ABSTRACT
Water security covers a wide range of issues and risks to people, the natural and built environment, the economy, and interactions between these. This breadth creates an interconnected complexity and the potential for perceived intractability. Tackling water security meaningfully therefore requires a judgement in balancing the holistic nature of water security with reductionist understanding of key processes and elements. This paper demonstrates that systems thinking can be adapted to achieve this through different systems framings of water security, tailored to local context. Through four case studies, we show how systems thinking has been adapted and applied to fit the contextual analysis and management of multiple water security issues. We show how this approach has dissolved disciplinary and sectoral silos; changed the spatial scale at which water security is addressed; improved data acquisition and analysis to better understand relationships between sub-systems; and integrated socio-ecological issues of justice and power with more traditional bio-physical understandings of water security. This is an important step towards turning systems thinking into systems transformation and making the concept of water security actionable and accessible to policy, planning, and practice.
1. Introduction
The water security paradigm has received increased attention over the past two decades in policy, development and academic debates (Cook and Bakker Citation2012; Lautze and Manthrithilake Citation2012). This is largely due to it spanning several interests and research areas, such as water resources, environmental studies, engineering, public health, and social science, amongst others (Cook and Bakker Citation2012). Additionally, the consideration of water security under the influence of future socio-economic and environmental scenarios has made it popular for sustainable development studies and assessment of future risk and uncertainty. Lastly, it underpins and is integral to addressing a wide range of Sustainable Development Goals (SDGs). This is perhaps a given considering that the SDGs mirror the challenges of sustainable development and were therefore designed to reflect the interconnected and indivisible nature of current global issues. Hence, systems thinking (ST) is particularly relevant for tackling SDGs having themselves been created in a systematic way (Eustachio et al. Citation2019). In many cases, the links and trade-offs between interconnected SDG goals at three levels (policy, resources, and sub-systems) are related to water both inside and outside of SDG6 ‘clean water and sanitation for all’ – nearly a quarter of these trade-offs impact water, therefore ST for water security offers the potential to avoid conflicts between sustainability objectives (Pham-Truffert et al. Citation2020; Le Blanc Citation2015).
There is general disagreement on the theoretical and conceptual foundations that explain water security (Chiluwe and Claassen Citation2020) and because of this, multiple definitions exist inclusive of various criteria, subject, and scope (e.g., Allan, Catherine, and Pahl-Wostl Citation2013; Bakker and Morinville Citation2013; Everard Citation2014; GWP Citation2000; UNESCO Citation2017; UN Water Citation2013). The compounding effect of this diversity of understanding is that the water security concept has increased in intricacy over time and now embodies a complex, multidimensional, and interdependent range of issues (Mishra et al. Citation2021). With such a breadth of issues, there is no single framing or approach that can do justice to all of them. Some common water security themes do exist such as: provision of potable water, water for productive activities, and security and independence (Lautze and Manthrithilake Citation2012). But ultimately, the breadth of water security makes it difficult to recognise what it means to achieve water security on-the-ground, particularly as the interconnections with other disciplines often appear endless. Consequentially, the big unanswered question is still ‘water security, how?’ (Lankford et al. Citation2013).
As an approach able to handle this increasing intricacy, we argue that ST is crucial and necessary to tackle water security. As a family of tools whose most important features are recognising and dealing with complexity and uncertainty (Dias and Jowitt Citation2020), ST enables multiple facets of water security to be addressed by understanding the relationships between the social, economic, physical, and natural structures and behaviours (Meadows Citation2008) of systems. The application of ST provides researchers with a means to organise, understand, and address both the tangible and intangible aspects of water security where there are competing water ontologies and epistemologies. The analytical skills and methods associated with ST approaches exist on a spectrum where the attribution of ‘systemicity’ varies (Checkland Citation2008); hard ST being where problems can be described as a set of interacting systems that can be engineered, soft ST being a process of inquiry used to organise complexity as a learning and critical system – promoting a conscious reflection on the contextual assumptions behind problem definition and solutions (Ulrich Citation2013). It is important to recognise that this creates a disparity in the philosophical traditions underpinning ST approaches such as positivistic versus phenomenological or critical framings (Checkland and Holwell Citation2004; Ulrich Citation2012); frames are a predisposition to distinguish some aspects of a situation rather than others and to classify and value these in an accustomed way (Vickers Citation1995). Therefore, these framings provide different entry points to understanding systems; hence, some ST approaches are favoured (and/or created) by some disciplines over others. The richness of systemic approaches fit for different problem-contexts has been acknowledged in the ST world for a long time (Boulding Citation1956; Jackson Citation1990; Citation2009).
ST itself is not novel as a concept applied to water security, but it is predominantly applied to sub-systems, or a set of sub-systems without broader water security system framings. Reductionist approaches can support and provide important insights but might not capture the overall emergent behaviour of the water security system. For example, Chen and Wei (Citation2013) state that ‘a water security system includes flood control and disaster mitigation, water resource security, water environment security’ as well as sub-systems such as society, economy, water resources, ecology, and environment. Whereas Kees et al. (Citation2018), more extensively, include a four-tier hierarchy of water security; pressures (environmental and socio-economic), states (water quantity, water supply infrastructure, flood protection infrastructure, sanitation infrastructure, water quality), impacts (water supply dependencies, water supply, flood protection, sanitation, ecology, aesthetic), and responses (institutional frameworks, planning, operational management).
If we were to, and as is often done, zoom in and select an individual sub-system (or multiple sub-systems), we could still apply ST, but in a reductionist sense to analyse a particular water security issue. This is arguably reflected in many current systems approaches to water security, where although the approach is systemic, the issues and scope tend to remain in individual problem silos of reduced water security elements. Whilst it is noted that both the reductionist approach and systems approach are complementary (Meadows Citation2008) and required in order to form a whole picture of water issues, the goal is to capture enough of the overall emergent behaviour of a water security system so that it is reflective of the real experience of water users and therefore can promote targeted systems transformation. Furthermore, framing the water security system in cross-cutting interdisciplinary themes rather than reductionist disciplinary silos.
