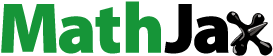
ABSTRACT
This study aims to present a multi-criteria decision analysis (MCDA) for comprehensive performance evaluation of the alternative design of timber–concrete composite (TCC) floor system. Considered objectives are serviceability and sustainability performance with associated criterion as (1) comfort class regarding springiness and vibrations, (2) architectural quality with associated criterion as open spaces, (3) environmental aspect with associated criterion as CO2 emissions and (4) cost aspect with associated criterion as the total costs. Analytical Hierarchy Process (AHP) and Complex Proportional Assessment (COPRAS) as the methods in the multi-criteria analysis have been combined for (1) determining the weighting of criteria based on the survey results, (2) verifying the consistency ratio of decision matrix made by experts and (3) for ranking and selecting the optimal concept design among design candidates. According to the results, the TCC floor with the span length of 7.3 m belonging to comfort class A has got the highest ranking. However, sensitivity analysis indicates that the TCC floor with a 9.0 m span length belonging to comfort class A shall be selected as the optimal concept design. The study contributes by developing a complete concept design tool for TCC floor systems using AHP combined COPRAS methods to handle both beneficial and non-beneficial criteria.
Introduction
Floor system as the major structural element in residential multi-storey wooden buildings regarding its volume mass has the significant potentials for being improved towards the sustainable structural element. Floor systems in multi-storey wooden buildings are now undergoing a revival in two different areas (Moya et al., Citation2017). On the one hand, the development of new Engineered Wood Products and, on the other, architectural and design innovation with improved overall sustainability (Russell-Smith et al., Citation2015) using both composite building materials and systems (Movaffaghi, Pyykkö, and Yitmen Citation2020) and low carbon footprint materials (Woodard and Milner Citation2016; Stocchero et al. Citation2017; Balasbaneh and Bin Marsono Citation2018; Balasbaneh, Marsono, and Khaleghi Citation2018).
Timber–concrete composite (TCC) floor systems with two major different configurations are emerging as an efficient solution for the floors in multi-storey wooden buildings. They generally comprise a cross-laminated timber (CLT) plate or glulam beams connected to a topping of concrete. In this paper, the research focus is on the glulam beams supported TCC floor system. TCC floor systems offer several advantages compared to the traditional timber floor system in terms of serviceability performances, i.e. stiffness, vibrational behaviour, fire and acoustic insulation (Naud et al. Citation2019). Besides TCC floor systems provide structural improvements over conventional light-frame wood flooring systems including bending and shear stiffness, strength and reduction of floor thickness (Dias et al. Citation2016; Movaffaghi, Pyykkö, and Yitmen Citation2020). Another advantage of the TCC floor system is the rigidity of the TCC floor in the plane of the floor, which means that horizontal loads caused by, for example, wind, can be distributed evenly over the vertical load-bearing elements such as columns and shear walls. The efficiency of TCC floor systems depends largely on the properties of the shear connection between timber and concrete. Connectors range from low stiffness, where partial composite action is achieved, to rigid connectors where almost full composite action fulfilled.
The building sector could consider an understanding of long-span TCC floor systems where improved vibration and acoustic performance is required, while at the same time achieving sustainable building solutions (Neve and Spencer-Allen, Citation2015). Flexibility in architectural design is of paramount importance. An open floor plan achievable by long-span floor systems allows a great variety of uses of the building which may change over the time. Flexibility in high-rise buildings is even more important since the same building could be used for different purposes at each storey. Long-span TCC floor systems involve efficient construction methods for industrialised multi-storey wooden buildings. They are not only robust and reliable but also economical and sustainable with minimum impact on the environment (Khorsandnia et al., Citation2016; Naud et al. Citation2019). It is very important to understand the environmental suitability and sustainability of these type of long-span composite structures (Balasbaneh, Marsono, and Khaleghi Citation2018) with a reduction in material usage.
