ABSTRACT
Welch EB, Gibbons HL, Brattebo SK, Corson-Rikert HA. 2017. Distribution of aluminum and phosphorus fractions following alum treatments in a large shallow lake. Lake Reserve Manage. 33:198–204.
Alum was added to the middle 40% of 5200 ha Grand Lake St. Marys (mean depth 1.6 m) in western Ohio during 2 successive years. About <8% to 2% of the added aluminum (Al) remained in the water column immediately after treatment, and aluminum-phosphorus (Al-P) was effectively formed in the targeted treated sediment. Sediment Al and Al-P were significantly higher inside than outside the treated area with an Al-added to Al-P–formed ratio of 51, double the initial effectiveness expected (102) from the 2 year Al dose of 95 g/m2. Sediment total P was also higher inside than outside the treatment area. The source of P that formed Al-P was more likely undetermined soluble reactive P diffused from deeper sediment and to a lesser extent external P loading. Mobile iron-P (Fe-P), usually the principal mobile fraction, was relatively low with no noticeable depletion (inside-outside), and loosely sorbed P was undetectable. Biogenic P (living algae and detritus) was also relatively low and showed no noticeable depletion. Some of the greater variability in sediment Al outside than inside the treated area may have been caused by resuspension and redistribution of the alum floc. Two of the 3 outside sites on the lee side of the treated area had higher sediment Al, whereas 2 of 3 outside sites on the windward side had lower Al. Nevertheless, the alum floc remained largely in the treated area for at least 3 months to >1 year, forming sediment Al-P.
The effectiveness of alum treatments in shallow Washington lakes has been well documented (Welch and Cooke Citation1999, Cooke et al. Citation2005). An analysis of 114 alum-treated lakes, of which 32% had mean depths ≤4 m, showed that treatment effectiveness lasted 11 years on average (Huser et al. Citation2016a). Sediment cores taken in 1998 in the Washington lakes, several years after their treatments, revealed clear markers of aluminum (Al) and newly formed Al-phosphorus (Al-P) at about 6 cm depth in 6 of 7 lakes (Rydin et al. Citation2000). The exception was Green Lake, which had a high density of milfoil and carp and an uneven distribution of alum floc during application because of equipment limitations and high winds. A second treatment in spring 2004, before milfoil was abundant, was more completely distributed. Cores taken shortly after treatment and in 2015 showed that formed Al-P had proceeded to settle (mix) through 30 cm of sediment column. None of these lakes are large (average ∼100 ha) or have a long wind fetch. Hence, there was still doubt about the permanence of an alum floc in a large shallow lake with long wind fetch in which the alum floc may resuspend and redistribute, leaving parts of the sediment surface untreated.
Grand Lake St. Mary's (GLSM) in western Ohio is large (5200 ha) and shallow (1.6 m mean depth) with a long wind fetch, 16 km east–west (). Large blooms of Planktothrix with summer chlorophyll and total phosphorus (TP) averaging >100 μg/L characterized this hypereutrophic lake. External TP loading was estimated at 38.4 × 103, 36.3 × 103, and 131.6 × 103 kg/yr for 2009, 2010, and 2011, respectively (Filbrun et al. Citation2013), values that represent 0.73, 0.70, and 2.53 g/m2 per year. This lake was as enriched as the top 10% of previously alum-treated lakes evaluated by Huser et al. (Citation2016a); therefore, GLSM represents an extreme test for the effectiveness of alum to inactivate sediment P within a specific target area. The central 40% of GLSM's area, with a mean depth of 2.2 m, was treated with alum during spring, 8–30 June 2011 (21.5 mg/L Al) and 12–30 April 2012 (23.6 mg/L Al), totaling 94.8 g/m2 Al.
Figure 1. Water column sampling and alum sediment core sites in GLSM on, respectively, 25 April and 9 August 2012.
