Abstract
Grand Lake St. Marys, hereafter Grand Lake, is a large shallow reservoir in western Ohio that has become hypereutrophic in recent decades owing to external phosphorus (P) loading. To restore water quality in Grand Lake, managers must decide whether to reduce external P loading from tributaries or continue to reduce internal P loading from sediments using aluminum sulfate additions. To help managers identify the most effective strategies for water quality improvements in Grand Lake, we quantified the reservoir's seasonal P dynamics using tributary and in-reservoir P measurements during 2009–2011. We also tested the short-term effects of reduced external P loading on Grand Lake's water quality using a pond experiment performed at the adjacent St. Marys State Fish Hatchery. The highest P concentrations in Grand Lake (1660, 391, and 311 μg/L in 2009, 2010, and 2011, respectively) occurred in summer when external P loads from tributaries were minimal, suggesting internal P release from sediments was the major source of P to fuel cyanobacterial blooms. Results from our pond experiment showed that decoupling the reservoir from external P loading during summer months does not improve short-term water quality. We conclude that external P loading during winter and spring, coupled with internal P loading from sediments during summer, drive the extremely high P concentrations in summer that support dense cyanobacterial blooms. Long-term improvement in Grand Lake water quality will first require major reductions to the external P loads from tributaries, followed by manipulations to reduce internal P loading from sediments.
Anthropogenic eutrophication and climate change threaten the health of coastal and inland waters on a global scale (Rabalais et al. Citation2009, Lewis Citation2011, Paerl et al. Citation2011). Shallow inland lakes and reservoirs are particularly vulnerable to eutrophication because their productivity can respond rapidly to nutrient enrichment, and reductions in external phosphorus (P) loading often fail to improve water quality because of internal P regeneration from sediments (Jacoby et al. Citation1982, Cooke et al. Citation1993, Søndergaard et al. Citation1999, Citation2003, Citation2012). Grand Lake St. Marys, hereafter Grand Lake, is a large and shallow polymictic reservoir located in western Ohio that has long served as a regional tourist destination for water recreation and sport fishing (Clark Citation1960), and it provides drinking water for the city of Celina. During summers 2009 and 2010, however, the Ohio Environmental Protection Agency (EPA) posted no-contact advisories at Grand Lake following the detection of microcystin concentrations that greatly exceeded the 20 μg/L threshold for moderate health alert in recreational waters (; WHO Citation2003). To restore water quality in Grand Lake, managers must decide whether to allocate resources toward reducing external P loading from tributaries, continue to reduce internal P loading from sediments, or do both. Accordingly, our goal is to understand the timing and relative importance of external P loading from tributaries and internal P loading from sediments as they pertain to the initiation and persistence of harmful algal blooms.
Figure 1 Seasonal variation in total microcystin concentrations in Grand Lake during 2009–2011. Water column and surface samples were collected from nearshore sites (State Park public beaches, the City of Celina Public Works [CPW] water treatment plant intake pipe, and the Ohio Department of Natural Resources [ODNR] office boat ramp) and offshore sites (e.g., near Safety Island). Samples were collected by ODNR, Ohio EPA (OEPA), USGS, and CPW and analyzed by CPW and GreenWater Laboratories (Palatka, FL) using enzyme-linked immunosorbent assays after sonication. Gray-filled diamonds show saturated tests, such that field microcystin concentrations were actually greater than those displayed here. The horizontal dashed lines represent the value for a moderate health alert in recreational waters (20 μg/L; WHO Citation2003).
![Figure 1 Seasonal variation in total microcystin concentrations in Grand Lake during 2009–2011. Water column and surface samples were collected from nearshore sites (State Park public beaches, the City of Celina Public Works [CPW] water treatment plant intake pipe, and the Ohio Department of Natural Resources [ODNR] office boat ramp) and offshore sites (e.g., near Safety Island). Samples were collected by ODNR, Ohio EPA (OEPA), USGS, and CPW and analyzed by CPW and GreenWater Laboratories (Palatka, FL) using enzyme-linked immunosorbent assays after sonication. Gray-filled diamonds show saturated tests, such that field microcystin concentrations were actually greater than those displayed here. The horizontal dashed lines represent the value for a moderate health alert in recreational waters (20 μg/L; WHO Citation2003).](/cms/asset/3d2020fd-2d23-4628-a71d-1317b24f6764/ulrm_a_823469_o_f0001g.gif)
Excessively high total phosphorus (TP) concentrations within Grand Lake during summer (Hoorman et al. Citation2008) currently drive dense annual blooms of cyanobacteria capable of producing toxins (Anabaena, Aphanizomenon, Microcystis, and Planktothrix). To restore recreational use of Grand Lake, managers are currently focused on reducing the concentrations of P available to fuel phytoplankton growth. The amount of P in Grand Lake, whether in the sediments or the water column, is ultimately derived from watershed inputs; therefore, a logical approach is to reduce P loading into the reservoir from its tributaries. For example, the Ohio EPA released a total maximum daily load (TMDL) report for Grand Lake that showed tributary TP loads must be reduced 80–99% during most seasons to improve water quality (OEPA Citation2007). Grand Lake's watershed, however, supports one of the most economically valuable concentrated livestock farming regions in the United States, and the most valuable region within Ohio (USDA Citation2009). Reducing external P loads would likely require restricting maximum crop and livestock yields, continuing to develop infrastructure and incentive programs for exporting manure-derived P from the watershed, and/or intercepting and harvesting P from tributaries before it enters the reservoir (e.g., as struvite; Forrest et al. Citation2008). Because these strategies represent long-term solutions to an immediate problem, managers have also pursued short-term improvement plans to abate internal P loading and bioavailability, including sediment dredging, rough fish removal, and alum (aluminum sulfate) addition. Alum treatment has received the most public attention because the Ohio EPA initiated expensive partial treatments in 2011 and 2012. In each year, the middle 40% of the reservoir area (19 km2) was treated at 40% of the dose needed to inactivate P in sediments (44 g Al/m2; TetraTech Citation2011, Citation2012).
We determined the relative importance of external and internal P loading for seasonal P dynamics in Grand Lake during the 2 years prior to alum addition and during the first alum treatment year. We used available tributary and in-reservoir P measurements to determine the relative importance of external and internal P loading for seasonal P dynamics in the reservoir. We also test the potential short-term (3 month) effects of reduced summer P loading on primary productivity and water quality in Grand Lake by conducting a manipulative experiment using ponds at the adjacent St. Marys State Fish Hatchery (SFH). Ponds at the St. Marys SFH receive water and sediments directly from Grand Lake during filling and hence serve as excellent mesocosms (Spivak et al. Citation2011) to test biological and water quality responses to varying levels of P loading into the reservoir. Phosphorus dynamics within the hatchery ponds are presumably driven by the same processes as for Grand Lake except for differences in physical forces that scale with size. For example, wind mixing probably has a greater role in sediment resuspension in Grand Lake, which has a 14.5-km fetch, as compared to the smaller hatchery ponds.
