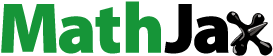
ABSTRACT
In this study, samples of cold in-place recycling mix (CIR) with steel fibres were manufactured and heated by electromagnetic induction. Heating was conducted to accelerate the curing process and make the CIR mixtures more competitive against other less-sustainable road materials. Heating can also be applied after years of service life to reduce the viscosity of the binder and make it flow through cracks, healing damage. Cylindrical Marshall samples were heated by induction for different durations immediately after production and their mechanical performance was assessed over different curing periods. In addition, 3-point bending healing tests were carried out on cured semicircular samples. The results showed that the remaining water content in samples heated for 180 s was lower than in control samples (not heated) after 21 days. The Marshall stability and flow after compaction increased by 147% and 144%, respectively, when heating was applied for 240 s. Healing tests produced encouraging results with strength recoveries higher than 50%.
1. Introduction
One of the main objectives of highway administrations is the preservation and maintenance of road networks to provide the highest quality of service. Most of these road networks are composed of light-traffic rural roads made with bituminous wearing courses. For the preservation and maintenance of these types of pavements, an effective technique is the in-place recycling of old wearing courses with bitumen emulsion (Asphalt Institute Citation1983, Citation1989, National Academies of Sciences, Engineering, and Medicine Citation2011, Nivedya and Veeraragavan Citation2017).
Cold in-place recycling (CIR) is a surface recycling technique in which the upper part of the aged asphalt pavement is milled and mixed with bitumen emulsion giving rise to a stabilised base course. The upper part of the milled pavement is called reclaimed asphalt pavement (RAP) (Asphalt Institute Citation1983, Citation1989, Kishore Kumar et al. Citation2008, Nivedya and Veeraragavan Citation2017).
Using CIR mixtures is an economical method for pavement preservation and maintenance. CIR mixtures also provide many environmental benefits such as conservation of non-renewable resources, reduction of emissions, reduction of fuel consumption, and elimination of generated materials for disposal (National Academies of Sciences, Engineering, and Medicine Citation2011, Nivedya and Veeraragavan Citation2017).
However, the implementation of CIR layers also has a major structural disadvantage. The strength of a CIR layer is usually very limited in the short term, because it needs a curing period to increase the layer low initial stiffness (Jenkins et al. Citation2007, Ebels Citation2008, Pérez et al. Citation2013). During this period, the CIR layer loses water, while a densification process takes place, produced by traffic loads (secondary compaction). As a result, the strength of the CIR layer increases. The low initial stiffness in the CIR layer could lead to great difficulties to lay and compact a hot mix asphalt overlay, as machinery can overload it. Also, immediately after it has been opened to heavy traffic, significant permanent deformation and failure may occur, creating an irreversible negative effect on the structural and functional state of the CIR layer (Pérez et al. Citation2016, Tabaković et al. Citation2016, National Academies of Sciences, Engineering, and Medicine Citation2017, Nivedya and Veeraragavan Citation2017, Pinto and Buss Citation2018, Roos Citation2018, Wang et al. Citation2018).
Many studies have been conducted with the aim of minimising the above problems by increasing the low initial stiffness and resistance of CIR mixtures to permanent deformation. There are published studies on different additives, such as cement (Oruc et al. Citation2007, Thanaya Citation2007, Al-Hdabi et al. Citation2014a, Citation2014b), waste fly ash (Al-Hdabi et al Citation2014b), binary blended cementitious filler (Dulaimi et al. Citation2016, Citation2017), as well as modified asphalt emulsions (Chávez-Valencia et al. Citation2007). Despite this extensive research, up to the present time, the problems relating to the curing period of CIR mixtures have not been addressed. Therefore, developing a new procedure to increase the early life strength of these materials would be a great contribution to the CIR mixture research.
It is possible to use induction heating to accelerate the curing process of CIR mixtures. The process involves adding conductive particles to the asphalt mixture and subjecting it to an alternating electromagnetic field with frequencies in the order of kilohertz. This induces electrical micro-currents that increase the temperature by the Joule principle. When the heat energy diffuses into the asphalt mixture, it increases the temperature of the embedding bitumen, reducing its viscosity and accelerating its flow through cracks and cavities. This technique has been successfully used to heal damage in hot asphalt mixtures and recover a considerable part of their initial resistance (García et al. Citation2009, Citation2011a, Citation2011b, Citation2012, Gómez-Meijide et al. Citation2016).
The feasibility of this technology has been proven at laboratory and full-scale through projects, such as HEALROAD, a 1.2M€ EU-funded investigation, which proved the concept at full-scale after exhaustive laboratory work and the construction, accelerated damage and heating/healing of a 400 m trial test section at BASt facilities, in Germany. The first full-scale induction machine has been also manufactured obtaining satisfactory on-site results.
Nevertheless, no published induction heating research or technical experiences have been found for the accelerated curing of CIR mixtures by induction heating.
The objectives of the present study are as follows:
Investigate the feasibility of using induction heating in CIR mixtures to reduce their water content, increase their strength, and consequently shorten their curing process. For this purpose, cylindrical Marshall samples were heated by induction for 0, 60, 120, 180, and 240 s after production and their mechanical performance was assessed after 0, 1, 3, 7, and 21 days.
Study the feasibility of applying induction heating to heal damage in CIR materials and extend their service life. For this objective, semicircular samples were split and healed by different heating durations, comparing the strength before and after applying the induction treatment.
2. Description of the proposed technology
In order to make the RAP material heatable by electromagnetic induction, it is necessary to introduce in it conductive metal fibres. These can be spread by a spreader equipment which is followed by conventional water and bitumen emulsion tanks and the conventional recycling equipment. The fibres are mixed with water, bitumen emulsion and the RAP material inside the recycling equipment. The recycled material is transported to the rear of the equipment where is extended and pre-compacted. At the moment, first light compaction of the material is carried out by rollers and then, the induction heating treatment is applied across the lane width by several passes of the induction heating equipment before second rollers compaction is performed. After several years of service, induction heating can be newly applied to seal cracks and extend the service life of the CIR layer, reducing maintenance and rehabilitation costs.
The main challenge to apply the induction heating is the design and manufacturing of a full-scale portable induction machine with power enough to produce satisfactory results. The first prototype of these machines was already produced and tested during the HEALROAD project mentioned above, showing great potential to increase fast the temperature of the road ().
3. Experimental method
3.1. Materials
The RAP was obtained from the milling of the upper part of highway asphalt pavements. shows its size distribution, obtained according to Standard UNE-EN-12697-2 (AENOR Citation2007a), and the gradation limits provided by the Spanish Specifications for Highway Maintenance (PG-4) for CIR mixtures (Ministerio de Fomento Citation2002).
