Abstract
Matern S, Robichon C, Nikolaus R, Monk CT, Arlinghaus R. 2023. Environmental determinants of coarse woody habitat in gravel pit lakes. Lake Reserv Manage. 39:259–272.
Coarse woody habitat (CWH) is an important structural habitat in freshwater ecosystems. In natural lakes, CWH accumulates over centuries alongside the succession of littoral tree communities. Newly created gravel pit lakes have difficulties in accumulating CWH due to their young age. Additionally, CWH presence might be negatively affected by shoreline development, where wood is removed to facilitate recreational activities such as angling. We studied 26 gravel pit lakes with an age ≤ 55 yrs in Lower Saxony, Germany, to quantify CWH density and to understand the impact of environmental factors, including lake morphology, lake age, wind direction, abundance of riparian trees, and the presence or absence of fisheries management, on CWH density. We sampled small and large CWH in the littoral zone of the study lakes using a transect-based approach. Density of CWH was lower in German gravel pit lakes than in North American natural lakes. In gravel pit lakes, we detected increasing densities of small CWH with increasing numbers of large trees on the shore and with increasing littoral slopes in lakes managed for recreational fisheries. Large CWH density was positively affected by lake age, by the density of large trees on the shore and with wind from land, and again by steep littoral slopes in lakes managed for recreational fisheries. We recommend that recreational fisheries managers and individual anglers maintain CWH in shallow littoral zones to promote fish habitats in generally low-structured gravel pit lakes.
Littoral zones link terrestrial and aquatic ecosystems (Naiman and Décamps Citation1997, Schindler and Scheuerell Citation2002, Pusey and Arthington Citation2003) and provide structural heterogeneity and habitat complexity in aquatic ecosystems (Eadie and Keast Citation1984, Kovalenko et al. Citation2012). In addition to emerged and submerged macrophytes, coarse woody habitat (CWH) represents a third littoral structure that can generate suitable habitats for colonization by various species (Sass Citation2009, Czarnecka Citation2016). Invertebrates, such as Gammaridae and Chironomoidae, regularly use CWH, with the highest diversity and biomasses found on highly decayed dead wood (Benke and Wallace Citation2003, Smokorowski et al. Citation2006, Dossi et al. Citation2020). Lake fish also use CWH regularly (Lewin et al. Citation2004, Citation2014, Matern et al. Citation2021, Maday et al. Citation2023), for purposes such as spawning (Nash et al. Citation1999, Lawson et al. Citation2011), feeding (Czarnecka et al. Citation2014), and potentially as refuge from predation (Newbrey et al. Citation2005, Roth et al. Citation2007, Smokorowski and Pratt Citation2007, Ahrenstorff et al. Citation2009). Hence, CWH abundance and complexity in lentic waters impact fishes’ abundance, spatial distribution, and feeding ecology (Newbrey et al. Citation2005, Ahrenstorff et al. Citation2009, Sass et al. Citation2012, Radinger et al. Citation2023).
Gravel pit lakes, also known as quarry lakes, are created through anthropogenic excavation of littoral resources such as sand or gravel (Mollema and Antonellini Citation2016, Blanchette and Lund Citation2016). In regions with a sparse abundance of natural lakes, gravel pit lakes dominate the lake landscape and can constitute the most frequent lentic waterbody type (Søndergaard et al. Citation2018, Nikolaus et al. Citation2020, Seelen et al. Citation2021). Gravel pit lakes are quickly colonized by organisms and can act as important secondary habitats for biodiversity conservation in agriculture-dominated landscapes (Santoul et al. Citation2004, Rey-Boissezon and Joye Citation2012, Biggs et al. Citation2017, Nikolaus et al. Citation2021). However, due to the young age of less than 100 yrs (Søndergaard et al. Citation2018, Matern et al. Citation2019, Seelen et al. Citation2021), the colonization and succession of the gravel pit lakes are ongoing (Müllerová et al. Citation2022). The density and complexity of CWH can thus be expected to be lower than in natural lakes, because CWH in lakes is known to accumulate over time, typically remaining in lakes for several centuries, depending on the type of tree and branch complexity and density (Guyette and Cole Citation1999).
CWH recruitment has already been studied in natural lakes (e.g., Christensen et al. Citation1996, Marburg et al. Citation2006), but no studies exist for gravel pit lakes. Lake size, littoral water depth, riparian tree density, wind intensity, beaver presence, and human-induced shoreline development have all been identified as relevant factors impacting CWH recruitment, abundance, and complexity in lentic ecosystems (Christensen et al. Citation1996, Mallory et al. Citation2000, Bozek Citation2001, Marburg et al. Citation2006, Sass Citation2009). In this context, residential development and other human actions, such as fishing site construction, have been reported to reduce CWH density in North American lakes (Christensen et al. Citation1996, Marburg et al. Citation2006). As many gravel pit lakes in Germany are intensively used by humans (Meyerhoff et al. Citation2019), it is possible that CWH density is negatively affected by both the young age of artificial lakes and shoreline development actions.
Many gravel pit lakes in Europe are managed by and for recreational fisheries (Arlinghaus et al. Citation2015, Zhao et al. Citation2016, Umweltbundesamt Citation2021, Seelen et al. Citation2022). The intensive use of nearshore habitats by anglers and other recreationists can negatively affect littoral wildlife and change the habitat quality of the littoral zone (O’Toole et al. Citation2009, Kaufmann et al. Citation2014a, Schafft et al. Citation2021, Nikolaus et al. Citation2022). As angling activities are often shore-bound, it is also possible that anglers, or fishing clubs more generally, remove CWH to clean shorelines in an attempt to reduce the potential for snagging of fishing lines or hooks (Arlinghaus et al. Citation2023). One indication for such effects would be a greater accumulation of CWH in steeper sloped shorelines where shoreline anglers have less access to sunken CWH than in shallower shorelines. CWH can also be supplemented as management action to improve habitat conditions. Anglers and managers also believe in the functionality of habitat management (Klefoth et al. Citation2023), but rather invest in fish stocking (Arlinghaus et al. Citation2022).
We investigated the CWH recruitment in the littoral zones of 26 gravel pit lakes in Lower Saxony, Germany. We tested the following hypotheses:
1. The CWH density in the littoral zone of gravel pit lakes is positively influenced by lake size, lake age, and riparian tree abundance, especially with wind exposure.
