ABSTRACT
Slagle ZJ, Allen MS. 2018. Should we plant macrophytes? Restored habitat use by the fish community of Lake Apopka, Florida. Lake Reserv Manage. 00:00–00.
Freshwater resources are impaired worldwide, and managers frequently use habitat restoration to mitigate anthropogenic disturbances to freshwater systems. Restoration attempts have not often been evaluated with respect to their benefits to the sportfish population, especially in lentic systems. Recent restoration techniques at Lake Apopka, Florida, have included planting of macrophytes, particularly spatterdock (Nuphar advena), to increase fish habitat, stabilize sediments, and sequester nutrients. We assessed fish community habitat use of recently restored habitat with comparison to both unrestored (non-vegetated) and natural vegetated littoral habitats. We compared relative abundance and species richness of the fish community across habitat treatments. We electrofished >30 transects in each of these 3 habitat treatments (i.e., natural, planted, and unrestored), collecting relative abundance data for all fishes and habitat data including vegetation species and percent area coverage (PAC). Natural and planted habitat held greater PAC of vegetation than unrestored sites. Natural habitat supported the greatest relative abundance (as indexed by catch per unit effort) and the greatest species richness of fishes, while planted habitats and unrestored habitats supported fewer relative numbers and lower richness. Planted habitats are being utilized by the fish community, but were not significantly different from unrestored sites. Increased diversity of macrophytes in planted habitats could increase the benefits for these populations. Managers should consider continued monitoring and evaluation of planted sites to increase our understanding of the benefits of habitat restoration.
Aquatic habitat restoration is growing in importance as the scientific community recognizes the impacts of anthropogenic habitat degradation. Impaired lake ecosystems are common across the globe due to factors such as excessive nutrient inputs, acidification, and water level stabilization (NRC Citation1992, Cooke et al. Citation2005). Habitat restoration efforts generally seek to reestablish important habitats, processes, and communities that have been negatively impacted by eutrophication and other threats. Often, lake managers seek to restore benefits from recreational freshwater fisheries (Lee and Jones Citation1991). These efforts require evaluation to inform future work, increase the beneficial effects from restoration projects, and wisely spend limited agency funding.
Many previous evaluations of fishery responses to habitat restoration have focused on lotic environments and salmonid habitat (reviewed by Roni et al. Citation2008; e.g., Mouton et al. Citation2012, Thomas et al. Citation2015, van Zyll de Jong and Cowx Citation2016), while fewer restoration projects on lentic systems have been evaluated. In Florida, some restoration monitoring efforts have lacked sufficient data to assess whether the restoration was successful (Dierberg et al. Citation1988, Dierberg and Williams Citation1989). Other researchers in Florida have evaluated fishery responses to drawdowns, dredging, macrophyte removal, and herbicide treatments (Colle and Shireman Citation1980, Moyer et al. Citation1995, Allen et al. Citation2003, Bonvechio and Bonvechio Citation2006, Jordan and Arrington Citation2014, Dotson et al. Citation2015), while macrophyte planting as a restoration technique has not been previously assessed. However, the Florida Fish and Wildlife Conservation Commission (FWC) has planted macrophytes in at least 13 lakes beginning in the 1980s (Dotson et al. Citation2015). Managers may plant macrophytes with the expectation that planting will increase fish habitat or population abundance, but these effects are unknown.
Macrophytes provide numerous benefits for aquatic ecosystems, and reduced macrophyte coverage reduces these benefits. Plants provide habitat for fish, invertebrates, and zooplankton (Savino and Stein Citation1982, Carpenter and Lodge Citation1986, Dibble et al. Citation1996, Petr Citation2000). Macrophyte beds act as wave breaks, limiting sediment resuspension and increasing water clarity (Kristensen et al. Citation1992, Gasith and Hoyer Citation1998, Burks et al. Citation2002, Van Donk and Van de Bund Citation2002). Macrophytes also sequester nutrients, thereby limiting phytoplankton growth (Blindow Citation1992, Van Donk and Van de Bund Citation2002, Phillips et al. Citation2016). Macrophytes can also improve aesthetics, reduce nuisance plant and algae growth, and generally moderate environmental disturbance (NRC Citation1992, Cooke et al. Citation2005).
In areas where macrophytes are reduced in abundance or eliminated entirely, managers may choose to plant macrophytes. However, evaluation of macrophyte planting restoration efforts is rare, and very few references in the literature exist (Cooke et al. Citation2005, Strakosh et al. Citation2009)—especially in regard to the fish community use of restored habitat in comparison with natural and unrestored habitats. Managers also do not always consider what types of habitat different species of macrophytes provide; macrophyte species differ in structure (e.g., emergent vs. submerged, size of interstitial spaces) and therefore provide different benefits for different species and sizes of fishes (Gotceitas and Colgan Citation1990, Killgore et al. Citation1993, Stahr and Shoup Citation2015, Kuehne et al. Citation2016).
We assessed fish community habitat use of recent habitat restoration efforts with comparison to both unrestored and natural littoral habitats in a large degraded lake in Florida. We compared relative abundance and species richness across habitat treatments. We tested the hypothesis that planted habitats would support richer communities and greater numbers of fish than unrestored habitats, but expected naturally vegetated habitats to be most diverse and hold the most fish.
