Abstract
Whitmore TJ, Riedinger-Whitmore MA, Reed ZE, Curtis JH, Yang H, Evans DE, Cropper NR, Alvarado KS, Lauterman FM, Scott A, Leonard CR, Franklin DL. 2020. Paleolimnological assessment of six lakes on the Kissimmee Chain, with implications for restoration of the Kissimmee–Okeechobee–Everglades system, Florida, USA. Lake Reserv Manage. 36:218–242.
The Kissimmee Basin in south central Florida contains a large, freshwater network that includes the Kissimmee River and nearly 2 dozen lakes that are headwaters of the Florida Everglades. Management of these lakes is an important part of Everglades restoration. We report a paleolimnological investigation of 6 lakes in the Upper Kissimmee Basin. Engineering activities connected the lakes and permanently altered hydrology in the 19th and 20th centuries. The lakes were naturally meso-eutrophic, but changes in lake levels and nutrient loading contributed to different degrees of eutrophication. Cyanobacteria were present historically at low levels in Lakes East Tohopekaliga, Cypress, and Tohopekaliga, but increased during the 20th century. Lake Jackson lacked cyanobacteria until recently, but Lakes Kissimmee and Marian have had high levels of cyanobacteria since predisturbance times. Profound changes in the lakes occurred after engineering activities eliminated natural large fluctuations in water levels that periodically dried large portions of the basins. Salt-tolerant biological indicators previously alternated with freshwater organisms. Large water-level fluctuations moderated aquatic-plant standing crops and reduced organic matter accumulation. Lakes Kissimmee and Marian showed greatest evidence of former associated wetlands, but lacked large variations in water levels. We recommend disconnecting these lakes from each other and from the Kissimmee River to reestablish large, natural fluctuations in water levels that were part of healthy ecosystem function. Former wetlands should be restored to slow the downstream cascade of nutrients to Lake Okeechobee and the Everglades. This study demonstrates that paleolimnology is useful for assessing hydrological changes that potentially affect lake restoration efforts.
The Kissimmee Chain of Lakes is a series of shallow, connected warm-temperate lakes in central Florida. Lakes on the chain are headwaters of the Kissimmee–Okeechobee–Everglades system, which extends from Orange County southward through Lake Okeechobee to the tip of the Florida Everglades, where discharge from the Everglades affects nearshore coral reefs. The lakes are situated in the ∼6164 km2 Kissimmee Basin, which contains the Kissimmee River, as well as associated streams and wetlands. Historically, the lakes were surrounded by freshwater marshes, pine flatwoods, and wet prairies that were connected by broad, shallow wetlands and creeks (South Florida Water Management District Citation2019). Water levels fluctuated seasonally, which facilitated the connection of upper to lower lakes through an extensive marsh complex (US Army Corps of Engineers Citation1996). A major effort to connect the lakes to the Kissimmee River and to Lake Okeechobee through canal construction began by the early 1880s. Canals were constructed to lower water levels and drain the northern Everglades region, to lessen flood impacts, to expand areas available for agriculture and ranching, and to reduce water flow and flooding in the lower Everglades. Water levels dropped by ∼1.8 m in lakes throughout the Upper Kissimmee Basin (US Army Corps of Engineers Citation1996), and connections between lakes and associated wetlands were severed (Atlantic and Gulf Coast Canal and Okeechobee Land Company 1885). Throughout the 1900s, wetland areas were drained for agriculture. Marshes adjacent to lakes were reduced to narrow littoral zones, which affected plant and animal communities (US Army Corps of Engineers Citation1996). Modern areas of the lakes are substantially smaller than their historic sizes because of drainage activities (Osceola County Community Development Department Citation2015). Upstream urbanization and agriculture led to increases in nutrient loading in some lakes. Point-source inputs had pronounced effects on Lake Tohopekaliga, which in turn affected Lake Cypress (Huber et al. Citation1982, Dierberg et al. Citation1988, James et al. Citation1994, Williams Citation2001). Subsequent channelization of the Kissimmee River by the Army Corps of Engineers in the 1960s had adverse ecological and water-quality consequences for Upper Basin lakes. Current management and nutrient mitigation of lakes on the Kissimmee Chain are important parts of the restoration initiative for the Florida Everglades, the largest Ramsar Convention wetland complex in the United States.
Paleolimnology has a well-established role in ecosystem management because it provides long-term environmental information that is often undocumented (Smol Citation1992, Brenner et al. Citation1993, Battarbee and Bennion Citation2011, Bennion et al. Citation2011, Davidson and Jeppesen Citation2013, Larocque-Tobler Citation2016). Predisturbance conditions in lakes of the Upper Kissimmee Basin are unknown, which has posed a challenge for establishment of appropriate restoration targets for the lakes. This study used paleolimnological methods to analyze a total of 18 sediment cores from Lakes Kissimmee, Cypress, Marian, Jackson, Tohopekaliga, and East Tohopekaliga on the Kissimmee Chain (). The objective was to describe water quality and ecological conditions, including aquatic plants, algae, and cyanobacteria, during reference periods that represented low human impact on the lakes during the early 1800s, and to document subsequent changes. Additionally, the purposes were to assess changes in nutrient deposition rates, and to determine whether associated wetlands were present that might have contributed significant amounts of colored dissolved organic carbon to the lakes and attenuated algal production. Our intention was to assist the Osceola County Public Works Department and the Florida Department of Environmental Protection (Florida DEP) in defining appropriate restoration goals for the lakes.
Figure 1. Location of the 6 study lakes in the Upper Kissimmee Basin. Modified from a map prepared by Kissimmee Division, Ecosystem Restoration Department, South Florida Water Management District (Citation2005).
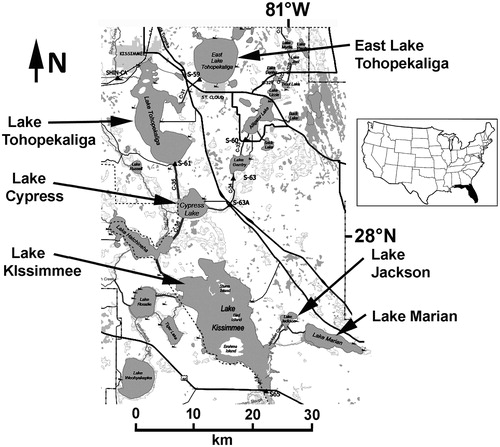
Study sites
Lakes on the Kissimmee Chain are managed by the South Florida Water Management District, the US Army Corps of Engineers, the Florida Fish and Wildlife Conservation Commission, the Osceola County Public Works Department, the Florida DEP, and the US Fish and Wildlife Service. Water quality is highly variable, but recently measured values are shown in . All lakes have high dissolved color values, and therefore are subject to target chlorophyll a values of 20 µg/L (Florida DEP Citation2013). Lakes Cypress, Jackson, Kissimmee, and Marian are considered impaired because limnetic chlorophyll a values exceed this standard, whereas East Tohopekaliga and Tohopekaliga are not impaired (Osceola County Community Development Department Citation2015). Because of their better water quality, East Tohopekaliga and Tohopekaliga are designated Class III lakes that are protected for fish consumption, recreation, and wildlife. Tohopekaliga, however, demonstrated extreme hypereutrophic conditions in recent decades (Huber et al. Citation1982). Lakes Tohopekaliga and Cypress require management of invasive Hydrilla. Lake Cypress is impaired in terms of total phosphorus and total nitrogen concentrations, as well as for chlorophyll a. East Lake Tohopekaliga does not require management to control Hydrilla, but it has extensive vegetation that creates problematic floating tussocks. Although Lake Jackson is impaired, it is nearly surrounded by the Three Lakes Wildlife Management Area and by private agricultural lands. Lakes Marian and Kissimmee sustain the highest limnetic total P and chlorophyll a concentrations (). Lake Kissimmee was placed on the federal list of impaired waters in 2006, and it is subject to management for invasive Hydrilla.
Table 1. Coring locations and modern mean water quality for study lakes.
Historical influences on the lakes
In 1884, Lake Topekaliga and other lakes along the Kissimmee River were described as having “pure, clear water” (Atlantic and Gulf Coast Canal and Okeechobee Land Company 1885). Prairie lands and hardwood forests were situated along the lake shores, with large tracts of cypress (Taxodium) in intermittent swamps. Sawgrass (Cladium jamaicense), organic-rich sediments, and aquatic plants were reported in wetlands near East Lake Tohopekaliga. A continuous marsh extended from Lake Cypress to the north bay of Lake Kissimmee.
Cattle ranches were well established in the Kissimmee Basin by the 1860s (Otto Citation1985). More extensive impacts began with settlement after the Civil War (c. 1865), when the region was further developed for agriculture and cattle ranching. Kissimmee City was founded on the northern shore of Lake Tohopekalgia in 1883, and the city of St. Cloud was founded in 1909 on the southern shore of East Lake Tohopekaliga.
Canal construction to join Upper Basin lakes to the Kissimmee River began in the 1880s. The St. Cloud Canal was dug through marshes to drain East Lake Tohopekaliga into Lake Tohopekaliga. Prior to 1884, East Lake Tohopekaliga was surrounded by cypress swamps and marshes that were left stranded above water levels when the lake was lowered, and logging took place on the shores (Atlantic and Gulf Coast Canal and Okeechobee Land Company 1885). East Lake Tohopekaliga fell 1.1 m in 30 d from the drainage. Sugar-cane agriculture was established in drained areas of East Lake Tohopekaliga by 1883. On the western and southern shores of Lake Tohopekaliga, dredging of wetlands and logging occurred in 1883 and 1884 (Atlantic and Gulf Coast Canal and Okeechobee Land Company 1885). Lake Tohopekaliga dropped 0.8 m from drainage, and lakes south of it had lowered water levels. The water level of Lake Okeechobee fell 0.45 m in 1 year.
Population increases occurred after World War II in the cities of Kissimmee and St. Cloud. Point-source discharges into Lake Tohopekaliga began in the 1950s (Williams Citation2001). By 1969, 4 wastewater treatment plants discharged 82.5 metric tons of phosphorus and 140 metric tons of nitrogen per year into Lake Tohopekaliga and its tributaries. Water-quality deterioration was evident by 1969. By 1982, limnetic total P averaged 322 μg/L and chlorophyll a averaged 71 μg/L in Lake Tohopekalika (Huber et al. Citation1982), and nutrients affected lakes that were downstream. In 1988, wastewater discharge into the lake was discontinued.
Massive flooding in 1947 after a hurricane prompted the Army Corps of Engineers to deepen and straighten the Kissimmee River to implement flood-control measures (South Florida Water Management District Citation2014). In the 1960s, the river was extensively channelized, which had serious consequences in terms of shortened water-residence times, higher nutrient concentrations, and ecological impacts on fish and wildlife in Upper Basin lakes and in Lake Okeechobee. In 1999, the South Florida Water Management District and the US Army Corps of Engineers began the Kissimmee River Restoration Project, which is scheduled to restore 71 km of the natural meandering system by 2020.
