Abstract
The aim of this study was to test the usefulness of polyurethane foam (PUF) passive air samplers as stationary, and, for the first time, as personal samplers for one week’s sampling period of polycyclic aromatic hydrocarbons (PAHs) in occupational air. Routine monitoring of workplace exposure is commonly performed with active sampling. However, active samplers can sometimes be unsuitable; e.g., it is difficult to make time-integrated measurements, longer than one day, and they can be noisy and obstructive. Indoor air quality on ships is an important aspect of the environment which has not been studied extensively on ships. For seafarers, good indoor air quality becomes particularly important as the ship represents both a working and living environment. In this study, measurements were carried out on two occasions on two different ships, at different workplaces, and for various personnel categories. On each ship, measurements were performed before and after a change of the type of fuel that the ships were operating on. We found a considerable wide range of PAHs exposure levels for the various workplaces and personnel categories on the ships. The stationary measurements, sum 32 PAHs, ranged from 33-39,000 ng m−3 and the personal exposure measurements ranged from 61-8,400 ng m−3. The results point to that the content of PAHs in the fuel can affect the indoor air environment on the entire ship. Further, the results demonstrate that the PUF sampler can serve as a simple and usable screening tool for estimating and tracking point sources of PAHs in micro-environments. Moreover, this study contributes to increased knowledge of exposure to and sources of PAHs for seafarers.
Introduction
Monitoring of polycyclic aromatic hydrocarbons (PAHs) in occupational environments is important given the potential adverse health effects associated with high exposure among workers. The US Environmental Protection Agency (US EPA) has identified a group of 16 PAHs as priority pollutantsCitation1 and some are classified by the International Agency for Research on Cancer (IARC) as being carcinogenic (benzo(a)pyrene), probably carcinogenic (dibenzo(a,h)anthracene), or possible carcinogenic (e.g., naphthalene and benzo(a)anthracene) in humans.Citation2 There are indications that compounds other than the known carcinogenic native PAHs, e.g., alkylated PAHs, contribute to PAHs toxicityCitation3 and thus should be included in PAHs exposure studies.
Measuring an individual’s exposure with personal sampling in the respiratory zone of the worker is critical for assessing risks, as exposure varies considerably with inter- and intra-individual variations. The best way to sample PAHs is with active sampling in the breathing zone of the worker.Citation4 With active sampling, both gas phase and particle phase can be collected appropriately. The ability to sample both phases is particularly important for PAHs measurements, since PAHs constitute a group of semi-volatile compounds of which some exists mainly in gaseous phase, whereas others are mainly particulates at ambient temperatures. Despite these advantages, the use of active sampling methods for routine monitoring (especially personal sampling) in workplaces is limited. The reason is that active methods are expensive (multiple concurrent measurements are therefore prevented), often inconvenient and obstructive for the workers to wear. Especially when it comes to time-integrated sampling for longer times, for example, several days or weeks, and in difficult work situations. Further, in potentially explosive areas, it may not be allowed to use battery-powered sampling pumps. Thus, in many cases they are either difficult or impossible to use.Citation4 This may be the reason why there are few studies on PAHs exposure in working environments.
Passive air samplers (PAS) using polyurethane foam (PUF) have proven to be good alternatives to active samplers for monitoring ambient and indoor environments.Citation5–11 The PUF sampler can also accumulate particles and is considered a promising technique for this cause. There are few studies that focus on the use of PUF-PAS for air sampling in work environments.Citation4,Citation12,Citation13
Indoor air quality on ships is an important aspect of the working environment which has not been studied extensively. For seafarers, good indoor environment becomes particularly important as the ship represents both a working and living environment. On long trips that last for weeks or even months there are limited opportunities to change the indoor environment, or to leave the ship. As a result, the personnel have little opportunity to avoid or influence exposure to substances that are present in the air in the ship’s work, living and recreational spaces. The air quality on a ship is characterized almost exclusively by the presence of substances that originate in the ship’s fuel, lubricants and engine exhaust. Although a number of studies from different countries have focused on health aspects for seafarers (e.g.,Citation14–18) few studies have focused on the air quality,Citation17 and, to the best of our knowledge, there is no study on the air quality with respect to PAHs on board.