Methods
Study site
Lake Apopka is one of the major impaired water bodies in Florida. The lake is one of the largest in Florida at greater than 12,000 ha and is fed by a natural spring, rainfall, and storm water runoff (). During the early 1900s, Lake Apopka was a premier fishing destination (Schneider and Little Citation1973, Reddy and Graetz Citation1987, Schelske and Brezonik Citation1992). Anthropogenic nutrient additions into the lake, including from citrus groves, muck farms, and the Winter Garden sewage treatment plant, increased during the same time period (Clugston Citation1963, Schneider and Little Citation1973, Schelske et al. Citation2005). These increased nutrient discharges combined with alterations to water flow led to decreased water quality, greatly decreased macrophyte coverage, declining recreational fisheries, and the creation of thick muck layers that cover the bottom of the lake (Schneider and Little Citation1973, Reddy and Graetz Citation1987, Schelske and Brezonik Citation1992). These problems were first documented in 1947, and various restoration efforts began in the late 1970s (Schneider and Little Citation1973, Schelske et al. Citation2005).
Figure 1. Map of Lake Apopka, with locations of each transect origin. Natural sites were primarily located near Gourd Neck Springs, where most of the sand bottom in the lake is found.
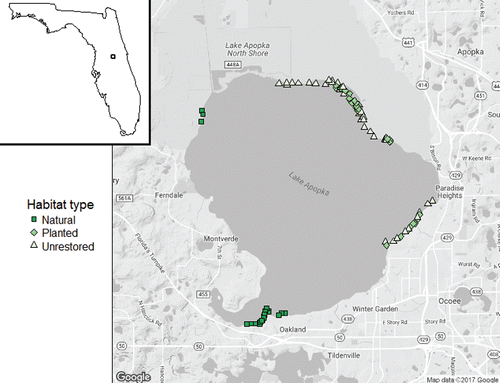
A range of management strategies has been attempted to improve water quality and fish habitat in Lake Apopka including a marsh flow-way (Lowe et al. 1992, Coveney et al. Citation2002), macrophyte planting (Hoge et al. Citation2003), removal of undesirable fish (Schaus et al. Citation2010), and reduction of external nutrient inputs (Coveney et al. Citation2005). Results of current in-lake methods to improve water quality are thus far ambiguous; researchers are divided as to whether the current management strategies are reducing muck and improving water quality (Bachmann et al. Citation1999, Canfield et al. Citation2000, Lowe et al. Citation2001, Coveney et al. Citation2005, Hoyer et al. Citation2012). Macrophyte planting in Apopka has taken place since 1991 (most recently in 2012–2014) with the objectives of expanding fish and wildlife habitat and improving water quality (Hoge et al. Citation2003; Jaggers B, FWC, biologist, Jul 2015, pers. comm.). These plantings have shown survival and expansion in some cases in Lake Apopka (Coveney Citation2016), but it is unknown whether benefits to fish communities have occurred.
Site classification
We identified 3 different habitat treatments (i.e., natural, planted, or unrestored) based on several criteria (). Natural sites represented bottom substrate and plant species that typify the makeup of the littoral zone prior to the degradation of the lake; these species include giant bulrush (Schoenoplectus californicus), maidencane (Panicum hemitomon), and eelgrass (Vallisneria americana; Clugston Citation1963, Schneider and Little Citation1973). Planted sites were areas where the FWC had planted rows of spatterdock (Nuphar advena), identified by PVC pipes marking the beginning and end of each planted row and by visual inspection with FWC agents (Jaggers B, FWC, biologist, Jul 2015, pers. comm.). Unrestored sites substrate consisted primarily of organic detritus and were often unvegetated or were sparsely vegetated by cattail (Typha spp.; Hoge et al. Citation2003).
Figure 2. Examples of the 3 different habitat types sampled in this study, including (a) unrestored, (b) planted, and (c) natural. Unrestored sites either lacked vegetation or were dominated by cattail, while spatterdock dominated planted site vegetation. Natural site macrophytes were diverse and included giant bulrush, maidencane, and eelgrass.
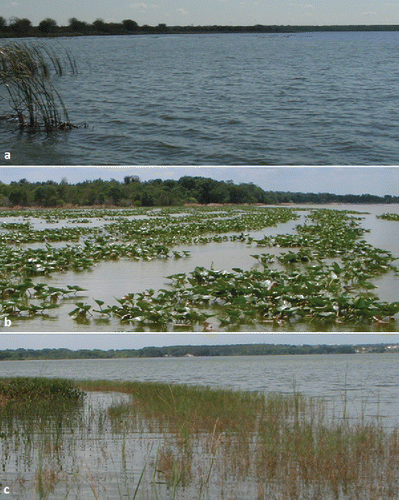
We classified natural sites using a combination of sediment and flora. We sampled sediment using a transparent PVC pipe attached to a one-way valve that allows pressure to bleed off and a vertical sample of the substrate to be extracted (Davis and Steinman Citation1998). As we retrieved the sample, we inserted a rubber stopper into the bottom of the transparent PVC pipe (prior to removal from the water) to prevent substrate from escaping. In these samples, we identified the substrate as either sand (indicating a hard bottom similar to the original lake bottom) or muck and detritus (which makes up the majority of the lake bottom and limits a variety of plant life). We classified sites with sand as the dominant substrate as natural sites, while other substrates were classified as either planted or unrestored. We only classified a site as natural if the site had both sand bottom and the presence of at least one of three natural macrophyte species (maidencane, giant bulrush, or eelgrass) in the immediate vicinity (i.e., within ∼10 m).