Architects, structural engineers, and technical and production managers are encouraged to adopt multi-criteria decision analysis (MCDA) for ranking and selecting concept design in the early design stage. Designing TCC floor systems involve several performance objectives and associated criteria that need to be considered simultaneously. Besides designing TCC floor systems require an evaluation of multifunctional objectives involving serviceability and sustainability including comfort class, architectural, environmental and cost performances. With advances in technology and the growing performance expectations from building systems and structural components, application of MCDA is receiving increasing attention in the construction industry (Balcomb and Curtner Citation2000; Hopfe et al. Citation2012). Presently, engineers are shifting from traditional design methods that were mostly based on experience, to the application of systematic decision-making procedures to achieve the optimal performance in their design alternatives (Brauers et al. Citation2008; Zavadskas, Liias, and Turskis Citation2008; Si et al. Citation2016). This study as the continuation of the previous study (Movaffaghi, Pyykkö, and Yitmen Citation2020) deals with developing a complete concept design tool for TCC floor systems using MCDA. Alternative designs are 7.3 m as medium and 9.0 m as long-span lengths, each involving three different comfort classes A, B and C in descending order. Classification of the floors into three comfort classes A, B and C is according to the proposal of Toratti and Talja (Citation2006) where class C is the base class. Each comfort class has its maximum limiting values for the point load deflection for a 1 kN static load at the most flexible points of the slab. The maximum deflection for comfort class A, B and C are 0.12, 0.25 and 0.50 mm, respectively. By applying the point load deflection criteria for each vibration class, the minimum bending stiffness fulfilling the limiting values have been extracted from the deflection formula. Considered objectives are serviceability and sustainability performance with associated criterion as (1) comfort class regarding springiness and vibrations, (2) architectural quality with associated criterion as open spaces, (3) environmental aspect with associated criterion as CO2 emissions and (4) cost aspect with associated criterion as the total costs. A survey has been administered to the technical and production managers working at 20 major industrialised house-building companies on both Swedish and European market. Analytical Hierarchy Process (AHP) and Complex Proportional Assessment (COPRAS) as the methods in MCDA have been combined for (1) determining the weighting of criteria based on experts’ judgement, (2) verifying the consistency ratio of decision matrix from experts’ judgement and (3) ranking and selecting the optimal concept design among design candidates. Movaffaghi, Pyykkö, and Yitmen (Citation2020) have developed a framework for multi-criteria based approach for designing optimal TCC floor system in industrialised multi-storey wooden buildings. The flowchart of the framework for multi-criteria based approach of TCC floor systems that have been used in this study is presented in (Movaffaghi, Pyykkö, and Yitmen Citation2020).
Figure 1. Flowchart of the developed framework for the application of MCDA (Movaffaghi, Pyykkö, and Yitmen Citation2020).
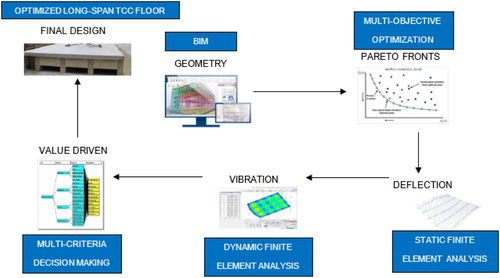
This article is structured as follows: firstly, structural performance of alternative TCC floors with two span lengths and three different comfort classes have been analysed to fulfil the serviceability requirements according to the Euro code 5. Secondly, AHP and COPRAS as the methods in MCDA have been combined for determining the weighting of the criteria, verifying the consistency ratio of decision matrix and prioritising, and selecting the optimal concept design. To examine the extent of variation in the predicted concept design when parameters are systematically varied over some range of interest, a sensitivity analysis has been performed. Finally, results are discussed, and some important conclusions are drawn and recommendations for future work are suggested.
TCC floor system fulfilling serviceability requirements
The serviceability performance with associate criteria as static deflection and fundamental natural frequency are often the governing design factor for TCC floor systems (Tannert et al. Citation2017). Meanwhile, there is a close relationship between static deflection and fundamental natural frequency of the floor (Suárez-Riestra et al. Citation2019). Thus, the deflection criteria both under short-term and long-term loading and fundamental natural frequency need to be considered for the design of the TCC floors. At present, the most widely accepted analytical approach for the evaluation of serviceability performance of the TCC floors is the so-called γ-method, which is provided in Annex B of Eurocode 5 (CEN, Citation2004). According to the γ-method, the effective bending stiffness of the composite section depends largely on the degree of composite action. For simplification, the shear connectors are assumed to be uniformly distributed along the span. The method makes use of a factor, called γ-factor, which ranges from 0 to 1. The γ-factor can be regarded as the degree of composite action, it being 0 for no composite action and 1 for full composite action. Since concrete cover in TCC floor systems works mainly in compression, recent works have attempted to minimise the thickness of the concrete cover by exploiting the high compressive strength (fc≈120–180 MPa) and high elastic modulus (Ec≈50–60 GPa) of Ultra-High Performance Fiber Reinforced Concrete (Ferrier, Labossiere, and Neale Citation2009; Graybeal, Citation2008; Baril et al. Citation2016).
