Abstract
The chemical group of polycyclic aromatic compounds (PAC), including the better-known subgroup of polycyclic aromatic hydrocarbons (PAH) and the heterocyclic aromatic compounds (NSO-PAC, heterocycles), comprise several thousand individual compounds. It is hard to find a comprehensive overview in the literature of these PACs that includes a substantial amount of relevant properties. Here an attempt is made to summarize the most studied but also some less well-known PACs. In addition to basic data such as recommended names, abbreviations, CAS numbers, molecular formulas, chemical structures, and exact mono-isotopic molecular weights, physico-chemical properties taken from the literature like boiling points, vapor pressures, water solubilities, Henry's Law constants, n-octanol-water partition coefficients (log KOW), and pKa are summarized. Selected toxicological data are listed indicating carcinogenic and mutagenic activity or effects on different organisms.
PAC nomenclature is a complex topic, so suggestions for practical use are made. Regarding available data, estimated (instead of measured) values should be used with caution because considerable deviations from experimentally determined values can occur. For an enhanced understanding of the behavior of single PACs in comparison with each other, some of the properties mentioned above are plotted vs. the number of rings or the degree of alkylation. Also, some physico-chemical data are correlated with different functional groups as substituents of the PAHs.
This article reveals that rather little is known about the less common PACs, e.g., higher molecular weight compounds, alkylated or otherwise substituted aromatics, for instance, keto-, oxo-, amino-, nitro-, cyano-PAHs, or some heterocyclic aromatic compounds, including their derivatives. It mirrors the limited state of knowledge about the variety of PACs that do not belong to the 16 EPA PAHs.
INTRODUCTION
The group of polycyclic aromatic hydrocarbons (PAH) is an extensively studied class of compounds in many fields of work, but the designation PAC is less used and hardly known despite its usefulness. It subsumes the group of PAHs and the group of heterocyclic aromatic compounds (NSO-PACs, heterocycles). It is important to realize that the term PAH does not cover heterocyclic compounds (Citation1).
There are thousands of polycyclic aromatic compounds (PACs) of interest to scientists (outside the synthetic field), mainly because of their ubiquity in the environment and in fossil materials and because of the deleterious influence on human health that some of them are known to possess. This also means that many scientists that do not count among the “hard-core” chemists study them with great intensity and immensely expand our knowledge on them and their occurrence, their transformation in the environment, including metabolism in various organisms. Work of this kind is amply documented in the contributions to this issue. Despite excellent research being done by them, some of these scientists may not have had a deep-going chemical introduction to PAC chemistry and therefore feel on uncertain ground when it comes to rules of naming the compounds, numbering of atoms, or drawing of structures.
Despite several very useful books on the topic of PAC chemistry, it is hard to find a comprehensive list of the majority of these PACs that can be at hand at all times to help beat such uncertainties. We have been encouraged to compile a range of data, not only on the 16 EPA PAHs but on such PACs that are commonly found in samples of various origins. Here, an attempt is made to give an overview of both well-known and less frequently encountered PACs. Recommended names, abbreviations, CAS numbers, molecular formulas, chemical structures, exact mono-isotopic molecular weights, and physico-chemical properties such as boiling points, vapor pressures, water solubilities, Henry's Law constants, n-octanol-water partition coefficients log KOW, and pKa are tabulated, if available. Finally, some known basic toxicological data are given including toxicity equivalent factors (TEF), as far as known. Lethal dose data (LD50, rat, mammals, oral pathway) are collected here as well as effect concentration (EC50) data from common toxicological test systems such as Vibrio fischeri (luminous bacteria) and Daphnia magna (water flea, water compartment).
Confusion about PAH nomenclature and names can be observed in the scientific community even at conferences such as the International Symposium on Polycyclic Aromatic Compounds (ISPAC). Also, it is frequently found that the preferred IUPAC name is not used by Chemical Abstracts. In order to reduce the uncertainty created by this undesirable situation, suggestions for PAC nomenclature for future practical use are made here.
USE OF THE PAC TABLE
Selected PACs are listed in Table 1 (see supplemental material). The compounds are ordered with respect to increasing ring number. First PAHs and particularly relevant alkylated PAHs are tabulated followed by PAHs with functional groups such as keto, hydroxy, nitro, amino, and cyano groups as well as carboxylic acids. Since PAHs often occur together with their heterocyclic analogs, PACs from the groups of oxygen, nitrogen, and sulfur heterocycles are also included.
