Abstract
Line-diffuser hypolimnetic oxygenation (HO) was initiated in North Twin Lake, Washington, in 2009 to mitigate loss of coldwater fishery habitat due to temperature–dissolved oxygen “habitat squeeze” and to reduce internal phosphorus cycling. Active tracking, net-captures, and hydroacoustic analyses demonstrated that trout populations rapidly expanded into increased hypolimnetic habitat within the first few years of oxygenation; however, long-term fishery benefits and many basic ecological aspects of HO have yet to be established. Diet and food web analyses indicate significant changes in feeding ecology of principal coldwater fish species in North Twin in 2012 compared to preoxygenation (2005) and to unoxygenated South Twin in 2012. North Twin rainbow trout (Oncorhynchus mykiss) consumed significantly more large-bodied Daphnia during midsummer 2012 than in South Twin, where rainbow trout fed primarily on littoral amphipods. Additionally, relative gut weight for brook trout (Salvelinus fontinalis) in August 2012 was significantly higher in North Twin compared to South Twin, apparently due to increased access to hypolimnetic zooplankton. Golden shiner (Notemigonus crysoleucas) diets also seem to include more zooplankton in oxygenated North Twin. Littoral-focused largemouth bass (Micropterus salmoides) diets were not altered by HO. Observed changes in feeding ecology following HO have significant implications for future fishery management in the Twin Lakes.
“Habitat-squeeze” is a common summer phenomenon affecting coldwater fish species such as trout in many dimictic, eutrophic lakes. High summer temperatures preclude trout from fully using the epilimnion, while colder waters of the hypolimnion may be also unusable due to hypoxic or anoxic conditions (Coutant Citation1985, Aku and Tonn Citation1999, Swales Citation2006). This habitat squeeze limits lake volume available for feeding, reduces or eliminates trout access to food sources in the benthos, and provides hypoxia-tolerant zooplankton with a refuge from planktivorous trout (Taggart Citation1984, Doke et al. Citation1995, Wang et al. Citation1996). Restoration of adequate hypolimnetic dissolved oxygen (DO) through hypolimmnetic aeration or oxygenation may increase forage area and access to food resources, improve benthic habitat, and reduce crowding-related stressors (Doke et al. Citation1995, Dinsmore and Prepas Citation1997, Aku and Tonn Citation1999).
Hypolimnetic oxygenation (HO) increases DO in the hypolimnion and is an effective management strategy for improving and maintaining coldwater fish habitat with minimal alteration to thermal structure of the water column (Cooke et al. Citation2005). Benthos and zooplankton diversity and density may also increase with oxic conditions in sediments, creating additional food sources for coldwater fish (Aku and Tonn Citation1999, Cooke et al. Citation2005); however, few studies have addressed the potential effect of HO on trout diets, and apparently none have assessed potential effects on the diets of warmwater fish.
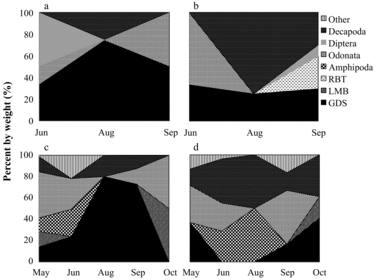
We hypothesized that rainbow and brook trout in the lake with HO would incorporate more pelagic prey into their diets and have higher relative gut weights than those in the untreated lake. Additionally, we anticipated that HO would minimally affect feeding ecology of the warmwater fish community. Diets were examined in a paired lake system in northeastern Washington (see Part I, Moore et al. Citation2014, this issue) that include a mixed cold- and warmwater fish community composed of rainbow trout (Oncorhychus mykiss), brook trout (Salvelinus fontinalis), largemouth bass (Micropterus salmoides), and golden shiner (Notemigonus crysoleucus). Our study includes side-by-side comparisons of an oxygenated and an untreated lake plus diet data prior to and following HO.
Study area
North Twin (NT) and South Twin (ST) lakes are mesotrophic, dimictic lakes located on the Colville Confederated Tribes (CCT) Reservation, 8.5 miles west of Inchelium, Washington (see Part I for details, Moore et al. Citation2014, this issue). By early July, epilimnetic temperatures typically exceed 21 C, and hypolimnia of both lakes are mostly anoxic. A line-diffuser HO was installed in NT in 2008 and was operated through summer stratification beginning in 2009.
