ABSTRACT
This study aimed to clarify the kinematics, particularly of the shoulder and hip joints, during preparation for manual wheelchair-to-bed transfer (i.e. when flipping up the arm and foot supports). This cross-sectional study included 32 able-bodied individuals. The kinematics of the shoulder and hip joints when the arm and foot supports were flipped up of manual wheelchair, were evaluated using a markerless inertial sensor-based motion capture system. We found that flipping the arm support upwards involved a large amount of abduction, internal and external rotation, flexion, and extension at the shoulder joint, whereas flipping the foot support upwards involved a large amount of flexion at the hip joint. The findings suggest that it is necessary to consider the range of motion required to flip up the arm and foot supports of manual wheelchairs, particularly in those with limited shoulder and hip range of motion such as older people, neuromuscular disorders, and orthopedic disorders.
KEYWORDS:
Introduction
Most people with impaired mobility use a wheelchair to ambulate. Over 130 million people worldwide use wheelchairs (Wheelchair Foundation, Citationn.d.). The proportion of wheelchair users increases with age (Gell et al., Citation2015; Kaye et al., Citation2000), with 2.9% of wheelchair users being older people, which is nearly seven times the number of wheelchair users in the working-age population in the United States (Kaye et al., Citation2000). The proportions of wheelchair users were 1.6%, 2.0%, 4.0%, and 6.6% in those aged 65–69 years, 70–74 years, 75–84 years, and ≥85 years, respectively (Kaye et al., Citation2000).
For wheelchair users, the ability to transfer between a wheelchair and other surfaces is an essential skill for mobility and independence in activities of daily living. Studies have reported that wheelchair users perform transfers 12–19 times per day (Finley et al., Citation2005; Gagnon et al., Citation2008; Mulroy et al., Citation2015). Using a proper transfer technique to perform transfers is important for preventing falls and improving wheelchair users’ participation in home and community activities, long-term health, and well-being (Koontz et al., Citation2011; Mortenson et al., Citation2012). Proper transfer techniques indicate wheelchair setup and body setup techniques (Worobey et al., Citation2018, Citation2020). The process of transferring from a wheelchair to a suitable surface involves multiple movement components: propelling the wheelchair to approach the target surface, pre-transfer preparation, transfer, sitting down, and maintaining a stable posture (Kitamura et al., Citation2021).
Two fundamental movements are involved in preparation for transfer. First, the arm support on the side to which the person needs to transfer is flipped up as it constitutes an obstacle for the requisite movement, and it would require significant effort and uncomfortable movements to pivot the buttocks over the arm support (Koontz et al., Citation2016; Toro et al., Citation2013). Second, the foot supports are flipped up to enable placement of the feet on a stable surface for safe and easy transfer. Flipping up the arm and foot supports involves combined movements at various joints, particularly large joints such as the shoulder (for flipping up the arm support) and hip (for flipping up the foot supports). However, studies suggest that the range of motion (ROM) of the joints of older people and those with certain disorders is insufficient for these movements (Ballinger et al., Citation2000; Eriks-Hoogland et al., Citation2009; McKay et al., Citation2017; Roach & Miles, Citation1991; Stathokostas et al., Citation2013). Previous studies have focused on the kinematics of wheelchair propulsion, transferring, and sitting down (Cloud et al., Citation2017; Finley et al., Citation2005; Gagnon et al., Citation2008; Kim et al., Citation2015; Koontz et al., Citation2002). However, there are no studies on the kinematics of pre-transfer preparation. This study, therefore, aimed to evaluate the kinematics of shoulder and hip joints during pre-transfer preparation.
Objective
This study aimed to clarify the kinematics, particularly of the shoulder and hip joints, during the preparatory movements (i.e., flipping up the arm and foot supports) involved in transferring from a manual wheelchair to a bed.
Methods
Study design, setting, and participants
This study used a cross-sectional design with convenience sampling. Thirty-two able-bodied individuals without any history of medical illnesses, such as orthopedic, neurological, respiratory, cardiovascular, or psychological diseases, participated in this study. The demographics and anthropometric data of these participants are listed in . This study protocol was approved by the institutional ethics committee (approval no. HM21-226). All participants provided written informed consent prior to study participation. This study was performed in accordance with the principles of the Declaration of Helsinki and its later amendments. This study was completed in accordance with the STROBE guidelines.
Table 1. Demographics and anthropometric data of all participants.
