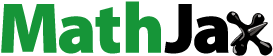
ABSTRACT
The chemical–physical hybrid cross-linked double network gel poly (acrylamide-co-acrylic acid)/Sodium alginate/Fe3+ (P(AM-co-AA)/SA/Fe3+, simplified as P-gel) was synthesised by free radical polymerisation combined with hydrogen bonding and ion coordination, which was in turn compounded with styrene–butadiene-styrene (SBS) to prepare P-gel gel/SBS modified asphalt. FT-IR, UV, XRD and SEM analyses confirmed the successful synthesis of P-gel. FM analysis revealed that the volume of P-gel increased after the long-term aging of asphalt. The physical, Dynamic Shear Rheometer (DSR), multi-stress creep recovery (MSCR), and time sweep tests showed that the addition of P-gel improved the road performance of the SBS modified asphalt. At a P-gel amount of 5%, the high-temperature performance grade (PG high temperature) of SBS modified asphalt improved from PG70 to PG76 and the recovery rate (64 oC) was increased by 17.08% and 41.13% at the stress of 0.1 and 3.2 kPa, respectively. The underlying mechanism behind these improvements in the mechanical properties of the composite modified asphalt was the different competitive relationship between the ‘softening’ effect of P-gel in asphalt and the ‘strengthening’ effect of the asphalt itself at different temperatures. This project provides an alternative strategy for preparing P-gel gel/SBS modified asphalt with enhanced mechanical properties for applications in the highway and construction industries.
Research highlights
P(AM-co-AA)/SA/Fe3+ gel compound SBS was applied to prepare modified asphalt.
P(AM-co-AA)/SA/Fe3+ gel/SBS asphalt had better viscoelastic properties.
P(AM-co-AA)/SA/Fe3+ gel/SBS asphalt had good anti-rutting and anti-fatigue property.
P(AM-co-AA)/SA/Fe3+ gel absorbs some light components of asphalt, causing volume expansion.
1. Introduction
Asphalt pavement is widely used in highways for its good anti-skating and noise reduction characteristics (Sidess and Uzan Citation2009). However, the conventional asphalt pavement is vulnerable to various forms of damage due to the lower performance of base asphalt (Liang et al. Citation2017, Wang et al. Citation2017). These issues have been mitigated to a certain extent by preparing modified asphalts for applications in the highway and construction industries. Among these, styrene–butadiene-styrene block copolymer (SBS) modified asphalt has been the most extensively applied version with its better improvement effect (Han et al. Citation2018, Li et al. Citation2019). Nevertheless, with a continuous increase in the load and number of vehicles, various damages to the SBS modified asphalt-based pavements still happen (Zeng et al. Citation2017, Li et al. Citation2018b), which is the strength limitation and occurrence of aging of SBS. Thus, finding ways to solve these problems is of utmost significance to design modified asphalt that can sustain a wide range of changing environmental conditions and remain least affected under the heavy traffic load for a longer duration (Wen et al. Citation2002, Xiao et al. Citation2014).
For enhancing the performance of SBS modified asphalt, composite modification using other materials has been reported several times, such as graphene nanoplatelets (Han et al. Citation2018, Li et al. Citation2018a, Han et al. Citation2021), nano-silica (Cai et al. Citation2018), carbon nanotubes (Wang et al. Citation2021), etc. The reason is that the particular functions they possess make them meet the requirements of asphalt modifiers. As a three-dimensional (3D) network structure (Geim and Novoselov Citation2007), the gel is a high molecular weight polymer that can expand volume after absorbing liquid components, and hence obtain excellent elastic property (Geim and Novoselov Citation2007), a feature similar to the way SBS acts in asphalt. In addition, the high degree of reactivity gives it good chemical/thermal stability, which could mitigate the deterioration of SBS modified asphalt after aging. However, the molecular structure types in traditional gels are too diverse and lack effective energy dissipation mechanisms (Ghavanini and Theander Citation2015), which results in lower mechanical strength and cannot meet the requirement of higher properties needed for pavement materials. Recently, by introducing an effective energy dissipation mechanism or improving the uniformity of the molecular structure, a combination of physical, chemical and natural macromolecular substances based on a simple chemical network has led to the production of a variety of high strength and flexible composite network structure gel such as double chemical cross-linked network gel (Le et al. Citation2016), chemical–physical hybrid cross-linked double network gel (Zhou et al. Citation2017, Liang et al. Citation2019) and bio-based gel (Yanturina et al. Citation2017).
Thus, in this study, a modified asphalt was prepared by combining the excellent mechanical properties of SBS modified asphalt and intriguing elastic, strength and toughness properties of the composite gel. For this purpose, based on the free radical polymerisation method combined with hydrogen bonding and ion coordination effect (Zhou et al. Citation2017, Liang et al. Citation2019), a chemical–physical hybrid cross-linked double-network gel from poly (acrylamide-acrylic acid copolymer)/sodium alginate/ferric ion (P(AM-co-AAc)/SA/Fe3+, simplified as P-gel) with high elasticity, strength and toughness was synthesised as reported previously (Li et al. Citation2018c, Liang et al. Citation2019), and was in turn applied as a modifier to prepare SBS composite modified asphalt. Microscopic characterisations of P(AM-co-AAc) and P-gel were performed by Fourier transform infra-red (FT-IR) spectroscopy, Ultraviolet–visible (UV) spectroscopy, X-ray diffraction (XRD), thermogravimetric analysis (TGA) and scanning electron microscopy (SEM). Microscopic characterisations of P-gel/SBS modified asphalt (original and long-term aging) were obtained by Fluorescence microscopy (FM). The tensile property, flowability, storage stability, viscoelastic, anti-rutting and anti-fatigue properties of P-gel/SBS modified asphalt were determined by physical, storage stability, dynamic shear rheometer (DSR), multi-stress creep recovery (MSCR), and time sweep tests, respectively.