Based on the proposed design tools by Movaffaghi, Pyykkö, and Yitmen (Citation2020), presented in , two long-span floor system, 7.3 and 9.0 m, have been designed to fulfil serviceability performances. The TCC floor system consists of glulam beams and reinforced concrete cover, joined by shear connectors. Cross-section of the investigated simply supported TCC floor system is shown in .
Serviceability performance with associated criteria as the maximum point load deflection caused by 1 kN point load at the middle of TCC floor and fundamental natural frequency have been analysed. Alternative designs are simply supported TCC floors with the dimensions each with three different comfort classes, A, B and C in descending order. The concrete layer has been chosen as 50 mm to fulfil minimum requirements for fire protection. A summary of the calculation results regarding the cross-section area of the glulam beam belonging to comfort class A, B and C and beam length 7.3 and 9.0 m is shown in .
Table 1. Cross-section area of the glulam beams for the floors belonging to comfort class A, B and C and beam length 7.3 and 9.0 (m) fulfilling associated criteria as maximum point load deflection and fundamental natural frequency.
Multi-criteria decision analysis
The technological cooperation of architects, structural engineers, and technical and production managers is the key to success in building design. Decision problems in the early design of TCC floors require a multi-criteria approach, involving a variety of often conflicting decision factors such as comfort class and span length. Thus, methods of MCDA can provide essential supporting tools for decision-making purposes (Zavadskas, Turskis, and Kildienė Citation2014; Ogrodnik Citation2019). MCDA deals with decisions involving the choice of an optimal concept design from several potential candidates in a decision, subject to several performance objectives and associated criteria (Cecconi et al. Citation2017). The aim of MCDA is to investigate the interaction of design parameters in the early stage of the design process in achieving a more value-driven design by considering the preferences of the stakeholders and experts. There exist several methods in multi-criteria analysis such as AHP and AHP combined COPRAS methods (Medineckienė, Turskis, and Zavadskas Citation2011). AHP has been used for the determination of the significance of criteria, which compares criteria and for alternative ranking based on Saaty’s scales for pairwise comparisons. COPRAS method has been applied to the various problems in construction (Kaklauskas et al. Citation2010; Tupenaite et al. Citation2010; Zavadskas et al. Citation2010). COPRAS method has been used for alternatives’ ranking and obtaining the most optimal solution (Stević Citation2016). COPRAS was first introduced by Zavadskas et al. (Citation1994). In decision-making, when it is necessary to consider the impact of multiple-criteria, which usually have different significance as beneficial or non-beneficial, COPRAS method provides an effective and understandable procedure than AHP-based ranking for such purposes (Popovic, Stanujkic, and Stojanovic Citation2012).
Performance objectives and criteria
Serviceability performance evaluation together with sustainability performance evaluation (Japan Research Institute Citation2003) need to be integrated in the design of TCC floors for the sustainable outcome. Sustainability performance in this paper has been divided into three categories, namely architectural, environmental and cost performances, as is shown in . For each performance objective/category, three most important criteria have been identified as the most important design aspects for TCC floors in multi-storey wooden buildings. Short and long-term deflections together with floors comfort class A, B and C revealing vibration responses of the floor to footfall-induced excitations (Yeoh et al. Citation2008) have been identified as the three most important criteria for serviceability performance evaluation. Open spaces by span lengths, floor thickness and daylight have been taken into consideration as the criteria for architectural performance evaluation. For environmental performance evaluation, global warming, resource use and indoor air quality have been identified as the most important. Finally, material, production and total costs have been identified as the most important criteria for cost performance in this study based on relevant literature studies, and authors’ experiences.
Figure 3. Performance objectives, categories and associated three most important criteria breakdown structure for response evaluation of TCC floor systems.