We hypothesized that (1) internal P loading drives summer increases in P concentrations in Grand Lake, similar to other shallow eutrophic lakes (Søndergaard et al. Citation1999), and therefore (2) decoupling the reservoir from external P loading during summer by reducing connectivity to its watershed does not improve short-term water quality.
Study sites
Grand Lake (40°31′43″N, 84°29′50″W) is located in Mercer and Auglaize counties in western Ohio (). It is the largest reservoir wholly contained in Ohio (surface area = 52.2 km2), has a long east–west fetch (14.5 km), and is extremely shallow (mean depth = 1.6 m). Based on surface and bottom temperature and dissolved oxygen (DO) measurements taken at a nearshore site during morning and afternoon of June–August 2009, the reservoir mixed each night by surface cooling (median vertical differences during morning were 0.0°C and 0.3 mg/L) and became weakly stratified on many days in the afternoon (median differences 0.3°C and 0.6 mg/L).
Figure 2 Locations of Grand Lake, its subwatersheds, and the St. Marys State Fish Hatchery (SFH) within Mercer and Auglaize counties, western Ohio. Land use data were extracted from the 2001 National Land Cover Database (Homer et al. Citation2007). Ohio Division of Wildlife (DOW) and Ohio EPA (OEPA; L-1, L-2, and L-3) sites are locations where water samples were collected for phosphorus measurements during 2009–2011, in addition to the south hatchery intake.
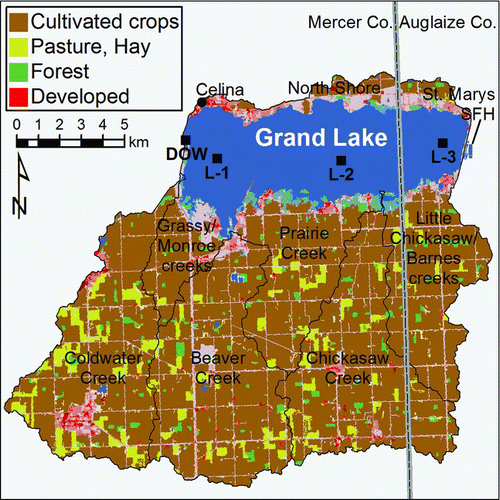
Grand Lake was constructed during 1837–1845 to supply water for the Miami-Erie Canal by damming the tributaries of the Wabash and St. Marys rivers, flooding the wetland area between them (Clark Citation1960, GLWWA Citation2008). More recently, the reservoir provided a variety of ecosystem services for humans, including drinking water for the city of Celina, economically valuable crappie (Pomoxis spp.) and channel catfish (Ictalurus punctatus) recreational sport fisheries, a location for boating and water sports, and a water source for rearing sport fish at the St. Marys SFH. In 2007 (before the Ohio EPA posted no contact advisories), annual travel and tourism industry income to Mercer and Auglaize counties was about $47.5 million and supported about 2400 local jobs (GLWWA Citation2008).
Grand Lake's 241 km2 watershed is located mainly south of the reservoir and contains several low-order streams, all <16 km long (). According to the 2001 National Land Cover Database (http://www.mrlc.gov/nlcd2001.php; Homer et al. Citation2007), 82% of the watershed land area was agricultural (cultivated crops, grassland, or pasture), 14% urban, 3% forested, and 1% wetlands. Cultivated crop production in Mercer and Auglaize counties was similar to statewide averages for farm size and crop yields during 1950–2007 (USBC Citation1952–Citation1994, USDA Citation1999–Citation2009); however, 2007 livestock densities within Grand Lake's watershed were much greater than county and statewide levels (; ). The high livestock density within Mercer County is due to the construction of many concentrated animal feeding operations since the 1990s, especially for poultry and pork production (). In 2007, Mercer County was ranked 3rd in the United States (2nd in Ohio) for poultry production and 50th (1st in Ohio) for pork production, with the total value of livestock, poultry, and their products ranking 35th of 3069 surveyed counties in the United Sates with a value of $441 million (USDA Citation2009). Consequently, Grand Lake's watershed produces large quantities of nitrogen (N)- and P-rich manures (460,240 tonnes in 2007) that exceed potential P uptake rates by cultivated crops within the watershed, even after 70% export of poultry manure (; OEPA Citation2007, GLWWA Citation2008). Application of commercial N and P fertilizers adds to this total.
Table 1 Comparisons of animal farming intensity and farm sizes among the state of Ohio, Auglaize and Mercer counties, and the Grand Lake St. Marys watershed during 2007 (GLWWA Citation2008, USDA Citation2009). Animal units (AU) are standardized to the average weight of a beef cow, using conversions listed in the Grand Lake St. Marys/Wabash Watershed Action Plan (GLWWA Citation2008).
Table 2 Comparisons of manure waste production and cropland application rates needed to distribute all manure wastes across Grand Lake St. Marys subwatersheds during 2007. Manure application rates are reported with adjustment for the estimated 70% export of poultry manure. All data were derived from the Grand Lake St. Marys/Wabash Watershed Action Plan (GLWWA Citation2008). All calculations exclude inorganic fertilizers.
Figure 3 Comparisons of (a) total (= All Cattle + Hogs/Pigs + Chickens + Turkeys) animal unit densities in Mercer and Auglaize counties to statewide densities, and (b) animal units/farm within Mercer County during 1950–2007. Numbers of animals derived from US Censuses of Agriculture reports (USBC Citation1952–Citation1994, USDA Citation1999–Citation2009) were standardized to animal units (1 AU = the average weight of a beef cow) using conversions listed in the Grand Lake St. Marys/Wabash Watershed Action Plan (GLWWA Citation2008).
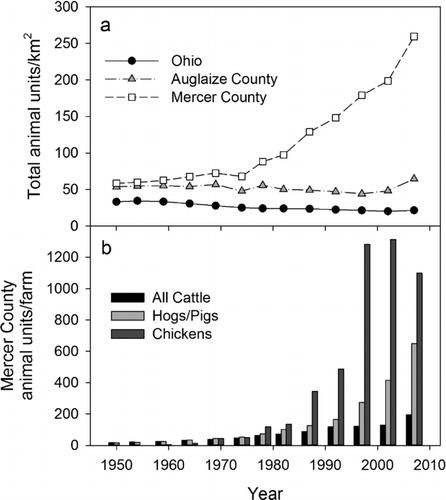
Grand Lake's nutrient concentrations and biological productivity () reflect its large, shallow morphometry and the long-term trends in agricultural land use within its watershed. Grand Lake has been highly productive since its construction, with an average TP concentration of 110 μg/L during the 1930s (Clark Citation1960). Prior to 1999, concentrations of TN, TP, and chlorophyll a were variable through time but have apparently doubled in the last decade (). Clark (Citation1960) reported that the reservoir was originally dominated by submersed macrophytes, with much of the nearshore area dominated by cattail (Typha spp.). Since about the 1970s, however, the reservoir has been characterized by a turbid phytoplankton state dominated by cyanobacteria (GLWWA Citation2008), and submersed macrophytes are now essentially absent. Currently, Grand Lake is among the most productive lakes and reservoirs in Ohio and the United States. In the 2007 National Lakes Assessment conducted by the US EPA, Grand Lake was in the 94th percentile for TN and TP concentrations of the 1252 inland lakes and reservoirs sampled, 97th percentile for chlorophyll a concentration, and had the third highest concentration of total microcystins (99th percentile; USEPA Citation2009).
