ABSTRACT
Welch EB, Gibbons HL, Brattebo SK, Corson-Rikert HA. 2017. Progressive conversion of sediment mobile phosphorus to aluminum phosphorus. Lake Reserve Manage. 33:205–210.
Sediment core data show that the 2004 alum treatment of Green Lake, Seattle, Washington, was not only initially effective at forming aluminum phosphorus (Al-P) and reducing mobile-P, but that the process of forming Al-P continued during the following 11 years. Increases in Al-P concentrations were determined by differences in levels at 0–10 and 0–20 (or 20+) cm between cores taken in 1998 (7 years after the first treatment), 2004 (6 months after the second treatment), and 2015. Most of the increase in Al-P formed since 1998 occurred between cores taken 6 months after the 2004 treatment and 2015. That increase was 60% at the deepest site and 84% at a shallower more central site. An increase in Al-P between 2004 and 2015 also occurred at 2 remaining shallower sites. Mobile-P (Fe-P and labile-P) decreased during this period, associated with increased Al-P, but the magnitude of change was less, probably because mobile-P had been largely depleted and P had diffused from deeper sediment. The increase in Al-P resulted in a decrease in the Al-added (dose) to Al-P–formed ratio in the top 25 cm at the deep site from 15:1, 6 months after the 2004 treatment, to 3.7:1, 11 years later. At the shallower more central site, that ratio decreased from 57:1 to 24:1 using the top 10 cm, or 28:1 to 10:1 in the top 20 cm. Higher ratios at the shallower site than at the deep site may be due to focusing of floc to deeper areas.
An important question regarding the longevity of alum treatments is the extent to which added aluminum (Al) continues to bind additionally available sediment phosphorus (P). Sediment mobile P was observed to compete for Al floc binding sites with other substances, thereby reducing its longer-term continued binding potential (James and Bischoff Citation2015). Mobile P was also found to diffuse from deeper sediment layers to bind with the added Al floc (Lewandowski et al. Citation2003); however, few examples show the long-term P-binding effectiveness of alum. The experience from 2 alum treatments to Green Lake in Seattle, Washington, over 25 years provides evidence that, at least under some conditions, mobile P will continue to bind to added Al several years after treatment, assuming the treatment was conducted according to specifications with adequate Al added. The Al doses for the 2 treatments to Green Lake were 33.5 g/m2 in 1991 and 92 g/m2 in 2004. Short-term effects of these treatments have been reported previously (Jacoby et al. Citation1994, Dugopolski et al. Citation2008).
Site description
Green Lake is 105 ha with mean and maximum depths of 3.9 and 8.2 m, respectively. The lake is unstratified in summer except for the deep pocket near the southeast shore; therefore, the water column remains largely oxic. The lake is an important recreational site in Seattle. The lake level was lowered by 2 m in 1911, and bottom sediment was subsequently dredged, removing 690 × 103 m3 for fill to expand park land. The deep pocket area is the result of that dredging.
External loading of TP is in runoff from an urban area that has changed little over the past 25 years; however, the lake has a deep, moderately P-enriched sediment layer. An average sediment total phosphorus (TP) concentration of 1.09 mg/g (±0.37) was determined in a sectioned 6 m core taken near the lake's center in 1959. Sediment above that 6 m depth was deposited since a volcanic eruption 6700 years ago, as determined by a 2.5 cm ash layer (Sylvester and Anderson Citation1964).
Methods
Sediment core samples were collected from 2 sites in April 1998 using a Wilner gravity corer with a 0.5 m tube; one site at the deep pocket (Index Station) and the other site near Station A toward the lake's northern end (). Cores were taken at the Index and A station sites in October 2004 and May 2015 as well as at a southern centralized (Station B) and western littoral site (Littoral Station) by a SCUBA diver inserting a 1 m long, 5 cm diameter tube carefully into the sediment (Dugopolski et al. Citation2008, Herrera Environmental Consultants, Inc. Citation2015). Core sections were collected at 1 cm intervals from the surface to 20 cm and also at 26 cm in 1998. Sectioned intervals in 2004, 6 months following the second treatment, were at 1 cm intervals to 10 cm depth and every 5 cm below that to 40 cm. Sample depths were fewer in 2015, at 1, 3, 5, 8, 12.5, 17.5, 22.5, and 27.5 cm.