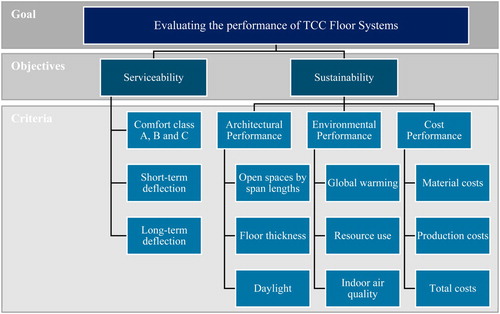
Among the three candidates’ criteria for each performance objective in , one criterion has been chosen for further studies as the most important criterion for designing TCC floors. Selection has been based on both authors’ experiences and reviewed literature (Dias et al. Citation2016; Martins et al. Citation2016; Jelusic and Kravanja Citation2017; Daňková, Mec, and Šafrata Citation2019; Sorelli et al. Citation2019; Xie et al. Citation2020). The indicators for the selected performance criteria are presented in .
Table 2. Selected criteria and corresponding indicators with its unit of measurements for response evaluation of TCC floors.
AHP method for multi-criteria decision analysis
According to Saaty (Citation1990), the basic purpose of AHP is to create one structure with all the essential criteria governing the outcome of a decision. AHP method allows the decision-maker to consider an arbitrary number of criteria of different kinds in the decision-making situation. The calculation steps of AHP are presented as follows (Saaty Citation1990). In the first step, the pairwise comparisons of the criteria are made of experts and stakeholders based on a nine-degree scale (Saaty Citation1980) presented in .
Table 3. Definition of fundamental weighting scale in the pairwise comparisons.
Let , … , n} be the set of n criteria. The result of the pairwise comparison on n criteria can be summarised in an (n × n) evaluation matrix A in which every element
is obtained from pairwise comparisons of the criteria according to the experts in the field, as shown in Equation (1). The aim of pairwise comparison is to find out the weighting of each criteria based on the decision-maker's experience without guessing games.
(1)
(1) In the second step, the mathematical process commences to normalise and find the relative weights for each criterion. The relative weights are given by the eigenvector w corresponding to the largest eigenvalue λmax, as
(2)
(2)
If the pairwise comparisons are completely consistent, the matrix A has rank 1 and λmax = n. Weights now can be obtained by column-based normalised pairwise comparison matrix by taking the average of each rows in the normalised matrix (Wang and Yang Citation2007). The reliability of the pairwise comparisons according to AHP is strictly related to the consistency of the pairwise comparison judgements. Meanwhile, the validity of outputs is based on the perspective of respondents from the design or production expert team. The consistency is defined by the relation between the entries of A. The consistency index CI is defined as
(3)
(3) The final consistency ratio CR, using which one can conclude whether the evaluations are sufficiently consistent, is calculated as the ratio of the CI and the random index RI, as indicated in the below equation
(4)
(4) where the RI represents the average consistency index, which is also named as the random index, as the average consistency of square matrices of various orders n which was filled with random entries computed by Saaty (Citation1997). Average consistency values of these matrices are given by Saaty and Vargas (Citation1991) as provided in . If the CR
0.1, the weighting and its consequence is accepted; otherwise, a new comparison matrix A is requested until CR
0.1.
Table 4. Values for RI (Saaty and Vargas Citation1991).
AHP combined COPRAS methods in multi-criteria decision analysis
The prioritisation and the utility degree of the design alternatives directly depend on a system of criteria, adequately describing the alternatives, values and weights of the criteria (Motuzienė et al. Citation2016). The method COPRAS are widely used for multi-criteria evaluation. The determination of significance and priority or ranking of design alternatives, by using the COPRAS method, contains the following steps (Zavadskas et al., Citation1994).
Stage 1: Expression of the Decision matrix D with n criterions and m design alternatives and the weights w for the n criteria calculated by AHP method in the previous section in terms of Equations (5) and (6), respectively.
(5)
(5)
(6)
(6) Stage 2: Normalisation of the decision matrix D matrix by Equation (7) and the weighted normalised matrix is calculated by Equation (8), respectively.