Recent water-level manipulation for sediment removal
Florida Fish and Wildlife Conservation Commission has lowered water levels and dewatered sediments in several of our study lakes to remove invasive plants and sediment by burning and scraping operations in recent years. Water levels in Lake Tohopekaliga were drawn down ∼1.8 m in 1971, 1979, 1987, and 2004, and scraping occurred in 1987 and 2004. During the 2004 operation, approximately 1350 ha of lake bottom was exposed, and 6.04 million m3 of organic material was removed (Lawrence K, Osceola County Public Works Department, March 2016, pers. comm.). In 1995, Lake Kissimmee was drained to half the size of its normal area for scraping and burning. Because Lake Cypress is controlled by the structure in Lake Kissimmee, its water level also fell. In 2004, East Lake Tohopekaliga was drawn down approximately 1.8 m, and 95% of the littoral zone was scraped. Drawdowns and sediment removal continue as a management practice in lakes of the Kissimmee Chain.
Materials and methods
Field methods and core collection
We conducted a systematic spatial survey of sediment distribution (Whitmore et al. Citation1996) in Lakes Cypress, East Tohopekaliga, Kissimmee, Jackson, Marian, and Tohopekaliga to identify optimal coring sites. Water depth at each site was measured by lowering a weighted, metered steel cable to the sediment/water interface. Total depth to hard, sandy sediments was measured at each site by inserting magnesium/zirconium rods into the sediment and subtracting the water depth to estimate soft-sediment thickness. Sediment cores of ∼1 m in length were recovered with a 1.83-m-long, 7-cm diameter piston corer (Fisher et al. Citation1992), and backup cores for pollen and macrofossil analyses were taken at the same stations using a 4-cm diameter, 1.83-m long cellulose acetate butyrate piston corer.
Cores were sectioned in the field at 5-cm intervals to 20 cm depth, then at 3-cm or 4-cm intervals to the base of the cores. Samples were stored in Nalgene plastic cups in the dark on ice until they were refrigerated in the laboratory. Diatom samples were collected in the field at the top of each sectioned interval and were preserved with alcohol.
Lead and cesium dating and core chronologies
210Pb dating of all sediment cores was performed at University College London by gamma spectrometry using an ORTEC HPGe GWL series well-type coaxial low-background intrinsic germanium detector (Appleby et al. Citation1986, Schelske et al. Citation1994). Unsupported 210Pb activity was calculated by subtracting 226Ra, as estimated from 214Pb, from the total 210Pb activity. 210Pb was determined via its gamma emissions at 46.5 keV, and 226Ra by the 295 keV and 352 keV gamma rays emitted by its daughter isotope 214Pb. 137Cs and 241Am, which are artificial radionuclides from weapons testing, were measured by their emissions at 662 kev and 59.5 kev to provide absolute time markers for c. 1963 (Appleby et al. Citation1986). Corrections were made for the effect of self-absorption of low-energy gamma rays within the sample (Appleby et al. Citation1992). For Lake Marian core 31_III_2018_MAR_328, radioisotopic analyses stopped just short of 210Pb supported levels, so excess 210Pb activity was extrapolated by linear regression for 2 samples. Sediment age/depth relationships were calculated using the constant rate of supply model (Appleby and Oldfield Citation1983), with occasional age adjustments based on the location of 137Cs peaks.
Diatom analyses
Diatom samples were digested in the laboratory with 35% H2O2 and K2Cr2O7 according to Van der Werff (Citation1953), and slides were prepared with Naphrax mounting medium. Approximately 500 valves were counted and identified at each level at 1500× magnification using compound microscopy. Diatoms were identified using standard floras (Patrick and Reimer 1966–1975, Krammer and Lange-Bertalot Citation1986–1991) and taxonomic websites (Diatoms of North America Citation2020). Zonation of diatom assemblages in sediment cores was determined by constrained incremental sum of squares (CONISS) hierarchical agglomerative cluster analysis using the statistical program R and the rioja package (Juggins Citation2012). The number of significant zones in each cluster analysis was determined with a broken-stick model (Bennett Citation1996) using the rioja package.
Historic water quality inferences were obtained with 2 diatom-based inference models. The models were constructed with a calibration set of 75 Florida lakes that we utilized in past studies (Riedinger-Whitmore et al. Citation2005, Kenney et al. Citation2014, Whitmore et al. Citation2015), but the models were updated slightly in the present study. Limnetic total P concentrations were inferred by weighted averaging inverse tolerance regression using log10 limnetic total P values and a calibration of 71 P-limited or nutrient-balanced lakes (r2 = 0.880, root mean square error [RMSE] = 0.186 log10 total P). In some lakes that have very high limnetic total P values, particularly those that have been N-limited, the limnetic total P model will not perform reliably. Consequently, N-limited lakes were removed from the calibration set for that model. Limnetic chlorophyll a concentrations were inferred by weighted averaging inverse tolerance regression using log10 limnetic chlorophyll a values for a calibration set of 73 lakes (r2 adj. = 0.831, RMSE = 0.265 log10 limnetic chlorophyll a). Statistical analyses for all diatom-based inferences were performed using R version 3.0.2 software (R Core Team Citation2014) and the package rioja (Juggins Citation2012). In the calibration set of lakes, the correlation coefficient between log total P and log chlorophyll a (the inferred variables) is approximately 0.86 (P < 0.001), so the models are interdependent and their inferences are not reported simultaneously. Selection of the appropriate model and model performance were evaluated by comparing water quality inferences with measured water quality during recent periods.
We used analog matching with dissimilarity cutoffs determined by Bray–Curtis (10% cutoff) and Monte Carlo simulation methods (cutoff = 0.617) to assess whether there were suitable analogs for the sediment core samples among lakes in the calibration set. Sediment cores that did not have sufficient analogs to interpret significant parts of their histories were interpreted by qualitative methods only. Analog matching was performed using R Version 3.0.2 software (R Core Team Citation2014) and the package rioja (Juggins Citation2012).
Sedimented algal and cyanobacterial pigment analyses
Approximately 7–10 g of wet sediment was taken from archived sediment samples for pigment analyses. Pigments were extracted using acetone, following Swain (Citation1985), Riedinger-Whitmore et al. (Citation2005), and Whitmore et al. (Citation2018). Acetone was added to sediment samples, and samples were shaken on a Thermo Scientific MaxQ 2000 shaker table following each acetone addition for approximately 30 mins. Samples were centrifuged at low speed (2000–3000 RPM, IEC HN SII centrifuge) for ∼10 mins, and the supernatant from each sample was removed using an adjustable volume pipette.
We assayed percent organic matter content of samples from all cores by loss on ignition at 550 C (Heiri et al. Citation2001). Loss-on-ignition data were used to estimate the organic matter content per gram wet sediment for each sample, and to determine pigment concentrations. Chlorophyll derivatives were estimated by measuring extracts at 665 nm (Swain Citation1985) using a Hitachi U-2800A ultraviolet–visible (UV-VIS) spectrophotometer, and are reported as absorbance per gram organic matter. Percent native chlorophyll, an indicator of pigment degradation in samples, was determined by measuring absorbance at 665 nm before and after acidification with HCl. Subsamples for total carotenoids were saponified using a 20% KOH/methanol mixture, extracted into petroleum ether, and measured at 448 nm. Total carotenoids were reported as absorbance per gram organic matter.
Subsamples for oscillaxanthin and myxoxanthophyll cyanobacterial pigments were extracted into petroleum ether, air dried, and redissolved in ethanol. Absorbance was measured at 412, 504, and 529 nm, and the trichromatic method was used to correct for phorbin contamination and to calculate oscillaxanthin and myxoxanthophyll at the major peaks of absorbance for each pigment. Pigment concentrations were expressed as micrograms pigment per gram organic matter.
Sediment chemistry analyses
Total P was assessed by colorimetry following EPA Method 365.1 for 10 cores in the IFAS Soil and Water Science Department Analytical Research Lab at the University of Florida (UF), Gainesville, FL, and for 8 cores on a Spectro Acros 2 ICP by Waters Agricultural Laboratory in Waycross, GA, using AOAC Method 957.02. Total N, total C, and stable isotope (δ13C and δ15N) analyses of organic matter were performed at the University of Florida Department of Geological Sciences on freeze-dried sediment. A Thermo Electron DeltaV Advantage isotope ratio mass spectrometer coupled with a ConFlo II interface linked to a Carlo Erba NA 1500 CNHS Elemental Analyzer was used for these analyses. Sample gases were measured relative to laboratory reference N2 and CO2 gases. CO2 values were standardized to CMUF REF (Carrara Marble–University of Florida Reference Gas), and N2 values were standardized to UF-N2 REF, both of which are UF in-house reference standards. δ13C was expressed as per mil (‰) deviation from the Vienna PeeDee Belemnite (VPDB) limestone standard, and δ15N was expressed as per mil (‰) deviation from the atmosphere.
Pollen, macrofossil, and ostracod analyses
Pollen and macrofossil analyses were performed on a minimum of 5 samples from each sediment core around the depths that corresponded to the predisturbance reference periods to determine the nature of reference aquatic-plant communities, and to investigate the presence of associated wetlands. The number of samples analyzed was limited by funding constraints, but analyses for 2 entire cores were provided voluntarily by student investigators on our projects.
Preparation of pollen samples followed Faegri and Iversen (Citation1989) and Bennett and Willis (Citation2001). Deflocculation of samples was done using a dilute detergent solution. A 10% HCl solution was added to remove carbonates. Samples were boiled in a KOH (or NaOH) solution to remove humic acids and unsaturated organic soil colloids. Samples were then washed through a 0.2-mm sieve with deionized water to remove coarse mineral particles and large organic matter. The supernatant was decanted into distilled water, and then a few drops of 10% HCl were added to prevent the formation of the precipitant Zn(OH)2. Remaining cellulose and organics were removed by acetolysis with anhydric acetic acid (9 parts) and H2SO4 concentrate (1 part), and samples were centrifuged at 1500 rpm for 20 min. Samples were washed with glacial acetic acid and heated briefly in 10% KOH to halt acetolysis and to prepare them for staining. Semipermanent mounts with silicone oil were prepared for microscopic analysis at 400×. Pollen was identified using standard references, including Willard et al. (Citation2004).
Macrofossil analysis was performed following methods outlined by Birks (Citation2017). Approximately 20–50 cm3 of sediment was measured for each sample by water displacement in a graduated cylinder, and then the sample was soaked in 10% sodium hexametaphosphate [(NaPO3)6] to disperse sediment particles from macrophyte remains. The sediment and deflocculant mixture was rinsed through sieves with screen sizes of 1 mm, 500 μm, 250 μm, and 125 μm using a shower-head attachment that distributed the point of water impact to reduce damage to the macrofossils. The 1-mm screen allowed for separation of larger seeds and macrophyte parts, and the 125-μm screen was used for recovery of small material, including small seeds and characeaen oospores. Screened material was suspended in water in a petri dish for examination under a dissecting binocular microscope. Identification was performed with the use of a macrofossil reference collection at the University of South Florida St. Petersburg.
Ostracods also were examined using stereomicroscopy in samples that had been cleaned and screened for macrofossil analysis and that disclosed the presence of ostracods. Ostracods were identified using references that included Furtos (Citation1936), Keyser (Citation1977), Teeter (Citation1980), and Pérez et al. (Citation2010).
