Abstract
In this study, the moisture sensitivity of different kinds of aggregates and bituminous binders is examined by comparing the performance between five empirical test methods for loose mixtures – static immersion test, rolling bottle test (RBT), boiling water test (BWT), total water immersion test and the ultrasonic method – with more fundamental surface energy-based test data. The RBT and BWT results showed that limestone aggregates perform better than granite aggregates and that, for unmodified binders, stiffer binders provide better moisture resistance compared with softer binder. Both tests were sensitive to aggregate type, binder type and anti-stripping agent type. Ranking of the mixtures by RBT and BWT was in general agreement with the surface energy-based tests, especially for mixtures that performed worst or best in RBT and BWT. The magnitude of the work of debonding in the presence of water was found to be aggregate type dependent which suggests the physico-chemical properties of aggregates may play a fundamental and more significant role in the generation of moisture damage, than bitumen properties.
1. Introduction
It has long been recognised that moisture can influence the physical and mechanical properties of bituminous paving mixtures (Hunter and Ksaibati Citation2002). In the coming years, damage in bituminous pavements caused by moisture may become increasingly important as global warming may lead to higher precipitation than currently experienced all over the world. According to a recent United Nations Intergovernmental Panel on Climate Change report (Christensen et al.Citation2007, Meehl et al.Citation2007), global average annual precipitation through the end of the century is expected to increase with changes in the amount and intensity of precipitation increasing pronouncedly in tropical and high-latitude regions.
Damage caused in part by moisture such as stripping, rutting, ravelling and fatigue cracking contributes significantly to failure in bituminous pavements. Because of a lack of fundamental understanding of the moisture damage problem, there are currently no satisfying solutions for this problem, only theories exist. Two of these theories are, however, commonly accepted. It is generally accepted that the two major factors that cause moisture-related problems include adhesive failure (i.e. bitumen stripping off the aggregate surface) and cohesive failure (i.e. the loss of mixture stiffness especially in the mastic). In addition, compatibility of the bitumen and the aggregate, the volumetric properties of binder and air voids, and the permeability of the mixtures are all important factors when considering the durability of bituminous paving mixtures.
As the performance of bituminous paving mixtures in the presence of water is a complex issue, numerous research studies have been carried out to simulate moisture damage. Over the years, bituminous technologists have been in search of a dependable laboratory test to predict the performance of bituminous paving mixtures in the field as far as moisture sensitivity is concerned (Solaimanian et al.Citation2003). These include tests to measure a change in physical and/or mechanical properties after immersion in water and to identify the degree of resistance to moisture damage through a specific combination of bitumen and aggregate. These tests can be generally divided into two tests: tests for loose bitumen-coated aggregate mixtures and tests for compacted asphalt mixtures.
Early work on the moisture damage problem could be traced back to at least the 1920s when the fact that water can reduce the adhesion between bitumen and aggregate and that the cohesion within the bituminous binder itself deteriorates became known to practitioners. As a result, several workers (Nicholson Citation1932, Hubbard and Shuger Citation1938, Lottman Citation1978) concentrated on solving this problem from different perspectives. As can be seen in Figure , numerous factors have been cited as causes of moisture damage in asphalt mixtures depending on whether the individual asphalt components (level 1), loose asphalt mixtures (level 1-2), compacted asphalt mixtures (level 2) or in-service pavement (level 3) performance is being considered. Because of this, many tests have been developed to examine moisture sensitivity from different aspects.
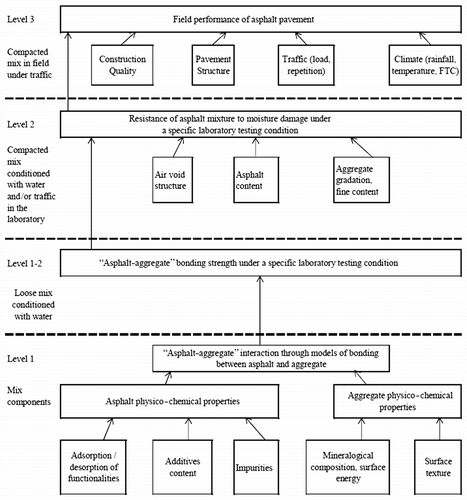
The focus of this study was limited to the examination of the aggregate–bitumen bonding strength using empirically based loose bitumen-coated aggregate moisture sensitivity tests and surface energy-based physico-chemical tests of constituent aggregate and bitumen in the laboratory. The loose bitumen-coated aggregate tests conducted included static immersion test, rolling bottle test (RBT), boiling water test (BWT), total water immersion test and ultrasonic test. The surface energy properties of the aggregates and bitumens were obtained using dynamic vapour sorption (DVS) and the dynamic contact angle (DCA) analyser tests, respectively.
The study examines the moisture sensitivity of aggregates and bituminous binders by comparing the performance between the five empirical test methods for loose mixtures with the more fundamental surface energy-based test data. Six different types of aggregates (three limestone and three granite aggregates) with known field performance and seven different kinds of bituminous binders (including five with anti-stripping agents) were selected for testing.
2. Materials, sampling and testing
Table shows a summary of the materials and methods used. They included two aggregate types, seven binder types and six test methods. Not all the aggregate–bitumen combinations were tested using all six test methods but the data included the performance of both the best and worst combinations.
Table 1 Materials and test methods.