Wheelchair used in the study
We used a standard manual wheelchair (NEO-1W, Nissin Medical Industries Co., Ltd., Aichi, Japan) with flip-up arm and foot supports and a quick-release mechanism for the arm supports (). The arm support could be manually flipped up after pulling a lever in front of the plug at the tip of the arm support. The foot supports could be manually flipped after lifting the feet off the foot supports. The dimensions of this wheelchair are listed in .
Figure 1. The quick-release mechanism of the arm supports of the wheelchair that was used in the study.
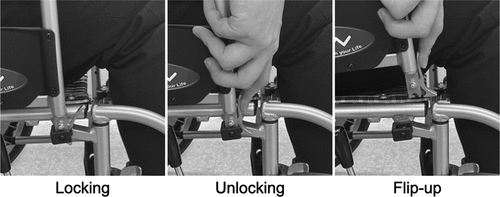
Table 2. The wheelchair dimensions.
Experimental procedures
We evaluated the kinematics of movements involved in preparation for transfer, focusing on flipping up the arm and foot supports.
The procedure for flipping up the arm support is shown in . As a starting position, participants sat in the wheelchair with their hands on their knees with the palms facing down, their feet on the foot supports, and their hips in neutral adduction/abduction and neutral rotation. First, participants unlocked the arm support by pulling the lever in front of the plug at the tip of the arm support with the right hand. Next, they flipped the arm support up while holding on to the lever. After flipping up the arm support, the participants returned their right hand to the starting position.
Figure 2. Procedure of flipping up the arm support.
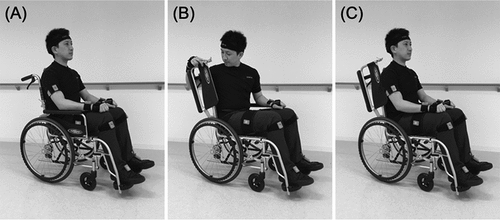
The procedure for flipping up the foot support is shown in . In this study, only the right foot support was flipped up. The starting position for this movement was the same as that for flipping up the arm support. First, participants bent their right knee from the starting position, lifted their right foot off the foot support, and placed it on the floor. Next, they held the end of the right foot support with their right hand and flipped up the foot support. After this, participants returned their right hand to the starting position.
Figure 3. Procedure of flipping up the foot support.
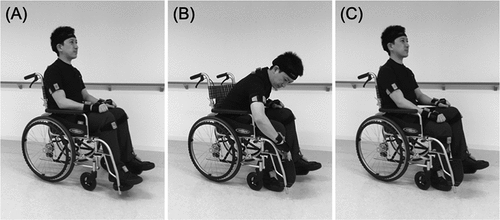
Participants practiced the flipping up of the arm and foot support three times before performing the single trial used in the present study.
Motion measurement
Xsens MVN Awinda (Xsens Technologies B.V., Enschede, The Netherlands), a markerless inertial sensor-based motion capture system, was used for motion measurement. This system was considered suitable for this study because the markers used with marker-based optical tracking systems are easily hidden by the wheelchair when flipping up the arm and foot supports. Several studies have previously reported on the validity and reliability of this system (Kasebzadeh et al., Citation2017; Kok et al., Citation2014; Starrs et al., Citation2012; Symonds et al., Citation2016). The Xsens MVN Awinda uses 17 wireless motion trackers to capture whole-body motion. These trackers are attached to the feet, lower legs, upper legs, pelvis, shoulders, sternum, head, upper arms, forearms, and hands. Data are wirelessly transmitted from each motion tracker to the Awinda Station with a sampling frequency of 60 Hz. This study used the calibration protocol with standing and walking modalities recommended by the product maker because the participants were able-bodied subjects. Specifically, prior to the measurement, the alignment between the corresponding sensors was calibrated by asking the participant to stand still and then walk a few meters back and forth for some time.
Data and statistical analyses
Changes in the joint angles of the right upper and lower limbs and trunk during flipping up of the arm and foot supports were calculated in the following joints: shoulder (adduction, abduction, internal rotation, external rotation, flexion, extension), elbow (flexion, extension), trunk (lateral flexion, rotation, flexion, extension of C7–T1, T8–T9, and L3–L4), hip (adduction, abduction, internal rotation, external rotation, flexion, extension), and knee (flexion, extension). To determine the starting time point of shoulder joint movement of the flip-up motion of the arm support, it was defined as the time point when the shoulder joint angle exceeded the range that occurs at rest. The range at rest was defined as mean ±2 standard deviations (SD) of angles occurring at rest. Since the shoulder joint motion occurs in three directions (adduction-abduction, internal-external rotation, and flexion-extension), the starting time point was defined as the time point when the range was exceeded in at least one of the three directions. The ending time point of the shoulder joint movement of the flip-up motion of the arm support was defined as the time point when the shoulder joint angle returned to within the range observed at rest. The starting and ending time point of the hip joint movement of the flip-up motion of the foot support were defined similarly to those of the shoulder joint.
