Abstract
Walnut Canyon Reservoir is a small source-water reservoir for the City of Anaheim, California. The reservoir receives raw water from the Metropolitan Water District of Southern California and is used for source water storage and emergency storage for the Lenain Filtration Plant. The reservoir naturally stratifies through the summer, with anoxia and elevated concentrations of phosphorus (P), nitrogen (N), manganese (Mn), and sulfide present in the hypolimnion. Water quality modeling using the 1-D DYRESM-CAEDYM model was conducted to evaluate different management strategies for the reservoir. Simulations evaluated the water column conditions in 2010 with (1) existing conditions, with operation of a SolarBee; (2) diffused aeration; (3) hypolimnetic oxygenation; and (4) flow routing with bottom withdrawal. Meteorological data from the nearby California Irrigation Management Information System stations were used to drive the hydrodynamic component of the model; the model was validated using available profile measurements and discrete sampling from April to July 2010. Diffused aeration, hypolimnetic oxygenation, and flow routing with bottom withdrawal were all predicted to improve dissolved oxygen conditions and lower concentrations of P, Mn, and other elements, although flow routing with bottom withdrawal could be implemented without incurring significant capital and operating costs; therefore, the flow routing strategy was implemented in spring 2011. Water column sampling demonstrated improved water quality in line with model predictions and indicated that flow routing with bottom withdrawal can serve as a practical and effective alternative to other engineered solutions under favorable hydraulic and source water quality conditions.
Stratification in lakes and reservoirs often results in anoxic or anaerobic conditions in bottom waters with accumulation of dissolved nutrients, sulfide, and reduced forms of iron (Fe) and manganese (Mn). In source drinking water reservoirs, these potential contaminants can constrain use of the water or increase treatment costs. As a result, diffused aeration systems or, in some cases, impeller-based mixing/circulation systems, are often used to enhance natural mixing processes and help deliver dissolved oxygen (DO) to the lower portions of the water column (Lorenzen and Fast Citation1977, Holdren et al. Citation2001).
These systems have significant capital costs, however. Diffused aeration systems also require potentially large inputs of power to operate and can thus have sizeable ongoing operation and maintenance costs. Impeller-type mixing systems (Punnett Citation1991) use less power, although they are less commonly used and may not be suited for some lakes (Lawson and Anderson Citation2007). Small solar-powered impeller systems are also available, although few published studies are available concerning their performance (Barkoh et al. Citation2011). Alternatively, hypolimnetic oxygenation systems have been installed in systems to deliver oxygen directly to the lower water column and maintain thermal stratification, but these systems also have substantial initial capital costs and have large ongoing operating and maintenance costs as well (Holdren et al. Citation2001).
Hypolimnetic withdrawal has also been used in some reservoirs to remove internally recycled nutrients as well as sulfide and other reduced chemical species (Macdonald et al. Citation2004, Nürnberg Citation2007). Removal of nutrients via this method has been found to reduce concentrations of chlorophyll and increase Secchi depth transparencies (Nürnberg Citation2007). Successful implementation of the method involves avoidance of large temperature changes that increase rates of sediment oxygen demand and internal nutrient recycling, however, as well as maintenance of the thermal stratification of the water column (Nürnberg Citation2007).
Selective withdrawal from discrete depths of the water column has also been used in some cases to manage cyanobacteria and taste-and-odor problems (Hoyer et al. Citation2009, Lehman et al. Citation2009). Phytoplankton have different light requirements, growth rates, and different sinking and physiological migration rates; greater stability of the water column tends to favor cyanobacteria while reduced stability favors diatoms and chlorophytes (Huisman et al. Citation2004, Hoyer et al. Citation2009). As a result, selective withdrawal has sometimes been implemented with the goal of destabilizing the water column, altering the light regime, and exporting nuisance algae. Rigosi and Rueda (Citation2012) recently completed a careful simulation study demonstrating that hydraulic effects (flushing) dominated phytoplankton community response to selective withdrawal, although environmental changes, especially differences in light regime, could also favorably alter the phytoplankton community. Environmental changes can be induced from hydraulically accelerated vertical displacement superimposed on natural sinking and migration rates (Rigosi and Reuda Citation2012).
Different approaches have thus been used to improve water quality depending on the specific situation and water quality goal. In this study, we evaluated several different strategies to improve water quality in a source water supply reservoir in southern California. We were particularly interested in the ability to mix the reservoir using turbulent kinetic energy (TKE) inputs due to inflow in conjunction with bottom withdrawal. Mixing energy to lakes and reservoirs is chiefly derived from wind shear on the water surface, although convective mixing and advective flow can also be important sources of TKE to surface waters (Martin and McCutcheon Citation1999).