Ulrich (Citation2012) observed that ‘holistic or whole-systems thinking – the quest for comprehensiveness – is a meaningful effort but not a meaningful claim’. In practical terms, one cannot expect to capture the whole complexity of a system, but one can strive to approach it with awareness of practical selectivity and partiality. By encouraging the water security systems thinker to welcome complexity, we attempt to aid the understanding of overlapping and percolating issues between disciplinary systems perspectives; the perspective of ST therefore allowing an interdisciplinary slice to be taken from the contextual water security issues in a given area. This paper demonstrates, through four international case studies, how ST can be adapted and applied to tackle a range of water security challenges in practice. The ST approaches for each case study exist within specific water security system framings of integration, behaviour, scale, and context (see ) all of which are elements of our three core concepts detailed in Section 2. The approach is focused on a narrative of problem identification and on-the-ground implementation, providing an overview of potential methodological components that can implement some systems framings for water security. The final discussion reflects on how ST can help structure problem articulation and understanding within the water security field, and subsequently recommendations on how to translate this into systems transformation – following the proposition that promoting holism is a means of facilitating better integrated and adaptive water resource decisions (Schenk et al. Citation2009; Mirchi et al. Citation2012; Hipel et al. Citation2013).
2. Core concepts
In keeping with the principles of ST (Senge Citation1990), our approach has been founded on a process of discovery and diagnosis to understand not just parts, but wholes and inter-relationships; an explorative approach enables us to develop problems related to water, aligning with the argument that the best solution is to define a problem, not to solve it (Fraser Citation2010). This is reflected through three core water security concepts that have been applied to underpin and enable a systems approach in all four case studies.
I: Water data is a foundation for an integrated understanding of the behaviour of a water security system. Effective action requires data and knowledge from across the whole water security system, including water use, quantity and quality. However, it is also essential to understand qualitative factors such as behaviour, attitudes, and stakeholder relationships to provide contextual setting in water value(s). This is demonstrated in Butte et al. (Citation2022) where the data gathering strategy for water security operates through multiple interfaces of data, the physical environment, project resources, stakeholders, and socio-economic context. Whilst integrating data from all aspects of the water security system is an important precursor to understanding, it is insufficient on its own. Observations must be turned into insights, for example, vulnerabilities, risks, costs of action or inaction, options, and policies. Moreover, these understandings and insights are further promoted by integrative methods that foster interdisciplinarity within data gathering strategies and outcome communication. Tools and systems are also needed to easily share, analyse, and convert this into information that communities and stakeholders can use to inform decisions about future needs, and have confidence in outcomes (Bartram et al. Citation2018).
II: Water security issues play out across a range of scales and sub-systems. Water security drivers, pressures, governance, and physical processes interact across multiple scales and sectors. Industrialisation, population growth, and urbanisation are increasing chemical and microbial water pollution which threatens public health and the environment. Hydrological extremes can lead to famine, death, spread disease, and exacerbate inequalities. These issues must all be tackled in a coherent framework as they are closely related, e.g. water pollution must be tackled at source and treated to protect public health and the environment and thereby increase water availability. A systems approach to water security therefore requires mapping and understanding these interactions at a range of spatial and temporal scales; within communities in rural and urban settings, within each catchment and between catchments. A range of tools can be used to identify these interactions, with system dynamics able to identify and map causal relationships (De Stercke et al. Citation2020), packet tracking can be used to better understand physical hydrological relationships (O’Donnell Citation2011; Pantha, Acharya, and Mohapatra Citation2021), whilst Problem-Driven Iterative Adaptation can be used to search for politically supportable and practically implementable reforms by engaging broad sets of actors (Andrews, Pritchett, and Woolcock Citation2013).
III: Developing water security pathways should be appropriate to local context and value(s). Consideration of only economic supply-demand does not capture the full value(s) of water; this is a major contributor to limited investment and choices that are detrimental to social welfare and human rights, and failure in water security (Castalia Citation2014). Individuals, communities, organisations, and other stakeholders understand, use, manage, and value water in multiple and complex ways (Haileslassie et al. Citation2020; Garrick et al. Citation2017). Restricted capabilities, blurred lines of responsibilities, conflict of interests, and unbalanced power amongst institutions, governance levels and legal frameworks, can make implementation of systems approaches to water security very difficult in practice. The political realities of each situation allow only limited pathways towards improved water security, which is itself also a contested concept. Better understanding of water insecurities in urban and rural contexts requires an acknowledgement of the different values, and a nuanced approach to defining the origins and mechanisms of security, in each context (Kjellén, Sinha, and Hebart-Coleman Citation2021).
Uptake of policy, practice and technology are improved when they align with local context and the values of multiple stakeholders (Vogl et al. Citation2017) and yet many stakeholders, especially communities, are unable to engage with, and take responsibility for, water security. We have developed the concept of a Water Collaboratory to address the challenge of applying systems approaches to tackle water security. The notion of Collaboratories follows the participatory reorientation of the notion of good practice in ST (Ulrich Citation2000). This provides a co-creative stakeholder engagement process for solving complex problems, that is inclusive and open to all stakeholders to meet on an equal basis, to jointly question, discuss, and construct new ideas to resolve water security issues. The Collaboratories featured in the case studies are experiencing different development transitions, which are emblematic of global challenges in water security, yet their approaches have been tailored to the local development context, ambition, culture, socio-political context, and setting.
3. Systems thinking for systems transformation
Moving from ST to systems transformation, and hence executing system change, in water security can only happen through direct engagement with the messiness of real situations. ST must aim to integrate more than information alone on the existence of water security problems; there is a necessary inclusion of knowledge of resources, incentives, and consequences in order to trigger (systems) action (Meadows Citation2008). Here, we present examples of how the application of ST concepts are helping to transform the way in which research teams, working within the framework of Water Collaboratories, are addressing locally defined water security challenges across the world. Each show an example of how these ideas have changed the team’s approach enabling them to develop new insights and pathways to systems transformation with their Collaboratories.
