Abstract
Schad AN, Dick GO. Aquatic vegetation community structure response to hydrilla management with triploid grass carp, herbicide, and native vegetation planting. Lake Reserve Manage. 34:417–425.
To aid conservation managers conducting hydrilla (Hydrilla verticillata (L.f.) Royle) management, we evaluated changes in aquatic vegetation community structure and dominance in dioecious hydrilla-infested ponds by applying several management tools and monitoring vegetation changes on an annual basis over a 4 yr period. The study was conducted in fully vegetated (dioecious hydrilla and native macrophytes) 0.3 ha mesocosm ponds using 4 levels of triploid grass carp (Ctenopharyngodon idella Valenciennes) stocking densities (0–129 per vegetated hectare), a one-time second-year herbicide treatment to reduce standing biomass of hydrilla and other submersed aquatic vegetation (SAV), and native plantings to provide the basis for replacing hydrilla as it was controlled. Grass carp stocking density had an inverse relationship with coverage of total vegetation, hydrilla, volunteer SAV, and volunteer emergents. All vegetation categories recovered from the one-time herbicide application, although this was suppressed by higher densities of grass carp. Grass carp did not significantly affect planted growth forms (SAV, floating-leaved, and emergent), with increases in mean coverage in all treatments through time, although coverage was lowest at the highest grass carp density. Vegetation dominance shifted from hydrilla to native in both control and low grass carp density treatments, with most SAV eliminated from treatments stocked with more than 72 per hectare.
Introduction
Hydrilla (Hydrilla verticillata (L.f.) Royle) infestations can be detrimental to water resource usages and ecosystem function, and habitually require management (Pieterse Citation1981, Langeland Citation1996). A variety of control measures have been developed for the species, but none thus far have proven self-sustainable, and hydrilla remains a long-term problem for many water resources (Gallagher and Haller Citation1990, Haller Citation2009). Because controlling hydrilla is commonly a component of a larger ecosystem management goal, impact on native vegetation requires consideration. Thus, efforts to improve sustained control methods continue, with focuses on identifying selective aquatic herbicides, finding host-specific biocontrol agents, and combining existing methods (Integrated Pest Management, IPM).
Aquatic herbicides are effective at controlling hydrilla, but alone generally provide only short-term control (Hestand and Carter Citation1978). Additionally, the most effective herbicides currently available for this species are only moderately selective, which can result in damage to native plants (Haller Citation2009). Herbicide-resistant aquatic weeds further complicate the continuous use of aquatic herbicides as a primary tool for hydrilla management (Koschinick et al. Citation2006, Richardson Citation2008).
Another method that can provide control of hydrilla is the use of triploid grass carp (Ctenopharyngodon idella Valenciennes; Michewicz et al. Citation1972, Hestand and Carter Citation1978, Chilton and Muoneke Citation1992, Bain Citation1993). Higher (>40 per vegetated hectare) stocking densities tend to be more effective at removal and suppression of hydrilla, but because triploid grass carp are generalist herbivores (Kilgen and Smitherman Citation1971), such densities may also remove most, if not all, native vegetation (Hanlon et al. Citation2000, Bonar et al. Citation2002, Dibble and Kovalenko Citation2009). Lower (<40 per vegetated hectare) densities of grass carp have been inconsistent in hydrilla control, possibly because established hydrilla is able to outgrow grazing pressures of lower numbers of fish (Hanlon et al. Citation2000, Pipalova Citation2006).
In some cases, resource managers have attempted to manage hydrilla using an IPM approach that combines aquatic herbicides and lower-density grass carp stockings (Haller Citation2009, Kirk and Manuel Citation2011, Sutton et al. Citation2013). Because grass carp show feeding preference for hydrilla over some other aquatic vegetation (Colle et al. Citation1978, Hestand and Carter Citation1978, Bonar et al. Citation1990, Pine and Anderson Citation1991, Parker and Hay Citation2005), targeted feeding on resprouting hydrilla has the potential to shift the balance from hydrilla-dominated communities to those dominated by less-palatable native species (Hanlon et al. Citation2000). This approach has yielded widely inconsistent results, with grass carp either not suppressing hydrilla or eliminating the entire aquatic plant community in many cases (Hanlon et al. Citation2000, Bonar et al. Citation2002, Pipalova Citation2006, Dibble and Kovalenko Citation2009).
