Abstract
The Gilgel Gibe reservoir, which was built to fulfill the energy demand and the electricity export ambitions of Ethiopia, was characterized by low macroinvertebrate diversity mainly due to water level fluctuations, trampling of the littoral zone by cattle, nutrient input, and siltation from the catchment flow. The downstream river system was also affected by the dam due to a strongly decreased discharge as the water is diverted to Gilgel Gibe II hydropower plant through a 25 km underground tunnel. The study was based on seasonal macroinvertebrate sampling, water quality analyses, measurement of daily water level fluctuation, and recording of major anthropogenic activities. Hierarchical cluster analysis and canonical correspondence analysis were performed to determine relationships between the macroinvertebrate community composition and the environmental variables in the reservoir. The cluster analysis, Kruskal-Wallis ANOVA, and MANOVA permutation tests, revealed that differences in physicochemical water quality parameters and ecological quality metrics based on macroinvertebrates existed between the samples taken during periods with low, medium, and high water level seasons. Remedial actions could be important at basin and reservoir level to solve the identified problems and to improve the ecological water quality.
In Ethiopia, a considerable number of dams are under construction to fulfill the energy demand of the country and its electricity export ambitions. Currently, there are 9 operational dams, and an additional 4 (Beles, Amerti-Neshi, Gilgel Gibe III, and Grand Renaissance Dam) are under construction.
The dynamics and structures of reservoirs present a pattern of organization between those of rivers and lakes (Calisto et al. Citation2005). In addition, ecological processes in the artificial lakes or reservoirs are much more complex and variable than those found in natural lakes (Agostinho and Gomes Citation1997), partly due to succession processes taking place after the development of the reservoir. Reservoirs are mainly influenced by the physicochemical and biological characteristics of their tributaries and by the land use pattern in the drainage basin (Torloni Citation1994). Age of a reservoir also plays a fundamental role on the diversity of macroinvertebrates community (Meiling et al. Citation2007, Jorcin and Nogueira Citation2008). Such influences could be systematically assessed using organisms like macroinvertebrates.
Macroinvertebrate communities can be used to assess existing hydrological and anthropogenic influences on a given reservoir. Specifically, some macroinvertebrate groups are important in the littoral zone, where they are supposed to control the biomass of algae (James et al. Citation2000; Martinez-Sanz et al. Citation2012), recycle detritus Kornijow et al. Citation1995) and provide a critical link in the food chain (Graynoth et al. Citation1986). Species composition and abundance of macroinvertebrates depend on multiple environmental factors (Weatherhead and James Citation2001). Macroinvertebrate communities in the littoral zone also vary due to water level fluctuations (Hynes Citation1961). The vegetation in the littoral zone of reservoirs plays an important role for macroinvertebrate communities by providing protection from predators and excessive water current and serving as a food source (Poznanska et al. Citation2009); however, young reservoirs can have poor macroinvertebrate communities (Meiling et al. Citation2007, Jorcin and Nogueira Citation2008).
The main aim of the present study was to determine factors affecting macroinvertebrates community composition in newly built reservoirs, using the Gilgel Gibe I reservoir as a case study, to improve the management strategy of this and similar reservoirs. In addition, the effect of the reservoir on the downstream Gilgel Gibe River was investigated to provide guidance on sustainable use of rivers for hydropower generation.
Table 1 Summary statistics of the water quality parameters in the Gilgel Gibe reservoir from 2006 to 2008 (FTU = Formazin Turbidity Units, DO = dissolved oxygen, BOD5 = 5-day biological oxygen demand, Min = minimum, Max = maximum, STDV = standard deviation and CI = confidence interval).
Study site
The Gilgel Gibe I reservoir, which only recently become operational, is located in southwest Ethiopia in the Gilgel Gibe River basin (). This reservoir is one of the first cascading dams built on the Omo-Gibe river basin. Gilgel Gibe II has no dam but receives water from Gilgel Gibe I after passing through a 25 km under-hill tunnel in the downstream side. Gilgel Gibe III (also called Gibe III) is located downstream from Gilgel Gibe II, a 243 m high dam. Upon completion, Gibe III will be the first largest hydroelectric plant in Africa with power output of about 1870 Megawatt (EEPCo Citation2012). Gilgel Gibe IV and V dams are also planned after the completion of the Gilgel Gibe III dam.
