Abstract
Fish habitats in many aging US reservoirs have become degraded and require broad-scale assessment to rate their status and facilitate rehabilitation efforts. To help prioritize habitat projects in reservoirs, we assembled a rating system for large reservoirs in the contiguous United States. Using responses to an online questionnaire about fish habitat impairment in 1299 large US reservoirs, we applied multivariate analyses to identify combinations of habitat impairment descriptors that quantified broad impairment types (i.e., a construct). Resulting constructs reflected point source pollution, nonpoint source pollution, excessive nutrients, algae blooms, siltation, limited nutrients, mudflats and shallowness, limited connectivity to adjacent habitats, limited littoral structure, nuisance species, anomalous water regimes, and large water level fluctuations. Scores were summed across constructs to create a composite number that rated overall reservoir habitat impairment. Construct and composite scores differed among geographic ecoregions of the United States. This rating system could provide a starting point for prioritizing reservoirs for habitat rehabilitation and enhancement projects.
Degradation of fish habitat has long been a concern of many reservoir ecologists, but the issue is quickly coming to the forefront as reservoirs age (Miranda and Krogman Citation2015, Pegg et al. Citation2015). Most US reservoirs were built in the 20th century, and as of 2014 their median age was 57 years (USACE Citation2009). Reservoirs are impounded rivers and receive more allochthonous inputs from incoming tributaries than do lakes, thereby experiencing ecological succession at an accelerated rate. Sediments and nutrients entering reservoirs slow and settle, accumulating in the benthic zone and entering the food web through photosynthetic and bacterial uptake (Thornton Citation1990). Fish habitat issues such as excessive sediments and nutrient loadings, degradation of submerged structure, and erosion of shorelines not adapted to continuous immersion may emerge in an aging reservoir and worsen over time (Agostinho et al. Citation1999).
State and federal agencies are expanding habitat activities and calling for implementation of nationwide programs to improve aquatic habitats (AFWA Citation2012). Many fish management agencies are expanding their reservoir habitat management programs with additional personnel that focus exclusively on habitat enhancement and rehabilitation, although the approaches differ among agencies depending on how local conditions influence aspects of reservoir habitat. These advances have come about because of recognition that aquatic problems in reservoirs are being largely driven by relatively rapid environmental changes and the realization that biotic manipulations and harvest management alone cannot sustain fish communities at desired levels (Miranda Citation2008).
Assessment systems for lakes and reservoirs generally have focused on in-reservoir water quality parameters indicative of trophic state (e.g., Vollenweider Citation1968, Schindler Citation1971, Carlson Citation1977, Ground and Groeger Citation1994, Burns et al. Citation1999). More recently, Kaufmann et al. (2014) outlined a rapid approach for quantifying aquatic habitats (i.e., littoral habitat complexity, fish cover, substrate, aquatic macrophytes, riparian vegetation, and human disturbances) in large-scale assessments, but this methodology was limited to the lakeshore. Habitat monitoring, management, and restoration are hindered by a scarcity of comprehensive quantitative indicators to track habitat condition and provide managerially or scientifically useful information. Quantitative indicators of reservoir fish habitat status, or reservoir ratings, could be used to grade habitat in reservoirs and measure progress toward goals. Managers could use the reservoir ratings to set priorities, evaluate strategies, and possibly provide a systematic, integrated approach to reporting performance of rehabilitation efforts. Moreover, by quantifying concepts about habitat condition, ratings may facilitate research into the causes of habitat impairment.
In view of this need, our objective was to rate US reservoirs relative to fish habitat condition. To score reservoir habitats, we applied a quantitative index similar to that developed by Miranda and Hunt (Citation2010), although we expanded the index to account for additional habitat impairment factors. We used the scores to explore how habitat impairment varies among and within geographic ecoregions of the United States.