The aim of the study presented in this paper is to evaluate the PUF-PAS sampler as a simple, silent and usable workplace screening tool, both stationary and personal, to yield time-integrated (3-7 days) air concentrations and tracking point sources of PAHs in micro-environments. Also, by using the sampler, to highlight sources, risky workplaces, and different working categories with elevated exposure levels of PAHs on ships.
Material and methods
Sampling campaigns
Details regarding the sampling campaigns; type of ship, fuel used, and measurements sites; stationary and personal, are provided in . Vessel 1 is an icebreaker that in wintertime provides assistance to other merchant vessels through ice in the Baltic Sea. The other ship, Vessel 2, is a cruise ship that mainly operates between Sweden and Finland in the Baltic Sea. During the first measurement campaign, the four main engines of Vessel 1 were operating on HFO (heavy fuel oil) and the auxiliary engines and boilers on marine gas oil (MGO). During the second campaign, the main and auxiliary engines were operating on MDO (Marine Diesel Oil). Vessel 2 was operating on HFO during the first campaign, and on a residual ultra-low Sulfur fuel oil (ULSFO) for the second campaign. The onboard stationary measurement sites were selected to represent both working and living places for the different personnel categories on board. The duration of the campaigns is presented in .
Table 1. Sampling campaign information and sampling parameters, stationary and personal measurements, on two Swedish vessels.
Air samplers for PAHs measurements
The polyurethane foam passive air sampler (PUF-PAS) used in this study is cylindrical in shape (PUF-cyl) (length: 10 cm, diameter: 2.2 cm) with a total surface area of 77 cm2 and density 0.030 g cm−3 (Klaus Ziemer GmbH, Germany). When used for sampling, the PUF-cyl is placed inside a protective cover net (diameter 2.2 cm, length 10 cm) with a mesh size of 1.0 mm (AB Derma, Sweden) (). The sampler for personal measurement is mounted onto a holder with a support plate that can be attached using safety pins to the person´s clothes close to the breathing zone (). The samplers for the stationary measurement sites were placed hanging at a height of approximately two meters above floors (). The aim was to place the sampler as close as possible to the person’s most frequent workplace or/and living area. Before use, each PUF-cyl is pre-cleaned via Soxhlet extraction using dichloromethane, dried in a vacuum desiccator, and stored in multiple layers of aluminum foil inside airtight Ziploc bags. Before use, and after the measurements prior to analysis, the samplers are stored in a freezer (−20 °C).
Figure 1. Total PAHs concentrations (ng m−3), including 16 US EPA PAHs and 16 alkylated PAHs, for respective measurement site, both stationary and personal measurements on two ship, and two measurement campaigns.
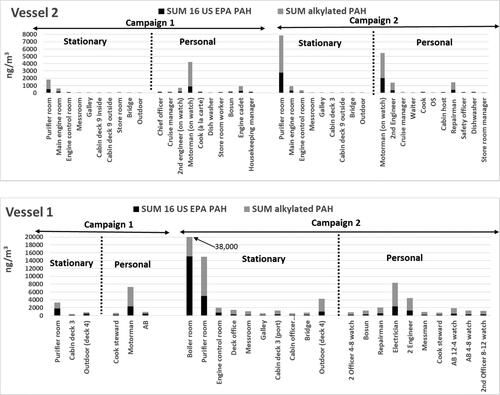
The published uptake-rates for the PUF-cyl sampler, two-week sampling time for workplaces, for 15 individual PAHs in gaseous- and particulate phases, respectively, were used for the calculations of concentration levels in this study ().Citation4 The alkylated species were calculated to closely related compounds of those published uptake-rates. The results are based on the assumption that the PAHs uptake-rates for PUF-PAS for one and two weeks are quite the same. However, there may be a greater uncertainty for naphthalene due to its volatility and short time to reach equilibrium.Citation19 All results show time-integrated mean concentrations over the sampling time.
Chemical analysis
The analytical procedures and instrumentation used in the PAHs analysis are described in detail elsewhereCitation4,Citation13,Citation20 and are given here in brief. Prior to extraction, a labeled internal standard mixture containing 16 deuterated U.S. EPA priority PAHs were spiked to the samples for determination of the recoveries of the quantified PAHs. The extraction equipment was Soxhlet using dichloromethane as solvent. The samples were purified using a combined column with on top 1 g sodium sulfate followed by 4 g activated silica and 2 g activated alumina. PAHs were separated and detected by means of high-resolution gas chromatography/low resolution mass spectrometry (HRGC/LRMS). The MS is an Agilent 5975 C connected to a 7890 A GC (Agilent Technologies, Inc., Santa Clara, Calif.) operating in electron impact (EI) mode, using selected ion monitoring (SIM). The GC column is a non-polar capillary column (60 m x 0.32 mm I.D. and 0.25 µm film thickness; J&W DB-5, Agilent). The concentration of 32 PAHs, including 16 US EPA priority PAHs and 16 alkylated species (), were determined.