Figure 1. Station locations for coring sites in Green Lake, WA (from Dugopolski et al. Citation2008).
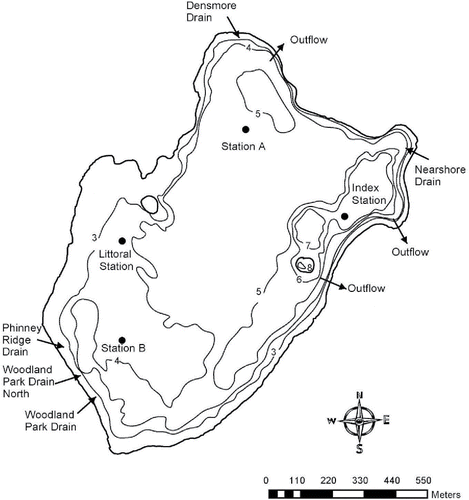
Each core section was analyzed for percent water, Al, TP, and the P fractions Al-P, calcium (Ca)-P, iron (Fe)-P, labile-P, and biogenic-P, the more mobile fraction of organic P. Only TP, Al, Fe-P, labile-P, and Al-P data are described here. These analyses were performed by IEH Analytical Laboratories, formerly Aquatic Research, Inc., Seattle, Washington, according to the scheme of Psenner et al. (Citation1988), Psenner and Puckso (Citation1988), and referenced in Rydin and Welch (Citation1998, Citation1999).
Results and discussion
A small number of cores were taken each year, and the seasons sampled varied; nevertheless, data trends were significant, even though the exact numerical values reported may have a larger associated error than the trends. Core data show that Al-P was much greater and mobile-P, as Fe-P and labile-P, was much lower throughout the sediment column at all 4 coring sites in 2015 than 6 months after treatment in 2004 (, Figs. and ). That change was also present at the Index and A station sites between 1998 and 2004, showing the effect of the 2004 treatment. The 2015 data show that the effect of the 2004 alum treatment, in terms of decreased mobile-P and increased Al-P, continued during the intervening 11 years, especially between 10 and 30 cm at the Index Station for Al-P formation and throughout the 30 cm core at the Station A site. There was usually a greater increase in Al-P formed between 1998 (7 years after the 1991 treatment) and 2004 and between 2004 (6 months after treatment) and 2015 than a decrease in mobile-P between those 2 periods (). At the Index Station, Al-P increased 0.667 mg/g and mobile-P decreased 0.410 mg/g at 0–20 cm depth between 1998 and 2015, whereas at A Station, Al-P increased by 0.386 mg/g and mobile-P decreased 0.285 mg/g over that period. Sixty percent of the Al-P increase at Index Station and 84% at Station A occurred between 2004, 6 months after treatment, and 2015, 11 years later. Thus, added Al continued to inactivate P and to greater depths in the sediment column well after the treatment, and the formed Al-P remained stable. Some of that increased Al-P may have been formed from added P diffused from deeper sediment because TP also increased (), a significant indication of the positive and long-term effect of the 2004 alum treatment.
Table 1. Average depth weighted sediment concentrations of TP, Al-P, and mobile-P in the top 10 and 20 cm depths at the Index Station, Station A, Station B, and the Littoral Station in 1998 (7 years post-alum), 2004 (6 months post-alum), and 2015. Concentrations in (mg/g DW).
Figure 2. Sediment aluminum-bound phosphorus at the Index Station and Station A, Green Lake, WA, in 1998, 2004 (6 months post-alum), and 2015.
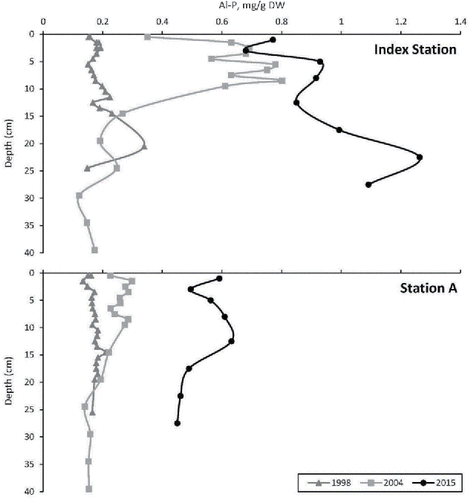
Figure 3. Sediment mobile phosphorus (Fe-P and labile-P) at the Index Station and Station A in Green Lake, WA, in 1998, 2004 (6 months post-alum), and 2015.