All individual joint angle data have been presented as mean (SD).
Results
Flipping up the arm support
A typical example of the sequence of changes in the shoulder joint angles during various shoulder movements when flipping up the arm support is shown in . First, abduction, internal rotation, and flexion occurred at the shoulder joint until the lever of the arm support was grasped. Subsequently, abduction, external rotation, and extension occurred at the shoulder joint until the arm support was completely flipped up. During this movement, the angles of shoulder external rotation and extension reached their maximum values, with mean (SD) values of 65.4° (14.8°) and 32.5° (14.4°), respectively. Finally, adduction, internal rotation, and flexion occurred at the shoulder joint until the participant returned to the starting position. During this movement, the angles of shoulder abduction, internal rotation, and flexion reached their maximum values, with mean (SD) values of 79.5° (8.6°), 55.5° (15.5°), and 90.8° (17.6°), respectively. The data of all joint angles when flipping up the arm support are listed in . In addition, the data of angular velocity and angular acceleration of the shoulder joint, which showed a large range of motion when flipping up the arm support, are shown in .
Figure 4. A typical example of the sequence of changes in the shoulder joint angles during various shoulder movements when flipping up the arm support.
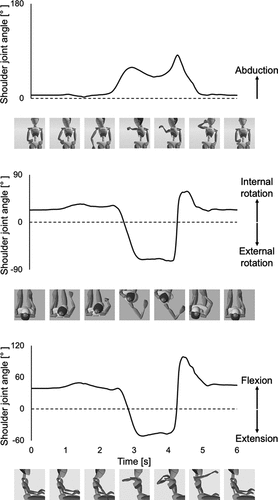
Table 3. Maximum values of each joint angle when flipping up the arm support.
Table 4. Maximum value of shoulder joint angular velocity and angular acceleration when flipping up the arm support.
Flipping up the foot support
A typical example of the sequence of changes in the hip joint angles during various hip movements when flipping up the foot support is shown in . First, internal rotation and flexion occurred at the hip joint until the foot support was completely flipped up. During this movement, the angles of hip internal rotation and flexion reached their maximum values, with mean (SD) values of 10.7° (6.8°) and 96.3° (9.2°), respectively. Next, external rotation and extension occurred at the hip joint until the participant returned to the starting position. The data of all joint angles when flipping up the foot support are listed in . In addition, the data of angular velocity and angular acceleration of the hip joint, which showed a large range of motion when flipping up the foot support, are shown in .
Figure 5. A typical example of the sequence of changes in the hip joint angles during various hip movements when flipping up the foot support.
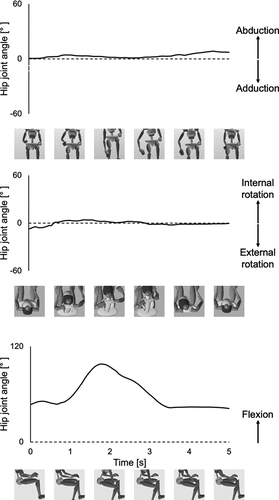
Table 5. Maximum values of each joint angle when flipping up the foot support.
Table 6. Maximum values of hip joint angular velocity and angular acceleration when flipping up the foot support.
Discussion
This study examined the kinematics during preparation for manual wheelchair-to-bed transfer with a focus on the movements involved in flipping up the arm and foot support. A considerable degree of abduction, internal and external rotation, flexion, and extension is needed at the shoulder joint for flipping up the arm support. In contrast, for flipping up the foot support, a large amount of flexion is required at the hip joint.