Table 3 Historical nutrient concentrations and biological productivity data for Grand Lake St. Marys. Individual measurements, ranges, or mean (range) are reported. ND = no data.
The St. Marys SFH, operated by the Ohio Department of Natural Resources, Division of Wildlife (ODNR-DOW), is located along the eastern shore of Grand Lake (). Water for filling the hatchery ponds was drawn from 2 intake pipes at 1 m depth and about 850 m apart. At the hatchery, 21 fish-rearing ponds are 0.4 ha (4,500 m3), one is 1.6 ha (12,600 m3), and 3 are 2.8 ha (59,200 m3) used for rearing fish. The 0.4 and 1.6 ha ponds range from 0.5 to 1.5 m deep, and the 2.8 ha ponds range from 0.5 to 3 m deep. All ponds were lined with clay during renovations in the mid-1990s, but the bottoms have since been covered by silty lake sediments delivered to ponds during filling events over many production years, as well as by organic matter produced within the ponds.
Materials and methods
Hypothesis 1: Internal loading drives increased summer P concentrations in Grand Lake
To determine the relative contributions of external and internal P loading to seasonal dynamics in Grand Lake, we compared monthly tributary TP loads to in-reservoir P masses during 2009–2011. We used US Geological Survey (USGS) stream flow measurements at Chickasaw Creek (40°29′13″N, 84°28′54″W; USGS Citation2012), combined with an intensive stream nutrient monitoring dataset provided by the National Center for Water Quality Research at Heidelberg University, Ohio (NCWQR Citation2012), to calculate daily water and external TP loads for Grand Lake. The tributary TP measurements were performed by NCWQR once daily or more frequently during high-flow events to accurately estimate TP flux into the reservoir. We calculated daily water and TP loads from Chickasaw Creek by multiplying discharge by the time interval surrounding measurements and the TP concentration in the sampled water. We then summed daily loads by month for each calendar year.
We estimated TP loads for Grand Lake's entire watershed using the direct measurements for Chickasaw Creek. Although water loads for the entire watershed were reasonably estimated by linear extrapolation from Chickasaw Creek based on relative land areas (TetraTech Citation2012), differences in farm animal densities across the different creek subwatersheds () could cause substantial differences in their relative P loads. To determine the best technique for extrapolating the Chickasaw Creek P loads to the entire watershed during 2009–2011, we compared our estimates of TP load to those performed by the Ohio EPA for multiple creeks and the entire watershed every 2 weeks during May 2010–May 2011 (Appendix C in TetraTech Citation2012). We compared those observed TP loads to our estimated TP loads calculated for the same period by extrapolating Chickasaw Creek measurements to the entire watershed using areal extrapolation with and without adjusting for proportional differences in farm animal densities. We found that simple areal extrapolation of Chickasaw Creek TP loads to the entire watershed provided the best estimates of total watershed TP loads. Thus, we linearly extrapolated the areal Chickasaw Creek TP loads to the entire watershed land area to calculate the daily TP loads (tonnes/km2/day) into Grand Lake during 2009–2011.
Following Kalff (Citation2002), we estimated mean water residence time within Grand Lake for each year by dividing Grand Lake's mean water volume (8.25 × 107 m3, ODNR-DOW) by the annual water load calculated from the USGS continuous measurements in Chickasaw Creek. This method provided a similar estimate of the mean water residence time for May 2010–May 2011 (236 d) as compared to the more detailed water mass balance provided in TetraTech (Citation2012; 212 d). Because we did not have measurements to complete water budgets for 2009 and 2010, we report the mean water residence times using our method to provide a first-order comparison of water residence times in the reservoir among these calendar years.
We estimated within-reservoir P masses for 2009, 2010, and 2011 using all available in-reservoir P measurements. We measured total reactive phosphate (TRP) concentrations weekly near the south hatchery intake () during April–September 2009 and 2010. The ODNR-DOW measured TP concentrations monthly during June–September 2009, and soluble reactive phosphorus (SRP) and TP concentrations monthly during April–September 2011 at a single site at the western end of Grand Lake (). The Ohio EPA measured SRP and TP monthly during April–October 2010 and every 1–4 weeks during March–October 2011 at offshore sites L-1, L-2, and L-3 (). We averaged concentrations across these 3 Ohio EPA sites for our analyses. For each year and set of measurements, we calculated the masses of SRP, TRP, or TP in the entire reservoir by multiplying the measured concentrations by a constant total water volume in Grand Lake (8.25 × 107 m3). We then compared seasonal changes in these in-reservoir P masses to monthly external TP loads to determine the relative importance of external and internal P loading in the reservoir during summer.
Hypothesis 2: Reduced summer P loading does not improve short-term water quality
To test whether managers should expect reduced P loading to improve Grand Lake's water quality in the short term (i.e., in one summer), we tested the effects of full and reduced reservoir connectivity to its watershed using a manipulative pond experiment performed at the St. Marys SFH during June–September 2009. The hatchery ponds are excellent models of the reservoir because they receive water, plankton, and sediments directly from the reservoir. Moreover, the ponds are identical in size and shape, and they can be independently filled and drained, affording exceptional experimental control and replication.
We designed our pond experiment to serve as a small-scale test of how reduced external P loading during summer might affect Grand Lake's water quality. We filled a block of nine 0.4 ha ponds and one 2.8 ha pond at the St. Marys SFH with Grand Lake water during 4–17 June. We then randomly assigned 3 of the 0.4 ha ponds to each of the following treatments: (1) twice refilling ponds with water from Grand Lake to establish full connectivity to external P loading; (2) entirely isolating ponds from Grand Lake (i.e., never refilling ponds), thereby wholly eliminating tributary P loading; and, (3) twice refilling ponds with water from the 2.8 ha pond to control for the physical effects of refilling the ponds on internal P loading from sediment resuspension. During each refilling event, ponds were first drained by lowering the sluice gates by 0.6 m (releasing about 2250 m3 of water, or about 50% of total volume) and then refilled by gravity according to their assigned treatment. The refilling events took place about 4 weeks apart during 15–17 July and 11–14 August 2009.
To more closely represent reservoir conditions, all nine 0.4 ha ponds were stocked with juvenile channel catfish (15 g) at 17,300 fish/ha on 15 June. These ponds also received about 187 kg artificial feed during the experiment to enhance catfish growth, releasing about 0.3 kg P into the ponds during the experiment (Filbrun et al. Citation2013). The 2.8 ha pond used to refill the smaller 0.4 ha ponds was not stocked with any fish and did not receive any artificial feed.