Table 2. Range of air concentrations determined via passive polyurethane foam (PUF-cyl) sampler (ng m−3) of individual, sum PAHs, 16 US EPA PAHs, and alkylated PAHs on two occasions on two ship at different workplaces (stationary measurements) and on various job categories (personal measurements).
Quality assurance
Consistent recoveries (50–120%) were obtained for all internal standard compounds that were added to and used for correction of the samples. A somewhat lower recovery (ca 50%) was obtained for Naphthalene that can be attributed to the higher volatility for this compound and thus slightly higher losses in sample work-up.
Field and laboratory blanks were analyzed in parallel with the samples. Minor residues of some 2–4 ringed PAHs occurred, however the amount occurring was <15% of the amount present in the samples. All results were corrected for the blanks.
The limits of detection were calculated as three times the standard deviation of the values for the blanks or the background noise of these blanks. A certified reference material (SRM 1649a urban dust) was used as quality control (QC). The measured levels of 13 PAHs lie, for most part, within 30% of the certified levels. The PUF-cyl method has been thoroughly tested in the laboratory for the conformity of duplicate samples in both the working environment (including seafarers) and the general environment, including personalized sampling for sampling times between 1 h and 2 weeks.Citation4,Citation12,Citation13 Good precision is realized for all duplicates of both the gaseous (precision: <10%) and particle bound (precision: 10–30%) PAHs.
Results
Air concentrations
shows the ranges of the air concentrations (ng m−3) of 32 individual PAHs components, all PAHs, 16 US EPA PAHs and alkylated PAHs for both stationary and personal exposure measurements on both vessels, and the two measurement campaigns, respectively. Total PAHs, including 16 US EPA PAHs and alkylated PAHs, for respective measurement site are depicted in . The full data set, for all samples and compounds, are presented in Tables S2-S5.
Eight 2- and 3-ring gas-phase PAHs constituents accounted for 60-90% of the total PAHs in all samples. Methylated naphthalene’s (2-methylnaphthalene and 1-methylnaphthalene) were the most abundant PAHs followed by naphthalene, phenanthrene, fluorene and other alkylated species viz. 2,3-dimethylnaphthalene, 2,3,5-trimethylnaphthalene and 1-methylfluorene. The concentrations of the particle-bound PAHs were low and accounted for <1% of total PAHs. The proportion of alkylated PAHs was high in most samples. These species constituted ca 40-80% of the total number of PAHs ().
There was a big difference in levels between the various stationary and personal measurements on the two vessels and the two measurement campaigns. The highest measured stationary measurement site (sum PAHs) was the boiler room on Vessel 1 during the second campaign (MDO; 39,000 ng m−3). Measurements in the boiler room were performed only on this vessel and campaign. High PAHs levels were measured in the purifier rooms of all four measurement campaigns (1,800–15,000 ng m−3). The levels in the engine rooms were also higher (600–2,000 ng m−3) compared to other measurement sites for each measurement campaign. Other measurement sites for each vessel and campaign appear to reflect the background levels of PAHs for that vessel. A rough comparison between the vessels, showed that the background levels were higher, a factor of 3–4 times, on Vessel 1.
The highest air concentrations, total 16 US EPA PAHs, obtained in this work are 50–150 times higher than those reported for urban, rural and indoor air in SwedenCitation9,Citation21,Citation22 and in restaurant kitchens in Sweden.Citation23 However, our values are comparable to those reported for fire fighters and police forensic specialists,Citation13,Citation24 and an alloy factory in Sweden.Citation12 The background levels, both vessels, in this study were equal to, or somewhat higher than the cited urban, rural and indoor air measurement studies in Sweden.