Those with limited shoulder ROM, such as older people, may find it challenging to flip up the manual wheelchair arm support. Advancing age has been associated with decreasing shoulder joint ROM. Gill et al. (Citation2020) investigated the ROM of active shoulder flexion and abduction. They found that the mean ROM of shoulder flexion was approximately 170° in individuals aged 20–24 years, 150° in those aged 65–69 years, and < 130° in those aged ≥85 years, whereas the mean ROM of shoulder abduction was approximately 160° in individuals aged 20–24 years, 140° in those aged 65–69 years, and < 120° in those aged ≥85 years. In addition, routinely and repeatedly flipping up the arm support might worsen shoulder joint ROM in older people with impaired ROM. This motion involves arm elevation almost to the height of the head. Studies have suggested that performing repetitive near-overhead movements is a risk factor for shoulder disorders (Harkness et al., Citation2003). In addition, for individuals with paraplegia and tetraplegia, the flip-up motion of wheelchair arm supports might be more difficult, despite its importance. They frequently use sitting pivot transfer from a wheelchair seat to the desired surface in daily life (Bromley, Citation2006). The flip-up motion of the arm support on a wheelchair is critical to safety perform sitting pivot transfer (Worobey et al., Citation2018, Citation2020). Nevertheless, those with paraplegia and tetraplegia have a higher rate of shoulder pain (Akbar et al., Citation2015; Bossuyt et al., Citation2018; Larsen et al., Citation2021; Richardson et al., Citation2021; Widerström-Noga et al., Citation2001) and limited range of motion of the shoulder joint (Ballinger et al., Citation2000).
Those with limited hip ROM, such as older people and individuals with orthopedic disorders, may find it challenging to flip up the manual wheelchair foot support. Significant hip joint motion (especially hip flexion) was required for flipping up the foot support. A study that investigated the ROM of various joints in 436 community-dwelling individuals (205 men, mean age 70 years; 231 women, mean age 71 years) reported maximum hip flexion angles of 114° for men and 102° for women, and both groups showed a similarly significant age-related decline in hip flexion (men: 6° per decade; women: 7° per decade) (Stathokostas et al., Citation2013). In patients with orthopedic conditions, such as those who have undergone hip arthroplasty, the hip joint ROM is limited postoperatively, and there is a risk of dislocation. Dislocation is one of the most common major complications of total hip replacement (Bozic et al., Citation2009). A previous study indicated that the kinetics and kinematics of dislocation-prone motion are that the center of gravity of the upper body is shifted significantly anteriorly to the center of the hip joint. Consequently, a high force output from the hip extensor muscles is required to maintain the sagittal plane equilibrium (Nadzadi et al., Citation2003). These problems might become more apparent because of the aging patient population with hip fractures, the underlying disease for total hip replacement (Dhanwal et al., Citation2011; Marks, Citation2010; Moon et al., Citation2011).
The findings of this study are significant and useful for clinical practice. Understanding the joint angles required for transfer preparation movements is useful as part of goal-oriented rehabilitation for new manual wheelchair users. Previous studies reported the need for quantitative measurement of shoulder and hip joint angles during pre-transfer preparation. Specifically, elucidating the relationship between the difficulty of subtasks comprising transfer and a patient’s physical and cognitive function is likely to contribute to establishing strategies for effective rehabilitation (Kitamura et al., Citation2022). Some wheelchair users are unable to achieve the required joint angles, and in such cases, users try to apply compensatory movements. Compensatory movements are defined as movements that are compensated for by functions other than those required for the original movement (Cirstea & Levin, Citation2000; Kasten et al., Citation2009). Our study findings may provide reference values for healthcare professionals when considering whether a user needs to perform compensatory movements. Specifically, they can assess whether the range of motion involved in the main joint of the movement is sufficient. A previous study reported that upper extremity reaching movements are compensated for with trunk movements (Cirstea & Levin, Citation2000).
Our findings are also useful when considering the novel design and development of assistive devices. Recently, a novel mechanisms related to transfer preparation movements has been proposed. Previous studies have reported mechanisms in which the arm support at the transfer side is lowered manually and electrically to the level of the wheelchair seat during transfer; foot supports are moved to the floor level during transfer without requiring manual operation (Gao et al., Citation2021; Tatemoto et al., Citation2020; Tee et al., Citation2021). Our findings might be collateral evidence that the direction of their development is correct. Although their feasibility, usability, and effectiveness will need to be verified in future research, the demand for these novel mechanisms will increase in the aging society.