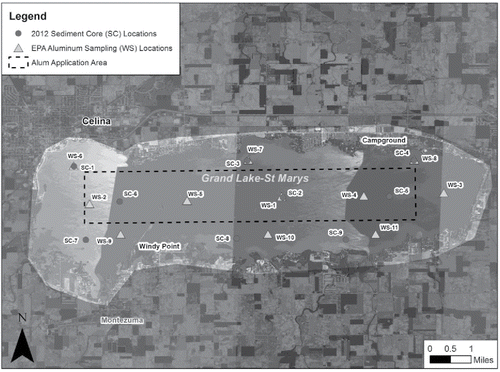
Internal loading is usually the principal source of P for algal biomass during summer in shallow lakes (Welch and Jacoby Citation2001). That source in GLSM was large, accounting for 91% of the total (internal and external) P load during summer 2010 before treatment, as observed by simple mass balance and calibrated to a non-steady state model (Perkins et al. Citation1997, Tetra Tech, Inc. Citation2013). There is no estimate of annual loading in 2010, but the gross internal load, calculated by mass balance after treatment, was 31% in 2011 and 45% in 2012 (Tetra Tech, Inc. Citation2013). Although the calculated summer sediment-to-water flux was only 4 mg/m2 per day before treatment, the lake's shallow depth accentuated its effect on TP water column concentration. Favorable results of the treatment were considered a low probability given the magnitude of external and internal loading, the presence of a large biomass of buoyant cyanobacteria during spring of both 2011 and 2012, and the limited area treated (due to limitation of funds). Presence of a large biomass of buoyant cyanobacteria can pose a problem for floc settlement, as observed in near shore enclosures experiments in 2010; thus, there was a need to determine the extent to which the alum floc remained in the treated area. The relatively positive observed results were unexpected. The calculated whole-lake summer internal flux of TP was reduced 55% following the 2 alum applications, even though 60% of the lake surface was untreated (Tetra Tech, Inc. Citation2013). Sediment cores and water samples taken inside and outside the treated area in 2012 show that some movement to outside the treated area probably occurred. Most of the Al added, however, could be accounted for in the treated area sediment, and only a relatively small fraction remained suspended in the water column, even under windy conditions with a long wind fetch.
Methods
Water samples were collected 25 April 2012, toward the end of treatment, at 4 sites inside and 7 sites outside the treated area (). Outside sites were ∼ 400–700 m from the treated areas, intended to represent ambient lake conditions. Depths sampled at each site were 0.5 m and near bottom, ranging from 1.1 to 1.7 m. Aluminum was analyzed on filtered and unfiltered samples by the Ohio Environmental Protection Agency according to standard methods (Eaton et al. Citation2005).
Sediment cores were collected 3 months following treatment on 9 August 2012 inside (n = 3) and outside (n = 6) the treated area to depths of 15 to 20 cm. The 3 inside cores were SC-2, SC-5, and SC-6, and the outside cores were SC-1, SC-3, SC-4, SC-7, SC-8, and SC-9 (). The cores were sectioned at 0–2, 2–4, 4–6, 6–10, 10–15, and, in 6 cores, 15–20 cm, including the 3 inside. Each section was analyzed for percent water, Al, and iron (Fe) according to standard methods (Eaton et al. Citation2005). Total P and P fractions Al-P, Ca-P, Fe-P, and biogenic P, the mobile fraction of organic P, were analyzed according to the scheme of Psenner et al. (Citation1988), Psenner and Puckso (Citation1988), and Rydin and Welch (Citation1998, Citation1999). All analyses were performed by Aquatic Research Inc., Seattle, Washington. Student t-tests were used to compare inside and outside means for Al in water and Al and Al-P in sediment.