Discussion
Concentrations and patterns of PAHs
There was a great variation in personal PAHs exposure between the different personnel categories on the vessels (). The highest levels of personal exposure in all four campaigns were found among the engine crew (4,200–7,300 ng m−3), with the highest individual value being an electrician working on Vessel 1 during the second campaign (8,400 ng m−3). Correspondingly, the highest stationary measurement levels were found in the engine room workplaces, especially the purifier room (15,000 ng m−3). Overall, the personal exposure levels for the seafarers well reflect the background levels measured in the respective workplaces onboard.
As seen in previous studies, the indoor air quality on both vessels were characterized almost exclusively by the presence of substances that originate in the ship’s fuel, lubricants and engine exhaust. Here, Vessel 1 changed from HFO to MDO, and Vessel 2 from HFO to ULSFO. When comparing the same stationary measurement sites from campaigns 1 and 2 on Vessel 1, the PAHs levels are higher for the second campaign. This may mean that the content of PAHs in the fuel can affect the environment on the entire ship, and thus that MDO would be a worse choice of ship fuel concerning the exposure levels of PAHs than HFO. For Vessel 2 there was a slightly higher PAHs content, in the purifier room during the second campaign (7,800 ng m−3) than the first (1,800 ng m−3). In comparison to other corresponding measurement sites between the two campaigns, including the engine room areas, there was a very little variation in PAHs content (engine areas 600–900 ng m−3; other areas 30-110 ng m−3). Thus, the difference in fuel, reduction of sulfur content has not affected the PAHs levels to some extent at different locations on the vessel.
The significance of the two fuels for the indoor air environment on the vessels was also made by comparing PAH patterns in the air against reported levels in the two fuels.Citation25 Ship fuels may contain notable levels of both native PAHs and alkylated PAHs. This empathizes maximizing the number of PAHs to be included in exposure studies on ships. The reported PAHs content in HFO and MDO fuels, respectively, contain ca 75% and 80% of alkylated species (Table S6). The proportion of alkylated PAHs at the different measurement sites during all four campaigns is between 40 and 80%. When comparing our results with studies in general and of the work environment, the alkylated PAHs fraction can typically be about 5–20%.Citation13,Citation21 Thus, the high proportion of alkylated PAHs in all samples also point to the significance of the fuels for the indoor environment on vessels.
Considerations and accuracy of PUF-cyl for estimating air concentrations
The uptake of compounds from an atmosphere may be influenced by several variables. In addition to samplers’ dependent variables, such as sampling medium, size and design of the sampler important variables may include concentration of target compounds as well as environmental parameters such as UV-light, the relative humidity, temperature and wind speed.
The samplers´ dependent variables can be especially important when used as personal sampler and this is important to consider in dirty work environments e.g., as it may be for certain occupational categories on ships. An open sampler design can then be particularly sensitive to contamination. We believe that the safety cover net used in this study () can prevent and reduce the risk from direct contact with contaminated surfaces for the PUF-PAS.
The level of target compounds in the air and interference from other chemical components can affect the uptake of substances.Citation26 Such an effect would, in this study, be important especially for the engine and purifier room spaces. In these spaces, where fuel and lubrication oils are handled, both volatile and semi-volatile organic substances may be elevated. Another factor that can affect uptake is the variation in the concentration gradient around the sampler, for example, if the sampler is exposed to a high level of the substances and then to a significantly lower level. Such an influence would probably affect the low molecular weight PAHs most, the intermediate very little and particulate PAHs probably negligible.
Thus, the influence of both interferences of other substances and large level differences may be the case for several of the occupational categories, which may have worked in engine room areas where the levels are significantly higher compared to other places. The significance of these parameters needs to be further tested.
Some PAHs components tend to be photodegraded by UV sunlight.Citation27 Depletion by UV sunlight is not a major concern in indoor measurements. However, even in indoor studies it is advisable to choose a sampling place in the shade, avoiding direct sunlight. In the outdoor sampling location, the samplers were well protected from both sunlight and wind.
Reportedly, wind speed constitutes an important parameter for the uptake of compounds in PUF-PAS.Citation28 Both high and low wind speeds may affect the uptake of compounds, but the influence of wind speed can be reduced by using a protective chamber (e.g., Citation11,Citation19). The open PUF-PAS design, used in this study, has previously been tested as a workplace sampler even outdoors.Citation13 The results of that study indicate a very little impact of wind speed. Since the seafarers, in our study, have mainly spent their time indoors and the negligible reported impact outdoors, we believe that the impact of the wind has been negligible for the results of this study.