(7)
(7)
(8)
(8) Stage 3: Calculation of the sum Bi of the beneficial criteria values by Equation (9) and non-beneficial criteria Ci values by Equation (10), respectively, where k is the number of beneficial criteria that will be maximised, and m is the total number of criteria
(9)
(9)
(10)
(10) Stage 4: Determination of the relative significance Qi of each alternative according to Equation (11) and the utility degree UDi of each alternative by Equation (12)
(11)
(11)
(12)
(12) Stage 5: The priority order of compared alternatives is determined based on their relative significance Qi. The alternative with higher relative importance has higher priority (rank) and is the most acceptable alternative according to the below equation
(13)
(13) illustrates implementing AHP combined COPRAS methods for evaluation of the TCC floors.
Analysis and results
For the ranking of six design alternatives of TCC floors, AHP combined COPRAS methods has been utilised. In this paper, AHP method has been applied for determining the weighs of criteria, based on pairwise comparisons. To set the criteria weights and determine their priority, a short survey with six questions was administered to the 20 technical and production managers at industrialised house-building companies at both Swedish and European market. Only technical and production managers were included in the survey for satisfying the condition CR < 0.1. In the survey it has been requested from the technical and production managers, to compare the criteria pairwise based on their experience/views using the fundamental weighting scale according to . They have compared pairwise the following criteria: maximising open spaces; minimising total costs; minimising CO2 emissions and maximising comforts. The list of the six survey questions involving pairwise comparisons is shown in . Summarised results from the pairwise comparisons are shown in .
Table 5. Survey questions for pairwise comparisons of the criteria in MCDA.
Table 6. Summarised results from pairwise comparisons by the 20 technical and production managers.
The weightings for the four criteria have been obtained as Equation (6) and are summarised in .
Table 7. The weightings of four criteria that have been obtained by normalising evaluation matrix A in Equation (1) by AHP method.
To ensure the consistency of the subjective judgements of the respondents in their answers to the pairwise comparisons (matrix A or Equation (1)) in the survey, the CR has been evaluated by the eigenvalue problem in Equation (2). Eigenvalue problem in Equation (2) has been solved in MATLAB and the eigenvalues λ are the entries in the main diagonal according to Equation (14). As it can be seen in Equation (14), the eigenvalue problem has both positive and negative non-zero real eigenvalues and λmax is equal to 4.2600 as the highest positive eigenvalue. CR has been calculated according to Eq. (4) to CR=0.0963 that satisfies the condition CR < 0.1 according to Saaty (Citation2008)
(14)
(14) Then COPRAS method has been applied for ranking and selecting the concept design for TCC floors by Equations (5) to (13). Alternative designs are 7.3 m as medium and 9.0 m as long-span lengths, involving three different comfort classes A, B and C in descending order. The summary of the response evaluation of the design alternatives is shown in .
Table 8. The summarised results for the response evaluation of the design alternatives.
The priority order of compared alternatives according to the COPRAS method has been determined based on their relative significance as in Equation (11). Thereby, the alternative with the highest relative importance has chosen as the most acceptable alternative according to Equation (13). TCC floor with the span length of 7.3 m belonging to comfort class A has got the highest ranking. Applying sensitivity analysis is highly important to ensure about the reliability of the final decision. Sensitivity analysis illustrates different “What- if” scenarios impact on final decision. However, open spaces and resource-saving criteria are more crucial in the choice of span length for TCC floors. Higher resource saving and open spaces can be achieved by long-span floor system where a few non-apartment-separating load-bearing walls can be reduced/removed. This means that both total costs and CO2 equivalent can be reduced by long-span floor system. Thus, weighting for the open spaces has been increased by 0.15 units in the next coming sensitivity analysis. In this scenario, TCC floor with the span length of 9.0 m belonging to comfort class A gets the highest ranking according to AHP combined COPRAS methods and shall be chosen as the optimal concept design for further development.
Conclusions and recommendations
In this research, a relatively innovative, systematic and practical method is suggested to support the decision-making process for the evaluation of the performance criteria of TCC floor systems in industrialised multi-storey wooden buildings. For this purpose, major goal, performance objectives as well as criteria which compose a performance TCC floor system were identified. The novelty of this paper as the continuation of the previous study is about developing a complete concept design tool for TCC floor system using AHP combined COPRAS methods to handle both beneficial as area and natural frequency and non-beneficial as cost and CO2 emissions criteria.