Gilgel Gibe I dam construction started in 1986 and was finished in 2004. This reservoir is designed for a live storage of 657 million m3 and a dead storage of 182 million m3 water. It operates at reservoir water levels between 1653 and 1671 m a.s.l. and has an average inflow of 50 m/s (Pietrangeli and Pallavicini Citation2007). Before the construction of the Gilgel Gibe I dam, reservoir area was comprised of farmland, grazing land, and bush land. The theoretical buffer zone around the reservoir is 0.5 to 1 km wide; nonetheless, the buffer area is prone to intensive grazing and tillage (authors’ observation). The maximum area of the reservoir covers about 60 km2, which would occur when the water reaches nearly 1671 m a.s.l. At normal operating schedules, the reservoir area is estimated to cover 50 km2 (MME-EELPA Citation1997). According to Jabbar et al. (Citation2007), the Gilgel Gibe area is known for high cattle densities (201–400 cattle/km2).
Materials and methods
Environmental and macroinvertebrate data were collected at 4 sites in the Gilgel Gibe I reservoir from 2006 to 2008. From each site, 2 samples were collected in each year to total 24 samples taken from the reservoir. In addition, to assess the effect of damming on the Gilgel Gibe River, environmental and macroinvertebrate data (8 samples) were also obtained from 2 sites, one 5 km upstream from the reservoir and one 0.4 km downstream from the dam. Macroinvertebrates were collected using the kick-sampling method in the littoral zone starting from 1 m depth toward the edge of the reservoir. Animals were sorted alive and preserved in 70% ethanol. In the laboratory, macroinvertebrates were identified to family level using a 10–40× dissecting microscope with a fiberoptics light source (US EPA Citation2004).
The family level biotic index (FBI) was calculated based on the formula FBI = (Σni ti)/N, where ni is abundance of taxon i, ti is the tolerance value of taxon i, and N is the abundance of all macroinvertebrates together (Hilsenhoff Citation1988). The Biological Monitoring Working Party (BMWP) index at each sampling site was calculated by summing the values of the sensitivity scores given to each macroinvertebrate family (Armitage et al. Citation1983).
Physicochemical parameters including temperature and conductivity (Knick Portamess 911 conductivity meter), pH (Knick Portamess 911 pH meter), oxygen saturation (Knick Portamess 911 oxygen probe), and turbidity (portable Wagtech turbidity meter) were measured onsite at each sampling location. The 5-day biochemical oxygen demand (BOD5), nitrate–nitrogen (described as nitrate), ammonium–nitrogen (described as ammonium), and orthophosphate phosphorus (described as phosphate) were measured in the laboratory according to standard methods (APHA-AWWA-WPCF Citation1995; ). Daily reservoir water levels from January 2005 to December 2008 were obtained from the Ethiopian Electric Power Corporation (EEPCo 2012).
Figure 2 Water level in the Gilgel Gibe reservoir from 2005 to 2008. The bar along the water level graph represents the box and whisker plot. Whiskers indicate 95% confidence interval, the box indicates inter quartile range, and the bar inside the box indicates mean water level.
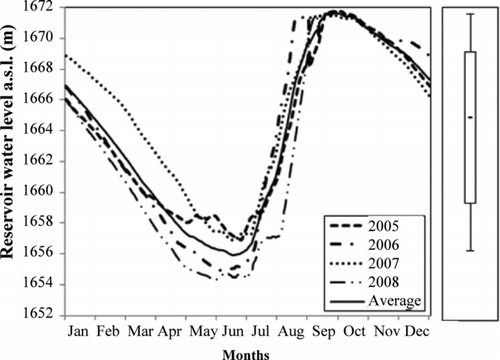
Hierarchical cluster analysis was performed to determine similarities between macroinvertebrate samples collected during different seasons and at different sites. Canonical correspondence analysis (CCA) was performed to determine relationships between the macroinvertebrate community composition and the environmental variables in the reservoir. Kruskal-Wallis ANOVA and MANOVA permutation tests were performed to assess differences in water quality parameters and macroinvertebrate metrics among low, medium, and high water levels and between the sites upstream and downstream from the reservoir.
