ABSTRACT
Emamgholizadeh S, Bateni SM, Nielson JR. 2018. Evaluation of different strategies for management of reservoir sedimentation in semi-arid regions: a case study (Dez Reservoir). Lake Reserv Manage. 00:00–00.
Reservoir sedimentation is a major concern, especially in arid and semi-arid areas, such as Iran. Dez Reservoir, with a primary storage capacity of 3315 × 106 m3, located on the Dez River, is an important dam in Iran that suffers from sedimentation problems. Large volumes of sediments are impounded every year in this reservoir, particularly during hyper-concentrated floods. The accumulation of sediments in the Dez Reservoir has negatively impacted its utilities, such as power generation, flood control, and water supply. In this study, several different strategies of sedimentation management were assessed, including watershed management, sluicing, flushing, density current venting, and bypass. The results show that sedimentation in the Dez Reservoir can be reduced by 11–15% by venting density currents, which entails discharging incoming density currents through irrigation gates before they get impounded in the reservoir. Sedimentation can be reduced by approximately 2% by pressure flushing, which would decrease sediment depositions near the power plant intake. By construction of a submerged dam 9 km upstream of Dez Dam Reservoir, the bypass technique can be used to release 10.8 × 106 m3 of sediments per year and discharge turbid flow during the flood season. Finally, implementing watershed management strategies in the Sezar catchment could reduce the inflowing sediment to Dez Reservoir by 15%. Dez Reservoir does not have sluice gates and, therefore, the free flushing and sluicing methods cannot be used as mitigation strategies.
Demand for water is progressively increasing worldwide at a projected rate of 2–3% per year over the coming decades (ICOLD Citation2013). Reservoirs worldwide, which currently have an aggregate storage of about 6197 km3, are a substantial component of the water supply infrastructure (Lehner et al. Citation2011). During the last several decades, the number of new reservoirs has increased (Sumi and Kantoush Citation2010). This increase in the number of reservoirs eventually results in an increase in the number of reservoir sediment management problems, such as sedimentation, especially in semi-arid areas where high sediment yields are typically prominent.
Sedimentation in reservoirs leads to a number of negative consequences, including losses in storage capacity, impaired reservoir operation, diminished useful life of the reservoir, and reduced reservoir water supply, flood control, power generation, navigation, irrigation, fishing, and recreation (Alatorre et al. Citation2010, Kondolf et al. Citation2014, Adam et al. Citation2015, Rashid et al. Citation2015, Alighalehbabakhani et al. Citation2017, Shukla et al. Citation2017). Yoon (Citation1992) showed that on average almost 1% of the total storage volume of reservoirs worldwide is lost annually due to sedimentation. The rate of storage loss in some parts of Asia is generally greater than the world average of 1%, and varies significantly from country to country due to geological conditions and different land covers (Shuguang et al. Citation2002).
Different strategies can be used to manage reservoir sedimentation. However, the specific solution depends on the individual project characteristics. For example, drag line, dredging, and hydro-suction can be effective choices for relatively small reservoirs, but for large reservoirs, these techniques might be too costly and time prohibitive. Therefore, choosing the appropriate method must be done with respect to the individual site characteristics and the feasibility of the proposed solution (Jones et al. Citation1981). Many reservoirs in Iran suffer from sedimentation problems, which occur at much faster rates than predicted (). The average annual loss of storage capacity is 0.69% per year. This annual loss is mainly due to the sparse forest cover in the upper parts of the catchments, which contributes to higher erosion yields. Dez Reservoir is one of Iran's major reservoirs that has been affected by sedimentation. The purposes of this study were to evaluate 5 strategies, namely (1) watershed management, (2) sluicing, (3) density current venting, (4) free and pressure flushing, and (5) sediment bypass techniques, to determine which techniques work best to control reservoir sedimentation in Dez Reservoir.
Data and methods
Study area
The Dez watershed is located in an arid region in southwestern Iran. Sezar and Bakhtiary are the 2 subbasins of this watershed. The mean annual precipitation at the Dez watershed is 496.3 mm. About 88% of total annual rainfall in the Dez watershed occurs from November until March. The highest and lowest precipitation occurs in January and June, respectively. The mean annual temperature in this watershed is 22 C.
A variety of land covers such as alpine, forest, and semi-steppe can be seen in the Dez watershed. The northern part of both subbasins is covered with moderate to dense forests. The study area is covered mainly by shale, sandstone, marl, conglomerate, and limestone formations, and its soil textures are loam, sandy loam, sandy clay loam, and loamy coarse sand. Dez Dam was constructed mainly for power generation, irrigation, and flood control. The irrigation demand downstream (with an area of 125 × 107 m2) is 1.21 × 109, 1.19 × 109, 3.13 × 109, and 1.21 × 109 m3 in autumn, winter, spring, and summer, respectively.
The catchment area of the Dez Reservoir is 17,365 km2, which is nearly 1% of the land area of Iran. Dez River flows through the Zagros and Bakhtiary mountains before reaching the Khuzestan plain. Dez River is fed by 2 main tributaries, namely the Sezar and the Bakhtiary, which respectively supply 58% and 42% of the Dez Reservoir water (). The intense rainfall, lack of plant cover, and steep slopes of the basin are the main factors of soil erosion in the study area. Erosion in the study area is significant, especially in the Sezar subbasin where there are bare, unprotected slopes. Dez Reservoir is one of the most important large reservoirs in Iran. Construction of Dez Dam on the Dez River began in January 1960 and reached completion in November 1962. Dez Dam is located approximately 25 km north of the city of Dezful, and 22 km from the city of Andimeshk, in the Khuzestan province ().
Figure 2. The plan view of Dez watershed, Dez Reservoir, and 2 tributaries of Sezar and Bakhtiary on Dez River.
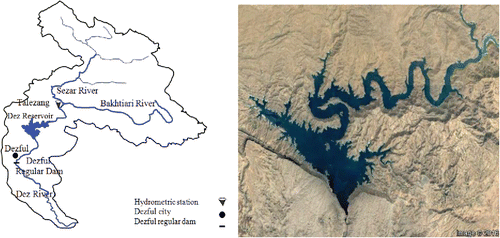
The elevation of the reservoir bottom in 1962 at the dam was 148 m and 3 low-level outlets were constructed at the base of the dam, having a centerline at elevation 222.7 m. The original purpose of these low-level outlets was to supply irrigation water to downstream areas during periods of low-flow discharge. Additionally, these outlets allow the release of water from the reservoir during emergency conditions. It is unknown, however, whether the outlet valves were designed to discharge sediment. Nonetheless, they have been frequently used for this purpose since 1994. Also, twin power plant intakes with inverters are set at elevation 270 m (47.3 m above the outlets).