The AHP combined COPRAS methods accomplish encouraging consequences for the evaluation of performance criteria in planning and design phases of TCC floor systems. This research primarily provides the required procedures for the design team including technical and production managers in accomplishing serviceability, and sustainability performances of TCC floor systems, by utilising an MCDA. In developing a systematic decision-making process, the design team can primarily describe TCC floor systems’ goals, objectives, criteria, indicators and limitations. Before beginning an MCDA procedure, the design team should decide on the significance of each of the criteria since performance priorities will affect the determinations during the overall design procedure. For this purpose, the design team need to compare the performance criteria, pairwise comparisons values and consistency ratios. The developed approach achieved through the presented research could provide as a guideline to the design team and promote to the evaluation of potential TCC floor systems or other structural elements. To examine the extent of the variation in predicted concept design, it is important to perform a sensitivity analysis as it suggested in the developed approach in this study.
The Climate Declaration Act for the new buildings is planned to enter into force in Sweden on 1 January 2022. The purpose is to reduce the climate impact in the construction phase. The new law surely will change the opinion of the technical and production managers to the advantage of sustainable design to change the outcome of this paper. This fact was relied after analysing the survey results obtained from one of the technical managers of a participated company in the survey.
Though this research suggested a variety of prospects for a complete concept design tool, it has limitations as well. Firstly, the performance objectives and associated criteria identified for evaluation were constrained. Secondly, objectives and criteria were limited to multi-storey wooden buildings. Thirdly, this research focused on the performance criteria of medium to long-span TCC floor systems challenged during the planning and early design phases. For future studies, it could be investigated to what extent the determined multifunctional objectives and performance criteria have a major influence on total building performance and the comfort level of inhabitants. Further analysis that could be included in the future studies shall involve size and topology optimisation of the concept design to reduce both material, production costs and climate impact as an important process in the product development.
Disclosure statement
No potential conflict of interest was reported by the author(s).
References
- Balasbaneh, A. T., and A. K. Bin Marsono. 2018. “New Residential Construction Building and Composite Post and Beam Structure Toward Global Warming Mitigation.” Environmental Progress & Sustainable Energy 37 (4): 1394–1402.
- Balasbaneh, A. T., A. K. B. Marsono, and S. J. Khaleghi. 2018. “Sustainability Choice of Different Hybrid Timber Structure for Low Medium Cost Single-story Residential Building: Environmental, Economic and Social Assessment.” Journal of Building Engineering 20: 235–247.
- Balcomb, J. D., and A. Curtner. 2000. “Multi-criteria Decision-making Process for Buildings.” Collection of Technical Papers. 35th Intersociety Energy Conversion Engineering Conference and Exhibit (IECEC) (Cat. No. 00CH37022). IEEE.
- Baril, M. A., L. Sorelli, J. Réthoré, F. Baby, F. Toutlemonde, and L. Ferrara. 2016. “Effect of Casting Flow Defects on the Crack Propagation in UHPFRC Thin Slabs by Means of Stereovision Digital Image Correlation.” Construction and Building Materials 129: 182–92.
- Brauers, W. K. M., E. K. Zavadskas, F. Peldschus, and Z. Turskis. 2008. “Multi-objective Decisionmaking for Road Design.” Transport 23 (3): 183–193.
- Cecconi, F. R., L. C. Tagliabue, S. Maltese, and M. Zuccaro. 2017. “A Multi-criteria Framework for Decision Process in Retrofit Optioneering Through Interactive Data Flow.” Procedia Engineering 180: 859–869.
- CEN1995-1-1. 2004. Eurocode 5 - Design of timber structures - Common rules and rules for buildings. European Committee for Standardization.
- Daňková, J., P. Mec, and J. Šafrata. 2019. “Experimental Investigation and Performance of Timber-Concrete Composite Floor Structure with Non-metallic Connection System.” Engineering Structures 193: 207–218.
- Dias, A., J. Skinner, K. Crews, and T. Tannert. 2016. “Timber-Concrete-Composites Increasing the Use of Timber in Construction.” European Journal of Wood and Wood Products 74 (3): 443–451.
- Ferrier, E., P. Labossiere, and K. W. Neale. 2009. “Mechanical Behavior of an Innovative Hybrid Beam Made of Glulam and Ultrahigh-performance Concrete Reinforced with FRP or Steel.” Journal of Composites for Construction 14 (2): 217–223.