To measure the effects of reduced summer P loading on Grand Lake, we compared a variety of physical, chemical, and biological response variables among our pond treatments. We measured water temperature continuously using data loggers (HOBO loggers, Onset Computer Corporation, Bourne, MA) suspended 0.5 m deep in the center of each pond. St. Marys SFH staff measured dissolved oxygen (DO) every 2 days during morning (06:00–08:00 h) and afternoon (12:00–13:00 h) using a YSI model 86 hand-held meter (Yellow Springs Instruments, Yellow Springs, OH). TRP, ammonium, and nitrate concentrations were measured weekly in unfiltered pond water samples collected near the kettle in each pond using a plastic tube sampler. TRP was measured by the stannous chloride/ammonium molybdate method (APHA Citation2005), ammonium was measured using the orthophthaldialdehyde method (Protocol B, Holmes et al. Citation1999), and nitrate was measured by St. Marys SFH staff using the nitrate electrode method (APHA Citation2005). We estimated phytoplankton production and respiration in each pond weekly by measuring oxygen differences in transparent and opaque bottles incubated in situ, following Jacob and Culver (Citation2010). We also measured Secchi depth in each pond weekly as a proxy for light penetration, along with early afternoon (13:00–14:00 h) pH.
We tested for differences in water quality metrics using a mixed effects model,
where Y is the response variable of interest, T is the fixed effect of the pond treatment, D is the fixed effect of time, T × D is the fixed effect of the treatment × time interaction, Z is the random effect of pond, and ϵ is the residual error. We tested all data for normality using one-sample Kolmogorov-Smirnov tests, and we visually inspected residual plots to assess equality of variances. Most data were loge-transformed to meet these assumptions. For variables with nonlinear responses through time, we also included the fixed effects of time2 and treatment × time2. Differences were considered significant at P ≤ 0.05. We performed all statistical tests using SPSS Statistics Version 17.0 (SPSS, Chicago, IL).
Results
Hypothesis 1: Internal loading drives increased summer P concentrations in Grand Lake
We compared 2009–2011 monthly external TP loads to in-reservoir P masses in Grand Lake to determine the relative importance of external and internal P loading for seasonal P dynamics in the reservoir. The annual external TP loads into Grand Lake during 2009, 2010, and 2011 were 38, 36, 132 tonnes, respectively (). Within years, tributary TP loads during January–May accounted for 61–79% of the annual loads delivered to the reservoir (). By comparison, in-reservoir SRP, TRP, and TP masses were generally low during April and May, then increased and remained elevated during June–September, despite low external TP loads in these months (). Peak in-reservoir P masses occurred during July and September 2009 (, left panel); June, August, and September 2010 (, middle panel); and June and July 2011 (, right panel). Nearshore sites (hatchery intake and ODNR-DOW) had higher P concentrations as compared to the Ohio EPA offshore sites (L-1, L-2, and L-3), although seasonal patterns of in-reservoir P masses were robust to site effects and the pools of P measured.
Table 4 Comparison of monthly external water and total phosphorus (TP) loads into Grand Lake during 2009–2011.
Figure 4 Panels (a) and (b) show seasonal and interannual variation in 2009–2011 Chickasaw Creek discharge and phosphorus concentrations, respectively. Panel (c) shows comparisons between monthly external TP loads (bars) for the entire Grand Lake watershed and in-reservoir total phosphorus (TP, solid lines), total reactive phosphorus (TRP dotted lines), and/or soluble reactive phosphorus (SRP dashed) masses. The vertical lines (panel c, right) show the start and finish of the alum treatment during 2011. Chickasaw Creek discharge measurements were performed by USGS, whereas nutrients were measured by the National Center for Water Quality Research (NCWQR). In-reservoir P measurements were performed on water samples collected near the south hatchery water intake (green), from the Ohio Department of Natural Resources, Division of Wildlife (DOW) boat ramp at the western end of the reservoir (blue), and from Ohio EPA (OEPA) sites (L-1, L-2, and L-3, averaged; red). Note that the scale for in-reservoir TP in tonnes is different for 2009 because of higher P concentrations measured in that year.
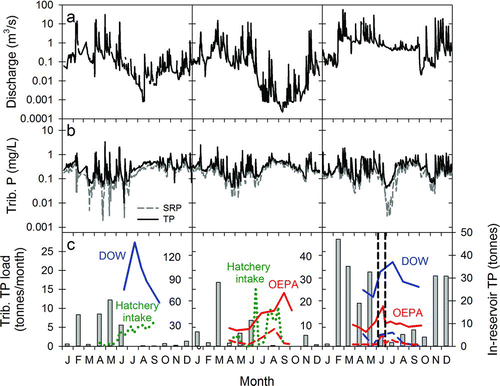
Chickasaw Creek discharge varied across years and seasons (), demonstrating that weather ultimately drives water residence time and external P loading, which in turn influences internal P dynamics. The mean water residence times were 513, 555, and 140 d for the 2009, 2010, and 2011 calendar years, respectively. Within years, January–Maywater loads accounted for 59–82% of the total annual water loads (). Concentrations of SRP and TP in Chickasaw Creek generally increased during high discharge events ().
Figure 5 Comparisons of 2009 (a, b) total reactive phosphate (TRP), (c, d) ammonium; and (e, f) nitrate concentrations among 0.4 ha ponds that that were twice refilled with water from Grand Lake (GL), a 2.8 ha pond, or were isolated at the St. Marys SFH. Vertical dashed lines show when the 0.4 ha ponds were partially drained and refilled with their assigned source water. Error bars are omitted from pond measurements to improve clarity. Note that the scales for nitrate concentrations differ between panels (e) and (f).
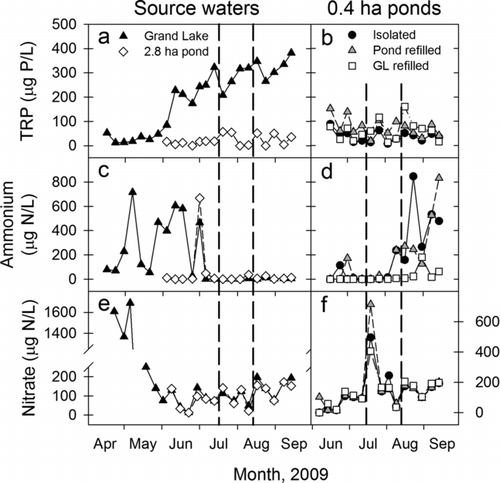
Hypothesis 2: Reduced summer P loading does not improve short-term water quality
To test whether decoupling Grand Lake from its watershed during summer would improve water quality, we compared water quality among the hatchery ponds with different levels of connectivity to the reservoir. TRP concentrations in the 0.4 ha ponds did not differ across reservoir-connectivity treatments (MEM; treatment and treatment × time, both P > 0.31; ) or through time (time, P = 0.47). Mean TRP concentrations ranged from 8 to 89 μg P/L in isolated ponds, 19 to 153 μg P/L in 2.8 ha pond-refilled ponds, and 14–161 μg P/L in Grand Lake-refilled ponds (). Grand Lake's TRP concentration averaged 175 μg P/L when the 2.8 and 0.4 ha ponds were initially filled (). Subsequently, TRP concentrations increased sharply in Grand Lake during June–September but remained lower in the 2.8 ha pond through September (). Ammonium and nitrate concentrations were generally low in the 0.4 ha ponds and did not differ among watershed-connectivity treatments (MEM; treatment and treatment × time, all P > 0.11; ). Ammonium concentrations increased sharply in August and September when phytoplankton populations declined (MEM; time, P < 0.001), whereas an anomalous nitrate spike occurred in all ponds on 20 July (). Consistent with patterns in spring tributary loading, ammonium and nitrate concentrations were greatest in Grand Lake prior to June, before the pond experiment began (). During mid-July through September, ammonium and nitrate concentrations were relatively low in Grand Lake and the 2.8 ha pond (0–50 and 20–200 μg N/L, respectively).