2. The presence of recreational-fisheries management negatively influences the amount of CWH, especially in shallow areas due to CWH removal as management action for improved angling access.
3. Due to their young age, CWH density in gravel pit lakes is lower than in natural lakes.
Methods
Study site and management of the lakes
All 26 sampled gravel pit lakes were located in Lower Saxony, Germany (), with 16 gravel pit lakes managed by angling clubs and 10 unmanaged gravel pit lakes owned by private persons or nature conservation agencies (Table S1). Angling clubs buy or rent the fishing rights of gravel pit lakes, often during or shortly after the excavation process. Subsequent utilization and management encompass fish stocking with a desired native species mix (Matern et al. Citation2019, Citation2022), recreational angling including harvest regulations (e.g., minimum-length and daily bag limits; Arlinghaus et al. Citation2016), and habitat management (e.g., creating angling sites or dead wood supplement for fish; Arlinghaus and Mehner Citation2005, Sass Citation2009). Importantly, no dead wood supplementations as (fisheries) management action were done in the studied lakes before sampling. In the unmanaged lakes recreational fishing was prohibited and the lakes did not receive initial fish stocking or shoreline development to create fishing sites. However, most of our gravel pit lakes were accessible to the public, and recreational visitors (e.g., walkers and dog walkers) were documented at all lakes.
Data collection
We sampled littoral CWH abundance and environmental variables in each lake either during June and July 2017 or August 2018 at multiple transects per lake, following 2 approaches: (1) For all lakes, prior to sampling, the location for the first transect was chosen randomly, and subsequently other transects were placed equidistantly along the whole shoreline. Distances between sampling points were constant within each lake and varied between 100 and 200 m depending on lake size. (2) In 8 of the studied lakes, additional transects were placed randomly within the lake (6 to 9 per lake) based on objectives in other studies not reported here. Thus, mainly due to variation in lake size but also due to the additional transects sampled, the number of transects varied across lakes. We kept the additionally sampled transects in the dataset to increase the sample size of transects, while a robustness analysis revealed similar results also without these transects. We divided each transect into a riparian zone plot and a littoral zone plot (). The riparian plot measured 10 × 10 m and all wood was counted and classified into 3 height categories (small: <3 m, medium: 3–10 m, and high: >10 m). The littoral zone plot measured 6 m in width and was investigated to a maximum water depth of 3 m; in cases of shallow zones, plot length was set to maximum 10 m. In the 6 lakes investigated in August 2018 the littoral zone plot measured only 2 m in width because of sampling time constraints. Water depth after every meter was measured with a tape measure attached to a stick to calculate the littoral slope. The total length of the littoral plot was noted to calculate the size of the littoral plot. We assessed all CWH structures including all sticks, even those less than 5 cm in length (), by snorkeling. We defined 3 criteria for CWH classification: (1) CWH length ≥ 50 cm; (2) CWH diameter ≥ 5 cm; and (3) complexity ≥ 2. Complexity was determined according to the maximum number of branch orders (1 = main trunk/1st order branch; 2 = 2nd order branch; 3 = 3rd order branch; 4 = 4th order branch; and 5 = 5th order branch; following Newbrey et al. Citation2005). Each CWH structure that fulfilled at least 2 of the 3 criteria was classified as large CWH and measured in detail (length, diameter, complexity, and percentage of submersion). All other CWH structures were classified as small CWH and their length was noted.
Figure 2. Sampling design adapted and modified after Newbrey et al. (Citation2005) and Kaufmann et al. (Citation2014b). In 2018 the width of the littoral zone plot was reduced to 2 m due to time constraints.
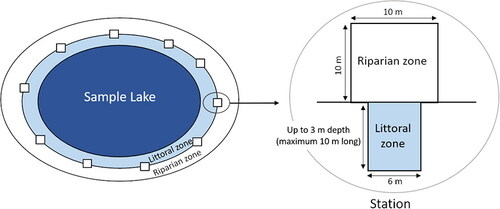
Figure 3. Diversity of coarse woody habitat (CWH) structures in gravel pit lakes: Length, bole diameter, and complexity were criteria used to define structures as either small CWH (A) and (B) or large CWH (C) and (D). CWH structures fulfilling at least 2 of the following criteria were classified as large CWH: (1) CWH length ≥50 cm; (2) CWH diameter ≥5 cm, and (3) complexity ≥2. All other CWH structures were classified as small CWH.
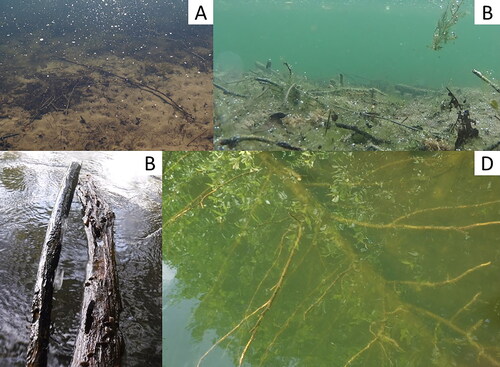
We measured lake area using QGIS (QGIS Development Team Citation2019) and used contour maps to extract information on mean and maximum lake depth (compare Matern et al. Citation2019, Nikolaus et al. Citation2021). At the water surface in the middle of the lake we measured pH value and Secchi disk transparency and took a water sample to analyze total phosphorus concentration in the lab (ISO Citation2004). For this study, we used the end of excavation to calculate gravel pit age (years). The proportion of forest within 100 m around each lake (buffer zone) was calculated in QGIS 3.4.1 with GRASS 7.4.2 using ATKIS land use data with a 10 × 10 m grid scale (© GeoBasis-DE/BKG 2013; AdV Citation2006). We used the ATKIS objects categorized as forest (economically) and wood (naturally), which included "AX_Wald” (ATKIS-ID 43002), “AX_Gehoelz” (ATKIS-ID 43003), “AX_Vegetationsmerkmal” with attribute “Baumbestand, Laubholz” (ATKIS-ID 540011021), “AX_Vegetationsmerkmal” with attribute “Baumbestand, Nadelholz” (ATKIS-ID 540011022), “AX_Vegetationsmerkmal” with attribute “Baumbestand, Laub- und Nadelholz” (ATKIS-ID 540011023), and “AX_Vegetationsmerkmal” with attribute “Gehölz” (ATKIS-ID 540011050).