2. Experimental
2.1. Experimental materials
All the reagents and chemicals used in this project were of analytical grade (unless mentioned) and applied as received without additional purification. Base asphalt (AH-90#) was supplied by Dalian West Pacific Petrochemical Co., Ltd. China. Linear SBS (YH-791) was provided by Shandong heavy traffic bridge Engineering Co., Ltd. China. Acrylic acid (AAc), N, N-methylenebisacrylamide (MBA, 99%, Electrophoresis grade), Potassium persulfate (KPS), SA (CP, viscosity: 200 ± 20 MPa·s) and N, N, N, N-tetramethylethylenediamine (TMEDA, Biotech grade) were obtained from Shanghai Macklin Biochemical Technology Co., Ltd. China. Acrylamide (AM) was obtained from Tianjin Zhiyuan chemical reagent Co., Ltd. China. Ferric chloride hexahydrate (FeCl3•6H2O) was obtained from the Tianjin Xinbote chemical Co., Ltd. China. Sodium chloride (NaCl) was obtained from the Tianjin Damao chemical reagent factory, China.
2.2. Preparation method
2.2.1. Preparation of P-gel
The preparation process of the P-gel is shown in , where the first step involved the preparation of double network gel P(AM-co-AAc)/SA. 40 ml of deionised water was taken in a 250 ml beaker to which AAc (1.12 g), AM (5.6 g), and SA (0.4 g) were added and stirred at 500 rpm until complete dissolution. Then, MBA (cross-linking agent, 0.01 g), KPS (initiator, 0.064 g) and TMEDA (reducing agent, 0.1 g) were added and stirred until fully dissolved. Nitrogen gas was then introduced to the mixed solution for 10 min to remove excess air, and then the beaker was sealed and placed in the 45 oC thermostat for 6 h to obtain P(AM-co-AAc)/SA. Then, Fe3+ was introduced into P(AM-co-AAc)/SA for coordination. Firstly, 0.3 M Fe3+ aqueous solution was added to P(AM-co-AAc)/SA and soaked for 24 h. Then, 0.1536 M NaCl solution was added to this mixture and further soaked for 24 h to obtain P-gel as reported previously (Li et al. Citation2018c, Liang et al. Citation2019). The obtained P-gel was then dried in an oven followed by grinding it into powder. At the same time, a control group P(AM-co-AAc) was prepared to analyse the effect of SA/Fe3+ addition on the structure and chemistry of the material.
The synthesis mechanism of P-gel is shown in . First of all, AM and AAc initiate the free radical polymerisation reaction through the redox system under the action of crosslinking agent, initiator and reducing agent to form a chemical network of P (AM-co-AAc) (Li et al. Citation2018c, Liang et al. Citation2019), which contains the SA molecules in the shape of curled and folding chain. As SA, AAc and AM molecular chains include carboxylic groups (-COO-), carboxyl groups (-COOH) and amide groups (O = C-NH2), respectively, the weak hydrogen bond can be formed with each other, resulting in the physical cross-linked network structure of P(AM-co-AAc)/SA. When Fe3+ is introduced to this mixture, -COO- can interact with Fe3+ through ion coordination (Zhou et al. Citation2017) to form a high-strength coordination bond. Among them, monocarboxylic anion (-COO-) is a bidentate ligand, and the coordination number of Fe3+ is 6 (Liu et al. Citation2018). Since P (AM-co-AAc) and SA have multiple -COO- groups, they can be regarded as multidentate ligands. In this case, Fe3+ and P (AM-co-AAc) and SA will form multiple coordination bonds with different -COO- groups, as shown in (a-h). In the coordination bond types shown in (a-d), Fe3+ only coordinates with -COOH on the single molecular chain of P (AM-co-AAc) or SA to form monodentate coordination or bidentate chelation structure. Hence it cannot let the two networks connect. Among the other four types shown in (e-h), Fe3+ simultaneously coordinates with -COO- on different molecular chains to form a bidentate, tridentate or tetradentate bridging structure. Therefore, establishing connections between the two network structures and creating a more robust physical network (the network connections have many possible types that can exist simultaneously, i.e. P(AM-co-AAc)/P(AM-co-AAc), SA/SA and P(AM-co-AAc)/SA). Therefore, increasing the proportion types of coordination bond shown in (e-h) is the key to improving the structural strength of P-gel. In addition, some unreacted free Fe3+ are also available in the solution, which can be easily washed away upon immersing the gel in NaCl solution (Zhou et al. Citation2017, Liang et al. Citation2019), thereby increasing the ratio of the multi-tooth bridge structure and further improving the strength of the network structure.
2.2.2. Preparation of P-gel/SBS modified asphalt
shows the procedure for the preparation of P-gel/SBS modified asphalt. Firstly, the base asphalt was heated in an oven at 130 oC for 2 h to remove any water present. Then, P-gel powder according to different percentages (mass percentage of base asphalt) was mixed with SBS (5%, mass percentage of base asphalt) and added to the asphalt, and passed through a high-speed shear emulsifier (5000 rpm) at 165 oC for 40 min. Subsequently, the asphalt was placed in a high-speed disperser (500 rpm) for 60 min, and the stabiliser (0.1%, mass percentage of base asphalt) was added in the last 10 min. Finally, the sample was heated at 160 oC in an oven and allowed static for 2 h to complete the development of P-gel/SBS modified asphalt. The SBS modified asphalt was prepared using the same preparation method (without adding P-gel) as the control group. The composition and abbreviated forms of asphalts prepared in this study are shown in .
Table 1. Different modified asphalt components, added amount and simplified name.
2.3. Characterisation and performance analysis
For the micro-characterisation of P-gel, the FT-IR and UV (Perkin Elmer spectrum 100 and PerkinElmer Lambda 950, PerkinElmer Co., Ltd.) were used to analyse the changes in the chemical composition of the material by the introduction of SA and Fe3+. The XRD (BRUKER D8 ADVANCE, Germany BRUKER Co., Ltd.) was used to analyse the influence of SA and Fe3+ introduction on the crystal structure of the gel. The SEM (Sigma 500, Carl Zeiss AG, accelerating voltage: 10 kV) was used to observe the influence of the introduction of SA and Fe3+ on the surface morphology of P(AM-co-AAc). The TGA (Mettler TGA/DSC3+, METTLER TOLEDO) was used to test the heat resistance of the P-gel to determine its stable temperature range. FM (BX53, Olympus) was used to observe the distribution and existence of P-gel in the SBS modified at the original and long-term aging state, respectively.