This study had some limitations. First, to obtain the underlying data concerning transfer preparation for manual wheelchair users, we recruited healthy participants. Flipping up the arm and foot supports involves combined movements at various joints, with shoulder and hip joints requiring relatively large movements. However, previous studies have suggested that the ROM in these joints in older people and in those with certain disorders may not be sufficient for these movements (Ballinger et al., Citation2000; Eriks-Hoogland et al., Citation2009; McKay et al., Citation2017; Roach & Miles, Citation1991; Stathokostas et al., Citation2013). Future research is warranted to investigate the differences in kinematics between healthy young people, older people, and individuals with limited joint ROM. Second, we standardized their sitting posture. The sitting posture of patients is known to vary. For example, sitting in a slouched position decreases glenohumeral abduction strength, whereas sitting in an upright posture increases glenohumeral forward flexion (Kanlayanaphotporn, Citation2014; Kebaetse et al., Citation1999). This point should be clarified to the effect of differences in sitting postures. Third, we used a standard manual wheelchair with flip-up arm and foot supports and did not use electric wheelchairs in this study. Kinematic data may change depending on the type of wheelchair (seat shape and cushion softness). Previous studies have reported that the specifications of manual wheelchairs have a certain level of variability (Paul et al., Citation2022; Puspitasari et al., Citation2021). From another perspective, in a previous study of 897,000 older wheelchair users, 864,000 were found to use manual wheelchairs, and 47,000 used electric wheelchairs (multiple answers allowed) (Kaye et al., Citation2000). This finding is not applicable to electric wheelchairs because electric wheelchairs tend to have different specifications compared with manual wheelchairs. Further studies should be conducted to determine the effect of differences in types of wheelchairs.
Conclusions
A significant degree of abduction, internal and external rotation, flexion, and extension at the shoulder joint is needed to flip up a manual wheelchair arm support. Similarly, a large amount of flexion at the hip joint is required to flip up a manual wheelchair foot support. Therefore, it is necessary to consider the ROM required to flip up the arm and foot supports, particularly in individuals with limited shoulder and hip ROM, such as older people and those with neuromuscular and orthopedic disorders.
Supplemental Material
Download PDF (102.2 KB)Acknowledgements
We thank the staff of the Department of Rehabilitation at Fujita Health University Hospital and the students of the Faculty of Rehabilitation, School of Health Sciences, Fujita Health University, Akane Hyuga, Haruka Hoshimoto, and Chika Momohira for their contribution to data acquisition for this study.
Disclosure statement
No potential conflict of interest was reported by the author(s).
Data availability statement
The data that support the findings of this study are available from the corresponding author upon reasonable request.
Supplementary material
Supplemental data for this article can be accessed online at https://doi.org/10.1080/10400435.2024.2315410.
Additional information
Funding
References
- Akbar, M., Brunner, M., Ewerbeck, V., Wiedenhöfer, B., Grieser, T., Bruckner, T., Loew, M., & Raiss, P. (2015). Do overhead sports increase risk for rotator cuff tears in wheelchair users? Archives of Physical Medicine and Rehabilitation, 96(3), 484–488. https://doi.org/10.1016/j.apmr.2014.09.032
- Ballinger, D. A., Rintala, D. H., & Hart, K. A. (2000). The relation of shoulder pain and range-of-motion problems to functional limitations, disability, and perceived health of men with spinal cord injury: A multifaceted longitudinal study. Archives of Physical Medicine and Rehabilitation, 81(12), 1575–1581. https://doi.org/10.1053/apmr.2000.18216
- Bossuyt, F. M., Arnet, U., Brinkhof, M. W. G., Eriks-Hoogland, I., Lay, V., Müller, R., Sunnåker, M., Hinrichs, T., & SwiSCI Study Group. (2018). Shoulder pain in the Swiss spinal cord injury community: Prevalence and associated factors. Disability and Rehabilitation, 40(7), 798–805.
- Bozic, K. J., Kurtz, S. M., Lau, E., Ong, K., Vail, T. P., & Berry, D. J. (2009). The epidemiology of revision total hip arthroplasty in the United States. Journal of Bone and Joint Surgery, 91(1), 128–133. https://doi.org/10.2106/JBJS.H.00155
- Bromley, I. (2006). Tetraplegia and paraplegia: A guide for physiotherapists. Elsevier Health Sciences.