The bulk specific density of RAP obtained according to UNE-EN 1097-6 (AENOR Citation2006a) was 2.56 g/cm3 and the residual bitumen content was 4.8%. In addition, the recovered asphalt has a ring and ball temperature of 64.4°C according to the UNE-EN 1426 (AENOR Citation2006b) and a penetration of 20.32 × 10−1 mm according to UNE-EN 1427 (AENOR Citation2006c).
Steel wool fibres () with diameters between 16 and 72 μm and with lengths between 0.15 and 5 mm were added to the RAP. The material used for the steel wool was low-carbon steel, with a bulk specific density of 7 g/cm3. The volumetric content of steel wool used in the present research was 0.6% of the total CIR mixture. Fibres used as additives in other types of mixtures can have large absorption coefficients, e.g. organic, cellulosic, or mineral fibres used in stone mastic asphalt (SMA) to retain bitumen after mixing (Nicholls Citation1998). However, in this study, the fibres do not have such a function. Instead, they will be heated under the action of an induced electromagnetic field. For this reason, steel fibres with a negligible water absorption coefficient were used.
The bitumen emulsion used for the manufacturing of the samples was C60B5 REC (Ministerio de Fomento Citation2014), which corresponds to a cationic slow-setting emulsion with 60% bitumen content and 100-pen grade bitumen.
3.2. Manufacturing of samples and application of induction heating
For the present investigation, three types of samples were manufactured:
Cylindrical samples for immersion-compression tests: Dry RAP was mixed with water, bitumen emulsion and steel fibres at room temperature (20°C) for 2 min. Then the mixture was compacted into 101.6 mm diameter × 101.6 mm height cylindrical specimens by applying static compaction, according to Spanish Standard NLT-162 (Ministerio de Obras Públicas y Transportes Citation2002c). This compaction involved a preload of 1 MPa for 1 min followed by a 2 min stage, during which the load was increased at a constant rate until 21 MPa was reached. This load was maintained for 2 min before reducing the load linearly until 0 kN was reached. Finally, following PG-4 specifications (Ministerio de Fomento Citation2002), the samples were cured for 3 days at 50 ± 2°C.
Cylindrical samples for Marshall tests: In this case, the mixing was performed under the same conditions explained above but following Spanish Standard NLT 159 (Ministerio de Obras Públicas y Transportes Citation2002a); a compaction of 50 blows on each side of the specimens by a Marshall hammer was applied. Hence, 101.6 mm diameter × 57 mm height samples were obtained. As these samples were used to assess the feasibility of induction heating to accelerate the curing process, different series were heated after compaction for 0, 60, 120, 180, and 240 s and then cured into a climatic cabinet at 20 ± 2°C for 0, 1, 3, 7, and 21 days.
Semicircular samples for induction-healing tests: Semicircular samples were obtained by manufacturing first Marshall samples, as explained in the previous case, which were then cut diametrically by a radial saw suitable for concrete and stone materials. Additionally, a notch of 4 mm width and 10 mm depth was cut at the midpoint of the diameter to ensure that a vertical crack is produced from that point during the 3-point bending tests. As healing treatments are supposed to be applied after long service durations, these samples were cured for 3 days in an oven at 50 ± 2°C, following PG-4 specifications (Ministerio de Fomento Citation2002).
3.2.1. Optimum water content. Modified proctor test
According to the Asphalt Recycling and Reclamation Association (Citation2003), there is no mixing design procedure for CIR that is universally accepted. Instead, several procedures are available to obtain the optimum emulsion and water contents (Tebaldi et al. Citation2014, Jenkins and Collings Citation2017, Mogadan and Mollashahi Citation2017). For this investigation, the Spanish specification for highway maintenance (PG-4) for CIR mixtures was used (Ministerio de Fomento Citation2002).
The RAP was heated at 60°C during 24 h in order dry it and to homogenise the water content of the samples, according to PG-4 specifications. Once the material was cooled back to ambient temperature (20°C), the RAP maximum dry density and the optimal fluids content (OFC) were obtained by Modified Proctor compaction tests, according to Standard UNE-EN-103501(AENOR Citation1994). According to the Spanish PG-4 specification, to manufacture the specimens of CIR, the water content that must be added to the RAP samples is obtained by means of the following equation (Ministerio de Fomento Citation2002):(1)
(1) where AWC is the water content added to the RAP, OFC is the optimum fluids content, and EC is the emulsion content added to the RAP.
3.2.2. Optimum binder content. Immersion-compression test
In the Spanish PG-4 specifications (Ministerio de Fomento Citation2002), the job mix formula of CIR mixtures must be verified by assessing the effect of water on the unconfined compression strength of the samples. This assessment was carried out according to the Spanish Standard NLT-162 (Ministerio de Obras Públicas y Transportes Citation2002c), somehow derived from the French Duriez test (NF P98-251) (Association Française de Normalisation Citation2004). Ten 101.6 mm diameter × 101.6 mm height cylindrical specimens were manufactured. Five specimens were immersed in water at 49°C for 4 days, and the other five were maintained at room temperature without being submerged in water. They were later subjected to unconfined compression tests according to the NLT-161 standard (Ministerio de Obras Públicas y Transportes Citation2002b), and the average value of the strength in each group was determined. The retained strength ratio (RSR) was obtained as follows:(2)
(2) where UCSwet is the unconfined compression strength of the group immersed in water at 49°C (MPa) and UCSdry the unconfined compression strength of the group that was not submerged. According to Spanish specifications (PG-4), this ratio must be higher than 75% in CIR mixtures employed in road pavements with T1 and T2 traffic categories (). In roads with T3 and T4 traffic categories, this ratio must be higher than 70%. Furthermore, PG-4 defines the minimum UCS as 3.0 MPa for the dry group and 2.5 MPa for the wet group for T1 and T2 traffic categories. In the case of roads with traffic categories T3 and T4, the minimum UCS is 2.5 MPa for the dry group and 2.0 MPa for the wet group ().
Table 1. Immersion-compression test results and PG-4 specifications for assessing the effect of water on CIR mixtures.
In Spain, CIR mixtures can be used as a binder course or as a base course, with a hot mix asphalt layer on top. Induction treatment can be used in these cases after compacting the CIR layer and before laying down the wearing HMA. At a later stage, when the pavement is cracked, it was explained that the same technology can be applied to heal the material.