Regardless of watershed-connectivity treatment, all the 0.4 ha ponds were equally as productive (measured as net primary production or Secchi transparency) as Grand Lake and the 2.8 ha pond once phytoplankton blooms became established (). Phytoplankton productivity did not change following the water exchange events, and Secchi depth, afternoon pH, and algal productivity and respiration did not differ across treatments through time (MEM; treatment × time or treatment × time2, all P > 0.37; Fig. 6). In Grand Lake, phytoplankton productivity increased during April–June, peaked in July when we observed a dense Planktothrix bloom, and subsequently decreased in August and September (Fig. 6e). In the 2.8 ha pond, phytoplankton productivity generally reflected the pattern in Grand Lake both in the magnitude and direction of productivity oscillations.
Figure 6 Comparisons of 2009 (a, b) Secchi transparency depth; (c, d) afternoon pH; (e, f) net phytoplankton primary productivity; and (g, h) respiration for source waters and among 0.4 ha ponds that were twice refilled with water from Grand Lake (GL), a 2.8 ha pond, or were isolated at the St. Marys SFH. Vertical dashed lines show when the 0.4 ha ponds were partially drained and refilled with their assigned source water. Error bars are omitted from pond measurements to improve clarity.
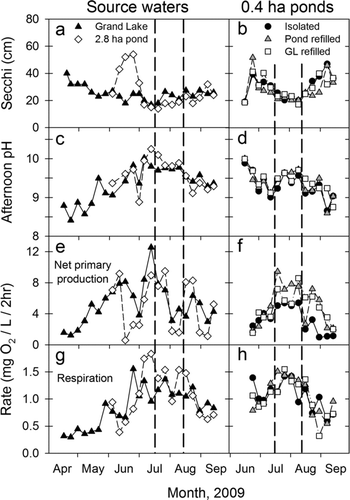
We found no differences in morning DO concentrations at the surface and bottom of the 0.4 ha ponds among treatments through time (MEM; treatment × time, both P > 0.34); hence, mixing induced by the water exchange events in July and August apparently did not affect DO concentrations over multiple days. Water clarity increased in all the 0.4 ha ponds as phytoplankton populations collapsed after initial pond filling, causing hypoxia (DO < 2 mg/L) to set up for 2–3 days in late June. After phytoplankton populations recovered, ponds in all treatments had 0–14 mg/L DO (median = 6 mg/L) at the surface and bottom during morning. In Grand Lake, morning DO concentration ranged from 0 to 12 mg/L (median = 7 mg/L), whereas concentrations at the bottom of the 2.8 ha pond approached 0 mg/L on several dates in July and August. Surface water temperatures ranged from 20 to 33°C in Grand Lake and all ponds during the experiment.
Discussion
Many shallow eutrophic lakes at north temperate latitudes have increased P concentrations during summer that can only be attributed to internal loading from sediments (Barbiero and Kann Citation1994, Boros et al. Citation2009, Søndergaard et al. Citation2012, Spears et al. Citation2012). Internal P loading is problematic for lake managers because poor water quality resulting from dense cyanobacterial blooms in summer often persists years or even decades after reductions in external P loading (Søndergaard et al. Citation2012, Spears et al. Citation2012). Although it is difficult to identify the causal mechanisms of internal P loading during summer, many complementary processes are likely involved, including (1) increased biological activity with increased temperature that reduces oxygen concentrations in surficial sediments (thereby releasing iron-bound P from sediments) and increased bacterial mineralization of organic P to phosphate in interstitial sediment water; (2) increased pH from photosynthesis that increases solubility of iron–phosphate compounds; (3) increased bioturbation of sediments by benthic-oriented fishes; and (4) wind mixing (Istvánovics Citation1988, Søndergaard et al. Citation1999, 2012, Boros et al. Citation2009, Spears et al. Citation2012). High rates of internal P loading are especially persistent in lakes dominated by a turbid algal state because they experience net oxygen loss at the sediment–water interface, and they have high rates of organic matter production in the overlying water column (Søndergaard et al. Citation1999, Citation2012, Spears et al. Citation2012).
Grand Lake's physical, chemical, and biological characteristics during 2009–2011 were similar to those of other shallow temperate lakes with P dynamics driven by summer internal P loading. Factors conducive to high internal P loading in Grand Lake during summer include (1) its morphometry (shallow depth, large surface area, and long fetch); (2) the flat and intensely agricultural surrounding lands (which do not shelter the reservoir from winds and produce high external P loads); (3) high in-reservoir summer temperature and primary production (that co-vary with elevated pH and decreased light penetration); and (4) large populations of bioturbating rough fish, including common carp (Cyprinus carpio) and gizzard shad (Dorosoma cepedianum). Moreover, the increases in water column P concentrations that occurred during summers 2009 through 2011 in months with the lowest tributary P loads can only be attributed to internal P loading, supporting our first hypothesis. Based on the observed seasonal pattern of P dynamics, we contend that external P loading from tributaries during late winter–early spring triggers late spring cyanobacterial blooms that then persist and intensify throughout summer with P regeneration from sediments. Internal P regeneration is especially problematic in Grand Lake because the reservoir does not stratify on a seasonal basis, so the released P becomes immediately available to fuel cyanobacterial growth and intensify blooms. In turn, the internal pool of mobile P in the sediments (about 1.8 g/m2, or 20% of TP in sediments; TetraTech Citation2012) likely originates from decades of previous external P loading.
Our pond experiment effectively served as a general, small-scale test of how Grand Lake water quality might respond if it were decoupled from external P loading during summer, and a specific test of whether refilling hatchery ponds with water containing lower P concentrations leads to improved water quality. Although we do not have direct measurements of internal P loading in the hatchery ponds as compared to Grand Lake, we have long studied the effects of phosphate addition on water quality and biological productivity in hatchery ponds, mainly for aquacultural production of zooplanktivorous fish (Culver et al. Citation1993, Jacob and Culver Citation2010). Results from these studies suggest that net P retention by sediments in ponds is high during spring because additions of inorganic P required to elicit increases in lower trophic level productivity were depleted from the water column within 1 week after application. In the current study, we found complementary evidence that P retention by sediments in the ponds during summer was greater (or at least there was less P release) than in Grand Lake. TRP concentrations were consistently greater at the reservoir sites compared to all ponds in 2009, despite similar temperatures and primary productivity. Presumably, greater sediment resuspension caused by wind-induced mixing in Grand Lake compared to the ponds forced the difference in the magnitude of internal P loading, although other factors such as differences in fish communities and density and the amounts of mobile P in sediments may also be important.
