Martínez-Lara E, et al. Effects of a human milk oligosaccharide, 2′-fucosyllactose, on hippocampal long-term potentiation and learning capabilities in rodents. J Nutr Biochem. 2015;26:455–65. doi:10.1016/j.jnutbio.2014.11.016.
- Vázquez E, Barranco A, Ramírez M, Gruart A, Delgado-García JM, Jimenez ML, et al. Dietary 2’-fucosyllactose enhances operant conditioning and long-term potentiation via gut-brain communication through the vagus nerve in rodents. PLoS One. 2016;11:1–14. doi:10.1371/journal.pone.0166070.
- Jacobi SK, Yatsunenko T, Li D, Dasgupta S, Yu RK, Berg BM, et al. Dietary isomers of sialyllactose increase ganglioside sialic Acid Concentrations in the Corpus Callosum and cerebellum and modulate the colonic Microbiota of formula-Fed piglets. J Nutr. 2016;146:200–8. doi:10.3945/jn.115.220152.
- Kao ACC, Harty S, Burnet PWJ. The Influence of prebiotics on neurobiology and behavior. Int Rev Neurobiol. 2016;131:21–48. doi:10.1016/bs.irn.2016.08.007.
- Oliveros E, Ramirez M, Vazquez E, Barranco A, Gruart A, Delgado-Garcia JM, et al. Oral supplementation of 2′-fucosyllactose during lactation improves memory and learning in rats. J Nutr Biochem. 2016;31:20–7. doi:10.1016/j.jnutbio.2015.12.014.
- Mudd AT, Fleming SA, Labhart B, Chichlowski M, Berg BM, Donovan SM, et al. Dietary Sialyllactose influences Sialic Acid Concentrations in the prefrontal cortex and magnetic resonance imaging Measures in Corpus Callosum of Young pigs. Nutrients. 2017;9:1297. doi:10.3390/nu9121297.
- Obelitz-Ryom K, Bering SB, Overgaard SH, Eskildsen SF, Ringgaard S, Olesen JL, et al. Bovine Milk Oligosaccharides with sialyllactose improves cognition in preterm pigs. Nutrients. 2019;11:1335. doi:10.3390/nu11061335.
- Berger PK, Plows JF, Jones RB, Alderete TL, Yonemitsu C, Poulsen M, et al. Human milk oligosaccharide 2’-fucosyllactose links feedings at 1 month to cognitive development at 24 months in infants of normal and overweight mothers. PLoS One. 2020;15:e0228323. doi:10.1371/journal.pone.0228323.
- Hauser J, Pisa E, Arias Vásquez A, Tomasi F, Traversa A, Chiodi V, et al. Sialylated human milk oligosaccharides program cognitive development through a non-genomic transmission mode. Mol Psychiatry. 2021. doi:10.1038/s41380-021-01054-9.
- McGuire MK, Meehan CL, McGuire MA, Williams JE, Foster J, Sellen DW, et al. What’s normal? Oligosaccharide concentrations and profiles in milk produced by healthy women vary geographically. Am J Clin Nutr. 2017: ajcn 139980. doi:10.3945/ajcn.116.139980.
- Nishtar S, Gluckman P, Armstrong T. Ending childhood obesity: a time for action. Lancet (London, England). 2016;387:825–7. doi:10.1016/S0140-6736(16)00140-9.
- Isganaitis E, Venditti S, Matthews TJ, Lerin C, Demerath EW, Fields DA. Maternal obesity and the human milk metabolome: associations with infant body composition and postnatal weight gain. Am J Clin Nutr. 2019;110:111–20. doi:10.1093/ajcn/nqy334.
- Alderete TL, Autran C, Brekke BE, Knight R, Bode L, Goran MI, et al. Associations between human milk oligosaccharides and infant body composition in the first 6 mo of life. Am J Clin Nutr. 2015;102:1381–8. doi:10.3945/ajcn.115.115451.
- Reinert KRS, Po’e EK, Barkin SL. The relationship between executive function and obesity in children and adolescents: a systematic literature review. J Obes. 2013;2013:820956. doi:10.1155/2013/820956.
- Galván M, Uauy R, López-Rodríguez G, Kain J. Association between childhood obesity, cognitive development, physical fitness and social-emotional wellbeing in a transitional economy. Ann Hum Biol. 2014;41:101–6. doi:10.3109/03014460.2013.841288.