- Cirstea, M. C., & Levin, M. F. (2000). Compensatory strategies for reaching in stroke. Brain: A Journal of Neurology, 123(5), 940–953. https://doi.org/10.1093/brain/123.5.940
- Cloud, B. A., Zhao, K. D., Ellingson, A. M., Nassr, A., Windebank, A. J., & An, K. N. (2017). Increased seat dump angle in a manual wheelchair is associated with changes in thoracolumbar lordosis and scapular kinematics during propulsion. Archives of Physical Medicine and Rehabilitation, 98(10), 2021–2027. https://doi.org/10.1016/j.apmr.2017.02.014
- Dhanwal, D. K., Dennison, E. M., Harvey, N. C., & Cooper, C. (2011). Epidemiology of hip fracture: Worldwide geographic variation. Indian Journal of Orthopaedics, 45(1), 15–22. https://doi.org/10.4103/0019-5413.73656
- Eriks-Hoogland, I. E., de Groot, S., Post, M. W., & van der Woude, L. H. (2009). Passive shoulder range of motion impairment in spinal cord injury during and one year after rehabilitation. The Japanese Journal of Rehabilitation Medicine, 41(6), 438–444. https://doi.org/10.2340/16501977-0355
- Finley, M. A., McQuade, K. J., & Rodgers, M. M. (2005). Scapular kinematics during transfers in manual wheelchair users with and without shoulder impingement. Clinical Biomechanics, 20(1), 32–40. https://doi.org/10.1016/j.clinbiomech.2004.06.011
- Gagnon, D., Nadeau, S., Noreau, L., Eng, J. J., & Gravel, D. (2008). Trunk and upper extremity kinematics during sitting pivot transfers performed by individuals with spinal cord injury. Clinical Biomechanics, 23(3), 279–290. https://doi.org/10.1016/j.clinbiomech.2007.09.017
- Gao, H., Tang, Y., Wang, Z., & Wu, Q. (2021). Design of a multifunctional nursing wheelchair. Journal of Physics: Conference Series, 1885(5), 052044. https://doi.org/10.1088/1742-6596/1885/5/052044
- Gell, N. M., Wallace, R. B., LaCroix, A. Z., Mroz, T. M., & Patel, K. V. (2015). Mobility device use in older adults and incidence of falls and worry about falling: Findings from the 2011-2012 national health and aging trends study. Journal of the American Geriatrics Society, 63(5), 853–859. https://doi.org/10.1111/jgs.13393
- Gill, T. K., Shanahan, E. M., Tucker, G. R., Buchbinder, R., & Hill, C. L. (2020). Shoulder range of movement in the general population: Age and gender stratified normative data using a community-based cohort. BMC Musculoskeletal Disorders, 21(1), 1–9. https://doi.org/10.1186/s12891-020-03665-9
- Harkness, E. F., Macfarlane, G. J., Nahit, E. S., Silman, A. J., & McBeth, J. (2003). Mechanical and psychosocial factors predict new onset shoulder pain: A prospective cohort study of newly employed workers. Occupational and Environmental Medicine, 60(11), 850–857. https://doi.org/10.1136/oem.60.11.850
- Kanlayanaphotporn, R. (2014). Changes in sitting posture affect shoulder range of motion. Journal of Bodywork and Movement Therapies, 18(2), 239–243. https://doi.org/10.1016/j.jbmt.2013.09.008
- Kasebzadeh, P., Hendeby, G., Fritsche, C., Gunnarsson, F., & Gustafsson, F. (2017). IMU dataset for motion and device mode classification. In 2017 International Conference on Indoor Positioning and Indoor Navigation (IPIN) (pp. 1–8). https://doi.org/10.1109/IPIN.2017.8115956
- Kasten, P., Rettig, O., Loew, M., Wolf, S., & Raiss, P. (2009). Three-dimensional motion analysis of compensatory movements in patients with radioulnar synostosis performing activities of daily living. Journal of Orthopaedic Science, 14(3), 307–312. https://doi.org/10.1007/s00776-009-1332-0
- Kaye, H. S., Kang, T., & LaPlante, M. P. (2000). Mobility device use in the United States. Disability statistics report (Report No. 14). U.S. Department of Education, National Institute on Disability and Rehabilitation Research.