For the performance of P-gel/SBS modified asphalt, physical property tests (ductility, softening point and penetration) were used to analyse the four modified asphalt types (SBS, 1% P-gel/SBS, 3% P-gel/SBS and 5% P-gel/SBS modified asphalt) and conducted to analyse the effect of the addition of P-gel and its variable amount on the conventional properties (tensile property, flowability and consistency) of the SBS modified asphalt. Then, the storage stability test was used to analyse whether the P-gel during mixing is strong enough to resist phase separation in the condition in which it is stored. In addition, DSR test (DHR-2, TA instruments) was used to test the four modified asphalt types and conducted to analyse the effect of the P-gel and its variable amount on the viscoelastic properties of the SBS modified asphalt. Furthermore, MSCR test (DHR-2, TA instrument) was used to test the four modified asphalt types and conducted to analyse the effect of the addition of P-gel and its variable amount on the anti-rutting property of the SBS modified asphalt. Finally, the time sweep test (DHR-2, TA instrument) was used to test the four modified asphalt types and conducted to analyse the effect of the addition of P-gel and its variable amount on the anti-fatigue property of the SBS modified asphalt.
3. Results and discussion
3.1. Microscopic representation of P-gel and P-gel/SBS modified asphalt
shows the FT-IR spectra of P(AM-co-AAc) and P-gel. For P(AM-co-AAc), the absorption peak at 3204.8 cm−1 is due to the stretching vibration of N-H bond (Duan et al. Citation2019a). The absorption peaks at 2921.1 cm−1 and 1452.1 cm−1 are ascribed to the stretching vibration and bending vibration of the C–H bond, respectively (Nallasamy and Mohan Citation2004, Duan et al. Citation2019b). The absorption peak at 1642.5 cm−1 is caused by the stretching vibration of C = O connected to -NH2 while the one at 1609.7 cm−1 can be attributed to the asymmetric tensile vibration of -COO- (Cabaness et al. Citation1971). After adding SA and Fe3+, the original absorption peaks at 3204.8 cm−1 and 1609.7 cm−1 shifted to 3224.4 cm−1 and 1615.2 cm−1, respectively. These results indicate that -COO- (from AAc and SA) forms the hydrogen bond with O = C-NH2 (Liang et al. Citation2019). In addition, no new absorption peaks appeared, indicating that no true chemical reaction occurred between SA and Fe3+ and P(AM-co-AAc), and they merely physically mixed.
shows the UV spectra of P(AM-co-AAc) and P-gel. Compared with the spectrum of P(AM-co-AAc), it can be seen that P-gel has one absorption peak at 457 nm, which is caused by the complex produced from the coordination of Fe3+ with -COO- on P(AM-co-AAc) and SA (Li et al. Citation2018c).
shows the XRD patterns of P(AM-co-AAc) and P-gel, which suggest that P(AM-co-AAc) has a strong diffraction peak at 2θ = 22.27o, and the peak shape is relatively sharp, with the interplanar spacing of d = 0.3988 nm calculated from Bragg's formula (Zhu et al. Citation2012). The sharp diffraction peak indicates the highly consistent structure of P(AM-co-AAc). In contrast, the diffraction peak of the P-gel showed a particular shift (2θ = 23.19o, d = 0.3832 nm) with a gentler peak, indicating that the structure types of P-gel are more complicated. This is because the introduction of SA and Fe3+ can generate hydrogen bonds and multiple coordination bond types with P(AM-co-AAc), respectively, which promote the diversified structure types of P-gel. At the same time, the decreased interplanar spacing (Δd = 0.0186 nm) indicates that the formation of hydrogen bonds and coordination bonds has shortened the distance between adjacent molecular chains and played a role in crosslinking networks.
(a-c) shows the SEM test results of P(AM-co-AAc) while those of P-gel are shown in Fig. (d-f). From (a-c), it can be seen that P(AM-co-AAc) has a dense layered structure, with a smooth surface and a certain degree of undulation. (e-f) shows the same dense morphological structure, but the layered structure is more apparent and reduced spacing. This is due to the coordination of Fe3+ with SA and P (AM-co-AAc). At the same time, the roughness of the gel surface increased significantly, which is caused by the addition of SA.
Figure 7. SEM images of P(AM-co-AAc) (a: 1000X, b: 5000X and c: 20,000X) and P-gel (d: 1000X, e: 5000X and f: 20,000X).
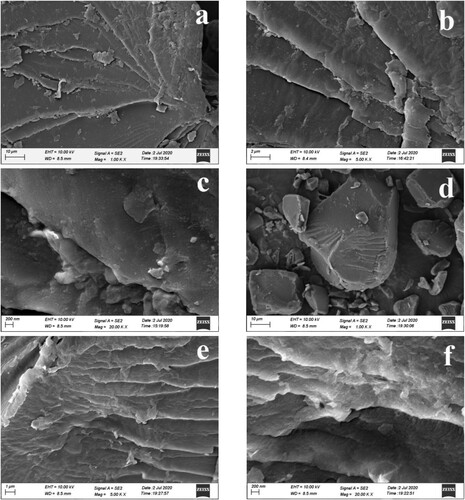
shows the TGA patterns of P(AM-co-AAc) and P-gel, which suggests that the residual mass of the two gels increases with increasing temperature. Still, a gentle area appears during the heating process, which divides the weight loss process of the material into two stages. The first stage is caused by the continuous evaporation of the water absorbed during the gel preparation process. According to the SEM analysis (), the dense surface of the two gels makes it difficult to evaporate the water during the drying process fully. The second stage is the decomposition process of the two kinds of gels. Among them, the characteristic decomposition temperatures of P (AM-co-AAc) and P-gel are 168.22 oC and 179.31 oC, respectively, indicating that SA and Fe3+ can improve the thermal stability of the gel. At the same time, this characteristic decomposition temperature of P-gel is greater than the maximum temperature (165 oC) used during the preparation of modified asphalt, which proves that P-gel can be stable during the preparation of modified asphalt.