- Wang C, Chan JSY, Ren L, Yan JH. Obesity reduces cognitive and motor functions across the lifespan. Neural Plast. 2016;2016; doi:10.1155/2016/2473081.
- Li N, Yolton K, Lanphear BP, Chen A, Kalkwarf HJ, Braun JM. Impact of early-life weight status on cognitive abilities in children. Obesity. 2018;26:1088–95. doi:10.1002/oby.22192.
- Litten-Brown JC, Corson AM, Clarke L. Porcine models for the metabolic syndrome, digestive and bone disorders: a general overview. Animal. 2010;4:899–920. doi:10.1017/S1751731110000200.
- Odle J, Lin X, Jacobi SK, Kim SW, Stahl CH. The suckling piglet as an agrimedical model for the study of pediatric nutrition and metabolism. Annu Rev Anim Biosci. 2014;2:419–44. doi:10.1146/annurev-animal-022513-114158.
- Lind NM, Moustgaard A, Jelsing J, Vajta G, Cumming P, Hansen AK. The use of pigs in neuroscience: modeling brain disorders. Neurosci Biobehav Rev 2007;31:728–51. doi:10.1016/j.neubiorev.2007.02.003.
- Gieling ET, Nordquist RE, van der Staay FJ. Assessing learning and memory in pigs. Anim Cogn. 2011;14:151–73. doi:10.1007/s10071-010-0364-3.
- Conrad MS, Dilger RN, Johnson RW. Brain growth of the domestic pig (Sus scrofa) from 2 to 24 weeks of age: a longitudinal MRI study. Dev Neurosci. 2012;34:291–8. doi:10.1159/000339311.
- Conrad MS, Johnson RW. The domestic piglet: An important model for investigating the neurodevelopmental consequences of early life insults. Annu Rev Anim Biosci. 2015;3:245–64. doi:10.1146/annurev-animal-022114-111049.
- Mudd AT, Dilger RN. Early-life nutrition and neurodevelopment: use of the piglet as a translational model. Adv Nutr. 2017;8:92–104. doi:10.3945/an.116.013243.
- Fleming SA, Mudd AT, Hauser J, Yan J, Metairon S, Steiner P, et al. Dietary oligofructose alone or in combination with 2′-fucosyllactose differentially improves Recognition memory and hippocampal mRNA expression. Nutrients. 2020;12:2131. doi:10.3390/nu12072131.
- Fleming SA, Mudd AT, Hauser J, Yan J, Metairon S, Steiner P, et al. Human and bovine milk oligosaccharides elicit improved recognition memory concurrent with alterations in regional brain volumes and hippocampal mRNA expression. Front Neurosci. 2020;14:1–14. doi:10.3389/fnins.2020.00770.
- Peter B, De Rijk EPCT, Zeltner A, Emmen HH. Sexual maturation in the female Göttingen minipig. Toxicol Pathol. 2016;44:482–5. doi:10.1177/0192623315621413.
- van der Staay FJ, Gieling ET, Pinzón NE, Nordquist RE, Ohl F. The appetitively motivated “cognitive” holeboard: A family of complex spatial discrimination tasks for assessing learning and memory. Neurosci Biobehav. Rev. 2012;36:379–403. doi:10.1016/j.neubiorev.2011.07.008.
- Mudd AT, Salcedo J, Alexander LS, Johnson SK, Getty CM, Chichlowski M, et al. Porcine milk oligosaccharides and Sialic Acid Concentrations vary throughout lactation. Front Nutr. 2016;3; doi:10.3389/fnut.2016.00039.
- Nadel L, Hardt O. Update on memory systems and processes. Neuropsychopharmacology. 2011;36:251–73. doi:10.1038/npp.2010.169.
- Gieling ET, Antonides A, Fink-Gremmels J, ter Haar K, Kuller WI, Meijer E, et al. Chronic allopurinol treatment during the last trimester of pregnancy in sows: effects on Low and normal birth weight offspring. PLoS One. 2014;9:e86396. doi:10.1371/journal.pone.0086396.
- Antonides A, Schoonderwoerd AC, Scholz G, Berg BM, Nordquist RE, van der Staay FJ. Pre-weaning dietary iron deficiency impairs spatial learning and memory in the cognitive holeboard task in piglets. Front Behav Neurosci. 2015;9; doi:10.3389/fnbeh.2015.00291.
- Elmore MRP, Dilger RN, Johnson RW. Place and direction learning in a spatial T-maze task by neonatal piglets. Anim Cogn. 2012;15:667–76. doi:10.1007/s10071-012-0495-9.