Details concerning the nomenclature of PAHs are discussed by Ehrenhauser in this issue (Citation21) and will not be repeated here. Yet we would like to point to the particular importance of the CAS number since it unambiguously identifies a compound. A call for a more wide-spread use of these numbers in research publications, at least for compounds of lesser prominence, is made (Citation21).
Routinely formulas are encountered, also in reputable journals, that are not drawn according to the basic rules of IUPAC. We would like to highlight the most often committed errors and emphasize the following basic rules. The rings should always be oriented with one carbon atom at the top (and not two carbon atoms as often seen with phenanthrene) and in many cases the numbering system starts at the top carbon atom of the most top right ring (the right upper quadrant) continuing clockwise. From this perspective, some exceptions such as phenanthrene (including anti-clockwise numbering for historical reasons), fluoranthene, acenaphthylene, benzo[a]anthracene, and picene exist. In general, the double bonds should be organized in such a way that the ring at the bottom left (lower left quadrant) carries three double bonds (six π-electrons) which alternatively can be drawn as a circle within the six-membered ring. This circular symbol should indeed only be used if six π-electrons are available in the ring-structure, hence, e.g., phenanthrene should be drawn with circles in the two terminal rings but not in the center one (only two π-electrons are present).
Naming always begins with a parent basic unit such as naphthalene, phenanthrene, anthracene, pyrene, chrysene, benzo[a]anthracene, perylene, fluoranthene, fluorene, etc., and for larger molecular weight PACs, additional rings or functional groups are added to the basic units according to the corresponding rules for nomenclature. It is noted that, for a correct graphic representation, the structure may need to be rearranged and is then drawn with the longest row of rings horizontally and preferably most other additional atoms in the upper right quadrant (in case rotation and mirroring are necessary). For five-membered rings, cutoff hexagons (such as in Table 1 (see supplemental material)) should be drawn instead of regular pentagons. The letters for the locants of additional bound groups (the “a” in, e.g., benzo[a]pyrene) should always be italicized and set in square brackets. The letters are separated by a comma unless they are neighboring, e.g., cyclopenta[cd]pyrene (no comma) but dibenzo[a,l]pyrene (with comma). Here special care should be taken because it can easily happen that (automatized?) changes by the publisher introduce or delete a comma, perhaps because of the lack of understanding of the meaningful difference between the two writings. For the names, the current rules suggest always using an “o” for benzo (and never “benz” such as commonly found in, e.g., benz[a]anthracene). Also often seen is that at least one “h” in the names is lost (e.g., in naphthalene). The complex rules and many exceptions in the nomenclature of PACs may be one reason for the confusion in the literature in this field until today and we recommend the use of the nomenclature in Table 1 (see supplemental material).
![Figure 1 Comparison of experimental (EXP) and estimated (EST) data using the EPI suite (Citation9) for naphthalene, pyrene, benzo[a]pyrene, coronene, and 2-aminonaphthalene; estimated values should be used with care.](/cms/asset/bb5322af-b04a-475f-9dd3-3e4ecdfbae5e/gpol_a_994071_f0001_b.gif)
In the following, information on the use of Table 1 (see supplemental material) is given. Italic numbers in Table 1 (see supplemental material) refer to estimated values using the EPI Suite estimation model (Citation9) in contrast to normal script showing experimental data. The estimated values should be used with care as the comparison between experimental (EXP) and estimated (EST) data in shows. It becomes clear that true boiling points can differ by more than 100°C from the calculated value (e.g., benzo[a]pyrene), vapor pressures by about an order of magnitude (e.g., pyrene), water solubilities by about two orders of magnitude (e.g., benzo[a]pyrene), Henry's Law constants by about three orders of magnitude (e.g., benzo[a]pyrene), and log KOW values by about one log unit (e.g., coronene).
Some physico-chemical properties of the PAHs (including some alkylated derivatives) are plotted in – to demonstrate how they depend on the number of rings. Boiling points often lie in the following ranges (): 200–300°C (2-ring PAH), 300–400°C (3-ring PAH), 300–450°C (4-ring PAH), 350–500°C (5-ring PAH), and 500–600°C (6–10-ring PAH). Vapor pressures show a continuous decrease from 2-ring PAHs to 10-ring PAHs from about 1.00E+02 to 1.00E-11 Pa () and water solubilities from some tens to about 1.00E-04 mg/L (). the n-octanol-water partition coefficient log KOW increases from about 3–10 from 2-ring PAHs to 10-ring PAHs ().