Stable carbon isotopes versus C/N ratio plots
We plotted δ13C values versus C/N ratios of organic matter in sediment cores to investigate changes over time in the primary producer communities that contributed organic matter to the sediments. We included in those plots some representative δ13C and C/N values for aquatic macrophytes, algae, and cyanobacteria. Our intention was not to determine which specific taxa were present in the past, but rather we sought to assess general changes in past macrophyte life-form groups and algal/cyanobacterial populations. Funding did not permit analysis of δ13C and C/N values of modern plants and algae in lakes of our study, so we used published δ13C values for primary producer taxa and functional groups collected from similar highly productive Florida lakes. Floating-leaved and emergent plants, as well as surface bloom-forming algae and cyanobacteria, use atmospheric CO2 as a carbon substrate (δ13C about −8‰; Gu and Alexander Citation1996), so δ13C values of organic matter derived from those groups should be similar across lakes. In contrast, the δ13C values of submerged primary producers can vary across lakes as a function of the δ13C of dissolved inorganic carbon in the water, which is influenced by carbonate dissolution in the watershed, inputs of allochthonous carbon, and the in-lake rate of primary production (Brenner et al. Citation1999). Most of the δ13C and C/N data we used came from the Brenner et al. (Citation2006) study of Lake Panasoffkee, Sumter County, Florida, a shallow, eutrophic lake that lies in karst bedrock, similar to the lakes of our study. Lake Panasoffkee receives substantial amounts of bicarbonate-rich groundwater input. Thus, our plots of δ13C versus C/N values display relative positions for the modern taxa, and stratigraphic changes in the sedimented organic matter values in cores are interpreted in general terms with respect to those taxa.
Brenner et al. (Citation2006) reported δ13C and C/N values for Typha, Sagittaria, Hydrocotyle, Pontederia, Scirpus, Salix, Nymphaea, Ceratophyllum, Najas, Potamogeton, Vallisneria, and Hydrilla, and for periphyton and epiphytic algae. Stable isotope values for submerged aquatic primary producers can vary seasonally, but that study reported averages from summer and winter seasons, which displayed little difference. We used δ13C values for Pistia from the Florida Everglades (Wang et al. Citation2002), and we assumed a C/N ratio similar to that for Nymphaea, because both genera have similar structural tissue for the floating life form (much aerenchyma tissue). We found few published δ13C values for cyanobacteria in our region, and those displayed a wide range depending on taxonomic affinity and location in the water column. Rosen et al. (Citation2017) reported that a 2016 cyanobacterial surface bloom on Lake Okeechobee was caused mostly by Microcystis aeruginosa, so we used the reported δ13C value for that species (Gu and Alexander Citation1996), which is distinctly negative compared with subsurface algae and cyanobacteria as a consequence of atmospheric CO2 fixation. We assumed a C/N ratio the same as that for algae (<∼10).
Results
Field survey and core collection
Sediment distribution is highly variable in shallow Florida lakes because of resuspension and transport. These processes can affect the completeness of sediment records (Whitmore et al. Citation1996). Sediment surveys and replicate cores were needed to ensure that one or more intact historical records would be obtained from each lake. In addition, lake drawdowns and sediment removal operations in recent decades posed significant risks that portions of records would be missing.
Sediments were probed at 62 stations in Lake Cypress, 59 stations in East Lake Tohopekaliga, 34 stations in Lake Jackson, 50 stations in Lake Kissimmee, 26 stations in Lake Marian, and 74 stations in Lake Tohopekaliga to find suitable coring sites. In total, 18 primary cores were taken from the 6 lakes between November 2016 and March 2018. Three cores were retrieved from Lake Cypress, 3 from East Tohopekaliga, 3 from Lake Kissimmee, 2 from Lake Jackson, and 2 from Lake Marian. Five sediment cores were collected from Lake Tohopekaliga: one from the northern basin, 2 from the central basin, and 2 from the southern basin.
We selected one core to represent each study lake in this article because including all 18 cores would have been too excessive for presentation and would not have been more informative. Many cores did not yield complete records. We used sediment dating results to select cores that had more intact profiles, and evaluated that based on radioisotopic activities and total 210Pb inventories, among other evidence. Some cores contained more sand lenses than other cores, and diatom breakage was occasionally too extensive for interpretation in portions of the profiles. Sediment records showed good concurrence within lakes, but certain records were more intact and informative. We chose the most intact record to represent each of the 6 lakes, but occasionally explain that some evidence, such as Ruppia seeds or ostracods, were more abundant in other cores that had less complete records. We also chose cores from the central portions of basins for this presentation rather than cores from peripheral areas and embayments.
Lake Cypress
Radioistopic activities for Lake Cypress core 29_XII_2017_CYP_3 are shown in , and dates are shown in Table S1. In the lower 60 cm of Lake Cypress core 29_XII_2017_CYP_3 (), prior to the 1880s, salt-tolerant diatom taxa such as Mastogloia smithii, Campylodiscus clypeus, and Anomoeoneis sphaerophora var. sculpta indicated that extremely low water levels occurred at times (Whitmore et al. Citation2018). These taxa co-occurred with Cyclotella meneghiniana, a eutrophic species (optimum total P = 61 µg/L; Whitmore et al. Citation2018) that is less salt-tolerant. Mixed assemblages suggested extreme variations in water levels. Excessive diatom breakage, which precluded quantitative assessment, occurred several times in predisturbance samples of one core from Lake Cypress, and it was associated with brief periods of dry conditions prior to the 1880s. Constrained incremental sum of squares hierarchical agglomerative cluster analysis (CONISS) and the broken-stick model showed that a statistically significant change in diatom assemblages occurred c. 1881 (). Salt-tolerant taxa disappeared in all 3 cores around the time of engineered water-level changes, after which there was a transition to dominance by Aulacoseira granulata, a eutrophic species (optimum total P = 45 µg/L; Whitmore et al. Citation2018) that requires open waters for suspension in the water column. Another change occurred in the early 1900s to dominance by hypereutrophic, tychoplanktonic Pseudostaurosira brevistriata and Staurosira construens var. venter (optimum total P, respectively, = 127 µg/L and 89 µg/L), which our studies have shown is often associated with cyanobacteria in the water column (Whitmore et al. Citation2018). Tychoplanktonic taxa spend much of their life histories growing on surfaces, but they are intermittently suspended in the water column by waves and currents. Diatom-based water quality inferences from Lake Cypress were not available because the mixed salt-tolerant assemblages in the lower halves of the cores had no analogs among lakes in the calibration set (Table S1).
Figure 2. Diatom profile with CONISS zonation for core 29_XII_2017_CYP_3 from Lake Cypress. Lower panels show sedimented algal and cyanobacterial pigment profiles from Lake Cypress core 18_XI_2016_CYP_40.
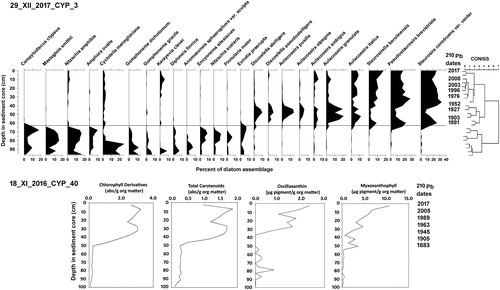
Pigment concentrations in the sediments are shown for core 18_XI_2016_CYP_40 (). Chlorophyll and carotenoid pigment values were stable below 50 cm in the core, then increased rapidly more than 3-fold after 1883 when Lake Cypress was connected to the Kissimmee Chain by canals. The cyanobacterial pigments oscillaxanthin and myxoxanthophyll were present occasionally prior to 1945, then increased more than 10-fold to the present time (). Total P deposition rates increased 19-fold, and total N increased 3.5-fold since c. 1855.
The plot of δ13C versus C/N ratios showed that sedimented carbon at the base of the core was composed primarily of submerged aquatic plants and epiphytic algae (). Sediment carbon sources initially were largely floating-leaved vegetation, and then in the top 40 cm of the core (since c. 1945), sources were cyanobacteria and/or algae that used atmospheric CO2 for photosynthesis. In predisturbance samples of core 18_XI_2016_CYP_40, Taxodium (cypress) pollen represented about 5% of the assemblage, which was a low-level indication that there were associated swamps in the area. Taxodium pollen was comparatively rare in the other 2 cores (Table S7). Pollen from other wetland taxa Morella (bayberry), Nyssa (water tupelo), and Salix (willow) also were present. Pollen data from the predisturbance period indicated the presence of Pontederia, Typha, Sagittaria, Nymphaea, Nuphar, and Myriophyllum. Macrofossil analyses showed abundant Chara in lowermost predisturbance samples, which was evidence of relatively clear waters (Table S13). Chara declined rapidly prior to the 1880s and was replaced by Nymphaea, a shift that often occurs with eutrophication and diminished light availability (Blindow Citation1992, Sayer et al. Citation2010a, Citation2010b, Bennion et al. Citation2018). Plant macrofossils during the predisturbance period also included remains of Najas and Potamogeton.
Figure 3. Plots of δ13C versus C/N ratios in sedimented organic matter for cores from Lakes Cypress, East Tohopekaliga, Jackson, and Tohopekaliga, the 4 lakes that showed prominent changes in primary producer communities over time. Specific taxa are placed in relative positions based on δ13C and C/N values reported for studies of other Florida lakes, and are used here to provide general indication of trends in community change.
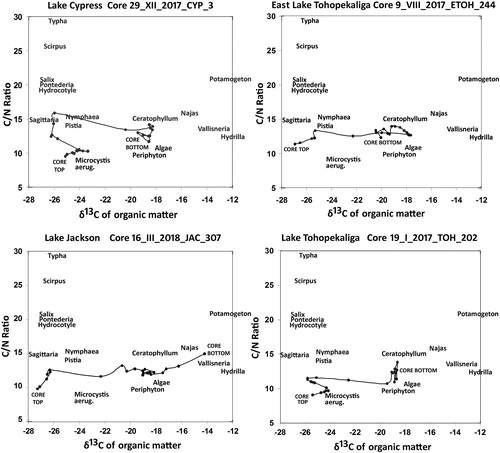
East Lake Tohopekaliga
Radioistopic activities for East Lake Tohopekaliga core 9_VIII_2017_ETOH_244 are shown in , and dates are shown in Table S2. In profiles of 2 cores from East Lake Tohopekaliga, diatoms were nearly absent from segments of ∼10 cm in each record because of substantial breakage. This breakage occurred in core 9_VIII_2017_ETOH_244 around the 25–35 cm depth (). Diatoms below that level, principally those toward the left in , were largely benthic and epiphytic taxa. Several benthic taxa below the 30-cm level were noteworthy for high salinity tolerances, and those included Nitzschia scalaris, Mastogloia smithii, Anomoeoneis sphaerophora var. sculpta, and particularly Campylodiscus clypeus. Prior to 1879, salt-tolerant diatoms co-occurred with Cyclotella meneghiniana and Aulacoseira ambigua, which are eutrophic indicators (A. ambigua optimum total P = 49 µg/L; Whitmore et al. Citation2018) that typically occur as plankton in the open-water limnetic zone of lakes. These mixed assemblages suggested extreme fluctuations in water levels. An increase to a high percentage (72%) of C. clypeus above the 40-cm level indicated that the large preservation hiatus in was a prolonged low water-level event. CONISS showed statistically significant changes in diatom assemblages after c. 1879 (). These changes occurred around the time (1883) that water levels were changed permanently by canal construction. Dominance shifted to planktonic Aulacoseira granulata until c. 1980, then to tychoplanktonic Pseudostaurosira brevistriata and Staurosira construens var. venter, which indicated persistent cyanobacteria (Whitmore et al. Citation2018). Diatom-based water quality inferences for the early history of East Lake Tohopekaliga were not available because mixed salt-tolerant assemblages in the lower portions of the cores resulted in those samples having no analogs in the calibration set (Table S2).