Reasons for variable results may include uncertainties regarding the density of grass carp needed to control specific infestations, changing grass carp densities due to attrition, incremental stocking practices, and plant susceptibility to other herbivores. While grass carp are known to prefer feeding on some species (palatable) over others (unpalatable), the presence of unpalatable native species in waterbodies in which hydrilla is being managed by grass carp may not be sufficient for replacing hydrilla as it declines (Colle et al. Citation1978, Bonar et al. Citation1990, Hofstra and Clayton Citation2001, Parker and Hay Citation2005). This may be particularly true in constructed or highly disturbed natural systems in which native vegetation communities are poorly developed and may lack different growth forms (Smart and Doyle Citation1995). In these cases, supplementing the native aquatic plant community is a consideration that may improve sustainable efficacy of the herbicide/grass carp IPM approach.
In this study, we evaluated changes in aquatic vegetation community structure in response to a combination of hydrilla management applications and native aquatic plant establishment. The study was conducted in fully vegetated (hydrilla and native macrophytes) earthen ponds and focused on hydrilla management using 4 grouped levels of grass carp stocking densities (none, low, medium, and high), a one-time herbicide treatment to reduce standing hydrilla and other submersed aquatic vegetation (SAV) biomass, and plantings of native vegetation to replace hydrilla and grass carp–palatable natives that occurred in the ponds. The primary goals were to evaluate how each grass carp density affected the aquatic vegetation communities over a 4 yr period and make inferences on which treatment promoted a shift of dominance from hydrilla to natives.
Materials and methods
The study was conducted at the U.S. Army Corps of Engineers Research and Development Center’s Lewisville Aquatic Ecosystem Research Facility (LAERF), located in Lewisville, Texas (latitude 33°04′45″N, longitude 96°57′30″W). Eight rectangular, earthen ponds with surface areas from 0.26 to 0.32 ha, volumes from 2,459 to 3,555 m3, and mean depths of about 1.0 m (Smart Citation1995) were used in the study. Prior to the study, in September 2010, vegetation coverage in the ponds was visually estimated at 100%, consisting primarily of dioecious hydrilla and a group of volunteer native macrophyte species, including longleaf pondweed (Potamogeton nodosus Poir.), southern waternymph (Najas guadalupensis (Spreng.) Magnus), muskgrass (Chara vulgaris L.), delta arrowhead (Sagittaria platyphylla (Engelm.) J. G. Sm.), squarestem spikerush (Eleocharis macrostachya Britton), and knotgrass (Paspalum distichum L.). Each pond was equally divided lengthwise by a welded-wire fence barrier covered with semipermeable landscape fabric to prevent movement of grass carp, resulting in 16 experimental units ranging from 0.13 to 0.16 ha surface area and depths of each ranging from 0 to 2 m deep. Ponds were filled by gravity flow with water from nearby Lewisville Lake with levels maintained throughout the study.
In May 2011, triploid grass carp ranging in length from 25 to 30 cm and averaging 352 g were stocked at 4 rates to provide 4 replicates for each of the following treatments: control (C) with no fish stocked; low density (LD) of 40–43 fish per vegetated hectare (approximately 17 per acre); medium density (MD) of 72–81 fish per vegetated hectare (approximately 31 per acre); and high density (HD) of 110–129 fish per vegetated hectare (approximately 48 per acre). Grass carp regularly schooled near water inflows, making periodic visual counts possible for verifying numbers of fish in each study pond, with supplemental stockings made once per year to maintain numbers as needed within a target range of +/− 10%. One MD pond and 1 HD pond were removed from analysis because the lack of confirmation of grass carp numbers during the study, leaving 3 replicates for each of those treatments for final analyses.
In May 2012, approximately 1 yr after stocking grass carp, all ponds were treated via surface spray with a USEPA-registered contact aquatic herbicide, Aquathol K (endothall: dipotassium salt form of endothall [7-oxabicyclo (2.2.1) heptane-2, 3-dicarboxylic acid]) at 1 mg/L active ingredient to remove above-ground biomass of hydrilla and other SAV, principally P. nodosus and N. guadalupensis. This rate is considered adequate for short-term control of hydrilla and other SAV naturally occurring in the ponds (Skogerboe and Getsinger Citation2001) and successfully eliminated standing biomass within 2 weeks of treatment. The herbicide application was made to provide conditions needed to evaluate grass carp suppression of recovering vegetation (both hydrilla and native volunteer) and set the stage for supplementing the native plant community with grass carp–tolerant species.