Temperature may also affect the PUF-cyl uptake. Harner et al.,Citation29 suggested that the passive sampling medium-air partition coefficient will increase by a factor of 2.5 to 3 for every 10 °C decrease in temperature. Cao and HewittCitation30 proposed an increase of 20 °C will increase the diffusion coefficient by only ca 4%. Thus, the temperature could have affected the results somewhat for the purifier room (ca 30–40 °C) and the outdoor location for the November measurement on Vessel 2 during the first campaign (around 6 °C). This means that the air levels presented herein in the purifier rooms and the engine crew who worked there may be somewhat underestimated, and for the outdoor measurements in November, the result may be slightly overestimated. The influence of temperature for extreme workplaces such as boiler and purifier rooms in this study need to be further investigated with concurrent passive PUF-cyl and active (pumped) methods. Another aspect of the temperature is that it can have significant effect on gaseous-particle partitioning and therefore it is likely to affect volatility and thus can facilitate the release of gaseous PAHs from the fuels in the fuel management in the purifier room. This can create elevated levels of PAHs at these sites.
Achieving detectable and accurate values for benzo(a)pyrene is crucial for monitoring PAHs in workplaces, as the occupational exposure limit (OEL) is based on the time-weighted average concentration of this compound. Uptake-rates of particulate PAHs are contradictory in the literature. Some studies point to the same uptake for gaseous-phase as particulate PAHs (e.g., Citation4,Citation11–13), including the one we used to calculate air levels.Citation4 In contrast, several studies have shown that particle-bound fraction has a considerably lower uptake, which is ca 10% of that associated with the gaseous phase PAHs.Citation10,Citation19 A reason for such differences in results could be a consequence of different protective sampler container designs used in those studies.Citation31 We believe that the protective cover net, the open sampling design used in this study, would negligible affects the uptake of both gaseous and particulate PAHs. More PUF-PAS uptake-rate calibration studies with different contaminant levels and environmental conditions are needed to confirm this assumption.
Another factor that may affect uptake for particulate PAHs are differences in aerosol conditions (e.g., amount, size and type of particles) and in which particle fraction PAHs are associated. Most of the PAHs are associated with the finest particles (<1.5 µm), which are expected to diffuse in a similar manner to gaseous compounds.Citation32 It is difficult when measuring in an environment to predict the appearance and content of the aerosol. Therefore, when using PUF-PAS for particulate compounds we can, in the worst case, estimate the air concentrations within a factor of approximately five to ten. Thus, the sampler does not meet the standard quality criteria for determining exposure and assessing workplace risks. The sampler nevertheless shows that the particulate PAHs levels including benzo(a)pyrene at all measurement sites, in this study, are low and well below the OEL.
Naphthalene, the second PAHs substance with an OEL value, is important to include in exposure studies. It is difficult to sample this substance with PUF-PAS due to its volatility and short time to reach equilibrium or undergoing degradation in PUF-PAS.Citation11,Citation19 The sampling time of one week in this study is significantly shorter than the 2-3 weeks that this compound takes to reach equilibrium.Citation19 However, depending on its volatility, the results for this substance should be considered as crude estimate. Possibly, performance reference compounds (PRCs)Citation33 can be used and improve the ability to sample and quantitatively determine this substance as well.
Toxicity concern and health risks
In order to estimate the carcinogenic risks of PAHs mixtures to the seafarers, the equivalents of benzo(a)pyrene (B(a)Peq), with toxic equivalent factors (TEF) for benzo(a)pyrene set to 1, were evaluated by multiplying concentrations of each PAH with their TEF value.Citation34,Citation35 These authors report TEF for 16 US EPA PAHs compounds and they are ranked according to cancer potency relative to benzo(a)pyrene. Alkylated PAHs, to date, do not have TEFs but will likely be developed in the future. Thus, B(a)Peq calculations were only performed for parent PAHs. It is important to point out that alkylated PAHs are often more toxic than corresponding parent PAHsCitation3 and this can underestimate the overall cancer risk.