- Graybeal, B. 2008. “Flexural Behaviour of an Ultrahigh-Performance Concrete I-Girder.” J. Bridge Eng 13 (6): 602–610.
- Hopfe, C. J., M. T. Emmerich, R. Marijt, and J. Hensen. 2012. “Robust Multi-criteria Design Optimization in Building Design.” Proceedings of Building Simulation and Optimization, Loughborough, UK. 118–125.
- Japan Research Institute. 2003. Environmental Performance Indicators Guideline for Organizations. Tokyo, Japan.
- Jelusic, P., and S. Kravanja. 2017. “Optimal Design of Timber-Concrete Composite Floors Based on the Multi-parametric MINLP Optimization.” Composite Structures 179: 285–293.
- Kaklauskas, A., E. K. Zavadskas, J. Naimavicienė, M. Krutinis, V. Plakys, and D. Venskus. 2010. “Model for a Complex Analysis of Intelligent Built Environment.” Automation in Construction 19 (3): 326–340.
- Khorsandnia, N., H. Valipour, J. Schänzlin, and K. Crews. 2016. “Experimental Investigations of Deconstructable Timber-Concrete Composite Beams.” Journal of Structural Engineering 142 (12): 04016130.
- Martins, C., A. M. Dias, R. Costa, and P. Santos. 2016. “Environmentally Friendly High-performance Timber–Concrete Panel.” Construction and Building Materials 102: 1060–1069.
- Medineckienė, M., Z. Turskis, and E. K. Zavadskas. 2011. “Life-cycle Analysis of a Sustainable Building, Applying Multi-criteria Decision Making Method.” In The 8th International Conference “Environmental Engineering”: Selected Papers, edited by D. Čygas and K. D. Froehner, pp. 957–961.
- Motuzienė, V., A. Rogoža, V. Lapinskienė, and T. Vilutienė. 2016. “Construction Solutions for Energy Efficient Single-family House Based on Its Life Cycle Multi-criteria Analysis: A Case Study.” Journal of Cleaner Production 112: 532–541.
- Movaffaghi, H., J. Pyykkö, and I. Yitmen. 2020. “Value-driven Design Approach for Optimal Long-span Timber-concrete Composite Floor in Multi-storey Wooden Residential Buildings.” Civil Engineering and Environmental Systems 37 (3): 100–116.
- Moya, L., L. Domenech, A. Cardoso, and V. Baño. 2017. “Proposal of Visual Strength Grading Rules for Uruguayan Pine Timber.” Eur. J. Wood Wood Prod 75: 1017–1019.
- Naud, N., L. Sorelli, A. Salenikovich, and S. Cuerrier-Auclair. 2019. “Fostering GLULAM-UHPFRC Composite Structures for Multi-storey Buildings.” Engineering Structures 188: 406–417.
- Neve, O., and L. Spencer-Allen. 2015. “Shaking Up Dance Floor Design with Timber-Concrete Composites.” Proceedings of the Institution of Civil Engineers-Construction Materials 168 (4): 204–212.
- Ogrodnik, K. 2019. “Multi-criteria Analysis of Design Solutions in Architecture and Engineering: Review of Applications and a Case Study.” Buildings 9 (12): 244.
- Popovic, G., D. Stanujkic, and S. Stojanovic. 2012. “Investment Project Selection by Applying COPRAS Method and Imprecise Data.” Serbian Journal of Management 7 (2): 257–269.
- Russell-Smith, S. V., M. D. Lepech, R. Fruchter, and Y. B. Meyer. 2015. “Sustainable Target Value Design: Integrating Life Cycle Assessment and Target Value Design to Improve Building Energy and Environmental Performance.” Journal of Cleaner Production 88: 43–51.
- Saaty, T. L. 1980. The Analytic Hierarchy Process: Planning, Priority Setting, Resources Allocation. London: McGraw-Hill.
- Saaty, T. L. 1990. “How to Make a Decision: The Analytic Hierarchy Process.” European Journal of Operation Research 48 (1): 9–26.
- Saaty, T. L. 1997. “That Is Not the Analytic Hierarchy Process: What the AHP Is and What It Is Not.” Journal of Multicriteria Decision Analysis 6: 324–335.