Aggregates with known field performance from six different sources were sampled for testing. They included three limestone aggregates (L1, L2 and L3) and three granite aggregates (G1, G2 and G3). Based on field experience, the limestone aggregates tend to be more resistant to moisture damage than granite aggregates. Therefore, it was expected that a discriminating laboratory test should be able to distinguish between the mixtures based on the selected aggregates.
In most of the existing test standards for evaluating moisture resistance of loose asphalt mixtures, the most commonly used aggregate size ranges from 6.3 to 9.5 mm. Therefore, for each of the six aggregate types selected for testing, only material passing the 9.6-mm sieve size but retained on the 6.3-mm sieve was used.
A total of seven bitumen types were used for coating the aggregates. Two of the seven binders were refined bitumen from Venezuelan crude with penetration grades of 40/60 and 160/220. The measured penetration of the 40/60 pen bitumen was 57 compared with 198 for the 160/220 pen bitumen. Similarly, the measured softening points were 50.7 and 38.0°C, respectively, for the 40/60 pen and 160/220 pen binders. The penetration of the modified binders was not tested. The 40/60 pen bitumen was made by blending 24% of a 160/220 pen binder with 76% of a 30/45 pen binder.
The five modified binders used all had 0.5% (by weight of binder) liquid anti-stripping agent added as adhesive promoters. The anti-stripping agents added included four amine-based types (AAS1, AAS2, AAS3 and AAS4) and one non-amine anti-stripping agent (NAAS). Anti-stripping agents reduce surface tension between the bitumen and aggregate in a mixture thereby promoting increased adhesion of the bitumen to the aggregate surface. The anti-stripping agents also work by promoting better wetting of bitumen onto the aggregate which also contribute to better adhesion. The five modified binders were prepared by blending the 40/60 pen binder with four amines and one NAAS. It must be noted that because of time and material constraints, not all aggregate–bitumen combinations were tested.
3. Test methods
3.1 Static immersion test
The static immersion test was conducted in accordance with ASTM D1664 (AASHTO T182). During the test, a 100 g sample of aggregate with sizes ranging from 6.3 to 9.5 mm coated with 5.5 g of bitumen was immersed in distilled water at 25°C for 16–18 h in a 500-ml glass bottle. The sample was then observed through the glass to estimate the percentage of total visible area of aggregate that remains coated as above or below 95%. Three replicates of 100 g aggregate coated with bitumen were tested, and the average percentage coated estimated. Some of the disadvantages of the test are (1) the test is subjective and therefore has high variability, and (2) the test does not involve any strength tests that directly relate to mixture performance. As shown in Table , only two binders (40/60 pen and 160/220 pen) were evaluated using the static immersion test.
3.2 Rolling bottle test
The RBT was conducted in accordance with BS EN 12697-11 (bituminous mixtures – test methods for hot mix asphalt – part 11: determination of the affinity between aggregate and bitumen). The RBT is a subjective test in that affinity is expressed by visual estimation of the degree of bitumen coverage on uncompacted bitumen-coated mineral aggregate particles after the influence of mechanical stirring action in the presence of water. To perform the test, dust-free aggregate samples weighing 170 g were dried in an oven at 105 ± 5°C overnight to constant mass and then coated with 5.7 g of molten binder. Mixing of the aggregates with a binder was conducted at 120 ± 5°C. The aggregate–binder mixture was then cooled loose at room temperature. The loose mixture was stored at ambient temperature for 12–64 h before testing. Each of the test bottles was filled to about half their volume with deionised water, and about 150 g of the loose aggregate–mixture was placed in each bottle. The whole assembly was put in the bottle roller rotating at a speed of 60 rotations per minute for about 6 h. At the end of the 6-h period, the aggregate particles were emptied from the test bottle into a test bowl which was then filled with fresh, deionised water to a level just above the top of the surface of the particles. Subsequently, the test bowl was placed on a white surface. The purpose of adding fresh water was to allow for optimal visual determination of binder coverage on the aggregate particles. At least three replicates of each sample were tested.
At the end of the test, the degree of bitumen coverage of the aggregate particles was estimated by visual observation and recorded to the nearest 5%. The degree of bitumen coverage was defined as the average proportion of the surface area of the aggregate particles that are covered with bitumen, expressed as a percentage ( = 100–the percentage of stripping). The degree of bitumen coverage on the aggregate particles was visually estimated by two experienced technicians independently. The procedure (i.e. rotation in the bottle roller and measuring of bitumen coverage) was repeated for three more cycles (24, 48 and 72 h) with fresh water replacing the fouled water in the test bottle at the end of each cycle and the degree of bitumen coverage estimated as discussed earlier. For each rolling time (6, 24, 48 and 72 h), the mean value of each technician's recordings of the average degree of bitumen coverage obtained on the three part samples (three bottles) was calculated to the nearest 5%, and the results were averaged to obtain the average degree of bitumen coverage for a given mixture.
3.3 Boiling water test
The BWT was performed in accordance with ASTM D3625-96(2005) (Standard Practice for Effect of Water on Bituminous-Coated Aggregate Using Boiling Water). Compared with the static immersion test and RBT, the BWT is a quicker approach to evaluate the moisture sensitivity of the bitumen and aggregate combination because it takes only about 60 min to condition compared with more than 72 h for RBT or 16–18 h in the case of the static immersion test. Like the static immersion test, the BWT cannot be used as a measure of field performance because such correlation has not been established. At least three replicates of each sample were tested.