Run-of-the river reservoirs that are operated for hydroelectric power generation and have large advective flows and short hydraulic detention times are often relatively well-mixed vertically (Martin Citation2007, Nogueira et al. Citation2012), although source and emergency drinking water storage reservoirs generally have longer detention times and are often naturally thermally stratified. Installation and operation of a diffused aeration or other system is often a standard practice for storage reservoirs to supplement natural wind and convective mixing. Under the right hydraulic and reservoir basin characteristics, routing flows through a source water storage reservoir can supplement natural wind- and convective-mixing processes and potentially improve water quality without installation or operation of diffused aeration or other systems.
Numerical modeling is increasingly used to guide lake and reservoir management; recent examples include an assessment of the effects of expansion of the Los Vaqueros Reservoir in California (Martin et al. Citation2013), modeling of water age and thermal structure of Lake Mead under changing water levels (Li et al. Citation2010), effects of hypolimnetic oxygenation in Lake Serraia in Italy (Toffolon and Serafini Citation2013), and the modeling of surface flow and transport in Lake Pontchartrain in Louisana (Chao et al. Citation2012).
The objectives of this study were to (1) evaluate alternative management strategies to improve water quality in Walnut Canyon Reservoir, California, through numerical modeling; (2) implement the most cost-effective strategy, and (3) assess effectiveness through water column measurements.
Study site
Walnut Canyon Reservoir is a small source drinking water reservoir operated by the City of Anaheim, California (; ). The reservoir receives raw water from the Metropolitan Water District of Southern California (MWD) delivered to north and/or south inlets at 242.9 and 247.5 m a.s.l. (). Water is withdrawn through a 4-tier outlet structure at the south end of the reservoir by 1.84 m diameter ports at 226.2, 235.6, 243.0, and 250.2 m a.s.l.
Table 1 Basin characteristics of Walnut Canyon Reservoir.
The reservoir has traditionally been operated as an emergency source supply capable of meeting 30–45 days of demand, with source water flows typically routed from MWD's Santiago Lateral through junction and bypass structures directly to the Lenain Filtration Plant (i.e., bypassing the reservoir; ). Treatment plant staff had historically operated a diffused aeration system in the lake through much of the year, although they installed a SolarBee (a floating, solar-powered circulator) in 2009 in an effort to reduce operation and maintenance costs and increase system reliability. Poor water quality resulted, including anoxia in the hypolimnion with high concentrations of nutrients, Mn2+, Fe2+, and sulfide as HS−, as well as high springtime concentrations of geosmin. These poor water quality factors, combined with constrained use of the reservoir and increased treatment costs, led to this assessment of in-lake management alternatives.
Methods
Analytical calculations of thermal stability and TKE inputs
Temperature profiles were used with the lake elevation–area relation to calculate the thermal stability of the water column. Thermal (Schmidt) stability is the amount of work needed to overcome buoyant forces due to stratification and completely mix the water column without loss or addition of heat (Idso Citation1973). Schmidt stability, S (J/m2), was calculated as (Idso Citation1973):
(1) where g is the acceleration due to gravity (m/s2); A0 is the surface area of the lake (m2); Az is the lake area at depth z (m); z* is the depth where the mean density occurs, ρz is the density (kg/m3) calculated from the temperature at depth z; ρ* is the volume-weighted mean density of the water column; and dz is the depth interval. The density of water at a given temperature was calculated using the equation of state for water (Martin and McCutcheon Citation1999). The effect of salinity on water density was negligible because electrical conductance of the water column was low and essentially constant with depth on all sampling dates (data not shown), such that vertical density differences (Equationequation 1
(1) ) arose only due to temperature effects.
TKE inputs into the reservoir due to wind shear were calculated for comparison with water column stability and mixing energy inputs due to flow (and momentum) routing through the reservoir. TKE due to wind shear, TKEwind (W/m2), was calculated as (Martin and McCutcheon Citation1999):
(2)
where Ck* is a dimensionless factor that accounts for the efficiency of wind energy in mixing the surface layer, taken here as 0.23; ρw is the density of water (kg/m3); CD is a dimensionless drag coefficient (1.3 × 10−3); ρa is the density of air (1.2 kg/m3); and Uw is the wind speed 10 m from the water surface (m/s).
The TKE input into the water column due to routing of flow into the reservoir was calculated from (Imboden Citation1980):
(3)
where TKEinflow is the turbulent kinetic energy input due to inflow (W/m2); Qinflow is the flow rate (m3/s); Uinflow is the flow velocity at the face of the inlet pipe (m/s); ρw is the density of water (kg/m3); and Ao is the surface area of the lake (m2).