(a-f) shows the FM test results of SBS, 1% P-gel/SBS and 5% P-gel/SBS modified asphalts. (a and b) (SBS modified asphalt) can be seen to form an obvious network structure, which is due to the swelling development and interconnection of SBS after absorbing light components in asphalt. The dot-shaped substances around the network structure are powdery SBS particles formed after shearing. The same network structure can also be observed from (c-f), and there are different amounts of uniformly dispersed massive substances, which is the added modifier P-gel. Due to the large amount of P-gel added (5%) in the asphalt in (e and f), the density of the bulk material is higher than that of (c and d).
Figure 9. FM of asphalt (original): SBS at 10X10 times (a) and 10X20 times (b), 1% P-gel/SBS at 10X40 times (c) and 10X20 times (d) and 5% P-gel/SBS at 10X10 times (e) and 10X20 times (f).
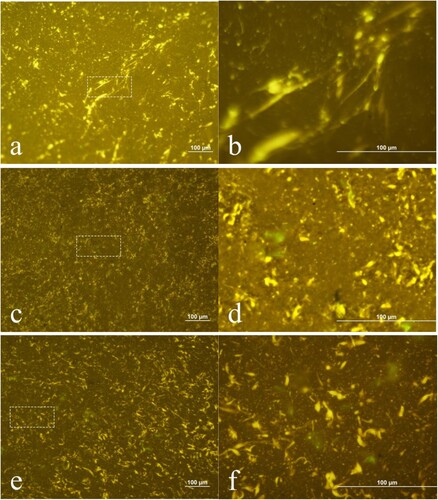
After aging treatment via rotating thin film oven test (RTFOT) and PAV of the three types of asphalt, FM test was performed and compared with those of the original asphalt samples and the results are shown in . It can be seen from (a and b) that the SBS network structure of the original asphalt has been broken down to a certain extent after long-term aging, and the same situation can also be seen in (d-i). This phenomenon may be because, during the aging process, SBS undergoes a certain degree of thermal and oxidative decomposition, resulting in the breakdown of the network structure (Han et al. Citation2019). Compared with (d) and (f), it can be found that the volume of P-gel expands to a extent. This phenomenon is more evident in the sample having high contents of P-gel (5% P-gel/SBS modified asphalt (h and i)). This proves that P-gel could absorb some light components in the asphalt leading to the expansion in the volume of the modified asphalt. Interestingly, after a long-term thermal oxygen aging treatment, P-gel did not decompose, indicating its excellent thermal stability, consistent with the TGA test results ().
Figure 10. FM test results of three kinds of asphalts (SBS: a and b at 10X10 times and c at 10X40 times, 1% P-gel/SBS: d and e at 10X10 times and f at 10X40 times and 5% P-gel/SBS: g and h at 10X10 times and i at 10X40 times) under different aging conditions (original: a, d and g and long-term aging state: b, c, e, f, h and i).
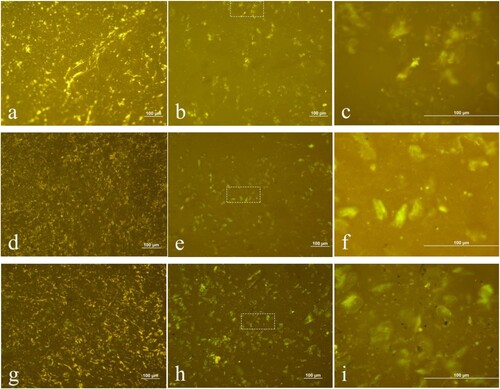
3.2. Physical test
In order to analyse the effect of P-gel and the amount of addition on the tensile property, flowability and consistency of SBS modified asphalt, the physical test (ductility, softening point, and penetration) were applied to four kinds of modified asphalts according to the test standards (T0605-2011, T0606-2011 and T0604-2011 of JTG E20-2011, respectively) (MOT Citation2019a). The test results are shown in .
As shown from , compared with SBS modified asphalt, the addition of P-gel can increase the ductility and softening point and decrease the penetration of the asphalt, and this effect changes with the amount of P-gel added. Within the scope of the study, adding 1% P-gel has the most significant impact on increasing the ductility of SBS modified asphalt (ductility = 17.83 cm), which is about 37.18% higher than that of SBS modified asphalt (ductility = 13.00 cm). As the amount of P-gel added increases, the softening point of SBS modified asphalt is increased while ductility and penetration are decreased. Compared with the softening point and penetration (3.818 mm) of the SBS modified asphalt (74.05 oC), the addition of 5% P-gel amount results in increasing the softening point of the SBS modified asphalt to 78.50 oC (6.01% increased) while penetration reduced to 3.023 mm (20.82% decreased). These results show that the addition of P-gel can improve the tensile property, flowability and consistency of the SBS modified asphalt. As the amount of P-gel added increases, the effect of enhancing tensile property decreases while enhancing flowability and consistency increases. This phenomenon is because P-gel can absorb certain light components in the asphalt, thereby increasing the consistency of the SBS modified asphalt. At the same time, the volume of P-gel swollen to a certain extent after absorbing light components, thus playing a ‘skeleton’ role in the SBS modified asphalt. However, when the amount of P-gel is too large (5%), a large amount of light components in the SBS modified asphalt are absorbed resulting in a decrease in its own fluidity, and the macroscopic performance is that the tensile property of the material is reduced (Du et al. Citation2013).
3.3. Storage stability test
Phase separation between modifier and asphalt often occurred during storage, pumping and pavement use of modified asphalt(Ouyang et al. Citation2006). Therefore the storage stability of modified asphalt is an important research issue. The high-temperature storage stability test was used to analyse whether the modifier (P-gel) during mixing is strong enough to resist phase separation in the condition in which it is stored. According to the test standard, modified asphalt is considered to have good high-temperature storage stability if the variation in softening point between the top and bottom of the treated bitumen (ΔT) after the experiment is less than 2.5 oC (MOT Citation2019b).
shows the storage stability test results of different types of modified asphalt. It can be found that the softening points of both the upper and lower parts of the four modified asphalts after treatment showed significant differences (ΔT all greater than 2.5 oC). The poorer thermal storage stability of the SBS-modified asphalt (ΔT = 26.2 oC) could be attributed to the lower addition of the stabiliser. Compared to SBS modified asphalt, the addition of P-gel resulted in a slight increase in ΔT, with the highest value at 5% (ΔT = 27.6 oC). The rise in ΔT indicates the presence of segregation of P-gel in the asphalt, but the minor change indicates a lower degree of segregation. This phenomenon may be because P-gel reduces the mobility of the asphalt after absorbing some light components in the asphalt, as evidenced by the increase in the softening point of the upper and lower parts of the P-gel/SBS modified asphalt. Due to the presence of segregation, asphalt samples in the subsequent tests were prepared with sufficient mixing to ensure material consistency.