Figure 4. Diatom profile with CONISS zonation for core 9_VIII_2017_ETOH_244 from East Lake Tohopekaliga. Lower panels show sedimented algal and cyanobacterial pigment profiles.
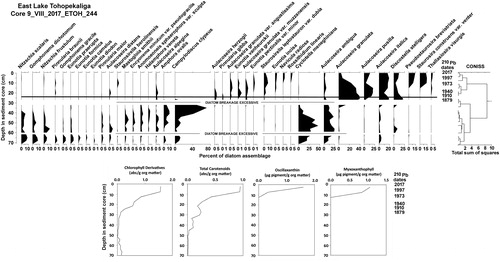
Percent native chlorophyll values for core 9_VIII_2017_ETOH_244 indicated fairly consistent pigment preservation throughout the core. Chlorophyll derivatives and total carotenoid values were low below the 45-cm level in the core (no date available), then increased from c. 1879 to the 1970s (). Myxoxanthophyll and oscillaxanthin cyanobacterial pigments appeared only above the 10-cm level (c. 1973: ), which was consistent with the timing of diatom indication of cyanobacteria influence.
δ13C and C/N ratios () showed that carbon deposited in predisturbance basal sediments was largely from submerged vegetation and epiphytic algal sources. Sedimented carbon sources progressed to floating-leaved vegetation toward the core top, and a recent decrease in C/N ratios possibly indicated cyanobacterial influence. Pollen grains in predisturbance samples were generally sparse, and Taxodium (cypress) and Morella (bayberry) pollen were low in abundance despite the reported presence of associated swamps before the 1880s. Pollen grains, however, did show evidence of emergent and floating-leaved Pontederia, Sagittaria, Nymphaea, Nuphar, and sedges, as well as submerged Myriophyllum and Potamogeton. Macrofossil evidence from predisturbance samples showed abundant Chara, which indicated clear or shallow waters. In the 41–44-cm sample from the core, seeds of Ruppia maritima were observed, which indicated very high solute concentrations prior to c. 1865.
Lake Jackson
Below the 70-cm level in core 16_III_2018_ JAC _307 (), salt-tolerant diatoms were present as in Lakes Cypress and East Tohopekaliga. These taxa included Nitzschia scalaris, Anomoeoneis sphaerophora var. sculpta, Campylodiscus clypeus, and Halamphora coffeaeformis. These species co-occurred with eutrophic taxa that included Cyclotella meneghiniana, Aulacoseira ambigua, and A. granulata, with the latter two being planktonic forms that indicated deeper limnetic waters. Water-level fluctuations were suggested by these mixed assemblages. Diatoms between the 70- and 40-cm levels showed extensive breakage that suggested a long period of extremely low water levels, as in East Lake Tohopekaliga. Radioistopic activities for core 16_III_2018_ JAC _307 are presented in . Unsupported 210Pb activity showed an abrupt decline at the 12.5-cm level (c. 1975), which suggested a possible hiatus caused by sediment transport. As a result, we adopted a conservative approach and rejected the calculated 210Pb dates, and assigned a nominal reference date of “c. 1900” to the depth at which unsupported 210Pb activity reached zero. The error term for excess 210Pb activity in the 20–24-cm interval was greater than the excess 210Pb activity value, so we set the date of “c. 1900” for 24 cm depth in the core. Diatom assemblages changed significantly above the section of extensive diatom breakage. The planktonic, eutrophic taxa Aulacoseira ambigua and A. granulata became dominant just before c. 1900, and salt-tolerant taxa no longer were present. Diatom assemblages near the top of the core showed an increase in some periphytic taxa (e.g., Karayevia clevei) without prominent indication of change in water quality, and the tychoplanktonic taxa that we associate with competitive exclusion by cyanobacteria were not present. As in Lakes Cypress and East Tohopekaliga, quantitative water quality inferences were not available because salt-tolerant assemblages in the lower portions of the cores resulted in samples having no analogs in the calibration set (Table S3). Equivocal dates did not permit estimation of changing rates of nutrient deposition.
Figure 5. Diatom profile for core 16_III_2018_ JAC _307 from Lake Jackson. Lower panels show sedimented algal and cyanobacterial pigment profiles. CONISS was not performed because of the broad gaps in diatom presence.
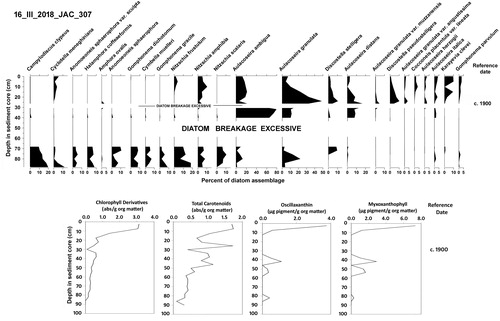
Chlorophyll derivative values were low (<1.0 abs/g org matter) in sediments deposited prior to c. 1900 (∼30 cm) in core 16_III_2018_JAC_307, which indicated low historical algal productivity (). Chlorophyll derivatives and total carotenoid concentrations increased to ∼2–3 abs/g above the 20-cm level. Percent native chlorophyll, the indicator of pigment preservation quality, declined in the upper 28 cm of the core, which showed that pigment concentration increases were not an artifact of preservation. Myxoxanthophyll and oscillaxanthin cyanobacterial pigments were present occasionally at low concentrations below the 15 cm level in the core, but they increased to 7.3 and 3.8 μg/g organic matter, respectively, in the top 10 cm.
δ13C versus C/N ratios () indicated that carbon deposited in sediments at the bottom of the core was largely from submerged aquatic vegetation that might have included Vallisneria, Ceratophyllum, and Najas, as well as carbon from algae or periphyton. Sedimented carbon signatures changed to sources that probably included floating-leaved vegetation and algae/cyanobacteria toward the core top. Pollen analysis of predisturbance samples at 70–100 cm deep in the core showed very low numbers of grains of the wetland Taxodium (cypress), Nyssa (water tupelo), Salix (willow), and Morella (bayberry), but pollen was present from grasses and asters, such as are found in natural grasslands and dry prairies in the vicinity of the lake today (Table S9). Pollen analysis revealed Myriophyllum in predisturbance samples, and plant macrofossils included Chara, Najas, and some seeds of Nymphaea. Macrofossil remains showed that Chara was abundant in the 80–85 cm sample, but disappeared above that level in the core (Table S15).
Lake Tohopekaliga
Lake Tohopekaliga core 19_I_2017_TOH_202 was retrieved in the central basin of this 3-lobed lake. Radioistopic activities for core 19_I_2017_TOH_202 are shown in , and dates are shown in Table S6. Diatom assemblages below the 60-cm level contained a large number of planktonic taxa that included Cyclotella meneghiniana, Synedra radians, and Cyclotella distinguenda. In addition, these samples included Anomoeoneis sphaerophora var. sculpta, Mastogloia smithii, M. braunii, and M. smithii var. lacustris, which indicated high solute concentrations that resulted from low water-level conditions (). We interpreted these mixed assemblages as being caused by large fluctuations in water level prior to the 1890s. CONISS and the broken-stick model showed a statistically significant change in diatom assemblages above the 60-cm level (c. 1890), and taxa indicative of high salinity conditions were no longer present. Eutrophic and planktonic species of Aulacoseira spp. became dominant around the 50-cm level in the core (c. 1916). After c. 1931, diatom dominance shifted to more highly eutrophic benthic taxa that included Pseudostaurosira brevistriata, Staurosirella berolinensis, and Staurosira construens. This transition suggested increased influence of cyanobacteria on planktonic diatom assemblages (Whitmore et al. Citation2018). Salt-tolerant diatoms below the 50-cm level resulted in samples that had no analogs in the calibration set (Table S6), so quantitative water quality inferences were not available.
Figure 6. Diatom profile with CONISS zonation for core 19_I_2017_TOH_202 from Lake Tohopekaliga. Lower panels show sedimented algal and cyanobacterial pigment profiles.
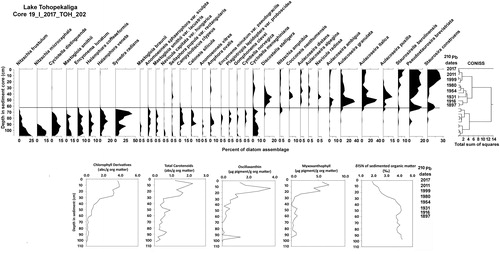
Chlorophyll derivative concentrations were <0.3 abs/g organic matter below the 65-cm level (prior to 1897), and they increased steadily to about 4.0 abs/g organic matter at the top of the core (). Total carotenoid concentrations varied around 0.5 abs/g organic matter below the 40 cm level in the core (c. 1950), then increased to 1–2 abs/g organic matter in the topmost samples. Oscillaxanthin and myxoxanthophyll cyanobacterial pigments were present and variable around 0.1–1 μg pigment/g organic matter below the 40 cm level, then increased to ∼2 μg pigment/g organic matter of oscillaxanthin and 5 μg pigment/g organic matter of myxoxanthophyll in the topmost samples. Although δ15N values of sedimented organic matter tended to be unchanged in all other lakes of this study, δ15N values in Tohopekaliga core 19_I_2017_TOH_202 showed a decline () that is a typical response to nitrogen-fixing cyanobacteria (Brenner et al. Citation1999, Riedinger-Whitmore et al. Citation2005). The decline in δ15N values was consistent with diatom evidence of increased cyanobacteria presence (Whitmore et al. Citation2018).
δ13C versus C/N ratios indicated that submerged aquatic vegetation, periphyton, and algae were important sources of sedimented carbon prior to 1897, but signatures changed rapidly thereafter to reflect sources that included cyanobacteria, algae, and possibly floating-leaved vegetation ().
Pollen from grasses, sedges, and asters in predisturbance samples (∼100–60 cm depth) indicated that marshes or open grasslands were present in the vicinity (Table S12). Pollen from wetland taxa, which included Taxodium, Salix, and Morella, were present only in very low numbers. Pollen from Pontederia, Myriophyllum, and Typha domingensis were present. Plant macrofossils in predisturbance samples (∼113–60 cm depth) showed abundant Chara, which indicated clear or shallow waters. Nymphaea appeared around 65 cm depth in the core as Chara declined (Table S18). Macrofossil analyses also showed Ruppia maritima seeds in predisturbance sediments of 3 cores from Lake Tohopekaliga, which indicated periods of very high solute concentrations during predisturbance times. Ruppia appeared in 2 cores from the southern basin and in one core from the central basin. In one core from the southern basin, up to 111 Ruppia seeds were found in analyzed samples.
Ostracods showed evidence of very high solute concentrations as a result of extreme water-level fluctuations during predisturbance times. In the 113–89 cm levels of core 19_I_2017_TOH_202, freshwater to oligohaline ostracods included Darwinula stevensoni, Cyprideis salebrosa, Physocypria krapelini, Limnocythere floridensis, Limnocythere inopinata, and Cypridopsis vidua. Several of these taxa plus Cypretta brevisaepta and Cypridopsis okeechobei were present in predisturbance samples from a core in the southern basin. Ostracods were not observed again after the 1890s in any sediment samples. Their disappearance likely resulted from stabilized water levels that precluded high dissolved solute concentrations, but reduction of macrophytes and increased sediment deposition also might have contributed to their decline (Keyser Citation1977, Holmes Citation1992, Ruiz et al. Citation2013). Total P accumulation rates increased 4- to 12-fold in the central basin, and total N accumulation rates increased 4- to 23-fold.