The general wind direction at each lake was calculated using data from the Deutscher Wetterdienst ([DWD] Citation2018), which provides historical station observations at 10 min intervals for Germany. We used data running from 2015 to 2017 to have a measure of wind direction representing multiple years before this study. For each lake, we used the data of the nearest meteorological station (mean distance ± SD: 22.1 ± 10.3 km). Wind directions were categorized according to the 8 cardinal and ordinal directions. To break branches off trees, a wind power of 8 Beaufort or higher is needed (DWD Citation2018), and we therefore considered the most prevalent wind direction with a power of 8 Beaufort or higher as the general wind direction for each lake. For each plot, the wind exposure was assessed by calculating the angle (degrees) between the general wind direction and the shoreline of the plot (0°; 45°; 90°; −45°; −90°). Positive values indicate wind coming from land (90° being orthogonal to the shoreline) and negative values indicate wind coming from water (–90° being orthogonal to the shoreline). An angle of 0° indicates wind parallel to the shoreline.
Data analysis
We calculated the density of small and large CWH per area (number [N]/m) of each littoral plot. We tested all environmental variables (lake area, lake age, wind direction, forest cover in the 100 m buffer around the lake, littoral slope of the plot, transect water depth, density of small riparian trees [<3 m high], density of medium-sized riparian trees [3–10 m high], density of tall riparian trees [>10 m high], and density of all riparian trees) for collinearity using a stepwise variance inflation factor (VIF) selection. We chose a strict VIF cutoff value of 2.5 in line with literature (Johnston et al. Citation2018), to reduce collinearity, but kept important environmental variables. A robustness check revealed that even a more liberal cutoff value of 5 (Craney and Surles Citation2002) did not change the results compared to the cutoff value of 2.5. Based on the results from the VIF analysis, we removed the following variables: transect water depth, density of small riparian trees, and density of all riparian trees from the further analysis. We ran 2 linear mixed-effects models to predict the number of small and large CWH separately as a function of lake age, wind direction, percentage of forest cover in the 100 m buffer around the lake, density of medium-sized riparian trees, density of tall riparian trees, littoral slope of the plot, and fisheries management (present/absent; see for CWH classification). We integrated the log-transformed littoral sampling area as offset to correct for different sampling efforts in the linear mixed-effects models from the package glmmTMB with negative binomial distribution: linear parameterization as family (version 1.0.1; Brooks et al. Citation2017). We included interaction effects between density of medium-sized riparian trees and wind direction, density of tall riparian trees and wind direction, and the littoral slope of the plot and management in both models to test hypotheses 1 and 2, and used lake as a random factor to avoid the pseudoreplication of transects within lakes. We used the “stepAIC” function from the package MASS (version 7.3–51.4; Venables and Ripley Citation2002) for model selection in both directions and added the results to the Supplement (Table S2, Table S3). The significance level was set to an alpha value of 0.05. All statistical analyses were conducted using the software R 3.6.2 (R Core Team Citation2019).
No data on CWH densities were available from German natural lakes. We therefore used data from temperate, natural lakes in North America as the best available surrogate. Coarse woody habitat data were extracted from Christensen et al. (Citation1996) with a bole diameter ≥ 5 cm and from Pearce et al. (Citation2022) with a bole diameter ≥ 10 cm, and CWH densities were compared with the sampled gravel pit lakes. We included all large CWH structures from the sampled gravel pit lakes with matching bole diameter definitions to achieve comparable datasets and compared CWH densities using a nonparametric Wilcoxon test (Wilcoxon Citation1945).
Results
Lake environments and CWH abundance
The sampled gravel pit lakes varied in size between 0.9 ha and 19.5 ha (mean ± SD: 6.5 ± 5.2 ha) with a mean lake depth between 0.6 and 11.9 m (mean ± SD: 4.6 ± 2.5 m; ). The gravel pit lakes were on average mesotrophic with a total phosphorus concentration of 29.9 ± 30.6 µg/L (mean ± SD) and a Secchi disk transparency of 2.7 ± 1.5 m (mean ± SD; Table S4). The age of the gravel pit lakes ranged from 7 to 55 yrs (mean ± SD: 27.5 ± 13.2 yrs). The slope of the littoral plot varied between 1.1° and 40.6° on transect level and 4.8° and 27.2° on lake level. Between 0% and 72.6% of the 100 m buffer around the lakes was covered by forest (mean ± SD: 16.1 ± 21.5%; ). We detected 12,160 small CWH structures distributed over all gravel pit lakes, with 259 out of 291 transects containing small CWH structures. In total, 4012 of the small CWH structures (33.0%) measured between 1 and 20 cm, 4791 small CWH structures (39.4%) measured between 21 and 50 cm, and 3357 small CWH structures (27.6%) were longer than 50 cm. The density of small CWH was 1.47 ± 1.77 N/m (mean ± SD) and ranged from 0.17 to 6.18 N/m in the gravel pit lakes. We further found 620 large CWH structures distributed over all gravel pit lakes and in 193 out of 291 sampled transects. Large CWH structures measured 181 ± 149 cm (mean ± SD) in length with a bole diameter of 4.8 ± 6.9 cm (mean ± SD). The complexity of the large CWH structures was 2.5 ± 1.3 (mean ± SD) with 93.0 ± 16.4% (mean ± SD) being submerged. The density of large CWH was 0.07 ± 0.10 N/m (mean ± SD) and ranged from 0.004 to 0.53 N/m in the gravel pit lakes.
Table 1. Environmental description of the studied gravel pit lakes.
Table 2. Riparian wood and tree descriptors and coarse woody habitat (CWH) densities of the studied gravel pit lakes.