Results from our pond experiment support our second hypothesis that reduced external P loading during summer does not improve short-term water quality in Grand Lake. When the ponds were initially filled in June 2009, a dense bloom of Planktothrix had already become established in Grand Lake, which likely stored a large pool of P. In combination with the high TRP concentration in the initial fill water (175 μg P/L) and potential P regeneration from sediments, P levels were sufficient in ponds to support dense phytoplankton populations for the remainder of the summer, even in the ponds in the isolated treatment. These results support the conclusion that simply reducing external P loading during summer will not improve water quality in the short term because external P loading in winter and spring, coupled with internal P loading during summer, currently drive summer P dynamics. Of course, P release from uneaten and egested artificial feed added to ponds is also a source of P to fuel algal productivity in ponds, but this source was likely inconsequential compared to the higher loads of P added to ponds during the pond refilling events. These outcomes further highlight the importance of external and internal P loads for reducing water quality in shallow lake and pond ecosystems.
Alum (aluminum sulfate) addition alters expectations for Grand Lake's remediation. Treating lakes with alum has long been used by managers to increase sediment adsorption capacity for P, thereby reducing phosphate concentrations that fuel cyanobacterial blooms (Cooke et al. Citation1993). In deep temperate lakes and reservoirs that stratify, alum binds first to phosphate and secondarily to organic colloidal particles in the water column as the amorphous aluminum hydroxide “sweep floc” sinks in one pass through the epilimnion before covering the sediments at the lake bottom to prevent classic iron-mediated P release during summer hypoxia (O’Melia Citation1972, Cooke et al. Citation1993, Wetzel Citation2001). The effectiveness of alum addition to shallow polymictic lakes, however, has been less studied. Cooke et al. (Citation1993) argued that alum should be more effective for treating shallow polymictic lakes because control of sediment P release occurs throughout the water column. The sorption capacity of the aluminum hydroxide floc may become saturated in highly turbid lakes, however, such that continual wind mixing would yet cause net P release from sediments. Also, pH > 9 caused by photosynthesis in eutrophic lakes, as commonly observed in Grand Lake, can liberate phosphate from the aluminum hydroxide floc (Stumm and Morgan Citation1970, Van Hullebusch et al. Citation2003, Cooke et al. Citation2005).
Alum addition to improve short-term water quality in shallow lakes has shown variable effectiveness. Welch and Schrieve (Citation1994) reported that alum additions to a series of 6 shallow eutrophic lakes in Washington were generally effective for reducing P concentration, internal P loading, and cyanobacterial biomass for up to 5 years after treatment. Similarly, Reitzel et al. (Citation2003) showed that low-dose alum addition reduced phosphate concentration and algal biomass in a 1-month enclosure experiment in shallow Lake Sønderby, Denmark. Conversely, alum treatments of other lakes have failed because of P regeneration from senescent organisms during summer, sustained external P loading and/or high pH. Examples of treatment failures for these reasons include the 1984 alum treatment of Lake Wapato, Washington (Cooke et al. Citation1993, Welch and Schrieve Citation1994); Lake Lyngby, Denmark (Sønnichsen Citation1977); and Lake Courtille, France (Van Hullebusch et al. Citation2003).
In Grand Lake, several factors may reduce the anticipated effectiveness of alum treatment. First, although orthophosphate comprises a large fraction of the water column TP during summer, particulate matter stores of P that can sustain phytoplankton growth are unaffected by the treatment except through enmeshment by the sweep floc. Second, high productivity in summer elevates afternoon pH (up to 10), which dramatically increases the solubility of aluminum-P complexes (Stumm and Morgan Citation1970). Sodium aluminate was added in solution with alum to buffer acidification caused by the initial reaction of aluminum sulfate with water to produce aluminum hydroxide (TetraTech Citation2012), but it will not buffer elevated pH driven by photosynthesis. Third, the process of alum application to this large reservoir takes several weeks, during which time weather events and biological dynamics (e.g., establishment of P stores in zooplankton or cyanobacterial blooms) can change. Daily loads of external P added to the reservoir during and after alum addition, especially in spring, may be unaffected by this treatment. Finally, even if alum wholly binds all in-reservoir P, external loading can rapidly regenerate P stores in the reservoir on a yearly time scale. For example, assuming an average summer TP concentration of 500 μg/L in the water column and 1.8 g/m2 of mobile P in the sediments (TetraTech Citation2012), we estimate that Grand Lake stores roughly 135 tonnes of P during summer. During a wet year, external sources can load a similar amount of P (e.g., 132 tonnes in 2011).
In contrast to chemically based or rough fish removal approaches to improving shallow lake water quality, forcing lakes to a clear water state through “top-down” biomanipulation represents a potential alternative for reducing summer internal P loading and improving water quality (Scheffer et al. Citation1993, Boros et al. Citation2009, Søndergaard et al. Citation2012). For example, removing carp and gizzard shad that are zooplanktivorous as juveniles can increase large-bodied zooplankton populations that suppress phytoplankton growth through grazing (Carpenter et al. Citation1985, Havens Citation1993). In turn, increased light penetration to sediments promotes growth of submersed macrophytes and benthic algae that compete for soluble P and oxidize and stabilize sediments (Scheffer et al. Citation1993, Søndergaard et al. Citation1999). Perhaps more importantly, reducing populations of adult carp and gizzard shad also reduces sediment bioturbation, thereby reducing subsequent erosion by wind mixing (Heerdt and Hootsmans Citation2007) and translocation of detrital P into the water column (Havens Citation1993, Vanni Citation2002, Boros et al. Citation2009). Nevertheless, removing enough fish biomass from Grand Lake to accomplish this transition would be impractical because of its size and the buffering effects of “bottom-up” nutrient enrichment of algal growth. We expect that high external P loads would counteract the effects of culling the fish, and a large pool of mobile P in the sediments would retard transition to a clear water state (Scheffer et al. Citation1993, Søndergaard et al. Citation1999).
Alum addition and rough fish removal are short-term mitigation strategies, applied with variable success to shallow lakes, to abate internal P loading following reductions in external loading (Welch and Schrieve Citation1994, Reitzel et al. Citation2003, Van Hullebusch et al. Citation2003). Our summary of agricultural trends and direct tributary monitoring by the NCWQR show that external P loads into Grand Lake are likely the highest they have ever been and continue to increase, adding adequate external P loads each year to initiate cyanobacterial blooms during spring. Thus, regardless of strategies to reduce internal P loading, managers should expect cyanobacterial blooms and associated poor water quality to persist annually as long as external P loading remains high.