3.4. Rheological properties tests
As a viscoelastic material, asphalt exhibits different rheological properties at different temperatures, and this characteristic plays a significant effect in the practical application of pavement. In order to accurately determine the rheological properties of asphalt, the DSR test was applied.
3.4.1. DSR test
According to the DSR test standard of asphalt (AASHTO Citation2020) at different temperatures, various physical quantities related to asphalt rheological properties are obtained. The complex modulus (G*) represents the property of resisting deformation, and the larger value indicates the higher stress required for the deformation of asphalt. The phase angle (δ) reflects the magnitude of asphalt deformation lagging behind the stress change. The storage modulus (G’) represents the asphalt's elastic property, and the larger value indicates the higher property (Han et al. Citation2018). The loss modulus (G'’) represents the viscous property of the asphalt, and the larger value indicates the higher property. Rutting factor (RF, G*/sin δ) characterises the high-temperature resistance of asphalt, and the value is also used as the high-temperature performance parameter in the Standard Specification for performance graded asphalt (PG) (ASTM Citation2021). The conversion relationships between G’, G'’, tan δ, RF, G* and δ are shown as Eq. (1-4).
(1)
(1)
(2)
(2)
(3)
(3)
(4)
(4)
In order to analyse the influence of P-gel and its amount on the high-temperature rheological properties of SBS modified asphalt, the test was conducted by frequency sweep (0.1-10 Hz) at different temperatures (46-82 oC) and performed in seven steps. Each step had a 6 oC rise in temperature.
shows the effect of the P-gel and addition amount on G’ of SBS modified asphalt. From (b), it can be found that at the same frequency (10 Hz), the G’ of four types of the modified asphalt decreased with increasing temperature, and the G’ of 5% P-gel/SBS modified asphalt was the largest among all the samples. As the amount of P-gel decreases, the G’ of modified asphalt is gradually decreased, and both are smaller than that of the SBS modified asphalt. Compared with SBS modified asphalt, the G’ of 5% P-gel/SBS modified asphalt has increased by 12.86% at 46 oC. From (b), it can be found that at the same temperature (46 oC), the G’ of the four modified asphalt types all increased as the loading frequency increases. The G’ of 5% P-gel/SBS modified asphalt is the largest among these samples, the same as (a). As the amount of P-gel decreases, the G’ of modified asphalt is gradually decreased, and both are smaller than that of the SBS modified asphalt. The changing trend in G’ shows that the addition of P-gel can improve the elastic property of SBS modified asphalt at higher contents.
Figure 13. Effect of P-gel and addition amount on the G’ of SBS modified asphalt: (a) different temperatures (46-82 oC) at constant frequency (10 Hz), (b) different frequencies (0.1-10 Hz) at constant temperature (46 oC).
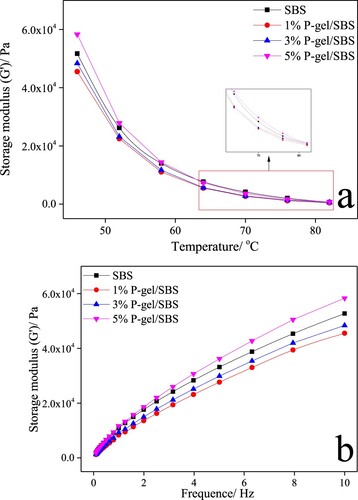
shows the effect of the P-gel and addition amount on G'’ of SBS modified asphalt, consistent with the results reported in . From (a), it can be found that at the same frequency (10 Hz), the G'’ of the four modified asphalts decreased with increasing temperature. The G'’ of 5% P-gel/SBS modified asphalt among the various samples is the largest. As the addition amount of P-gel decreases, the G'’ of modified asphalt is gradually decreased, and both are smaller than that of the SBS modified asphalt. Compared with SBS modified asphalt, the G'’ of 5% P-gel/SBS modified asphalt increases by 16.22% at 46 oC. From (b), it can be found that at the same temperature (46 oC), the G'’ of the four modified asphalt types all increase as the loading frequency increases. Among these samples, the G'’ of 5% P-gel/SBS modified asphalt is the largest. As the amount of P-gel decreases, the G'’ of modified asphalt is gradually decreased, and both are smaller than that of the SBS modified asphalt. The test results of G'’ indicate that the addition of P-gel can improve the viscosity property of SBS modified asphalt to a certain extent, but a higher amount of P-gel is required.
3.4.2. PG (high temperature)
In order to analyse the influence of the P-gel and its amount on the PG of the SBS modified asphalt at high temperature, according to the standard specification of PG (the rutting factor (RF) of the original asphalt is greater than 1.0kPa and the short-term aging state is greater than 2.2kPa) (AASHTO Citation2021) the RF of different modified asphalts was calculated according to Eq. 3.
shows the RF of four types of modified asphalt in original and short-term aging states at different temperatures. At 82 oC, the RF values of the four modified asphalt types in the original state are less than 1 kPa, while these were higher than 1 kPa at a lower temperature (76 oC). After short-term aging, the RF of these modified asphalts increased. However, only the RF of 5% P-gel/SBS modified asphalt was greater than 2.2 kPa, which meets the PG (high temperature) evaluation standard and belongs to PG76. In contrast, those of the other three types of modified asphalt was greater than 2.2 kPa at 70 oC only, and hence they belonged to PG70. These results show that higher contents of P-gel addition (5% within the scope of this study) can improve the high-temperature performance of the SBS modified asphalt.
Table 2. RF of original and short-term aging states asphalts at different temperatures with the various content of P-gel.