Lake Kissimmee
Lake Kissimmee core 27_XII_2017_KIS_2 was retrieved from the main basin of the lake. Radioistopic activities for core 27_XII_2017_KIS_2 are shown in , and dates are presented in Table S4. The diatom assemblage profile () shows that Aulacoseira spp. were present at ∼10% of assemblages or greater throughout the entire core. Pseudostaurosira brevistriata and Staurosira construens var. venter, which are most abundant during advanced eutrophication with cyanobacteria (Whitmore et al. Citation2018), were present consistently at percentages similar to Aulacoseira spp. These diatoms indicated that Lake Kissimmee was eutrophic and has sustained high productivity since well before 1826. Salt-tolerant diatoms were not present in the Lake Kissimmee cores, and analog matching results showed that diatom assemblages throughout the core had many analogs (5–13, generally >10) among lakes in the calibration set (Table S4). Diatom-based limnetic total P inferences obtained by weighted averaging for the predisturbance period were approximately 61 μg/L at the base of the core during the early 1800s, and they generally increased to the present time (, Table S4). The Florida DEP provided a modern limnetic total P average of 64.1 μg/L for 2000–2006 () for comparison, and our diatom-based limnetic total P inference for that time period was ∼76.6 μg/L. We note that the lake has highly variable water quality, with a limnetic total P range of 9–700 μg/L during 2000–2006 (Polk County Water Atlas Citation2019), so although our inference is somewhat high, there might be a question about representativity of the modern reported mean. Total P accumulation rates in core 27_XII_2017_KIS_2 increased approximately 74-fold from 1886 to the present time, and total N accumulation rates increased approximately 12-fold.
Figure 7. Diatom profile with CONISS zonation for core 27_XII_2017_KIS_2 from Lake Kissimmee. Lower panels show sedimented algal and cyanobacterial pigment profiles. Lower right panel presents diatom-based limnetic total P inferences.
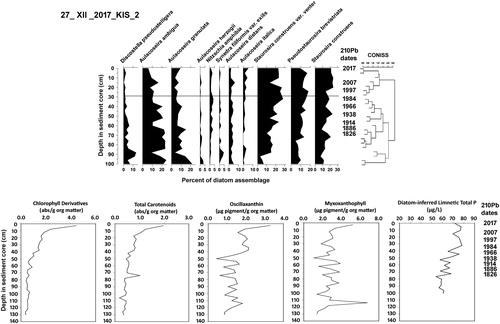
Chlorophyll derivative values were <1 abs/g organic matter below ∼50 cm (prior to 1880s) in core 27_XII_2017_KIS_2, then increased gradually above the 50 cm level to 4.4 abs/g organic matter at the top of the core (). Total carotenoid values were <1 abs/g organic matter below the 20 cm level (c. 1990), but increased to 2 abs/g organic matter. Most notably, the cyanobacterial pigments myxoxanthophyll and oscillaxanthin were found throughout the Lake Kissimmee cores at values ranging from 1 to 6 μg pigment/g organic matter, which indicated that cyanobacteria populations were continuously present since prior to the 1880s.
δ13C versus C/N ratios () were essentially unchanged throughout the length of the sediment core (since prior to 1826), and they suggested that carbon deposited in sediments was consistently influenced by cyanobacteria and algae, and possibly to some extent by floating-leaved vegetation as well.
Figure 8. Plots of δ13C versus C/N ratios in sedimented organic matter for cores from Lakes Kissimmee and Marian from the early 1800s until 2017. Both lakes lacked macrofossil evidence and were consistently dominated by algae and cyanobacteria over time. Specific taxa are placed in relative positions based on δ13C and C/N values reported for studies of other Florida lakes, and are used here to provide general indication of trends in community change.
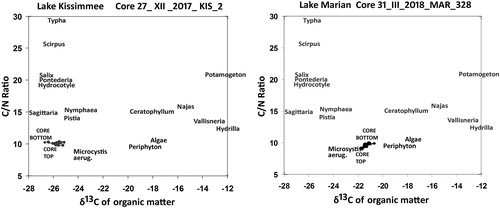
Pollen from predisturbance samples showed high amounts of Taxodium grains (∼17% of assemblages), which indicated that swamps were associated with Lake Kissimmee prior to 1826. Pollen from the wetland taxa Nyssa, Salix, and Morella supported that conclusion (Table S10). Plant macrofossils showed virtually no evidence of submerged aquatic vegetation, but seeds from floating-leaved nymphaeids were present (Table S16), which indicated turbid waters from algae/cyanobacteria, or high dissolved organic carbon concentrations (color) from associated swamps.
Lake Marian
Radioisotopic activities for Lake Marian core 31_III_2018_MAR_328 are shown in , and dates are shown in Table S5. In Lake Marian, as in Lake Kissimmee, diatoms showed highly productive conditions throughout the entire 1.5 m profile, with no evidence of salt-tolerant diatoms that would suggest water-level fluctuations (). The eutrophic, planktonic diatom Aulacoseira ambigua represented 50–60% of diatoms in the assemblages from 150 to 100 cm depth in the core. A. ambigua progressively declined above the 100-cm level (c. 1898) to 10% of the assemblage at the top of the core, while Pseudostaurosira brevistriata, Staurosira construens, and Staurosirella berolinensis increased and represented 10–20% of the assemblage at the core top. This shift is consistent with increased competitive exclusion of planktonic diatom taxa by cyanobacteria (Whitmore et al. Citation2018). Diatom samples in the core had 7–9 analogs in the calibration set before c. 1837, and 12–15 analogs at all depths higher than that in the core (Table S5).
Figure 9. Diatom profile with CONISS zonation for core 31_III_2018_MAR_328 from Lake Marian. Lower panels show sedimented algal and cyanobacterial pigment profiles. Lower right panel presents diatom-based limnetic chlorophyll a inferences.
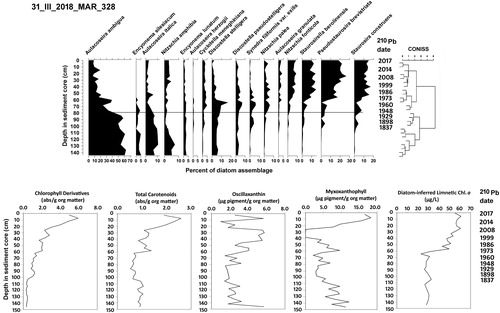
The Florida DEP provided a mean of measured limnetic total P for 2000–2006 of 155 μg/L, and our diatom-inferred value for that period was 82.1 μg/L, which substantially underestimated the reported value. The modern measured value is beyond the reliable range of our total P inference model, and we suspect at least intermittent nitrogen limitation in Lake Marian. As a result, we rejected use of the limnetic total P inference model for Lake Marian. Instead, we applied the weighted-averaging model that is used to infer chlorophyll a from diatom assemblages in sediments. The Florida DEP reported a measured mean chlorophyll a value of 54.5 μg/L for 2000–2006, and our inferred value was 53.6 μg/L for that period, which indicated reliable performance. Limnetic chlorophyll a inferences for Lake Marian are shown in and Table S5. Inferred limnetic chlorophyll a at the base of the core, which was deposited well before c. 1837, was 28.3 μg/L. Limnetic chlorophyll a, therefore, increased significantly in Lake Marian from the early 1800s to the present time.
Chlorophyll derivatives and total carotenoid concentrations were <1.0 abs/g organic matter c. 1837 (). Chlorophyll derivatives increased progressively after c. 1898 to ∼5 abs/g organic matter at the top of the core. Total carotenoids increased rapidly after c. 1960–1973 to ∼2 abs/g organic matter at the top of the core (). Myxoxanthophyll and oscillaxanthin cyanobacterial pigment concentrations showed considerable variation over time, but prior to c. 1837, they were nearly as high as during recent decades. Myxoxanthopyll increased rapidly after c. 1999.
As in Lake Kissimmee, stable isotopes and C/N ratios of organic matter in sediments showed that algae and cyanobacteria, and possibly some floating-leaved macrophytes, were the primary sources of sediment organic matter from prior to 1837 up to the present time (). Macrofossil remains were very sparse, with no indication of submerged aquatic vegetation, and only a few sclereids of nymphaeids were found in one core in deposits that dated to the early 1900s (Table S17). Pollen grains of Taxodium (∼13–17% of assemblages) as well as Morella (bayberry) were abundant in samples prior to 1837 (Table S11), which indicated that swamps were well established in the vicinity of Lake Marian. Pollen and macrofossil evidence, therefore, supported an interpretation of waters that were high in algae/cyanobacteria concentrations, as well as possible high color from dissolved organic carbon from associated swamps.
Discussion
Predisturbance water quality and eutrophication
Eutrophication occurred in all of the study lakes to varying degrees. This was evidenced by increased deposition rates of phosphorus and nitrogen, by increased sedimented pigment concentrations of algae and cyanobacteria, and by diatom autecology and statistical inferences of water quality. δ13C versus C/N plots and plant macrofossils in Lakes Cypress, East Tohopeliga, Jackson, and Tohopekaliga showed a shift from submerged plant communities to floating-leaved taxa, then to algae/cyanobacteria, a progression that is typical of light limitation caused by eutrophication (Sayer et al. Citation2010a, Citation2010b, Bennion et al. Citation2018). Multiple lines of evidence showed that all lakes in this study were meso-eutrophic to eutrophic during predisturbance times. None of the lakes in this study held oligotrophic waters during their reference periods in the early 1800s. Qualitative diatom evidence based on well-documented autecological preferences in the literature, as well as the limnetic total P optima reported for dominant taxa (Whitmore et al. Citation2018), showed that Lakes Cypress, East Tohopekaliga, Jackson, and Tohopekaliga had high productivity during their predisturbance periods in the early 1800s. Predisturbance inferences for limnetic total P in Lake Kissimmee (61 μg/L) and for limnetic chlorophyll a in Lake Marian (28.3 μg/L) were within the eutrophic range for lakes.
High productivity in these lakes during their predisturbance periods is consistent with their geological setting. Paleolimnological studies have shown that a significant number of Florida lakes, particularly alkaline lakes with color, were productive during predisturbance periods because of naturally high edaphic nutrient supply (e.g., Brenner et al. Citation1993, Riedinger-Whitmore et al. Citation2005, Kenney et al. Citation2014). For such lakes, nutrient criteria standards <40–45 µg/L total P would not be appropriate. Naturally high productivity in lakes on the Kissimmee Chain suggests that relatively small amounts of nutrient additions could occur before modern nutrient criteria standards would be exceeded. Paleolimnological studies have demonstrated naturally high productivity and sustained cyanobacterial populations in other regions as well (McGowan et al. Citation1999, Brooks et al. Citation2001, Simmatis et al. Citation2020).