3.2.3. Volumetric properties
After the optimum emulsion content, added water and bitumen content were selected according to the procedure explained in sections 3.2.1 and 3.2.2. One series of three samples was mixed and compacted according to the immersion-compression procedure. Another series of three samples was manufactured following the Marshall procedure. Immediately after compaction, the specimens of the two series were subjected to a 3-day curing process in an oven at 50°C. Then, the air and remaining water voids were calculated in each series according to the standard UNE-EN 12697-8 (AENOR Citation2007d) as follows:(3)
(3) where Va is the air and remaining water content, ρb is the bulk specific density obtained by the saturated surface dry water displacement method according to the standard UNE-EN 12.697-6 (AENOR Citation2007c), and ρm is the maximum specific density according to the standard UNE-EN 12.697-5 (AENOR Citation2007b) measured in loose mixtures.
3.2.4. Marshall test
To evaluate the effect of induction heating on the curing and resistance of CIR mixtures, the Marshall test procedure was carried out according to a modified version of the Spanish Standard NLT 159 (Ministerio de Obras Públicas y Transportes Citation2002a). Different series of three cylindrical samples of 101 mm diameter and 57 mm height were manufactured, subjected to different induction heating durations (0, 60, 120, 180, and 240 s), and stored in a climatic cabinet at 20 ± 2°C for 0, 1, 3, 7, and 21 days before the testing. After these curing durations, the specimens were tested at 20 ± 2°C and the Marshall stability (S, kN) and flow (F, mm) were obtained.
3.2.5. Water content of Marshall samples
After compaction, the Marshall samples were left for 1 h inside the moulds before demoulding. The application of induction heating to the samples was carried out immediately after demoulding. All samples were weighed immediately before (Pb) and after (Pa) the application of induction heating durations (0, 60, 120, 180, and 240 s) to obtain the weight of water evaporated. The water content after induction treatment (wi) was obtained using the following expression:(4)
(4) where Piw is the initial weight of water inside the sample after compaction and demoulding.
As mentioned above, after induction treatment, the samples were cured at 20 ± 2°C into a climatic cabinet for 0, 1, 3, 7, and 21 days. At the end of each curing time, the samples were again weighed immediately before Marshall testing (PbM) to obtain the weight of water evaporated during the curing time. The water content after curing (wc) of the samples was obtained using the following expression:(5)
(5) Nevertheless, it must be noted that at 0 days of curing time, it was not possible to carry out the Marshall tests on samples immediately after induction heating, because first, these had to be cooled down into a climatic cabinet for 2 h at 20 ± 2°C before testing. During such cooling time at high temperature, the samples heated by induction continued losing water and, consequently, the water content before testing was lower even for samples tested at day 0. The samples not heated by induction were also cooled down into the climatic cabinet for 2 h at 20 ± 2°C before testing at day 0 to have the same curing period.
3.2.6. Induction healing assessment. Semicircular 3-point bending test
Semicircular 3-point bending tests were carried out to evaluate the effect of induction heating on the healing of CIR mixtures (). The tests specimens were preconditioned at −20 ± 2°C for 24 h before testing. Two supporting metallic pieces at the straight (bottom) edge and a loading steel ball at the midpoint of the semicircular arch comprised the test setup. The spacing between the two metallic supports was 60 mm (). The test was carried out inside a climatic chamber at −20 ± 2°C at a loading rate of 5 mm/min.
The feasibility of using induction heating for the healing of CIR bituminous mixtures was analysed in 3 steps: (1) semicircular samples were split by 3-point bending test at −20 ± 2°C registering the initial maximum load resisted, Fo; (2) both parts were gently placed together at ambient temperature and 30, 60, 90, 120, 150, and 180 s of induction heating were applied; and (3) 3-point bending test was repeated at −20 ± 2°C obtaining a new maximum load, (Fa). The healing level reached for each CIR mixture sample (Sh) was expressed as the ratio between the maximum loads registered before and after the healing treatment, as follows:(6)
(6)
4. Results
4.1. Mix design
The modified Proctor test showed a maximum dry density of 1.94 g/cm3 for the optimum water content of 5.75%. Hence, this amount of water was fixed for the manufacturing of all samples, including water from asphalt emulsion and added water.
The binder content for CIR specimens was selected according to Spanish PG-4 specifications (Ministerio de Fomento Citation2002) and based on the immersion-compression test. presents the five CIR mixtures manufactured with different residual bitumen contents (1.50%, 1.75%, 2.00%, 2.25%, and 2.50%) and their corresponding added water contents obtained by EquationEquation 1(1)
(1) . The results of the dry and wet unconfined compression strengths (UCSdry and UCSwet) as well as the RSR obtained by each mixture can also be found in the table.
It can be observed that all mixtures met the requirement of RSR > 75% for traffic categories T1 and T2 specified in (annual average daily heavy traffic (AADHT) > 2000 and ≥ 200, respectively) but only samples 1, 3, and 4 met the UCSdry and UCSwet requirements for categories T3, T4, and shoulders (AADHT < 200 and 50, respectively). Among them, mixture number 3 was selected as having the best combination of compression strength and resistance to water damage (UCSdry = 2.61 MPa, UCSwet = 2.30 MPa, and RSR = 88.25%). Such mixture, used for all tests included in the present investigation, had a residual bitumen content of 2.0%, bitumen emulsion content of 3.33%, and an added water content of 1.92%. This CIR mixture can be used a as a binder course or as base course of traffic categories T3, T4, and shoulders ().
4.2. Volumetric properties
First, it was checked that after 3 days, the samples were practically dry at 50°C. Three samples were cured for 2 additional days, registering a negligible average weight loss of (0.02%).
Results showed that the average bulk specific density of immersion-compression samples compacted by static compaction was 2.24 g/cm3 and the average maximum specific density was 2.45 g/cm3. Substituting these values into Equationequation 3(3)
(3) , an air void content of 8.57% was obtained. This air void content was achieved after curing the samples for 3 days at 50°C. Therefore, it includes the voids initially filled with water and air before curing.
For Marshall samples, the results showed an average bulk specific density of 2.11 g/cm3, average maximum specific density of 2.45 g/cm3, and an obtained air void content of 13.86%, which correspond to an open mixture. The differences between the static compaction and Marshall hammer compaction indicate that when using the Marshall hammer, the water was not properly drained, reducing the compaction level of the specimens.