3.1. India: National Capital Territory Delhi and the Upper Yamuna River Basin
The various nested sub-systems at play within the National Capital Territory (NCT) of Delhi create a need for systemic data gathering and aggregation, being foundational for integrated understanding of the behaviour of water security. The ST transformation is unique in NCT Delhi, where water security management has to develop within a nested system of governance, water basins, and other securities. These nested system structures are characterised by hierarchy, connectedness, and aggregation driven by the rising cycle of supply and demand; the main issue concerning water security for Delhi being the widening urban water gap due to higher demand and lower supply. Key issues within the component of demand are increasing urban population and discoordination between infrastructure planning and water provision. Within the component of supply, problems of water availability due to quality, infrastructure provision and access to fresh water are all entangled within upstream-downstream relationships. The city of Delhi itself has multiple municipalities and a centralised water governance system. Within this city, governance coordination is lacking and access to water is as an extremely political issue. Inequality also exists in the right to water between poorer and wealthier areas due to lack of investment and infrastructure (Kumar et al. Citation2021).
These are all largely physical attributes and ST is used practically for transformation from supply augmentation to demand and resource management by providing a better representation of the water security system through data. Hence, aiming to improve the integrations between organisations and information through understanding interconnectedness and embeddedness. This is key as the complex water management system is a root cause for many water security problems this area faces. The representation of the systems needed for data structure and aggregation is the focus of the ST transformation, with relationships and interconnections explored in line with the natural flow direction of the Yamuna river (see ).
Figure 2. Upper Yamuna River Basin (UYRB) Water Security Data System from National Capital Territory (NCT) Delhi to Hathnikund Barrage.
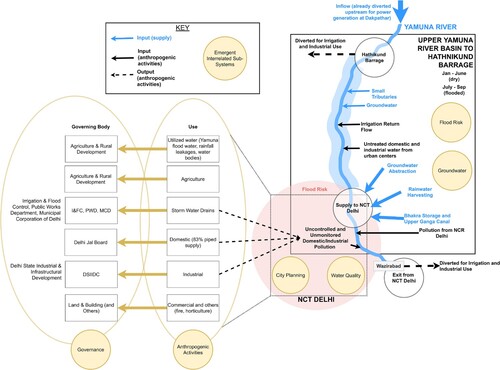
The main systemic linkages between the Upper Yamuna River Basin (UYRB) and NCT Delhi, are contained within six dominant interrelated sub-systems that describe the emergent behaviour of water security processes and interactions in this area (as shown in ): groundwater, flood risk, water quality, city planning, anthropogenic activities, and governance. The diversion of water at various places along the length of Yamuna for power generation, irrigation, and drinking water purposes plays a key role in water availability, water quality, and is driven by anthropological activity. Crucially, no water is allowed to flow downstream of the diversion barrages and the river remains dry in many stretches, relying on contributions from groundwater accrual, confluence of few small tributaries, irrigation return flow, and untreated or partially treated domestic and industrial wastewater from various urban centres.
NCT Delhi is a key actor in the systems interactions in the UYRB. The flow of the Yamuna within NCT Delhi is influenced by discharge from the Hathnikund Barrage; when excess water is released from Hathnikund, a backflow is created effecting the major city drains and causing urban flooding and water quality issues. Water quality of the Yamuna is significantly impacted by anthropogenic activities within NCT Delhi. This is a product of multiple factors: the gap between sewage generation and treatment; uncontrolled and unmonitored discharges of wastewater predominantly from domestic sources into the river (where 18 out of 22 major drains join); and riverbed encroachments, especially during dry season. The National Capital Region (NCR) that surrounds Delhi also majorly contributes to the pollution load in the River Yamuna (CPCB Citation2013).
Integrated solutions to these linked issues in the UYRB will not be fulfilled unless there is reliable data to represent the system. The systems transformation achieved here has been the revealing of the value and place of data and information for understanding the intersection of sub-systems within water security, working together with key organisations. Demonstrating that water data is foundational for an integrated understanding of the behaviour of water security. The presence of these dominant sub-systems also exposes how systems transformation could be achieved through targeted action and data collection of identified leverage points. For example, groundwater; a significant contributor to both NCT Delhi and the Yamuna River. It is essential for river recharge and a key water supply for NCT Delhi, with deficit in drinking water supply for Delhi being partially augmented through groundwater resources. Other leverage points include stakeholder consultation and involvement in demand reduction, and infrastructure for water distribution (e.g. aged supply lines that require both coverage and leakage renewal) and water treatment (e.g. the construction of decentralized wastewater treatment for an extended sewer network).
3.2. Malaysia: the Johor River Basin
The systems transformation in the Johor River Basin (JRB) demonstrates the play of issues that intersect, connect, and evolve over a range of scales and sub-systems. The starting approach to water security in this basin has previously been largely reduced to solvable elements with set boundaries and individual targeted solutions, i.e. viewing water security solutions as products of non-dynamic binary quality and quantity issues confined to disconnected and distinct governance scales such as federal (Malaysia), state (Johor), and basin (JRB). Water security was initially addressed in silos for particular issues and drivers (see ). This streamlined the development of technical solutions into disconnected paths of water quality verses water quantity, not providing, or allowing room for, integrated and interdisciplinary water security perspectives.
As a basin faced with multiple internal drivers, such as increasing population, industry, and agriculture, in combination with exogenous environmental drivers of local climate variability and global climate change, all set within a political relationship of water transfer (to Singapore) – the JRB has multiple development futures at the hands of its various stakeholders, which were previously unrepresented as influential elements of the water security system. It has been observed through ST transformation that the overlapping boundaries of issues, drivers, and stakeholders introduce varying systems scales that are naturally integrated with one another, prompting the need for research and solutions that are equally integrated.