Field surveys and bathymetric data from Talezang hydrometric station were used to calculate the sediment yield from Dez watershed in order to estimate the sedimentation in Dez Reservoir. Talezang hydrometric station is located 15 km upstream of Dez Dam. Its upstream catchment area is 16,130 km2. The suspended sediment load at this station, which generally consists of fine materials (clay, silt, and fine sand) was measured with a depth integrating sampler (DH-49, US Geological Survey). The sediment samplings were performed at 1/4, 1/2, and 3/4 of the width of the river and finally their mean was reported as the suspended sediment concentration.
The average discharge of the Dez River at Talezang hydrometric station is 270 m3/s, which is obtained by multiplying the Dez River cross-section area by the average flow velocity at Talezang hydrometric station. The flow velocity was measured by an impeller current meter with an accuracy of ±1.5% of reading above 0.15 m/s and ±0.004 m/s below 0.15 m/s. The suspended sediment concentration (S) versus the discharge (Q) at Talezang station is shown (). The bed load, which moves along the bed by rolling, sliding, or saltation was not measured in this station. However, it can be approximated as 10–20% of the suspended load (Torabi-Pode Citation1999, Azarang and Bajestan Citation2015). Monthly variations of suspended sediment load and discharge at Talezang hydrometric station are also shown (). It is evident that the changes in Qs are consistent with those of Q. During the high inflow season (spring and winter), Qs is also high. In contrast, during the low inflow season (summer and fall), Qs is low as well. In April, Q and Qs reach their peak values of 676 m3/s and 3.14 × 109 kg/month, respectively. Overall, Qs at Talezang hydrometric station varies significantly in different months, mainly depending on the inflow ().
Figure 3. The relation between suspended sediment concentration (S, kg/m3) and discharge (Q, m3/s) at Talezang hydrometric station in Dez River.
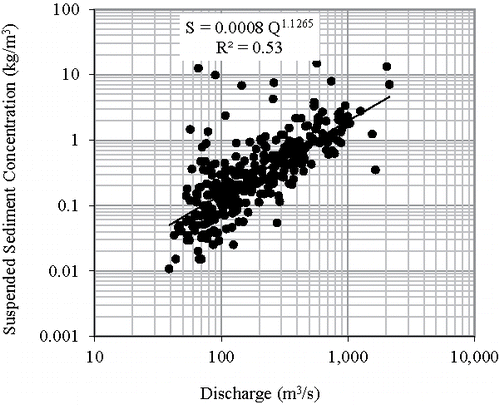
Figure 4. Monthly variations of discharge (Q) and suspended sediment load (Qs) at Talezang hydrometric station.
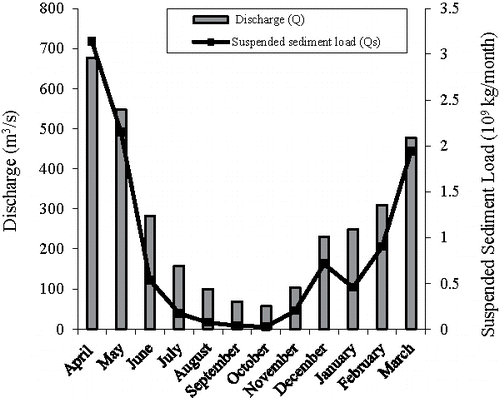
The observed annual suspended sediment load from 1955 to 2011 shows a long-term average annual suspended load of 8.8 × 109 kg per year. Assuming a density of 1100 kg/m3 for fine submerged sediments and a multiplier of 1.15 for bed loads, an annual infilling sediment load of 9.2 × 106 m3 per year was obtained. Most likely, this value is an underestimate, because only a fraction of suspended sediment measurements were taken at high discharges. The calculations based on bathymetric data and field surveys show the sediment yield over the 36-year period (1962–1997) was 568.8 × 106 m3, which corresponds to an annual rate of 15.8 × 106 m3/yr, and a specific sediment yield of 910 m3/km2/yr for the entire watershed (or 1 kg/m2/yr). After several years of impounding, the incoming sediment has formed a delta at the inlet, which is growing toward the dam body (Emamgholizadeh and Samadi Citation2008). The reservoir bed at the face of the power plant intakes has increased from the original elevation of 170 m in 1962 to 260 m in 2007, which is only 8 m below the level of the power plant intakes. Thus, the sediment level near the dam has risen at a rate of about 2 m per year. In fact, there is a concern that the sediment will eventually enter the intakes and damage the turbine runners and other power generation components. In the original design of Dez Reservoir, a volume of 840 × 106 m3 was considered as the dead storage of the reservoir. The dead storage was estimated to fill up with sediment after about 70 yr of operation (in 2032). This estimate was achieved by considering several plans such as sediment retention structures and reforestation programs for upstream areas of Dez Dam. However, these mitigation plans were not carried out, which caused the dead storage of Dez Reservoir (840 × 106 m3) to fill up in 2015 (i.e., earlier than anticipated).
The original gross storage volume of Dez Reservoir was 3315.6 × 106 m3 at a water surface elevation of 350 m, while the available gross volume in 2002/2003 was equal to 2698.5 × 106 m3 (this was determined from reservoir surveys and contour maps), which corresponds to a volume loss of about 19%. Bed profile of the reservoir from the survey data is plotted for different years (). The size distribution and density of the deposited sediment within Dez Reservoir were determined using gravity cores of the sediment. Results indicated that the median particle size of all samples taken from near dam to the delta region was less than 0.01 mm, and samples are composed of 60% silt and 40% clay (Emamgholizadeh and Fathi-Moghdam Citation2014).
Watershed management
As a result of runoff from snowmelt and rainfall, soil erosion (gully, sheet, and rill erosion) occurs in watersheds. The eroded soil particles are transported through river systems and are deposited in lakes, reservoirs, or oceans. When the rates of sediment erosion are accelerated by human activities, this process can lead to losses in ecosystem services (Molina-Navarro et al. Citation2014). In areas that suffer from the soil erosion problem, a quantitative evaluation of soil erosion and also an assessment of possible mitigation and management strategies are needed by policy makers and planning agencies (De Roo et al. Citation1996). Different techniques such as empirical models (e.g., Modified Pacific Southwest Inter-Agency Committee [MPSIAC], Erosion Potential Method [EPM], Fournier, Douglas, Kirby) and field surveys (the bathymetric survey of the reservoir or measured sediment loads at the hydrometric stations) can be used to determine the erosion rate of a watershed (Morris and Fan Citation1998, Aksoy and Kavvas Citation2005). In this study, the bathymetric survey of the Dez Reservoir was used to estimate the sediment yield of Dez watershed. Also, the Geographic Information System (GIS) and the MPSIAC model were applied to generate the sediment yield map of Dez watershed (). The MPSIAC model was calibrated with the bathymetric survey data.