In Lakes Cypress, East Tohopekaliga, Tohopekaliga, and Marian, diatom communities changed in response to increased cyanobacteria presence. Tychoplanktonic taxa increased as cyanobacteria became well established and planktonic taxa, including Aulacoseira spp., were subject to competitive exclusion (Whitmore et al. Citation2018). Although this shift might seem paradoxical in hypereutrophic conditions because of potential light limitation of algae that grow on surfaces, frequent mixing in Florida’s shallow, wind-stressed lakes affords tychoplanktonic taxa the opportunity to meet minimum light requirements. Recognition of this community shift might serve as an early warning indicator of pending system changes in modern Florida lakes, and it might be used to better inform decisions about lake management (Reavie Citation2019). This diatom community change was not evident in Lake Jackson, however, and sedimented pigments showed that cyanobacteria increased in that lake only recently. Lakes Kissimmee and Marian sustained substantial cyanobacteria populations throughout their entire records since the early 1800s.
Associated wetlands and their possible influence on lake color
Carpenter et al. (Citation1998) showed strong effects of dissolved organic color on chlorophyll concentrations and primary production in lakes because of light attenuation in the water column. Persistent and highly colored humic and fulvic acids that contribute to colored dissolved organic carbon (C-DOC) in lakes often have allochthonous sources from nearby wetlands, although pine flatwoods in Florida often contribute C-DOC because shallow, impervious clay layers direct tannins laterally to nearby waterbodies. In contrast, autochthonous DOC from the breakdown of phytoplankton and macrophyte biomass is less highly colored and is easily degraded. Because of its potential for moderating primary production, Williamson et al. (Citation1999) maintained that there is a clear need to incorporate C-DOC into lake management approaches. However, C-DOC is not equally effective in controlling algal production in lakes across a wide range of trophic states. Carpenter et al. (Citation1998) stated that eutrophication is responsible for a broad range of P inputs to lakes, and this strong environmental gradient tends to mask the effects of C-DOC in multiple-lake comparisons. Karlsson et al. (Citation2009) concluded that light availability, as influenced by C-DOC, controls productivity most effectively in low-productivity lakes, whereas nutrient availability controls productivity in lakes that are eutrophic and dominated by pelagic production. Based on these studies, it seems appropriate to conclude that the potential moderating effect of C-DOC on primary productivity is likely to be more effective during periods of low disturbance than it is when lakes have undergone advanced eutrophication. This suggests the importance of protecting associated wetlands for lakes that have not been subject to high nutrient loading, and for implementing wetland restoration after high nutrient concentrations have been reduced.
At the beginning of the 1800s, wetlands covered nearly 50% of the surface area of Florida (Fernald and Purdum Citation1998). Subsequent logging and alteration of lake levels caused substantial loss of wetlands associated with Florida’s 8000 lakes. When the present study was initiated, the Osceola County Public Works Department conducted a statistical evaluation of water quality measures for a large set of lakes to determine whether C-DOC has a moderating influence on phytoplankton concentrations in this region. Limnetic concentrations of total nitrogen, total phosphorus, and chlorophyll a were compared for 2 categories: lakes with low color concentrations (i.e., <75 platinum cobalt units; PCU) and lakes with high color concentrations (>75 PCU). Results showed that nutrient concentrations were likely to be higher under high color conditions, but concentrations of chlorophyll a were likely to be lower under high color conditions. The Osceola County and Florida DEP concluded that C-DOC has the potential to alter relationships between watershed loadings and in-lake concentrations of chlorophyll a in Kissimmee Basin lakes.
Lakes Kissimmee and Marian showed high pollen concentrations of Taxodium (cypress), Nyssa (water tupelo), Salix (willow), and Morella in bottommost core samples, which indicated clear association of swamps with the lakes. Macrofossil evidence was scarce, except for floating-leaved nymphaeids that can grow in waters with high color or turbidity. There was little evidence for submerged aquatic-plant communities. Our conclusion is that during predisturbance times in Lakes Kissimmee and Marian, waters were high in C-DOC that probably moderated algal productivity.
In contrast, pollen in reference samples from Lakes East Tohopekaliga and Tohopekaliga showed relatively little evidence of swamp taxa, and macrofossils showed substantial amounts of Chara and Ruppia that indicated clear waters (Dressler et al. Citation1987, Bennion et al. Citation2018). This also was the case with Lakes Cypress and Jackson, but they lacked Ruppia in early deposits. Because historical drainage reports indicated that some swamps were present near Cypress, East Tohopekaliga, and Tohopekaliga (Atlantic and Gulf Coast Canal and Okeechobee Land Company 1885), it might be appropriate to consider restoration efforts to support associated wetlands near those lakes. We concluded from pollen evidence, however, that Lake Jackson had fairly clear waters and apparently lacked associated swamps that might have contributed significant amounts of C-DOC.
Regarding the question of whether restoring more natural water levels and colored dissolved organic carbon concentrations might mitigate algal productivity and alter restoration strategies in lakes on the Kissimmee Chain, we recommend that this approach should first be implemented for Lakes Kissimmee and Marian, because paleolimnological evidence showed greater original wetland presence for those lakes.
Historical changes in lake water levels
Modern water levels in lakes of the Kissimmee Chain are substantially lower than they were prior to engineered drainage activities that began in the 1880s. Decreases in water levels during the 19th and 20th centuries contributed to management challenges, such as a greater potential for internal nutrient loading from sediment resuspension over a larger portion of the lake areas (e.g., Nagid et al. Citation2001), a reduced volume of water that would make the lakes more susceptible to eutrophication for a given increase in nutrient loading, and a potential loss of allochthonous C-DOC from associated wetlands that might have helped to moderate algal production by light limitation.
A potentially greater disturbance resulted from reducing the large natural variations in water levels that prevailed prior to engineered changes. It was speculated that water levels in Kissimmee Chain lakes fluctuated during predisturbance times up to 3 m (US Army Corps of Engineers Citation1996), and that was confirmed by the present study. Salinity preferences of diatoms, macrofossils, and ostracods showed that these fluctuations greatly exceeded seasonal water-level variations, and that they occasionally led to total drying in portions of the lakes. Large water-level fluctuations would have increased habitat diversity and helped to maintain the integrity of the lake ecosystems, and natural fires during low water levels would have burned organic matter in exposed littoral areas (US Army Corps of Engineers Citation1996). Early natural fires were evident from charcoal that appeared periodically in diatom samples of the present study, although charcoal was not analyzed in detail. Multiple indicators in all sediment cores showed that Lakes Cypress, East Tohopekaliga, Jackson, and Tohopekaliga changed significantly after the 1880s because they failed to undergo very low water-level events that concentrated solutes on a periodic basis. Modern water levels are held relatively constant (within 0.6–0.9 m) by the South Florida Water Management District (Osceola County Community Development Department Citation2015). This has promoted organic matter accumulation, and favored proliferation of certain nuisance plants such as Hydrilla over native plants that depend on seasonal water-level variations for propagation. Results of the present study show that the cessation of natural water-level fluctuations had greater impact on lake processes, water quality, and community compositions than generally has been recognized. Changes in water-level fluctuations were shown to affect the structure and function of shallow lakes in other paleolimnological studies as well (e.g., Levi et al. Citation2016).
The most prominent diatom indicator of high solute conditions during extreme low water-level events in Lakes Cypress, East Tohopekaliga, Jackson, and Tohopekaliga was Campylodiscus clypeus. Pouličková and Jahn (Citation2007) described this species as widespread mostly in hypersaline waters, but at specific conductance values beginning as low as 2000–3000 μS/cm, and where dominant anions tend to be carbonate or sulfate rather than chloride. Carvalho et al. (Citation1995) reported that Campylodiscus clypeus occurred most often in hypersaline sites with Anomoeoneis sphaerophora var. costata. In the present study we observed Anomoeoneis sphaerophora var. costata, as well as two of its salt-tolerant varieties.
Ruppia maritima seeds were abundant in cores from Lakes Tohopekaliga and East Tohopekaliga prior to 1880. Although Ruppia is often described as an estuarine plant in areas of lower salinity, it is actually a freshwater plant that has pronounced salinity tolerance, and it occurs in clear waters <2.0 m deep (Zieman Citation1982, Kantrud Citation1991). We have collected Ruppia, for example, in the littoral zone of a freshwater Florida lake that has a specific conductance of about 1500 μS/cm. Ruppia seeds are ingested by waterfowl and can be transported 280 km (Charalambidou et al. Citation2003), so it could have been distributed from coastal areas to these lakes during low water-level stands. Its presence in the sediment record of inland lakes provides clear indication of former elevated concentrations of dissolved solutes.
Ostracods provided additional evidence of elevated dissolved solute concentrations at the base of several Lake Tohopekaliga cores. Darwinula stevensoni, Limnocythere inopinata, and Cypridopsis vidua occur in fresh to oligohaline waters (Keyser Citation1977, Holmes Citation1992, Ruiz et al. Citation2013). Cypridopsis okeechobei has an optimum range of 0.3–0.5 ppt salinity (Keyser Citation1977, Holmes Citation1992). Cypretta brevisaepta is an oligohaline indicator that occurs below 0.5 ppt, but it has an optimum range of 0.5–1 ppt (Keyser Citation1977). Limnocythere inopinata and Cypridopsis vidua prefer organic substrates with vegetative cover and are often associated with Chara (Matsuda et al. Citation2015), as they were in core samples prior to c. 1890. Ostracods were absent in Lake Tohopekaliga after c. 1890, which signaled the disappearance of naturally low water levels. It appears evident that the hydrological alterations that began in 1883 prevented extremely low water levels after that time.
Many shallow Florida lakes are subject to very large fluctuations in water level, in part because of elution, or downward leakage in karst bedrock (Deevey Citation1988), which contributes to falling levels during drier periods. Natural fluctuations up to 3.5 m have been demonstrated in multiple-year studies of Florida lakes (Hoyer et al. Citation2005), and up to 10 m in lakes that are astatic (Deevey Citation1988). Large water-level fluctuations are not caused so much by precipitation in a given year, but by cumulative interannual precipitation patterns that affect groundwater levels on the order of decades (Deevey Citation1988).
Because most Florida lakes are shallow (<5 m), water-level fluctuations can greatly affect lake area and volume. We calculated the percent volume curve for modern Lake Tohopekaliga using contour areas and depths indicated in a contemporary morphometric map (UF/IFAS Citation2019). A drop of 2 m in current water level would reduce the lake to 23% of its volume, and would expose ∼37% of the lake area to drying. A drop of 3 m in Tohopekaliga would reduce the current volume to 4%, and would expose 70% of the lake bed to drying, which could easily increase total dissolved solids sufficiently to support salt-tolerant taxa. Although downward elution likely would have removed some solutes as well as water during drier periods (Deevey Citation1988), the lakes in this study achieved impressively high salinities, as indicated by diverse biological evidence, so evaporation had to exceed leakage by a substantial amount.
Lakes Kissimmee and Marian did not show evidence of extreme water-level fluctuations like the other lakes, and they demonstrated the clearest evidence of associated wetlands. We note that predisturbance water levels in East Tohopekaliga (70.3 ft, 21.4 m) and Tohopekaliga (64.6 ft, 19.7 m) stood at higher elevations than in Kissimmee (59.1 ft, 18.0 m; surveyed prior to the NGVD elevation standard: Atlantic and Gulf Coast Canal and Okeechobee Land Company 1885). Although the predisturbance water level of Lake Cypress was not reported, it would have been between 60 and 64 ft (18.3–19.5 m). It appears that local hydrological factors made Kissimmee and Marian less subject to drying than East Tohopekaliga, Tohopekaliga, and Cypress, which stood at higher elevations. Lake Jackson is situated between Kissimmee and Marian, but it showed evidence of high solutes and dry periods that Kissimmee and Marian did not. Jackson has been bordered by dry prairies historically, so it has a different edaphic setting. Jackson and Cypress are the smallest and volumetrically shallowest lakes in this study: a 2-m water-level drop would currently reduce both lakes to <1% of their volume, and a 3-m drop would cause the lakes to go dry.