Field sampling
We used timed boat electrofishing to sample the 3 different habitats. We conducted approximately 10 electrofishing samples per treatment in 3 seasons (fall 2015, winter 2015–2016, and spring 2016) for at least 30 samples per treatment. At each site, we electrofished using a boat-mounted electrofishing unit (GPP 9.0; Smith-Root, Inc., Vancouver, WA) with 2 boom probes and 2 netters. We electrofished for 300s of actual shock time for each sample, allowing the netters to occasionally pulse the current on and off to more effectively capture fish. We determined proper voltage, pulse width, and rate, and adjusted according to local lake conditions, targeting 4.0 amps. We identified all fish to species; we measured sportfish (e.g., Florida bass [Micropterus floridanus], bluegill [Lepomis macrochirus], and black crappie [Pomoxis nigromaculatus]) for total length (TL) and enumerated all other fishes.
We collected habitat samples at the beginning and end of each transect to serve as habitat covariates. We estimated the coverage and composition of aquatic macrophytes by throwing a 0.25 m2 PVC quadrat in 3 haphazard locations adjacent to the boat: once off the bow and once off each side. We then identified the top 3 macrophyte species in each quadrat based on coverage according to Hoyer et al. (Citation1996). We also visually estimated the total percent area coverage (PAC) in each quadrat. We followed these procedures at the beginning and end of each transect, for 6 quadrat samples per transect. We also visually estimated the area coverage of aquatic vegetation across the entire transect by averaging 3 or 4 field crew members' individual estimates of PAC to the approximate width of the electrofishing field. We also took photos at the beginning and end of each transect for reference, each looking toward the transect.
Analysis
We used generalized linear models (GLMs) to assess the relative abundance of fish species and the PAC of macrophytes. We assumed that catch per unit effort (CPUE) and PAC were distributed following a compound Poisson–gamma distribution (i.e., Tweedie distribution; Tweedie Citation1984). We chose this approach because the high abundance of zero counts in our data precluded the use of parametric statistics (i.e., the data were not normally distributed). The Tweedie distribution has the advantage of incorporating zero and nonzero data into a single model framework, and has application to fisheries CPUE data with many zero counts (Candy Citation2004, Shono Citation2008). We estimated the Tweedie index parameter p for each model using Fourier inversion (Dunn and Smyth Citation2008). We modeled total fish relative abundance and species relative abundance with GLMs; we compared the fit of each with a null model and likelihood ratio tests (LRTs; Anderson Citation2008, Bolker Citation2008). We then tested for significant differences between habitats using Tukey's range tests for each model (Tukey Citation1949). We also followed these same methods to model macrophyte metrics (e.g., transect PAC, mean quadrat PAC, and Simpson's index). We used Program R (R Core Team Citation2014) and the R package cplm (Compound Poisson Linear Models) for analyses (Zhang Citation2013).
We used Simpson's index and species richness estimators (Chao1 and Jackknife1) to compare diversity of fishes and macrophytes across the 3 habitat treatments. Simpson's index (D) is a commonly used measure of diversity that emphasizes dominance of a species within an assemblage (Simpson Citation1949, Magurran Citation2004). Simpson's index is reported as [−ln(D)] following Rosenzweig (Citation1995) and represents the likelihood that 2 random individuals are the same species. A sample with a higher Simpson's has greater diversity (less dominated by one species) than a sample with a lower Simpson's index. We additionally used the Chao1 estimator (Chao Citation1987) to estimate the minimum species richness by habitat using our fish abundance data; this reduces bias compared to observed species richness by additionally estimating undetected species (Shen et al. Citation2003, Chao et al. Citation2009, Chiu et al. Citation2014). We used the Jackknife1 estimator of species richness for macrophytes, as it only requires presence/absence data (Heltshe and Forrester Citation1983, Magurran Citation2004). We used the R package vegan (Oksanen Citation2013) for diversity analyses.
Results
The 3 habitat treatments differed substantially in macrophyte cover and composition. In randomly placed quadrat throws, we only observed spatterdock in planted habitats and cattail in unrestored habitats (with 2 exceptions: one observation of frogs bit [Limnobium spongia] in a planted sample and one in an unrestored sample). We observed 8 species in natural habitats, including giant bulrush, eelgrass, maidencane, pickerelweed (Pontederia cordata), Egyptian paspalidium (Paspalidium geminatum), and cattail. GLMs for transect PAC, quadrat PAC, and Simpson's index were highly significant (LRTs; P < 0.01) compared to null models (i.e., plant coverage or diversity does not vary by habitat treatment). Natural habitats also had a greater mean Simpson's index, although natural and planted habitats were only marginally significantly different from each other (Tukey's range test; P = 0.054; ). Planted and natural habitats had similar PAC and Simpson's index and were significantly different from unrestored habitats based on Tukey's range tests for transect PAC and quadrat PAC (P < 0.01; ). Macrophyte species richness (Jackknife1 estimator ± SE) was greatest in natural habitats (8.97 ± 0.97) and similar across planted and unrestored habitats (2.97 ± 0.97 for both).