To perform the test, 600 g of clean oven-dried aggregates was fully coated with 30 g of molten binder. About 300 g each of the aggregate–bitumen mixture was submerged under boiling water in a glass beaker. The mixture was boiled for 10 min. The percentage of the total visible area of the aggregate that retained its original coating of bitumen was used as an estimate of moisture damage. As shown in Table , only two binders (40/60 pen and 160/220 pen) were evaluated using the BWT.
3.4 Total water immersion test
The total water immersion test is performed in the laboratory to compare the performance of bitumen doped with an adhesion agent against the non-doped bitumen. This is necessary to check each aggregate with non-doped and doped bitumen to assess the effectiveness of the additive or whether the aggregate needs additive in the binder to provide proper adhesion. Three replicates of each sample were tested.
The test assesses the average percentage of binder coverage after immersion in 40°C water after 3 h of soaking. The test is an improvement on the static immersion test. It uses water at 40°C rather than room temperature (25°C) used in the static immersion test to provide a better result. As shown in Table , only two binders (40/60 pen and 160/220 pen) were evaluated using the total water immersion test.
3.5 Ultrasonic method
The ultrasonic method was developed to measure the resistance to stripping of coated aggregates (Hveem Citation1943, Andersland and Goetz Citation1956, Thelen Citation1958, Jimenez Citation1974). The test requires a polished stone test piece be coated with 2 g of bitumen that gives a 0.12-mm thick binder film. About 30 pieces of aggregates are required. By subjecting to ultrasound under water, the bitumen is stripped mechanically from the stone. The degree of stripping is determined either by weighing the stripped test piece or by visual assessment after 5, 10, 15 and 20 min of conditioning in the ultrasonic bath. At least three replicates of each sample were tested.
3.6 DCA analyser test
A DCA analyser (Thermo Scientific CAHN Radian DCA, Newington, NH, USA) was used to determine the surface energy components of the binders. Surface energy components of each bitumen was estimated using the contact angles that a set of three probe liquids with known surface energy components make with bitumen (in solid state) under dynamic conditions. The probe liquids used included water, glycerol and diiodomethane, and their surface energy components are shown in Table .
Table 2 Surface energy components (mJ/m2) of probe liquids for DCA test.
During the DCA test, a clean 40 mm × 24 mm × 0.45 mm no. 15 microscope glass slide is coated with bitumen and hung from the balance of the DCA equipment with the help of a crocodile clip. A beaker containing a probe liquid is placed on a movable stage positioned under the glass slide. The bitumen-coated glass slide is then immersed up to a maximum depth of 5 mm (advancing) and then withdrawing (receding) from the liquid by moving the stage up and down, respectively, at a constant speed of 40 μm/s while continuously recording the change in mass of the bitumen-coated slide against depth of immersion. The results are used to compute the contact angle between the bitumen and the probe liquid. All the DCA measurements were obtained at room temperature (23 ± 2°C and 50 ± 5% relative humidity). Three replicates of each bitumen–probe liquid combination were tested.
Detailed discussion of the DCA method for estimating surface energy of bitumen is provided elsewhere (Bhasin Citation2006, Bhasin et al.Citation2006, Ahmad Citation2011). Basically, the approach uses the measured mass–depth relationships to estimate the forces acting on a bitumen-coated slide as it is being immersed or removed from a probe liquid to determine contact angles between the binder and at least three probe liquids. The contact angle results from the three probe liquids are used in Equation (1) to obtain three simultaneous equations from which the three surface energy components (γLW, γ+ and γ− ) could be estimated. The estimated surface energy components are then used to determine the total surface energy () of the binders using Equation (2) from which the cohesive bond strength (equals to twice
) of the binder could also be obtained.
3.7 DVS test
A DVS system (DVS Advantage 2, Surface Measurement Systems, Middlesex, UK) was used to determine the surface energy components of the aggregates using sorption isotherms obtained at 25°C. Detailed discussion of the approach has been provided elsewhere (Ahmad Citation2011). Six oven-dried aggregates (only fractions passing 5-mm sieve and retained on 2.36-mm sieve) and three probe liquids (octane, ethyl acetate and chloroform) with known surface energy components were used for the DVS tests. Table lists the surface energy components of the probe liquids. Basically, the DVS approach for determining surface energy properties of aggregates involves measuring the weight gain of an aggregate sample (usually less than about 10 g in weight) kept in a sealed DVS sample chamber containing a probe liquid vapour (at partial pressures ranging from 0% to 95%). Only a single replicate of each aggregate–probe liquid combination was tested as each sorption isotherm took more than a week to complete.
Table 3 Surface energy components (mJ/m2) of probe liquids for DVS test.
For each aggregate–probe liquid combination, mass gain in the aggregates is monitored, using an ultra-sensitive balance, at 14 different partial pressures until equilibrium mass is reached at each partial pressure stage. The results (i.e. equilibrium mass) are plotted against partial pressure to generate sorption isotherms from which the specific surface area (SSA) and spreading equilibrium pressures (Bhasin Citation2006) for each of the three probe liquids could be estimated. The results are then used to estimate the surface energy components of the aggregates as discussed in the following.
Similar to Equation (1) for determining surface energy of binders, Equation (3) was used to generate three simultaneous equations which could be solved to obtain the three surface energy components by using the total surface energy and the spreading pressure of each probe liquid. The total surface energy of the aggregates is given by Equation (4), using the surface energy components of the aggregates.