The European Community has suggested the B(a)Peq on PM10 at an annual standard in ambient air of 1 ng m−3.Citation36 This cancer potency value was exceeded at several of the stationary and personal measurement sites in this study. The highest measured B(a)Peq value in this study was in the boiler room on Vessel 1 during the second campaign (ca 30 ng m−3). Measurements in the boiler room were performed only on this vessel and campaign. High values were measured in the purifier rooms on both vessels during all four campaigns (ca 1.5–7 ng m−3). Noteworthy, the motorman on Vessel 1, campaign 1, has a value as high as 20 ng m−3. Other seafarers with elevated values are the motorman on the other three campaigns (ca 3 ng m−3), as well as the repairman (2.0 ng m−3) and the electrician (ca 4 ng m−3) during the first campaign on Vessel 2. It is reasonable to believe that the high cancer potency values for some of the occupational categories may be due to the engine personnel working in the high-level workplaces in the engine rooms during the measurement campaigns. The annual standard B(a)Peq values (1 ng m−3) have been exceeded in urban sites all over the world e.g., Athens, Greece (1.6 ng m−3)Citation37 Zabrze, Poland (7.94 ng m−3),Citation38 Mexico City, Mexico (2.17 ng m−3) and Shanghai, China.Citation39 Thus, the results of our study are similar to, and in some cases significantly higher than, the studies cited. B(a)Peq calculations for different workplaces and occupational categories are sparse. An exposure study on chefs to PAHs showed B(a)Peq values of about 0.6 ng m−3.Citation20 Contribution of the cancerogenic potency of benzo(a)pyrene alone of the PAHs mixture is commonly >50% (e.g.,Citation20,Citation40) In our study, it looks different because the levels of the particulate PAHs fraction in the study were for most measurement sites low (<1%) compared to gas phase PAHs, and, thus, in most cases, increasing the significance for the gaseous phase fraction of PAHs of the cancerogenic potency. For some of the measurement sites, gas phase PAHs (mainly naphthalene and phenanthrene) could account for over 80% of the total cancer risk. In addition, the intermediate PAHs, fluoranthene, with a significant portion also in the gaseous phase accounted for between 20 and 60% of the total cancer activity. Benzo(a)pyrene had an important role in some of the places, e.g., the outdoor location on Vessel 1, campaign 1 (47%) and for the personal measurement on the captain of the cruise ship (Vessel 2, campaign 1) (54%). For most of the samples benzo(a)pyrene constituted less than 20% of the cancerogenic activity. These results underline the importance of including both particulate and gas phase PAHs when evaluating risk to human health. As mentioned earlier, alkylated PAHs are often more pervasive and toxic than their parent PAHs and these compounds also contribute to the cancerogenic potency risk. Since these accounted for 30-70% of the total PAHs content, the cancer risk evaluation done in this study could have significantly underestimated the real overall health risk. Although there is uncertainty with PUF-PAS in quantitatively determining particle bound PAHs that have the greatest cancer risk, the study clearly shows that there are workplaces and occupational categories on vessels where the PAHs exposure may pose adverse health risks.
In summary, the PUF-cyl passive sampler is a simple and usable screening tool for estimating air concentrations and tracking point sources of PAHs in micro-environments. The sampler is easy to use even as a personal sampler for several days and can easily be handled by the subject themselves. The uptake of compounds from an atmosphere may be influenced by several variables. Also, depending on uncertainty in sampling of particulate PAHs, that have the largest cancerogenic potency, the passive sampler does not meet the standard quality criteria required for exposure assessment in occupational settings. The influence of uptake due to temperature and other differences between sites with different PAHs levels need to be further investigated. Nevertheless, the study has shown major differences in PAHs burdens between places and difference in exposure to different occupational categories, and pointed out the risks to various workplaces and seafarer categories on ships.
Supplemental Material
Download Zip (1.3 MB)Acknowledgements
We would like to express our sincere gratitude to the shipping companies for giving us access to the ships and to the crew members who generously agreed to carry the samplers and assisted with the measurements during our stay on-board.
Additional information
Funding
References
- USEPA. Guidelines for carcinogen risk assessment, EPA/630/P-03/001F, 2005, http://www.epa.gov/raf/publications/pdfs/CANCER%20GUIDELINES%20FINAL%203-25-05.pdf. U.S Environmental Protection Agency.
- IARC, International Agency for Research on Cancer, IARC Monographs on the Evaluation of Carcinogenic Risks to Humans (Lyon, France: IARC, WHO, 2006), Vol 92.
- J. G. M. Van Rooij, and F. J. Jongeneelen, “Review of Skin Permeation Hazard of Bitumen Fumes,” Journal of Occupational and Environmental Hygiene 4, no. sup1 (2007): 237–44.