In June 2012, when new sprouts of hydrilla and native vegetation were observed, 11 species of native aquatic plants were transplanted into each pond (). Plants selected were those shown to be capable of contributing a persistent pressure on hydrilla by preemptively occupying niches (Smart Citation1995) and/or had shown evidence of tolerance to grass carp grazing in vegetation establishment efforts (Dick et al. Citation2016). Prior to planting, plants were grown to root-bound condition in commercial nursery pots following guidelines described in Dick et al. (Citation2013). Plants were de-potted and placed at approximate 1.8 m on-centers along 2 depth tiers accessible to grass carp in each pond. Emergent species were planted in the shallower tier at 0.3 m deep, while floating-leaved and submersed species were planted at 0.6 m depths. Six containerized individuals of each species were planted as 3 pairs at randomly assigned positions along the appropriate depth tier, with 1 plant of each pair protected from herbivory with a 58 cm diameter, PVC-coated welded-wire cylinder to exclude grass carp and other large herbivores such as waterfowl and turtles for initial establishment.
Table 1. Eleven native aquatic plant species were transplanted into units with and without herbivore protection to evaluate their suitability for replacing hydrilla being controlled by triploid grass carp.
Vegetation coverage resulting from these plantings totaled 1.32 m2 (0.12 m2 per species) in each pond, or about 0.10% the area of each. These small-scale plantings followed native vegetation founder colony establishment techniques developed by Dick et al. (Citation2013), where grass carp–tolerant transplants would grow and spread rapidly into areas where hydrilla (or other vegetation) recovery was kept in check by grass carp. Results of individual species survival and growth with and without protection are reported and discussed in detail in Dick et al. (Citation2016), with Nymphaea odorata, Echinodorus cordifolius, Vallisneria americana, and Justicia americana generally demonstrating the greatest resistance to grass carp herbivory.
In November 2010, water levels in ponds were lowered to facilitate GIS mapping of vegetation cover and distribution, primarily to verify hydrilla was present throughout each. Thereafter, vegetation community structure was assessed at full water level (so as not to disturb vegetation or grass carp) with visual estimates of percent coverage constructed in the field using reference points and later transferred to aerial photography (Google Earth Pro, 1 April 2011) and GIS mapped (ESRI, Redlands, CA). Percent coverage was estimated in 10% increments (plus 0% or 5% when coverage was absent or low) in each mapped area for 7 categories: hydrilla, volunteer SAV, planted SAV, charophytes, planted floating–leaved, volunteer emergents, and planted emergents. Total percent coverage for each category was then calculated for each pond. For category dominance trend analysis, percent coverage was assigned in accordance with the category’s canopy-forming abilities as described in Smart et al. (Citation2009); additional criteria to be assigned dominance was contribution to >50% coverage of a particular mapped area. Vegetation categories were expressed as percent coverage of the total pond area to standardize data. To standardize growing season or in-year vegetation differences for factor and treatment comparisons, surveys were taken toward the end of the growing season (Sep) each year.
Percent coverages of vegetation categories were analyzed by creating generalized linear models with binomial (events/trials data) as the distribution and probit as the link function (probit regression or probit GLM). In this analysis, there was 1 between-units factor (grass carp stocking density at 4 levels: C, LD, MD, HD) and 1 within-units factor (survey year). Response variables included percent coverage of hydrilla, volunteer SAV, volunteer emergent, total vegetation, planted SAV, planted floating–leaved, and planted emergent, as well as hydrilla dominance and native dominance (combined volunteer and planted). Main effects and 2-way interactions were included in each probit regression. Tests for main model effects were done using Wald Chi-Square. Main effect estimated marginal means were also pairwise contrasted for multiple comparisons (with Bonferroni adjustment) to explore differences between levels of factors of interest where appropriate. All analyses were done using IBM SPSS version 22; α = 0.1.
Results
More than 90% coverage by aquatic vegetation, including hydrilla, was estimated in all ponds at study onset in September 2010. Through the study period, hydrilla, volunteer SAV, volunteer emergent, and total vegetation coverage were significantly different between grass carp densities and within sampling dates, while hydrilla, SAV, and total coverage also demonstrated a significant interaction with independent variables with greater declines in coverages occurring at higher grass carp densities over time (, ). While volunteer SAV (mostly P. nodosus and N. guadalupensis) occurred in C throughout the study, by its end, neither MD nor HD supported volunteer SAV; 2 of the 4 LD ponds were void as well. A similar trend was seen in native emergent volunteer communities, which consisted of 3 principal species at the onset of the study: S. platyphylla, E. macrostachya, and P. distichum. While these 3 species persisted as emergent co-dominants in C and LD throughout the study, by 2013, P. distichum was the most common of the 3 in MD and HD treatments, with near absence of S. platyphylla in both treatments.