3.8 Bond energy parameters
The surface energy parameters obtained from Equations 123(4) were used to estimate three bond energy parameters: binder cohesion, dry bond strength and work of debonding; the results are used to assess the moisture sensitivity of an asphalt mixture by computing the four moisture compatibility ratios at various aggregate–bitumen combinations (Bhasin Citation2006, Bhasin et al.Citation2006).
Binder cohesion is the cohesive bond strength of the binder material and is estimated as twice the total surface energy. Dry bond strength (Equation (5)) is defined as the interfacial work of adhesion between bitumen and aggregate. A bigger value of dry bond strength suggests greater adhesion between the two materials, and hence more resistance against debonding in the absence of moisture. The work of debonding (Equation (6)) is the reduction in bond strength of a bitumen–aggregate system in the presence of moisture. A smaller value (magnitude) of this parameter for a given bitumen–aggregate system is indicative of a better moisture damage performance of that system.
3.9 Compatibility ratios
Equations (7) and (8) were used to compute the moisture compatibility ratios (ER1, ER1 × SSA, ER2 and ER2 × SSA). Higher values of the ratios suggest higher resistance to moisture damage.
4. Results and discussion
4.1 Static immersion test
During the test, the percentage of total visible area of aggregate that remains coated after 16–18 h of soaking is estimated as above or below 95%. The results are presented in Figure . The results indicated that 100% of the aggregate remained coated at the end of the test for all the limestone aggregate mixtures. For the granite mixtures, the percentage coated area observed for each aggregate was above 95% with the exception of G3 aggregate that showed about 90% coated area.
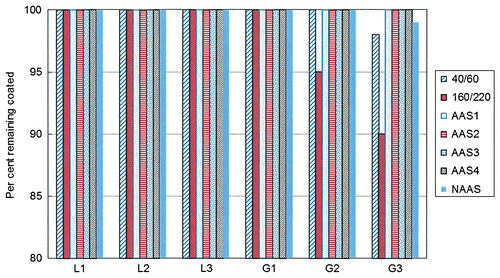
The results suggest that most of the aggregate–binder combinations showed similar bonding (>95% of coated aggregates) properties after undergoing the static immersion test. The exception was the combinations of G3 granite. Combinations of G3 with 40/60 pen binder, 160/220 pen binder and NAAS resulted in per cent-stripped aggregate of 10%, 2% and 1%, respectively. The combination of G1 with 160/220 pen binder also lost 5% of binder. In terms of moisture sensitivity, the static immersion test results suggest that G3 granite with 160/220 pen binder was the worst mixture. This result is in agreement with previous studies (Vuorinen and Hartikainen Citation2001, Airey and Chio Citation2002) that used similar aggregates as used in this study. Results for the mixtures containing amine-based anti-stripping agents with retained binder >95% appear to be in agreement with previous research (Ahmad Citation2011). Even though the static immersion test ranked the G3–60/200 pen combination as worst in terms of moisture sensitivity, the test appears not to be sensitive to different aggregate types as it ranked L1, L2, L3, G1 and G2 aggregates with all the binders, except 160/220 pen, equally.
4.2 Rolling bottle test
In the rolling bottle method, the degree of bitumen coverage of the coated aggregate particles was checked after 6, 24, 48 and 72 h of conditioning in a bottle roller. The results are presented in Figure .
Mixtures containing the unmodified binders showed higher binder loss than the modified binders containing anti-stripping agents. Binder losses in the mixtures containing the 160/220 pen binder were the highest for each aggregate type tested (Figure ). Binder losses in the 40/60 pen mixtures were just slightly < 160/220 pen binder – both were higher than the mixtures containing anti-stripping agents for most of the aggregates considered.
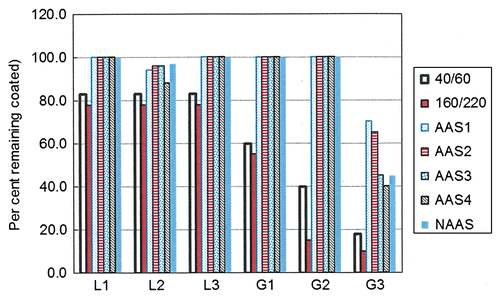
The use of the anti-stripping agents caused measurable reduction in binder loss for all the aggregates considered; the effect was more pronounced in the G3 mixtures. For example, the use of AAS1 resulted in only 30% binder loss compared with 90% binder loss for the 160/220 pen mixtures. The results show that the anti-stripping agents used in this study were effective in reducing moisture sensitivity in moisture-susceptible aggregates such as G3.
The results also show that the RBT is sensitive to changes in aggregate and binder property including binder modification. Compared with the static immersion test, the RBT appears more discriminatory as it was able to show small differences in moisture susceptibility in the good performing L2 limestone aggregates. For example, ranking in this case was in increasing order of resistance: 160/220 pen, 40/60 pen, amine-based anti-stripping agent and NAAS, which was to be expected.
Compared with the static immersion test, the sensitivity of the RBT was higher. Figure shows the binder loss versus conditioning time obtained for mixtures containing 40/60 pen binders, which illustrates the sensitivity of the RBT to different aggregate types. The limestone aggregates (L1, L2 and L3) appeared to perform better than granite aggregates (G1, G2 and G3). The results show G3 as the worst performing aggregate again as expected based on field performance.