Predictors of CWH recruitment
For both models no variables were dropped after model selection ( and ). We detected large CWH density in gravel pit lakes to be significantly affected by lake age (P = 0.049) and the interaction of littoral slope and management (P < 0.001; ). Densities of large CWH increased with lake age, and in managed lakes large CWH densities increased with increasing littoral slope, while large CWH densities in unmanaged lakes were not affected by littoral slope (). Furthermore, large CWH densities were positively affected by tall trees on the shore (P = 0.06) and an interaction of tall trees on the shore and wind direction (P = 0.07, , ); however, effects were not significant at a significance level of 0.05. Small CWH density in gravel pit lakes was significantly affected by tall trees on the shore (P < 0.001) and the interaction of littoral slope and management (P < 0.001; ). Densities of small CWH increased with the number of large trees on the shore and similarly for large CWH, the density of small CWH increased in managed lakes with increasing littoral slope, and small CWH densities in unmanaged lakes were not affected by littoral slope (). Furthermore, and similar to large CWH densities, small CWH densities were positively affected by lake age, but the effect was not significant (P = 0.09; , ).
Figure 4. Marginal effect plots for the densities of large, coarse woody habitat (CWH) in gravel pit lakes impacted by lake age (a), the interaction of large tree density on the shore and wind direction (b), and the interaction of littoral slope and lake management (c). The shaded region indicates the confidence intervals.
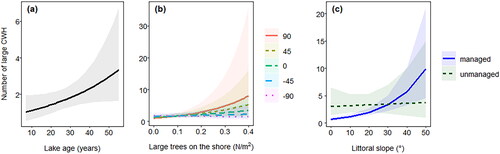
Figure 5. Marginal effect plots for the densities of small, coarse woody habitat (CWH) in gravel pit lakes impacted by large tree density on the shore (a), lake age (b), and the interaction of littoral slope and lake management (c). The shaded region indicates the confidence intervals.
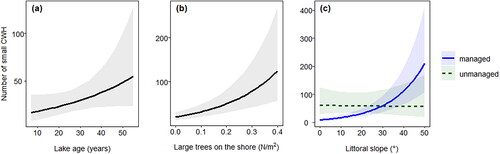
Table 3. Model output from the linear mixed-effects model predicting densities of large coarse woody habitat (CWH) in gravel pit lakes.
Table 4. Model output from the linear mixed-effects model predicting densities of small coarse woody habitat (CWH) in gravel pit lakes.
We detected significantly lower densities of CWH structures with a bole diameter ≥ 5 cm in gravel pit lakes in Germany compared to natural lakes in the United States (Wilcoxon test: W = 126, P = 0.035) and significantly lower densities of CWH structures with a bole diameter ≥ 10 cm compared to natural lakes in Canada (Wilcoxon test: W = 9, P < 0.001, ).
Figure 6. Comparison of coarse woody habitat (CWH) densities in gravel pit lakes in Lower Saxony, Germany, and (a) natural lakes in northern Wisconsin and Upper Michigan, United States, with a bole diameter ≥ 5 cm (data extracted from Christensen et al. Citation1996) and (b) natural lakes in Ontario, Canada with a bole diameter ≥ 10 cm (data extracted from Pearce et al. Citation2022). Boxes represent the 25th to the 75th percentile, with the median represented by the thick horizontal line; whiskers display 1.5 times the interquantile range and filled dots display outliers. Circles represent the observed data.
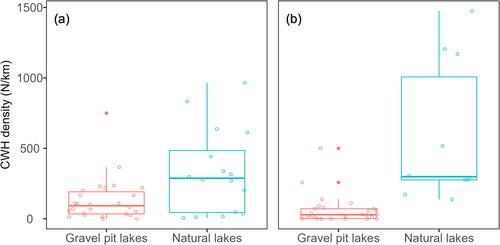
Discussion
We studied the recruitment of small and large CWH in gravel pit lakes and found, in agreement with hypothesis 1, that lake age and the number of tall trees, especially with wind exposure, affected CWH densities. We further detected, in support of hypothesis 2, an influence of recreational-fisheries management on CWH, with lowest densities in shallow water areas of managed lakes. Finally, and agreeing with hypothesis 3, CWH densities were significantly lower in German gravel pit lakes compared to natural lakes in Wisconsin and Ontario. Therefore, all 3 hypotheses received empirical support in our work.
We detected tall trees on the shore as one of the main drivers for CWH densities in gravel pit lakes, especially in combination with wind direction (and power). Broken branches of riparian trees and trees felled by wind are a key source of littoral dead wood in littoral habitats of lakes (Sass Citation2009), and expectedly, they also influence CWH in gravel pit lakes. Relatedly, forest cover and riparian coarse woody habitat have been reported to positively affect CWH density in lakes in Wisconsin, United States (Jennings et al. Citation2003, Marburg et al. Citation2006). However, our variable of forest cover in a 100 m buffer around the lakes did not add significant information to the model, most likely because only trees in the immediate riparian zone contribute to littoral CWH densities. Furthermore, the succession in the riparian zone of gravel pit lakes is often still in progress (Müllerová et al. Citation2022), and potentially outdated land use data are not useful as descriptors of littoral CWH. In addition to riparian trees, beavers have been identified as a further important source of littoral CWH in lakes and rivers (Sass Citation2009). However, we only detected beavers at a single gravel pit lake in our dataset and therefore did not integrate this in our analysis. Lake shape has also been revealed as a good predictor of littoral CWH (Marburg et al. Citation2006). In our transect-based sampling design, we would expect effects of lake shape only in certain transects (e.g., bays), but less on a whole-lake level. We therefore integrated other environmental variables (e.g., forest cover in the 100 m buffer and lake size) that appropriately describe data in our analysis.
The gravel pit lakes in our study varied in age between 7 and 55 yrs, and, as expected, we found increased CWH densities with increasing lake age, most likely because an older age allows trees to develop and branches and other structures to occasionally die off and fall into the lake. In line with this argument, CWH densities were significantly higher in natural temperate lakes in North America that have existed for more than 10,000 yrs (Mandrak and Crossman Citation1992) compared to the relatively young gravel pit lakes in this study (≤ 55 yrs). Similar to the natural lakes from North America, natural lakes in northern Germany are remnants from the last ice age, while natural lakes worldwide have also resulted from other processes (e.g., tectonic shifts). Hence, the literature data used for comparison do not represent natural lakes worldwide, but allow for an appropriate comparison. Our findings demonstrate the effect of lake age on littoral CWH densities in initially CWH-free waterbodies, while waterbodies such as reservoirs might have high CWH densities due to their genesis by flooding areas that are potentially covered by trees. In gravel pit lakes CWH must accumulate (over time) through growth and decay of riparian vegetation. In North American lakes wood input rates ranged from 0.5 to 1.9 logs/km/yr (Marburg et al. Citation2009) with retention times of up to several centuries (Guyette and Cole Citation1999). Hence, our findings are in line with the literature and we can conclude that CWH densities in gravel pit lakes are impacted by lake age.