In this sense, the ST lens has proved an important integrative tool for the water security work and research in the JRB. Allowing space to explore, understand, and integrate water issue boundaries; water quantity, water quality, the underlying governance and values perceived. Discovering that there are other elements and interconnections that significantly contribute towards water security within the basin than quantity and quality alone. This is especially the case for the integration of actors and influence into the water security network of the JRB. As illustrated by reconfiguration of water security outputs created by systems transformation in , the causal interactions among the issues, drivers, stakeholders, and elements of water security in JRB were systemically mapped out in a participatory process – unveiling the complexity and overlapping boundaries of the water security issues. The result of this framing has altered the focus and approach to identifying water security solutions for the JRB; highlighting areas of crossdisciplinarity that can be integrated to produce better research outcomes and establish water governance solutions that enable integrated river basin management in the JRB.
The means in which water security issues play out across a range of scales and sub-systems has been realised through the systems transformation process within the JRB. The ST lens has made room for a union of water quality and quantity in research and practice, as well as a new outlook for the framing of what more integrated water governance solutions may look like. This has only been achievable through the emergence of connections between varying scales and sub-systems; crucially demonstrating the role and placement of stakeholders within this system as both key linkages and key leverage creators. Alongside this, ST has enabled an improved view of how drivers of fragmented governance, community and behaviour aggravate and contribute towards water security issues in the JRB. ST therefore offers a better review of horizontal interactions across ministries (at federal level) and divisions (at state level), as well as the hierarchical interactions among federal, state and basin level.
3.3. The Central Ethiopian Complex: Upper Awash, Abbay, Central Rift Valley, and Addis Ababa
The water security issues in the Central Ethiopian Complex (CEC) are spread over several territories connected through water issues such as scarcity, quality, land use, economy, and risk. All of these exist inside a complex governance structure, with differing and competing political ideologies. In order to form a management scale that is practical for implementing and understanding solutions, the interconnectedness of these problems is required to be shown in an understandable way for stakeholders and decision-makers; observing the web of relationships and actors over the areas (and hence applying a systems framing to these) of the Abbay, Central Rift Valley, and Upper Awash basins, and the capital city of Addis Ababa are crucial in harnessing the water security discourse of this area. In summary, this has proved essential in developing water security discourse that represents local context and values, and therefore the pathways that are appropriate.
The problemscapes concept (see Polaine et al. Citation2022) has been adopted for integrating complexity with the decision-making arena in the CEC as well as identifying drivers and impacts in achievable leverage areas. It is a semi-systems framework for understanding issue networks of water security problem interactions that moves away from the relationships solely confined to the basin, a fixed physical unit, and towards a conceptual transient problem area. The investigation of the problematical situation is arguably the first step in any systems approach and is necessary to identify systemic interventions (Checkland and Poulter Citation2006). This systems perspective builds on conceptual problemshed ideas presented by Tony Allan (Citation1998) and reprised by Peter Mollinga (Citation2007). Essentially that, in order to capture the multi-dimensionality of water problems, we must move away from the narrowly water-centred framing approach that is the basin (Mollinga Citation2020). This is done by treating the analysis boundaries of water problems as open, empirical questions over pre-defined spatial boundaries (Mollinga, Meinzen-Dick, and Merrey Citation2007). Constructing the problemscape has both conceptual and material outputs, with the key difference between problemsheds and problemscapes being that outputs and methodology are based on a hybrid of causal loop diagram (CLD) mapping and spatial representation (see ).
At a first level, and similarly to the situation in the JRB, we have found that this approach has integrated the disciplinary work of those concerned with problems in hydrology, water quality, sociology, political economy, and governance. Once again showing that the ST lens can be a vehicle for fostering interdisciplinary research, and therefore providing a horizontally unified approach to problem solving. As part of the explorative methodology, and hence exposure of connections between issues, the process of group problem articulation and explanation of these problems as emergent behaviours of various elements and drivers through participatory CLD across disciplines (Zhang et al. Citation2021; Schmitt Olabisi et al. Citation2018) allows for these varied disciplines to see linkages between research and systemic transformation.
However, this needs to be furthered in order to account for more than this broad representation of feedback structure given by CLD alone (Lane Citation2008). The development of the problemscape points to a systems approach that is purposefully suited to broad problem areas and can be of more use in the areas of action for decision makers (the water problem reality). This approach is currently being developed by research partners and stakeholders working in the Central Ethiopia area. The problemscape, being unconstrained by hydrological boundaries, not only exposes leverage points but – importantly – leverage points that are non-water insights to water problems. This approach has driven systemic transformation reflective of enabling water security pathways to be developed that are appropriate to local context and values.
3.4. Colombia: socio-ecological justice systems
The case study in Colombia takes a different approach to the previous examples, having been working within a socio-ecological systems (SES) framework for some time, the utilisation of ST here has largely been towards developing systems transformation within SES to include justice and power dimensions of water security for decision making and governance within a critical ST perspective. The SES approach is central to the lens of water governance analysis and practice in Colombia as a means of considering the inseparability of social and bio-physical dimensions in the production of specific hydro-social configurations (Heynen, Kaika, and Swyngedouw Citation2005). Crucially, realising that the paradigms that frame the knowledge and information of the two respective social and ecological systems create borders between these that are artificial and arbitrary (Hernández et al. Citation2019).
SES was proposed by Ostrom (Citation2007, Citation2009) to improve the analysis of complex relationships between human and natural resources for management, policy, and strategy. Specifically, as a tool of analysis that examines nested attributes of (and feedback between) resources systems and their outcomes and how these affect incentives of users in governance systems over time (Ostrom Citation2007). The SES perspective is essential for water security problem framing in the Upper Cauca River Basin (UCRB), Colombia; a diverse range of actors, extractive practices, and environmental protection institutions require a systems framework that can integrate the impacts and influences amongst these. Building and evolving the SES approach to encompass ideas of power, justice (inequity and inequality), and intersectionality, has led to the development of the socio-ecological justice (SEJ) framework (see ). The latter attempts to make visible the justice dimension taking place in different society-nature relationship models pointed out by Muradian and Gómez-Baggethun (Citation2021). Thus, the SEJ framework centres the analysis on the diverse set of possible relations (i.e. extractive, utilitarian, caring, spiritual, etc.) that human beings and social groups have historically established with nature.