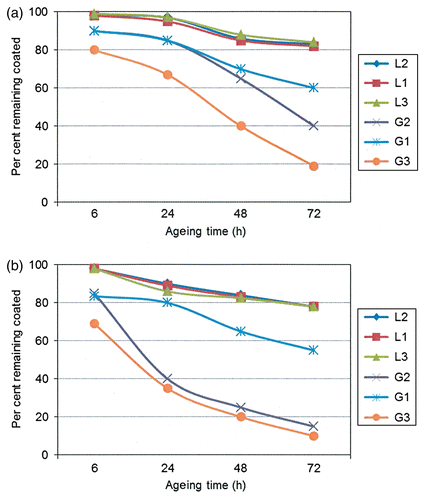
From the curves in Figure , it could be seen that the percentages of bitumen coverage decreased slowly with testing time for limestone; on the contrary, percentages for granite reduce sharply during the test period. For instance, during the first 6 h, L2 showed only a 2% binder loss while G3 showed about 20% loss. In addition, the percentage of binder loss for G3 at 6 h is equal to that for the limestone aggregates at 72 h. Among the granite, G1 showed the best bonding properties as illustrated by the 10%, 15%, 30% and 40% of binder loss for 6, 24, 48 and 72 h, respectively. Although the total loss of binder for G2 was more than G1, these two aggregates had almost the same percentage of binder loss at the first 24 h. It can be concluded that considering 40/60 pen binder, L3 is the best aggregate with the least binder loss and G3 is the worst. Similar results as for the 40/60 pen binders were obtained for the softer 160/220 pen binder as shown in Figure .
4.3 Boiling water test
The BWT estimates the percentage of bitumen coverage after 10 min of boiling. Figure presents the result of G3 with 40/60 pen binder. The results are quite obvious; practically all of the binder has been stripped of aggregates.
For all the mixtures tested, an attempt was made to estimate percentage binder loss after conditioning in water. The results are shown in Figure . It can be seen that G3, again, exhibited the worst bonding property. Considering the limestone aggregates, the performance of L1 and L2 was similar for both 40/60 pen and 160/220 pen binders but minor differences could be seen with L3 where 160/220 pen binder showed higher stripping as expected. In terms of granite, the 160/220 pen binder seems to have a better bond property than the 40/60 pen binder except for G1 that had 5% more binder loss. As 160/220 pen is softer than 40/60 pen binder, it was expected that the 160/220 pen mixtures should have higher (worse) moisture susceptibility. The reason for this anomaly could be because 160/220 pen has a lower softening point and therefore may tend to coat the aggregates during the test. More tests may be needed to confirm this in future studies.
4.4 Total water immersion test
Figure shows the average percentage of binder coverage after immersion in 40°C water for 3 h obtained during the total water immersion test. From Figure , it could be seen that the limestone aggregates had very little binder loss compared with the granite aggregates. The percentages of binder loss for limestone were all < 5% for the two binder types. The results were completely the opposite for the granite where the percentages of binder loss were quite high. Unexpectedly, the G1 mixtures showed nearly the same bonding properties as the limestone with only 3% loss for 40/60 pen binder and 5% for 160/220 pen binder. As in the tests previously discussed, G3 aggregates were the worst performing aggregate with 20% and 30% binder losses, for 40/60 pen and 160/220 pen, respectively.
4.5 Ultrasonic method
By subjecting to ultrasound under water, the bitumen is stripped mechanically from the stone. As the ultrasonic bath cannot be used for no more than 10 min at a time, a 5-min cycle duration was used. The degree of stripping was determined by visual assessment after 5, 10, 15 and 20 min of conditioning in the ultrasonic bath.
Figure shows typical results after three cycles of conditioning obtained using the granite aggregate G3. The left plate shows samples of 40/60 pen mixtures while the right shows results for the 160/220 pen binder. The results were not expected as G3 has poor moisture resistance based on field performance. Similar results were observed for all the other aggregate binder combinations. It is obvious that the test is not as sensitive as some of the tests previously discussed.
5. Discussion
5.1 Sensitivity of qualitative test to aggregate type
The results of the five loose bitumen-coated aggregate mixture tests presented in this study show that limestone aggregates in general performed better than granite aggregates based on most of the laboratory-based empirical test methods. The results agree with findings from previous studies (Airey et al.Citation2008, Grenfell et al.Citation2012) that indicate that limestone has a better bonding property compared with granite aggregates. The results are also supported by the more fundamental surface energy-based methodology.
Several reasons may account for limestone aggregates better bonding properties in comparison with granite aggregates. Limestone aggregates are generally hydrophobic, and thus have higher affinity for bitumen than water leading to a lower possibility of stripping. Granite is an igneous rock formed from magma that contains a high silica and alumina content and is therefore generally hydrophilic. Consequently, asphalt mixtures containing granite aggregates are more likely to strip than limestone aggregates. The test results of this study support this quite well.
All three types of limestone (L1–L3) have quite low water sensitivity as shown by the 25% or less binder loss obtained for these aggregates in the five qualitative tests considered. Because of the lower water sensitivity, not all the tests were able to discriminate clearly between the limestone mixtures. For instance, the static immersion test was not sensitive enough to distinguish the differences between these three limestone aggregates. The BWT and the RBT provided better ranking of the limestone aggregates in relation to their bonding properties.
In contrast to the limestone mixtures, the three mixtures containing granite aggregates (G1–G3) exhibited comparatively higher water sensitivity. The percentages of binder loss were all very high (up to 90%) especially for the RBT and BWT. Furthermore, nearly all the tests could distinguish the differences between these three aggregates with the BWT and RBT being the most sensitive. The total water immersion test also provided reasonable reference to distinguish between the three granite aggregates.