In summary, adding high amounts of P-gel in the test temperature range (46-82 oC) can improve the viscoelastic properties and PG (high temperature) of SBS modified asphalt. However, as the amount of P-gel decreased, this modification effect decreased correspondingly, and even the performance of SBS modified asphalt decreased. This may be because the P-gel absorbs some light components in asphalt to cause volume expansion and exist as elastic blocks in asphalt, then plays the role of ‘softening’ effect. At the same time, the decrease in the content of lighter components in asphalt leads to an increase in the proportion of heavier components and results in a reduction of the flowability of the asphalt, similar to the change in components during asphalt aging. In this process, the mechanical strength of asphalt has been improved to a certain extent, thereby playing a role of ‘strengthening’. These two kinds of processes exist and interact in the asphalt simultaneously, as shown in . When the addition amount of P-gel is low (1%), P-gel's ‘softening’ effect dominates, making the viscoelastic property of asphalt decrease. However, as the amount of P-gel increased (5%), the ‘strengthening’ effect of asphalt itself began to dominate so that the viscoelastic properties of asphalt could be restored to a certain extent. When the temperature rises, the modulus of asphalt itself decreases, but the modulus of P-gel itself marginally changes and can provide a particular supporting effect. Thus the viscoelastic properties of asphalt are increased upon increasing the temperature.
3.4.3. MSCR test
In order to analyse the influence of the P-gel and its amount on the rutting resistance of SBS modified asphalt at high temperature, a more scientific and representative MSCR test was conducted(AASHTO Citation2019). The test stress was under 0.1 kPa (20 loading cycles and the first 10 cycles at 0.1 kPa are for conditioning the specimen) and 3.2 kPa (10 loading cycle) levels, respectively. According to the test stander (JTG E20-2011)(MOT Citation2019a), the anti-rutting property of asphalt mixtures should be tested at 60 oC, and 64 °C is the closest temperature based on the PG distribution of asphalt. Therefore the temperature of MSCR tests was selected as 64 oC. The time-strain relation of different asphalts under corresponding loading stress are shown in .
Figure 16. Effect of P-gel and addition amount on SBS modified asphalt time-strain relation (64 oC) at: (a) 0.1 kPa and (b) 3.2 kPa.
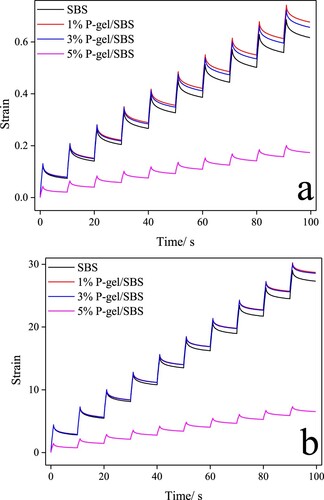
It can be found from (a) that under the stress of 0.1 kPa, the strain of the four types of modified asphalt increases stepwise with increasing the loading cycle, which is identical to the vehicular behaviour on the road surface under actual operation. Among them, the total strain (TS) of 1% P-gel/SBS modified asphalt is the largest (TS = 0.676) and is very similar to the TS of SBS modified asphalt (TS = 0.616). As the addition amount of P-gel increases (3%), the TS of the SBS modified asphalt begins to be decreased (TS = 0.656). As the addition amount of P-gel increases to 5%, the TS of SBS modified asphalt is significantly decreased (TS = 0.174, reduced by 71.84% compared with the SBS modified asphalt). After increasing the test stress to 3.2 kPa ( (b)), the time-strain relation of the four types of modified asphalt is the same as that shown in (a), but the growth rate of the dependent variable increases significantly. The TS of 1% P-gel/SBS modified asphalt is also the largest (TS = 28.71) and very similar to the TS of SBS modified asphalt (TS = 27.32). As the addition amount of P-gel increases (3%), the TS of the SBS modified asphalt begins to be decreased (TS = 28.56). As the addition amount of P-gel increased to 5%, the TS of SBS modified asphalt is significantly decreased (TS = 6.53, reduced by 76.10% compared with the SBS modified asphalt). These results show that the addition of an initial small amount of P-gel reduces the ability of the SBS modified asphalt to resist deformation. As the amount of P-gel added increases, the negative effect is gradually disappeared, which is similar to the change rules of DSR test results.
Based on the peak strain (γp), residual strain (γnr) and loading stress (τ) in each cycle of the MSCR test, the recovery rate (R) and creep compliance (Jnr) representing the asphalt's recovery ability can be obtained by Eq. 5 and 6. The R represents the degree of asphalt recoverability after being subjected to external force, and the Jnr represents the size (permanent deformation) of the asphalt non-recoverable part accumulated by an external force. Both of them are used to characterise the rutting resistance of asphalt (AASHTO Citation2019). The results of R and Jnr of SBS, 1% P-gel/SBS, 3% P-gel/SBS and 5% P-gel/SBS modified asphalt at 64 oC are shown in .
(5)
(5)
(6)
(6) Comparing the R and Jnr of four modified asphalt types at 64 oC, it can be found that the R has been increased to different degrees, and the Jnr decreased. Comparing the R of four types of modified asphalt, the 1% P-gel/SBS modified asphalt is the smallest under both loading stresses, though it is similar to that of SBS modified asphalt (ΔR0.1 = −3.70% and ΔR3. 2 = −3.48%). As the amount of P-gel increases (3%), the R of the SBS modified asphalt begins to increase (ΔR0.1 = −2.78% and ΔR3.2 = 3.33). When the amount of P-gel is increased to 5%, the R of SBS modified asphalt increased significantly (ΔR0.1 = 8.70% and ΔR3.2 = 16.01%, increased by 17.08% and 41.13%, respectively). Comparing the Jnr of four types of modified asphalt, the 1% P-gel/SBS modified asphalt is the largest under both stress levels, but it is very similar to that of SBS modified asphalt (ΔJnr0.1 = 0.060 kPa−1 and ΔJnr3.2 = 0.062 kPa−1). As the addition amount of P-gel increases (3%), the Jnr of the SBS modified asphalt increases (ΔJnr0.1 = 0.040 kPa−1 and ΔJnr3.2 = 0.039 kPa−1). When the addition amount of P-gel increased to 5%, the Jnr of SBS modified asphalt showed a very significant change (ΔJnr0.1 = 0.442 kPa−1 and ΔJnr3.2 = 0.650 kPa−1, reduced by 71.75% and 76.11%, respectively).