Rainbow and brook trout subsistence fisheries were developed in Twin Lakes in the 1950s and are currently being managed as put–grow–take (B. Cross B, Fisheries Manager, CCT, 2013 pers. comm.). Additionally, largemouth bass and golden shiner were illegally introduced to the lakes in the 1980s (Christensen and Moore Citation2008). Fathead minnow (Pimephales pormelas) and redside shiner (Richardsonius balteatus) are also present in small numbers within the lakes. Both trout species represent the coldwater fish community, avoiding temperatures >21 C (Horak and Tanner Citation1964, Overholtz et al. Citation1977) and DO <4 mg/L (Spoor 1999). Largemouth bass and golden shiner are considered warmwater fish that tend to occupy littoral areas of the Twin Lakes (Christensen and Moore Citation2007), where temperatures usually exceed 21 C by early July. Preoxygenation details of the Twin Lakes fish communities are in Christensen and Moore (2007, 2008).
Materials and methods
Sampling stations were located at approximately the deepest point in each lake. Temperature and DO profiles were taken at 1 m intervals using a multiparameter probe (Hydrolab Minisonde 5a Water Quality Multiprobe equipped with a Hydrolab Surveyor Model 4a recording device).
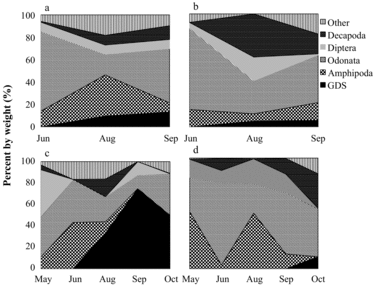
Fish sampling and enumeration are based on methods utilized by Christensen and Moore (Citation2007). Fish were collected by gillnets and electrofishing. Stomachs were collected from rainbow trout, brook trout, golden shiner, and largemouth bass from NT and ST in June, August, and September 2005. In 2012, fish stomachs were collected at both lakes in May, June, August, and September, with additional trout and bass stomachs collected in October 2012. Brook trout, rainbow trout, and pelagic golden shiner were sampled using sinking monofilament gillnets. Nets had varying mesh sizes and were set in both lakes overnight at varying depths for each sampling date. Nets were extended perpendicular to the shore in randomly assigned near-shore and pelagic areas, the pelagic defined as that portion of the lake beginning at the 8 m depth contour and extending 200 m toward the center of the lake. If fewer than 10 individuals per species size class were collected after a single night of sampling, nets were reset until this target minimum was met. Trout and golden shiner captures were sacrificed at the time of sampling, and stomachs were extracted whole. Pelagic golden shiners were collected only in 2012.
Largemouth bass and littoral golden shiners in littoral zones were sampled with a Smith-Root GPP 5.0 DC electrofishing boat equipped with umbrella boom-shaped anodes and hanging cable cathodes; duty cycle and range were adjusted to induce suitable fish taxis. Sampling occurred in the early evening and night for each lake in locations designated by Christensen and Moore (Citation2007). Captured fish were first measured for length, then stomach contents were sampled using a 60 cc syringe as a modified gastric lavage (Kamler and Pope Citation2001, Christensen and Moore Citation2007). A target of 10 largemouth bass stomachs per size class were collected from each lake. Size classes sampled include <100, 100–199, 200–299, and >299 mm (Christensen 2005, 2007, Christensen and Moore Citation2007). Occasionally, largemouth bass were also caught in gillnets set for trout and golden shiner; bass sampled in October were caught exclusively with gillnets. Littoral golden shiners were sampled only in 2005.