Figure 3. Mean of estimated plant coverage (PAC) in transect samples, quadrat samples (top panel), and Simpson's index (bottom panel) for macrophyte species encountered in random quadrat throws by habitat generated by a generalized linear model with the Tweedie distribution (Zhang Citation2013). Error bars depict the 95% confidence interval from the GLMs and different letters indicate significant differences between groups based on Tukey's range tests.
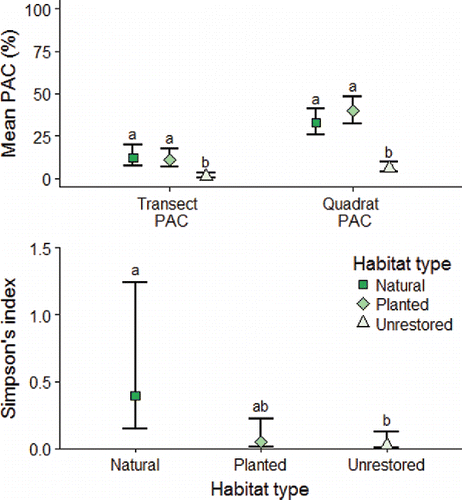
Natural habitat samples had greater relative numbers of fish compared to planted and unrestored habitat samples (). The median catch per unit effort (CPUE ± coefficient of variation, CV) for all fishes in natural habitats was 66.1 (±1.4) fish per hour, compared to 14.3 (±1.2) fish per hour in planted habitats and 8.1 (±1.3) fish per hour in unrestored habitats (). Additionally, natural habitats held much greater relative numbers of each sportfish compared to the other habitats (). These trends held true for the GLMs as well (). All models were highly significant compared to null models using likelihood ratio tests (P < 0.01). Mean fish-per-hour for all species (from GLMs) was significantly greater in natural habitat samples, while planted and unrestored habitats had similar relative abundances (). Mean relative abundance of each sportfish was greatest in natural habitats and lowest in both restored and unrestored habitats according to the individual species GLMs and Tukey's range tests.
Table 1. Median (±CV) fish caught per hour by habitat treatment and species. We failed to catch sportfish in many samples across habitats, but tended to catch more fish per hour in the natural treatment samples.
Table 2. Mean (±95% C.I.) fish caught per hour estimated from generalized linear models by habitat treatment and species. Each habitat model was significant compared to a null model (CPUE being equal among all treatments) according to likelihood ratio tests.
Figure 4. Estimated mean relative abundance (CPUE) of all fish, bluegill, Florida bass, and black crappie divided by habitat treatment, generated by a generalized linear model with the Tweedie distribution (Zhang Citation2013). Error bars depict the 95% confidence interval from the GLMs and different letters indicate significant differences between groups based on Tukey's range tests.
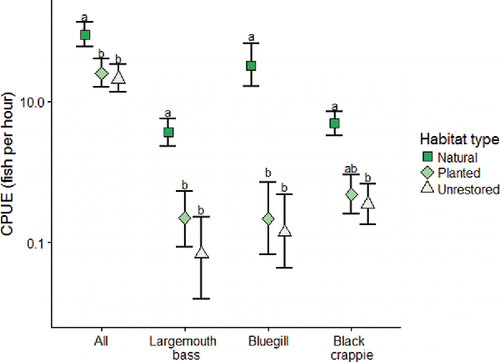
Fish species richness was greatest in natural habitats, lower in planted habitats, and lowest in unrestored habitats, although species diversity did not follow the same trends. Mean (±SE) estimated minimum species richness (Chao1 estimator) was 25.6 (±3.4) species for natural habitats, 22.6 (±3.4) in planted habitats, and 17.8 (±1.8) in unrestored habitats (). Observed species richness followed these trends as well, but sample sizes (in this case, numbers of fish in each habitat) were vastly different and observed species richness is therefore biased. Simpson's indices for fish were similar across all habitats (), although unrestored habitats were slightly higher.
Table 3. Observed species richness, estimated minimum richness (Chao1 estimator), and estimated diversity (Simpson's index) for fish samples by habitat type. Species richness generally increased from unrestored to natural habitats, while diversity was slightly greater in unrestored habitats.
Discussion
We found natural habitats in Lake Apopka to contain the greatest relative abundance of fish and the greatest richness, while planted (i.e., restored) habitats and unrestored habitats generally held lower abundances and diversities. We also found that natural habitats were more diverse in flora. Our results were similar to Johnson and Crumpton (Citation1998), who found greater biomass of fishes in “grass” and “bulrush” sites compared to “cattail” or “no vegetation” sites in Lake Apopka. Other studies have found greater diversity and abundance of fish in systems with a diverse macrophyte community compared to cattail-dominated systems (Gifford Citation2002). Cattail-dominated systems are additionally associated with decreased macroinvertebrate biomass and density compared to diverse communities (Schummer et al. Citation2012, Lawrence et al. Citation2016).