Numerical modeling
The 1-D hydrodynamic-water quality model DYRESM-CAEDYM (Hipsey et al. Citation2006, Imerito Citation2007) was used to predict temperature and water quality in Walnut Canyon Reservoir for the 2010 study period. DYRESM-CAEDYM is used worldwide to simulate the physical, chemical, and biological processes and properties of lakes and reservoirs. DYRESM uses a Lagrangian layer scheme to simulate temperature and density profiles based on shortwave and longwave heat inputs, heat loss due to evaporation, back-radiation and convection, and mixing energy due to wind-forcing and advective and convective forces (Imerito Citation2007).
Daily meteorological data (shortwave heat flux, wind speed, air temperature, dew point temperature, and cloud cover) for 2010 were taken from California Irrigation Management Information Systems (CIMIS) stations #75 and 78 (at Irvine and Pomona, CA, respectively) and averaged as input to the model (CIMIS 2010). Wind speed was corrected to 10 m elevation using the relationship of Mackay and Yeun (Citation1983). Daily water inflows and outflows were taken from monthly treatment plant records and assumed to be uniform within each month. Influent water quality was defined based on available measurements. An isothermal water column at 12 C was used as the initial water column condition for January 2010.
Four scenarios were evaluated using 2010 meteorological and flow conditions in the initial phase of this study: (1) existing (reference) conditions, with SolarBee in operation; (2) diffused aeration; (3) hypolimnetic oxygenation; and (4) flow routing with bottom withdrawal. In scenario 1, existing (reference) conditions, which also served as the model validation, the model was set up to reproduce the reservoir's 2010 operating conditions, including limited inflow/outflow and operation of the SolarBee. The operation of the SolarBee was simulated using the impeller mixing subroutine within DYRESM with a 0.91 m diameter impeller and 6.1 m draft tube delivering a rated flow of 0.189 m3/s. Scenario 2 involved the continuous operation of a 160-port diffuser at the deepest location in the reservoir, flowing air at a rate of 0.04 m3/s at the diffuser. This flow rate was chosen based on scaling of other reported air flow rates successful in lake mixing (Ashley et al. Citation1987). Scenario 3 evaluated hypolimnetic oxygenation, simulated by setting a small positive oxygen flux from the sediments at 0.3 g/m2/d to offset sediment oxygen demand and the oxygen demand that results from aerobic respiration and decomposition reactions within the hypolimnion. Scenario 4 investigated the influence of routing the average flow of the MWD delivery (approximately 38,000 m3/d) through the reservoir at the south inlet (247.5 m a.s.l.) with withdrawal from the lowest outlet port at 226.2 m a.s.l. (30.9 m depth at crest elevation).
Field sampling and laboratory measurements
Measurements of water column conditions and water quality were conducted from 29 April 2010 through 28 September 2011. Sampling frequency was limited during the winter (monthly or less frequently) but increased to weekly to biweekly during the summer. Measurements of temperature, DO, electrical conductance, and pH were made using an YSI sonde generally mid- to late-morning at ∼1–2 m depth intervals, and transparency was measured using a Secchi disk. Water samples were collected using a Kemmerer sampler at 3 depths (surface, mid, and bottom) at the deepest location near the outlet. Samples were returned to the lab and analyzed for ammonium-N (NH4-N), dissolved phosphate-P (PO4-P), total iron (Fe), total manganese (Mn), sulfide, geosmin, and 2-methylisoborneol (2-MIB) following Standard Methods (APHA/AWWA/WEF 2012).
Results
Analytical calculations
Calculations of thermal stability and the TKE inputs available from wind and advective flow provide useful insights into mixing energy available through routing of flow through the reservoir. Schmidt thermal stability was calculated using Equationequation 1(1) for 3 dates in 2010 (29 Apr, 14 Jun, and 22 July) based on measured water surface elevations, hypsography, and temperature profiles (; ). Temperature profile measurements indicate presence of thermal stratification in April, with an 18.5 C epilimnion extending down to about 5 m depth and a cooler (∼15 C) hypolimnion present below 20 m depth ().
Table 2 Physical conditions present on calibration sampling dates.
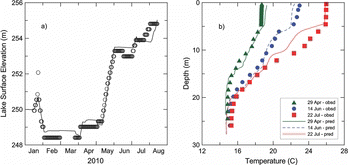
Significant work would be needed to overcome the net buoyant force associated with the temperature/density gradients; onset of thermal stratification in the spring yielded a Schmidt stability of 112 J/m2 on 29 April 2010. The TKE input to the water column available due to wind, at a mean daily wind speed of 1.95 m/s, was calculated from Equationequation 2(2) to be 3.33 × 10−6 W/m2 (). In comparison, work available to mix the water column from advective inflow was an order of magnitude greater (3.1 × 10−5 W/m2). Subsequent warming of the epilimnion later in the spring and summer () resulted in further increases in thermal stability of the water column, reaching a value of 533 J/m2 by 22 July ().