Methods
Large reservoirs within the contiguous United States were defined by the Reservoir Fisheries Habitat Partnership (RFHP) as any river impoundment equaling or exceeding 100 ha in surface area (http://www.reservoirpartnership.org). Using this simple definition, our initial sampling frame included > 4300 water bodies ≥ 100 ha documented in the National Inventory of Dams (USACE Citation2009).
Data collection
We developed an online survey that included 52 questions about habitat impairment, including aspects of habitat availability, water quality, water regime, and degradation processes (). Questions were expanded from a previous survey (Miranda and Hunt Citation2010) based on extensive literature review. A 6-point Likert-type scale was used to collect responses with ratings from 0 to 5: 0 = no impairment, 1 = low impairment, 2 = low-to-moderate impairment, 3 = moderate impairment, 4 = moderate-to-high impairment, and 5 = high impairment. Respondents were instructed to rate impairment based on the reservoir's state during the past 5 years and relative to similar reservoirs within their geographic region. The link for the online survey was made available through state natural resource agencies to all biologists responsible for managing fish in reservoirs. After an introduction outlining the survey's purpose, as well as the voluntary and confidential nature of responses, respondents were asked to complete the survey for reservoirs under their jurisdiction. The survey was conducted via the host SurveyMonkey (http://www.surveymonkey.com) between June and December 2010, including a follow-up period when nonrespondents were contacted multiple times to encourage participation.
Table 1 Variables representing diverse sources of fish habitat impairment in large US reservoirs.
Table NaN Variables representing diverse sources of fish habitat impairment in large US reservoirs. (Continued)
This survey depended on the perception of professionals, but perceptions may vary geographically depending on exposure. The extent of this effect depends on the extent of the geographic area. To promote equivalence of responses among participants in our study, each question was coupled with an expanded narrative to help focus the respondent. Moreover, respondents were instructed to exclude little-known reservoirs, including privately owned and small reservoirs that were not frequently monitored.
Rating system
All survey responses were examined for completeness and duplication (i.e., one entry per reservoir), and incomplete (i.e., >30 % item nonresponse; Schafer Citation1997) or duplicated cases were removed from analyses. Remaining missing values were estimated using multiple imputation (MI procedure; SAS Citation2009).
We hypothesized that the responses to the 52 questions (i.e., observed variables) were intercorrelated and measured multiple facets (i.e., latent constructs) of habitat impairment. To identify the latent constructs, we submitted the 52 observed variables to oblique coordinate analysis (OCA), a clustering procedure similar to principal coordinate analysis but not requiring that axes be orthogonal (Harman Citation1976). In an ordinary principal coordinate analysis all components are computed with the same set of variables, although in each component each variable is given a different weight, and components are orthogonal (i.e., uncorrelated) relative to each other. In OCA, each cluster component is computed with a different and separate set of variables, and components are oblique (i.e., correlated) relative to each other. We chose an oblique approach because habitat impairments are often interrelated and co-occurring, and therefore statistically dependent. Additionally, OCA typically yields more interpretable scales, an important part of our objective. The OCA was applied with the VARCLUS algorithm (SAS Citation2009), beginning with all variables in a single cluster component and iteratively splitting components until no additional components with eigenvalue > 0.9 could be defined. The OCA was applied to a distance matrix created using Gower's similarity coefficient (appropriate for ordinal scale data; Gower Citation1971) on the 52 observed variables, yielding a number of latent impairment constructs. Reliability of latent constructs was assessed using Cronbach's alpha coefficient for internal consistency (Cronbach Citation1951), and observed variables reducing a constructs’ internal consistency were removed.
We rated reservoirs with a composite index similar to that described by Miranda and Hunt (Citation2010). Ratings (i.e., 0–5 Likert score) for each observed variable included in each construct were averaged and rounded to the nearest integer, yielding a range of possible construct scores from 0 to 5. Construct scores were then added to compute an overall composite rating score as:
where f′m = score of construct m of the n constructs that make up the composite rating; fm = average of the j variables that make up the mth construct; and vi = score of variable i of the j variables that make up fm.