Results
Water Al
During the applications, the alum floc was observed to settle in minutes, leaving a relatively clear water column; however, water Al concentration, determined near the end of the second treatment, averaged slightly higher inside than outside the treated area (). There was little difference (mean 0.07 mg/L, range 0–0.34) in total Al (TAl) between surface and bottom. Most of the TAl was in dissolved form with a larger fraction inside (81%) than outside (63%). The difference in mean TAl between inside and outside was ∼0.4 mg/L (2.08–1.69 mg/L), <2% of the Al dose (23.6 mg/L) added in 2012, although the means were not significantly different (P = 0.062) owing to the large inside variability. If the outside sites represented background ambient conditions at ∼400–700 M from the treated area, then that difference between inside and outside TAl (<2%) would indicate that nearly all the Al added was in the sediment. Maximum wind speeds of 13–32 km/h (8–20 mph), however, may well have intermixed treated water with ambient lakewater. In that case, residual TAl remaining in the water was probably near 8% of the second dose (overall mean TAl 1.85/23.6), although that would assume the background ambient level was zero. The actual pretreatment background is unknown.
Table 1. Mean total and dissolved Al concentrations (mg/L ± SD) and range at surface and bottom, inside and outside a 4000 ha mid-lake area treated with alum at 23.6 mg/L Al during 2–30 April 2012. Water samples for Al were collected on 25 April.
Sediment
Sediment Al content was rather uniform, especially in the top 6 cm with <5% variation for the 3 inside cores. Thus, Al averaged over the top 6 cm was greater inside than outside the treatment area by 4.76 mg/g (). Although the inside-to-outside difference averaged over 6 and 10 cm was similar (4.42 mg/g, or 10%), the variability was greater inside for 10 cm than for 6 cm (67% vs 4.5% of mean). Below 10 cm (to 15 or 20 cm), the difference between means inside and outside diminished (3.4 mg/g), indicating that most of the added Al was above 10 cm, although the variability among cores suggest some vertical mixing. The mean of all 9 inside observations from 0 to 6 cm (0–2, 2–4, and 4–6 cm × 3 cores) was significantly greater than the mean of the 18 (0–2, 2–4, 4–6 cm × 6 cores) outside concentrations (P = 0.018). Summed over the top 6 cm column, the areal difference was 47 g/m2 (637–590 g/m2) compared with an addition from the 2 treatments of 94.8 g/m2.
Table 2. Mean sediment concentrations (± SD) of water, Al, Al-P, Ca-P, and Fe-P averaged over the top 6 cm (0–2, 2–4, and 4–6 cm) following alum treatments in 2011 (21.5 mg/L Al) and 2012 (23.6 mg/L Al) to a mid-lake 4000 ha area. Three cores were taken inside and 6 outside the treated area following the 2012 treatment ().
Sediment Al concentrations in 3 cores outside the treated area on the windward side averaged less than the other 3 outside cores on the leeside: 520 vs. 590 g/m2. Using only leeside cores, the difference between Al inside and outside was 117 g/m2 compared to 47 g/m2 using all 6 outside cores. Variability, however, was greater among the 3 than among all 6 cores; thus, using all 6 outside cores was considered more representative of possible floc transport.
Aluminum-P content of the top 6 cm of sediment inside the treated area was, in nearly all samples, higher than outside and was less variable than Al content (). The mean of all concentrations at 9 sediment depths (0–2, 2–4, and 4–6 cm; 3 × 3) inside was significantly greater than the mean of the 18 outside concentrations at all depths (6 × 3; P = 0.0002). The areal means were 7.76 ± 0.14 g/m2 inside and 6.83 ± 2.3 g/m2 outside. Using the average of the 3 outside cores with slightly lower concentrations gave essentially the same mean mass per area (6.74 ± 3.3 g/m2) as using all 6 outside (6.83 ± 2.3 g/m2), further supporting the use of all 6 outside cores to determine the amount of Al added. The difference between the mean of inside samples and mean of the 6 samples outside (0.93 g/m2) gave an Al-added to Al-P–formed ratio of 50.5 (47/0.93 g/m2). If the actual Al dose (94.8 g/m2) were used, the Al-added:Al-P–formed ratio would be 102, indicating that the Al added had formed about twice as much Al-P as expected from the dose.
Sediment TP was rather low compared to most eutrophic lakes. Concentrations in the top 6 cm were significantly greater (P = 0.05) inside (mean = 709 ± 52 μg/g, dry weight) than outside (mean = 622 ± 89 μg/g). Higher sediment TP inside than outside was proportional to Al-P formed. Aluminum-P was 32% of TP inside and 26% outside.