Course woody habitat densities in gravel pit lakes managed for recreational fisheries were strongly impacted by littoral slope, while no effect of littoral slope on CWH densities was found in unmanaged gravel pit lakes. In North American lakes, shoreline development typically for housing and to support boating has been detected as a major factor influencing littoral CWH (Christensen et al. Citation1996, Jennings et al. Citation2003, Marburg et al. Citation2006). Our gravel pit lakes were largely free from boating and housing. However, in Germany, angling clubs regularly meet to complete annual clean-up activities on the shoreline, and in this context very likely remove fallen trees and other dead wood to improve access to anglers for shoreline fishing. Moreover, individual anglers likely remove wood that is entangled in the fishing line during fishing operations or may otherwise remove wood that is accessible in shallow water through wading into the shallow littoral (Arlinghaus et al. Citation2023). It is likely that these actions explain the significant interaction term we found among fisheries management and littoral slope, as the clean-up activities are more easily completed in shallow nearshore areas, leaving wood at steeply sloped shores but not in shallow sloped ones. As a limitation, we only differentiated between angler-managed and unmanaged gravel pit lakes, but we cannot refer the lower CWH densities in the shallow nearshore areas to specific actions (e.g., CWH removal by individual anglers or CWH removal as management measure). In line with our findings, Mallory et al. (Citation2000) also found higher CWH densities in deeper areas of the littoral zone and human impacts on CWH density and distribution. Our findings support the assumption that the littoral CWH densities were lowest in shallow and easily reachable parts of the lake, while CWH densities increased with steeper slopes of the banks.
Limitations
We sampled environmental data as representatively as possible, but weather stations were sometimes several kilometers away from lakes (mean distance ± SD: 22.1 ± 10.3 km). However, our sampling area was characterized by an overall wind direction from the west, which was also true for all weather stations. Accordingly, our wind data should appropriately represent the wind conditions at each lake (Christensen et al. Citation1996, Marburg et al. Citation2006). Wind and wave action could relocate tree-specific CWH after it enters the lakes, but we were unable to measure these details within our study lakes.
Our analysis revealed a significant effect of lake age on densities of large CWH but not on small CWH. By contrast, the effect of large trees on the shoreline was only significant on small CWH densities but not on large CWH densities. Future research may improve upon this study by including very old and highly vegetated gravel pit lakes. Nevertheless, our study demonstrated that lake age and trees on the shoreline affect CWH recruitment in gravel pit lakes, supporting findings from other studies (Christensen et al. Citation1996, Marburg et al. Citation2006).
Conclusions and implications for management
We conclude that CWH densities in the littoral zone of gravel pit lakes are mainly driven by lake age and the number of tall trees on the shore, especially with wind exposure. We further conclude that CWH clearance in shallow lake areas as a measure of recreational-fisheries management negatively affects CWH densities. CWH in the littoral zone represents an important habitat for fishes and other aquatic organisms in gravel pit and natural lakes (Sass et al. Citation2012, Czarnecka Citation2016, Matern et al. Citation2021, Maday et al. Citation2023). CWH supplementations can also promote abundance of certain fish species (Theis et al. Citation2023), but do not necessarily promote overall lake fish abundance (Sass et al. Citation2012, Radinger et al. Citation2023). In certain situations, the removal of littoral CWH has been found to decrease the abundance of typical lake fish species (Helmus and Sass Citation2008, Gaeta et al. Citation2014) and can also negatively affect aquatic invertebrates (Benke and Wallace Citation2003, Czarnecka Citation2016), especially when alternative habitats are missing (Smokorowski et al. Citation2021). We thus recommend that anglers and fisheries managers retain CWH in the small and low structured littoral zones of gravel pit lakes. Moreover, planting of shorelines to increase the presence of trees and fostering rehabilitation of beavers could increase the CWH density in gravel pit lakes.
Author contributions
Sven Matern: conceptualization of the study, data analysis and interpretation, manuscript preparation. Charlotte Robichon: conceptualization of the study, data generation, data analysis and interpretation, manuscript preparation. Robert Nikolaus: conceptualization of the study, data generation, data analysis and interpretation, manuscript editing. Christopher T. Monk: data analysis and interpretation, manuscript editing. Robert Arlinghaus: conceptualization of the study, data interpretation, manuscript preparation, funding.
Acknowledgments
The authors thank all the people participating in the fieldwork, namely, Leander Höhne, Andreas Maday, and Stéphane Mutel. We thank Angelsportverein Leer u. Umgebung e.V., Bezirksfischereiverband für Ostfriesland e.V., Angler-Verein Nienburg e.V., ASV Neustadt am Rübenberge e.V., Fischereiverein Hannover e.V., Niedersächsisch-Westfälische Anglervereinigung e.V., Stiftung Naturschutz im Landkreis Rotenburg (Wümme), Henning Scherfeld, FV Peine-Ilsede u. Umgebung e.V., SFV Helmstedt u. Umgebung e.V., Verein der Sportfischer Verden (Aller) e. V., Verein für Fischerei und Gewässerschutz Schönewörde u. Umgebung e.V., Steffen Göckemeyer, Thomas Reimer, Melanie and Heinz H. Nordmeyer, Achaz von Hardenberg, Johann Augustin, Dieter Klensang, Elke Dammann, Holcim Germany, and the Angler Association of Lower Saxony for participating in this study. We thank the 3 reviewers for their constructive feedback that further improved the article. This work was jointly supported by the German Federal Ministry of Education and Research (BMBF) and the German Federal Agency for Nature Conservation (BfN) with funds granted by the German Federal Ministry for the Environment, Nature Conservation and Nuclear Safety (BMU; grant number: 16LC1320A).
Disclosure statement
No potential conflict of interest was reported by the author(s).