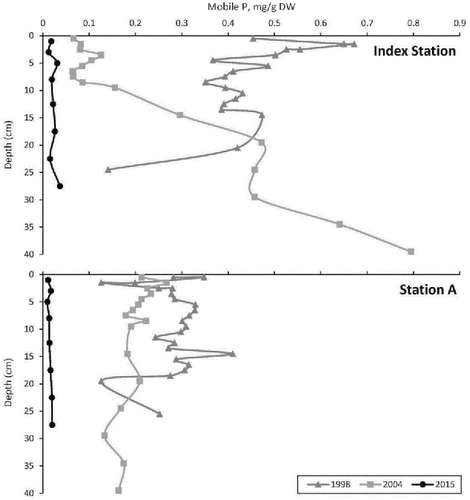
The Al-added to Al-P–formed ratio continued to decrease over time accordingly. Al-P concentrations at the Index Station averaged 0.173, 0.649, and 0.842 mg/g, respectively, over the top 10 cm in cores collected in 1998, 2004, and 2015, respectively (). Note that Al-P content had not increased from 1998 levels much deeper than 10 cm 6 months after the 2004 treatment (). These concentrations converted to 1.57, 7.72, and 10.0 g/m2 for the 3 observation years, respectively. The difference between 1998 and 2004 (after treatment) was 6.15 g/m2, the approximate amount of Al-P initially formed by the 2004 alum treatment. The ratio of Al added in 2004 (92 g/m2) to the Al-P formed (6.15 g/m2) was 15:1, which indicates the effect of sediment P initially inactivated with Al by the 2004 treatment. Observed Al-P content could not be used to more accurately estimate Al-P formed because cores were not deep enough to establish background levels.
The 2015 cores show that additional Al-P was formed at the Index Station because sediment cores were collected 6 months after the 2004 treatment (). The Al-P concentration in 2015 averaged 0.975 mg/g or 29 g/m2 through the top 25 cm of sediment. More Al-P was actually formed than this amount because cores did not extend deep enough to determine the depth at which Al-P matched background levels (i.e., pre-treatment conditions in 1998; ). The difference between 29 g/m2 and the average amount down to 25 cm in 1998 (4.35 g/m2) is 24.7 g/m2, which yields an Al-added to Al-P–formed ratio of 3.7:1 (92/24.7). That ratio is much lower than the ratio of 15:1 initially observed after the 2004 treatment and could be even lower if the depth at which Al-P returned to pre-treatment levels were known.
Al-P concentrations in the top 10 cm at A Station in 2004 and 2015 were lower than at the Index Station, averaging 0.179, 0.263, and 0.573 mg/g in 1998, 2004, and 2015, respectively (, ). These concentrations represented, respectively, 1.40, 3.21, and 7.0 g/m2 Al-P on an areal basis. The averages were similar in the top 0–20 cm; 0.181, 0.243, and 0.573 mg/g, which converted to 3.42, 6.75, and 15.9 g/m2, respectively. Although the progression of increasing Al-P over the period was substantial, less Al-P was formed at A Station than at the Index Station, probably because floc was focused to deeper areas (, ). Al-added to Al-P–formed ratios of 51:1 and 28:1 represent the effect through 10 and 20 cm, respectively, 6 months after the 2004 treatment. Effectiveness increased during the intervening years, with much lower ratios of 24:1 and 10:1 for the 10 and 20 cm sediment depths. As noted for the Index Station, the actual ratios at Station A may be slightly lower because Al-P formed did not clearly reach background (1998 levels) within the analyzed 30 cm depth (). Al-P concentrations were only slightly higher at B Station than at A Station, producing similar effectiveness of Al-added to Al-P–formed ratios of 26:1 and 10:1 for 10 and 20 cm sediment depths between 2004 and 2015. Although Al-P concentrations were higher at the Littoral Station than at A or B stations in 2004, they were lower in 2015, so the increase in effectiveness of Al-P formed during the 11 years was less (). There were no core data from the B or Littoral stations in 1998. These binding ratios may continue to decline according to a relationship based on a core depth index and dose that predicted ratios of 7:1 to 9:1 (Huser Citation2012).