Resulting impairment construct scores (f′m) were summarized nationwide and by reservoir habitat ecoregions (Krogman and Miranda Citation2015). Ecoregions included Xeric (XER), Western Mountains (WMT), Northern Plains (NPL), Temperate Plains (TPL), Southern Plains (SPL), Upper Midwest (UMW), Coastal Plains (CPL), Southern Appalachian (SAP), and Northern Appalachian (NAP). Ecoregions were originally established by the US Environmental Protection Agency (USEPA) and are an aggregation of Omernik's Level III ecoregions, often but not necessarily contiguous to one another (USEPA Citation2006). We summarized impairment constructs for each ecoregion by reporting the proportion of reservoirs scoring high (i.e., 4 or 5) on each construct and summarizing composite ratings as regional medians.
Results
We received 1599 responses, including partially completed surveys and duplicate entries; 7 states did not participate and were primarily in the far northern United States where natural lakes predominate. Of the responses received, 1299 matched our study scope (i.e., surface area ≥100 ha and not a natural lake fitted with a water control structure) and were complete enough for analysis. Most surveys had low item nonresponse, with 78% containing no missing items and 99% containing <10% missing items. Considering that the National Inventory of Dams identifies 4300 regulated waterbodies ≥100 ha, our sample represented at least 30% of reservoirs ≥100 ha distributed throughout the contiguous United States. Reservoirs ranged in surface area from 100 to 156,000 ha, in mean depth from 0.3 to 181.2 m, and in age from 10 to 178 years.
Impairment constructs
Oblique component analysis of the 52 observed variables identified 12 constructs that explained 61.4% of the variation in responses (). Further analysis of each construct with Cronbach's alpha suggested that 3 of the 52 observed variables, including insufficient bank shading, oxygen stratification, and residual effects of upstream impoundments, reduced the reliability of some constructs. These variables were removed not because they are unimportant, but because they showed low correlation with the retained variables. The resulting constructs had alpha coefficients ranging from 0.57 to 0.90, with two-thirds of constructs having an alpha >0.80 (i.e., good internal consistency; ). Only the limited nutrients construct had an alpha coefficient <0.60 (i.e., questionable/poor internal consistency; Cronbach Citation1951), but the construct was retained because it represented a unique impairment that may have been better measured by variables not included in the original survey. Each construct was assigned a name carefully selected to describe its composing and/or top-loading variables, and then a construct definition was developed to provide the reader with greater context of the construct interpretation.
Table 2 Latent constructs representing major reservoir habitat impairments. Each latent construct comprises several observed variables coded numerically and defined in Table 1. The internal consistency of the construct is reflected by Cronbach's alpha (α) coefficient.
The siltation construct had the greatest proportion of highly impaired reservoirs (i.e., with construct scores of 4 or 5), followed by excessive nutrients, mudflats/shallowness, and large water fluctuations constructs (). The point source pollution construct had the fewest highly impaired reservoirs, followed by nuisance species and limited nutrients constructs. The proportion of reservoirs scoring high on individual impairment constructs differed by ecoregion (). The large water fluctuations construct had the greatest proportion of highly impaired reservoirs in NAP, NPL, WMT, XER, and SPL. The mudflats/shallowness construct had the greatest number of highly impaired reservoirs in CPL but was a common impairment across the United States. The excessive nutrients construct had the greatest number of highly impaired reservoirs in TPL and UMW, whereas siltation had the greatest number of highly impaired reservoirs in SAP, SPL (tie with large water fluctuations construct), and CPL (tie with mudflats/shallowness construct). The point source pollution construct was influential only in the XER ecoregion. Generally, each ecoregion was defined by a unique set of major impairments ().