Figure 1. Vegetation cover class coverage (mean + SD) between 4 grass carp densities (C = control, LD = low density, MD = medium density, HD = high density) before (2010) and after (2011) stocking grass carp; denoted with letters are statistically similar groupings (α = 0.1) of main effects year (upper-case) and grass carp density (lower-case), where appropriate (least significant difference).
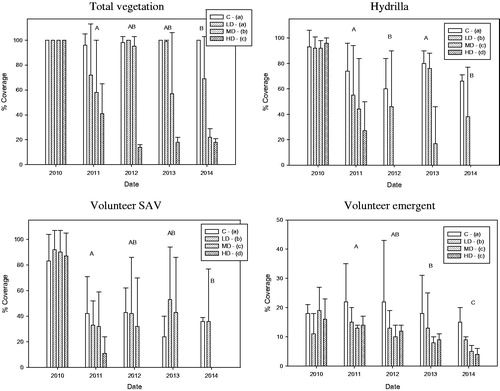
Table 2. Results of the probit regression for vegetation community structure class percent coverage response to grass carp densities over time; significance is given in bold.
Percent coverages for all 3 planted growth forms were significantly different within sampling year, due to vegetation spread, but not between grass carp density, although some effects were observed (). Planted vegetation increased coverages from year to year among all grass carp stocking rates during the study (). This dynamic was more pronounced, or had exceptions in certain cases, with particular growth forms. For example, by the end of the study planted SAV mean coverages increased from HD (0%) < C (0.93%) < MD (3.25%) < LD (3.62%), creating a bell-shaped curve on predictor variable grass carp stocking rates. Nearly all planted SAV coverage was attributable to V. americana, floating-leaved vegetation included a single species, N. odorata, and emergents included all planted species except for E. quadrangulata.
Table 3. Results of the probit regression for planted vegetation cover class percent coverage response to grass carp densities over time; significance in bold.
Table 4. Raw and percent planted vegetation coverage (mean ± SD) between 4 grass carp densities (C = control, LD = low density, MD = medium density, HD = high density).
Both hydrilla and native plant dominance were significantly affected by grass carp stocking density, sampling year, and factor interaction (, ). Hydrilla dominance statistically unique grouped means increased from C < LD < MD & HD, while native dominance increased from C & LD < MD < HD; (LSD). In C treatments, total mean vegetation coverage remained at 100%, but mean hydrilla dominance had declined from 50% (2011) to 29% (2014); by that same time, native vegetation dominance increased from 50% to 71%. However, the reduction was less pronounced when looking at the ratio of hydrilla (29%) to native SAV (36%) mean dominance after 4 yr, where hydrilla remained dominant in deeper portions of the ponds. In LD treatments, total mean vegetation coverage had declined from 100% (2010) to 69% (2014), and even though hydrilla remained present, it was not dominant at study’s end (0–1%). In MD and HD treatments, total mean vegetation coverage had declined from 100% in both to 22% and 18%, respectively, and was composed primarily of planted emergent and floating-leaved species. Little SAV remained in MD (3.2%, V. americana) and none in HD treatments, with large swaths of the ponds left devoid of vegetation.
Figure 2. Hydrilla and native vegetation mean percent dominance ± SD between grass carp treatments 2011–2014; bare area not pictured.
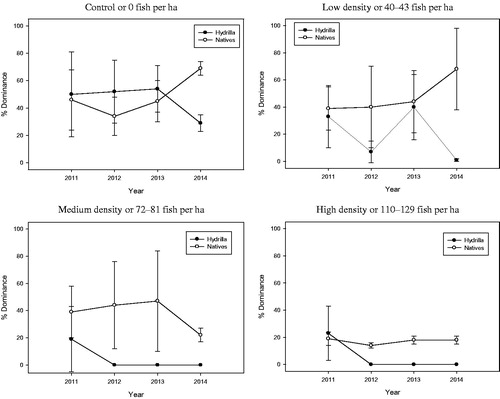
Table 5. Results of the probit regression for hydrilla and native vegetation dominance percent coverage response to grass carp densities over time; significance in bold.