Studies have shown that high sediment yields are associated with arid/semi-arid, seasonal Mediterranean, and tropical regions (Walling and Webb Citation1983). Arid and semi-arid areas are particularly sensitive to erosion because of scarce vegetation cover, steep topography, and intense rainfall after a long dry season (Bronstert et al. Citation2014). Comparisons between sediment yields of different regions with different climates (arid, humid temperate, and humid tropical) show that arid basins export 21 times more sediment than humid tropical basins, and 36 times more than humid temperate watersheds (Reid and Frostick Citation1987). Sediments cause problems in reservoirs and lakes. In particular, sediments can deteriorate water quality, and have negative effects on biological and physical processes in these receiving systems (Walling and Webb Citation1996). While these erosion processes are natural, human activities increase sediment transport to reservoirs through soil degradation. Estimates show that nearly 2 × 1013 m2 of land have undergone human-induced soil degradation. Additionally, 1100 × 1010 m2 and 550 × 1010 m2 of land area experience soil degradation respectively due to water and wind erosion (Lal Citation2001).
Different techniques have been used to reduce soil erosion and sediment yield. These techniques can be mainly classified into 3 categories (Morris and Fan Citation1998): (1) structural or mechanical measures, such as check dams, debris basins, and sediment retention basins; (2) vegetative or agronomic measures; and (3) operational measures. Some of these watershed management programs, however, can be costly, requiring large expenditures. They can also fail to achieve a reduction in sediment yield for various reasons, which can include cultural or economic issues, or the misidentification of the physical nature of the problem (Morris and Fan Citation1998).
Hydraulic flushing
Hydraulic flushing is a method of removing deposited sediment from a reservoir, by releasing water from the reservoir, so that the resulting drawdown scours the accumulated sediment and transports it downstream. Depending on the level of drawdown, hydraulic flushing is classified into 2 general categories: free flushing and pressure flushing (Fan Citation1985).
Free flushing is done by discharging the water of the reservoir, allowing riverine flow to transport sediment through the impoundment (Morris and Fan Citation1998). This method is recommended when the reservoir can reach its lowest drawdown level, so that the onset of the monsoon or a large storm could provide flood flows to scour deposited sediments and transport them downstream (Tate and Farquharson Citation2000). This method has been used in many reservoirs, including Tapu in Taiwan (Lai and Chang Citation2001), Madesimo Reservoir in Italy (Brignoli et al. Citation2017), Gebidem Reservoir in Switzerland (Meile et al. Citation2014), Baira Reservoir in India (Chaudhry et al. Citation2014), Gmund Reservoir in Austria (White Citation2001), Hengshan and Honglingjin Reservoirs in China, Mangahao Reservoir in New Zealand, Santo Domingo Reservoir in Venezuela (Atkinson Citation1996), and SefidRoud Reservoir in Iran (Morris and Fan Citation1998, Hajiabadi and Zarghami Citation2014).
In contrast to free flushing, in pressure flushing the water level in the reservoir is only slightly lowered. Due to considerable shear flow at the outlet, deposited sediments are removed from the reservoir, typically leaving a funnel-shaped crater or scour cone (Emamgholizadeh Citation2010, Fathi-Moghadam et al. Citation2010). Once the cone is formed, very little sediment will be entrained in the subsequent discharge (Di Silvio Citation1990). Generally, the dimensions of the scour cone are influenced by many factors such as sediment characteristics, submerged angle of repose, inflow and outflow water discharge, and outlet geometry (White Citation2000).
Sluicing
Sluicing or sediment routing operations can be used to prevent sediment deposition in reservoirs. Flood flows typically bring high sediment concentrations into reservoirs. By opening sluice gates (sluicing), impoundment time can be decreased, and the flood flows with high sediment concentrations can be routed downstream of the reservoir. This strategy has been used at certain sites, especially in China. The concept of sediment routing is summarized in the Chinese slogan “discharge the muddy water, impound the clear water” and this strategy has been practiced in many reservoirs in China including the Sanmenxia Dam on the Yellow River (Zhang et al. Citation1976, Chen et al. Citation2017). It has also been applied at the Three Gorges Reservoir (Wang et al. Citation2005, Wang and Chunhong Citation2009) and Loiza Reservoir in Puerto Rico (Morris and Fan Citation1998). For sluicing to be effective, the drawdown level of the reservoir should be below half of the dam height, and the sluice capacity should be more than the mean annual flow (Pitt and Thompson Citation1984). Reservoir drawdown is necessary to maximize flow velocity so that suspended sediment can pass through the impounded reach without deposition (Morris and Fan Citation1998). Also, enough surplus inflow must be available to discharge sediment, and the outlet should preferably be located near the original riverbed level (Basson and Rooseboom Citation1997).
Density current venting
Density currents occur when the density of the current is larger than that of the ambient fluid. As a result, the density current continues as an underflow and plunges into the deeper water (Bournet et al. Citation1999, Üneş et al. Citation2015). The intrusion of density currents into a reservoir could influence the sediment trap efficiency if the sediments are vented through a low-level outlet in the dam or where the sediments deposit within the reservoir.
Density currents can be vented from a reservoir by releasing water through a low-level outlet. This process can release more than half of the total sediment load in an individual flood. The effectiveness of this method depends on the appropriate location of the low-level outlets in the dam body and also the timely release of the density current as it reaches the dam face (Morris and Fan Citation1998). Fan and Morris (Citation1992) suggested that this method is well suited for large reservoirs with multiyear storage capacity where drawdown is unwanted.
Sediment bypass technique (SBT)
In this method, sediment is routed to the dam tail water with bypass tunnels during floods, and therefore reduces reservoir sedimentation (Auel and Boes Citation2012, Albayrak et al. Citation2013). SBT has been shown to be more effective than other methods. An advantage of SBT is that it has positive ecological effects because sediment conveyance may significantly decelerate or even stop riverbed erosion, and consequently increase the morphological variability downstream (Boes et al. Citation2014). SBT has been implemented at several reservoirs such as the Asahi Dam (Kataoka Citation2003, Sumi et al. Citation2004, Mitsuzumi et al. Citation2009) and Miwa Dam (Suzuki Citation2009, Sumi et al. Citation2012).