However, the results are similar to those obtained by Babagoli et al. (Citation2016) for Marshall CIR samples with a 3% of slow-setting emulsion and 3.8% of water compacted with 50 blows per face. In this case, the bulk specific density was 2.08 g/cm3 and the air void content was 12.5%. Similar results of air voids ranging between 13% and 13.6% and bulk specific densities ranging between 2.073 and 2.087 g/cm3 were obtained by Cross (Citation1999) in CIR mixtures compacted using a Marshall hammer with 50 blows per side. In this regard, the fact that the samples after compaction have a high water content is appropriate for the investigation, as high water content is useful to study the feasibility of induction heating to accelerate the curing period.
4.3. Water content
First, a series of three samples was manufactured and weighed before and after compaction (right before demoulding). Results showed an average water loss of 1.83 g due to this process and before applying any induction heating. This value was subtracted from the initial design water weight (25.8 g) to calculate the initial weight of water inside the sample (Piw) before induction heating.
presents the average water loss and the average water content measured for all samples immediately after induction heating (for 0, 60, 120, 180, and 240 s) obtained by Equationequation 4(4)
(4) . Considering that the initial design water weight was 25.8 g, then Piw = 25.8 g − 1.83 g = 23.97 g (2.83% by weight of total CIR mixture).
Table 2. Water content immediately after induction heating (%).
In , it can be observed that after 180 and 240 s of induction heating, the evaporated water was already greater than 10 g, which is more than one third of the initial amount, and the remaining water content was less than 2.00%. The Tukey-Kramer multiple comparison analysis showed that at 95% confidence level, there were significant differences between all water content pairs of means except for the remaining water contents of 1.59% and 1.58%. This implies that 180 and 240 s of induction heating durations produce the same remaining water content and that all other induction heating durations produce different remaining water contents.
On the other hand, shows the water content measured after 0, 1, 3, 7, and 21 curing days obtained by Equationequation 5(5)
(5) . As explained before, at 0 curing day (control mixtures), the water content was measured 2 h after induction heating. Thus, at 0 curing day, there are different water content values in and .
As can be observed in , after 1, 3, 7, and 21 curing days, the samples reduced progressively the water content. The water content reduces remarkably fast during the first week, reaching very similar values of less than 0.5% after 21 days. This was not observed for the control mixtures (which were not heated by induction), whose water content remained between 0.5% and 1%. In addition, it can be observed that mixtures heated for 180 and 240 s already reached a steady-state water content below 0.5% during the first curing day. This steady-state water content was verified because the Tukey-Kramer multiple comparison analysis showed that at 95% confidence level, there were no significant differences between all pairs of remaining water content means corresponding to 1, 3, 7, and 21 days. Thus, the water content obtained 2 h after heating the samples for 180 and 240 s (0.55% and 0.59%, respectively) was already lower than the steady-state content reached by the non-heated control mixtures after 21 days. By applying 120 s of induction heating, the water content after 2 h of treatment was higher (0.93%) but still in the same order of magnitude obtained by the control mixtures after 3 days.
4.4. Marshall parameters
4.4.1. Marshall stability
(a) shows the values of Marshall stability versus the curing time in days (0, 1, 3, 7, and 21 days) for five different heating induction durations applied after manufacturing of samples (0, 60, 120, 180, and 240 s). It was expected that although the drying level was different at early stages after applying induction heating, the long-term-curing mechanical performance was approximately the same. However, as shown in , the application of induction heating produced, in general, increases in the Marshall stability at any curing time. In other words, the curing process becomes more effective when a longer heating time is applied after manufacturing. A difference of approximately 7 kN between samples non-heated and heated for 240 s was maintained even when the samples reached a steady-state stability after 21 days (the measured stabilities were 10.40 kN and 17.28 kN, respectively). This steady stability was verified because the Tukey-Kramer multiple comparison analysis showed that at 95% confidence level, there were no significant differences between all pairs of stabilities corresponding to 1, 3, 7, and 21 days. (b), shows that there is a linear correlation between the stability at 21 days and the induction heating time.
Figure 7. (a) Variation of Marshall stability values with curing time; (b) correlation between stability after 21 days and induction heating time after manufacturing.
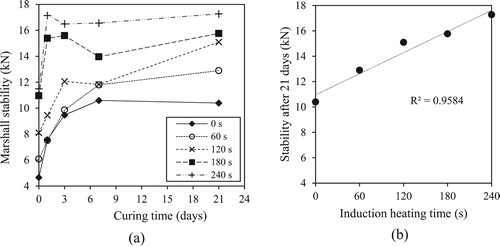
summarises the percentage increase in stability at each curing time, compared to the control (non-heated) samples. The results show that induction heating produced significant increases, especially at early curing stages (0 and 1 day after manufacturing) and when at least 120 s heating were applied. Hence, the stability at day 0 (2 h after induction heating) increased by 74.0%, 135.4%, and 146.8% when heating of 120, 180, and 240 s was applied (from 4.66 kN to 8.11 kN, 10.97 kN, and 11.50 kN, respectively). Moreover, it must be highlighted how the stability at day 0 (2 h after induction heating) after applying 180 s induction heating exceeded the stability reached by the control mixtures after 21 days.
Table 3. Increase in Marshall stability at each curing time by applying different induction heating durations.
In addition, the steady-state stabilities are reached in a shorter time when the material is heated after manufacturing. Hence, the stability of the control samples (not heated) increased until 7 days after manufacturing, while for the case of mixtures heated for 180 and 240 s, such steady state was reached in less than 1 day. Therefore, induction heating makes the curing of CIR materials not only more effective but also faster.
For comparison, it is worth mentioning that Babagoli et al. (Citation2016) compacted 63 mm high CIR samples and left them in the moulds for 24 h at 60°C and another 24 h at room temperature. Finally, the samples were extracted from the moulds and left for 5 days at room temperature. According to the authors, after such curing process, the Marshall stability obtained at room temperature was 8.2 kN. For the case of the present research, by applying 120 s of induction heating, the stability (day 0) of the 57 mm high samples was 8.11 kN. By applying a size correction factor of 1.19 specified by the Spanish Standard NLT 159, the result is 9.65 kN, which is 19% higher than the reference value.
4.4.2. Marshall flow
(a) shows the Marshall flow values obtained at each curing time and when different induction heating treatments were applied after manufacturing the samples. It was found that, in general, induction treatment tended to increase the flow at any curing time. Again, this effect was more significant at the early curing stages. Hence, the flow 2 h after induction treatment (0 days of curing time) could be increased by 86% with just 120 s and 144% with 240 s. (b) shows that there is a linear correlation between the flow at 21 curing days and the induction heating time.
Figure 8. (a) Variation of Marshall flow values with curing time; (b) correlation between flow after 21 days and induction heating time after manufacturing.