In such a web of relationships, asymmetrical power relations amongst social actors have negative impacts not only on people’s lives and well-being but also on ecosystems function, integrity, and ecosystem services provision alike. Therefore, those negative impacts create conflicts related to access to natural goods such as water, but also struggles for conflicting value systems that develop into vindications for justice to under-represented social groups or communities, and more recently to degraded natural entities. This framework is a process for understanding more holistically the interactions between bio-physical and social systems, and the power relationships embedded in such interactions of the UCRB. It also allows us to have a critical understanding of the limitations of a ‘model’ Collaboratory when set in contexts of conflict and power asymmetries. Real-world conflicts are far away from the Habermasian Ideal Speech Situation (Habermas Citation1991).
One may argue that a SEJ framework is a lens that allows for the systemic observation and analysis of complex entities such as SES where the bio-physical and social sub-systems continuously interact. Nonetheless, our current economic capitalist sub-system is the main driver of socio-ecological degradation leaving behind impoverished social groups and degraded or destroyed ecosystems where inequity and inequality scenarios are common ground around the global south (Scheidel Citation2018). In this sense, a specific territory such as the UCRB, becomes an open book where the SEJ framework can read and analyse the dynamics and effects of conflicting water valuation systems, water grabbing by those most powerful, water pollution as a consequence of social metabolism, and health risks and hazards for those humans and non-humans equally vulnerable.
SEJ may be first identified and then put into practice by analysing socio-ecological data from the territory through the SEJ lenses. A very practical proposal is to plot/represent both social and ecological or bio-physical data sets in multi-layered GIS. For instance, by having mining sites, along with rural water supply systems, natural land cover variation, water permits concentration, and conflict-prone hotspots in the territory, a web of key relations (implying power asymmetries, instrumental goals or grabbing agents) may arise. In this sense, the metaphor of the territory as an open book read and analysed by an appropriate conceptual framework such as SEJ framework allows us to better grasp the complexity and dynamics of these water security territories (Lopez, Boelens, and Vos Citation2019).
The use of this framework begins the process of establishing a new core concept for ST within water security; specific society-nature models and relationships in a given territory shape the interaction and relation networks amongst stakeholders and with ecosystems. Thus, hydro-social systems dynamics, conflicts over water rights and access, the consequent losses felt by social groups and perceived losses on the natural base of aquatic ecosystems are all mobilising forces for action that may trigger claims for SEJ and its concomitant struggles to reshape or recover a fairer scenario. Therefore, in a new concept of recognition of water security becoming a highly contested feature in such complex and dynamic systems, pertinent questions such as ‘Water security for whom, including natural entities?’, and ‘How can it be achieved and sustained?’ will most likely be at the heart of SEJ vindications and conflicts management.
4. Discussion and recommendations
Ultimately, the implementation and success of using ST for water security problem framing stems from its ability to integrate multiple influence systems together and produce improved and more sustainable solutions. As observed by Jowitt (Citation2020), by striving for sustainability in engineering solutions we naturally adopt a systems approach. To discuss the level of application and the value of the ST lens to water security in these contexts, we refer back to how this has helped to identify and acknowledge the importance of these other sub-systems through the three core concepts: water data is a foundation for an integrated understanding of the behaviour of water security; water security issues play out across a range of scales and sub-systems; developing water security pathways should be appropriate to varied local context and values.
We observe that in our work in Delhi, ST has helped to understand the value of data and information in the context of intersecting sub-systems; demonstrating the role of data as a system, a feeder to other systems, and also a shaping of our knowledge of the system. In Johor, ST has provided a vehicle for the merging of water quality and quantity outputs for integrated working and solutions, allowing water security in the JRB to be understood as a product of interlocking, intersecting, and overlapping scales and sub-systems. This framing has also enabled an appropriate scale to be created, outside of the hydrological unit, for tackling complex water security issues. Somewhat similarly, in the CEC, taking a wide systems view beyond the catchment and water engineering as a discipline has exposed casual linkages that allow innovative responses to water security to be considered which would not be otherwise be on the table. In the UCRB, we have shown how incorporation of issues such as justice and power alongside socio-ecological issues are not just pivotal to understand water security, but failure to incorporate them into the water security system can act as a barrier to enabling systems transformation; the inclusion of these issues is crucial in creating pathways that are appropriate to local context and values.
The systems transformation across these four global settings is different in each context. This variation is not only a demonstration of the different system framings, but also the capacities of ST adoption and implementation. The understandings of water security systems interaction(s) for water security move from interdisciplinarity, to data sub-systems, to boundaries, to values – the increasing complexity demanding increased ST capacity from those involved. Whilst the various systems outputs (the figures) are mainly for conceptualising this systems transformation, the figures for each Collaboratory are valuable both as outputs and as processes of collaborative creation – all of these being useful tools to support more holistic water security thinking.
As well as solutions and insights, the different applications of ST in these four catchments have identified a number of barriers that need to be overcome if this approach is to be used in practice for water security. Our recommendations for providing the enabling conditions that can mitigate these barriers can be broken down into creating, articulating, and using a systems framing, similar to the steps described by Jackson (Citation2006).Footnote1 The operationalisation of a ST framework for those involved, if inexperienced, is a demanding process. Time, resources, and facilitation are required in order to initiate and operationalise ST. At an early stage in ST application, it is therefore necessary to develop a shared interdisciplinary language, and a commitment to long-term dynamic thinking and communication from all parties involved. Related to this is the need to challenge and expand or integrate existing disciplinary framings of the system in order to develop a shared framing of contextual water security that ultimately allows system-scale problems to be addressed. Hence, the degree of interdisciplinarity amongst research team members and stakeholders is a useful indicator of the capacity and readiness to systems transformation within a river basin. Another challenge arises when considering the communication of ST with stakeholders. To bring ST to stakeholders, creation and communication should be a co-generation process with emphasis on application of useful outputs rather than theoretical considerations. Interaction with water security systems should be the focus not intervention, engaging with the stream of the situation in what soft system methodology describes as moving from Mode 1 to Mode 2 (Checkland Citation2008).Footnote2
Achieving water security is often considered an intractable and, in the face of changing climatic and socio-economic conditions, a continuous challenge. Very much intwined in water security discourse is the uncertainty of water availability and distribution under climate change impacts. In order to understand and adapt to these uncertainties, interconnections across levels of policy, resource, and sub-systems (as discussed in Pham-Truffert et al. Citation2020) must be recognised to create integrated and resilient solutions. ST is shown to be a powerful tool to help address this. However, the resulting systems transformation is a long-term process requiring continuous guiding and encouragement of direction; allowing for the capacity to dynamically respond as more is revealed about the system(s), actors become more aware of their role within the system, and as the system responds to change from exogenous factors. Hence, the concept of ST itself in combination with the complexity of water security demands a lot from all involved in the process, but especially those who are the implementors of systems transformation; usually entrenched in disciplinary silos and not used to connecting systematically. Whilst we recognise that ST is an extremely useful approach to enable in water security understanding, research, and action – we also stress the need to further develop these tools and methods for policy makers, consultants and communities in order to facilitate and mainstream the implementation of ST in water security.