Differences in catchability between habitats could have affected our relative abundances, but our findings are robust to this possibility. Catchability (i.e., the proportion of fish caught out of the population in a specific area) differs across macrophyte coverages (Bayley and Austen Citation2002), and unrestored habitats had both lower fish abundance and lower macrophyte coverage compared to the other sampled habitats ( and ). However, catchability of Florida bass and bluegill was actually greater in transects of lower macrophyte coverage (Bayley and Austen Citation2002), and we would expect to more easily catch these species in more open environments such as the unrestored habitat samples. Catchability of bluegill and Florida bass in Florida is relatively constant across a range of vegetation densities and seasons (Hangsleben et al. Citation2013), and fish abundance is typically greater in vegetated areas of lakes (Dibble et al. Citation1996). We attempted to control for catchability by using identical methods across all 3 habitats (e.g., 2 netters, similar speed and shock intensity, similar transect depths). We also sampled across 3 seasons, sampling within a short time period within seasons. We therefore believe that CPUE was a valid index of relative abundance in our study.
This study also suffers from spatial overlap and isolation across different treatments. Natural site samples were by necessity taken along the south shore of Lake Apopka (), the only area of the lake that consistently supports diverse flora; unrestored and restored samples were taken on the east and northeast shores of the lake, where restoration efforts were focused. We cannot say conclusively that differences in macrophytes are the sole driver of fish community richness and abundance, because natural sites differ in other physiochemical and biological properties that could affect the fish community (e.g., sand substrate, vs. muck and detritus for both unrestored and restored sites). Macrophytes' interactions with the water column create differences in dissolved oxygen, pH, and temperature from surrounding unvegetated areas (Killgore et al. Citation1993, Petr Citation2000). Fish respond to these changes differently depending on plant density and fish density (Miranda and Hodges Citation2000, Dunham et al. Citation2002, Wilson et al. Citation2015). We did not monitor water quality parameters across treatments. We observed broadly similar water depths across all 3 treatments, but we did not measure water depths in this study. Macrophyte habitat is one of many factors that drive fish habitat selection. Future studies should measure water quality parameters and other habitat characteristics to better elucidate differences between natural, restored, and unrestored sites.
Habitat may be limiting in Lake Apopka for the Florida bass population. Low levels of aquatic macrophytes may limit sportfish populations in larger lakes (Wiley et al. Citation1984, Hoyer and Canfield Citation1996a, Citation1996b). In particular, juvenile bass mortality could be increased through limited refugia (Canfield and Hoyer Citation1992, Killgore et al. Citation1993), and restoration of aquatic vegetation in reservoirs has been shown to increase densities of age-0 largemouth bass (Micropterus salmoides) in some systems (Strakosh et al. Citation2009, Stahr and Shoup Citation2015), while loss of macrophytes is associated with decreased juvenile abundance (Parsons et al. Citation2009). While response of the sportfish population will always be difficult and time consuming to measure in a system as large as Lake Apopka (Rogers and Allen Citation2012), continued habitat restoration efforts may increase the benefits for native sportfish populations by increasing refugia for juvenile sportfish.
Managers should consider planting mixed communities of macrophytes in addition to (or in combination with) established planted sites. Loss of plant biodiversity can cause large, cascading changes in the functionality of an ecosystem (Knops et al. Citation1999). We found that a diverse flora (i.e., more than one species) supported greater abundance and richness of fish species compared to the current planted monocultures of spatterdock. Mixed macrophyte communities also have greater resistance to invasions of undesirable and invasive species (Stenberg et al. Citation1997, Hoge et al. Citation2003), and planting mixed macrophyte communities could serve to extend the benefits from restoration measures such as drawdowns (where long-term benefits can be limited by rapid invasion of cattail; e.g., Moyer et al. Citation1995). Spatterdock is also a floating or emergent type of macrophyte; submersed macrophytes can provide varied benefits for fish communities (Gotceitas and Colgan Citation1990, Killgore et al. Citation1993, Stahr and Shoup Citation2015, Kuehne et al. Citation2016). The planting of mixed stands of macrophytes could have greater, longer-lasting benefits for the fish and invertebrate community compared to the status quo of planting spatterdock-only stands in Lake Apopka. Any additional plantings should also be monitored by management for survival and possible improvements to sportfish habitat.
Restoration activities, particularly macrophyte plantings, suffer from a lack of long-term evaluation, and wider cooperation between scientists and management organizations is needed (Minns et al. Citation1996). Future studies should consider evaluation of diverse planting vs. monoculture planting for improvements in ecosystem benefits, including greater numbers and diversity of fishes, macroinvertebrates, and greater resilience to invasion. Future studies should also be aware of the varied and unpredictable responses of fish communities to restoration efforts (Pine et al. Citation2009). Existing monitoring data should also be used for evaluation of restoration efforts in Lake Apopka (Spurgeon et al. Citation2016), although no long-term evaluation of macrophyte planting currently exists for Lake Apopka. With continued experimentation and evaluation, the most effective restoration practices can be identified and used to further improve the sportfish population, both in Lake Apopka and wherever habitat restoration techniques are employed.
Acknowledgments
We thank FWC for project funding and K. Zarada, J. Procopio, Y. Quintana, G. Scholten, M. Hosabettu, and D. Dreier for field support.
Additional information
Funding
References
- Allen MS, Tugend KI, Mann MJ. 2003. Largemouth bass abundance and angler catch rates following a habitat enhancement project at Lake Kissimmee. Florida. North Am J Fish Manag. 23:845–855.
- Anderson DR. 2008. Model based inference in the life sciences: a primer on evidence. New York (NY): Springer.
- Bachmann RW, Hoyer MV, Canfield DE Jr. 1999. The restoration of Lake Apopka in relation to alternative stable states. Hydrobiologia 394:219–232.