Mean wind speeds varied somewhat over these dates and correspondingly yielded somewhat different TKEwind inputs, but the increase in thermal stability clearly underscores the inability of wind to mix the water column (). Advective flows could potentially increase TKE input to the reservoir by 10–20 times that available due to wind. Moreover, while wind shear acts on the water surface, advective flows can deliver TKE within the water column at depths dependent on water surface elevation and influent structure elevations, and thus potentially deliver TKE directly to the metalimnion and weaken stratification.
These calculations do not include the export of cool hypolimnetic water through bottom withdrawal, however, which would also weaken thermal stability. Numerical modeling was thus conducted to provide a more complete analysis of the energy and heat budgets for the reservoir, effects of flow routing with bottom withdrawal, and other alternative management strategies.
Numerical simulations
Model validation
As the first step in this analysis, model validation was conducted using available water column, inflow, and related data for 2010. Limited availability of data required some assumptions, and therefore model default values were used without further calibration. The DYRESM model has previously been found to reliably predict water surface elevation, temperature, and salinity without calibration, however. The model reasonably reproduced observed trends in surface elevation of the lake, especially given the assumption of uniform daily flow within each month due to the monthly treatment plant flow records (). The root mean square error (RMSE) for water surface elevation was 0.34 m. The lake level increased in early to mid-January due to net inflows, although net withdrawals lowered the lake level to about 248.4 m a.s.l. for most of February and March. Water levels increased substantively in May to over 253 m, were largely unchanged in July, and then increased further in August ().
Over the April–August 2010 time period for which initial profile measurements were available, the model also adequately reproduced temperature (RMSE = 0.71 C) and thermal stratification in the lake (). The measured and predicted temperature profiles reveal a warm epilimnion about 5 m thick, with a 15 m thick metalimnion, and a cool hypolimnion below 20 m depth during summer stratification ().
The CAEDYM ecology-water quality model is less robust than DYRESM due to the ecological and biogeochemical complexity and unique features of a given lake or reservoir (Martin et al. Citation2013). DO concentrations in April 2010 were well reproduced by the model (, triangles), although substantial deviations were found below the epilimnion in June and July () following large net inflows from MWD that greatly increased the water surface elevation in May 2010 (). Specifically, while both modeled and measured DO concentrations dropped to <0.1 mg/L in the deepest water, measured values declined more rapidly with depth than modeled values (). The model thus significantly underpredicted the rate of DO depletion within the metalimnion during the summer, seemingly correlated with MWD inflows ().
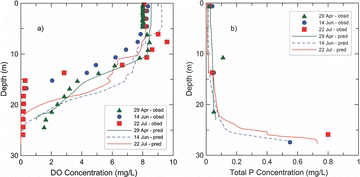
These marked deviations are thought to be due to delivery of detrital organic matter and low levels of chlorine into the epilimnion, with potentially further algal mortality and induced oxygen demand. MWD provides some chlorination of source water distributed to other agencies to control spread of quagga mussel (Dreissena rostriformis) veligers. Total chlorine measured on inflows ranged from <0.1 to 0.3 mg/L over the April–August 2010 time period. Predicting the effects of chlorine on the biology and chemistry of the reservoir was beyond the capability of the model, however. For the purposes of this study, the discrepancy between measured and modeled metalimnetic DO concentrations was considered tolerable, given the circumstances, because the model did correctly reproduce temperature profiles () and reasonably represented DO concentrations in the epilimnion and hypolimnion ().
While neither chlorophyll a concentrations nor direct measures of the phytoplankton community were conducted as part of this study, Secchi disk transparencies were quantified. Secchi disk depths were reasonably reproduced by the model during the summer months (Jun–Aug 2010), with measured values of 3.4–4.2 m versus predicted values of 3.0–3.7 m (RMSE = 0.38 m), but transparencies were underpredicted during the spring when MWD inflows increased water surface elevation and free chlorine levels.
DYRESM-CAEDYM also predicted low total phosphorus (P) concentrations in the epilimnion and markedly higher concentrations above the sediments, consistent with measured values (). High concentrations of total nitrogen (N; principally as NH4-N) and Mn2+ above the bottom sediments were also both predicted and observed (data not shown). The DYRESM-CAEDYM model used in this study does not simulate sulfide production, although our previous work on other lakes in the region typically find S2− concentrations tend to track increases in NH4-N and PO4-P at ratios of 1–2:1 S:NH4-N and 2–3:1 S:PO4-P on a mass basis. Thus, predicted bottom water concentrations of PO4-P of ∼0.6 mg/L () suggested sulfide concentrations of 1.2–1.8 mg/L and compared favorably with available measured value of 1.53 mg/L on 22 July 2010.