Conclusions
Two major types of changes occurred in these lakes during the late 19th and 20th centuries. All lakes in this study were meso-eutrophic prior to the mid 1800s, but eutrophication was observed in 5 of the 6 lakes, as shown by multiple lines of evidence. East Lake Tohopekaliga showed the least evidence of nutrient changes, and eutrophication was most pronounced in Lake Marian. Onset of eutrophication began in the late 1800s immediately after engineered hydrological changes, and it increased distinctly during the 20th century because of nutrient loading that likely arose from urbanization, land-use change, agriculture, and cattle ranching. Engineered connection of the lakes facilitated a downstream cascade of nutrients, and the loss of former connecting wetlands reduced the biological filtering of nutrients that occurred prior to disturbance.
The second and possibly more prominent change in 4 of the 6 lakes was that hydrology was altered dramatically, both by lowering water levels with respect to former associated wetlands and, importantly, by permanently preventing the lakes from undergoing natural large fluctuations in water levels. This was evident in Lakes Cypress, East Tohopekaliga, Jackson, and Tohopekaliga, but was not the case in Lakes Kissimmee and Marian. Large natural fluctuations influenced the types of native plant communities that were present, whereas more static water levels in recent times have contributed to organic matter accumulation and high standing crops of aquatic plants. Although lake levels have been lowered occasionally in recent decades so littoral sediments can be scraped and burned, this is mechanically doing the work that natural processes did before engineered activities prevented large natural fluctuations in water levels.
Current management approaches often emphasize in-lake nutrient concentrations and mitigation without adequately considering other significant changes in ecology or ecosystem function, but restoration cannot be effective when it regards system changes as univariate (Davidson et al. Citation2018). Contemporary challenges for management of these lakes include satisfying stakeholder needs related to the sport-fishing economy, recreational uses, water supply, and flood prevention. The lakes, however, are distinctly out of balance with respect to nutrient content and recycling, standing crops of native and invasive plants, and the accumulation of nutrient-rich organic sediments. Considerable energy and costs are required for periodic drawdowns, scraping of sediments, and management of aquatic plants. The sustained connection of the lakes, which originated as a 19th-century paradigm, also facilitates transport of nutrients through the Kissimmee–Okeechobee–Everglades system, and it contributes to downstream restoration challenges.
Based on the findings of this study, we recommend disconnecting the lakes from each other and from the Kissimmee River to reestablish large, natural fluctuations in water levels that were part of healthy ecosystem function. Some structures might need to be retained but modified to prevent flooding in developed areas. The current downstream nutrient cascade challenges local management agencies to deal with nutrient loads that arise partly outside of their jurisdictions. Hydrological restoration would return nutrient loading to being more of a local issue. Former wetlands between the lakes should be restored because this would reestablish biological communities that sequester nutrients that currently enter the lakes and river system, and that subsequently reach Lake Okeechobee, the Everglades, and offshore coral reef systems.
The focus of the 19th and early 20th centuries was on making engineered changes in natural systems for perceived human benefit, but that led to many unanticipated problems in lakes on the Kissimmee Chain. We advocate for a management paradigm in the 21st century that places greater emphasis on sustainability, reduced energy expenditure, and restoring the benefits that were provided by natural ecosystem functions.
Supplemental Material
Download MS Word (255.5 KB)Acknowledgments
We are particularly grateful to Susan Gosselin (Osceola County Public Works Department) and to Kimberly Lawrence (Reedy Creek Improvement District) for their support of our study. Three anonymous reviewers provided helpful feedback. Neil Rose arranged for radioisotopic dating at the Environmental Change Research Centre at University College London. Mark Brenner provided valuable suggestions that helped to improve the article. Kendal Jackson assisted in the field.
Additional information
Funding
References
- Appleby PG, Nolan PJ, Gifford DW, Godfrey MJ, Oldfield F, Anderson NJ, Battarbee RW. 1986. 210Pb dating by low background gamma counting. Hydrobiologia. 143(1):21–27. doi:10.1007/BF00026640.
- Appleby PG, Oldfield F. 1983. The assessment of 210Pb data from sites with varying sediment accumulation rates. Hydrobiologia. 103(1):29–35. doi:10.1007/BF00028424.
- Appleby PG, Richardson N, Nolan PJ. 1992. Self-absorption corrections for well-type germanium detectors. Nucl Inst Methods B. 71(2):228–233. doi:10.1016/0168-583X(92)95328-O.
- Battarbee RW, Bennion B. 2011. Palaeolimnology and its developing role in assessing the history and extent of human impact on lake ecosystems. J Paleolimnol. 45(4):399–404. doi:10.1007/s10933-010-9423-7.
- Bennett KD. 1996. Determination of the number of zones in a biostratigraphical sequence. New Phytol. 132(1):155–170. doi:10.1111/j.1469-8137.1996.tb04521.x.
- Bennett KD, Willis KJ. 2001. Pollen. In: Smol JP, Birks HJ, Last WM, editors. Tracking environmental change using lake sediments volume 3: terrestrial, algal, and siliceous indicators. Dordrecht, The Netherlands: Kluwer Academic Publications; p. 5–32.
- Bennion H, Battarbee RW, Sayer CD, Simpson GL, Davidson TA. 2011. Defining reference conditions and restoration targets for lake ecosystems using palaeolimnology: a synthesis. J Paleolimnol. 45(4):533–544. doi:10.1007/s10933-010-9419-3.
- Bennion H, Sayer CD, Clarke SJ, Davidson TA, Rose NL, Goldsmith B, Rawcliffe R, Burgess A, Clarke G, Turner S, Wiik E. 2018. Sedimentary macrofossil records reveal ecological change in English lakes: implications for conservation. J Paleolimnol. 60(2):329–348. doi:10.1007/s10933-017-9941-7.
- Birks HH. 2017. Plant macrofossil introduction. In: Reference module in earth systems and environmental sciences. New York: Elsevier.
- Blindow I. 1992. Decline of charophytes during eutrophication: comparison with angiosperms. Freshwater Biol. 28(1):9–14. doi:10.1111/j.1365-2427.1992.tb00557.x.
- Brenner M, Hodell DA, Leyden BW, Curtis JH, Kenney WF, Gu BH, Newman JM. 2006. Mechanisms for organic matter and phosphorus burial in sediments of a shallow, subtropical, macrophyte-dominated lake. J Paleolimnol. 35(1):129–148. doi:10.1007/s10933-005-7881-0.
- Brenner M, Whitmore TJ, Curtis JH, Hodell DA, Schelske CL. 1999. Stable isotopes (δ13C and δ15N) of sedimented organic matter as indicators of historic lake trophic state. J Paleolimnol. 22(2):205–221. doi:10.1023/A:1008078222806.
- Brenner M, Whitmore TJ, Flannery MS, Binford MW. 1993. Paleolimnological methods for defining target conditions in lake restoration: Florida case studies. Lake Reserv Manage. 7(2):209–217. doi:10.1080/07438149309354272.
- Brooks SJ, Bennion H, Birks H. 2001. Tracing lake trophic history with a chironomid-total phosphorus inference model. Freshwater Biol. 46(4):513–533. doi:10.1046/j.1365-2427.2001.00684.x.
- Carpenter SR, Cole JJ, Kitchell JF, Pace ML. 1998. Impact of dissolved organic carbon, phosphorus, and grazing on zooplankton biomass and production in experimental lakes. Limnol Oceanogr. 43(1):73–80. doi:10.4319/lo.1998.43.1.0073.
- Carvalho LR, Sims PA, Battarbee RW, Cox EJ, Juggins S. 1995. Campylodiscus clypeus (Ehrenb.) Ehrenb. in inland saline lakes In: Robertson AM, Hicks S, Akerlund A, Risberg J, Hackens T, editors. Landscapes and life: studies in honour of Uwe Miller. PACT. 50:471–484.
- Charalambidou I, Santamaria L, Langevoord O. 2003. Effect of ingestion by five avian dispersers on the retention time, retrieval and germination of Ruppia maritima seeds. Funct Ecol. 17(6):747–753. doi:10.1111/j.1365-2435.2003.00787.x.
- Davidson TA, Bennion H, Reid M, Sayer CD, Whitmore TJ. 2018. Towards better integration of ecology in palaeoecology: from proxies to indicators, from inference to understanding. J Paleolimnol. 60(2):109–116. doi:10.1007/s10933-018-0032-1.
- Davidson TA, Jeppesen E. 2013. The role of palaeolimnology in assessing eutrophication and its impact on lakes. J Paleolimnol. 49(3):391–410. doi:10.1007/s10933-012-9651-0.
- Deevey ES. Jr. 1988. Estimation of downward leakage from Florida lakes. Limnol Oceanogr. 33(6):1308–1320. doi:10.4319/lo.1988.33.6.1308.
- Diatoms of North America. 2020. Diatoms of North America. https://diatoms.org/.
- Dierberg FE, Williams VP, Schneider WH. 1988. Evaluating water quality effects of lake management in Florida. Lake Reserv Manage. 4(2):101–111. doi:10.1080/07438148809354818.
- Dressler RL, Hall DW, Perkins KD, Williams NH. 1987. Identification manual for wetland plant species of Florida: used by the Florida department of environmental protection in determining the landward extent of waters of the State Institute of Food and Agricultural Sciences. SP-35. Univ. of Florida, Gainesville, FL; 297p.
- Faegri K, Iversen J. 1989. Finding the grain: laboratory technique. In: Faegri K, Kaland PE, Kryzwinski K, editors. Textbook of pollen analysis. 4th ed. Caldwell, NJ: The Blackburn Press; 328p.
- Fernald EA, Purdum ED. 1998. Water resources Atlas of Florida. Tallahassee, FL: Florida State University, Institute of Science and Public Affairs; 282p.
- Fisher MM, Brenner M, Reddy KR. 1992. A simple, inexpensive piston corer for collecting undisturbed sediment/water interface profiles. J Paleolimnnol. 7:157–161.
- Florida Department of Environmental Protection (Florida DEP). 2013. Implementation of Florida’s Numeric Nutrient Standards. Tallahassee: Florida DEP; 86p.
- Furtos NF. 1936. Fresh-water Ostracoda from Florida and North Carolina. Am Midl Nat. 17(2):491–522. doi:10.2307/2419975.
- Gu BH, Alexander V. 1996. Stable carbon isotope evidence for atmospheric CO2 uptake by cyanobacterial surface scums in a eutrophic lake. Appl Environ Microb. 62(5):1803–1804. doi:10.1128/AEM.62.5.1803-1804.1996.
- Heiri O, Lotter AF, Lemcke G. 2001. Loss on ignition as a method for estimating organic and carbonate content in sediments: reproducibility and comparability of results. J Paleolimnnol. 25(1):101–110. doi:10.1023/A:1008119611481.
- Holmes J. 1992. Non-marine ostracods as quaternary paleoenvironmental indicators. Prog Phys Geogr. 16(4):405–431. doi:10.1177/030913339201600402.