- Dilger RN, Johnson RW. Behavioral assessment of cognitive function using a translational neonatal piglet model. Brain Behav Immun. 2010;24:1156–65. doi:10.1016/j.bbi.2010.05.008.
- Izquierdo A, Brigman JL, Radke AK, Rudebeck PH, Holmes A. The neural basis of reversal learning: An updated perspective. Neuroscience. 2017;345:12–26. doi:10.1016/j.neuroscience.2016.03.021.
- Pagani JH, Brown KL, Stanton ME. Contextual modulation of spatial discrimination reversal in developing rats. Dev Psychobiol. 2005;46:36–46. doi:10.1002/dev.20041.
- Watson DJ, Sullivan JR, Frank JG, Stanton ME. Serial reversal learning of position discrimination in developing rats. Dev Psychobiol. 2006;48:79–94. doi:10.1002/dev.20106.
- Saperstein LA, Kucharski D, Stanton ME, Hall WG. Developmental change in reversal learning of an olfactory discrimination. Psychobiology. 1989;17:293–9. doi:10.1007/BF03337783.
- Willing J, Drzewiecki CM, Cuenod BA, Cortes LR, Juraska JM. A role for puberty in water maze performance in male and female rats. Behav Neurosci. 2016;130:422–7. doi:10.1037/bne0000145.
- Wang B, Yu B, Karim M, Hu H, Sun Y, McGreevy P, et al. Dietary sialic acid supplementation improves learning and memory in piglets. Am J Clin Nutr. 2007;85:561–9. doi:10.1093/ajcn/85.2.561.
- Fleming S, Chichlowski M, Berg B, Donovan S, Dilger R. Dietary sialyllactose does not influence measures of recognition memory or diurnal activity in the young pig. Nutrients. 2018;10:395. doi:10.3390/nu10040395.
- Palmano K, Rowan A, Guillermo R, Guan J, McJarrow P. The role of gangliosides in neurodevelopment. Nutrients. 2015;7:3891–913. doi:10.3390/nu7053891.
- Vickers MH, Guan J, Gustavsson M, Krägeloh CU, Breier BH, Davison M, et al. Supplementation with a mixture of complex lipids derived from milk to growing rats results in improvements in parameters related to growth and cognition. Nutr Res. 2009;29:426–35. doi:10.1016/j.nutres.2009.06.001.
- Chen Y, Zheng Z, Zhu X, Shi Y, Tian D, Zhao F, et al. Lactoferrin promotes early neurodevelopment and cognition in postnatal piglets by upregulating the BDNF signaling pathway and polysialylation. Mol Neurobiol. 2015;52:256–69. doi:10.1007/s12035-014-8856-9.
- Wang B. Molecular mechanism underlying sialic acid as an essential Nutrient for brain development and cognition. Adv Nutr. 2012;3:465S–472S. doi:10.3945/an.112.001875.
- Laube C, van den Bos W, Fandakova Y. The relationship between pubertal hormones and brain plasticity: implications for cognitive training in adolescence. Dev Cogn Neurosci. 2020;42:100753. doi:10.1016/j.dcn.2020.100753.
- Koss WA, Belden CE, Hristov AD, Juraska JM. Dendritic remodeling in the adolescent medial prefrontal cortex and the basolateral amygdala of male and female rats. Synapse. 2014;68:61–72. doi:10.1002/syn.21716.
- Drzewiecki CM, Willing J, Juraska JM. Synaptic number changes in the medial prefrontal cortex across adolescence in male and female rats: A role for pubertal onset. Synapse. 2016;70:361–8. doi:10.1002/syn.21909.
- Jia S, Lu Z, Gao Z, An J, Wu X, Li X, et al. Chitosan oligosaccharides alleviate cognitive deficits in an amyloid-β1–42-induced rat model of Alzheimer’s disease. Int J Biol Macromol. 2016;83:416–25. doi:10.1016/j.ijbiomac.2015.11.011.
- Burokas A, Arboleya S, Moloney RD, Peterson VL, Murphy K, Clarke G, et al. Targeting the microbiota-gut-brain axis: prebiotics have anxiolytic and antidepressant-like effects and reverse the impact of chronic stress in mice. Biol Psychiatry. 2017;82:472–87. doi:10.1016/j.biopsych.2016.12.031.