- Bayley PB, Austen DJ. 2002. Capture efficiency of a boat electrofisher. Trans Am Fish Soc. 131:435–451.
- Blindow I. 1992. Long- and short-term dynamics of submerged macrophytes in two shallow eutrophic lakes. Freshw Biol. 28:15–27.
- Bolker B. 2008. Ecological models and data in R. Princeton (NJ): Princeton University Press.
- Bonvechio KI, Bonvechio TF. 2006. Relationship between habitat and sport fish populations over a 20-year period at West Lake Tohopekaliga. Florida. North Am J Fish Manag. 26:124–133.
- Burks RL, Lodge DM, Jeppesen E, Lauridsen TL. 2002. Diel horizontal migration of zooplankton: costs and benefits of inhabiting the littoral. Freshw Biol. 47:343–365.
- Candy SG. 2004. Modelling catch and effort data using generalised linear models, the Tweedie distribution, random vessel effects and random stratum-by-year effects. CCAMLR Sci. 11:59–80.
- Canfield DE, Bachmann RW, Hoyer MV. 2000. A management alternative for Lake Apopka. Lake Reserv Manage. 16:205–221.
- Canfield DE, Hoyer MV. 1992. Aquatic macrophytes and their relation to the limnology of Florida lakes. Gainesville (FL): University of Florida Department of Fisheries and Aquaculture, Center for Aquatic Plants.
- Carpenter SR, Lodge DM. 1986. Effects of submersed macrophytes on ecosystem processes. Aquat Bot. 26:341–370.
- Chao A. 1987. Estimating the population size for capture-recapture data with unequal catchability. Biometrics 43:783–791.
- Chao A, Colwell RK, Lin C-W, Gotelli NJ. 2009. Sufficient sampling for asymptotic minimum species richness estimators. Ecology. 90:1125–1133.
- Chiu C-H, Wang Y-T, Walther BA, Chao A. 2014. An improved nonparametric lower bound of species richness via a modified Good–Turing frequency formula. Biometrics. 70:671–682.
- Clugston JP. 1963. Lake Apopka, Florida, a changing lake and its vegetation. Florida Acad Sci. 26:168–174.
- Colle DE, Shireman JV. 1980. Coefficients of condition for largemouth bass, bluegill, and redear sunfish in hydrilla-infested lakes. Trans Am Fish Soc. 109:521–531.
- Cooke GD, Welch EB, Peterson SA, Nichols SA. 2005. Restoration and management of lakes and reservoirs. New York (NY): Taylor & Francis.
- Coveney, MF. 2016. Water quality changes in Lake Apopka, Florida, and the St. Johns River Water Management District's restoration program. Palatka (FL): St. Johns River Water Management District.
- Coveney MF, Lowe EF, Battoe LE, Marzolf ER, Conrow R. 2005. Response of a eutrophic, shallow subtropical lake to reduced nutrient loading. Freshw Biol. 50:1718–1730.
- Coveney MF, Stites D, Lowe E, Battoe L, Conrow R. 2002. Nutrient removal from eutrophic lake water by wetland filtration. Ecol Eng. 19:141–159.
- Davis, WP, Steinman AD. 1998. A lightweight, inexpensive benthic core sampler for use in shallow water. J Freshw Ecol. 13:475–479.
- Dibble ED, Killgore KJ, Harrel SL. 1996. Assessment of fish–plant interactions. In: Miranda L, DeVries D (eds). Multidimensional approaches to reservoir fisheries management. Proceedings of the Third National Reservoir Fisheries Symposium, Chattanooga, Tennessee, USA, 12–14 June 1995. Vol. 16. Bethesda (MD): American Fisheries Society. p. 357–372.
- Dierberg FE, Williams VP. 1989. Lake management techniques in Florida. USA: costs and water quality effects. Environ Manage. 13:729–742.
- Dierberg FE, Williams VP, Schneider WH. 1988. Evaluating water quality effects of lake management in Florida. Lake Reserv Manage. 4:101–111.
- Dotson JR, Bonvechio KI, Thompson BC, Johnson WE, Trippel NA, Furse JB, Gornak S, McDaniel CK, Pouder WF, Leone EH. 2015. Effects of large-scale habitat enhancement strategies on Florida bass fisheries. In: Tringali MD, Long JM, Birdsong TW, Allen MS (eds). Black bass diversity: multidisciplinary science for conservation. Bethesda (MD): American Fisheries Society Symposium 82 p. 387–404
- Dunham JB, Cade BS, Terrell JW. 2002. Influences of spatial and temporal variation on fish–habitat relationships defined by regression quantiles. Trans Am Fish Soc. 131:86–98.
- Dunn PK, Smyth GK. 2008. Evaluation of Tweedie exponential dispersion model densities by Fourier inversion. Stat Comput. 18:73–86.
- Gasith A, Hoyer MV. 1998. Structuring role of macrophytes in lakes: changing influence along lake size and depth gradients. In: Jeppesen E, Søndergaard M, Søndergaard M, Christoffersen K (eds). Structuring role of submerged macrophytes in lakes. New York (NY): Springer. P. 381–392
- Gifford AM. 2002. The effect of macrophyte planting on amphibian and fish community use of two created wetland ecosystems in central Ohio. MS thesis. The Ohio State University: Columbus (OH).