After capture, fish were weighed to the nearest gram and length measured to the nearest millimeter. Stomach samples were preserved in 70% alcohol, iced for transport, and stored at 4 C until analyzed. Prey fish present in stomach samples were counted and identified to species using a dichotomous key (Wydoski and Whitney Citation2003). When golden shiners were found as prey, we estimated their wet weights with length–weight regression formulas developed separately for each lake. Wet weights of other prey fish species were estimated using published length–weight regressions (Anderson and Neumann Citation1996). Macroinvertebrate prey were counted and identified to family or order using a dichotomous key (Merritt and Cummins Citation1996). Macroinvertebrate prey lengths were measured to the nearest 0.5 mm; if 10 or more of the same prey species were present in a stomach sample, a subset of 10 individuals from that species was measured (Liao et al. Citation2001).
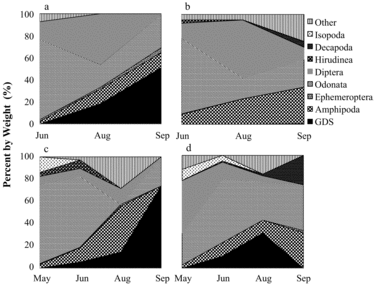
Dry weights for macroinvertebrates and zooplankton were estimated from length–weight regressions available in the literature (Dumont and Balvay 1978, Smock Citation1980, Benke et al. Citation1999, Johnston and Cunjak Citation1999, Watkins et al. 2011). Wet weights of macroinvertebrate prey were assumed to be 5 times greater than estimated dry weights (Morin and Dumont Citation1994). Dry weights of zooplankton were considered to be 7% of wet weights (Lawrence et al. Citation1987, Pelham et al. Citation2001). Percent by weight was determined for each prey species in the stomach samples (Chipps and Garvey Citation2007), and total gut weight was also calculated for each fish (Aku and Tonn Citation1999).
We used the Schoener's Diet Overlap Index (SDOI) to determine if dietary patterns varied between lakes before and during treatment (Schoener Citation1970, Pelham et al. Citation2001). This index ranges from 0 to 100, with 0 representing no common prey and 100 indicating identical diets; an overlap value of 60 or more is considered biologically significant, that is, likely to significantly impact fish growth, health, reproduction, or interspecific competition (Wallace Citation1981, Cortes Citation1997). Empty stomachs were excluded from overlap analyses (Pelham et al. Citation2001). We also compared diets within and between golden shiners and rainbow trout for both lakes and for each year, using 1-way and 2-way ANOVAs with SAS 9.3 (SAS Institute 2012), respectively, assuming significance at p < 0.05.
Stomach fullness was quantified as relative gut weight (RGW), with weight of stomach contents expressed as percentage of fish weight (Hyslop Citation1980, Aku and Tonn Citation1999):
(1) where, SCWi = weight of stomach contents of fishi (g), and BWi = total body weight of fishi (g). RGW for each species was compared by lake and month for 2005 and 2012 with a 2-way ANOVA in SAS 9.3 (SAS Institute 2012). Empty stomachs were included in RGW analyses.
Results and discussion
High epilimnetic temperatures and low hypolimnetic DO confined both trout species to the metalimnia of both lakes during midsummer 2005 and in ST in 2012 (Part I, Moore et al. Citation2014, this issue). Since full seasonal HO was initiated in NT in 2009, trout have been observed utilizing expanded hypolimnion habitat, while those in ST remain confined to the metalimnion between about 5 and 7 m during midsummer (Part I, Moore et al. Citation2014, this issue). The presence of anoxic hypolimnia in both lakes during 2005 and again in ST in 2012 also suggests Daphnia had a refuge from trout predation while anoxia persisted (Doke et al. Citation1995, Wang et al. Citation1996).