The average Al-added to Al-P–formed ratio observed in 8 Washington lakes at 7 to 21 years after alum treatments was 10.7 ± 0.7 (Rydin et al. Citation2000). Also, Al-P formed above background occurred at depth intervals <10 cm in all 8 Washington lakes. Binding ratios have ranged from 2.1:1 to 13:1 in other lakes with well-defined Al-added markers. Why the depth of Al-P formed was eventually much greater in Green Lake (>30 cm; ) is not clear but may possibly be due to bioturbation by carp, which are likely much more abundant in Green Lake than in the other alum-treated lakes studied. For example, a fisheries study in the 1970s showed no carp in Long Lake, Kitsap County, one of the cited 8 lakes. A recent study of an alum-treated lake with abundant carp and soft sediment measured the average carp bioturbation depth at 13 cm with maximums of 25 and 28 cm (Huser et al. Citation2015), which suggests that carp bioturbation may not have been sufficient to mix sediment beyond 30 cm, as observed in Green Lake. Also, alum doses (measured as Al) were much lower in all but 2 of the other Washington lakes studied than the 2004 treatment in Green Lake, ranging from 11 to 27 g/m2. Medical Lake is the one other Washington lake studied with a high dose (122 g/m2 in 1977), and the peak Al-P formed by 1998 was distinctive at a depth of only 6.5–7.5 cm (Rydin et al. Citation2000). Thus, the high Al-P formed through the top 30 cm in Green Lake may not be readily explained by either carp bioturbation or the high dose. However, the lack of a definite marker in Green Lake from the 1991 treatment, as was observed in the other Washington lakes, may well have been due to carp bioturbation because Green Lake sediment is much less dense (water content ∼90%) than the earlier cited lake (Huser et al. Citation2015) with measured bioturbation (70%–80% water), and carp densities were larger in Green Lake. Unfortunately, no pretreatment P fraction data before the 1991 treatment were available to compare, as was possible with the 2004 treatment discussed earlier.
The actual Al dose in 2004 (92 g/m2) was used to judge Al-P binding effectiveness, assuming that the alum distribution was even and conservative across the lake. Although the treatment was closely supervised, the distribution was probably not precisely even for a variety of reasons, so all individual cores may not have received the same actual dose. An alternative is to determine the Al added from an identifiable bulge in Al concentration in the sediment profile. That method was not possible, however, because determined total Al concentrations were inconsistent among cores from the 3 dates, and there was no indication of an Al bulge in the 2015 cores. Observed Al added from cores has usually been less than the dose added; an average of only 50% was recovered after 8–22 years following treatment in the 8 Washington lakes (Rydin et al. Citation2000). Recovery in the Minneapolis Chain of Lakes ranged from 46% to 185% with higher recoveries in deeper stratified lakes because of focusing (Huser Citation2012). Thus, the Al-added to Al-P–formed ratios estimated using the actual dose may be too high by a factor of 2, although the progressive trend in Al-P formation between 1998 and 2004 (after the treatment) and between 2004 and 2015 would not change.
Sediment TP did not increase after the 1991 treatment, averaging 1.13 and 0.97 mg/g at the Index and A stations, respectively, to a depth of 20 cm, as shown in the 1998 cores (, ), similar to the average to a depth of 6 m at a central site determined in 1959 (1.09 mg/g). However, sediment TP had increased to an average of 1.59 and 1.25 mg/g to the 20 cm depth at the same 2 sites (Index and A stations) by 2004 after the second treatment and to 1.80 and 1.36 mg/g over the same depth interval by 2015 (). These values represent an increase at the index site of 40% and 60% by 2004 and 2015, respectively, from 1998 levels, and a 30% and 40% increase at A Station. Such an increase would be expected to show up only in the top few centimeters if caused by an increase in external sources of TP. That the increase occurred to a sediment depth of 20 cm suggests that the added P diffused largely from historic TP reserves at greater depths, a process suggested by Rydin et al. (Citation2000). Some of the increased TP probably contributed to the increased Al-P formed.
Figure 4. Sediment total phosphorus at the Index Station and Station A in Green Lake, WA, in 1998, 2004 (6 months post-alum), and 2015.