Results and discussion
In this study, both engineering (free and pressure flushing, sluicing, density current venting, and sediment bypass) and non-engineering (watershed management) methods were assessed for sedimentation control in Dez Reservoir.
Watershed management method
Geographic Information System (GIS) and the MPSIAC model (calibrated with survey data) were used to estimate the sediment yield in Dez Reservoir (). Based on the sediment yield map, almost 35% of the basin was identified as erodible area with high sediment production. Also, Emamgholizadeh and Samadi (Citation2008) indicated that 55% of the total inflow sediment discharge in Dez Reservoir comes from the Bakhtiary tributary, and the remaining 45% is brought in by the Sezar River. The Bakhtiary subcatchment is an uninhabited mountainous area with no rural population and no access roads, which makes it difficult to implement sediment management measures. Thus, most studies on erosion reduction measures such as sediment trapping, check dams, and reforestation have focused on the northwest portion of the Dez watershed (i.e., the Sezar River subcatchment). According to Dezab and Acres (Citation2005), approximately 15% of the annual sediment yield of the watershed can be controlled by watershed management measures. Sediment management measures, however, would not lead to an immediate reduction of inflow sediment discharge because the construction of check dams, roads, and forest planting would take several years.
In general, implementing a watershed management program for Dez basin would be time consuming and costly, and therefore this strategy may not the best solution for the sediment problems in Dez Reservoir. Khuzestan Water and Power Authority (KWPA) studied the construction of a dam on the Bakhtiary River. The construction of this dam was begun in 2009 and is scheduled to be completed by 2018. It is a double curvature concrete arch dam with height of 315 m and storage capacity of 4845 × 106 m3. This dam is located 75 km upstream of Dez Dam, and will likely trap 55% of the annual sediment load and it will reduce the sedimentation problem of Dez Reservoir.
Hydraulic flushing method
The accumulation of sediment in the Dez Reservoir has reduced its storage capacity and is threatening to reach a level where it will enter the power intakes (). According to the hydrography study carried out in 1983, the sediment level was at 220 m, which was only 2.7 m under the centerline of irrigation outlets. The hydrography study in 1997 showed that the sediment level had increased to 247.5 m, which is 24.8 m above the centerline of irrigation outlets. Dez Dam was not equipped with bottom outlets for the purpose of sediment release. To initiate flushing techniques, 3 irrigation outlets could be used, which are located in the body of the dam. For this dam, the free flow flushing is not feasible, mainly because of its key role in power generation and frequency control of the national power network. For the first time, in 1994, it was decided to utilize the irrigation outlets as sluiceways to release the accumulated sediment (). The irrigation outlets decreased the sediment elevation to 3 m below the centerline of the irrigation outlets. They were open for 65 d over the flood season. The irrigation outlets were used for flushing accumulated sediment again on 7 February 1995, 7 and 24 May 1997, 14 April 1998, 13 March 2000, and 18 June 2003.
Unfortunately, the sediment concentration released from outlets was not measured, except during the pressure flushing operation in 2000 (), when it was measured hourly. As can be seen, the maximum sediment concentration was about 1128 g/L, which is approximately equal to the specific weight of sediment. After 36 h of pressure flushing, the outflow sediment concentration was reduced to 2 kg/m3, and after 56 h, the outflow sediment concentration was 0 g/L. Also, according to these sediment concentration measurements, about 0.9 × 106 m3 of the deposited sediment was removed from the reservoir during the pressure flushing operation in 2000. In early June 2003, the result of sediment level monitoring near the dam showed that sediments had previously accumulated to an elevation of 256 m (30 m above the centerline of the irrigation outlets). Therefore, the Dez Dam authorities decided to once again implement the pressure flushing technique on 17 June 2003 via the irrigation outlets. This time, after about 4 h of flushing, the outflow discharge from the irrigation outlet was clear and a flushing cone had developed in the vicinity of the dam wall.
According to the bathymetric survey, the radius of the flushing half-cone was nearly 250 m after it reached equilibrium (). The bathymetric map was used to assess the scour cone geometry. Based on the longitudinal profile, the radius of flushing half-cone was nearly 250 m. Also, the volume of the flushing half-cone (i.e., the volume of the sediment discharge during the pressure flushing operation) was approximately 0.3 × 106 m3. At the distance of 10 m from the dam, the bed had a steep slope of 45%. From 10 to 100 m, the bed slope became milder and reduced to about 12%. At the distance farther than 100 m from the dam, the bed slope decreased to less than 6%. Field measurements in several reservoirs, namely Bikou, Kongazhue, Fen He, Yan Gou Gorge, and Qington Gorge in China showed that the scour cone had a slope of 7–30.6% (Fang and Cao (Citation1996).
Figure 9. The longitudinal bed profile of the reservoir centerline after flushing in 2003. Adapted from Emamgholizadeh and Samadi (Citation2008).
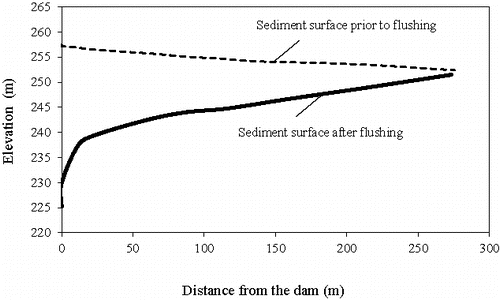
Flushing efficiency (E) is defined as the ratio of volume of the scoured sediment (Vs) to the volume of water flowing during flushing (Vw) (i.e., as E = Vs/Vw; Qian Citation1982). The flushing efficiency in Dez Reservoir was equal to 0.00890 and 0.00908 in the years 2000 and 2003, respectively. Morris and Fan (Citation1998) reported that the flushing efficiencies of Guernsey (USA), Warsak (Pakistan), Liujiaxia (China), and Shuicaozi (China) reservoirs vary from 0.00017 to 0.012. It is evident that the flushing efficiency in Dez Reservoir falls within the range reported by Morris and Fan (Citation1998).
Flushing of the accumulated fine sediments from reservoirs and releasing them into downstream rivers may damage downstream aquatic communities (Wohl and Cenderelli Citation2000). Hence, flushing time is important both economically and environmentally (Brandt Citation2000). According to Morris and Fan (Citation1998), flushing flows should be scheduled by taking into account factors such as the life history of important species in the river, the natural flow variation before impoundment, and water availability. For example, properly timed flushing flows can cleanse gravels in preparation for spawning, but a late release may wash out eggs. Kereselidze et al. (Citation1985) suggested that flushing should be done immediately before fish spawn. Mikhalev (Citation1971) stated that time of flushing should be before the beginning of the yearly flood. In the Sefidrood Reservoir in Iran, flushing is done at the end of the irrigation season (from mid-Sep to Oct) because it is the period of minimum water demand.