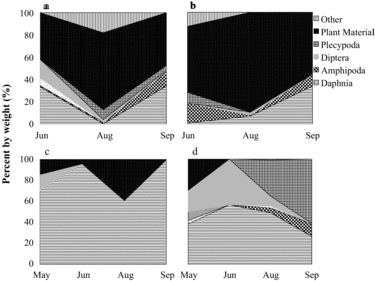
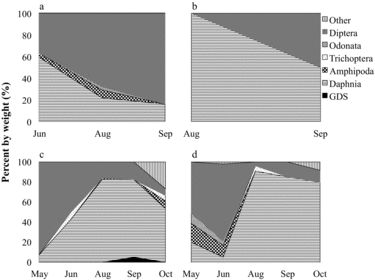
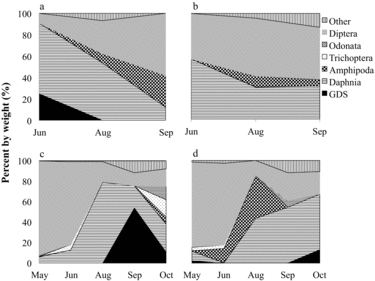
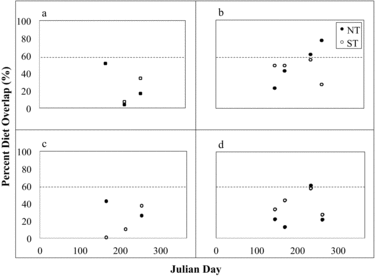
Largemouth bass
Too few of the small largemouth bass (<100 mm) were collected in 2005 or 2012 to warrant diet and RGW analyses. Diets of larger largemouth bass size classes varied seasonally and with size class in both lakes before and during HO (–). Smaller prey items such as odonates, amphipods, and dipterans were important components in diets of the smaller size classes, while large prey items such as decapods and golden shiners were more important in diets of larger bass. SDOI scores suggest bass diets varied regardless of lake, month, or year, with a mean diet overlap of 55.2 and 45.1% between lakes in 2005 and 2012, respectively. Largemouth bass RGW for the >299, 200–299, and 100–199 mm size classes showed a high degree of seasonal variation, with no clear pattern before or during treatment. Given their highly varied diet and low SDOI overlap scores before and during oxygenation, there is no evidence that HO had any influence on bass diets.
Golden shiners
Golden shiners primarily consumed plant material, dipterans, daphnids, plecypods, and other invertebrate prey (). There seems to have been a diet shift between 2005 and 2012 of decreasing plant material consumption and increasing daphnia intake, most notably in NT. In 2005, consumption of daphnids by littoral golden shiners was not significantly different between lakes (p = 0.6146). In 2012, pelagic NT golden shiners consumed significantly more daphnids than those in ST (p = 0.0028). Golden shiner SDOI values indicate a biologically significant diet overlap between lakes in 2005 (69%) when averaged across months. In 2012, diet overlap (50%) was not biologically significant when averaged across months due to higher Daphnia consumption in NT golden shiners (see later discussion).
An apparent increase in Daphnia consumption by NT golden shiners in 2012 may be an artifact of sampling methods, but this warrants further study. In 2005, golden shiners were sampled from the littoral zone while electrofishing for bass; in 2012, they were caught in near-shore and pelagic gill nets during trout sampling. Golden shiners typically migrate laterally from the littoral to the pelagic zone at sunset, allowing them to take advantage of vertical migration by Daphnia moving from the hypolimnion into the epilimnion (Hall et al. Citation1979). Golden shiners captured in the littoral zone during evening electrofishing in 2005 may have been individuals that did not move offshore. For future studies, golden shiners will be sampled from both littoral and pelagic zones of the Twin Lakes.
Increased Daphnia consumption by NT golden shiners in 2012 does not seem to be correlated with zooplankton abundance given that preliminary findings indicate that zooplankton have not increased in NT compared to ST (B. Cross, Washington State University, unpubl. data). HO may possibly be encouraging expanded utilization of Daphnia prey by golden shiners. Golden shiner RGW in both 2005 and 2012 varied between lakes and months (2005 lake*month p = 0.0057; 2012 lake*month p = 0.0040) without a consistent pattern, providing no clear evidence that HO altered stomach fullness.
Brook trout
Brook trout prey generally consisted of Daphnia, dipterans, and amphipods (). Daphnia consumption was greatest in early summer for 2005 and in midsummer for 2012. Brook trout had biologically significant diet overlap between lakes in 2005 (66%) and during thermal stratification in 2012 (82%), indicating that HO has had a minimal influence on brook trout diet. Oxygen delivery rates for 2012 were substantially reduced (P. Gantzer, Gantzer Water Resources Engineering LLC, 2013, pers. comm.), however, and hypolimnetic DO was not as high as observed in 2009 or 2011 (Part I, Moore et al. Citation2014, this issue). These results may therefore not be representative of full trout access to the hypolimnion.