Table 3. R and Jnr of different modified asphalts at 0.1 and 3.2 kPa (64 oC).
In summary, adding a higher amount of P-gel (5%) can improve the rutting resistance of SBS modified asphalt because the asphalt component changed and became stiffer. In addition, the P-gel could act as an elastic skeleton in the asphalt and hence can function as a buffer and energy storage point upon exposure to the effect of external forces, thereby improving the rutting resistance of asphalt.
3.4.4. Time sweep test
In order to evaluate the influence of P-gel addition and its amount on the anti-fatigue property of SBS modified asphalt, a time sweep test was performed on the four types of modified asphalts after long-term aging (Anderson et al. Citation2001, Wang et al. Citation2016, Safaei and Castorena Citation2017). The number of loading times at which the complex modulus (G*) is reduced to 50% of the initial value is used as the fatigue life of the asphalt at 25 oC and 0.25 MPa strain (Wang et al. Citation2016). The test results are shown in .
It can be seen from that as the number of loading increases, the complex modulus of the modified asphalts decreases and the G* is reduced to 50% of the initial value, the load number of 5% P-gel/SBS modified asphalt is the largest in the test range. As the amount of P-gel decreases, the load number of modified asphalt is decreased, and both are smaller than that of the SBS modified asphalt. Compared with SBS modified asphalt (32396 times), the load number of 5% P-gel/SBS modified asphalt is increased by 21.28% (39291 times), which shows that a high amount of addition of P-gel can improve the anti-fatigue property of asphalt. These results once again confirmed the data obtained in DSR and MSCR tests discussed above.
In the process of repeated shear deformation of asphalt, the energy dissipation of elastic and viscous parts is inevitable (Wang et al. Citation2016). This process can be expressed by the change rate of dissipated energy (dissipated energy rate of change, DR) between adjacent loading times, which can be calculated by Eq. 7 and 8 (Wang et al. Citation2016).
(7)
(7)
(8)
(8) Where,
represents the energy consumed during the i-th load,
represents the energy consumed during the (i-1)-th load,
represents the i-th strain during the secondary load,
represents the complex modulus during the i-th load, and
represents the phase angle of the i-th load. The calculation results of different modified asphalts are shown in .
It can be seen from that in the test environment, there are two turning points in the DR-load number curves of the modified asphalts, thus dividing the whole process into three parts. In the first part, the damage to the asphalt is marginal and hence can be ignored. During the second part, the asphalt accumulated damage as the number of loads increased, and the second turning point represents the damage limit of asphalt. It can be found that 5% P-gel/SBS modified asphalt has the largest load number (41938 times) in the second turning point. As the addition amount of P-gel decreases, the load number of modified asphalt is gradually decreased. Both were smaller than SBS modified asphalt, which has a similar pattern of change as shown in . Compared with SBS modified asphalt (33826 times), the load number of 5% P-gel/SBS modified asphalt is increased by 23.98%.
These results show that the addition of P-gel can improve the anti-fatigue property of SBS modified asphalt, though a higher amount is required (within the scope of this study). This phenomenon is also the result of the higher relaxation capacity of the modified asphalt imparted by the P-gel.
3.5. Cost of preparation
The cost of P-gel was calculated regarding the price of each chemical at a 5% addition amount (compared with 5% SBS modified) and shown in . According to the investigations, the unit price of base asphalt and SBS is 632 $/ton and 3162 $/ton, respectively. It can be found from that the extra cost of 5% P-gel is 99.194 $/ton (SBS modified asphalt), a 12.56% decrease compared to 5% SBS modified asphalt.
4. Conclusions
In summary, this study reported the synthesis of P-gel based on a chemical–physical hybrid cross-linking double network and compounded it with SBS to prepare modified asphalt. Through the micro-characterisation (FT-IR, UV, XRD, SEM, TG and FM), physical, storage stability and mechanical properties tests (DSR, MSCR and time sweep tests), the following conclusions can be deduced from this study:
TG and FM test results showed that the P-gel could not be decomposed in asphalt during the preparation and aging process. The volume of the P-gel can be expanded, thereby achieving the role of the elastic skeleton.
The addition of 5% P-gel can improve the viscoelastic, anti-rutting and anti-fatigue properties of SBS modified asphalt (within the scope of this study).
Comparing the mechanical properties of SBS modified asphalt with different P-gel amounts, it is found that the higher dose has a noticeable improvement effect on asphalt viscoelastic, anti-rutting and anti-fatigue properties.
The ‘softening’ effect of P-gel due to absorbing some light components and the ‘strengthening’ effect of asphalt due to losing light components combine to influence the final road performance of the modified asphalt, which changes with the variable amount of P-gel.
Credited to the better mechanical properties and resistance against rutting, using the P-gel as a modifier could be of potential value and effectively applied in pavement and construction to prepare an alternative type of modified asphalt. However, the modified asphalt obtained by the preparation process used in this study should be concerned with the segregation problem of the modifier.
Disclosure statement
No potential conflict of interest was reported by the author(s).
Additional information
Funding
References
- AASHTO, 2019. T 350-19, standard test method for multiple stress creep and recovery (MSCR) of asphalt binder using a dynamic shear rheometer. American Association of State Highway and Transportation Officials.
- AASHTO, 2020. T 315-20, determining the rheological properties of asphalt binder using a dynamic shear rheometer (DSR). American Association of State Highway and Transportation Officials.
- AASHTO, 2021. M 320-21, standard specification for performance-graded asphalt binder. American Association of State Highway and Transportation Officials.
- Anderson, D., et al., 2001. Evaluation of fatigue criteria for asphalt binders. Transportation Research Record: Journal of the Transportation Research Board, 1766, 48–56.
- ASTM, 2021. D 6373-21, Standard Specification for Performance Graded Asphalt Binder American Society of Testing Materials.
- Cabaness, W.R., Lin, T.Y.-C., and Párkányi, C, 1971. Effect of pH on the reactivity ratios in the copolymerisation of acrylic acid and acrylamide. Journal of Polymer Science Part A-1: Polymer Chemistry, 9 (8), 2155–2170.