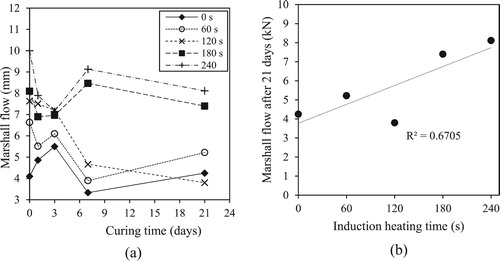
On the contrary, in (a), a tendency of decreasing the Marshall flow values with the increase in curing time can be observed. However, this tendency was not always verified. For example, for 180 and 240 s induction heating durations, the Tukey-Kramer multiple comparison analysis showed that at 95% confidence level there were no significant differences between all pairs of flow means corresponding to 0, 1, 3, 7, and 21 days. For 120 s, there were only significant differences between the flow mean corresponding to 21 days and the flow means corresponding to 0, 1, and 3 days.
4.4.3. Marshall ratio
(a) shows the values of the Marshall ratio (MR) (kN/mm) obtained by dividing the stability values between the flow values (full dots). The MR values are a function of the induction heating durations (0, 60, 120, 180, and 240 s) for 0, 1, 3, 7, and 21 curing days. Some authors (Cross Citation1999, Pérez et al. Citation2012, Xu et al. Citation2016) used the MR as an indicator of rigidity of asphalt concrete mixtures. (b) shows the stability versus flow values for the induction heating durations.
Figure 9. (a) Effect of induction heating time on MR and remaining water content after induction (wi); (b) relation between values of Marshall parameters.
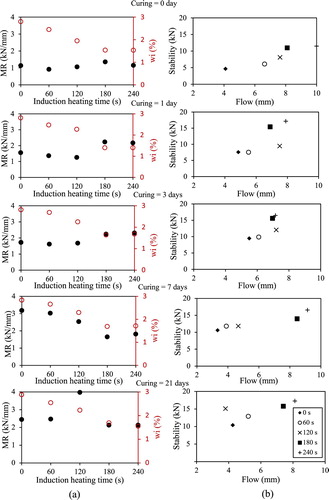
As can be observed in (a), there is no clear correlation between the MR and induction heating time. This was confirmed because the Tukey-Kramer multiple comparison analysis showed that at 95% confidence level, for 0, 1, 3, 7, and 21 curing days, there were no significant differences between all pairs of MR corresponding to 0, 60, 120, 180, and 240 s. This means that the increase in flow values observed previously when applying induction heating was not related to the loss of stiffness.
On the other hand, in (b), it can be observed that for 0, 1, 3, 7, and 21 curing days, both parameters (stability and flow) increase as the induction heating time increases. This effect may be produced by the reactivation of the aged bitumen in RAP, reducing its viscosity and increasing its flow capacity to blend it with the virgin bitumen of the emulsion. Therefore, this reactivation produces a more effective aggregate coating and a better aggregate–binder adhesion. Consequently, the CIR mixture becomes stronger. This is a beneficial effect because it allows the use of the material at higher loading levels without producing plastic deformations.
Another explanation to these results might be that induction heating may cause oxidation and loss of volatile fractions within the bitumen of the emulsion. This might produce short-time aging and therefore bitumen hardening. Consequently, the CIR mixture would become stiffer. Nevertheless, in general, the increase in both Marshall parameters in does not produce an increase in MR (rigidity). In addition, García et al. (Citation2011a) used the gel permeation chromatography method to validate that there was no ageing of bitumen during the induction heating process. The authors demonstrated that the molecular weight distribution of bitumen did not change because of the induction heating process. Because of such reasons, this hypothesis does not seem completely feasible.
(a) shows the MR as a function of the curing days (0, 1, 3, 7, and 21 days) for 0, 60, 120, 180, and 240 s of induction heating durations (full dots). On the other hand, (b) shows the stability versus flow values for the same conditions.
Figure 10. (a) Effect of curing time on MR and remaining water content after curing (wc); (b) relation between values of Marshall parameters.
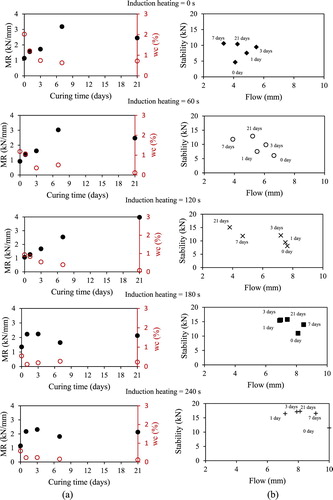
As can be observed in (a), a stronger correlation was found between the MR values and curing days. In (a), for 0, 60, and 120 s of induction heating time, the rigidity values increase with the curing days. The strongest correlation was found for 120 s induction heating time. In this case, the MR was 1.06 kN/mm at 0 days and 3.97 kN/mm at 21 days, respectively, which indicates an increase of up to 274.5%. However, for 180 and 240 s, MR tends to stabilise. These observations were confirmed as the Tukey-Kramer multiple comparison analysis showed that at 95% confidence level, for induction heating durations of 0, 60, and 120 s, there were significant differences between pairs of MR corresponding to 0, 7, and 21 days. For heating durations of 180 and 240 s, there were no significant differences between all pairs of MR.
On the other hand, in (b), in general, there is a tendency to decrease the flow as the curing days increase. This tendency is more perceptible for 60 and 120 s of induction heating durations. This means that the decrease in flow values with the passing of curing days can be related to the increase in stiffness.
4.5. Relation between water content, MR, and Marshall parameters
(a) shows the relations between the remaining water content (wi) immediately after induction heating durations with MR for 0, 1, 3, 7, and 21 curing days (empty dots). As mentioned before, in (a), MR (rigidity) does not vary significantly. (b) shows the relations between stability and flow for different induction heating durations. As can be observed, in general, the stability and flow increase ((b)) as the remaining water content after induction decreases ((a)). This effect is more noticeable for heating durations between 0 and 180 s. For the samples heated for 240 s, both the stability and flow increased, although no significant reduction in water content was observed. This indicates that induction treatment produces a beneficial effect not only because of drying the sample but also because of something else. As explained before, the authors have hypothesised that during the heating process the aged bitumen in RAP is reactivated. Thus, the viscosity of bitumen reduces, increasing its flow capacity and obtaining a more effective coating.