5. Conclusion
Systems thinking (ST), underpinned by core elements of behaviour, integration, scale, and context, has been applied to four case studies around the world. We show how ST has: (i) broken down disciplinary and sectoral silos in Johor; (ii) enabled work to be undertaken at a spatial scale appropriate to the complexity of the water security challenge in Addis Ababa; (iii) improved data acquisition and analysis in Delhi to better understand interactions and relationships between sub-systems; and (iv) allowed the integration of socio-ecological issues of justice and power with bio-physical understanding of catchment processes in the UCRB. The figures attempt to conceptualise how these have been achieved, but also have value in themselves as both an output and a process. Demonstrating that this approach provides a platform to produce integrated sustainable solutions for water security and address systems level problems that require synthesis, not reductionism (Jowitt Citation2020).
Creating of systems frames and adoption of ST are necessary precursors to systems transformation for addressing water security problems, both built on the fundamental foundation of interdisciplinarity. Widespread adaptation of ST for water security requires a shared language from which a shared framework and understanding of the system can be developed. Data acquisition and monitoring programmes must be designed to understand the system (especially interactions between sub-systems) and to reflect system-level outcomes to avoid incentivising siloed actions that conflict other objectives. A range of empirical, physically based, and conceptual tools are available to support ST, but more work needs to be done to build capacity amongst actors and make these tools more accessible, to mainstream ST into practice.
Disclosure statement
No potential conflict of interest was reported by the author(s).
Additional information
Funding
Notes
1 Jackson (Citation2006) describes four phases of a meta-methodology of creative holism: creativity, choice, implementation, and reflection.
2 Moving from Mode 1 to Mode 2 indicates that the systems user is immersed in the situation rather than simply being an observer. Hence, the focus is on interaction not intervention.
References
- Allan, T. 1998. “Watersheds and Problemsheds: Explaining the Absence of Armed Conflict Over Water in The Middle East.” Middle East Review of International Affairs, 5. https://ciaotest.cc.columbia.edu/olj/meria/meria398_allan.html#txt.
- Allan, T., Jun Xia Catherine, and C. Pahl-Wostl. 2013. “Climate Change and Water Security: Challenges for Adaptive Water Management.” Current Opinion in Environmental Sustainability 5 (6): 625–632. doi:10.1016/j.cosust.2013.09.004.
- Andrews, M., L. Pritchett, and M. Woolcock. 2013. “Escaping Capability Traps Through Problem Driven Iterative Adaptation (PDIA).” Center for Global Development Working Paper No. 299, HKS Working Paper No. RWP12-036. doi:10.2139/ssrn.2102794.
- Bakker, K., and C. Morinville. 2013. “The Governance Dimensions of Water Security: A Review. Philosophical Transactions of the Royal Society A: Mathematical.” Physical and Engineering Sciences 371 (2002): 20130116. doi:10.1098/rsta.2013.0116.
- Bartram, J., C. Brocklehurst, D. Bradley, M. Muller, and B. Evans. 2018. “Policy Review of the Means of Implementation Targets and Indicators for the Sustainable Development Goal for Water and Sanitation.” NPJ Clean Water 1 (3), doi:10.1038/s41545-018-0003-0.
- Boulding, K. E. 1956. “General Systems Theory – The Skeleton of Science.” Management Science 2 (3): 197–208.
- Butte, G., Y. T. Solano-Correa, M. V. Peppa, D. Ruiz-Ordónez, R. Maysels, N. Tuqan, X. K. Polaine, C. Montoya-Pachongo, C. L. Walsh, and T. P. Curtis. 2022. Data Gathering Strategies for Water Security: A Proposed Framework Approach. doi:10.1002/essoar.10511218.1.
- Castalia (Castalia Strategic Advisors for Evidence on Demand). 2014. Barriers to Infrastructure Service Delivery in Sub-Saharan Africa and South Asia. [pdf]. https://assets.publishing.service.gov.uk/media/57a089baed915d3cfd0003d0/P813_EOD_Castalia_Barriers.pdf.
- Checkland, P. 2008. Soft Systems Methodology: A 30-Year Retrospective. Chichester: Wiley.
- Checkland, P., and S. Holwell. 2004. “‘Classic’ OR and ‘Soft’ OR – An Asymmetric Complementarity.” In Systems Modelling: Theory and Practice, edited by M. Pidd, 56–58. Chichester: John Wiley & Sons.
- Checkland, P., and J. Poulter. 2006. Learning for Action: A Short Definitive Account of Soft Systems Methodology and its use for Practitioners, Teachers and Students. Hoboken: Wiley.
- Chen, Z., and S. Wei. 2013. “Application of System Dynamics to Water Security Research.” Water Resources Management 28 (2): 287–300. doi:10.1007/s11269-013-0496-8.
- Chiluwe, Q., and M. Claassen. 2020. “Systems Perspectives on Water Security: An Applied Review and Conceptual Framework.” Environmental Policy and Governance 30 (6): 332–344. doi:10.1002/eet.1889.
- Cook, C., and K. Bakker. 2012. “Water Security: Debating an Emerging Paradigm.” Global Environmental Change 22 (1): 94–102. doi:10.1016/j.gloenvcha.2011.10.011.