Given the small number of brook trout sampled in 2005, RGW comparisons are limited to September 2005, during which there was no significant difference in RGW between lakes (p = 0.4915). In 2012, RGW varied with lake and month (p = 0.0138), with NT brook trout having significantly higher RGW (0.043) compared to ST (0.006) during peak thermal stratification in August (p = 0.0295). August 2012 NT brook trout RGW likely increased as a consequence of HO and their renewed ability to access zooplankton in the hypolimnion, especially during the day. In July 2012, NT daphnia were distributed uniformly through the metalimnion and hypolimnion during daylight hours, while ST daphnia sought refuge in the anoxic hypolimnion where DO was insufficient (B. Cross, Washington State University, unpubl. data). Increased Daphnia consumption in salmonids following HO has also been observed in Amisk Lake, Alberta (Aku and Tonn Citation1999).
Rainbow trout
Rainbow trout primarily consumed daphnids, amphipods, dipterans, and golden shiners (). In 2005, Daphnia consumption decreased through the sampling season while intake of dipterans increased. Rainbow trout in both lakes consumed amphipods in August and September 2005. In 2012, Daphnia consumption was highest during midsummer and greater in NT compared to ST. ST rainbow trout consumed significantly more amphipods than NT rainbow trout during peak stratification in August 2012 (p < 0.0001; ). During the same sampling period, NT rainbow trout consumed significantly more Daphnia than rainbow trout in ST (p = 0.0043).
Diet overlap between lakes can be evaluated by comparing SDOI in NT and ST for each sampling date. For 2005, the SDOI does indicate biologically significant diet overlap between lakes for rainbow trout and golden shiners, ranging from 66 to 75%. In 2012, the SDOI indicates decreasing diet overlap between lakes, from 90% in May to 59% in August, mainly due to higher amphipod consumption in ST. Amphipod consumption observed in 2005 and 2012 in ST for rainbow trout implies these fish are feeding in the littoral zone in August when epilimnetic temperatures exceed 21 C. Wang et al. (Citation1996) observed that rainbow trout consumed nonplanktonic littoral prey in a Minnesota lake in midsummer when access to large-bodied Daphnia decreased as a result of hypolimnetic anoxia.
Increased access to Daphnia following HO is not reflected in rainbow trout RGW. In 2005, there was no significant difference in RGW between lakes (p = 0.2198) or months (p = 0.3270). In 2012, RGW varied significantly between lakes and months (p < 0.0001), with NT in early summer and fall having highest average RGW relative to that in ST. RGW was not significantly different between lakes during peak thermal stratification in August (p = 0.4268), when greatest benefit from oxygenation would be expected.
Diet overlap between fish species
Largemouth bass showed few diet similarities with trout or golden shiner. Bass diet overlap only exceeded the 60% threshold with rainbow trout in NT in September 2012 (67%) and in ST in August 2005 (63%). Minimal diet overlap with other fish in the system is expected given that bass are primarily a littoral-feeding species and that larger bass are predominantly piscivores. HO seems to have had no effect on diet overlap between bass and other fish.
Brook and rainbow trout diet overlap averaged across months was biologically significant before (66%) and during HO (64%), an expected finding given the importance of daphnids and dipterans in diets of both species. When rainbow trout fed on golden shiners or high numbers of amphipods, there was not a biologically significant diet overlap between rainbow and brook trout.
Rainbow trout and golden shiner diets generally did not overlap significantly between lakes before or during HO (); however, golden shiners did represent a major zooplankton consumer within the Twin lakes system. In August 2012, because consumption of Daphnia by rainbow trout and golden shiners in NT was not significantly different (p = 0.2702), diet overlap between the 2 species was biologically significant at 62%. Like rainbow trout, diet overlap for golden shiner and brook trout diet was generally low () except in NT in August and September 2012 in NT. At that time, both species fed on large numbers of late season Daphnia, and diet overlap was biologically significant at 61% and 77% for brook trout and golden shiners, respectively.