Discussion
Changes in vegetation coverage and community structure throughout the study were related primarily to grass carp density. As expected, the higher the fish density the greater the loss of targeted hydrilla and nontargeted native vegetation (Hanlon et al. Citation2000, Bonar et al. Citation2002, Dibble and Kovalenko Citation2009). Total vegetation coverage remained similar over time in C (no grass carp), where recovery of plants following herbicide treatment resulted in continued full coverage throughout most of the study. The LD treatments (40–43 fish per vegetated hectare) exhibited high-coverage vegetation communities during the first 3 yr, and declined variably after the fourth, with some supporting considerably less vegetation than others. The MD treatments (72–81 fish per vegetated hectare) exhibited coverage variability during the first 3 yr, but were more similar to one another after the fourth, with vegetation communities made up primarily of volunteer emergents and planted floating-leaved and emergents. An exception to this was the occurrence of 1 planted SAV species (V. americana) in 2 of the MD ponds (Dick et al. Citation2016). The HD treatments (110–129 fish per vegetated hectare) exhibited little variability (or vegetation) during the entire study.
Throughout the study, in general, vegetation coverages remained in the C/LD groups, but declined dramatically in the MD/HD groups. The main difference between these groups was total vegetation coverage, where the line between the “all or nothing” dynamic (Hanlon et al. Citation2000) can be distinguished. When an ecosystem management’s goal is to remove hydrilla from a system, MD/HD may provide the most desirable results, with above-ground hydrilla essentially eliminated after 4 yr. Accompanying loss of volunteer and planted SAV in most of those same ponds, however, showed potential consequences of using these stocking rates, with the ecosystem left with little, if any, SAV and the benefits derived from vegetation. With large increases in bare areas in these treatments, those areas were left susceptible to future colonization by plants or other species as harmful as, or more harmful than, hydrilla. As an example, during the winter 2014 following the study, we noted Asian clams (Corbicula fluminea L.), long known to occur at LAERF but never becoming established in ponds, had become abundant in HD and present in MD, but not in C/LD. It appeared removal of one invasive aquatic species without addressing the resultant ecosystem changes resulted in infestation by another.
When an ecosystem management’s goal is to reduce hydrilla dominance in an ecosystem, LD, and to a much lesser extent C, provided results that left the vegetation community intact, but not dominated by hydrilla. The gradual decline in hydrilla coverage in C ponds could be attributable to the herbicide application and increased competition by volunteer and planted native vegetation (Smart et al. 1994, Smart Citation1995). In LD, hydrilla coverage declined throughout the study, as did volunteer SAV, while other native vegetation increased (principally planted native emergent and floating-leaved species), potentially attaining a holistic goal of controlling targeted vegetation and replacing damaged nontargeted vegetation with species less susceptible to management applications.
This study also showed the potential for ameliorating nontargeted vegetation damage by supplementing the community with species with low susceptibility to grass carp. The results indicate planting SAV not previously occurring in the ponds was generally most successful with grass carp stocking rates between groups (LD and MD, 40–81 fish per vegetated hectare) compared to C (no grass carp) or HD (above 110 fish per vegetated hectare; ). Planted SAV coverages were not as pronounced in control treatments perhaps due competitive interactions with hydrilla and other volunteer vegetation. Vallisneria americana was the most common planted SAV to establish and spread in LD and MD treatments, implying lower susceptibility to grass carp grazing than other planted and volunteer SAV. Therefore, even though MD resulted in removal of hydrilla and volunteer SAV species, survival and growth of V. americana showed replacing hydrilla with a desirable native plant is possible when grass carp are used for control, but might take time and/or more intense planting efforts.
The planted floating-leaved species used in this project, Nymphaea odorata, increased coverage annually in all treatments (). While grazing by grass carp was observed, it was not sufficient to prevent establishment and spread, even in the absence of more palatable species regardless of stocking rates. This result indicated N. odorata, and perhaps other floating-leaved species known to produce oxalate crystals or other herbivore defense chemicals, can deter substantial feeding by grass carp and may serve as good candidates for establishing vegetation to replace hydrilla and other species of lost SAV (Kuo-Huang Citation1990). Similarly, some planted emergent species were not controlled by grass carp, resulting in steady increases in mean coverages of planted emergents 2 yr after planting in all treatments. While all planted emergent species increased coverage over time in C/LD, only a few were able to spread in MD/HD, including Justicia americana, Echinodorus cordifolius, and Schoenoplectus tabernaemontani. Justicia americana, particularly, is a commonly used “restoration” plant, which in part may be successful because of its relative unpalatability to grass carp and other herbivores (Dick et al. Citation2016).