The impact of the degree of alkylation is exemplarily shown in for naphthalene. It can be observed that increasing alkylation implies increasing hydrophobicity and lower vapor pressure. The polarity is significantly dependent on the presence of functional groups and an example for this is given in , where log KOW data for naphthalenes possessing different functional groups are plotted and some quinolines as the analogous nitrogen heterocycle. As expected, the quinolines show lower log KOW values than the corresponding naphthalenes, and alkylation increases the lipophilicity. The polar functional groups increase the hydrophilicity in the order NO2<COOH<OH<CN<NH2<dione which is relevant for studies on the uptake of environmental pollutants by organisms.
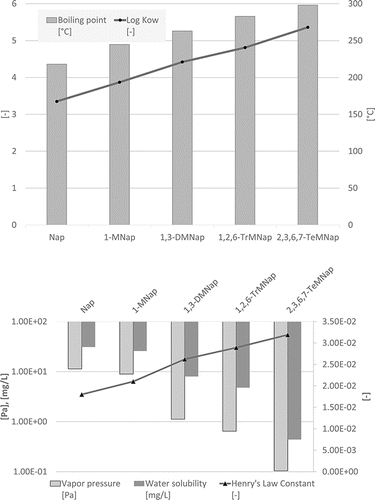
Since PAHs are used as markers for source identification in environmental work, some relevant aspects pertaining to such use are mentioned here in an extremely condensed way: retene or picene indicate biogenic origin, a dominance of alkylated PAHs over the parents is typical for a petrogenic origin, and appreciable concentrations of parent PAHs and linear polycyclic aromatic compounds (such as anthracene) vs. the corresponding angular isomers (such as phenanthrene) are indicative of a pyrogenic origin. Petrogenic alkylated PAHs show a bell-shaped distribution in contrast to a slope distribution of petrogenic compounds (see the contribution by Stout et al. (Citation22)). Also, petrogenic PAHs are enriched in compounds containing five-membered (non-aromatic) rings condensed onto the aromatic rings. These differences can be observed in the varying PAH patterns of coals during coal formation: compounds reflecting their biogenic origin dominate in coals of low maturity, followed by an increasing alkylation in the bituminous coal state and finally, with increasing thermal and diagenetic impact, a pyrogenic pattern is observed in anthracite. Other markers are discussed in Stout et al. (Citation22). Selected notes about markers are added in brackets in Table 1 (see supplemental material) following the compound names. Again it is noted that this list does not raise any claim to completeness, but we rather plan to continuously proceed to add data to it in the future.
Several PACs have been evaluated for their toxicity and the corresponding data are summarized in the last columns of Table 1 (see supplemental material). It is obvious that generally higher molecular weight PAHs (e.g., dibenzopyrenes) are more toxic than lower molecular weight compounds. Some alkylated compounds also show significant adverse effects (e.g., 5-methylchrysene or 6-methylpyrene). Not much is known about PAH toxicity for compounds with more than seven rings. Additionally, some PAHs with different functional groups such as nitro- or amino-PAHs and N-PAC and O-PACs are known for their toxicity. The table also uncovers that there are many compounds where we do not know anything about possible toxic effects. Obviously, here a research need is identified. In the column “toxicity”, “+” indicates that the compound has been evaluated officially to be carcinogenic by sufficient and limited evidence and “(0)” with inadequate evidence for evaluation. “I” indicates that at least in one study the compound was proven to be carcinogenic, mutagenic, or cytotoxic.
ACKNOWLEDGMENTS
Dr. Albrecht Seidel is kindly acknowledged for checking PAC nomenclature and Erik Achten for graphics assistance.
Supplemental Material
Supplemental data for this article can be accessed on the http://dx.doi.org/10.1080/10406638.2014.994071 publisher's website.
GPOL_A_994071_supplemental_material.zip
Download Zip (302.2 KB)REFERENCES
- Andersson, J.T. “PAH or PAC, that is the Question.” Polycyclic Aromatic Compounds 29 (2009): 1–2
- http://www.sisweb.com/referenc/tools/exactmass.htm (accessed November 20, 2014)
- chem.sis.nlm.nih.gov/chemidplus/ (accessed November 20, 2014)
- www.GSBL.de (accessed November 20, 2014)
- Greim, H. Gesundheitsschädliche Arbeitsstoffe, Toxikologische arbeitsmedizinische Begründungen von MAK-Werten und Einstufungen, DFG, Wiley-Verlag GmbH and Co. (Weinheim, Germany: KgaA, 2008); Vol 35. Lieferung.