Although previous studies have indicated limited potential for diet overlap and competition between trout and golden shiners in Twin Lakes, increased Daphnia consumption by golden shiners in NT during August and September 2012 suggests there may be competitive interactions during peak thermal stratification. Brook trout RGW increased in NT in August 2012, which may be related to reduction of the hypolimnetic anoxic zooplankton refuge following HO. HO may have reduced the necessity for littoral feeding by rainbow trout; however, lower DO delivery rates for 2012 did not fully oxygenate the entire hypolimnion (Part I, Moore et al. Citation2014, this issue), so that some anoxic layers may have still provided at least partial refuge for zooplankton from fish predation. Brook and rainbow trout body condition factors have not been significantly different between NT and ST since oxygenation began (B. Cross, Washington State University, unpubl. data). One explanation is that increased interspecific competition between trout and golden shiners may be masking potential benefits of expanded midsummer habitat and prey access provided by HO. Another possibility is that stocking rates for both lakes are somewhat high, leading to higher intraspecific and higher interspecific competition for food resources among the trout species.
Declines in lentic salmonid populations are commonly correlated with increases in nonsalmonid zooplanktivores, such as golden shiners (Schneidervin and Hubert Citation1987, Gipson and Hubert Citation1991, Flick and Webster Citation1992). Like lentic brook and rainbow trout, golden shiners are specialized zooplanktivores that actively size-select for large Daphnia (Hall et al. Citation1979, Elser et al. Citation1995, Muller-Solger et al. Citation1997). Increases in golden shiner populations are often associated with a sharp decline in lake-wide Daphnia densities (Muller-Solger et al. Citation1997). Although golden shiner and trout may be spatially segregated during thermal stratification, Daphnia predation by both species may occur throughout the water column.
Several studies have also noted decreased rainbow trout density, attributable to increased interspecific competition for shared Daphnia resources as golden shiner populations increased (Elser et al. Citation1995, Muller-Solger et al. Citation1997). Overall, better access to hypolimnetic zooplankton, especially during daylight hours, should favor salmonids over more surface-oriented species, such as golden shiners. Rainbow trout are more surface-oriented compared to brook trout (Behnke Citation2002), which may explain the greater response in RGW by brook trout. HO may expand lentic salmonid habitat and prey access, but HO effects on interspecific competition between salmonids and nonsalmonids should also be considered. Managers of mixed warm- and coldwater fisheries should also consider strategies to increase predation on golden shiner. For example, slot limits for bass encourage recruitment, and preservation of larger piscivorous bass that prey on golden shiners.
In summary, HO seems to have had little effect on largemouth bass, a littoral-oriented, warmwater species. HO may have increased pelagic feeding opportunities for golden shiners based on their higher Daphnia consumption in NT in 2012. Golden shiner RGW varied seasonally in both lakes and in both years, and there was no clear evidence of HO influence. Brook trout diet did not change before or during treatment, but RGW was significantly higher in NT relative to ST during August 2012, indicating a possible benefit of HO. Because Daphnia were heavily utilized as prey by both NT golden shiners and trout, diet overlap in August and September 2012 was biologically significant.
Competition between golden shiners and trout may be lessening the fishery benefits of HO, although other factors are possible. For example, oxygen delivery rates by the HO system in 2012 were insufficient to maintain a completely oxic hypolimnion (Part I, Moore et al. Citation2014, this issue), so that some hypolimnetic refuge for zooplankton from fish predation may have existed. In addition, long-term benefits of HO, especially in potentially enhancing zooplankton communities, are not likely to have been fully realized in only a few years. Continued fish diet analyses conducted in years with a completely oxic hypolimnion may be required to fully confirm HO effects on fish diets. We suggest that managers consider bioenergetics modeling to better match fish stocking rates to lake carrying capacity for preventing potential over-utilization of zooplankton resources after implementation of HO.
Acknowledgments
The authors thank Ed Shallenberger for his fisheries expertise and Brian Keleher, Allen Hammond, and Montana Pakootas for assistance with field work.
Funding
We also thank the Colville Confederated Tribes for partially funding this work and for the use of gillnets, measuring equipment, and electroshocking boat.