From these outcomes, we can infer one of several approaches may prove successful dependent on ecosystem management goals. The first, to shift general hydrilla dominance to native dominance without impacting vegetation coverage, our controls indicated that a combination of aquatic herbicide and supplementing the native plant community may enable reduction of hydrilla coverage, although this effect was prominent only in shallower areas of the ponds. Alternatively, using low densities of grass carp (approximately 40 per vegetated hectare) in conjunction with herbicide and native plantings reduced hydrilla coverage and dominance, but permitted some native species to persist and replace lost hydrilla. In our study, this treatment resulted in substantial declines in hydrilla dominance without accompanying loss of total vegetation coverage. However, Hanlon et al. (Citation2000), after an evaluation of 38 stocked Florida lakes, suggest anything above 25–30 fish per vegetated hectare can achieve full SAV eradication within 10 yr, a reminder to proceed with caution in this type of management strategy. Another approach, a more rapid removal of hydrilla with increased native species bystander risk, would be to increase the stocking density to between 70 and 80 fish per vegetated hectare (MD in our study) for more thorough removal of hydrilla, with limited herbicide applications and extensive revegetation efforts to establish unpalatable species. Our results suggest stockings in this range will potentially permit establishment of certain emergent, floating-leaved, and SAV species to counter the loss of hydrilla and other vegetation. Stocking at higher rates (110 or more per hectare) will eliminate hydrilla and other SAV, but may permit establishment of only a few species (floating-leaved and emergent) highly unpalatable to grass carp.
Acknowledgments
This work was funded by the U.S. Army Corps of Engineers Aquatic Plant Control Research Program (APCRP).
References
- Bain M. 1993. Assessing impacts of introduced aquatic species: grass carp in large systems. Environ Manage. 17:211–224. doi:10.1007/BF02394691.
- Bonar SA, Bolding B, Divens M. 2002. Effects of triploid grass carp on aquatic plants, water quality, and public satisfaction in Washington State. N Am J Fish Manage. 22:96–105. doi: 10.1577/1548-8675(2002)022%3c0096:EOTGCO%3e2.0.CO;2.
- Bonar SA, Sehgal HS, Pauley GB, Thomas GL. 1990. Relationship between the chemical composition of aquatic macrophytes and their consumption by grass carp (Ctenopharyngodon idella). J Fish Biol. 36:149–157. doi:10.1111/j.1095-8649.1990.tb05591.x.
- Chilton EW III, Muoneke MI. 1992. Biology and management of grass carp (Ctenopharyngodon idella, Cyprinidae) for vegetation control: a North American perspective. Rev Fish Biol Fish. 2:283–320. doi:10.1007/BF00043520.
- Colle DE, Shireman JV, Rottmann RW. 1978. Food selection by grass carp fingerlings in a vegetated pond. Trans Am Fish Soc. 107:149–152. doi:10.1577/1548-8659(1978)107%3c149:FSBGCF%3e2.0.CO;2.
- Dibble ED, Kovalenko K. 2009. Ecological impact of grass carp: a review of the available data. J Aquat Plant Manage. 47:1–15.
- Dick GO, Smart RM, Dodd LL. 2013. Propagation and establishment of native plants for vegetative restoration of aquatic ecosystems. Vicksburg (MS): U.S. Army Engineer Research and Development Center Environmental Laboratory Technical Report ERDC/EL TR-13-9.
- Dick GO, Smith DH, Schad AN, Owens C. 2016. Native aquatic vegetation establishment in the presence of grass carp. Lake Reserv Manage. 32:222–230. doi:10.1080/10402381.2016.1167147.
- Gallagher JE, Haller WT. 1990. History and development of aquatic weed control in the United States. Weed Sci. 5:115–192.
- Haller WT. 2009. Hydrilla. In: Gettys LA, Haller WT, Bellaud M, editors. Biology and control of aquatic plants: a best management practices handbook. Marietta (GA): Aquatic Ecosystem Restoration Foundation. p. 89–93.