Table 2 Summary statistics of macroinvertebrates in the Gilgel Gibe reservoir from 2006 to 2008 (Min = minimum, Max = maximum, STDV = standard deviation, and CI = confidence interval).
Results
Seasonal fluctuation of the water level in the reservoir
The Gilgel Gibe reservoir water level fluctuates depending on the season. In the rainy season, the water level of the dam starts to increase and reaches a maximum in September (); from the end of September, the water level starts to decline because of decreased river discharges due to low rainfall and the use of the reservoir water to operate the 3 turbines for electricity production. The first quartile (), the interquartile, and the third quartile ranges of the average water level were 1657–1660, 1660–1669, and 1669–1672 m a.s.l., termed as low, medium, and high, respectively (). At the end of the rainy season (Sep and Oct), livestock did not enter the littoral zone for drinking water because they could easily find water closer to the grazing fields.
Water quality in the reservoir
Kruskal-Wallis ANOVA () indicated that temperature, turbidity, conductivity, dissolved oxygen (DO), ammonium, and phosphate in the reservoir significantly differed between low, medium, and high water levels (p < 0.05). Ammonium and phosphate concentrations increased with decreasing reservoir water level. DO was higher at high and low reservoir water levels and lower during intermediate water levels. Other water quality parameters such as pH, BOD, and nitrate did not differ significantly between different water levels. MANOVA permutations also indicated that multivariate means of the water quality parameters significantly differed between low, medium, and high water levels (p < 0.001).
Macroinvertebrate communities in the reservoir
In the Gilgel Gibe I reservoir, 20 macroinvertebrate taxa were detected (). Chironomidae were present in all samples, and Oligochaeta were common in the samples collected when the reservoir water level was low. Among the Ephemeroptera, Plecoptera, and Trichoptera (EPT) taxa, only the Ephemeroptera taxon was present during high water levels. Coleoptera and Hemiptera were also more common during high water levels. In general, the maximum taxa richness was registered when the water level was high. The values of the ecological quality metrics based on the macroinvertebrates present in the Gilgel Gibe I reservoir were summarized (). From the cluster analysis of macroinvertebrate data (), 3 major clusters could be distinguished, which corresponded with the water level of the reservoir.
Table 3 Summary statistics of macroinvertebrate metrics in the Gilgel Gibe reservoir (Min = minimum, Max = maximum, STDV = standard deviation, CI = confidence interval, % E = percentage of Ephemeroptera, BMWP = biological monitoring working party, FBI = family level biotic index).
Figure 3 Bray-Curtis dendrogram of hierarchical cluster analysis of the macroinvertebrate communities collected in the Gilgel Gibe reservoir from 2006 to 2008.
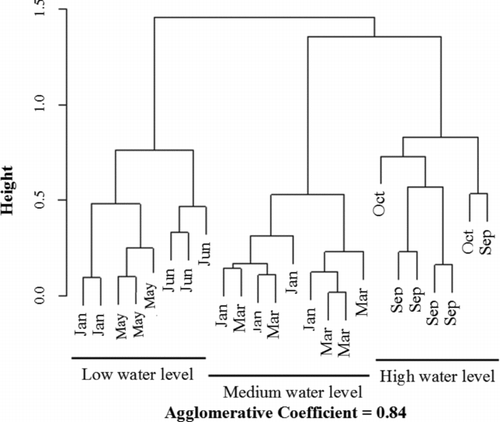
The first and the second axes of the CCA biplot explained 52% of the variability in the dataset (). The correlation coefficients (R) between macroinvertebrate communities and environmental variables in the first and second axes on the CCA were, respectively, 0.982 and 0.959. Macroinvertebrate samples collected in January and March were strongly related with elevated concentrations of phosphate and nitrate. In contrast, macroinvertebrate communities sampled in September and October were mainly characterized by higher water levels in the reservoir and the presence of submerged vegetation. Samples collected in June corresponded with a higher ammonium concentration ().