(a) shows the relations between the water content (wc) after being cured for 0, 1, 3, 7, and 21 days and MR for 0, 60, 120, 180, and 240 s of induction heating time (empty dots). (b) shows the relation between stability and flow for different curing days. As can be observed in (b), in general, while the remaining water content decreases, the flow tends to decrease. Consequently, MR (rigidity) increases.
Therefore, the effect of induction heating on MR is not as significant as that of curing time. Hence, although the mixtures become stronger but not stiffer immediately after induction treatment, their stiffness continues to increase with curing time. This behaviour is similar to that produced in the rehabilitation of a pavement with CIR mixtures, where the stiffness increases as the water content in the CIR mixture decreases.
4.6. Self-healing
presents the 3-point bending test results obtained by the samples before and after applying induction treatment. Although the tests were carried out at −20°C, the samples presented high deformation and particle loss in general. Consequently, the produced cracks were not completely clean and the vertical and load–deformation curves registered during the tests were highly irregular. In addition, samples 3, 5, and 7 collapsed during the first 3-point bending test and had to be rejected.
Table 4. Results of self-healing experiment in CIR mixtures.
Despite the difficulties mentioned above, in , a relation between the healing recovery (obtained by Equationequation 6(6)
(6) ) and induction heating time can be observed. In general, the healing level tends to increase with the induction time, although considerable data scattering was detected after 90 s. However, it can be said that at least 50% recovery is reached when a single induction treatment is applied.
Ajam et al. (Citation2017) carried out a study on the healing capacity of hot mix asphalt in very similar conditions (type of induction coil, selected current, coil–sample distance, etc.). Their results showed that after 90 s of induction heating, the obtained healing was also between 40% and 50%, which is very similar to the results shown in . However, after 120 s, the reported healing levels were close to 100%, while in the present investigation, the levels remained significantly lower.
Further investigation is necessary to determine if the healing of CIR is actually less effective and efficient than HMA.
5. Feasibility analysis
Previous results indicate that by applying induction heating technology, it is possible to use CIR mixes and open the road in the same day without risk of permanent deformations. In other words, it supposes a transition from a material similar to a cold mix asphalt (only applicable in low-and-light traffic roads), to a material similar to a hot mix asphalt (applicable in high-and-heavy-traffic roads), but avoiding high-energy-consuming and low-sustainable processes, such as drying the aggregates, mixing the components at 160°C for several minutes, transport the material at high temperature or laying and compacting it while producing fumes.
Assuming the dimensions of a highway CIR-mixture lane as: lane width = 350 cm (maximum Spanish road lane width); lane length = 100 cm; thickness = 5.7 cm (the same of the Marshall sample), the CIR section volume would be 350 × 100 × 5.7cm = 199,500 cm3≈0.2 m3. By considering a CIR mixture density of 2.2 t/m3, the amount of material to be heated is 0.44 t.
On the other hand the volume of a Marshall sample is 462.12 cm3. Then, dividing 199,500 cm3/462.12 cm3 = 431.71. Therefore, the volume (or the weight) of the highway section is 431.71 times of the one Marshall sample. Hence, the induction energy consumptions per ton of mixture when the material is heated for 60, 120, 180 and 240 s is respectively E60 = 2.8 kW × (60/3600) h × 431.71/0.44 t = 45.90 kWh/t; E120 = 91.81 kWh/t, E180 = 137.71 kWh/t and E240 = 183.61 kWh/t.
According to the literature (Chappat and Bilal Citation2003), the total energy consumption to manufacture and construct a layer of CIR mixture is equal to 38.61 kWh/t. Hence, if we add the process of induction heating, the total energy consumptions per ton of mixture is ET60 = 84.51 kWh/t, ET120 = 130.42 kWh/t, ET180 = 176.32 kWh/t and ET240 = 222.22 kWh/t for respective induction times of 60, 120, 180 and 240 s.
According to the same sources (Chappat and Bilal Citation2003, and Feng et al. Citation2014) the total energy consumption to manufacture and construct a hot mix asphalt layer is 188.89 kWh/t, while for the Swedish Transport Administration this energy consumption is 191.2 kWh/t (Martinsson et al Citation2016). Therefore, for induction heating times of 60, 120 and 180 s the total CIR mixture energy consumption is still lower than that necessary for hot mix asphalt.
In addition, the fact that a high-and-heavy traffic road can be opened the same day that the CIR mixture is constructed, instead of several days after, can save significant social costs caused by disruptions, extended travel time, etc. Moreover, it is important to consider that the generated greenhouse gases (GHG) emissions for a CIR mixture are only 10 kg/t; while the GHG emissions for bituminous concrete are 54 kg/t (Chappat and Bilal Citation2003). Also, it is also very important to take into account that, once the steel fibres are inside the CIR layer, the induction heating technology can be applied several times to repair cracks and extend the service life of the layer, with the consequent savings in maintenance and rehabilitation costs/emissions (Lizasoain-Arteaga et al Citation2019). Finally, the technology does not necessarily have to be applied in complete long road sections. It can be also implemented at a smaller scale, for example in maintenance works of specific damaged sections, such as patchings, bridge joints, crossings, etc.
For all these reasons, the feasibility of the technology can be assumed. Nevertheless, the present research is a first step in the development of this technology. The results at laboratory level were encouraging and showed that the technology might have high industrial potential. However, further investigation at full scale is still necessary to accurately assess aspects such as the actual on-site mechanical, economic and environmental performance.
6. Conclusions
This study investigated the feasibility of improving the mechanical behaviour of CIR mixtures during the curing period using induction heating. In addition, the healing capacity of CIR mixtures subjected to electromagnetic induction was also studied. After examining the obtained results, the following conclusions were drawn:
Induction heating is an effective method of reducing the amount of water in CIR mixtures once they have been already compacted.
The Marshall stability increased when induction heating was applied. The difference between heated and non-heated samples was particularly significant at early curing stages. The steady-state Marshall stability was also reached faster in mixtures heated by induction.
The Marshall flow of samples after induction also increased with heating time. However, as this increase was proportional to the increase in stability, in general, the rigidity of the material remained practically constant. Therefore, the material became stronger but not stiffer immediately after heating.
Induction heating makes the curing of CIR materials not only more effective but also faster. The authors have hypothesised that heating the aged binder in RAP after compaction might induce its reactivation by increasing its flow capacity to blend it with the virgin bitumen of the emulsion, obtaining a better coating and aggregate–binder adhesion.
With the passing of curing days, in general, the Marshall flow tended to decrease; consequently, the MR (rigidity) increased. This natural process was simply analogous to that of conventional CIR mixtures.
After analysing the results of the healing test, it can be concluded that at least 50% recovery can be achieved in CIR mixtures by induction heating.