- Chen D, Yang X, Yang J, Lai G, Yong T, Tang X, et al. Prebiotic effect of fructooligosaccharides from Morinda officinalis on Alzheimer’s disease in rodent models by targeting the microbiota-gut-brain axis. Front Aging Neurosci. 2017;9:403. doi:10.3389/fnagi.2017.00403.
- Savignac HM, Corona G, Mills H, Chen L, Spencer JPE, Tzortzis G, et al. Prebiotic feeding elevates central brain derived neurotrophic factor, N-methyl-d-aspartate receptor subunits and d-serine. Neurochem Int. 2013;63:756–64. doi:10.1016/j.neuint.2013.10.006.
- Yen C-H, Wang C-H, Wu W-T, Chen H-L. Fructo-oligosaccharide improved brain β-amyloid, β-secretase, cognitive function, and plasma antioxidant levels in D-galactose-treated balb/cJ mice. Nutr Neurosci. 2017;20:228–37. doi:10.1080/1028415X.2015.1110952.
- Savignac HM, Couch Y, Stratford M, Bannerman DM, Tzortzis G, Anthony DC, et al. Prebiotic administration normalizes lipopolysaccharide (LPS)-induced anxiety and cortical 5-HT2A receptor and IL1-β levels in male mice. Brain Behav Immun. 2016;52:120–31. doi:10.1016/j.bbi.2015.10.007.
- Williams S, Chen L, Savignac HM, Tzortzis G, Anthony DC, Burnet PW. Neonatal prebiotic (BGOS) supplementation increases the levels of synaptophysin. GluN. 2A), -subunits and BDNF proteins in the adult rat hippocampus. Synapse. 2016;70:121–4. doi:10.1002/syn.21880.
- Fleming SA, Monaikul S, Patsavas AJ, Waworuntu RV, Berg BM, Dilger RN. Dietary polydextrose and galactooligosaccharide increase exploratory behavior, improve recognition memory, and alter neurochemistry in the young pig. Nutr Neurosci. 2017;22:499–512. doi:10.1080/1028415X.2017.1415280.
- Gronier B, Savignac HM, Di Miceli M, Idriss SM, Tzortzis G, Anthony D, et al. Increased cortical neuronal responses to NMDA and improved attentional set-shifting performance in rats following prebiotic (B-GOS®) ingestion. Eur Neuropsychopharmacol. 2018;28:211–24. doi:10.1016/j.euroneuro.2017.11.001.
- Kao A, Spitzer S, Anthony DC, Lennox B, Burnet PWJ. Prebiotic attenuation of olanzapine-induced weight gain in rats: analysis of central and peripheral biomarkers and gut microbiota. Transl Psychiatry. 2018;8:66. doi:10.1038/s41398-018-0116-8.
- Jorgensen JM, Young R, Ashorn P, Ashorn U, Chaima D, Davis JCC, et al. Associations of human milk oligosaccharides and bioactive proteins with infant growth and development among Malawian mother-infant dyads. Am J Clin Nutr. 2020: 1–12. doi:10.1093/ajcn/nqaa272.
- Sampson TR, Mazmanian SK. Control of brain development, function, and behavior by the microbiome. Cell Host Microbe. 2015;17:565–76. doi:10.1016/j.chom.2015.04.011.
- Schmidt K, Cowen PJ, Harmer CJ, Tzortzis G, Errington S, Burnet PWJ. Prebiotic intake reduces the waking cortisol response and alters emotional bias in healthy volunteers. Psychopharmacology (Berl). 2015;232:1793–801. doi:10.1007/s00213-014-3810-0.
- Thompson RS, Roller R, Mika A, Greenwood BN, Knight R, Chichlowski M, et al. Dietary prebiotics and bioactive milk fractions improve NREM Sleep, enhance REM Sleep rebound and attenuate the stress-induced decrease in diurnal temperature and Gut microbial alpha diversity. Front Behav Neurosci. 2017;10:240. doi:10.3389/fnbeh.2016.00240.
- Xu L-Z, Xu D-F, Han Y, Liu L-J, Sun C-Y, Deng J-H, et al. BDNF-GSK-3β-β-Catenin Pathway in the mPFC Is involved in antidepressant-like effects of Morinda officinalis oligosaccharides in rats. Int J Neuropsychopharmacol. 2017;20:83–93. doi:10.1093/ijnp/pyw088.
- Li H, Wang P, Huang L, Li P, Zhang D. Effects of regulating gut microbiota on the serotonin metabolism in the chronic unpredictable mild stress rat model. Neurogastroenterol Motil. 2019;31:e13677. doi:10.1111/nmo.13677.