- Umweltchemikalien, G. Rippen, ecomed Sicherheit, Verlagsgruppe Hüthig Jehle Rehm GmbH, 2014 (Estimations: EPISuite).
- NSO-Heterozyklen, Altlastenforum Baden-Württemberg e.V. Heft 12, E. Schweizerbartsche Verlagsbuchhandlung (Nägele u. Obermiller Stuttgart), Eds. Arbeitskreis Erfahrungsaustausch Sanierung und Beurteilung von Altlasten, Germany (2007).
- International Agency for Research on Cancer (IARC). World Health Organization, IARC Monograph on the Evaluation of Carcinogenic Risks to Humans, Vol. 92, “Some Non-heterocyclic Polycyclic Aromatic Hydrocarbons and Some Related Exposures,” Lyon, France (2010).
- www.Chemspider.com (accessed November 20, 2014); (EPI-Suite; Henrys Law Constant - Bond Method).
- USEPA RPF assessment 2010 draft; http://cfpub.epa.gov/ncea/iris_drafts/recordisplay.cfm?deid=194584 (accessed November 20, 2014).
- Calculated using Advanced Chemistry Development (ACD/Labs) Software V11.02 (copyright 1994–2014 ACD/Labs).
- Fetzer, J.C. “Large (C > = 24) Polycylic Aromatic Hydrocarbons, Chemistry and Analysis,” A Series of Monographs on Analytical Chemistry and its Applications, vol. 35, in Chemical Analysis, ed. J.D. Windefordner (Toronto: John Wiley & Sons, 2000).
- http://oehha.ca.gov/air/toxic_contaminants/pdf_zip/PAHs_Final.pdf (accessed November 20, 2014)
- Toxnet.nlm.nih.gov (accessed November 20, 2014)
- Valla, J.A., E. Mouriki, A.A. Lappas, and I.A. Vasalos. “The Effect of Heavy Aromatic Sulfur Compounds on Sulfur in Cracked Naphtha.” Catalysis Today 127 (2007): 92–8.
- Sunthankar, A.V. and B.D. Tilak. “A New Synthesis of Thiophenes and Thiapyrans - Part II. Monomethylthionaphthenes and Monochlorothionaphthenes.” Proceedings - Indian Academy of Sciences, Section A 32A (1950): 396–401.
- Kruber, O. and A. Ilaeithel. “Zur Kenntnis des Steinkohleteer-Anthracenols.” Chemische Berichte 86 (1953): 366–71.
- Bryson, A. “The Effects of Substituents on the pKa Values and N-H Stretching Frequencies of 1- and 2-Naphthylamines.” Journal of the American Chemical Society 82 (1960): 4862–71.
- ACD/LogP, Software Version 11.02, Advanced Chemistry Development, Inc., Toronto, ON, Canada, www.acdlabs.com, 2014.
- Lutskii, A.E. “Vodorodnaya Svyaz I Fizicheskie Svoistva 8-oksihkinolina.” Zhurnal Obshchei Khimii 24 (1954): 561–2; Chemical Abstracts 49:32411
- Ehrenhauser, F. “PAH and IUPAC Nomenclature.” Polycyclic Aromatic Compounds 35 (2015): 161-76.
- Stout, S.A., S.D. Emsbo-Mattingly, G.S. Douglas, A.D. Uhler, and K.J. McCarthy. “Beyond 16 Priority Pollutant PAHs: A Review of PACs used in Environmental Forensic Chemistry.” Polycyclic Aromatic Compounds 35 (2015): 285-315.
- Karcher, W. “Spectral Atlas of Polycyclic Aromatic Compounds, Vol. 2 Including Data on Physic-Chemical Properties, Occurrence and Biological Activity.” ed. W. Karcher (Dordrecht, the Netherlands: Kluwer Academic Publishers, 1988).
- Brack, W. and K. Schirmer. “Effect-Directed Identification of Oxygen and Sulfur Heterocycles as Major Polycyclic Aromatic Cytochrome P4501A-Inducers in a Contaminated Sediment.” Environmental Science & Technology 37 (2003): 3062–70.