Ratings
The ratings ranged from 0 to 46 with a median score of 18 out of a maximum possible score of 60. The distribution of ratings was approximately normal with 10th and 90th percentiles of 9 and 30, respectively (). Median ratings by ecoregion were 17 in CPL, 17 in NAP, 20.5 in NPL, 17 in SAP, 17 in SPL, 24 in TPL, 17 in UMW, 16 in WMT, and 22 in XER. Nevertheless, there was extensive variability in ratings within regions and nationally, with a wide range of ratings occurring in each ecoregion (). Saylorville Lake, a flood control reservoir in central Iowa, had the highest impairment rating nationwide (i.e., 46), scoring particularly high on the nonpoint source pollution, excessive nutrients, siltation, and anomalous water regime constructs. Four reservoirs shared the lowest possible impairment rating (i.e., 0): Big Lake, Arizona; Marlette Lake, Nevada; Hog Park Reservoir, Wyoming; and Prairie Lake, Illinois.
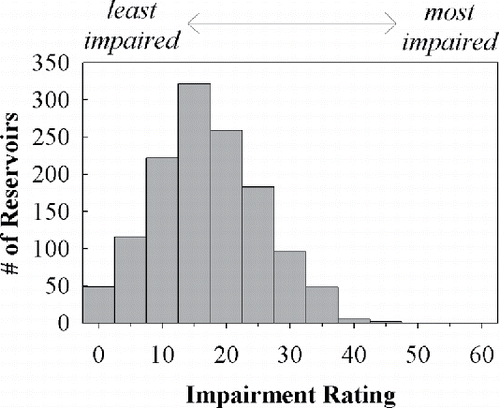
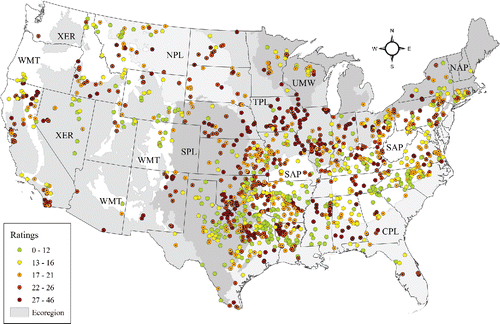
Discussion
The composite reservoir habitat ratings provide a measure of the overall habitat impairment status of a reservoir and can be used for rapid assessment and for comparing reservoirs. The rating system provides a method to quickly identify high-quality reservoirs for protection and degraded reservoirs for rehabilitation. Distinction between high-quality and degraded reservoirs can be relatively unambiguous; reservoirs with low rating scores generally have low scores on most of the constructs, whereas reservoirs with high rating scores have high scores on many constructs. Conversely, interpretation of ratings in the intermediate range is more ambiguous. Potentially, reservoirs with similar ratings can be impaired by different constructs, requiring scrutiny of construct scores to sort out the major sources of impairment. Comparison of rating scores among reservoirs may allow prioritization of restoration activities. Reservoirs with high scores may require immediate management attention, whereas those with low scores should have priority for conservation measures. Reservoirs with intermediate scores may require attention on a narrow range of high magnitude impairments or a broad range of low magnitude impairments.
The ratings captured a variety of fish habitat impairments in reservoirs nationwide, with relative importance of specific impairment components changing spatially across wide geographic areas. Ecoregions did not define reservoir condition, however, because the full range of impairment scores appeared in every ecoregion nationwide. Impairments such as sedimentation and nonpoint source pollution were widespread, affecting all or nearly all ecoregions to some degree, and were often associated with inputs from upstream watersheds. Impairments such as point source pollution and limited nutrients were identified by respondents as impairments in relatively few reservoirs.
Our analyses suggested that impairment due to large water level fluctuation was most common in the dryer areas of the contiguous United States, including the West (ecoregions WMT and XER) and Great Plains (SPL and NPL). Water is scarcer in these areas and is typically collected for irrigation (unpublished data from survey); water levels may fluctuate widely as incoming water is stored during the rainy season and released throughout the growing season. The water storage and allocation required to optimize water availability for irrigation can often conflict with the needs of fish in a reservoir by altering environmental cues or seasonal habitat availability (Ploskey Citation1986, Bunn and Arthington Citation2002, Dagel and Miranda Citation2012). Our analyses further indicated that large water level fluctuation was also the most important impairment in the Northeast; however, the extent of this impairment was relatively lower than in other regions.