- Gotceitas V, Colgan P. 1990. The effects of prey availability and predation risk on habitat selection by juvenile bluegill sunfish. Copeia. 1990:409–417.
- Hangsleben MA, Allen MS, Gwinn DC. 2013. Evaluation of electrofishing catch per unit effort for indexing fish abundance in Florida lakes. Trans Am Fish Soc. 142:247–256.
- Heltshe JF, Forrester NE. 1983. Estimating species richness using the jackknife procedure. Biometrics. 39:1–11.
- Hoge VR, Conrow R, Stites DL, Coveney MF, Marzolf ER, Lowe EF, Battoe LE. 2003. SWIM plan for Lake Apopka, Florida. Palatka (FL): St Johns River Water Management District.
- Hoyer MV, Canfield DE. 1996a. Lake size, aquatic macrophytes, and largemouth bass abundance in Florida lakes: a reply. J Aquat Plant Manag. 34:48–50.
- Hoyer MV, Canfield DE. 1996b. Largemouth bass abundance and aquatic vegetation in Florida lakes: an empirical analysis. J Aquat Plant Manag. 34:23–32.
- Hoyer MV, Canfield DE, Horsburgh CA, Brown K. 1996. Florida freshwater plants: a handbook of common aquatic plants in Florida lakes. Gainesville (FL): University Press of Florida.
- Hoyer MV, Wellendorf N, Frydenborg R, Bartlett D, Canfield DE. 2012. A comparison between professionally (Florida Department of Environmental Protection) and volunteer (Florida LAKEWATCH) collected trophic state chemistry data in Florida. Lake Reserv Manage. 28:277–281.
- Johnson WE, Crumpton JE. 1998. Lake Apopka fish population assessment. Final report for Contract #97W121 for St. Johns River Water Management District: Palatka, Florida.
- Jordan F, Arrington DA. 2014. Piscivore responses to enhancement of the channelized Kissimmee River, Florida. U.S.A. Restor Ecol. 22:418–425.
- Killgore KJ, Dibble ED, Hoover JJ. 1993. Relationships between fish and aquatic plants: a plan of study. Vicksburg (MS): U.S. Army Corps of Engineers Waterways Experiment Station Miscellaneous Paper A-93-1.
- Knops JMH, Tilman D, Haddad NM, Naeem S, Mitchell CE, Haarstad J, Ritchie ME, Howe KM, Reich PB, Siemann, et al. 1999. Effects of plant species richness on invasion dynamics, disease outbreaks, insect abundances and diversity. Ecol Lett. 2:286–293.
- Kristensen P, Sondergaard M, Jeppesen E. 1992. Resuspension in a shallow eutrophic lake. Hydrobiologia. 228:101–109.
- Kuehne LM, Olden JD, Rubenson ES. 2016. Multi-trophic impacts of an invasive aquatic plant. Freshw Biol. 61:1846–1861.
- Lawrence BA, Bourke K, Lishawa SC, Tuchman NC. 2016. Typha invasion associated with reduced aquatic macroinvertebrate abundance in northern Lake Huron coastal wetlands. J Great Lakes Res. 42:1412–1419.
- Lee GF, Jones RA. 1991. Effects of eutrophication on fisheries. Rev Aquat Sci. 5:287–305.
- Lowe EF, Battoe LE, Coveney MF, Schelske CL, Havens KE, Marzolf ER, Reddy KR. 2001. The restoration of Lake Apopka in relation to alternative stable states: an alternative view to that of Bachmann et al. (1999). Hydrobiologia. 448:11–18.
- Lowe EF, Battoe LE, Stites DL, Coveney MF. 1992. Particulate phosphorus removal via wetland filtration: an examination of potential for hypertrophic lake restoration. Environ Manage. 16:67–74.
- Magurran AE. 2004. Measuring biological diversity. 2nd ed. Malden (MA): Blackwell Publishing.
- Minns CK, Kelso JRM, Randall RG. 1996. Detecting the response of fish to habitat alterations in freshwater ecosystems. Can J Fish Aquat Sci. 53:403–414.
- Miranda LE, Hodges KB. 2000. Role of aquatic vegetation coverage on hypoxia and sunfish abundance in bays of a eutrophic reservoir. Hydrobiologia. 427:51–57.
- Mouton AM, Buysse D, Stevens M, Neucker T, Coeck J. 2012. Evaluation of riparian habitat restoration in a lowland river. River Res Appl. 28:845–857.
- Moyer EJ, Hulon MW, Sweatman JJ, Butler RS, Williams VP. 1995. Fishery responses to habitat restoration in Lake Tohopekaliga. Florida. North Am J Fish Manag. 15:591–595.
- [NRC] National Research Council. 1992. Restoration of aquatic ecosystems. Washington (DC): National Academies Press.
- Oksanen J. 2013. R package vegan: ecological diversity. https://cran.r-project.org/web/packages/vegan.
- Parsons JK, Couto A, Hamel KS, Marx GE. 2009. Effect of fluridone on macrophytes and fish in a coastal Washington lake. J Aquat Plant Manag. 47:31–40.
- Petr T. 2000. Interactions between fish and aquatic macrophytes in inland waters. A review. Rome: FAO Fisheries Technical Paper No. 396.
- Phillips G, Willby N, Moss B. 2016. Submerged macrophyte decline in shallow lakes: what have we learnt in the last forty years? Aquat Bot. 135:37–45.