The results indicate that bituminous mixtures containing limestone aggregates have better moisture resistance than granite aggregates. For this study, L2 limestone showed the best bonding properties while G3 granite performed the worst for most of the tests conducted.
5.2 DCA test – bitumen surface energy
Using Equation (1), the average contact angle values obtained from the DCA tests together with the surface energy components of the probe liquids (Table ) were used to determine the surface energy components of the binders. Table shows the summarised surface energy for the seven binders.
Table 4 Surface energy components (mJ/m2) of bitumen.
Some differences in surface energy components depending on binder type (grade or modification) could be seen. The Lifshitz van der Waals component for the softer 160/220 pen bitumen is lower (28.2 mJ/m2) than the stiffer 40/60 pen bitumen (30.6 mJ/m2). The corresponding Lifshitz van der Waals component for the modified binders ranged from 30.8 to 32.2 mJ/m2. The two unmodified binders appear to be neutral (negligible Lewis acid and Lewis base components) which is in contrast to the modified binders that exhibited significant Lewis base component. Given that the modified binders all contain 40/60 pen, the surface energy results show the effect of the anti-stripping additives makes the bitumen more basic. The total surface energy of the binders was similar except for the 160/220 pen binder which showed a relatively low surface energy.
5.3 DVS test – aggregate surface energy
Two key surface energy parameters were obtained by analysing the DVS sorption isotherms: SSA and the spreading pressure. Both parameters are useful inputs for the surface energy calculation models. In addition, SSA is used in the computation of one of the four compatibility ratios. Table provides the surface energy components for the tested aggregates obtained from the DVS software. Also shown in Table are the SSA values that were obtained using octane as the probe liquid. No easily discernible trends could be seen by looking at the individual surface energy components of the aggregates, hence the need for estimating bond energy parameters as discussed in the following.
Table 5 Surface energy components (mJ/m2) of aggregate.
5.4 Bond energy parameters
Surface energy components of the individual asphalt mixture constituents by themselves provide only limited insight into the moisture damage problem. Therefore, the aggregate surface energy components were used in combination with the surface energy components of the binders and water (Table ) to compute the dry bond strength (Figure ) as well as the work of debonding (Figure ) for each combination of aggregate and bitumen tested.
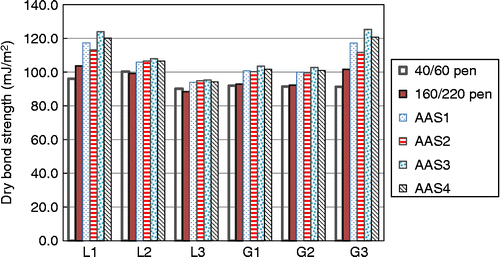
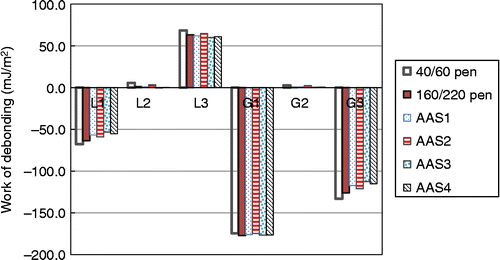
As previously indicated, dry bond strength represents the interfacial work of adhesion between bitumen and aggregate: a bigger value of dry bond strength suggests greater adhesion between the two materials in the absence of moisture. From Figure , for each aggregate type, a trend of increasing dry bond strength was observed when the unmodified binder (40/60 pen) is compared with the modified binder; the increase was highest in L1 (30%) and G3 (37%). The results in Figure also show that with the exception of the two aggregates L1 and G3, the dry bond strengths for the various aggregate–bitumen combinations are similar averaging about 98.37 mJ/m2. The dry bond strengths for L1 and G3 appear to be significantly higher compared with the rest of the aggregates, which suggest greater sensitivity of these aggregates to anti-stripping agents. These results suggest that for the bitumen mixtures considered in this study, the effects of both aggregate type and the type of binder and/or modification play an important role in aggregate–bitumen adhesion.
Figure shows the reduction in bond strength in the presence of moisture (work of debonding) for the various aggregate–bitumen systems. The magnitude of the work of debonding was found to be aggregate type dependent, as indicated by the cluster of data around each aggregate type, which suggests the physico-chemical properties of aggregates may play a fundamental and more significant role in the generation of moisture damage, than bitumen properties. The magnitude of work of debonding averaged 59.2 ± 5.44, 1.83 ± 2.28, 63.21 ± 3.05, 175.80 ± 1.04, 1.24 ± 1.10 and 120.84 ± 7.62, respectively, for aggregate L1, L2, L3, G1, G2 and G3. In the presence of moisture, mixtures with positive work of debonding are considered more stable than those with negative work of debonding. On this basis alone, mixtures comprising of aggregate L1, G1 or G3 that exhibited negative work of debonding would be expected to be less stable than the other three mixtures showing positive work of debonding. Furthermore, aggregate–bitumen combinations with smaller magnitudes of work of debonding are indicative of better moisture resistance. Again, on this basis alone, mixtures comprising of aggregate L1, G1 and G3 appear to be the least resistant to moisture for the various binders used. For the remaining aggregates, the reduction in bond strength is comparable for a given bitumen type, suggesting that aggregate effects might be more influential than binder effects. It is interesting to note the decrease in work of debonding for the modified binders (AAS1–AAS4) compared with the unmodified 40/60 pen. For instance, considering L1, the work of debonding decreased up to 25% from 68 mJ/m2 for the unmodified to values ranging from 53.3 to 58.9 mJ/m2 for the modified. This suggests that for majority of the aggregates considered, the work of debonding parameter is sensitive to the effects of the anti-stripping additives used. The results also suggest that the high dry bond strengths obtained for L1, G2 and G3 are mostly lost in the presence of water and illustrate the importance of considering both the wet and dry bonding of adhesion when evaluating moisture resistance of asphalt mixtures. This was the motivation for considering the compatibility ratios as discussed in the following.