- CPCB (Central Pollution Control Board). 2013. Monitoring of Indian National Aquatic Resources Series: MINARS/35/2013-14. (2013) Central Pollution Control Board, Ministry of Environment & Forests. New Delhi: Government of India. Status of Water Quality in India – 2011.
- De Stercke, S., V. Chaturvedi, W. Buytaert, and A. Mijic. 2020. “Water-Energy Nexus-Based Scenario Analysis for Sustainable Development of Mumbai.” Environmental Modelling and Software 134: 1–17. doi:10.1016/j.envsoft.2020.104854.
- Dias, P., and P. Jowitt. 2020. “What is the Body of Knowledge for Engineers Involved with Civil Engineering Systems? – A 2020 Vision’.” Civil Engineering and Environmental Systems 37 (4): 149–153. doi:10.1080/10286608.2020.1858065.
- Eustachio, J., A. Caldana, L. Liboni, and D. Martinelli. 2019. “Systemic Indicator of Sustainable Development: Proposal and Application of a Framework.” Journal of Cleaner Production 241: 118383. doi:10.1016/j.jclepro.2019.118383.
- Everard, M. 2014. “Water Security for Nature and People.” Environmental Scientist-Water Security 23: 3. https://www.the-ies.org/resources/water-security.
- Fraser, N. 2010. Scales of Justice: Reimagining Political Space in a Globalizing World. New York: Columbia University Press.
- Garrick, D., J. Hall, A. Dobson, R. Damania, R. Grafton, R. Hope, C. Hepburn, et al. 2017. “Valuing Water for Sustainable Development.” Science 358 (6366): 1003–1005. doi:10.1126/science.aao4942.
- GWP (Global Water Partnership). 2000. Towards Water Security: A Framework for Action. Stockholm: GWP. [pdf]. https://www.gwp.org/globalassets/global/toolbox/references/towards-water-security.-a-framework-for-action.-mobilising-political-will-to-act-gwp-2000.pdf.
- Habermas, J. 1991. Moral Consciousness and Communicative Action. Cambridge, MA: MIT Press.
- Haileslassie, A., E. Ludi, M. Roe, and C. Button. 2020. “Water Values: Discourses and Perspective.” In Encyclopedia of the UN Sustainable Development Goals, 946–955. doi:10.1007/978-3-319-70061-8_140-1
- Hernández, V. A. C., G. F. Vargas, A. Figueroa, and I. Restrepo. 2019. “El Enfoque De Sistemas Socioecológicos En Las Ciencias Ambientales.” Investigación Y Desarrollo 27 (2): 85–109. https://www.redalyc.org/journal/268/26864302004/html/.
- Heynen, N., M. Kaika, and E. Swyngedouw. 2005. In the Nature of Cities – Urban Political Ecology and The Politics of Urban Metabolism, edited by N. Heynen, M. Kaika, and E. Swyngedouw. London: Routledge.
- Hipel, K., L. Fang, T. Ouarda, and M. Bristow. 2013. “An Introduction to the Special Issue on Tackling Challenging Water Resources Problems in Canada: A Systems Approach.” Canadian Water Resources Journal 38 (1): 3–11. doi:10.1080/07011784.2013.773643.
- Jackson, M. C. 1990. “Beyond a System of Systems Methodologies.” The Journal of the Operational Research Society 41 (8): 657–668. doi:10.2307/2583472.
- Jackson, M. C. 2006. “Creative Holism: A Critical Systems Approach to Complex Problem Situations.” Systems Research and Behavioral Science 23 (5): 647–657. doi:10.1002/sres.799.
- Jackson, M. C. 2009. “Fifty Years of Systems Thinking for Management.” Journal of the Operational Research Society 60 (sup1): S24–S32. doi:10.1057/jors.2008.176.
- Jowitt, Paul W. 2020. “Systems and Sustainability.” Civil Engineering and Environmental Systems 37 (4): 253–263. doi:10.1080/10286608.2020.1839892.
- Kees, C. H., van Ginkel, A. Y. Hoekstra, J. Buurman, and R. J. Hogeboom. 2018. “Urban Water Security Dashboard: Systems Approach to Characterizing the Water Security of Cities.” Journal of Water Resources Planning and Management 144 (12): 04018075. doi:10.1061/(asce)wr.1943-5452.0000997.
- Kjellén, M., R. Sinha, D. Hebart-Coleman, E. Yaari, E. Muratore, A. Loeffen, L. Pories, et al. 2021. Chapter 9: Enabling Multi-value Approaches in Water Governance. In UN World Water Development Report 2021: Valuing Water, UN. doi:10.18356/9789214030140c015.
- Kumar, A., N. Singh, S. Cooper, A. Mdee, and S. Singhal. 2021. “Infrastructural Violence: Five Axes of Inequities in Water Supply in Delhi, India.” Frontiers in Water 3. doi:10.3389/frwa.2021.727368.
- Lane, D. C. 2008. “The Emergence and Use of Diagramming in System Dynamics: A Critical Account.” Systems Research and Behavioral Science 25 (1): 3–23. doi:10.1002/sres.826.
- Lankford, B., B. Bakker, M. Zeitoun, and D. Conway. 2013. Water Security: Principles, Perspectives and Practices. London: Earthscan.
- Lautze, J., and H. Manthrithilake. 2012. “Water Security: Old Concepts, New Package, What Value?” Natural Resources Forum 36 (2): 76–87. doi:10.1111/j.1477-8947.2012.01448.x.
- Le Blanc, D. 2015. “Towards Integration at Last? The Sustainable Development Goals as a Network of Targets.” Sustainable Development 23: 176–187. doi:10.1002/sd.1582.
- Lopez, R. R., R. Boelens, and J. Vos. 2019. “Hydrosocial Territories in Dispute: Flows of Water and Power in an Interbasin Transfer Project in Bolivia.” Water Alternatives 12 (1): 267–284.
- Meadows, D. 2008. Thinking in Systems. 1st ed. London: Earthscan.