References
- Aku PMK, Tonn WM. 1999. Effects of hypolimnetic oxygenation on the food resources and feeding ecology of cisco in Amisk Lake, Alberta. T Am Fish Soc. 128:17–30.
- Anderson RO, Neumann RM. 1996. Length, weight, and associated structural indices. In: Murphy BR, Willis DW, editors. Fisheries techniques. 2nd ed. Bethesda (MD) : American Fisheries Society. p. 447–482.
- Behnke RJ. 2002. Trout and salmon of North America. New York (NY) : Free Press.
- Benke AC, Huryn AD, Smock LA, Wallace JB. 1999. Length–mass relationships for freshwater macroinvertebrates in North American with particular reference to the Southeastern United States. J N Am Benthol Soc. 18:308–343.
- Chipps SR, Garvey JE. 2007. Assessment of diets and feeding patterns. In: Guy CS, Brown ML, editors. Analysis and interpretation of freshwater fisheries data. Bethesda (MD) : American Fisheries Society. p. 473–514.
- Christensen DR. 2005. Prey selectivity and population dynamics of a lentic fish community, Twin Lakes, Washington . [master's thesis]. [Pullman (WA) ]: Washington State University.
- Christensen DR. 2007. Food web and habitat influences affecting the survival of salmonids in Twin Lakes, . Washington [dissertation]. [Pullman (WA) ]: Washington State University.
- Christensen DR, Moore BC. 2007. Diet composition and overlap in a mixed warm- and coldwater fish community. J Freshw Ecol 23:195–204.
- Christensen DR, Moore BC. 2008. Differential prey selectivity of largemouth bass functional feeding groups in Twin Lakes, Washington. Lake Reserv Manage. 23:39–48.
- Cooke GD, Welsh EB, Peterson SA, Nichols SA. 2005. Restoration and management of lakes and reservoirs. . 3rd ed. Boca Raton (FL) : Taylor and Francis.
- Cortes E. 1997. A critical review of methods of studying fish feeding based on analysis of stomach contents: application to elasmobranch fishes. Can J Fish Aquat Sci. 54:726–738.
- Coutant CC. 1985. Striped bass, temperature, and dissolved oxygen: A speculative hypothesis for environmental risk. T Am Fish Soc. 114:31–61.
- Dinsmore WP, Prepas EE. 1997. Impact of hypolimnetic oxygenation on profundal macroinvertebrates in a eutrophic lake in central Alberta. I. Changes in macroinvertebrate abundance and diversity. Can J Fish Aquat Sci. 54:2157–2169.
- Doke, JL, Funk WH, Juul STJ, Moore BC. 1995. Habitat availability and benthic invertebrate population changes following alum treatment and hypolimnetic oxygenation in Newman Lake, Washington. J Freshw Ecol. 10(2):87–102.
- Dumont HJ, Balvay G. 1978. The dry weight estimate of Chaoborus flavicans (Meigen) as a function of length and instars. Hydrobiologia. 64:139–145.
- Elser JJ, Luecke C, Bret MT, Goldman CR. 1995. Effects of food web compensation after manipulation of rainbow trout in an oligotrophic lake. Ecology. 76:52–69.
- Flick WA, Webster DA. 1992. Standing crops of brook trout in Adirondack waters before and after removal of non-trout species. N Am J Fish Manage. 12:783–796.
- Gipson RD, Hubert WA. 1991. Factors influencing the body condition of rainbow trout in small Wyoming reservoirs. J Freshw Ecol. 6:327–334.
- Hall DJ, Werner JF, Gilliam GG, Mittelbach D, Howard D, Doner CG, Dickerman JA, Stewart AJ. 1979. Diel foraging behavior and prey selection in the golden shiner (Notemigonus crysoleucas). Can J Fish Aquat Sci. 36:1029–1039.
- Horak DL, Tanner HA. 1964. The use of vertical gill nets in studying fish depth distribution, Horsetooth Reservoir, Colorado. T Am Fish Soc. 93:137–145.
- Hyslop EJ. 1980. Stomach contents analysis: a review of methods and their application. J Fish Biol. 17:411–429.
- Johnston TA, Cunjak RA. 1999. Dry mass–length relationships for benthic insects: A review with new data from Catamaran Brook, New Brunswick, Canada. Freshwater Biol. 41:653–674.