- Kebaetse, M., McClure, P., & Pratt, N. A. (1999). Thoracic position effect on shoulder range of motion, strength, and three-dimensional scapular kinematics. Archives of Physical Medicine and Rehabilitation, 80(8), 945–950. https://doi.org/10.1016/s0003-9993(99)90088-6
- Kim, S. S., Her, J. G., & Ko, T. S. (2015). Effect of different hand positions on trunk and shoulder kinematics and reaction forces in sitting pivot transfer. Journal of Physical Therapy Science, 27(7), 2307–2311. https://doi.org/10.1589/jpts.27.2307
- Kitamura, S., Otaka, Y., Murayama, Y., Ushizawa, K., Narita, Y., Nakatsukasa, N., Kondo, K., & Sakata, S. (2021). Reliability and validity of a new transfer assessment form for stroke patients. PM&R, 13(3), 282–288. https://doi.org/10.1002/pmrj.12400
- Kitamura, S., Otaka, Y., Murayama, Y., Ushizawa, K., Narita, Y., Nakatsukasa, N., Matsuura, D., Kondo, K., & Sakata, S. (2022). Difficulty of the subtasks comprising bed-wheelchair transfer in patients with subacute strokes: A cohort study. Journal of Stroke and Cerebrovascular Diseases, 31(10), 106740. https://doi.org/10.1016/j.jstrokecerebrovasdis.2022.106740
- Kok, M., Hol, J. D., & Schӧn, T. B. (2014). An optimization-based approach to human body motion capture using inertial sensors. IFAC Proceedings Volumes, 47(3), 79–85. https://doi.org/10.3182/20140824-6-ZA-1003.02252
- Koontz, A. M., Cooper, R. A., Boninger, M. L., Souza, A. L., & Fay, B. T. (2002). Shoulder kinematics and kinetics during two speeds of wheelchair propulsion. Journal of Rehabilitation Research and Development, 39(6), 635–649.
- Koontz, A. M., Kankipati, P., Lin, Y. S., Cooper, R. A., & Boninger, M. L. (2011). Upper limb kinetic analysis of three sitting pivot wheelchair transfer techniques. Clinical Biomechanics, 26(9), 923–929. https://doi.org/10.1016/j.clinbiomech.2011.05.005
- Koontz, A. M., Tsai, C. Y., Hogaboom, N. S., & Boninger, M. L. (2016). Transfer component skill deficit rates among veterans who use wheelchairs. Journal of Rehabilitation Research and Development, 53(2), 279–294. https://doi.org/10.1682/JRRD.2015.02.0023
- Larsen, C. M., Juul-Kristensen, B., Kasch, H., Hartvigsen, J., Frich, L. H., Boyle, E., Østengaard, L., & Biering-Sørensen, F. (2021). The Danish spinal cord injury shoulder (DanSCIS) cohort: Methodology and primary results. Spinal Cord, 59(7), 821–831. https://doi.org/10.1038/s41393-020-00594-1
- Marks, R. (2010). Hip fracture epidemiological trends, outcomes, and risk factors, 1970-2009. International Journal of General Medicine, 3, 1–17. https://doi.org/10.2147/IJGM.S5906
- McKay, M. J., Baldwin, J. N., Ferreira, P., Simic, M., Vanicek, N., Burns, J., Baldwin, J., McKay, M., Ferreira, P., Nightingale, E., Pourkazemi, F., Sman, A., Hiller, C., Mousavi, S., Nicholson, L., Rose, K., Chard, A., Mackey, M., … 1000 Norms Project Consortium. (2017). Normative reference values for strength and flexibility of 1,000 children and adults. Neurology, 88(1), 36–43. https://doi.org/10.1212/WNL.0000000000003466
- Moon, A., Gray, A., & Deehan, D. (2011). Neck of femur fractures in patient’s aged more than 85 years-are they a unique subset? Geriatric Orthopaedic Surgery & Rehabilitation, 2(4), 123–127. https://doi.org/10.1177/2151458511414562
- Mortenson, W. B., Miller, W. C., Backman, C. L., & Oliffe, J. L. (2012). Association between mobility, participation, and wheelchair-related factors in long-term care residents who use wheelchairs as their primary means of mobility. Journal of the American Geriatrics Society, 60(7), 1310–1315. https://doi.org/10.1111/j.1532-5415.2012.04038.x
- Mulroy, S. J., Hatchett, P., Eberly, V. J., Haubert, L. L., Conners, S., & Requejo, P. S. (2015). Shoulder strength and physical activity predictors of shoulder pain in people with paraplegia from spinal injury: Prospective cohort study. Physical Therapy, 95(7), 1027–1038. https://doi.org/10.2522/ptj.20130606