References
- Ahrenstorff TD, Sass GG, Helmus MR. 2009. The influence of littoral zone coarse woody habitat on home range size, spatial distribution, and feeding ecology of largemouth bass (Micropterus salmoides). Hydrobiologia 623(1):223–233. doi: 10.1007/s10750-008-9660-1.
- [AdV] Arbeitsgemeinschaft der Vermessungsverwaltungen der Länder der Bundesrepublik Deutschland [Working Committee of the Surveying Authorities of the States of the Federal Republic of Germany] 2006. Documentation on the Modelling of Geoinformation of Official Surveying and Mapping (GeoInfoDok). Chapter 5; Section 5.4; Version 5.1; [cited 07 July 2023]. Available from https://www.adv-online.de
- Arlinghaus R, Cyrus E-M, Eschbach E, Fujitani M, Hühn D, Johnston F, Pagel T, Riepe C. 2015. Hand in Hand für eine nachhaltige Angelfischerei Ergebnisse und Empfehlungen aus fünf Jahren praxisorientierter Forschung zu Fischbesatz und seinen Alternativen. Vol. 28. Berlin: Berichte Des IGB.
- Arlinghaus R, Klefoth T, Matern S, Radinger J, Nikolaus R, Meyerhoff J, Schafft M, Cyrus E-M, Emmrich M, Hering D, et al. 2023. Biodiversität, Angeln und Gesellschaft: Wissensbasierte Empfehlungen für ein nachhaltiges Fischereimanagement an Baggerseen. Vol. 32. Berlin: Berichte des IGB.
- Arlinghaus R, Lorenzen K, Johnston BM, Cooke SJ, Cowx IG. 2016. Management of freshwater fisheries: addressing habitat, people and fishes In: Craig J, editor. Freshwater Fisheries Ecology. Oxford: Blackwell Science. p. 557–579.
- Arlinghaus R, Mehner T. 2005. Determinants of management preferences of recreational anglers in Germany: Habitat management versus fish stocking. Limnologica 35(1-2):2–17. doi: 10.1016/j.limno.2004.10.001.
- Arlinghaus R, Riepe C, Theis S, Pagel T, Fujitani M. 2022. Dysfunctional information feedbacks cause the emergence of management panaceas in social-ecological systems: the case of fish stocking in inland recreational fisheries. J Outdoor Recreat. 38:100475. doi: 10.1016/j.jort.2021.100475.
- Benke AC, Wallace JB. 2003. Influence of wood on invertebrate communities in streams and rivers In: Gregory SV, Boyer KL, Gurnell AM, editors. The ecology and management of wood in world rivers. Bethesda, Maryland: Am Fish Soc Symp. p.149–177.
- Biggs J, von Fumetti S, Kelly-Quinn M. 2017. The importance of small waterbodies for biodiversity and ecosystem services: implications for policy makers. Hydrobiologia 793(1):3–39. doi: 10.1007/s10750-016-3007-0.
- Blanchette ML, Lund MA. 2016. Pit lakes are a global legacy of mining: an integrated approach to achieving sustainable ecosystems and value for communities. Curr Opin Environ Sustain. 23:28–34. doi: 10.1016/j.cosust.2016.11.012.
- Bozek MA. 2001. A second life for trees in lakes: as useful in water as they were on land. Lakeline.
- Brooks ME, Kristensen K, van Benthem KJ, Magnusson A, Berg CW, Nielsen A, Skaug HJ, Machler M, Bolker BM. 2017. glmmTMB balances speed and flexibility among packages for zero-inflated generalized linear mixed modeling. The R J. 9(2):378–400. doi: 10.32614/RJ-2017-066.
- Christensen DL, Herwig BR, Schindler DE, Carpenter SR. 1996. Impacts of lakeshore residential development on coarse woody debris in North Temperate Lakes. Ecol Appl.6(4):1143–1149. doi: 10.2307/2269598.
- Craney TA, Surles JG. 2002. Model-dependent variance inflation factor cutoff values. Qual Eng. 14(3):391–403. doi: 10.1081/QEN-120001878.
- Czarnecka M. 2016. Coarse woody debris in temperate littoral zones: implications for biodiversity, food webs and lake management. Hydrobiologia 767(1):13–25. doi: 10.1007/s10750-015-2502-z.
- Czarnecka M, Pilotto F, Pusch MT. 2014. Is coarse woody debris in lakes a refuge or a trap for benthic invertebrates exposed to fish predation? Freshw Biol. 59(11):2400–2412. doi: 10.1111/fwb.12446.
- Dossi F, Leitner P, Graf W. 2020. Age matters: substrate-specific colonization patterns of benthic invertebrates on installed large wood. Aquat Ecol. 54(3):741–760. doi: 10.1007/s10452-020-09772-y.
- [DWD] Deutscher Wetterdienst [German Meteorological Service] 2018. Historical 10-minute station observation of max/min – mean wind speed and wind gust for Germany, version V1. [cited 07 July 2023]. https://www.dwd.de
- Eadie JMcA, Keast A. 1984. Resource heterogeneity and fish species diversity in lakes. Can J Zool. 62(9):1689–1695. doi: 10.1139/z84-248.
- Gaeta JW, Sass GG, Carpenter SR. 2014. Drought-driven lake level decline: effects on coarse woody habitat and fishes. Can J Fish Aquat Sci. 71(2):315–325. doi: 10.1139/cjfas-2013-0451.
- Guyette RP, Cole WG. 1999. Age characteristics of coarse woody debris (Pinus strobus) in a lake littoral zone. Can J Fish Aquat Sci. 56(3):496–505. doi: 10.1139/f98-177.
- Helmus MR, Sass GG. 2008. The rapid effects of a whole-lake reduction of coarse woody debris on fish and benthic macroinvertebrates. Freshwater Biol. 53(7):1423–1433. doi: 10.1111/j.1365-2427.2008.01974.x.
- [ISO] International Organization for Standardization. 2004. Water quality – determination of phosphorus – ammonium molybdat spectrometric method.
- Jennings MJ, Emmons EE, Hatzenbeler GR, Edwards C, Bozek MA. 2003. Is littoral habitat affected by residential development and land use in watersheds of Wisconsin lakes? Lake Reserv Manag.19(3):272–279. doi: 10.1080/07438140309354092.