5.5 Compatibility ratios
Based on an extensive field moisture damage performance versus laboratory surface energy, intrinsic adhesion study in the USA, a set of threshold values have been defined for the energy ratios in order to separate ‘good’ from ‘poor’ moisture damage performing aggregate–bitumen combinations (Little and Bhasin Citation2006). The threshold limits are 0.75 for ER1, 0.50 for ER2, 0.50 for ER3 and 0.35 for ER4. It must be noted that these threshold values are not absolute and therefore, in general, when evaluating mixtures for moisture sensitivity, aggregate–bitumen mixtures with higher compatibility ratio are preferred to those with lower ratios. Table shows the summary of the compatibility ratios together with the suggested threshold limits. Aggregate–bitumen combinations that classified as poor based on the threshold limits are in italic in Table . Some similarities and differences in predictions based on the compatibility ratios are apparent from Table . All the parameters identified G1, a granite, as the most moisture-sensitive mixture. In addition to G1, parameter ER2 identified most L2 and G3 mixtures as moisture sensitive. There was close agreement in the prediction of ER1 × SSA and ER2 × SSA.
Table 6 Compatibility ratios of aggregate–bitumen combinations.
From Table , the effect of several experimental factors including aggregate type, binder type and binder modification (anti-stripping agent) could be deduced. For the same aggregate type, each compatibility ratio was higher in the modified binder compared with the unmodified binder (40/60 pen). For example, considering aggregate L1 in Table , ER1 increased from 1.42 for the unmodified bitumen (40/60 pen) to between 1.92 and 2.33. Similar observations could be made for the other three compatibility ratios ER2, ER1 × SSA and ER2 × SSA. The results show that the anti-stripping agents improved moisture-damage resistance of the mixtures. Considering the unmodified binders, it can be seen from Table that compatibility ratios for the softer 160/220 pen binder were higher than the stiffer 40/60 pen binder which could be attributed to better wetting ability of the softer binder, and illustrates the complexity of the moisture damage problem. These results suggest that the surface energy parameters are sensitive to the positive effect of the anti-stripping agents on moisture resistance as well as to the stiffness properties of the unmodified binders. Therefore, the results of the surface energy tests could be used to evaluate the sensitivity of the empirical (qualitative) test methods considered in this study.
6. Comparison of test methods
6.1 Loose asphalt-coated mixture tests
Differences in moisture sensitivity of the various aggregate–bitumen mixtures were observed based on the test type. Figure shows a depiction of how four tests (static immersion test, RBT, BWT and total water immersion test) out of the five tests considered in this study compare in terms of sensitivity to aggregate and the two unmodified binders. A test was considered sensitive if it can distinguish between the various aggregates and/or the different bitumen used. The ultrasonic method was not shown for obvious reasons (Figure ). The results show that for binders considered, the BWT and the RBT appear to be the most sensitive. The two tests similarly ranked the mixtures containing limestone aggregate as generally better (higher retained bitumen coating) than those containing granite mixtures in most cases which agrees with field performance of these aggregate. The static immersion test and the ultrasonic tests appear to be the least sensitive tests for evaluating moisture sensitivity of loose bitumen-coated aggregate mixtures for the materials considered in this study.
6.2 Comparison of RBT results with surface energy parameters
As indicated in Section 6.1, the RBT was found to be one of the most sensitive tests for evaluating aggregate–bitumen bond in the presence of moisture. The RBT is a qualitative test and, therefore, does not allow a fundamental understanding of the moisture damage mechanism. Therefore, data from the more fundamental surface energy-based measurements (Table ) obtained for various aggregate–bitumen combinations were used in an attempt to verify whether the sensitivity of the RBT observed in this study was supported by fundamental physico-chemical properties of the constituent aggregate and bitumen.
A mixture is considered moisture resistant if at the end of the RBT, 95% of the test sample remain coated with bitumen. From Figure , all the limestone mixtures exhibited 95% or more coated aggregate at the end of the 72-h test. In contrast, all the granite mixtures had < 95% of their mixtures coated with bitumen after undergoing the RBT. The results are in general agreement with the compatibility ratios (especially ER1) obtained from surface energy tests (Table ) which also show that the most resistant mixtures were the limestone mixtures while the least resistant mixtures were the mixture containing granite. It should be noted that because of the qualitative nature of the RBT criteria, namely that a mixture with bitumen coverage >95% is considered a pass while that with < 95% bitumen coverage is considered a fail, the test may not be very sensitive for marginal mixtures that are neither very moisture resistant nor very moisture susceptible, as compared to the surface energy approach. The results show that the surface energy-based method is in general agreement with the RBT (in terms of best and worst) but is also more sensitive. The results of the study provide some theoretical basis that appears to suggest that the sensitivity of the qualitative RBT observed in this study is supported in part by the physico-chemical properties of aggregate and bitumen used. Thus, the tests may be useful for evaluating the moisture sensitivity of asphalt mixtures. No previous studies have reported a link between the RBT (or any of the loose bitumen-coated aggregate moisture sensitivity test) and surface energy of asphalt mixtures.