- Hoyer MV, Horsburgh CA, Canfield DE, Jr., Bachmann RW. 2005. Lake level and trophic state variables among a population of shallow Florida lakes and within individual lakes. Can J Fish Aquat Sci. 62(12):2760–2769. doi:10.1139/f05-177.
- Huber WC, Brezonik PL, Heany JP, Dickinson RE, Preston SD, Dwornik DS, DeMaio MA. 1982. A classification of Florida lakes. Final report to the Florida Department of Environmental Regulation, Report ENV-05-82-1. Tallahassee: Florida DEP; v. 1–2, 547p.
- James RT, O’Dell K, Smith VH. 1994. Water quality trends in Lake Tohopekaliga, Florida, USA: responses to watershed management. J Am Water Resources Assoc. 30(3):531–546. doi:10.1111/j.1752-1688.1994.tb03311.x.
- Juggins S. 2012. Rioja: analysis of quaternary science data, R package version (0.8-7). http://cran.r-project.org/package=rioja.
- Kantrud HA. 1991. Wigeongrass (Ruppia maritima): a literature review. Fish and Wildlife Research, Series 10. Washington, DC: U.S. Fish and Wildlife Service; 58p.
- Karlsson J, Bystrom P, Ask J, Ask P, Persson L, Jansson M. 2009. Light limitation of nutrient-poor lake ecosystems. Nature Lett. 460(7254):506–510. doi:10.1038/nature08179.
- Kenney WF, Whitmore TJ, Buck DG, Brenner M, Curtis JH, Di JJ, Kenney PL, Schelske CL. 2014. Whole-basin, mass-balance approach for identifying critical phosphorus-loading thresholds in shallow lakes. J Paleolimnol. 51(4):515–528. doi:10.1007/s10933-014-9771-9.
- Keyser D. 1977. Ecology and zoogeography of recent brackish-water Ostracoda (Crustacea) from South-West Florida. In Proc. Sixth International Ostracod Symposium, Saalfelden; p. 207–222.
- Krammer K, Lange-Bertalot H. 1986–1991. Bacillariophyceae Teil 1-4. In: Pascher A, editor. Susswasserflora von Mitteleuropa. Jena: Gustav Fischer Verlag.
- Larocque-Tobler I. 2016. Editorial: Using paleolimnology for management and restoration of lakes. Front Ecol Evol. 4:103. doi:10.3389/fevo.2016.00103.
- Levi EE, Bezirci G, Çakıroğlu Aİ, Turner S, Bennion H, Kernan M, Jeppesen E, Beklioğlu M. 2016. Multi-proxy palaeoecological responses to water-level fluctuations in three shallow Turkish lakes. Palaeogeogr Palaeoclimatol Palaeoecol. 449:553–566. doi:10.1016/j.palaeo.2016.02.052.
- Matsuda JT, Lansac-Toha FA, Martens K, Velho LFM, Mormul RP, Higuti J. 2015. Association of body size and behavior of freshwater ostracods (Crustacea, Ostracoda) with aquatic macrophytes. Aquat Ecol. 49(3):321–331. doi:10.1007/s10452-015-9527-2.
- McGowan S, Britton G, Haworth E, Moss B. 1999. Ancient blue-green blooms. Limnol Oceanogr. 44(2):436–439. doi:10.4319/lo.1999.44.2.0436.
- Nagid EJ, Canfield DE, Jr., Hoyer MV. 2001. Wind-induced increases in trophic state characteristics of a large (27 km2), shallow (1.5 m mean depth) Florida Lake. Hydrobiologia. 455(1/3):97–110. doi:10.1023/A:1011913200302.
- Osceola County Community Development Department. 2015. Osceola County Lakes Management Plan. 70p. https://www.osceola.org/core/fileparse.php/2731/urlt/092415_LakesManagementPlan.pdf.
- Otto JS. 1985. Florida’s cattle-ranching frontier: Manatee and Brevard counties (1860). Florida Histor Quart. 64(1):48–61.
- Patrick P, Reimer CW. 1966–1977. The diatoms of the United States. Monogr Phil Acad Sci. 1–2(13):Part 1, v.
- Pérez L, Lorenschat J, Brenner M, Scharf B, Schwalb A. 2010. Extant freshwater ostracodes (Crustacea: Ostracoda) from Lago Petén Itzá, Guatemala. Rev Biol Trop. 58(3):871–895.
- Polk County Water Atlas. 2019. Lake Kissimmee (Osceola), Lake East Tohopekaliga (Osceola), Lake Tohopekaliga (Osceola). https://polk.wateratlas.usf.edu/.
- Pouličková A, Jahn R. 2007. Campylodiscus clypeus (Ehrenberg) Ehrenberg ex Kutzing: typification, morphology, and distribution. Diatom Res. 22(1):135–146. doi:10.1080/0269249X.2007.9705701.
- R Core Team. 2014. R: A language and environment for statistical computing. Vienna, Austria: R Foundation for Statistical Computing. http://www.R-project.org/.
- Reavie ED. 2019. Paleolimnology supports aquatic management by providing early warnings of stressor impacts. Lake Reserv Manage. 36(3):210–217. doi:10.1080/10402381.2019.1645249.
- Riedinger-Whitmore MA, Whitmore TJ, Smoak J, Brenner M, Moore AM, Curtis J, Schelske CL. 2005. Cyanobacterial proliferation is a recent response to eutrophication in many Florida lakes: a paleolimnological assessment. Lake Reserv Manage. 21(4):423–435. doi:10.1080/07438140509354447.
- Rosen BH, Davis TW, Gobler CJ, Kramer BJ, Loftin KA. 2017. Cyanobacteria of the 2016 Lake Okeechobee waterway harmful algal bloom. U.S. Geological Survey Open-File Report 2017–1054; 34p. doi:10.3133/ofr20171054.
- Ruiz F, Abad M, Bodergat AM, Carbonel P, Rodrıguez-Lazaro J, Gonzalez-Regalado ML, Toscano A, Garćıa EX, Prenda J. 2013. Freshwater ostracods as environmental tracers. Int J Environ Sci Technol. 10(5):1115–1128. doi:10.1007/s13762-013-0249-5.
- Sayer CD, Burgess A, Kari K, Davidson TA, Peglar S, Yang HD, Rose N. 2010a. Long-term dynamics of submerged macrophytes and algae in a small and shallow, eutrophic lake: implications for the stability of macrophyte-dominance. Freshwater Biol. 55(3):565–583. doi:10.1111/j.1365-2427.2009.02353.x.
- Sayer CD, Davidson TA, Jones JI. 2010b. Seasonal dynamics of macrophytes and phytoplankton in shallow lakes: a eutrophication-driven pathway from plants to plankton? Freshwater Biol. 55(3):500–513. doi:10.1111/j.1365-2427.2009.02365.x.
- Schelske CL, Peplow A, Brenner M, Spencer CN. 1994. Low-background gamma counting: applications for 210Pb dating of sediments. J Paleolimnol. 10(2):115–128. doi:10.1007/BF00682508.
- Simmatis B, Nelligan C, Rühland KM, Jeziorski A, Castro V, Paterson AM, Smol JP. 2020. Tracking ∼200 years of water quality in Muskrat Lake, a eutrophic lake trout lake in Ontario (Canada) with cyanobacterial blooms. Lake Reserv Manage. 36(3):260–277. doi:10.1080/10402381.2020.1755749.
- Smol JP. 1992. Paleolimnology: an important tool for effective ecosystem management. J Aquat Ecosyst Stress Recov. 1(1):49–58. doi:10.1080/07438149709354309.
- South Florida Water Management District. 2005. Kissimmee Watershed Basemap. https://www.sfwmd.gov/sites/default/files/documents/kissimmee_watershed_basemap2005_25x33.pdf.
- South Florida Water Management District. 2014. Kissimmee chain of lakes management area ten-year general management plan (2014 through 2024). Land Stewardship Section. South Florida Water Management District; 120p.
- South Florida Water Management District. 2019. Kissimmeee River. https://www.sfwmd.gov/our-work/kissimmee-river.
- Swain EB. 1985. Measurement and interpretation of sedimentary pigments. Freshwater Biol. 15(1):53–75. doi:10.1111/j.1365-2427.1985.tb00696.x.
- Teeter JW. 1980. Ostracoda of the Lake Flirt Formation (Pleistocene) of Southern Florida. Micropaleontology. 26 (4):337–355. doi:10.2307/1485349.
- The Atlantic and Gulf Coast Canal and Okeechobee Land Company. 1885. Report of Engineer and Superintendent. 37 p.
- University of Florida Center for Aquatic and Invasive Plants (UF/IFAS). 2019. Bathymetric maps. http://plants.ifas.ufl.edu/manage/overview-of-florida-waters/public-waters/bathymetric-maps/.
- US Army Corps of Engineers. 1996. Kissimmee River, Florida, revitalization project: integrated project modification report and supplement to the final environmental impact statement. Jacksonville District: South Atlantic Division; 362p.
- Van der Werff A. 1953. A new method of concentrating and cleaning diatoms and other organisms. Int Ver Theor Angew Limnol Verh. 12(1):276–277. doi:10.1080/03680770.1950.11895297.
- Wang Y, Hsieh YP, Landing WM, Choi YH, Salters V, Campbell D. 2002. Chemical and carbon isotopic evidence for the source and fate of dissolved organic matter in the northern Everglades. Biogeochemistry. 61(3):269–289. doi:10.1023/A:1020282717732.
- Whitmore TJ, Brenner M, Schelske CL. 1996. Highly variable sediment distribution: a case for sediment mapping surveys in paleolimnological studies. J Paleolimnol. 15(3):207–221. doi:10.1007/BF00213041.
- Whitmore TJ, Lauterman FM, Smith KE, Riedinger-Whitmore MA. 2015. Limnetic total P transfer functions for lake-management applications: considerations for their design and use. Front Ecol Evol. 3:107. doi:10.3389/fevo.2015.00107.
- Whitmore TJ, Riedinger-Whitmore MA, Lauterman FM, Curtis JH. 2018. Cyanobacterial influence on diatom community lifeform dynamics in subtropical lakes of Florida USA. In: Bennion H, Whitmore TJ, Davidson TA, Sayer CD (eds.). Putting the ecology into palaeo. J Paleolimnol. 60(2):223–246. doi:10.1007/s10933-018-0018-z.
- Willard DA, Bernhardt CE, Weimer L, Cooper SR, Gamez D, Jensen J. 2004. Atlas of pollen and spores of the Florida Everglades. Palynology. 28(1):175–227. doi:10.2113/28.1.175.
- Williams VP. 2001. Effects of point source removal on lake water quality: a case history of Lake Tohopekaliga, Florida. Lake Reserv Manage. 17(4):315–329. doi:10.1080/07438140109354138.
- Williamson CE, Morris DP, Pace ML, Olson OG. 1999. Dissolved organic carbon and nutrients as regulators of lake ecosystems: resurrection of a more integrated paradigm. Limnol Oceanogr. 44(3 Part 2):795–803. doi:10.4319/lo.1999.44.3_part_2.0795.
- Zieman JC. 1982. The ecology of the seagrasses of south Florida: a community profile. FWS/OBS-82/25. Washington, DC: U.S. Fish and Wildlife Service, Office of Biological Services; 123 p.