If external loading were immediately reduced, how long should Grand Lake managers expect internal P loading to fuel cyanobacterial blooms and cause poor water quality? Depending on the store of mobile P in sediments, the P concentration gradient between sediments and the overlying water, the effectiveness of ongoing remediation projects, weather, and yet other factors, managers should expect water quality problems to continue for years or even decades. In Lake Søbygaard, Denmark, Søndergaard et al. (Citation1999) measured internal loading by sediments during summer for 15 years after 80–90% reductions in external loading and found evidence for P mobilization from as deep as 25 cm in sediments. Similarly, Spears et al. (Citation2012) reported a recovery period of Loch Leven, Scotland, of at least 20 years after 60% reduction in external loading. Similarly, TetraTech (Citation2012) estimated that at least 80% reductions to external and internal P loading in Grand Lake are needed to reduce algal production and restore water quality, and that it could take decades to reduce internal P loading to the target level. To restore Grand Lake and similar shallow lakes in the long-term, managers must first abate external P loading. Otherwise, toxic cyanobacterial blooms during summer will occur indefinitely.
Acknowledgments
We thank the USGS and the NCWQR at Heidelberg University for providing the extensive Chickasaw Creek monitoring data; the Ohio EPA for providing 2011 P concentrations in Grand Lake; M. Sudman at the Celina Water Treatment Plant, the Ohio EPA, the Ohio Department of Natural Resources, and USGS for providing microcystin concentration data; M. Pugh and staff at St. Marys SFH for performing water manipulations to the ponds in 2009 and measuring nutrient concentrations in 2009 and 2010; and R. Briland, C. Doyle, M. Kulasa, A. Martyn, and C. Reynolds for extensive help in the field and laboratory. We are grateful to S. Hale, D. Kane, K. Krieger, K. Page, T. Sindt, R. Zweifel, and 3 anonymous reviewers for providing reviews that greatly improved the quality of this manuscript. This research project was funded by the Federal Aid in Sport Fish Restoration Program (F-69-P, Fish Management in Ohio) administered jointly by the US Fish and Wildlife Service and the Ohio Department of Natural Resources, Division of Wildlife.
References
- [APHA] American Public Health Association, American Water Works Association, Water Environment Federation . 2005 . Standard methods for the examination of water and wastewater, 21st ed. Washington , DC
- Barbiero , R P and Kann , J . 1994 . The importance of benthic recruitment to the population development of Aphanizomenon flos-aquae and internal loading in a shallow lake . J Plankton Res. , 16 : 1581 – 1588 .
- Boros , G , Tátrai , I , György , ÁI , Vári , Á and Nagy , A S . 2009 . Changes in internal phosphorus loading and fish population as possible causes of water quality decline in a shallow, biomanipulated lake . Int Rev Hydrobiol. , 94 : 326 – 337 .
- Carpenter , S R , Kitchell , J F and Hodgson , J R . 1985 . Cascading trophic interactions and lake productivity . BioScience , 35 : 634 – 639 .
- Clark , CF. 1960 . Lake St. Marys and its management , Columbus , OH : Ohio Department of Natural Resources-Division of Wildlife Publication W-324 .
- Cooke , G D , Welch , E B , Martin , A B , Fulmer , D G , Hyde , J B and Schrieve , G D . 1993 . Effectiveness of Al, Ca, and Fe salts for control of internal phosphorus loading in shallow and deep lakes . Hydrobiologia. , 253 : 323 – 335 .
- Cooke , G D , Welch , E B , Peterson , S A and Nichols , S A . 2005 . Restoration and management of lakes and reservoirs, 3rd ed. , Boca Raton , FL : Taylor & Francis .
- Culver , D A , Madon , S P and Qin , J . 1993 . Percid pond production techniques: timing, enrichment, and stocking density manipulation . J Appl Aquacult. , 2 : 9 – 31 .
- Davic , R D , DeShon , J and Eicher , D . 1995 . 1994 Ohio water resource inventory: Volume 3: Ohio's public lakes, ponds, and reservoirs , Columbus , OH : Ohio Environmental Protection Agency Technical Bulletin MAS/1995-7-2-III .
- Davic , R D , Eicher , D and DeShon , J . 1997 . 1996 Ohio water resource inventory: Volume 3: Ohio's public lakes, ponds, and reservoirs , Columbus , OH : Ohio Environmental Protection Agency Technical Bulletin MAS/1997-10-2 .
- Filbrun , J E , Reynolds , C A and Culver , D A . 2013 . Effects of feeding rate on habitat quality in fish rearing ponds . J. World Aquacult. Soc. , 44 : 198 – 209 .
- Forrest , A L , Fattah , K P , Mavinic , D S and Koch , F A . 2008 . Optimizing struvite production for phosphate recovery in WWTP . J Environ Eng. , 134 : 395 – 402 .
- [GLWWA] Grand Lake/Wabash Watershed Alliance . 2008 . Grand Lake St. Marys/Wabash River: watershed action plan . Darke, Auglaize, and Mercer counties’ Soil and Water Conservation Districts and the City of Celina (OH)
- Havens , KE. 1993 . Responses to experimental fish manipulations in a shallow, hypereutrophic lake: the relative importance of benthic nutrient recycling and trophic cascade . Hydrobiologia. , 254 : 73 – 80 .
- Heerdt , G and Hootsmans , M . 2007 . Why biomanipulation can be effective in peaty lakes . Hydrobiologia. , 584 : 305 – 316 .
- Holmes , R M , Aminot , A , Kérouel , R , Hooker , B A and Peterson , B J . 1999 . A simple and precise method for measuring ammonium in marine and freshwater ecosystems . Can J Fish Aquat Sci. , 56 : 1801 – 1808 .
- Homer , C , Dewitz , J , Fry , J , Coan , M , Hossain , N , Larson , C , Herold , N , McKerrow , A , VanDriel , J N and Wickham , J . 2007 . Completion of the 2001 National Land Cover Database for the conterminous United States . Photogram Eng Remote Sensing. , 73 : 337 – 341 .
- Hoorman , J , Hone , T , Sudman , T Jr , Dirksen , T , Iles , J and Islam , K R . 2008 . Agricultural impacts on lake and stream water quality in Grand Lake St. Marys, western Ohio . Water Air Soil Pollut. , 193 : 309 – 322 .
- Istvánovics , V. 1988 . Seasonal variation of phosphorus release from the sediments of shallow Lake Balaton (Hungary) . Water Res. , 22 : 1473 – 1481 .
- Jacob , A P and Culver , D A . 2010 . Experimental evaluation of the impacts of reduced inorganic phosphorus fertilization rates on juvenile saugeye production . Aquaculture. , 304 : 22 – 33 .
- Jacoby , J M , Lynch , D D , Welch , E B and Perkins , M A . 1982 . Internal phosphorus loading in a shallow eutrophic lake . Water Res. , 16 : 911 – 919 .
- Kalff , J. 2002 . Limnology: inland water ecosystems , Upper Saddle River , NJ : Prentice Hall .
- Lewis , W M Jr. 2011 . Global primary production of lakes: 19th Baldi Memorial Lecture . Inland Waters , 1 : 1 – 28 .