Unlike the West, most habitat impairments in the Midwest and South emphasized constructs reflective of incoming water quality and land management in the reservoir's watershed rather than water storage. A reservoir's watershed is often the primary source of inputs into the reservoir, including nutrients, sediments, chemicals, and other pollutants (Kimmel and Groeger Citation1986, Kennedy and Walker Citation1990, Thornton Citation1990). Excessive nutrient inputs was the most important impairment in the Midwest, followed by siltation and nonpoint source pollution. Runoff from agricultural land contributes to all of these impairments; farm land covers >74% of Iowa (ISU 2013) and 60% of Illinois (IDNR Citation2013). In the South, siltation and mudflats/shallowness were the most important impairments, whereas excessive nutrient input was less important. Interestingly, this coincides with less land coverage by traditional agricultural land and greater land coverage by timber land. In the Southeastern states of Florida, Georgia, North Carolina, South Carolina and Virginia, 59% of the land was forested in 2007, with 97% of that considered timber land (Smith et al. Citation2009). In addition, the same region had 12% agricultural land (USDA Citation2009). In the South Central states of Alabama, Arkansas, Kentucky, Louisiana, Mississippi, Oklahoma, Tennessee, and Texas, 33% of the land was forested, with 93% of that considered timber land; the same region had 21% agricultural land (USDA Citation2009). Commercial forestry practices such as roadbuilding and clear-cutting during harvest could export additional sediment and, to a lesser degree, nutrients to waterways directly (Ensign and Mallin Citation2001) or indirectly by altering streamflow (Troendle and Olsen Citation1994). Thus, reservoirs in the Midwest and South show faster rates of sedimentation and eutrophication than in other regions and hence faster functional aging as defined by Miranda and Krogman (Citation2015). The close ties among land use, eutrophication, and functional age were effectively demonstrated for Kansas reservoirs by Carney (Citation2009).
The rating system and its components reflected a wide range of fish habitat issues that transcend those that can be readily measured during onsite quantitative surveys. Many of the habitat descriptors included in our survey measured constructs not typically quantified during onsite surveys, providing new perspective on reservoir fish habitat. Elements such as sediment and nutrient loading, resultant habitat diversity loss, loss of connectivity to adjacent habitats such as backwaters, water storage patterns, algal blooms, and nuisance species may not be captured by most time-limited onsite water quality and quantitative habitat surveys. Our rating system tapped into observational experience accumulated by field biologists. Whereas limitations are associated with relying on this type of knowledge, subjectively scored habitat indexes have been confirmed to show strong correlations among themselves and low-to-moderate correlations with biotic index scores (Hughes et al. Citation2010). A comparison of the Kaufmann et al. (Citation2014) quantitative survey of nearshore habitats with scores of our siltation, mudflats/ shallowness, and limited littoral structure constructs could help clarify the value of these two assessment tools. Further improvement in assessment accuracy may be obtained by upgrading to objective onsite quantitative habitat surveys but at a substantial rise in cost and perhaps without matching increases in evaluation accuracy.
Regional differences in impairment sources contributing to the ratings can inform more effective allocation of funding for habitat renovations. For example, a nonprofit organization like the Reservoir Fisheries Habitat Partnership, which provides funding for reservoir fish habitat improvement in reservoirs across the United States, may use the ratings to identify the least and most impaired reservoirs nationwide and to develop ecoregion-specific priority impairments. The latter may be a more conservative approach if impairment valuation scores differ among regions due to geographical shifts in professional perceptions, as alluded to earlier. A habitat improvement project that addresses priority impairments specific to the ecoregion (e.g., a project in the TPL ecoregion that addresses siltation and excessive nutrients through wetland construction) may be allocated funding over projects that do not address priority impairments (e.g., a project in the TPL ecoregion that installs brush piles and foregoes watershed considerations). The rating system as a whole provides a national snapshot of fish habitat in large reservoirs, enabling objective comparison of a wide variety of reservoirs for decision-making about national, regional, and local habitat management strategies.