Calcium-bound P was similar inside and outside the treated area because it is not part of mobile-P and would not have formed Al-P after Al addition (), supporting the validity of the inside-outside difference in Al and Al-P being due to the treatment. In comparison, Fe-P was also similar inside and outside (); the Fe-P fraction is usually mobile and should have declined as Al-P formed. Iron-P was very low in GLSM sediment, however, and was obviously a minor source for internal loading. Total Fe content was relatively large, providing a mean TFe:TP ratio of 52, 3.5 times greater than the limit for Fe control on P (Jensen et al. Citation1992).
Discussion
The alum floc was observed to readily settle through the water column immediately after application, despite high winds during the treatment of the central 40% of GLSM, but the clear-water condition was short-lived as wind mixed in water with high concentrations of algae. Nevertheless, only a relatively small fraction (<2–8%) of the applied Al remained in the water column near the end of the second application, with most in the sediment. A TAl residual of 8% of the dose is too high because the pretreatment was not zero, but possibly closer to the average TAl for 134 Ohio Lakes and reservoirs of 0.70 mg/L (0.029–10.3, n = 822; USGS Citation2016).
The rather fast settling of alum floc and water column clearing has been typically observed following other lake treatments that were properly dosed and buffered. The relatively small fraction of added TAl in the water column, possibly <5% assuming an average background of 0.70 mg/L, indicates that even in a large shallow lake during high winds, most of the floc seemed to reach the sediment.
Most of the TAl in lakewater near the end of treatment was in dissolved form, with a slightly higher dissolved fraction inside (81%) than outside (63%) the treated area. The high dissolved fraction is not unusual. Pretreatment TAl in Long Lake, Washington, was about 0.5 mg/L (less than half that in GLSM), with 70–100% in dissolved form. Concentrations actually decreased to 0.1–0.2 mg/L TAl after treatment (Cooke et al. Citation2005). Similarly high residual dissolved Al concentrations following treatments have been observed in other lakes; Campbell and Erie lakes in Washington (Entranco Citation1987) and Morey in Vermont (Smeltzer Citation1990).
The sediment core data show that between about 50% and 100% of the Al added (47 vs. 94.8 g/m2) was retained in the treated area, depending on whether observed Al in the top 6 cm of all 6 cores (590 g/m2) or only 3 outside (520 g/m2) cores were used to determine added Al, or if the 2-year dose were used. By using all 6 outside cores, the added-Al:Al-P–formed ratio was 51; using just 3 cores, the ratio was 116, which is even more than using the applied dose (102). The ratio of 51 is most reasonable because the ratio has been observed to decrease over time as Al continues to complex with P as more P becomes available, and half the total dose was applied a year earlier. Ratios of Al-added to Al-P–formed averaged 11 several years after treatment in the Washington lakes (Rydin et al. Citation2000). The Al-added:Al-P–formed ratio in Green Lake sediments decreased from 50:1 shortly after the 2004 treatment to 4:1 after 11 years (Welch et al. Citation2017). Thus, the ratio is expected to decrease further over time in GLSM.