- Saaty, T. L. 2008. “Decision Making with the Analytic Hierarchy Process.” International Journal of Services Sciences 1 (1): 83–98.
- Saaty, T. L., and L. G. Vargas. 1991. “Prediction, Projection and Forecasting.” Dordrecht: Kluwer Academic Publishers.
- Si, J., L. Marjanovic-Halburd, F. Nasiri, and S. Bell. 2016. “Assessment of Building-integrated Green Technologies: A Review and Case Study on Applications of Multi-criteria Decision Making (MCDM) Method.” Sustainable Cities and Society 27: 106–115.
- Sorelli, L., N. Naud, S. Lamonthe, C. Joly-Lapalice, and P. Blanchet. 2019. “Fostering Composite Structures of UHPC and Timber.” In International Interactive Symposium on Ultra-High Performance Concrete (Vol. 2, No. 1). Iowa State University Digital Press.
- Stević, Ž. 2016. “Supplier Selection Using AHP and COPRAS Method. Strategic Management and Decision Support Systems in Strategic Management.” Subotica, 21th International Scientific Conference SM2016, 231–238.
- Stocchero, A., J. K. Seadon, R. Falshaw, and M. Edwards. 2017. “Urban Equilibrium for Sustainable Cities and the Contribution of Timber Buildings to Balance Urban Carbon Emissions: A New Zealand Case Study.” Journal of Cleaner Production 143: 1001–1010.
- Suárez-Riestra, F., J. Estévez-Cimadevila, E. Martín-Gutiérrez, and D. Otero-Chans. 2019. “Experimental, Analytical and Numerical Vibration Analysis of Long-Span Timber-Timber Composite Floors in Self-Tensioning and Non-tensioning Configurations.” Construction and Building Materials 218: 341–350.
- Tannert, T., B. Endacott, M. Brunner, and T. Vallée. 2017. “Long-term Performance of Adhesively Bonded Timber-Concrete Composites.” International Journal of Adhesion and Adhesives 72: 51–61.
- Toratti, T., and A. Talja. 2006. “Classification of Human Induced Floor Vibrations.” Building Acoustics 13 (3): 211–221.
- Tupenaite, L., E. K. Zavadskas, A. Kaklauskas, Z. Turskis, and M. Seniut. 2010. “Multiple Criteria Assessment of Alternatives for Built and Human Environment Renovation.” Journal of Civil Engineering and Management 16 (2): 257–266.
- Wang, J. J., and D. L. Yang. 2007. “Using a Hybrid Multi-Criteria Decision Aid Method for Information Systems Outsourcing.” Computers and Operation Research 34 (12): 3691–3700.
- Woodard, A. C., and H. R. Milner. 2016. “Sustainability of Timber and Wood in Construction.” In Sustainability of Construction Materials, edited by Khatib, 129–157. Melbourne, Australia: Woodhead Publishing.
- Xie, Z., X. Hu, H. Du, and X. Zhang. 2020. “Vibration Behavior of Timber-Concrete Composite Floors Under Human-Induced Excitation.” Journal of Building Engineering 32: 101744.
- Yeoh, D., M. Fragiacomo, A. Buchanan, K. Crews, J. Haskell, and B. L. Deam. 2008. “Development of Semi-prefabricated Timber-Concrete Composite Floors in Australasia.” 10th World Conference on Timber Engineering WCTE 2008, Miyazaki, Japan, CD.
- Zavadskas, E. K., R. Liias, and Z. Turskis. 2008. “Multi-attribute Decision-Making Methods for Assessment of Quality in Bridges and Road Construction: State-of-the-Art Surveys.” The Baltic Journal of Road & Bridge Engineering 3 (3): 152–162.
- Zavadskas, E., F. Peldschus, and A. Kaklauskas. 1994. Multiple Criteria Evaluation of Projects in Construction" Institute of Technological and Economic Development (ITED), Vilnius Technical University. V. Technika 226.
- Zavadskas, E. K., Z. Turskis, and S. Kildienė. 2014. “State of Art Surveys of Overviews on MCDM/MADM methods.” Technological and Economic Development of Economy 20 (1): 165–179.
- Zavadskas, E. K., Z. Turskis, and J. Tamošaitiene. 2010. “Risk Assessment of Construction Projects.” Journal of Civil Engineering and Management 16 (1): 33–46.