Effect of the dam on the Gilgel Gibe River
The Gilgel Gibe River hydrology downstream from the dam has been strongly affected. Specifically, discharge, flow regime, water depth, and flow velocity have been altered. The estimated average discharge of the river just upstream from the reservoir was 13 m3/s during the dry season. Downstream from the dam, the discharge in the Gilgel Gibe River became <0.63 m3/s (approximately 5%). Among the water quality parameters used in this study (), temperature, turbidity, conductivity, DO, and phosphate significantly differed between upstream and downstream sites. Conductivity in the downstream site was exceptionally high (415 μS/cm) in comparison with the other sites in the Gilgel Gibe basin, while the upstream site had an average conductivity of 133 μS/cm. The percentage scrapers, EPT and chironomids as well as the Margalef and BMWP showed higher values in the upstream site (). MANOVA permutation tests indicated significant differences of macroinvertebrate communities and physicochemical parameters between the upstream and downstream site (p < 0.01).
Table 4 Average and standard deviation (STDV) of environmental variables and macroinvertebrate metrics at the upstream and downstream of the Gilgel Gibe reservoir from 2006 to 2008 (FTU = Formazin Turbidity Units, DO = dissolved oxygen, BOD = biochemical oxygen demand, % E = percentage of Ephemeropetera, BMWP = biological monitoring working party, and FBI = family level biotic index).
Discussion
The Gilgel Gibe I reservoir had high turbidity and elevated phosphate concentrations of 0.3–0.7 mg/L, which is much higher than the permissible 0.025 mg/L for lakes and reservoirs (US EPA Citation1986). It is therefore not unexpected that algal blooms occurred in the reservoir. Devi et al. (Citation2008) previously cautioned that the Gilgel Gibe I reservoir would become enriched with nutrients, which could induce eutrophication. In March 2008, measurements of chlorophyll a content ranged from 5 to 7 Kg/L, indicating a mesotrophic state (Duan et al. Citation2007).
The most important factor affecting macroinvertebrate communities in the Gilgel Gibe I reservoir was probably the water level fluctuation, as was noted by Hynes (Citation1961). When the reservoir water level reaches its maximum, the surrounding vegetation is submerged. As a result, the substratum of the littoral zone is covered with plants, which provides protection to macroinvertebrates from predators and also serves as a food source (Poznanska et al. Citation2009). During high water levels, cattle did not often enter the littoral zone to drink because they could easily access water near the grazing fields. During low water level in May and June, however, cattle often came to the littoral zone to drink (authors’ observation). Trampling muddied the substratum and, in addition, dung droppings increased the organic content of the reservoir, which probably contributed to the dominance of chironomids and oligochaetes during this period because these taxa are usually the dominant macroinvertebrates in reservoirs with a muddy substratum (Meiling et al. Citation2007, Shao et al. Citation2008, Poznanska et al. Citation2009).
Another factor that could explain the low macroinvertebrate diversity in the Gilgel Gibe I reservoir is its age. In most cases, the diversity of macroinvertebrate communities of young reservoirs was found to be poor (Meiling et al. Citation2007, Jorcin and Nogueira Citation2008). The Gilgel Gibe reservoir is only 5 years old, and the submerged land consisted of farmland, grazing land and bushes. It may take some time until the substrate becomes more favourable for benthic invertebrates colonization.
The Gilgel Gibe dam has seriously affected the downstream river ecosystem. The reduction of the discharge by 95% has reduced the flow velocity and exposed the bottom substrate, leaving only pool habitats. The intended discharge downstream from the dam was at least 1.3 m3/s (Pietrangeli and Pallavicini Citation2007); however, the actual flow was 0.63 m3/s, less than half of the intended flow. During sampling, people could easily access the middle of the watercourse for firewood collection, fishing, and stone collection, which was not the case before the construction of the dam. The change of the hydrological regime has therefore increased the human impact in the downstream part of the watercourse, which could have also contributed to a lower diversity of sensitive taxa like EPT and scrapers. De Jalon et al. (Citation2006) also found that scrapers were adversely affected after a dam was constructed.