- Johnston R, Jones K, Manley D. 2018. Confounding and collinearity in regression analysis: a cautionary tale and an alternative procedure, illustrated by studies of British voting behaviour. Qual Quant. 52(4):1957–1976. doi: 10.1007/s11135-017-0584-6.
- Kaufmann PR, Peck DV, Paulsen SG, Seeliger CW, Hughes RM, Whittier TR, Kamman NC. 2014a. Lakeshore and littoral physical habitat structure in a national lakes assessment. Lake Reserv Manag. 30(2):192–215. doi: 10.1080/10402381.2014.906524.
- Kaufmann PR, Hughes RM, Van Sickle J, Whittier TR, Seeliger CW, Paulsen SG. 2014b. Lakeshore and littoral physical habitat structure: a field survey method and its precision. Lake Reserv Manag. 30(2):157–176. doi: 10.1080/10402381.2013.877543.
- Klefoth T, Wegener N, Meyerhoff J, Arlinghaus R. 2023. Do anglers and managers think similarly about stocking, habitat management and harvest regulations? Implications for the management of community-governed recreational fisheries. Fish Res. 260:106589. doi: 10.1016/j.fishres.2022.106589.
- Kovalenko KE, Thomaz SM, Warfe DM. 2012. Habitat complexity: approaches and future directions. Hydrobiologia 685(1):1–17. doi: 10.1007/s10750-011-0974-z.
- Lawson ZJ, Gaeta JW, Carpenter SR. 2011. Coarse woody habitat, lakeshore residential development, and largemouth bass nesting behavior. N Am J Fish Manag. 31(4):666–670. doi: 10.1080/02755947.2011.608990.
- Lewin W-C, Mehner T, Ritterbusch D, Brämick U. 2014. The influence of anthropogenic shoreline changes on the littoral abundance of fish species in German lowland lakes varying in depth as determined by boosted regression trees. Hydrobiologia 724(1):293–306. doi: 10.1007/s10750-013-1746-8.
- Lewin W-C, Okun N, Mehner T. 2004. Determinants of the distribution of juvenile fish in the littoral area of a shallow lake. Freshwater Biol. 49(4):410–424. doi: 10.1111/j.1365-2427.2004.01193.x.
- Maday A, Matern S, Monk CT, Klefoth T, Wolter C, Arlinghaus R. 2023. Seasonal and diurnal patterns of littoral microhabitat use by fish in gravel pit lakes, with special reference to supplemented deadwood brush piles. Hydrobiologia 850(7):1557–1581. doi: 10.1007/s10750-023-05152-3.
- Mallory EC, Ridgway MS, Gordon AM, Kaushik NK. 2000. Distribution of woody debris in a small headwater lake, central Ontario, Canada. Fal. 148(4):587–606. doi: 10.1127/archiv-hydrobiol/148/2000/587.
- Mandrak NE, Crossman EJ. 1992. Postglacial dispersal of freshwater fishes into Ontario. Can J Zool. 70(11):2247–2259. doi: 10.1139/z92-302.
- Marburg AE, Bassak SB, Kratz TK, Turner MG. 2009. The demography of coarse wood in north temperate lakes. Freshw Biol. 54(5):1110–1119. doi: 10.1111/j.1365-2427.2008.02158.x.
- Marburg AE, Turner MG, Kratz TK. 2006. Natural and anthropogenic variation in coarse wood among and within lakes: coarse wood in lakes. J Ecol. 94(3):558–568. doi: 10.1111/j.1365-2745.2006.01117.x.
- Matern S, Emmrich M, Klefoth T, Wolter C, Nikolaus R, Wegener N, Arlinghaus R. 2019. Effect of recreational-fisheries management on fish biodiversity in gravel pit lakes, with contrasts to unmanaged lakes. J Fish Biol. 94(6):865–881. doi: 10.1111/jfb.13989.
- Matern S, Klefoth T, Wolter C, Arlinghaus R. 2021. Environmental determinants of fish abundance in the littoral zone of gravel pit lakes. Hydrobiologia 848(10):2449–2471. doi: 10.1007/s10750-021-04563-4.
- Matern S, Klefoth T, Wolter C, Hussner A, Simon J, Arlinghaus R. 2022. Fish community composition in small lakes: the impact of lake genesis and fisheries management. Freshw Biol. 67(12):2130–2147. doi: 10.1111/fwb.14001.
- Meyerhoff J, Klefoth T, Arlinghaus R. 2019. The value artificial lake ecosystems provide to recreational anglers: implications for management of biodiversity and outdoor recreation. J Environ Manage. 252:109580. doi: 10.1016/j.jenvman.2019.109580.
- Mollema PN, Antonellini M. 2016. Water and (bio)chemical cycling in gravel pit lakes: a review and outlook. Earth Sci Rev. 159:247–270. doi: 10.1016/j.earscirev.2016.05.006.
- Müllerová A, Řehounková K, Prach K. 2022. Succession of aquatic and littoral vegetation in disused sandpits. Land Degrad Dev. 33(2):257–268. doi: 10.1002/ldr.4142.
- Naiman RJ, Décamps H. 1997. The ccology of interfaces: riparian zones. Annu Rev Ecol Syst. 28(1):621–658. doi: 10.1146/annurev.ecolsys.28.1.621.
- Nash KT, Hendry K, Cragg-Hine D. 1999. The use of brushwood bundles as fish spawning media: brushwood bundles as fish-spawning material. Fish Manag Ecol. 6(5):349–356. doi: 10.1046/j.1365-2400.1999.00153.x.
- Newbrey MG, Bozek MA, Jennings MJ, Cook JE. 2005. Branching complexity and morphological characteristics of coarse woody structure as lacustrine fish habitat. Can J Fish Aquat Sci. 62(9):2110–2123. doi: 10.1139/f05-125.
- Nikolaus R, Matern S, Schafft M, Klefoth T, Maday A, Wolter C, Manfrin A, Lemm JU, Arlinghaus R. 2020. Einfluss anglerischer Bewirtschaftung auf die Biodiversität von Baggerseen: Eine vergleichende Studie verschiedener gewässergebundener Organismengruppen. Lauterbornia 87:153–187.
- Nikolaus R, Matern S, Schafft M, Maday A, Wolter C, Klefoth T, Arlinghaus R. 2022. Influence of protected riparian areas on habitat structure and biodiversity in and at small lakes managed by recreational fisheries. Fish Res. 256:106476. doi: 10.1016/j.fishres.2022.106476.