- [NCWQR] National Center for Water Quality Research . 2012 . Tributary data download , Heidelberg University . [cited 9 May 2012] Available from: http://www.heidelberg.edu/ academiclife/distinctive/ncwqr/data
- [OEPA] Ohio Environmental Protection Agency . 2007 . Total maximum daily loads for the Beaver Creek and Grand Lake St. Marys watershed .
- [OSU] Ohio State University . 2005 . Ohio agronomy guide, 14th ed. , Columbus , OH : Ohio State University . Extension Bulletin 472-05
- O’Melia , CR. 1972 . “ Coagulation and flocculation ” . In Physiochemical processes for water quality control , Edited by: Weber , W J Jr . 61 – 109 . New York , NY : Wiley and Sons .
- Paerl , H W , Hall , N S and Calandrino , E S . 2011 . Controlling harmful cyanobacterial blooms in a world experiencing anthropogenic and climatic-induced change . Sci Total Environ. , 409 : 1739 – 1745 .
- Rabalais , N N , Turner , R E , Días , R J and Justíc , D . 2009 . Global change and eutrophication of coastal waters . ICES J Mar Sci. , 66 : 1528 – 1537 .
- Reitzel , K , Hansen , J , Jensen , H S , Andersen , Fø and Hansen , K S . 2003 . Testing aluminum addition as a tool for lake restoration in shallow, eutrophic Lake Sønderby, Denmark . Hydrobiologia. , 506–509 : 781 – 787 .
- Scheffer , M , Hosper , S H , Meijer , M-L , Moss , B and Jeppesen , E . 1993 . Alternative equilibria in shallow lakes . Trends Ecol Evol. , 8 : 275 – 279 .
- Søndergaard , M , Jensen , J P and Jeppesen , E . 1999 . Internal phosphorus loading in shallow Danish lakes . Hydrobiologia. , 408/409 : 145 – 152 .
- Søndergaard , M , Jensen , J P and Jeppesen , E . 2003 . Role of sediment and internal loading of phosphorus in shallow lakes . Hydrobiologia , 506–509 : 135 – 145 .
- Søndergaard , M , Bjerring , R and Jeppesen , E . 2012 . Persistent internal phosphorus loading during summer in shallow eutrophic lakes . Hydrobiologia , doi: DOI 10.1007/s10750-012-1091-3
- Sønnichsen , T. 1977 . Toxicity of a phosphate-reducing agent (aluminum sulphate) on the zooplankton in the Lake Lyngby Sø . Verh Internat Verein Limnol. , 20 : 709 – 713 .
- Spears , B M , Carvalho , L , Perkins , R , Kirika , A and Paterson , D M . 2012 . Long-term variation and regulation of internal phosphorus loading in Loch Leven . Hydrobiologia. , 681 : 23 – 33 .
- Spivak , A C , Vanni , M J and Mette , E M . 2011 . Moving on up: can results from simple aquatic mesocosms experiments be applied across broad spatial scales? . Freshwater Biol. , 56 : 279 – 291 .
- Stumm , W and Morgan , J J . 1970 . Aquatic chemistry: an introduction emphasizing chemical equilibria in natural waters , New York , NY : Wiley and Sons .
- TetraTech . 2011 . Preliminary draft report: 2011 alum treatment and associated water quality monitoring for Grand Lake St. Marys , Columbus , OH : prepared for OEPA and USEPA .
- TetraTech . 2012 . Final draft: Effectiveness of a 2011 alum treatment, future phosphorus load reductions and associated water quality monitoring for Grand Lake St. Marys , Columbus , OH : prepared for OEPA and USEPA .
- Tobin , R L and Youger , J D . 1977 . Limnology of selected lakes in Ohio—1975 . US Geological Survey Water-Resources Investigations , Report 77–105
- [USBC] US Bureau of the Census . 1952 . Census of agriculture: 1950 , Washington , DC : US Department of Commerce .
- [USBC] US Bureau of the Census . 1956 . Census of agriculture: 1954 , Washington , DC : US Department of Commerce .
- [USBC] US Bureau of the Census . 1962 . Census of agriculture: 1959 , Washington , DC : US Department of Commerce .
- [USBC] US Bureau of the Census . 1967 . 1964 Census of Agriculture , Washington , DC : US Department of Commerce .
- [USBC] US Bureau of the Census . 1973 . 1969 Census of Agriculture , Washington , DC : US Department of Commerce .
- [USBC] US Bureau of the Census . 1977 . 1974 Census of Agriculture , Washington , DC : US Department of Commerce .
- [USBC] US Bureau of the Census . 1981 . 1978 Census of Agriculture , Washington , DC : US Department of Commerce .
- [USBC] US Bureau of the Census . 1984 . 1982 Census of Agriculture , Washington , DC : US Department of Commerce .
- [USBC] US Bureau of the Census . 1989 . 1987 Census of Agriculture , Washington , DC : US Department of Commerce .
- [USBC] US Bureau of the Census . 1994 . 1992 Census of Agriculture , Washington , DC : US Department of Commerce .
- [USDA] US Department of Agriculture . 1999 . 1997 Census of Agriculture , Washington , DC : National Agricultural Statistics Service .
- [USDA] US Department of Agriculture . 2004 . 2002 Census of Agriculture , Washington , DC : National Agricultural Statistics Service .
- [USDA] US Department of Agriculture . 2009 . 2007 Census of Agriculture , Washington , DC : National Agricultural Statistics Service .
- [USEPA] US Environmental Protection Agency . 1975 . Report on Grand Lake of St. Marys, Auglaize and Mercer counties, Ohio, EPA Region V. , Las Vegas , NV : National Eutrophication Survey . Working Paper No. 411
- [USEPA] US Environmental Protection Agency . 2009 . National lakes assessment: a collaborative survey of the nation's lakes , Washington , DC : Office of Water and Office of Research and Development Report 841-R-09-001 .
- [USGS] US Geological Survey . 2012 . USGS Ohio Water Science Center; [cited 9 May 2012] . Available from: http://oh.water.usgs.gov
- Van Hullebusch , E , Auvray , F , Deluchat , V , Chazal , P M and Baudu , M . 2003 . Phosphorus fractionation and short-term mobility in the surface sediment of a polymictic shallow lake treated with a low dose of alum (Courtille Lake, France) . Water Air Soil Pollut. , 146 : 75 – 91 .
- Vanni , MJ. 2002 . . Nutrient cycling by animals in freshwater ecosystems . Annu Rev Ecol Syst. , 33 : 341 – 370 .
- Welch , E B and Schrieve , G D . 1994 . Alum treatment effectiveness and longevity in shallow lakes . Hydrobiologia. , 275/276 : 423 – 431 .
- Wetzel , RG. 2001 . Limnology: lake and river ecosystems. 3rd ed. , San Diego , CA : Academic Press .
- [WHO] World Health Organization . 2003 . Guidelines for safe recreational water environments , Vol. 1 , Geneva , , Switzerland : coastal and fresh waters .