A preliminary feasibility analysis was carried out, showing that the technology has potential to enable the use of CIR materials in high-and-heavy traffic roads, while providing certain economic and environmental advantages compared to other common materials, such as HMA. Nevertheless, further investigation at full-scale is still necessary to accurately assess the actual on-site performance.
Acknowledgements
The authors would like to acknowledge the funding of the project BIA2016-80317-R from the Spanish Ministry of Economy and Competitiveness. The authors also would like to express their sincere gratitude to ARIAS INFRAESTRUCTURAS for the RAP and to ECOASFALT for the bitumen emulsion, which was generously donated for the present research.
Disclosure statement
No potential conflict of interest was reported by the authors.
References
- AENOR, Asociación Española de Normalización y Certificación, 1994. UNE 103-501-94. Geotecnia. Ensayos de compactación. Proctor modificado. Madrid: Asociación Española de Normalización y Certificación.
- AENOR, Asociación Española de Normalización y Certificación, 2006a. UNE-EN 1097–6. Ensayos para determinar las propiedades mecánicas y físicas de los áridos. Parte 6: Determinación de la densidad de partículas y la absorción de agua. Madrid: Asociación Española de Normalización y Certificación.
- AENOR, Asociación Española de Normalización y Certificación, 2006b. UNE-EN 1426. Betunes y ligantes bituminosos. Determinación de la penetración con aguja. Madrid: Asociación Española de Normalización y Certificación.
- AENOR, Asociación Española de Normalización y Certificación, 2006c. UNE-EN 1427. Betunes y ligantes bituminosos. Determinación del punto de reblandecimiento. Método del anillo y bola. Madrid: Asociación Española de Normalización y Certificación.
- AENOR, Asociación Española de Normalización y Certificación, 2007a. UNE-EN 12697–2. Métodos de ensayo para mezclas bituminosas en caliente. Parte 2: Determinación de la granulometría de las partículas. Madrid: Asociación Española de Normalización y Certificación.
- AENOR, Asociación Española de Normalización y Certificación, 2007b. UNE-EN 12697–5. Métodos de ensayo para mezclas bituminosas en caliente. Parte 5: Determinación de la densidad máxima. Madrid: Asociación Española de Normalización y Certificación.
- AENOR, Asociación Española de Normalización y Certificación, 2007c. UNE-EN 12697–6. Métodos de ensayo para mezclas bituminosas en caliente. Parte 6: Determinación de la densidad aparente de probetas bituminosas por el método hidrostático. Madrid: Asociación Española de Normalización y Certificación.
- AENOR, Asociación Española de Normalización y Certificación, 2007d. UNE-EN 12697–8. Métodos de ensayo para mezclas bituminosas en caliente. Parte 8: Determinación del contenido de huecos en las probetas bituminosas. Madrid: Asociación Española de Normalización y Certificación.
- Ajam, H., et al., 2017. Self-healing of dense asphalt concrete by two different approaches: electromagnetic induction and infrared radiation. Journal of Testing and Evaluation, 45 (6), 1993–1940. doi:10.1520/JTE20160612.
- Al-Hdabi, A., et al., 2014b. Laboratory studies to investigate the properties of novel cold-rolled asphalt containing cement and waste bottom ash. Road Materials and Pavement Design, 15 (1), 78–89. doi:10.1080/14680629.2013.852612.
- Al-Hdabi, A., Al Nageim, H., and Seton, L., 2014a. Performance of gap graded cold asphalt containing cement treated filler. Construction and Building Materials, 69, 362–369. doi:10.1016/j.conbuildmat.2014.07.081.
- Asphalt Institute, 1983. Asphalt cold-mix recycling. Manual series n° 21, Lexington, Kentucky.
- Asphalt Institute, 1989. Asphalt cold mix. Manual Series n° 14, Lexington, Kentucky.
- Asphalt Recycling and Reclamation Association, 2003. Guidelines for cold in-place recycling. Annapolis, MD: Asphalt Recycling and Reclamation Association.
- Association Française de Normalisation. Norme Française, 2004. NFP 98-251-1. Enrobés hydrocarbonés. Essais statiques sur mélanges hydrocarbonés, partie1: Essais Duriez sur mélanges hydrocarbonés à chaud. Paris: Association Française de Normalisation.
- Babagoli, R., Ameli, A., and Shahriari, H, 2016. Laboratory evaluation of rutting performance of cold recycling asphalt mixtures containing SBS modified emulsion. Petroleum Science and Technology, 34 (4), 399–313. doi:10.1080/10916466.2015.1135168.
- Chappat, S. and Bilal, J., 2003. Sustainable development. The environmental road of the future. Life cycle analysis. Energy consumption & greenhouse gas emissions.
- Chávez-Valencia, L.E., et al., 2007. Improving the compressive strengths of cold-mix asphalt using asphalt emulsion modified by polyvinyl acetate. Construction and Building Materials, 21 (3), 583–589. doi:10.1016/j.conbuildmat.2005.07.017.
- Cross, S, 1999. Experimental cold in-place recycling with hydrated lime. Transportation Research Record, 1684, 186–193. doi:10.3141/1684-22.
- Dulaimi, A., et al., 2016. New developments with cold asphalt concrete binder course mixtures containing binary cementitious filler (BBCF). Construction and Building Materials, 124, 414–423. doi:10.1016/j.conbuildmat.2016.07.114.
- Dulaimi, A., et al., 2017. High performance cold asphalt concrete binder course using alkali-activated binary blended cementitious filler. Construction and Building Materials, 141, 160–170. doi:10.1016/j.conbuildmat.2017.02.155.
- Ebels, L.J., 2008. Characterisation of material properties and behavior of cold bituminous mixtures for road pavements. Doctoral Dissertation. Stellenbosh University. South Africa.
- Feng, L., et al., 2014. Investigation and analysis on the two-year energy consumption on asphalt pavement in Lúan City in China. In: J. Harvey, J. Agnès and D. Jones, eds. International Symposium on pavement life cycle assessment. Davis, CA: University of California, Pavement Research Center, 14–16.
- García, A., et al., 2009. Electrical conductivity of asphalt mortar containing conductive fibers and fillers. Construction and Building Materials, 23 (10), 3175–3181. doi:10.1016/j.conbuildmat.2009.06.014.
- García, A., et al., 2011a. Induction heating of mastic containing conductive fibers and fillers. Materials and Structures, 44 (2), 499–508. doi:10.1617/s11527-010-9644-2.