Supplementary Materials
Supplemental data for this article can be accessed on the http://www.dx.doi.org/10.1080/10402381.2015.1121307.
Supplemental_ScoresbyReservoir.xlsx
Download MS Excel (217.7 KB)Acknowledgments
We thank Jeff Boxrucker of the Reservoir Fisheries Habitat Partnership for helpful assistance at various stages of this research, as well as the many fishery managers nationwide who provided data for this study. Ken Wagner and two anonymous reviewers provided helpful reviews that improved this manuscript.
Funding
Funding for this research was provided by the US Fish and Wildlife Service through the Reservoir Fisheries Habitat Partnership. Any use of trade, firm, or product names is for descriptive purposes only and does not imply endorsement by the US Government.
References
- Agostinho AA, Miranda LE, Bini LM, Gomes LC, Thomaz SM, Susuki HI. 1999. Patterns of colonization in neotropical reservoirs, and prognoses on aging. In: Tundisi JG, Straškraba M, editors. Theoretical reservoir ecology and its applications. Leiden (The Netherlands): Backhuys Publishers. p. 227–265.
- [AFWA] Association of Fish and Wildlife Agencies. 2012. National fish habitat action plan, 2nd ed. Washington (DC). 40 p.
- Bunn SE, Arthington AH. 2002. Basic principles and ecological consequences of altered flow regimes for aquatic biodiversity. Environ Manage. 30:492–507.
- Burns NM, Rutherford JC, Clayton JS. 1999. A monitoring and classification system for New Zealand lakes and reservoirs. Lake Reserv Manage. 15:255–271.
- Carlson RE. 1977. A trophic state index for lakes. Limnol Oceanogr. 22:361–369.
- Carney E. 2009. Relative influence of lake age and watershed land use on trophic state and water quality of artificial lakes in Kansas. Lake Reserv Manage. 25:199–207.
- Cronbach LJ. 1951. Coefficient alpha and the internal structure of tests. Psychometrika. 16:297–334.
- Dagel JD, Miranda LE. 2012. Backwaters in the upper reaches of reservoirs produce high densities of age-0 crappies. N Am J Fish Manage. 32:626–634.
- Ensign SH, Mallin MA. 2001. Stream water quality changes following timber harvest in a coastal plain swamp forest. Water Res. 35:3381–3390.
- Ground TA, Groeger AW. 1994. Chemical classification and trophic characteristics of Texas reservoirs. Lake Reserv Manage. 10:189–201.
- Gower JC. 1971. A general coefficient of similarity and some of its properties. Biometrics. 27:857–871.
- Harman HH. 1976. Modern factor analysis, 3rd ed. Chicago (IL): University of Chicago Press.
- Hughes RM, Herlihy AT, Kaufmann PR. 2010. An evaluation of qualitative indexes of physical habitat applied to agricultural streams in ten U.S. states. J Am Water Resour As. 46:792–806.
- [IDNR] Illinois Department of Natural Resources. 2013. Land cover database by categories: cropland; [cited 22 Aug 2013]. Available from: http://dnr.state.il.us/orep/ctap/map/category.htm
- [ISU] Iowa State University. 2013. Crop and land use: statewide data; [cited 22 Aug 2013]. Available from: http://www.extension.iastate.edu/soils/crop-and-land-use-statewide-data
- Kaufmann PR, Peck DV, Paulsen SG, Seeliger CW, Hughes RM, Whittier TR, Kamman NC. 2014. Lakeshore and littoral physical habitat structure in a national lakes assessment. Lake Reserv Manage. 30:191–215.
- Kennedy RH, Walker WW. 1990. Reservoir nutrient dynamics. In: Thornton KW, Kimmel BL, Payne FE, editors. Reservoir limnology: ecological perspectives. New York (NY): Wiley Interscience. p. 109–132.