The general agreement between predicted and available measured values (with the exception of metalimnetic DO) provided some confidence in the model as a useful screening tool for alternative management strategies for Walnut Canyon Reservoir.
Evaluation of in-lake management alternatives
The potential water quality improvements achieved through aeration (scenario 2), hypolimnetic oxygenation (scenario 3), and flow routing with bottom withdrawal (scenario 4) were evaluated. Full 1-year simulations were conducted that began by assuming isothermal conditions on 1 January 2010. The simulated temperature conditions for the 2010 operational scenario 1 (existing 2010 reference conditions with SolarBee 6.1 m draft tube) were cool, well-mixed conditions present during the short winters and warm surface water temperatures with strong stratification present during the summer (). Note that the y-axis is taken here as height above the bottom; this convention allows one to also inspect the changes in lake surface elevation due, in this case, to inflow–outflow and, to a lesser extent, also evaporation.
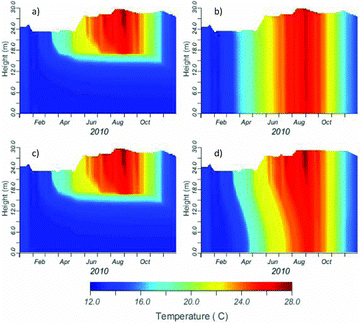
Aeration (scenario 2) resulted in temperature profiles that deviated markedly from the existing condition, yielding uniform temperatures present throughout the water column (i.e., no thermal stratification present; ). Temperatures throughout the water column during the summer were similar to those found in the epilimnion in the existing (2010 reference) condition (scenario 1). The predicted temperature profiles under hypolimnetic oxygenation (scenario 3) were essentially identical to that for the reference case () because this technique as modeled did not impart additional TKE to the water column. The simulation of flow routing with bottom withdrawal (scenario 4) predicted marked warming of the bottom waters during the spring and summer, although some weak stratification was present into August ().
The predicted DO profiles also varied for the different scenarios. A well-aerated epilimnion was predicted through the year for the existing (reference) condition, with an anoxic hypolimnion present beginning in March, although note that anoxia in the hypolimnion persisted until the last day of the year in this simulation (). Thus, upwelling of some sulfide would likely lower DO concentrations for period of time following late fall mixing. In contrast, aeration was able to maintain high DO levels throughout the water column through the entire simulation, with cool waters and an apparent algal bloom in April yielding uniform water column concentrations near 9 mg/L (). Hypolimnetic oxygenation and flow routing with bottom withdrawal were both predicted to maintain concentrations of >4 mg/L DO in the hypolimnion through the summer ().
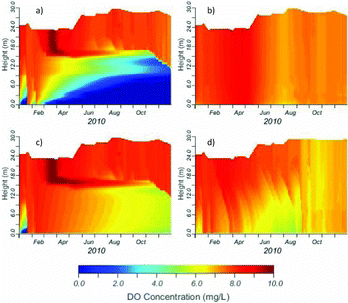
Predicted concentrations of dissolved PO4-P also varied markedly across the different treatments (, note differences in concentration scales). Under scenario 1, the existing 2010 (reference) conditions with operation of the SolarBee, PO4-P concentrations immediately above the deepest sediments were predicted to exceed 1.2 mg/L, with much lower concentrations elsewhere in the water column (). In contrast, under scenario 2, very low (<0.005 mg/L) dissolved PO4-P concentrations were predicted with aeration, with little vertical variation (). Under scenario 3, hypolimnetic oxygenation was predicted to reduce dissolved PO4-P concentration above the sediments by about 90%, reaching only 0.12 mg/L (), versus 1.2 mg/L under current conditions (). Under scenario 4, flow routing with bottom withdrawal was predicted to lower the dissolved PO4-P concentration in the bottom of the lake by more than 98%, reaching a maximum <0.01 mg/L (). Reductions in dissolved PO4-P are beneficial because less of this readily available form of P is available for mixing into the upper layers of the lake, thereby reducing the prospect for a subsequent algal blooms and possible taste-and-odor problems.
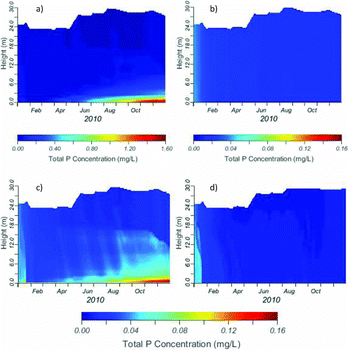
Secchi disk transparencies were predicted to be comparatively high under all scenarios, with mean annual values ranging from 4.27 ± 0.42 m for the existing 2010 condition to 4.56 ± 0.18 m with flow routing/bottom withdrawal. There were no statistically significant differences between the existing reservoir, aeration (4.31 ± 0.48 m), or hypolimnetic oxygenation (4.28 ± 0.42 m); flow-routing with bottom withdrawal yielded a statistically significantly higher transparency than the other scenarios (p < 0.001).