With regard to the above-mentioned studies, the best time for flushing at the Dez Reservoir should be determined by considering downstream conditions. Dezful Dam was constructed 23 km downstream of Dez Dam. This dam (with a height of 20 m) regulates the water that is released from Dez Dam. According to the hydrography, after 30 yr of operation, deposited sediment has reduced its storage volume by more than 40% (Emamgholizadeh Citation2010). Therefore, flushing in Dez Reservoir causes increased sedimentation in the Dezful Reservoir (see ), a loss of reservoir water storage, a threat of flooding downstream, and potential negative environmental impacts downstream. Samadi-Boroujeni et al. (Citation2010) stated that choosing the time of flushing must be done with respect to the downstream conditions such as the environmental and hydraulic issues that have been mentioned. Based on their findings, the irrigation gates should be opened for 6 h with a discharge of 30 m3/s. Also, the period between 2 flushing operations should be at least 6 h.
In pressure flushing, when the water level in the reservoir decreases, the flow velocity and bed shear stress increase. Therefore, the efficiency of this method increases and it produces a higher flushing rate and a larger cone (Fathi-Moghadam et al. Citation2010). The water level in Dez Reservoir reaches its minimum value of 317 m in June and 319 m in July (). In June and July, the inflow discharge is equal to 282 m3/s and 157 m3/s, respectively (). They are not flood months and even the inflow discharge in July is less than the mean annual inflow discharge of 271 m3/s. For more efficiency, it is recommended to implement pressure flushing in these 2 mo.
Sluicing
Sediment transport in many rivers in Iran, especially the Dez River, occurs mainly in the 4-mo flood season. In fact, 80–85% of the annual sediment load is transported by 55–62% of the annual runoff. A dam with sluice gates could potentially allow these flows with high sediment concentrations to pass through the reservoir and avoid much of the sediment deposition. As mentioned earlier, Dez Reservoir is used for power generation, flood control, and as a water supply for agriculture consumption. For these purposes, it is necessary to maintain adequate storage capacity in the reservoir, which may not be maintained if sluicing is implemented. Furthermore, Dez Dam does not have sluice gates. Given these limitations, sluicing would not be an effective method for Dez Reservoir.
Density current venting
Field measurements of density currents were carried out at 7 locations from December 2002 until June 2003 (). Governing variables such as temperature, density, depth (water pressure sensor RCM9), water velocity (Vale-port current device), and suspended sediment (vertical tube sampler) were measured. The accuracy of water velocity, temperature, density, and depth measurements were ±1% of measured velocity, ±0.05 C, ±2% of measured density, and ±1% of measured depth, respectively. The main objectives were to determine (1) the geometry of the density current (its thickness and width) as it travels through the lower part of the reservoir, and (2) the rate of suspended sediment conveyed to the dam wall, which can be calculated by multiplying the cross-section area by the velocity and sediment concentration. The measurements of suspended sediment concentration and the velocity at the cross-section A–A showed that the first density current occurred on 28 and 29 January 2003. The sediment concentration near the bed was almost 2 kg/m3, which reduced to 0.2 kg/m3 at about one-third of the depth. The thickness of the density current was nearly 15 m. The second turbidity current occurred on 23 and 24 April 2003. The sediment concentration near the bed was almost 1.5 kg/m3 and 4.5 kg/m3, and the thickness of the density current was about 20 m and 10 m on 23 and 24 April, respectively.
Figure 11. Daily discharge and suspended sediment concentration at Talezang hydrometric station in Dez River.
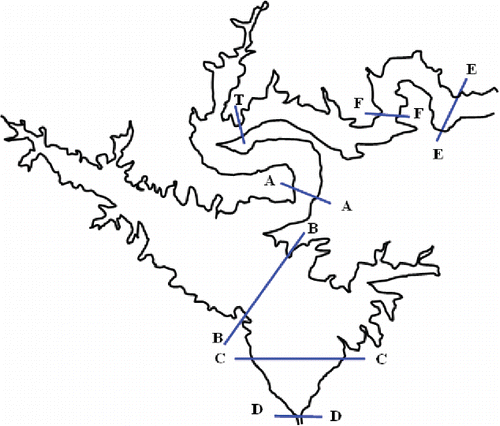
Occurrence of density currents in a reservoir depends on the concentration of the suspended sediments carried by the inflow discharge to the reservoir. Comparing the daily discharge with suspended sediment concentrations at Talezang hydrometric station in Dez River shows that the large suspended concentration within the density current occurs mostly at the high inflow time (). When the suspended sediment concentration is high at Talezang hydrometric station, it is expected to have density currents at the Dez Reservoir. Both discharge and sediment concentration decrease from 3 May 2003 to the end of the period (). According to the historical records from 1976 to 2014, on average the highest inflows of 477, 676, and 547 m3/s occur respectively in March, April, and May (). The water level in the reservoir and the average inflows are also shown in the same figure. When density currents in Dez Reservoir are released during the flood season, although some sediment will still deposit within the reservoir and the storage capacity will still reduce over time, it will occur at a much slower rate. The minimum and maximum water levels of operation in Dez Reservoir are 300 m and 350 m, respectively. Density currents are impounded every year, particularly during hyper-concentrated floods. It would be possible to discharge these density currents through the irrigation gates. The benefit in sediment reduction from this method would depend on the sediment concentration, inflowing discharge, and the fraction of sediment particles finer than 0.01 mm (Wang and Chunhong Citation2009). In this study, we used the field measurements of the density currents in the Dez Reservoir from 2002 to 2003. As mentioned previously, the field measurements of density currents in Dez Reservoir showed that 2 density currents reached the dam. The measured discharge (Q) and suspended sediment concentration (S) at Talezang hydrometric station were used to find the relationship between Q and S (). As shown, when S × Q ≥ 4057, density currents occur at Dez Reservoir. In other words, for high discharge and sediment concentration (points above the curve in ), the density currents flowed through the reservoir and reached the dam. But for lower discharge and sediment concentration (points placed below the curve), the density current was weak and did not reach the dam.
Figure 12. Daily discharge and suspended sediment concentration at Talezang hydrometric station in Dez River (labels of a and b show the first and second density currents).