7. Conclusions
The following conclusions were reached based on the results presented in this study:
Bituminous mixtures containing limestone aggregates have better moisture resistance than granite aggregates based on results from loose bitumen-coated moisture sensitivity tests.
For unmodified mixtures, stiffer binder (40/60 pen) provides better moisture resistance compared with softer binder (160/220), based on loose bitumen-coated moisture sensitivity tests.
Large differences in moisture sensitivity of the mixtures were observed based on the test method used: the BWT and the RBT were the most sensitive while the static immersion test and the ultra-sonic test were the least sensitive. The results for the total water immersion test had mixed success.
Ranking of the mixtures by RBT was in general agreement with those based on surface energy-based methods, especially for the ‘best’ performing and ‘worst’ performing mixtures, suggesting that the loss of adhesion at the aggregate–bitumen interface is a major mechanism of failure that occurs during the RBT.
The magnitude of the work of debonding in the presence of water was found to be aggregate type dependent which suggests that the physico-chemical properties of aggregates may play a fundamental and more significant role in the generation of moisture damage, than bitumen properties.
The five loose bitumen-coated moisture sensitivity tests considered in this study are qualitative. Therefore, future work comparing results of this study, especially the RBT, with performance testing such as the saturation ageing tensile stiffness test is recommended.
References
- Ahmad, N., 2011. Asphalt mixture moisture sensitivity evaluation using surface energy parameters. Dissertation (PhD). University of Nottingham, Nottingham.
- Airey, G.D. and Choi, Y.-K., 2002. State of the art report on moisture sensitivity test methods for bituminous pavement materials. Road Materials and Pavement Design, 3 (4), 355372.
- Airey, G.D., et al., 2008. The influence of aggregate, filler and bitumen on asphalt mixture moisture damage. Construction and Building Materials, 22 (9), 2015–2024.
- Andersland, O.B. and Goetz, W.L., 1956. Sonic test for evaluation of stripping resistance in compacted bituminous mixtures. Proceedings of the Association of Asphalt Paving Technologists, 25, 148–175.
- Bhasin, A., 2006. Development of methods to quantify bitumen–aggregate adhesion and loss of adhesion due to water. Dissertation (PhD). Texas A&M University, College Station, TX.
- Bhasin, A., et al., 2006. Limits on adhesive bond energy for improved resistance of hot-mix asphalt to moisture damage. Transportation Research Record: Journal of the Transportation Research Board (1970), 3–13.
- Christensen, J.H., et al., 2007. Regional climate projections. In: S.Solomon, D.Qin, M.Manning, Z.Chen, M.Marquis, K.B.Averyt, M.Tignor and H.L.Miller, eds. Climate change 2007: the physical science basis. Contribution of working group I to the fourth assessment report of the Intergovernmental Panel on Climate Change. Cambridge: Cambridge University Press, 849–926.
- Grenfell, J., et al., 2012. Optimising the moisture durability SATS conditioning parameters for universal asphalt mixture application. International Journal of Pavement Engineering, 13 (5), 433–450.
- Hubbard, P. and Shuger, L.W., 1938. Adhesion of asphalt to aggregate in presence of water. Highway Research Board Proceedings, 18 (1), 238–249.
- Hunter, E.R. and Ksaibati, K., 2002. Evaluating moisture susceptibility of asphalt mixes. Laramie, WY: University of Wyoming.
- Hveem, F., 1943. Quality tests for tsphalt: a progress report. Proceedings of the Association of Asphalt Paving Technologists, 15, 111–152.
- Jimenez, R.A., 1974. Testing for debonding of asphalt from aggregates. In: Transportation Research Record 515. Washington, DC: Transportation Research Board, National Research Council, 1–17.
- Little, D.N. and Bhasin, A., 2006. Using surface energy measurements to select materials for asphalt pavement. NCHRP project 9–37, final report.Washington, DC: Transportation Research Board, National Research Council.
- Lottman, R.P., 1978. NCHRP report 192: predicting moisture-induced damage to asphaltic concrete. Washington, DC: Transportation Research Board, National Research Council.
- Meehl, G.A., et al., 2007. Global climate projections. In: S.Solomon, D.Qin, M.Manning, Z.Chen, M.Marquis, K.B.Averyt, M.Tignor and H.L.Miller, eds. Climate change 2007: the physical science basis. Contribution of working group I to the fourth assessment report of the Intergovernmental Panel on Climate Change. Cambridge: Cambridge University Press.
- Nicholson, V., 1932. Adhesion tension in asphalt pavements, its significance and methods applicable in its determination. Proceedings of the Association of Asphalt Paving Technologists, 3, 28–49.
- Solaimanian, M., et al., 2003. Test methods to predict moisture sensitivity of hot-mix asphalt pavements. In: Moisture sensitivity of asphalt pavements, National Seminar, San Diego. Washington, DC: Transportation Research Board.
- Thelen, E., 1958. Surface energy and adhesion properties in asphalt–aggregate systems. In: Highway Research Board, Bulletin 192. Washington, DC: National Research Council, 63–74.
- Vuorinen, M. and Hartikainen, O.-K., 2001. A new ultrasonic method for measuring stripping resistance of bitumen on aggregate. Road Materials and Pavement Design, 2 (3), 297–309.