- Hanlon SG, Hoyer MV, Cichra CE, Canfield DE. 2000. Evaluation of macrophyte control in 38 Florida lakes using triploid grass carp. J Aquat Plant Manage. 38:48–54.
- Hestand RS, Carter CC. 1978. Comparative effects of grass carp and selected herbicides on macrophyte and phytoplankton communities. J Aquat Plant Manage. 16:43–50.
- Hofstra DE, Clayton JS. 2001. Evaluation of selected herbicides for control of exotic submerged weeds in New Zealand: 1. the use of endothall, triclopyr and dichlobenil. J Aquat Plant Manage. 39:20–24.
- Kilgen RH, Smitherman RO. 1971. Food habits of the white amur stocked in ponds alone and in combination with other species. Prog Fish Cult. 33:123–127. doi:10.1577/1548-8640(1971)33%5b123:FHOTWA%5d2.0.CO;2.
- Kirk JP, Manuel KL. 2011. Application of early detection and rapid response management techniques to control hydrilla. Vicksburg (MS): U.S. Army Engineer Research and Development Center Environmental Laboratory Technical Note ERDC/TN APCRP-BC-23.
- Koschnick TJ, Haller WT, Netherland MD. 2006. Aquatic plant resistance to Herbicides. Aquatics. 28:4–9.
- Kuo-Huang LL. 1990. Calcium oxalate crystals in the leaves of Nelumbo nucifera and Nymphaea tetragona. Taiwania. 35:178–190.
- Langeland KA. 1996. Hydrilla verticillata (L.f.) Royle (Hydrocharitaceae), “The perfect aquatic weed”. Castanea. 61:293–304.
- Michewicz JE, Sutton DL, Blackburn RD. 1972. The white amur for aquatic weed control. Weed Sci. 20:106–110.
- Parker JD, Hay ME. 2005. Biotic resistance to plant invasions? Native herbivores prefer non-native plants. Ecol Let. 8:959–967. doi:10.1111/j.1461-0248.2005.00799.x.
- Pieterse AH. 1981. Hydrilla verticillata - a review. Abstr Trop Agric. 7:9–34.
- Pine RT, Anderson WJ. 1991. Plant preferences of triploid grass carp. J Aquat Plant Manage. 29:80–82.
- Pipalova I. 2006. A review of grass carp use for aquatic weed control and its impact on water bodies. J Aquat Plant Manage. 44:1–12.
- Richardson, RJ. 2008. Aquatic plant management and the impact of emerging herbicide resistance issues. Weed Technology 22:8–15. doi:10.1614/WT-07-034.1.
- Skogerboe JG, Getsinger KD. 2001. Endothall species selectivity evaluation: southern latitude plant community. J Aquat Plant Manage. 39:129–135.
- Smart RM. 1995. Preemption: An important determinant of competitive success, pp. 231–236. In Proceedings, 29th annual meeting, Aquatic Plant Control Research Program. Miscellaneous Paper A-95-3, U.S. Army Engineer Waterways Experiment Station, Vicksburg, (MS).
- Smart RM, Doyle R. 1995. Ecological theory and the management of submersed aquatic plant communities. Vicksburg (MS): U.S. Army Engineer Research and Development Center Technical report APCRP: A-95-3.
- Smart RM, Barko JW, McFarland DG. 1994. Competition between Hydrilla verticillata and Vallisneria americana under different environmental conditions. Vicksburg (MS): U.S. Army Engineer Research and Development Center Technical report TR A-94-1.
- Smart RM, Dick GO, Honnell DR, Madsen JD, Snow JR. 1995. Physical and environmental characteristics of experimental ponds at the Lewisville Aquatic Ecosystem Research Facility. Vicksburg (MS): U.S. Army Engineer Research and Development Center Miscellaneous Paper A-95-2.
- Smart RM, Dick GO, Snow JR, Honnell DR, Smith DG, Smith, JK. 2009. Ecological effects of exotic and native aquatic vegetation. Vicksburg (MS): U.S. Army Engineer Research and Development Center Technical Report ERDC/EL TR-09-10.
- Sutton DL, Vandiver VV Jr, Hill JE. 2013. Grass carp: a fish for biological management of hydrilla and other aquatic weeds in Florida. UF/IFAS Extension Bull. 867: 1–6. https://edis.ifas.ufl.edu/pdffiles/FA/FA04300.pdf