Because sulfide concentrations tend to follow dissolved PO4-P (and NH4-N) concentrations, these findings further indicate that aeration, hypolimnetic oxygenation and bottom withdrawal also lower the prospects for S2− problems in the reservoir. Assuming 3:1 S:PO4-P on a mass basis, a deep hypolimnetic S2− concentration of almost 5 mg/L is predicted by late fall under current practices (i.e., scenario 1) and compares reasonably with a measured value of 5.6 mg/L in late September, while a maximum S2− concentration in late summer of <0.02 mg/L would be expected with aeration and flow routing with bottom withdrawal (scenarios 2 and 4, respectively). Somewhat higher S2− concentrations were predicted for hypolimnetic oxygenation as simulated here (∼0.5 mg/L), but remain about 85% lower than concentrations predicted for 2010 with operation of the SolarBee. In practice, oxygen delivery would generally be increased to prevent accumulation of sulfide in the bottom waters, while a fixed (and not quite adequate) rate was used in the simulation.
This modeling analysis indicates that scenario 4, routing of flow through the reservoir in conjunction with bottom water withdrawal, can mix the water column of Walnut Canyon Reservoir relatively well, generally maintain DO levels >4 mg/L above the sediments, and prevent accumulation of high concentrations of reduced chemical species and their delivery to the Lenain Filtration Plant. Based on these results, flow-routing with bottom withdrawal was implemented at Walnut Canyon Reservoir in spring 2011.
Measured water column conditions prior to and following flow routing-bottom withdrawal
Observed water column conditions in Walnut Canyon Reservoir showed strong differences in 2011 following implementation of flow routing with bottom withdrawal (scenario 4) compared with 2010 with only operation of a SolarBee (scenario 1). Measured temperature and DO profile data are presented as contour plots () for comparison with simulation results ( and 5). The fewer number of measured profiles introduce greater variation in the contour plots, especially evident in 2010 data, when compared with daily model simulation output.
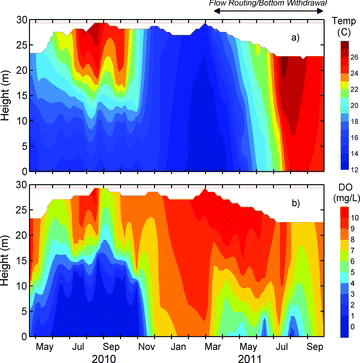
The reservoir exhibited strong thermal stratification in 2010 when only the SolarBee was operating (). Surface temperatures were routinely 25–26 C in the epilimnion and 15–16 C in the hypolimnion through the summer, with rapid cooling in October and mixing in November 2010 (). The water column was cool (12–14 C) and generally well-mixed in the winter (Dec–Mar) before warming in April–May (). With flows routed through the reservoir in 2011, only modest temperature gradients were present through the spring and summer, however, and contrasts strongly with the conditions present the previous year (). Flow routing with bottom withdrawal greatly increased TKE input to the water column and thus minimized the strength and duration of thermal stratification.
DO levels also displayed different conditions in summer 2010, when only the SolarBee was in place, compared with the summer of 2011 with flow routing with bottom withdrawal (). DO was essentially absent in the hypolimnion of the lake from June to October 2010 and was restored only in November when the lake mixed. Conversely, with flow routing and hypolimnetic withdrawal, DO remained high through March 2011 before declining modestly later in the spring, although it remained >4 mg/L through the entire water column through the summer with the exception of a single sampling date in July 2011 ().
Measured sulfide levels above the deepest sediments near the lowest intake also exhibited quite profound improvements with flow routing and bottom withdrawal (). Concentrations increased approximately linearly with time beginning in early June 2010 when DO levels dropped to <1 mg/L (); sulfide reached a maximum value of about 7 mg/L by late October 2010 (). Measurements were resumed the following March 2011 following implementation of flow routing with bottom withdrawal, with sulfide concentrations below detection through the spring and summer 2011.
Mn is another redox-sensitive element that can create treatment challenges for drinking water utilities. Concentrations of total Mn increased somewhat earlier than sulfide and reached a steady-state maximum value of ∼0.65 mg/L by July 2010, while concentrations remained <0.05 mg/L through summer 2011 with flow routing with bottom withdrawal except for the sampling date in late July 2011 () that also experienced a lower DO excursion ().
Total P concentrations exhibited strong differences as well, with concentrations above the bottom sediments approaching 1 mg/L through much of summer 2010, while concentrations were markedly lower during summer 2011 ().