Another effect observed downstream from the dam was a change in water quality. The phosphate concentration and the turbidity of the water downstream from the dam were reduced by 67 and 83%, respectively, when compared to the upstream river water. This is in agreement with a study by Ideva et al. (Citation2008) in Tanzania, where these authors indicated that concentrations of nitrate and phosphate drastically decreased downstream compared to a site upstream from the dam. Alterations of the nutrient content in the downstream part may affect the primary production of the river system (Ideva et al. Citation2008). Electrical conductivity in the downstream part increased by 210% compared to the upstream site. This is much more than in a Tanzanian reservoir, where the conductivity increased by 38% (Ideva et al. Citation2008).
The Gilgel Gibe I reservoir is characterized by a low macroinvertebrate diversity, probably mainly due to the muddy littoral bed and the water level fluctuations. Until the reservoir develops through succession and becomes colonized, the diversity of macroinvertebrates may remain low. Remedial actions could be important at large scale in the Gilgel Gibe river basin and around the reservoir. Delimitation of a riparian buffer zone along the watercourse, which is effective to minimize incoming pollutants and nutrients, could be a major remedy at the basin level (Ambelu et al. Citation2010). Another action could be the protection of the surrounding vegetation and strictly protecting the buffer zone from intensive grazing, farming, and bush clearance. A third remedy could be the establishment of a sediment trap at the mouths of the rivers before the entrance into the reservoir, as practiced along the River Eye, United Kingdom (Bouma and Schuijt Citation2001). The Gilgel Gibe I reservoir also affected the downstream river system by reducing the downstream discharge, which affected the macroinvertebrate community. The release of the intended environmental flow from the reservoir to the downstream river course of at least 1.3 m3/s could help to maintain the downstream river ecosystem.
Acknowledgments
The authors are grateful to Flemish Inter-University Cooperation (VLIR-UOS) and Jimma University, Department of Environmental Health Sciences and Technology for supporting this study. We are also grateful to Ethiopian Electric Power Corporation for providing us the reservoir water level data.
References
- Agostinho , A A and Gomes , L C . 1997 . “ Manejo e monitoramento de recursos pesqueiros: perspectivas para oreservatorio de Segredo ” . In Reservatorio de Segredo: bases ecologicas para omanejo , Edited by: Agostinho , A A and Gomes , L C . 319 – 364 . Maringa : EDUEM .
- Ambelu , A , Lock , K and Goethals , P LM . 2010 . Comparison of modelling techniques to predict macroinvertebrate community composition in rivers of Ethiopia . Ecol Info. , 5 : 147 – 152 .
- [APHA-AWWA-WPCF] American Public Health Association, American Waterworks Association, Water Pollution Control Facility . 1995 . Standard methods for the examination of water and wastewater. 19th , Washington, DC : American Public Health Association .
- Armitage , P D , Moss , D , Wright , J F and Furse , M T . 1983 . The performance of a new biological water quality score system based on macroinvertebrates over a wide range of unpolluted running-water sites . Water Res. , 17 : 333 – 347 .
- Bouma , J J and Schuijt , K . 2001 . “ Ecosystem valuation and cost-benefit analysis as tools in integrated water management ” . In Dams in European context , Edited by: MidttOmme , G H , Honningsvag , Repp B , Vaskinn , K and Westeren , T . 3 – 10 . Netherlands : Taylor and Francis .
- Calisto , M , Goulart , M , Barbosa , F AR and Rocha , O . 2005 . Biodiversity assessment of benthic macroinvertebrates along a reservoir cascade in the lower Sao Francisco river (Northeastern Brazil) . Braz J Biol. , 65 : 229 – 240 .
- De Jalon , D G , Sanchez , P and Camargo , J A . 2006 . Downstream effects of a new hydropower impoundment on macrophyte, macroinvertebrate and fish communities . Regul River. , 9 : 253 – 261 .
- Devi , R , Tesfahune , E , Legesse , W and Deboch , B . 2008 . Assessment of siltation and nutrient enrichment of Gilgel Gibe dam, South-western Ethiopia . Bioresour Technol. , 99 : 975 – 979 .
- Duan , H , Zhang , Y , Zhang , B , Song , K and Wang , Z . 2007 . Assessment of chlorophyll-a concentration and trophic state for lake Chagan using landsat TM and field spectral data . Environ Monit Assess. , 129 : 295 – 308 .