- Cai, L., Shi, X., and Xue, J., 2018. Laboratory evaluation of composed modified asphalt binder and mixture containing nano-silica/rock asphalt/SBS. Construction and Building Materials, 172, 204–211.
- Du, H., Quek, S.T., and Pang, S.D., 2013. Smart multifunctional cement mortar containing graphite nanoplatelet. Singapore: SPIE.
- Duan, S., et al., 2019a. Synthesis and evaluation of high-temperature properties of butylated graphene oxide composite incorporated SBS (C4H9-GO/SBS)-modified asphalt. Journal of Applied Polymer Science, 136 (46), 48231.
- Duan, S., et al., 2019b. Enhancing effect of microalgae biodiesel incorporation on the performance of crumb rubber/SBS modified asphalt. Journal of Cleaner Production, 237, 117725.
- Geim, A.K., and Novoselov, K.S., 2007. The rise of graphene. Nature Materials, 6 (3), 183–191.
- Ghavanini, F.A., and Theander, H., 2015. Graphene feasibility and foresight study for transport infrastructures.
- Han, M., et al., 2018. Effect of polystyrene grafted graphene nanoplatelets on the physical and chemical properties of asphalt binder. Construction & Building Materials, 174, 108–119.
- Han, M., et al., 2019. preparation of octadecyl amine grafted over waste rubber powder (ODA-WRP) and properties of Its incorporation in SBS-modified asphalt. polymers, 11 (655), 1–21.
- Han, M., et al., 2021. A review on the development and application of graphene based materials for the fabrication of modified asphalt and cement. Construction and Building Materials, 285, 122885.
- Le, J., Marasteanu, M., and Turos, M., 2016. Graphene Nanoplatelet (GNP) Reinforced Asphalt Mixtures: A Novel Multifunctional Pavement Material.
- Li, J., et al., 2018a. Preparation and properties of SBS-g-GOs-modified asphalt based on a thiol-ene click reaction in a bituminous environment. Polymers, 10 (1264), 1–21.
- Li, J., et al., 2018b. Comparative analysis, road performance and mechanism of modification of polystyrene graphene nanoplatelets (PS-GNPs) and octadecyl amine graphene nanoplatelets (ODA-GNPs) modified SBS incorporated asphalt binders. Construction and Building Materials, 193, 501–517.
- Li, J., et al., 2019. Studies on the properties of modified heavy calcium carbonate and SBS composite modified asphalt. Construction and Building Materials, 218, 413–423.
- Li, Y., Wu, S., and Amirkhanian, S., 2018c. Investigation of the graphene oxide and asphalt interaction and its effect on asphalt pavement performance. Construction and Building Materials, 165, 572–584.
- Liang, M., et al., 2017. Thermo-stability and aging performance of modified asphalt with crumb rubber activated by microwave and TOR. Materials & Design, 127, 84–96.
- Liang, Y., et al., 2019. Ultrastiff, tough, and healable ionic–hydrogen bond cross-linked hydrogels and their uses as building blocks To construct complex hydrogel structures. ACS Applied Materials & Interfaces, 11 (5), 5441–5454.
- Liu, K., Zhang, K., and Shi, X., 2018. Performance evaluation and modification mechanism analysis of asphalt binders modified by graphene oxide. Construction and Building Materials, 163, 880–889.
- MOT, 2019a. Standard test methods of bitumen and bituminous mixtures for highway engineering: JTG E20-2019. Ministry of Transport of the People's Republic of China.
- MOT, 2019b. T 0661-2019, standard test method of segregation test for polymer modified bitumen. Ministry of Transport of the People's Republic of China.
- Nallasamy, P., and Mohan, S., 2004. Vibrational spectra of cis-1.4-polyisoprene. Arabian Journal for Science and Engineering, 29, 17–26.
- Ouyang, C., et al., 2006. Low-density polyethylene/silica compound modified asphalts with high-temperature storage stability. Journal of Applied Polymer Science, 101 (1), 472–479.
- Safaei, F., and Castorena, C., 2017. Material nonlinearity in asphalt binder fatigue testing and analysis. Materials & Design, 133, 376–389.
- Sidess, A., and Uzan, J, 2009. A design method of perpetual flexible pavement in Israel. International Journal of Pavement Engineering, 10 (4), 241–249.
- Wang, C., et al., 2016. Identifying fatigue failure in asphalt binder time sweep tests. Construction & Building Materials, 121, 535–546.
- Wang, P., et al., 2017. Effect of multi-walled carbon nanotubes on the performance of styrene–butadiene–styrene copolymer modified asphalt. Materials & Structures, 50, 17.
- Wang, R., et al., 2021. Experimental study on mechanism, aging, rheology and fatigue performance of carbon nanomaterial/SBS-modified asphalt binders. Construction and Building Materials, 268, 121189.
- Wen, G., et al., 2002. Rheological characterisation of storage-stable SBS-modified asphalts. Polymer Testing, 21, 295–302.
- Xiao, F., et al., 2014. Rheological property investigations for polymer and polyphosphoric acid modified asphalt binders at high temperatures. Construction & Building Materials, 64, 316–323.
- Yanturina, R.A., Trofimov, B.Y., and Ahmedjanov, R.M., 2017. Structuring in Cement Systems with Introduction of Graphene Nano-Additivesed. Materials Science and Engineering Conference Series, 012017, 1–6.
- Zeng, W., et al., 2017. The Utilization of Graphene Oxide in Traditional Construction Materials: AsphaltThe Utilisation of Graphene Oxide in Traditional Construction Materials: Asphalt. Materials, 10, 48.
- Zhou, X., et al., 2017. Evaluation of thermo-mechanical properties of graphene/carbon-nanotubes modified asphalt with molecular simulation. Molecular Simulation, 43, 312–319.
- Zhu, C., Zhai, J., Wen, D. & Dong, S., 2012. Graphene oxide/polypyrrole nanocomposites: one-step electrochemical doping, coating and synergistic effect for energy storage. Journal of Materials Chemistry, 22, 6300-6306.
Appendix
Table S1. The price and cost of each material per ton.