Measurements of the phytoplankton community were not conducted as part of this study, although some measurements of taste-and-odor compounds were made. High geosmin concentrations were measured in April 2010, when concentrations reached almost 400 ng/L in the metalimnion and averaged >200 ng/L throughout the water column on 21 April 2010. In contrast, geosmin concentrations were below detection in April 2011 and reached a maximum of 9.1 ng/L in June 2011. This evidence indicates a favorable algal community shift away from taste-and-odor producing cyanobacteria as a result of flow routing with bottom withdrawal. A statistically significant increase in mean summer Secchi disk depth was also found (3.3 vs. 4.8 m, significant at p < 0.002). The scenario 4 approach thus markedly lowered algal taste-and-odor production and increased summer transparency when compared with operation of the SolarBee (scenario 1).
Schmidt thermal stability of the water column was calculated from sonde casts for the 2010–2011 study period using Equationequation 1(1) . Thermal stability increased from ∼100 J/m2 in April 2010 to a maximum value of ∼550 J/m2 in July–August, before declining through the fall to reach fully isothermal conditions in December 2010 (). While some thermal stability was present in spring and early summer with flow routing with bottom withdrawal in 2011, advective flows through the reservoir added energy (work) to the water column and thus substantially weakened thermal stratification.
Water column measurements were generally made in late morning when shortwave inputs are high yet natural TKE inputs due to wind are typically low (mid-to-late afternoon winds tend to impart the greatest wind shear and mixing force to the reservoir surface). As a result, these measured stability values are thought to be daily maximum values and not daily average values, which is especially relevant for 2011 when near-surface diurnal thermoclines dominate the calculated water column thermal stability. Notwithstanding, comparison of 2010 with 2011 values at comparable dates in the summer (Jun–Aug) indicates that flow routing with bottom withdrawal lowered the thermal stability of the water column by an average of 310 J/m2 (and up to 496 J/m2 in late Jul–early Aug; ). A substantial amount of work was thus provided by the routing of flow through the reservoir, with stability further weakened by removal of cool hypolimnetic water.
Water quality improvements were thus achieved without the need for installation and operation of a diffused aeration or other mixing system. Although only limited measurements were made in 2012, flow routing with bottom withdrawal continued to maintain high summer bottom water DO concentrations (>5.4 mg/L), increased Secchi depths (>4.3 m), and low concentrations of geosmin and 2-MIB (often below detection; maximum values of 6.3 and 9.0 ng/L, respectively).
Discussion
Analytical calculations and numerical simulations demonstrated the capability of flow routing with bottom withdrawal to substantially increase TKE inputs and help mix the water column in Walnut Canyon Reservoir. Numerical modeling further predicted increases in concentrations of DO and reductions in nutrients and reduced chemical species in the lower water column and in treatment plant influent without the need to install and operate diffused aeration or hypolimnetic oxygenation systems.
Based on these findings, flow routing with bottom withdrawal was implemented by the water utility the following year; subsequent monitoring demonstrated significant water quality improvements from use of this strategy relative to the existing (2010) conditions that included operation of a SolarBee. Favorable water quality conditions were also present in 2012; therefore, under suitable hydraulic and basin characteristics, flow routing with bottom withdrawal can yield marked improvements in water quality without the need for aeration or oxygenation systems. This effect is achieved through mixing of the water column and thus (1) delivering and maintaining adequate levels of DO in the hypolimnion to prevent accumulation of high levels of nutrients, sulfide, and other reduced species; and (2) favorably altering the phytoplankton community through flushing and alteration of the environmental conditions to limit cyanobacteria and production of taste-and-odor compounds. As with other destratification techniques, however, this approach does warm bottom waters, so it may not be appropriate for reservoirs with a cold-water fishery or those with downstream releases to sensitive habitat. This study also illustrates the value of numerical modeling as a tool in the development and assessment of lake management strategies.
Acknowledgments
The authors acknowledge the use of the models DYRESM and CAEDYM developed by the Centre for Water Research, University of Western Australia. We would also like to acknowledge and thank the Lenain Treatment Plant and City of Anaheim for their support and assistance in this study.
Funding
This project was partially supported by the USDA National Institute of Food and Agriculture through Hatch project CA-R-ENS-6696-H.
References
- [APHA/AWWA/WEF] American Public Health Association, American Water Works Association, and Water Environment Federation. 2012. Standard methods for the examination of water and wastewater. 22nd ed.
- Ashley KI, Hay S, Scholten GH. 1987. Hypolimnetic aeration: field test of the empirical sizing method. Water Res. 21:223–227.
- Barkoh A, Begley DC, Smith DG, Kurten GL, Fries LL, Schlechte JW. 2011. Can solar powered circulation control Prymnesium parvum blooms and toxicity in fish hatchery ponds? Harmful Algae. 10:173–180.