- Kamler JF, Pope KL. 2001. Nonlethal methods of examining fish stomach contents. Rev Fish Sci. 9:1–11.
- Lawrence SG, Malley DF, Findlay WJ, MacIver MA, Delbaere IL. 1987. Method for estimating dry weight of freshwater planktonic crustaceans from measures of length and shape. Can J Fish Aquat Sci. 44:264–274.
- Liao H, Pierce CL, Larscheid JG. 2001. Empirical assessment of indices of prey importance in diets of predacious fish. T Am Fish Soc. 130:583–591.
- Merritt RW, Cummins KW. 1996. An introduction to the aquatic insects of North America. . 3rd ed. Dubuque (IA) : Kendall-Hunt.
- Moore BC, Cross BK, Clegg EM, Lanouette BP, Skinner M, Preece E, Child A, Gantzer P, Shallenberger E, Christensen D, Nine B. 2014. Hypolimnetic oxygenation in Twin Lakes, WA. Part I: Distribution and movement of trout. Lake Reserv Manage 30:226–239.
- Morin A, Dumont P. 1994. A simple model to estimate growth rate of lotic insect larvae and its value for estimating population and community production. J N Am Benthol Soc. 13:357–367.
- Muller-Solger A, Brett MT, Luecke C, Elser JJ, Goldman CR. 1997. The effects of planktivorous fish (golden shiners) on the ciliate community of a mesotrophic lake. J Plankton Res. 19:1815–1828.
- Overholtz WJ, Fast AW, Tubb RA, Miller R. 1977. Hypolimnion oxygenation and its effects on the depth distribution of rainbow trout (Salmo gairdneri) and gizzard shad (Dorosoma cepedoanum). T Am Fish Soc. 106:371–375.
- Pelham ME, Pierce CL, Larscheid JG. 2001. Diet dynamics of the juvenile piscivorous fish community in Spirit Lake, Iowa, USA, 1997–1998. Ecol Freshw Fish. 10:198–211.
- SAS Institute. 2012. SAS 9.3. Cary [NC] : SAS Institute Inc.
- Schneidervin RW, Hubert WA. 1987. Diet overlap among zooplanktophagous fishes in Flaming Gorge Reservoir, Wyoming–Utah. N Am J Fish Manage. 7:379–385.
- Schoener TW. 1970. Nonsynchronous spatial overlap of lizards in patchy habitats. Ecology. 51:408–418.
- Singleton, VL, Gantzer P, Little JC. 2007. Linear bubble plume model for hypolimnetic oxygenation: full-scale validation and sensitivity analysis. Water Resour Res. 43:1–12.
- Smock LA. 1980. Relationships between body size and biomass of aquatic insects. Freshwater Biol. 10:375–383.
- Spoor WA. 1999. Distribution of fingerling brook trout, Salvelinus fontinalis (Mitchill), in dissolved oxygen concentration gradients. J Fish Biol. 36:363–373.
- Swales S. 2006. A review of factors affecting the distribution and abundance of rainbow trout (Oncorhychus mykiss Walbaum) in lake and reservoir systems. Lake Reserv Manage. 22:167–178.
- Taggart CT. 1984. Hypolimnetic aeration and zooplankton distribution: a possible limitation to the restoration of cold-water fish populations. Can J Fish Aquat Sci. 41:191–198.
- Wallace RK. 1981. An assessment of diet-overlap indexes. T Am Fish Soc. 110:72–76.
- Wang L, Zimmer K, Diedrich P, Williams S. 1996. The two-story rainbow trout fishery and its effect on the zooplankton community in a Minnesota lake. J Freshw Ecol. 11:67–80.
- Watkins J, Rudstam L, Holeck K. 2011. Length-weight regressions for zooplankton biomass calculations: A review and a suggestion for standard equations. Bridgeport (NY) : Cornell Biological Field Station Publications and Reports . [cited 14 April 2014]. Available from http://hdl.handle.net/1813/24566
- Wydoski RS, Whitney RR. 2003. Inland fishes of Washington. Singapore: University of Washington Press.