- Nikolaus R, Schafft M, Maday A, Klefoth T, Wolter C, Arlinghaus R. 2021. Status of aquatic and riparian biodiversity in artificial lake ecosystems with and without management for recreational fisheries: implications for conservation. Aquatic Conserv: Mar Freshw Ecosyst. 31(1):153–172. doi: 10.1002/aqc.3481.
- O’Toole AC, Hanson KC, Cooke SJ. 2009. The effect of shoreline recreational angling activities on aquatic and riparian habitat within an urban environment: implications for conservation and management. Environ Manage. 44(2):324–334. doi: 10.1007/s00267-009-9299-3.
- Pearce JL, Mallory EC, Smokorowski KE. 2022. Downed wood dynamics in the riparian and littoral zone of small lakes in tolerant hardwood forests. Can J For Res. 52(5):751–768. doi: 10.1139/cjfr-2021-0245.
- Pusey BJ, Arthington AH. 2003. Importance of the riparian zone to the conservation and management of freshwater fish: a review. Mar Freshwater Res. 54(1):1–16. doi: 10.1071/MF02041.
- QGIS Development Team. 2019. QGIS Geographic Information System. Open Source Geospatial Foundation [cited 07 July 2023]. Retrieved from http://qgis.osgeo.org
- R Core Team. (2019). R: a language and environment for statistical computing. R Foundation for Statistical Computing, Vienna, Austria. Retrieved from www.r-project.org/
- Radinger J, Matern S, Klefoth T, Wolter C, Feldhege F, Monk CM, Arlinghaus R. 2023. Ecosystem-based management outperforms species-focused stocking for enhancing fish populations. Science 379(6635):946–951. doi: 10.1126/science.adf0895.
- Rey-Boissezon A, Joye D. 2012. A temporary gravel pit as a biodiversity hotspot for aquatic plants in the Alps. Arch Des Sci. 65:177–190.
- Roth BM, Kaplan IC, Sass GG, Johnson PT, Marburg AE, Yannarell AC, Havlicek TD, Willis TV, Turner MG, Carpenter SR. 2007. Linking terrestrial and aquatic ecosystems: the role of woody habitat in lake food webs. Ecol Modell. 203(3-4):439–452. doi: 10.1016/j.ecolmodel.2006.12.005.
- Santoul F, Figuerola J, Green AJ. 2004. Importance of gravel pits for the conservation of waterbirds in the Garonne river floodplain (southwest France). Biodivers Conserv. 13(6):1231–1243. doi: 10.1023/B:BIOC.0000018154.02096.4b.
- Sass GG. 2009. Coarse woody debris in lakes and streams In: Likens GE, editor. Encyclopedia of Inland Waters. Oxford: Elsevier. Vol.1.
- Sass GG, Carpenter SR, Gaeta JW, Kitchell JF, Ahrenstorff TD. 2012. Whole-lake addition of coarse woody habitat: response of fish populations. Aquat Sci. 74(2):255–266. doi: 10.1007/s00027-011-0219-2.
- Schafft M, Wegner B, Meyer N, Wolter C, Arlinghaus R. 2021. Ecological impacts of water-based recreational activities on freshwater ecosystems: a global meta-analysis. Proc Biol Sci. 288(1959):20211623. doi: 10.1098/rspb.2021.1623.
- Schindler DE, Scheuerell MD. 2002. Habitat coupling in lake ecosystems. Oikos. 98(2):177–189. doi: 10.1034/j.1600-0706.2002.980201.x.
- Seelen LMS, Teurlincx S, Armstrong MR, Lürling M, van Donk E, de Senerpont Domis LN. 2022. Serving many masters at once: a framework for assessing ecosystem services delivered by quarry lakes. Inland Waters. 12(1):121–137. doi: 10.1080/20442041.2021.1944765.
- Seelen LMS, Teurlincx S, Bruinsma J, Huijsmans TMF, van Donk E, Lürling M, de Senerpont Domis LN. 2021. The value of novel ecosystems: disclosing the ecological quality of quarry lakes. Sci Total Environ. 769:144294. doi: 10.1016/j.scitotenv.2020.144294.
- Smokorowski KE, Pearce JL, Geiling WD, Pratt TC. 2021. Wood removals from lakes may not necessarily elicit fish population responses. North Am J Fish Manage. 41(1):142–157. doi: 10.1002/nafm.10543.
- Smokorowski KE, Pratt TC. 2007. Effect of a change in physical structure and cover on fish and fish habitat in freshwater ecosystems – a review and meta-analysis. Environ Rev. 15(NA):15–41. doi: 10.1139/a06-007.
- Smokorowski KE, Pratt TC, Cole WG, McEachern LJ, Mallory EC. 2006. Effects on periphyton and macroinvertebrates from removal of submerged wood in three Ontario lakes. Can J Fish Aquat Sci. 63(9):2038–2049. doi: 10.1139/f06-104.
- Søndergaard M, Lauridsen TL, Johansson LS, Jeppesen E. 2018. Gravel pit lakes in Denmark: chemical and biological state. Sci Total Environ. 612:9–17. doi: 10.1016/j.scitotenv.2017.08.163.
- Theis S, Ruppert JLW, Poesch MS. 2023. Coarse woody habitat use by local fish species and structural integrity of enhancements over time in a shallow northern boreal lake assessed in a Bayesian modelling approach. Ecol Solut Evid 4:e12200. doi: 10.1002/2688-8319.12200.
- Umweltbundesamt. 2021. Angelfischerei und Nachhaltigkeit in Österreich. Impulse zur nachhaltigen angelfischereilichen Nutzung von Gewässern. Wien.
- Venables WN, Ripley BD. 2002. Modern applied statistics with S. 4th ed. New York (NY): Springer.
- Wilcoxon F. 1945. Individual comparisons by ranking methods. Biometrics Bulletin. 1(6):80–83. doi: 10.2307/3001968.
- Zhao T, Grenouillet G, Pool T, Tudesque L, Cucherousset J. 2016. Environmental determinants of fish community structure in gravel pit lakes. Ecol Freshw Fish. 25(3):412–421. doi: 10.1111/eff.12222.