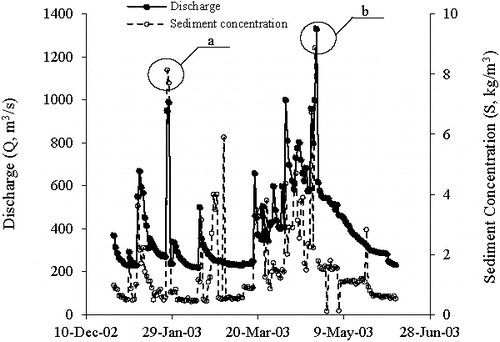
Figure 13. Relationship between the measured discharge (Q, m3/s) and suspended sediment concentration (S, kg/m3) (labels of a and b show the first and second density currents).
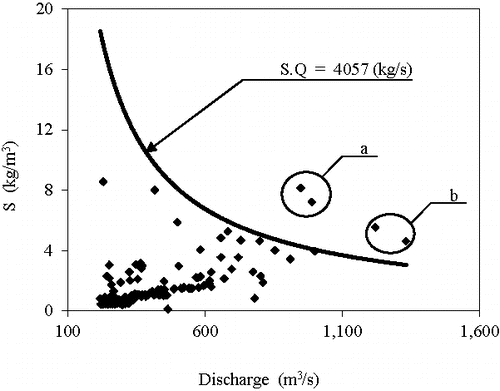
Based on the field measurements, 11–15% of the annual sediments that inflow into the Dez Reservoir are due to density currents. These currents travel through the lower reservoir downstream of the delta, reach the dam, and deposit sediments. Thus, opening an outlet and releasing these density currents could prevent some of the sedimentation (Dezab and Acres Citation2005). By the density current venting approach, it is possible to release nearly 1.74 × 106 m3 to 2.37 × 106 m3 of sediments of Dez Reservoir. In Dez Reservoir, the sediment releasing efficiency of the density current venting approach is about 21%. This finding is consistent with the Wang (Citation2015) and Wang and Chunhong (Citation2009) studies. Wang (Citation2015) stated that the sediment releasing efficiency for density current venting varies typically from 6% to 36%. Also, according to Wang and Chunhong (Citation2009), the efficiency of density current venting in Xiaolangdi Reservoir is about 20%.
Sediment bypass technique
Emamgholizadeh and Samadi (Citation2008) showed that the sediment bypass technique can be used in the Dez Reservoir to divert a part of the incoming sediment-laden waters. They proposed to construct a submerged dam with height of 57 m and a diversion tunnel with length of 15 km and diameter of 8.28 m upstream of Dez Dam (). This method would flush deposited sediments from the delta region and discharge turbid flow during the flood season. Sediment would be eroded and flushed through the bypass tunnel by a tractive force of the water, and carried out to the Andimeshk region. Performing a flush once per year, combined with a bypass tunnel discharging more than 307 m3/s, make it possible to flush 9.3 × 106 m3 of deposited sediment per year. If flushing is done when the turbidity currents occur (which is 2–4 times per year), it would be possible to remove 1.5 × 106 m3 of sediment from the reservoir. If the sediment bypass method is done at the proper time, it has a high efficiency (Morris and Fan Citation1998, Sumi et al. Citation2012). For example, in Miwa Reservoir, the efficiency of the sediment bypass method was up to 80% of the total inflow sediment (Kantoush et al. Citation2011). The efficiency of the sediment bypass technique method is high during the flood season (Kantoush et al. Citation2011).
Therefore, in Dez Reservoir, the best time for implementing sediment bypass would be March, April, and May in which inflow to the reservoir is high (). Although this technique is expensive due to the construction costs of a tunnel, it has less environmental impacts downstream because the sediment would be discharged to the Andimeshk region. The Andimeshk region was chosen because it is a non-agricultural plain with sandy soil. By transferring flushed sediment to the Andimeshk plain, it is possible to reclaim it without environmental problems.
Conclusions
Reservoir sedimentation is the main issue for most of the reservoirs around the world. Dez Reservoir, one of the largest reservoirs in Iran, suffers from sedimentation problems. The main reasons for sedimentation in this reservoir (and also similar reservoirs, which are located in arid and semi-arid areas) are scarce vegetation cover, steep topography, and intense rainfall. In Dez Reservoir, the sedimentation issue could be controlled by applying different strategies, including watershed management, sluicing, releasing density currents, free and pressure flushing, and sediment bypass. Sedimentation could be reduced by 11–15% with density current venting, and by 2% with pressure flushing. Free flushing and sluicing are not recommended due to (1) their negative effects on the downstream ecosystem, and (2) lack of low-level outlets in Dez Dam. The pressure flushing method for removing sediments can be localized, and thus it is the recommended method when there is a need for localized removal of deposited sediments such as sediments around a water intake inlet. To use the hydraulic flushing, sluicing, and density current venting methods efficiently, the bottom outlets should be designed with sufficient capacity.
The watershed of Dez Reservoir is composed of 2 large watersheds, namely Sezar and Bakhtiary. Because the entire catchment area of Dez Reservoir is very large, watershed management techniques would be too costly and time prohibitive, if applied to the entire area. Watershed management strategies are recommended for the Sezar portion of the catchment area and not the Bakhtiary basin because of access considerations (the Bakhtiary subcatchment is an uninhabited mountainous area with no rural population and no access roads). Lastly, by using the sediment bypass technique, it possible to flush nearly 10.8 × 106 m3 of deposited sediment per year.
Cost of the above-mentioned methods is an important factor that should be taken into account when evaluating different approaches. Pressure flushing and density current venting need to use irrigation outlets at the Dez Dam. The valves in the irrigation outlets were installed more than 54 yr ago, and therefore it is risky to open them. Before using the pressure flushing and/or density current venting techniques, irrigation valves should be repaired or replaced. The cost of recovering 1 m3 of Dez Reservoir via the pressure flushing or density current venting methods is 3.6 USD. The watershed management method and sediment bypass techniques cost 9.4 USD/m3 and 15.6 USD/m3, respectively (Dezab and Acres Citation2005, Emamgholizadeh and Samadi Citation2008). Overall, combination of engineering (such as bypass sediment bypass technique, density current venting, and hydraulic flushing) and nonengineering (such as watershed management) approaches is an effective way to mitigate the sediment problems in Dez Reservoir.
This study suggests that policy makers take advantage of watershed management measures such as construction of check dams and roads as well as forest planting in the Dez watershed and other similar watersheds to reduce soil erosion.
Acknowledgments
We gratefully acknowledge the support and funding provided by the Shahrood University of Technology and the University of Hawaii at Manoa to conduct this study.