Some uncertainty in the ratio may be due to transport of a portion of the alum floc out of the treated area due to wind-driven sediment resuspension. Resuspension of fine sediment in near shore areas leaving coarse material behind and refocusing the fines to deeper water areas is common in lakes. Even in a relatively small lake (Harriet, MN), alum added only to the littoral area was transported to deeper areas, reducing deep-water sediment-P release by 85% (Huser et al. Citation2011). Further, resuspension is an important mechanism for internal P loading in shallow lakes (Welch and Cooke Citation1995, Sondergaard et al. Citation2001). In GLSM, strong winds during treatment were largely from the southeast, which may have intermittently resuspended sediment in the treated area and transported it to the northwest. Wind speeds at GLSM, recorded at 07:00 and 19:00 h only, were consistently >4.5 m/s during spring–summer 2012. Higher unrecorded wind speeds were experienced at other times. As an example of wind-caused resuspension, winds >4.5 m/s were calculated to cause resuspension in 101 of 153 days during summer in Upper Klamath Lake, Oregon (26,500 ha, 2 m mean depth; Laenen and Letourneau Citation1996). Additionally, winds >8 m/s over a 4 km fetch produced resuspension in Lake Arre, Denmark (Kristensen et al. Citation1992), but Upper Klamath Lake is 5 times larger than GLSM and has greater sediment water content. Nevertheless, cores from sites 1 and 3, northeast and north of the treated area, had sediment Al concentrations much higher than those in cores 8 and 9 on the south and southeast, respectively (). Core sites 1 and 3 were 440 and 410 m from the treated area, and sites 8 and 9 were 675 and 775 m from the treated area. Resuspension may explain the relatively even distribution of constituents over the top 10 cm of the cores. In addition, resuspension was probably more pronounced in summer 2012 when the lake level was ∼0.43 m lower than in 2011 because of drought conditions. The Upper Klamath Lake study showed that at a wind speed of 4.5 m/s, bottom sheer stress would increase 4-fold by decreasing water depth 1 m.
Bioturbation by benthivorous fish can be an important mechanism that mixes the sediment column and may explain some of the uniform TAl concentrations to 10 cm and in some cores to 20 cm. Common carp were observed to mix sediment to an average depth of 13 cm, with maximums of 25 and 28 cm (Huser et al. Citation2016b), a possible occurrence in GLSM because it seems to have large populations of carp as well as gizzard shad (Filbrun et al. Citation2013).
The source of mobile P that formed Al-P is not obvious. Mobile Fe-P and loosely sorbed P are normally the source, but Fe-P was relatively low (38 μg/g) in GLSM sediment, and loosely sorbed P was undetectable (<2 μg/g). Iron-P ranged from 100 to 400 μg/g in sediments in 4 Wisconsin lakes and was completely depleted with the addition of Al at 100 g/m2 (Rydin and Welch Citation1999), only slightly more than the dose to GLSM. Mobile P (Fe-P) in Half Moon Lake, Wisconsin, was even higher at ∼2 mg/g and required 115 g/m2 Al to convert it to Al-P (James Citation2011). Background Fe-P below 10 cm in Green Lake, Seattle, Washington, ranged from 250 to 500 μg/g but was depleted to <100 μg/g above that depth by Al addition and corresponded directly with formed Al-P increasing from ∼250 μg/g to between ∼600 and 800 μg/g, shown in cores shortly after the 2004 alum treatment (Dugopolski Citation2008). By 2015, sediment Fe-P in Green Lake was nearly gone, and the Al-added:Al-P–formed ratio had decreased from 50:1 to 4:1. The dose for that second and well-distributed treatment in 2004 was 96 g/m2 Al (Welch et al. Citation2017).
Thus, the low and largely unchanged Fe-P content in GLSM sediment suggests that mobile-P producing internal loading and forming Al-P comes from some other source(s). Organic P in the form of living and decomposing algae from past blooms was a possible source, a fraction that should have occurred as biogenic P, but it averaged only 120 ± 9 μg/g inside and 112 ± 19 μg/g outside the treated area in GLSM. Biogenic P was rather high (∼600 μg/g) and was considered the source for high internal loading in Lake Vallentuna, Sweden, which also had very low Fe-P (100 μg/g). Biogenic P was not immediately affected by experimental Al addition, however, in contrast to Fe-P and loosely sorbed P (Rydin and Welch Citation1998). The lack of biogenic-P response to Al in Lake Vallentuna sediment seems consistent with results from GLSM. The higher TP inside than outside the treated area, proportional to Al:P formed, indicates that another P source was inactivated. That source was likely undetermined soluble reactive P diffused from deeper sediment as added Al continually sorbed P in surficial sediment, a process observed by Lewandowski et al. (Citation2003) in Lake Süser See sediment, and may well have been the source of Al-P in GLSM.