- Chao XB, Jia YF, Wang SSY, Hossain AKMA. 2012. Numerical modeling of surface flow and transport phenomena with applications to Lake Pontchartrain. Lake Reserv Manage. 28:31–45.
- [CIMIS] California Irrigation Management Information System. 2010. Department of Water Resources, State of California. . Available from: http://wwwcimis.water.ca.gov/
- Hipsey MR, Romero JR, Antenucci JP, Hamilton D. 2006. Computational Aquatic Ecosystem Model: CAEDYM v2.3 science manual. Centre for Water Research, University of Western Australia. 90 p.
- Holdren C, Jones A, Taggart J. 2001. Managing lakes and reservoirs. North American Lake Management Society and Terrene Institute, in cooperation with the Office of Water Assessment. Madison (WI): US Environmental Protection Agency. Watershed Protection Division.
- Hoyer AB, Moreno-Ostos E, Vidal J, Blanco JM, Palomino-Torres RL, Basanta A, Escot C, Rueda FJ. 2009. The influence of external perturbations on the functional composition of phytoplankton in a Mediterranean reservoir. Hydrobiologia. 636:49–64.
- Huisman J, Sharples J, Stroom JM, Visser PM, Kardinaal WEA, Verspagen JMH, Sommeijer B. 2004. Changes in the turbulent mixing shift competition for light between phytoplankton species. Ecology. 85:2960–2970.
- Idso SB. 1973. On the concept of lake stability. Limnol Oceanogr. 18(4):681–683.
- Imboden DM. 1980. The impact of pumped storage operation on the vertical temperature structure in a deep lake: a mathematic model. In: Clugston JP, editor. Proceedings of the Clemson Workshop on Environmental Impacts of Pumped Storage Hydroelectric Operations. US Dept of the Interior; Fish and Wildlife Service. FWS/OBS-80/28. p. 125–146.
- Imerito A. 2007. Dynamic Reservoir Simulation Model DYRESM v4.0 science manual. University of Western Australia, Centre for Water Research. 42 p.
- Lawson R, Anderson MA. 2007. Stratification and mixing in Lake Elsinore, California: An assessment of axial flow pumps for improving water quality in a shallow eutrophic lake. Water Res. 41:4457–4467.
- Lehman EM, McDonald KE, Lehman JT. 2009. Whole lake selective withdrawal experiment to control harmful cyanobacteria in an urban impoundment. Water Res. 43:1187–1198.
- Li Y, Acharya K, Chen D, Stone M. 2010. Modeling water ages and thermal structure of Lake Mead under changing water levels. Lake Reserv Manage. 26:258–272.
- Lorenzen M, Fast A. 1977. A guide to aeration/circulation techniques for lake management. US Environmental Protection Agency. . USEPA-600/3–77–004.
- Macdonald RH, Lawrence GA, Murphy TP. 2004. Operation and evaluation of hypolimnetic withdrawal in a shallow eutrophic lake. Lake Reserv Manage. 20:39–53.
- Mackay D, Yeun ATK. 1983. Mass transfer coefficient correlations for volatilization of organic solutes from water. Environ Sci Technol. 17:211–217.
- Martin B, Ding L, Hannoun IA, List EJ. 2013. Predicting effects of reservoir expansion with three-dimensional modeling: Case study of Los Vaqueros Reservoir. Lake Reserv Manage. 29:217–232.
- Martin JL. 2007. Energy production and reservoir water quality: A guide to the regulatory, technical and theoretical basis for required studies. Reston (VA): ASCE. 376 p.
- Martin JL, McCutcheon SC. 1999. Hydrodynamics and transport for water quality modeling. Boca Raton (FL): Lewis. 794 p.
- Nogueir, MG, Perbiche-Neves G, Naliato DAO. 2012. Limnology of two contrasting hydroelectric reservoirs (storage and run-of-river) in southeast Brazil. In: Samadi-Boroujeni H, editor. Hydropower: practice and application. InTech Publication. 320 p.
- Nürnberg GK. 2007. Lake responses to long-term hypolimnetic withdrawal treatments. Lake Reserv Manage. 23:388–409.
- Punnett RE. 1991. Design and operation of axial flow pumps for reservoir destratification. Washington (DC): US Army Corps of Engineers. . Report W-91–1. 34 p.
- Rigosi A, Reuda FJ. 2012. Hydraulic control of short-term successional changes in the phytoplankton assemblage in stratified reservoirs. Ecol Eng. 44:216–226.
- Toffolon M, Serafini M. 2013. Effects of artificial hypolimnetic oxygenation in a shallow lake. Part 2: Numerical modeling. J Environ Manage. 114:530–539.