- Mirchi, A., K. Madani, D. Watkins, and S. Ahmad. 2012. “Synthesis of System Dynamics Tools for Holistic Conceptualization of Water Resources Problems.” Water Resources Management 26 (9): 2421–2442. doi:10.1007/s11269-012-0024-2.
- Mishra, B., P. Kumar, C. Saraswat, S. Chakraborty, and A. Gautam. 2021. “Water Security in a Changing Environment: Concept, Challenges and Solutions.” Water 13 (4): 490. doi:10.3390/w13040490.
- Mollinga, P. 2020. “Knowledge, Context and Problemsheds: A Critical Realist Method for Interdisciplinary Water Studies.” Water International 45 (5): 388–415. doi:10.1080/02508060.2020.1787617.
- Mollinga, P., R. Meinzen-Dick, and D. Merrey. 2007. “Politics, Plurality and Problemsheds: A Strategic Approach for Reform of Agricultural Water Resources Management.” Development Policy Review 25 (6): 699–719. doi:10.1111/j.1467-7679.2007.00393.x.
- Muradian, R., and E. Gómez-Baggethun. 2021. “Beyond Ecosystem Services and Nature’s Contributions: Is It Time To Leave Utilitarian Environmentalism Behind?” Ecological Economics 185: 107038. doi:10.1016/j.ecolecon.2021.107038.
- O’Donnell, G., J. Ewen, and P. E. O’Connell. 2011. “Sensitivity Maps for Impacts of Land Management.” Physics and Chemistry of the Earth 36 (13): 630–637.
- Olabisi, S., S. Liverpool-Tasie, L. Rivers, A. Ligmann-Zielinska, J. Du, R. Denny, S. Marquart-Pyatt, et al. 2018. “Using Participatory Modeling Processes to Identify Sources of Climate Risk in West Africa.” Environment Systems and Decisions 38 (1): 23–32. doi:10.1007/s10669-017-9653-6.
- Ostrom, E. 2007. “A Diagnostic Approach for Going Beyond Panaceas.” Proceedings of the National Academy of Sciences of the United States of America 104 (39): 15181–15187. doi:10.1073/pnas.0702288104.
- Ostrom, E. 2009. “A General Framework for Analyzing Sustainability of Social-Ecological Systems.” Science 325 (5939): 419–422. doi:10.1126/science.1172133.
- Pantha, K., K. Acharya, S. Mohapatra, S. Khanal, N. Amatya, C. Ospina-Betancourth, G. Butte, et al. 2021. “Faecal Pollution Source Tracking in the Holy Bagmati River by Portable 16S rRNA Gene Sequencing.” NPJ Clean Water 4 (1): 1–10.
- Pham-Truffert, M., F. Metz, M. Fischer, H. Rueff, and P. Messerli. 2020. “Interactions Among Sustainable Development Goals: Knowledge for Identifying Multipliers and Virtuous Cycles.” Sustainable Development 28: 1236–1250. doi:10.1002/sd.2073.
- Polaine, X. K., A. Nicol, J. Amezaga, M. Berihun, M. Dessalegn, and A. T. Haile. 2022. “Problemscapes and Hybrid Water Security Systems in Central Ethiopia.” Frontiers in Water 4: 800926. doi:10.3389/frwa.2022.800926.
- Pope, K., M. Bonatti, and S. Sieber. 2021. “The What, Who and How of Socio-Ecological Justice: Tailoring a New Justice Model for Earth System Law.” Earth System Governance 10: 100124. doi:10.1016/j.esg.2021.100124.
- Scheidel, A., L. Temper, F. Demaria, and J. Martínez-Alier 2018. “Ecological Distribution Conflicts as Forces for Sustainability: An Overview and Conceptual Framework.” Sustainability Science 13 (3): 585–598. doi:10.1007/s11625-017-0519-0.
- Schenk, C., B. Roquier, M. Soutter, and A. Mermoud. 2009. “A System Model for Water Management.” Environmental Management 43 (3): 458–469. doi:10.1007/s00267-008-9254-8.
- Senge, P. M. 1990. The Fifth Discipline. The art and Practice of the Learning Organization. London: Random House.
- Ulrich, W. 2000. “Reflective Practice in the Civil Society: The Contribution of Critically Systemic Thinking.” Reflective Practice 1: 247–268.
- Ulrich, W. 2012. “Operational Research and Critical Systems Thinking – An Integrated Perspective Part 1: OR as Applied Systems Thinking.” The Journal of the Operational Research Society 63 (9): 1228–1247.
- Ulrich, W. 2013. “Critical Systems Thinking.” In Encyclopedia of Operations Research and Management Science. 3rd ed., edited by S. I. Gass, and M. C. Fu, 314–326. New York: Springer.
- UNESCO. 2017. International Hydrological Programme (IHP)-VIII Water Security: Responses to Local, Regional, and Global Challenges (2014-2021). Paris: UNESCO. [pdf]. https://en.unesco.org/sites/default/files/ihp_brochure_long_2017.pdf.
- United Nations University Institute for Water, Environment & Health (IWEH). 2013. Water Security & the Global Water Agenda: A UN-Water Analytical Brief. https://www.unwater.org/publications/water-security-global-water-agenda/.
- Vickers, G. 1995. The Art of Judgment: A Study of Policy Making. Centenary ed. Advances in Public Administration. Thousand Oaks, CA: Sage Publications.
- Vogl, A. L., J. H. Goldstein, G. C. Daily, B. Vira, L. Bremer, R. I. McDonald, D. Shemie, B. Tellman, and J. Cassin. 2017. “Mainstreaming Investments in Watershed Services to Enhance Water Security: Barriers and Opportunities.” Environmental Science & Policy 75: 19–27.
- Zhang, T., Q. Tan, S. Zhang, T. Zhang, and W. Zhang. 2021. “A Participatory Methodology for Characterizing and Prescribing Water-Energy-Food Nexus Based on Improved Casual Loop Diagrams.” Resources, Conservation and Recycling 164: 105124. doi:10.1016/j.resconrec.2020.105124.