These results clearly show that even in a shallow hypereutrophic lake greatly disturbed by wind, Al added via alum did reach and largely remain in the sediment and form Al-P. Moreover, the treatments substantially reduced summer whole-lake internal P loading, despite treating only 40% of the lake, necessitated by economic constraints. No substantial and persistent reduction in summer mean whole-lake TP was found, however: 187, 162, and 180 μg/L during 2010 (pre-treatment), 2011, and 2012, respectively. The 2012 concentration was higher due to the lower (by 0.43 m) lake level. Correcting for lake level, areal mean TPs were 229, 221, and 221 mg/m2 for the 3 respective summers. Nevertheless, alum treatments to shallow lakes have been largely successful, reducing internal loading by >70% (Cooke et al. Citation2005, Huser et al. Citation2016a). Further, in-lake treatments have substantially improved lake quality without reducing external loading (Welch and Jacoby Citation2001). Improved lake quality may have occurred in GLSM as well, at least short-term, had the whole lake received 95 g/m2 of Al, although its external TP loading is large, and it is more eutrophic than most previously alum-treated lakes.
There are nevertheless uncertainties with the long-term management of GLSM water quality, such as the overall effectiveness of the partial alum treatments on whole-lake TP and the need to reduce inflow TP, which averaged 253 and 290 μg/L during 2011 and 2012 (Tetra Tech, Inc. Citation2013; see Filbrun et al. Citation2013 for consideration of management alternatives). Our focus, however, was on the fate of added Al and the extent of its effectiveness in forming sediment Al-bound P, which remains bio-unavailable and hence inactivated. Although whole-lake internal loading was substantially reduced by treating only 40% of the lake, 60% of the lake still contained TP concentrations between 100 and 200 μg/L without inactivation of sediment P. The whole-lake sediment P release rate was reduced, but the effect on whole-lake TP was compromised because lake level was nearly 27% lower than normal in 2012, providing a concentrating effect of the residual lower sediment release rate. Although these constraints help explain the lack of improvement in whole-lake quality, the alum treatment itself was nevertheless effective because the added Al stayed largely in the treated area and substantially reduced internal loading. The reduction of internal loading by 55% was calculated by a calibrated mass balance model on a whole-lake basis. Thus, reduction would have been much greater in the treated area because 60% of the lake was untreated, although the fraction of pre-treatment internal load coming from the deeper central 40% versus the shallower 60% is unknown.
Acknowledgments
The authors appreciate the statistical assessment provided by R.W. Plotnikoff. Thanks to Emil Rydin and Brian Huser for their reviews of the manuscript and suggestions.
Funding
The authors would like to thank Ohio EPA and Ohio DNR for their overall project funding and direct implementation support toward themanagement ofGLSM; specifically, we wish to thank the support provided by Russ Gibson, Rick Wilson, and Scott Fletcher.
References
- Cooke GD, Welch EB, Peterson SA, Nichols SA. 2005. Restoration and management of lakes and reservoirs. 3rd ed. Boca Raton (FL): CRC Press
- Dugopolski RA, Rydin E, Brett MT. 2008. Short-term effects of a buffered alum treatment on Green Lake sediment phosphorus speciation. Lake Reserv Manage. 24:181–189.
- Eaton AD, Clesceri LS, Rice EW, Greenberg AE, Franson MAH. 2005. Standard methods for the examination of water and wastewater. 21st ed. American Public Health Association, Water Environment Federation, and American Water Works Association.
- Entranco. 1987. Final Phase II report: Erie and Campbell Lakes: restoration, implementation and evaluation. Bellevue (WA): Entranco Engr. Inc.
- Filbrun JE, Conroy JD, Culver DA. 2013. Understanding seasonal phosphorus dynamics to guide effective management of shallow, hypereutrophic Grand Lake, St. Marys, Ohio. Lake Reserv Manage. 29:165–178.
- Huser BJ, Brezonik P, Newman R. 2011. Effects of alum treatment in Minneapolis Chain of Lakes, Minnesota, USA. Lake Reserv Manage. 27:220–228.