- Nadzadi, M. E., Pedersen, D. R., Yack, H. J., Callaghan, J. J., & Brown, T. D. (2003). Kinematics, kinetics, and finite element analysis of commonplace maneuvers at risk for total hip dislocation. Journal of Biomechanics, 36(4), 577–591. https://doi.org/10.1016/s0021-9290(02)00232-4
- Paul, G., Steffan, I. T., Itoh, N., Bowman, R., & Bradtmiller, B. (2022). Design for all – design for disabled: How important is anthropometry? Work (Reading, Mass), 73(s1), S57–S65. https://doi.org/10.3233/WOR-211106
- Puspitasari, N. B., Putra, R. P., Ismail, R., & Prastawa, H. (2021). Implementation of user-centered design (UCD) method in product development of traveling wheelchair. Journal of Physics, Conference Series, 1858(1), 012036. https://doi.org/10.1088/1742-6596/1858/1/012036
- Richardson, A., Samaranayaka, A., Sullivan, M., & Derrett, S. (2021). Secondary health conditions and disability among people with spinal cord injury: A prospective cohort study. The Journal of Spinal Cord Medicine, 44(1), 19–28. https://doi.org/10.1080/10790268.2019.1581392
- Roach, K. E., & Miles, T. P. (1991). Normal hip and knee active range of motion: The relationship to age. Physical Therapy, 71(9), 656–665. https://doi.org/10.1093/ptj/71.9.656
- Starrs, P., Chohan, A., Fewtrell, D., Richards, J., & Selfe, J. (2012). Biomechanical differences between experienced and inexperienced wheelchair users during sport. Prosthetics and Orthotics International, 36(3), 324–331. https://doi.org/10.1177/0309364612448807
- Stathokostas, L., McDonald, M. W., Little, R. M., & Paterson, D. H. (2013). Flexibility of older adults aged 55-86 years and the influence of physical activity. Journal of Aging Research, 2013, 743–843. https://doi.org/10.1155/2013/743843
- Symonds, A., Holloway, C., Suzuki, T., Smitham, P., Gall, A., & Taylor, S. J. (2016). Identifying key experience-related differences in over-ground manual wheelchair propulsion biomechanics. Journal of Rehabilitation and Assistive Technologies Engineering, 3, 2055668316678362. https://doi.org/10.1177/2055668316678362
- Tatemoto, T., Saitoh, E., Tanabe, S., Koyama, S., Kumazawa, N., Furuzawa, S., Kato, T., Yoshimuta, H., Torii, K., Kiyono, K., Otaka, Y., & Kanada, Y. (2020). Lateral transfer assist robot (LTAR): Development of a proof-of-concept prototype. Technology and Health Care, 28(2), 175–183. https://doi.org/10.3233/THC-191762
- Tee, K. S., Yazid, M. A. Y. B., Low, E., Mohamad, E. J., Soom, C. F., Sam, T. H., & Pusppanathan, J. B. (2021). Development of a wheelchair lifting system with height levelling and side transfer. Journal of Tomography System and Sensor Application, 4(1), 50–56. http://www.tssa.my/index.php/jtssa/article/view/149
- Toro, M. L., Koontz, A. M., & Cooper, R. A. (2013). The impact of transfer setup on the performance of independent wheelchair transfers. Human Factors: The Journal of the Human Factors & Ergonomics Society, 55(3), 567–580. https://doi.org/10.1177/0018720812460549
- Wheelchair Foundation. (n.d.). Worldwide need: Wheelchair needs in the world. https://www.wheelchairfoundation.org/fth/analysis-of-wheelchair-need/
- Widerström-Noga, E. G., Felipe-Cuervo, E., & Yezierski, R. P. (2001). Relationships among clinical characteristics of chronic pain after spinal cord injury. Archives of Physical Medicine and Rehabilitation, 82(9), 1191–1197. https://doi.org/10.1053/apmr.2001.25077
- Worobey, L. A., Rigot, S. K., Boninger, M. L., Huzinec, R., Sung, J. H., DiGiovine, K., & Rice, L. A. (2020). Concurrent validity and reliability of the transfer assessment instrument questionnaire as a self-assessment measure. Archives of Rehabilitation Research and Clinical Translation, 2(4), 1–8. https://doi.org/10.1016/j.arrct.2020.100088
- Worobey, L. A., Zigler, C. K., Huzinec, R., Rigot, S. K., Sung, J., & Rice, L. A. (2018). Reliability and validity of the revised transfer assessment instrument. Topics in Spinal Cord Injury Rehabilitation, 24(3), 217–226. https://doi.org/10.1310/sci2403-217