- Pine WE, Martell SJD, Walters CJ, Kitchell JF. 2009. Counterintuitive responses of fish populations to management actions. Fisheries. 34:165–180.
- Core Team R. 2014. R: A language and environment for statistical computing. Vienna (Austria). https://www.r-project.org/.
- Reddy KR, Graetz DA. 1987. Internal nutrient budget for Lake Apopka – Final Report. Palatka (FL): Saint Johns Water Management District Special Publication SJ 91-SP6.
- Rogers MW, Allen MS. 2012. An ecosystem model for exploring lake restoration effects on fish communities and fisheries in Florida. Restor Ecol. 20:612–622.
- Roni P, Hanson K, Beechie T. 2008. Global review of the physical and biological effectiveness of stream habitat rehabilitation techniques. North Am J Fish Manag. 28:856–890.
- Rosenzweig ML. 1995. Species diversity in space and time. Cambridge (UK): Cambridge University Press.
- Savino JF, Stein RA. 1982. Predator–prey interaction between largemouth bass and bluegills as influenced by simulated, submersed vegetation. Trans Am Fish Soc. 111:255–266.
- Schaus MH, Godwin W, Battoe L, Coveney M, Lowe E, Roth R, Hawkins C, Vindigni M, Weinberg C, Zimmerman A. 2010. Impact of the removal of gizzard shad (Dorosoma cepedianum) on nutrient cycles in Lake Apopka. Florida. Freshw Biol. 55:2401–2413.
- Schelske C, Brezonik P. 1992. Can Lake Apopka be restored?. In: National Research Council. Restoration of aquatic ecosystems: science, technology, and public policy. Washington (DC): The National Academies Press. P. 393–398
- Schelske CL, Lowe EF, Battoe LE, Brenner M, Coveney MF, Kenney WF. 2005. Abrupt biological response to hydrologic and land-use changes in Lake Apopka, Florida. USA. AMBIO: J Hum Environ. 34:192–198.
- Schneider RF, Little JA. 1973. Rise and fall of Lake Apopka: a case study in reservoir mismanagement. In: Ackermann WC, White GF, Worthington EB, Ivens JL (eds). Man-made lakes: their problems and environmental effects. Washington (DC): American Geophysical Union. P. 690–694
- Schummer ML, Palframan J, McNaughton E, Barney T, Petrie SA. 2012. Comparisons of bird, aquatic macroinvertebrate, and plant communities among dredged ponds and natural wetland habitats at Long Point, Lake Erie. Ontario. Wetlands. 32:945–953.
- Shen T, Chao A, Lin C. 2003. Predicting the number of new species in further taxonomic sampling. Ecology. 84:798–804.
- Shono H. 2008. Application of the Tweedie distribution to zero-catch data in CPUE analysis. Fish Res. 93:154–162.
- Simpson EH. 1949. Measurement of diversity. Nature 163:688.
- Spurgeon JJ, Stewart NT, Pegg MA, Pope KL, Porath MT. 2016. Using standardized fishery data to inform rehabilitation efforts. Lake Reserv Manage. 32:41–50.
- Stahr KJ, Shoup DE. 2015. American water willow mediates survival and antipredator behavior of juvenile largemouth bass. Trans Am Fish Soc. 144:903–910.
- Stenberg J, Clark M, Conrow R. 1997. Development of natural and planted vegetation and wildlife use in the Lake Apopka Mash Flow-way Demonstration Project: 1990–1994. Palatka (FL): Saint Johns River Water Management District Special Publication SJ98–SP4.
- Strakosh TR, Gido KB, Guy CS. 2009. Effects of American water willow establishment on density, growth, diet, and condition of age-0 largemouth bass in Kansas reservoirs. Trans Am Fish Soc. 138:269–279.
- Thomas G, Lorenz AW, Sundermann A, Haase P, Peter A, Stoll S. 2015. Fish community responses and the temporal dynamics of recovery following river habitat restorations in Europe. Freshw Sci. 34:975–990.
- Tukey JW. 1949. Comparing individual means in the analysis of variance. Biometrics. 5:99–114.
- Tweedie MCK. 1984. An index which distinguishes between some important exponential families. In: Ghosh JK and Roy J, (eds). Statistics: applications and new directions. Proceedings of the Indian Statistical Institute Golden Jubilee International Conference. P. 604
- Van Donk E, Van de Bund WJ. 2002. Impact of submerged macrophytes including charophytes on phyto- and zooplankton communities: allelopathy versus other mechanisms. Aquat Bot. 72:261–274.
- Van Zyll de Jong M, Cowx IG. 2016. Long-term response of salmonid populations to habitat restoration in a boreal forest stream. Ecol Eng. 91:148–157.
- Wiley MJ, Gorden RW, Waite SW, Powless T. 1984. The relationship between aquatic macrophytes and sport fish production in Illinois ponds: a simple model. North Am J Fish Manag. 4:111–119.
- Wilson KL, Allen MS, Ahrens RNM, Netherland MD. 2015. Nonlinear and density-dependent fish habitat selection across physiochemical gradients in an invasive macrophyte habitat. Environ Biol Fishes. 98:1525–1539.
- Zhang W. 2013. Likelihood-based and Bayesian methods for Tweedie compound poisson linear mixed models. Stat Comput. 23:743–757.