- Huser BJ, Egemose S, Harper H, Hupfer M, Jensen H, Pilgrim KM, Reitzel K, Rydin E, Futter M. 2016a. Longevity and effectiveness of aluminum addition to reduce sediment phosphorus release and restore lake water quality. Water Res. 97:122–132.
- Huser BJ, Przemyslaw GB, Chinski CJ, Sorensen PW. 2016b. Effects of common carp (Cyprinus carpio) on sediment mixing depth and mobile phosphorus mass in the active sediment layer of a shallow lake. Hyrobiologia. 763:23–33.
- James WF. 2011. Variation in the aluminum: phosphorus binding ratio and alum dosage considerations for Half Moon Lake, Wisconsin. Lake Reserv Manage. 27:128–137.
- Jensen HS, Kristensen P, Jeppesen E, Skytthe A. 1992. Iron-phosphorus ratio in surface sediments as an indicator of phosphorus release from aerobic sediments in shallow lakes. Hydrobiologia. 235/236:731–743.
- Kristensen P, Sondergaard M, Jeppesen E. 1992. Resuspension in a shallow eutrophic lake. Hydrobiologia. 228:101–109.
- Laenen A, LeTourneau AP. 1996. Upper Klamath basin nutrient loading study – estimate of wind-induced resuspension of bed sediment during periods of low lake elevation. Portland (OR): US Geological Survey, Open File Report 95-414.
- Lewandowski J, Schauser I, Hupfer M. 2003. Long term effects of phosphorus precipitations with alum in hypereutrophic Lake Süsser See (Germany). Water Res. 37:3194–3204.
- Perkins WW, Welch EB, Frodge J and Hubbard T. 1997. A zero degree of freedom total phosphorus model; 2. Application to Lake Sammamish, Washington. Lake and Reserv. Manage. 13(2):131--141.
- Psenner R, Bostrȍ M B, Dinka M, Pettersson K, Puckso R, Sager M. 1988. Fraction of phosphorus in suspended matter and sediment. Arch Hydrobiol Suppl. 30:98–103.
- Psenner R, Puckso R. 1988. Phosphorus fractionation: advantages and limits of the method for the study of sediment P origins and interactions. Arch Hydrobiol Suppl. 30:43–59.
- Rydin E. Welch EB. 1998. Aluminum dose required to inactive phosphate in lake sediments. Water Res. 32:2969–2976.
- Rydin E, Welch EB. 1999. Dosing alum to Wisconsin lake sediments based on in vitro formation of aluminum bound phosphate. Lake Reserv Manage. 15:324–331.
- Rydin E, Huser BJ, Welch EB. 2000. Amount of phosphorus inactivated by alum treatments in Washington lakes. Limnol Oceanogr. 45:226–230.
- Smeltzer E. 1990. A successful alum/aluminate treatment of Lake Morey, Vermont. Lake Reserv Manage. 6:9–19.
- Sondergaard M, Jensen JP, Jeppesen E. 2001. Retention and internal loading of phosphorus in shallow, eutrophic lakes. Sci World J. 1:427–442.
- Tetra Tech, Inc. 2013. Preliminary assessment of effectiveness of the 2012 alum application. Prepared for USEPA, Region 5, Chicago, IL. February.
- [USGS] US Geological Survey. 2016. National Water Quality Portal. USGS, USEPA, and National Water Quality Monitoring Council 2005–2016. Accessed 10 August 2016.
- Welch EB, Cooke GD. 1995. Internal phosphorus loading in shallow lakes: importance and control. Lake Reserv Manage. 11:273–281.
- Welch EB, Cooke GD. 1999. Effectiveness and longevity of phosphorus inactivation with alum. Lake Reserv Manage. 15:5–27.
- Welch EB, Gibbons HL, Brattebo SK, Corson-Rikert HA. 2017 Forthcoming. Progressive conversion of sediment mobile P to aluminum P. Lake Reserv Manage.
- Welch EB, Jacoby JM. 2001. On determining the principal source of phosphorus causing summer algal blooms in western Washington lakes. Lake Reserv Manage. 17:55–65.