- García, A., et al., 2011b. A simple model to define induction heating in asphalt mastic. Construction and Building Materials, 31, 38–46. doi:10.1016/j.conbuildmat.2011.12.046.
- García, A., et al., 2012. Optimization of composition and mixing process of a self-healing porous asphalt. Construction and Building Materials, 30, 59–65. doi:10.1016/j.conbuildmat.2011.11.034.
- Gómez-Meijide, B., et al., 2016. Effect of air voids content on asphalt self-healing via induction and infrared heating. Construction and Building Materials, 126, 957–966. doi:10.1016/j.conbuildmat.2016.09.115.
- Jenkins, K.J. and Collings, D.S, 2017. Mix design of bitumen-stabilised materials – South Africa and abroad. Roads Materials and Pavement Design, 18 (2), 331–349. doi:10.1080/14680629.2016.1213511.
- Jenkins, K.J., Long, F.M., and Ebels, L.J, 2007. Foamed bitumen mixes = shear performance? International Journal of Pavement Engineering, 8 (2), 85–98. doi:10.1080/10298430601149718.
- Kishore Kumar, C., et al., 2008. Investigation of cold-in place recycled mixes in India. International Journal of Pavement Engineering, 9 (4), 265–274. doi:10.1080/10298430701551201.
- Lizasoain-Arteaga, E., et al., 2019. Environmental impact assessment of induction-healed asphalt mixtures. Journal of Cleaner Production, 208, 1546–1556. doi:10.1016/j.jclepro.2018.10.223.
- Martisson, K., et al., 2016. Calculation of energy and carbon dioxide on asphalt pavements. In: EAPA and Eurobitumen, organizer. 6th Euroasphalt & Eurobitume Congress, Jun 1–3, Prague, Czech Republic.
- Ministerio de Fomento, 2002. Reciclado de Firmes. Orden Circular 8/2001. Dirección General de Carreteras, Madrid, Spain.
- Ministerio de Fomento, 2014. Emulsiones Bituminosas. Artículo 214 del Pliego de Prescripciones Técnicas Generales para Obras de Carreteras y Puentes (PG-3), Madrid, Spain.
- Ministerio de Obras Públicas y Transportes, 2002a. Normas NLT. NLT 159. Método Marshall para mezclas asfálticas. Ensayos de carreteras. Dirección General de Carreteras, 2nd ed. Madrid, Spain.
- Ministerio de Obras Públicas y Transportes, 2002b. Normas NLT. NLT-161. Resistencia a compresión simple de mezclas bituminosas. Ensayos de carreteras. Dirección General de Carreteras, 2nd ed. Madrid, Spain.
- Ministerio de Obras Públicas y Transportes, 2002c. Normas NLT. NLT-162. Efecto del agua sobre la cohesión de las mezclas bituminosas compactadas. Ensayos de carreteras. Dirección General de Carreteras, 2nd ed. Madrid, Spain.
- Mogadan, B.B. and Mollashahi, H.F, 2017. Suggesting a simple design method for cold recycled asphalt mixes with asphalt emulsion. Journal of Civil Engineering and Management, 23 (7), 966–976. doi:10.3846/13923730.2017.1343200.
- National Academies of Sciences, Engineering, and Medicine, 2011. Recycling and reclamation of asphalt pavements using in-place methods. Washington, DC: The National Academies Press. doi:10.17226/14568.
- National Academies of Sciences, Engineering, and Medicine, 2017. Material properties of cold In-place recycled and full-depth reclamation asphalt concrete. Washington, DC: The National Academies Press. doi:10.17226/24902.
- Nicholls, J.C., 1998. Asphalt surfacings. London: E&FN SPON.
- Nivedya, M.K. and Veeraragavan, A., 2017. Bitumen-stabilised materials for sustainable road infrastructure. In: Babu G. Sivakumar, S. Saride, and B. Basha, eds, Sustainability issues in civil engineering. Springer transactions in civil and environmental engineering. Singapore: Springer, 35–50. doi: 10.1007/978-981-10-1930-2_3.
- Oruc, S., Celik, F., and Akpinar, M.V, 2007. Effect of cement on emulsified asphalt mixtures. Journal of Materials Engineering and Performance, 16 (5), 578–583. doi:10.1007/s11665-007-9095-2.
- Pérez, I., Medina, L., and del Val, M.A, 2013. Mechanical properties and behaviour of in-situ materials which are stabilised with bitumen emulsion. Road Materials and Pavement Design, 14 (2), 221–238. doi:10.1080/14680629.2013.779301.
- Pérez, I., Medina, L., and del Val, M.A, 2016. Nonlinear elasto-plastic performance prediction of materials stabilized with bitumen emulsion in rural road pavements. Advances in Engineering Software, 91, 69–79. doi:10.1016/j.advengsoft.2015.10.009.
- Pérez, I., Pasandín, A.R., and Medina, L, 2012. Hot mix asphalt using C&D waste as coarse aggregates. Materials and Design, 36, 840–846. doi:10.1016/j.matdes.2010.12.058.
- Pinto, I. and Buss, A, 2018. Layer coefficients of cold in-place recycled layers from performance data. International Journal of Pavement Engineering (In press). doi: 10.1080/10298436.2018.1473863.
- Roos, C., 2018. A design function for Bitumen stabilised material performance based on laboratory and field evaluation. Doctoral Dissertation. Stellenbosh University. South Africa.
- Tabaković, A., McNally, C., and Fallon, E, 2016. Specification development for cold in-situ recycling of asphalt. Construction and Building Materials, 102 (1), 318–328. doi:10.1016/j.conbuildmat.2015.10.154.
- Tebaldi, G., et al., 2014. Synthesis of standards and procedures for specimen preparation and in-field evaluation of cold-recycled asphalt mixtures. Roads Materials and Pavement Design, 15 (2), 272–299. doi:10.1080/14680629.2013.866707.
- Thanaya, I.N.A, 2007. Evaluating and improving the performance of cold asphalt emulsion mixes. Civil Engineering Dimension, 9 (2), 64–69. doi:10.9744/ced.9.2.pp.%2064-69.
- Wang, Y., et al., 2018. Cold recycling of reclaimed asphalt pavement towards improved engineering performance. Journal of Cleaner Production, 171, 1031–1038. doi:10.1016/j.jclepro.2017.10.132. doi: 10.1016/j.jclepro.2017.10.132
- Xu, B., et al., 2016. Study on Marshall design parameters of porous asphalt mixture using limestone as coarse aggregate. Construction and Building Materials, 124, 846–854. doi:10.1016/j.conbuildmat.2016.08.005.