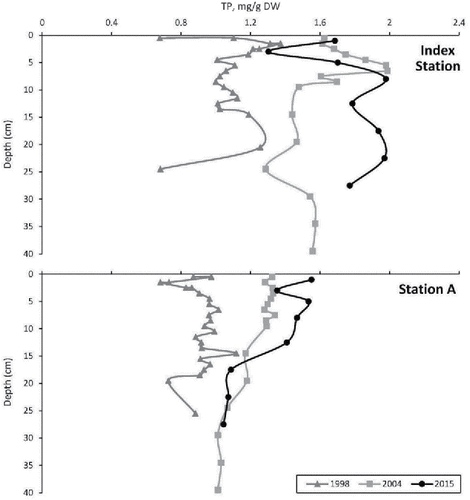
Biogenic P concentrations were similar in the top 25 cm in 1998, 2004 (6 months after treatment), and in 2015, ranging from 0.30 to 0.445 mg/g at the Index Station and 0.275 to 0.352 mg/g at A Station. There was no indication that much of the increase in Al-P came from biogenic-P. There was also no indication that biogenic-P formed much Al-P in laboratory experiments with sediments from Lake Vallentuna, which had a relatively high internal loading but little mobile Fe-P (Rydin and Welch Citation1998), yet biogenic-P has been routinely included in alum dose determinations (de Vicente et al. Citation2008).
Acknowledgments
The authors appreciate the interpretive advice from Emil Rydin and Brian Huser regarding their data from 1998, and to Brian Huser for suggestions on this manuscript. The authors also thank Rob Zisette and Rebecca Dugopolski of Herrera Environmental Consultants, Inc., for collecting cores in 2004 and 2015 and for providing some of the historical data.
References
- de Vicente I, Huang P, Andersen FØ, Jensen HS. 2008. Phosphate adsorption by fresh and aged aluminum hydroxide, consequences for lake restoration. Environ Sci Technol. 42:6650–6655.
- Dugopolski RA, Rydin E, Brett MT. 2008. Short-term effects of a buffered alum treatment on Green Lake sediment phosphorus speciation. Lake Reserv Manage. 24:181–189.
- Herrera Environmental Consultants, Inc. 2015. Phosphorus management plan: Green Lake alum treatment 2016. Seattle (WA): Herrera Environmental Consultants, Inc.
- Huser BJ. 2012. Variability in phosphorus binding by aluminum in alum treated lakes explained by lake morphology and aluminum dose. Water Res. 46:4697–4704.
- Huser BJ, Przemyslaw GB, Chinski CJ, Sorensen PW. 2015. Effects of common carp (Cyprinus carpio) on sediment mixing depth and mobile phosphorus mass in the active sediment layer of a shallow lake. Hydrobiologia. 763:23–33.
- Jacoby JM, Gibbons HL, Stoops KB, Bouchard D. 1994. Response of a shallow, polymictic lake to buffered alum treatment. Lake Reserv Manage. 10:103–112.
- James WF, Bischoff JM. 2015. Relationships between redox-sensitive phosphorus concentrations in sediment and the aluminum:phosphorus binding ratio. Lake Reserv Manage. 31:339–346.
- Lewandowski J, Schauser I, Hupfer M. 2003. Long term effects of phosphorus precipitations with alum in hypereutrophic Lake Süsser See (Germany). Water Res. 37:3194–3204.
- Psenner R, Bostrȍ m B, Dinka M, Pettersson K, Puckso R, Sager M. 1988. Fraction of phosphorus in suspended matter and sediment. Arch Hydrobiol Suppl. 30:98–103.
- Psenner R, Puckso R. 1988. Phosphorus fractionation: advantages and limits of the method for the study of sediment P origins and interactions. Arch Hydrobiol Suppl. 30:43–59.
- Rydin E, Huser B, Welch EB. 2000. Amount of phosphorus inactivated by alum in Washington lakes. Limnol Oceanogr. 45:226–230.
- Rydin E, Welch EB. 1998. Aluminum dose required to inactivate phosphate in lake sediments. Water Res. 32:2969–2976.
- Rydin E, Welch EB. 1999. Dosing alum to Wisconsin lake sediments based on possible in vivo formation of aluminum bound phosphorus. Lake Reserv Manage. 15:324–331.
- Sylvester RD, Anderson GC. 1964. A lake's response to its environment. American Society of Civil Engineers (ASCE). J Sanit Eng Div. 90:1–22.