References
- Adam N, Erpicum S, Archambeau P, Pirotton M, Dewals B. 2015. Stochastic modelling of reservoir sedimentation in a semi-arid watershed. Water Resour Manage. 29(3):785–800.
- Aksoy H, Kavvas ML. 2005. A review of hillslope and watershed scale erosion and sediment transport models. Catena. 64(2):247–271.
- Alatorre L, Beguería S, García-Ruiz JM. 2010. Regional scale modeling of hillslope sediment delivery: a case study in the Barasona Reservoir watershed (Spain) using WATEM/SEDEM. J Hydrol. 391(1):109–123.
- Albayrak I, Auel C, Boes R. 2013. Supercritical flow in sediment bypass tunnels. Proc 12th Int Symposium on River Sedimentation. p. 1867–1875.
- Alighalehbabakhani F, Miller CJ, Baskaran M, Selegean JP, Barkach JH, Dahl T, Abkenar SMS. 2017. Forecasting the remaining reservoir capacity in the Laurentian Great Lakes watershed. J Hydrol. 555:926–937.
- Atkinson E. 1996. The feasibility of flushing sediment from reservoirs. Report OD 137, UK TDR Project R5839. HR Wallingford, Oxfordshire, UK.
- Auel C, Boes R. 2012. Sustainable reservoir management using sediment bypass tunnels. Proc 24th ICOLD Congress. p. 224–241.
- Azarang F, Bajestan MS. 2015. Simulating the erosion and sedimentation of Karun Alluvial River in the Region of Ahvaz (southwest of Iran). Am J Eng Res. 4:233–245.
- Basson G, Rooseboom A. 1997. Dealing with reservoir sedimentation. Guidelines and case studies. ICOLD – CIGB Bull. 115.
- Boes R, Auel C, Hagmann M, Albayrak I. 2014. Sediment bypass tunnels to mitigate reservoir sedimentation and restore sediment continuity. P. 221–228. In: Schleiss AJ, de Cesare G, Franca MJ, Pfister M (eds). Reservoir sedimentation.CRC Press: Boca Raton (FL).
- Bournet P, Dartus D, Tassin B, Vincon-Leite B. 1999. Numerical investigation of plunging density current. J Hydraul Eng. 125(6):584–594.
- Brandt A. 2000. A review of reservoir desiltation. Int J Sediment Res. 15(3):321–342.
- Brignoli ML, Espa P, Batalla RJ. 2017. Sediment transport below a small alpine reservoir desilted by controlled flushing: field assessment and one-dimensional numerical simulation. J Soils Sediments. 17:2187–2201.
- Bronstert A, de Araújo J-C, Batalla RJ, Costa AC, Delgado JM, Francke T, Foerster S, Guentner A, López-Tarazón JA, Mamede GL. 2014. Process-based modelling of erosion, sediment transport and reservoir siltation in mesoscale semi-arid catchments. J Soils Sediments. 14:2001–2018.
- Chaudhry MA, Habib-ur-Rehman M, Akhtar MN, Hashmi HN. 2014. Modeling sediment deposition and sediment flushing through reservoirs using 1-D numerical model. Arab J Sci Eng. 39(2):647–658.
- Chen J, Zhou W, Han S, Sun G. 2017. Influences of retrogressive erosion of reservoir on sedimentation of its downstream river channel – a case study on Sanmenxia Reservoir and the Lower Yellow River. Int J Sediment Res. 32(3):373–383.
- De Roo A, Offermans R, Cremers NL. 1996. A single-event physically-based hydrological and soil erosion model for drainage basins. II: sensitivity analysis, validation and application. Hydrol Process. 10:1119–1126.
- Dezab and Acres. 2005. Dez Dam rehabilitation project, Task 1, reservoir operation review & sediment study. Report to Khuzestan Water and Power Authority, Ahwaz, Iran.
- Di Silvio G. 1990. Modeling desiltation of reservoirs by bottom-outlet flushing. P. 159–171. In: Shen HW (ed). Movable bed physical models. Springer: Dordrecht (Netherlands).
- Emamgholizadeh S. 2010. The sedimentation effects of Dezful regular dam on the power generation of Dez Dam. Proceedings of International Symposium on Hydraulic Physical Modeling and Field Investigation, 13–15 September 2010, Nanjing, China.
- Emamgholizadeh S, Fathi-Moghdam M. 2014. Pressure flushing of cohesive sediment in large dam reservoirs. J Hydrol Eng. 19(4):674–681.
- Emamgholizadeh S, Samadi H. 2008. Desilting of deposited sediment at the upstream of the Dez Reservoir in Iran. J Appl Sci Env Sanit. 3:25–35.
- Fan J. 1985. Methods of preserving reservoir capacity. Editorial UNESCO. p. 65–164.
- Fan J, Morris GL. 1992. Reservoir sedimentation. I: delta and density current deposits. J Hydraul Eng. 118(3):354–369.
- Fang D, Cao S. 1996. An experimental study on scour funnel in front of a sediment flushing outlet of a reservoir. Proceedings of the 6th Federal Interagency Sedimentation Conference, Las Vegas (NV).
- Fathi-Moghadam M, Emamgholizadeh S, Bina M, Ghomeshi M. 2010. Physical modelling of pressure flushing for desilting of non-cohesive sediment. J Hydraul Res. 48(4):509–514.
- Hajiabadi R, Zarghami M. 2014. Multi-objective reservoir operation with sediment flushing; case study of Sefidrud Reservoir. Water Resour Manage. 28(15):5357–5376.
- [ICOLD] International Commission on Large Dams. 2013. General synthesis. http://www.icold-cigb.net/GB/world_register/general_synthesis.asp.
- Jones K, Berney O, Carr D, Barrett E. 1981. Arid zone hydrology for agricultural development. Rome, Italy: FAO Irrigation and Drainage.
- Kantoush S, Sumi T, Murasaki M. 2011. Evaluation of sediment bypass efficiency by flow field and sediment concentration monitoring techniques. J Japan Soc Civil Eng, Ser B1 (Hydraul Eng). 67(4): I_169–I_174.
- Kataoka K. 2003. Sedimentation management at Asahi Dam. The 3rd World Water Forum, Kyoto-Shiga, Japan. p. 197–207.
- Kereselidze N, Kutavaya V, Tsagareli YA. 1985. Silting and flushing mountain reservoirs, exemplified by the Rioni series of hydroelectric stations. Power Technol Eng. 19(9):514–520.