- Kimmel BL, Groeger AW. 1986. Limnological and ecological changes associated with reservoir aging. In: Hall GE, Van Den Avyle MJ, editors. Reservoir fisheries management: strategies for the 80s. Bethesda (MD): American Fisheries Society. p. 103–109.
- Krogman RM, Miranda LE. 2015. A classification system for large reservoirs of the contiguous United States. Environ Monit Assess 184:174. doi:10.1007/s10661-014-4244-1
- Miranda LE. 2008. Extending the scale of reservoir management. In: Allen MS, Sammons S, Maceina MJ, editors. Balancing fisheries management and water uses for impounded river systems, Symposium 62. Bethesda (MD): American Fisheries Society. p. 75–102.
- Miranda LE, Hunt KM. 2010. An index of reservoir habitat impairment. Environ Monit Assess. 172:225–234.
- Miranda LE, Krogman RM. 2015. Functional age as an indicator of reservoir senescence. Fisheries. 40:170–176.
- Pegg MA, Pope KL, Powell LA, Turek KC, Spurgeon JJ, Stewart NT, Hogberg NP, Porath MT. 2015. Reservoir rehabilitations: seeking the fountain of youth. Fisheries. 40:177–181.
- Ploskey GR. 1986. Effects of water-level changes on reservoir ecosystems, with implications for fisheries management. In: Hall GE, Van Den Avyle MJ, editors. Reservoir fisheries management: strategies for the 80s. Bethesda (MD): American Fisheries Society. p. 86–97.
- SAS. 2009. SAS/STAT(R) 9.2 User's guide, 2nd ed; [cited 22 Aug 2013]. Available from: http://support.sas.com/documentation/cdl/en/statug/63033/HTML/default/viewer.htm#titlepage.htm
- Schafer JL. 1997. Analysis of incomplete multivariate data. London (UK): Chapman & Hall.
- Schindler DW. 1971. A hypothesis to explain the differences and similarities among lakes in experimental lakes area, northwestern Ontario. J Fish Res Board Can. 28:295–301.
- Smith WB, Miles PD, Perry CH, Pugh SA. 2009. Forest resources of the United States, 2007. Washington (DC): US Department of Agriculture, Forest Service, Washington Office, General technical report WO-78. 336 p.
- Thornton KW. 1990. Sedimentary processes. In: Thornton KW, Kimmel BL, Payne FE, editors. Reservoir limnology: ecological perspectives. New York (NY): Wiley Interscience. p. 43–70.
- Troendle CA, Olsen WK. 1994. Potential effects of timber harvest and water management on streamflow dynamics and sediment transport. Sustainable ecological systems: implementing an ecological approach to land management. Fort Collins (CO): USDA, Forest Service. General technical report RM-247. p. 34–41.
- [USACE] US Army Corps of Engineers. 2009. National Inventory of Dams (NID); [cited 1 Dec 2010]. Available from: http://geo.usace.army.mil/pgis/f?p=397:1:3514628094309333
- [USDA] US Department of Agriculture, National Agricultural Statistics Service. 2009. 2007 Census of Agriculture. Vol 32: Part 51, Chapter 1, United States summary and state data. Washington (DC): . AC/07/A-51.
- [USEPA] US Environmental Protection Agency. 2006. Wadeable streams assessment: a collaborative survey of the nation's streams. Washington (DC): EPA/841/B-06/002.
- Vollenweider RA. 1968. Scientific fundamentals of the eutrophication of lakes and flowing waters, with particular reference to nitrogen and phosphorus as factors in eutrophication. Paris (France): Organisation for Economic Co-operation and Development, Directorate for Scientific Affairs Technical Report DAS/SCI/68.27.
- Wetzel RG. 1990. Reservoir ecosystems: conclusions and speculations. In: Thornton KW, Kimmel BL, Payne FE, editors. Reservoir limnology: ecological perspectives. New York (NY): Wiley Interscience. p. 227–238.