- [EEPCo] Ethiopian Electric Power Corporation . 2012 . Gibe III Hydroelectric Project Official Website [cited 25 Feb 2013]. Available from: http://www.gibe3.com.et/
- Graynoth , E , Bennett , L C and Pollard , J C . 1986 . Diet of landlocked sockeye salmon (Oncorhynchus nerka) and trout in the Waitaki lakes, New Zealand . New Zeal J Mar Fresh. , 20 : 537 – 549 .
- Hilsenhoff , W L . 1988 . Rapid field assessment of organic pollution with a family-level biotic index . J N Am Benthol Soc. , 7 : 65 – 68 .
- Hynes , H BN . 1961 . The effect of water level fluctuations on littoral fauna . Int Ver Theor Angew Limnol. , 14 : 652 – 656 .
- Ideva , R J , Machiwa , J , Schiemer , F and Hein , T . 2008 . Effect of an impoundment on nutrient dynamics in the Kihansi River, Tanzania . Afr J Aquat Sci. , 33 : 181 – 188 .
- Jabbar , M , Negassa , A and Gidyelew , T . 2007 . “ Geographic distribution of cattle and shoats populations and their market supply sheds in Ethiopia ” . In Improving Market Opportunities , 54 Nairobi , , Kenya : ILRI (International Livestock Research Institute) . Discussion Paper No. 2
- James , M R , Hawes , I and Weatherhead , M . 2000 . Effects of settled sediments on grazer-periphyton macrophyte interactions in the littoral zone of a large oligotrophic lake . Freshwater Biol. , 44 : 311 – 326 .
- Jorcin , A and Nogueira , M G . 2008 . Benthic macroinvertebrates in the Paranapanema reservoir cascade (southeast Brazil) . Braz J Biol. , 68 : 1013 – 1024 .
- Kornijow , R , Gulati , R D and Ozimek , T . 1995 . Food preference of freshwater invertebrates: comparing fresh and decomposed angiosperm and filamentous alga . Freshwater Biol. , 33 : 205 – 212 .
- Martinez-Sanz , C , Fernandez-Alaez , C and Garcia-Criado , F . 2012 . Richness of littoral macroinvertebrate communities in mountain ponds from NW Spain: what factors does it depend on? . J Limnol. , 71 : 154 – 163 .
- Meiling , S , Xinqin , H , Zhicai , X , Xinghuan , J , Ruiqiu , L and Qinghua , C . 2007 . Comparative study on macroinvertebrate communities along a reservoir cascade in Xiangxi River Basin . Acta Ecol Sin. , 27 : 4963 – 4971 .
- [MME-EELPA] Ministry of Mines and Energy: Ethiopian Electric Light and Power Authority . 1997 . Gilgel Gibe hydroelectric project environmental assessment main report 100 Addis Ababa , , Ethiopia
- Pietrangeli , A and Pallavicini , I . Hydroelectric cascade plants in the Omo basin in Ethiopia . International Commission on Large Dams 75th annual meeting; Dam safety management: role of state, private companies and public in designing, constructing and operating of large dams. Saint Petersburg, Russia , pp. 10
- Poznanska , M , Kobak , J , Wolnomiejski , N and Kakareko , T . 2009 . Shallow-water benthic macroinvertebrate community of the limnic part of a low land Polish dam reservoir . Limnologica. , 39 : 163 – 17 .
- Shao , M L , Xie , Z C , Han , X Q , Cao , M and Cai , Q H . 2008 . Macroinvertebrate community structure in Three-Gorges reservoir, China . Int Rev Hydrobiol. , 93 : 175 – 187 .
- Torloni , C E . 1994 . Management of fishing resources in CESPs reservoirs . Acta Limnol Brasil. , 5 : 177 – 186 .
- [US EPA] US Environmental Protection Agency . 1986 . Quality Criteria for Water Washington, DC EPA 440/5-86-001
- [US EPA] US Environmental Protection Agency . 2004 . Wadeable Stream Assessment: Benthic Laboratory Methods , Washington, DC : US EPA, Office of Water and Office of Research and Development . EPA841- B-04-007
- Weatherhead , M A and James , M R . 2001 . Distribution of macroinvertebrates in relation to physical and biological variables in the littoral zone of nine New Zealand lakes . Hydrobiologia. , 462 : 115 – 129 .