- Kondolf GM, Gao Y, Annandale GW, Morris GL, Jiang E, Zhang J, Cao Y, Carling P, Fu K, Guo Q. 2014. Sustainable sediment management in reservoirs and regulated rivers: experiences from five continents. Earth's Future. 2(5):256–280.
- Lai J-S, Chang F-J. 2001. Physical modeling of hydraulic desiltation in Tapu Reservoir. Int J Sediment Res. 16(3):363–379.
- Lal R. 2001. Soil degradation by erosion. Land Degrad Dev. 12(6):519–539.
- Lehner B, Liermann CR, Revenga C, Vörösmarty C, Fekete B, Crouzet P, Döll P, Endejan M, Frenken K, Magome J, et al. 2011. Global reservoir and dam (GRanD) database. Technical Documentation, Version. 1. Bonn, Germany: Global Water System Project.
- Meile T, Bretz N-V, Imboden B, Boillat J-L. 2014. Reservoir sedimentation management at Gebidem Dam (Switzerland). Proceedings of River Flow 2014, Special Session on Reservoir Sedimentation. Leiden, Netherlands: CRC Press/Balkema. p. 245–255.
- Mikhalev MA. 1971. Control of silting in reservoirs on mountain rivers. 14th Congress, IAHR. p. 1011–1014.
- Mitsuzumi A, Kato M, Omoto Y. 2009. Effect of sediment bypass system as a measure against long-term turbidity and sedimentation in dam reservoir. 23rd ICOLD Congress, Brasilia, Brazil.
- Molina-Navarro E, Martínez-Pérez S, Sastre-Merlín A, Bienes-Allas R. 2014. Catchment erosion and sediment delivery in a limno-reservoir basin using a simple methodology. Water Res Manage. 28(8):2129–2143.
- Morris GL, Fan J. 1998. Reservoir sedimentation handbook: design and management of dams, reservoirs, and watersheds for sustainable use. McGraw Hill: New York (NY).
- Pitt J, Thompson G. 1984. The impact of sediment on reservoir life, p. 541–548. In: DE Walling, SSD Foster, P Wurzel, (eds). Challenges in African hydrology and water resources, Harare Symposium, July 1984, IAHS-AISH.
- Qian N. 1982. Reservoir sedimentation and slope stability; technical and environmental effects. pp. 639–690. Fourteenth International Congress on Large Dams, Transactions, Rio de Janeiro, Brazil.
- Rashid MU, Shakir AS, Khan NM, Latif A, Qureshi MM. 2015. Optimization of multiple reservoirs operation with consideration to sediment evacuation. Water Resour Manage. 29(7):2429–2450.
- Reid I, Frostick L. 1987. Flow dynamics and suspended sediment properties in arid zone flash floods. Hydrol Process. 1(3):239–253.
- Samadi-Boroujeni H, Haghiabi A, Ardalan E. 2010. Determination of appropriate hydraulic conditions to decrease the negative impacts of Dez Dam flushing operation on the downstream. Int J Water Resour Env Eng. 2(1):1–8.
- Shuguang L, Yonglai Z, Zhengji Z, Congxian L. 2002. Sediment load of Asian rivers flowing into the oceans and their regional variation. Marine Sci Bull. 4(1):61–70.
- Shukla S, Jain SK, Kansal M, Chandniha SK. 2017. Assessment of sedimentation in Pong and Bhakra reservoirs in Himachal Pradesh, India, using geospatial technique. Rem Sens Appl: Soc Env. 8:148–156.
- Sumi T, Kantouch S, Suzuki S. 2012. Performance of Miwa Dam sediment bypass tunnel: evaluation of upstream and downstream state and bypassing efficiency. 24th ICOLD Congress, Kyoto, Japan. p. 576–596.
- Sumi T, Kantoush SA. 2010. Integrated management of reservoir sediment routing by flushing, replenishing, and bypassing sediments in Japanese river basins, p. 831–838. Proceedings of the 8th International Symposium on Ecohydraulics; 12–16 September 2010, Seoul, Korea.
- Sumi T, Okano M, Takata Y. 2004. Reservoir sedimentation management with bypass tunnels in Japan. p. 1036–1043. Proceedings of the Ninth International Symposium on River Sedimentation; 18–21 October 2004, Yichang, China.
- Suzuki M. 2009. Outline and effects of permanent sediment management measures for Miwa Dam. 23rd ICOLD Congress, Brasilia, Brazil.
- Tate EL, Farquharson FA. 2000. Simulating reservoir management under the threat of sedimentation: the case of Tarbela Dam on the river Indus. Water Res Manage. 14(3):191–208.
- Torabi-Pode H. 1999. Rate equations for estimating sediment of the rivers of Khuzestan. MS thesis. Shahid Chamran University: Ahvaz (Iran).
- Üneş F, Joksimovic D, Kisi O. 2015. Plunging flow depth estimation in a stratified dam reservoir using neuro-fuzzy technique. Water Resour Manage. 29(9):3055–3077.
- Walling DE, Webb B. 1983. Patterns of sediment yield. P. 69–100. In: Gregory KJ (ed). Background to palaeohydrology: a perspective. Wiley: New York (NY).
- Walling DE, Webb B. 1996. Erosion and sediment yield: global and regional perspectives. Proceedings of an International Symposium; 15–19 July 1996, Exeter, UK. IAHS Publication No. 236. IAHS Press: Wallingford, Oxfordshire (UK).
- Wang G, Wu B, Wang ZY. 2005. Sedimentation problems and management strategies of Sanmenxia Reservoir, Yellow River, China. Water Resour Res. 41(9).
- Wang ZY. 2015. Mitigation strategies of reservoir sedimentation in China. World Hydropower Congress; 19–21 May 2015, Beijing, China.
- Wang ZY, Chunhong H. 2009. Strategies for managing reservoir sedimentation. Int J Sediment Res. 24(4):369–384.
- White WR. 2000. Flushing of sediments from reservoirs. Thematic Review IV:5.
- White WR. 2001. Evacuation of sediments from reservoirs. Thomas Telford. London (UK).
- Wohl EE, Cenderelli DA. 2000. Sediment deposition and transport patterns following a reservoir sediment release. Water Resour Res. 36(1):319–333.
- Yoon Y. 1992. The state and the perspective of the direct sediment removal methods from reservoirs. Int J Sediment Res. 7(20):99–115.
- Zhang H, Xia M, Chen S, Li Z, Xia H, Jiang N, Lin B. 1976. Regulation of sediments in some medium and small-sized reservoirs on heavily silt-laden streams in China. Transactions 12th International Conference on Large Dams.