- P. Bohlin, K. C. Jones, J. O. Levin, R. Lindahl, and B. Strandberg, “Field Evaluation of a Personal Passive Air Sampler for PAH Exposure in Workplaces,” Journal of Environmental Monitoring 12, no. 7 (2010): 1437–44.
- M. Shoeib and T. Harner, “Characterization and Comparison of Three Passive Air Samplers for Persistent Organic Pollutants,” Environmental Science & Technology 36, no. 19 (2002): 4142–51.
- F. Jaward, N. Farrar, T. Harner, A. Sweetman, and K. C. Jones, “Passive Air Sampling of Polycyclic Aromatic Hydrocarbons and Polychlorinated Naphthalenes across Europe,” Environmental Toxicology and Chemistry 23, no. 6 (2004): 1355–64.
- A. Motelay-Massei, T. Harner, M. Shoeib, M. Diamond, G. Stern, and B. Rosenberg, “Using Passive Air Samplers to Assess Urban-Rural Trends for Persistent Organic Pollutants and Polycyclic Aromatic Hydrocarbons. 2. Seasonal Trends for PAHs, PCBs and Organochlorine Pesticides,” Environmental Science & Technology 39, no. 15 (2005): 5763–73.
- E. C. Santiago, and M. G. Cayetano, “Polycyclic Aromatic Hydrocarbons in Ambient Air in the Philippines Derived from Passive Sampler with Polyurethane Foam Disk,” Atmospheric Environment 41, no. 19 (2007): 4138–47.
- P. Bohlin, K. C. Jones, H. Tovalin, and B. Strandberg, “Observations on Persistent Organic Pollutants in Indoor and Outdoor Air Using Passive Polyurethane Foam Samplers,” Atmospheric Environment 42, no. 31 (2008): 7234–41.
- K. Klanova, P. Eupr, J. Kohoutek, and T. Harner, “Assessing the Influence of Meteorological Parameters on the Performance of Polyurethane Foam-Based Passive Air Samplers,” Environmental Science & Technology 42 (2008): 550–5.
- T. Harner, K. Su, S. Genualdi, J. Karpowicz, L. Ahrens, C. Mihele, J. Schuster, J.- P. Charland, and J. Narayan, “Calibration and Application of PUF Disk Passive Samplers for Tracking Polycyclic Aromatic Compounds (PACs),” Atmospheric Environment 75 (2013): 123–8.
- P. Bohlin, K. C. Jones, and B. Strandberg, “ Field Evaluation of Polyurethane Foam Passive Air Samplers to Assess Airborne PAHs in Occupational Environments,” Environmental Science & Technology 44, no. 2 (2010): 749–54.
- B. Strandberg, A. Julander, M. Lewné, M. Sjöström, H. Koca. Akdeva, and C. Bigert, “Evaluation of Polyurethane Foam Passive Air Sampler (PUF) as a Tool for Occupational PAH Measurements,” Chemosphere 190 (2018): 35–42.
- T. Hemmingsson, I. Lundberg, R. Nilsson, and P. Allebeck, “Health-Related Selection to Seafaring Occupations and Its Effects on Morbidity and Mortality,” American Journal of Industrial Medicine 31, no. 5 (1997): 662–8.
- L. Kaerlev, H. L. Hansen, and P. S. Nielsen, “Cancer Incidence among Danish Seafarers: A Population Based Cohort Study,” Occupational and Environmental Medicine 62, no. 11 (2005): 761–5.
- K. Forsell, S. Hageberg, and R. Nilsson, “Lung Cancer and Mesothelioma among Engine Room Crew—Case Reports with Risk Assessment of Previous and Ongoing Exposure to Carcinogens,” International Maritime Health 58, no. 1–4 (2007): 5–13.
- M. Oldenburg, X. Baur, and C. Schlaich, “Occupational Risks and Challenges of Seafaring,” Journal of Occupational Health 52, no. 5 (2010): 249–56.
- K. Forsell, H. Eriksson, B. Järvholm, M. Lundh, E. Andersson, and R. Nilsson, “Work Environment and Safety Climate in the Swedish Merchant Fleet,” International Archives of Occupational and Environmental Health 90, no. 2 (2017): 161–8.
- P. Bohlin, O. Audy, L. Škrdlíková, P. Kukučka, P. Přibylová, R. Prokeš, Š. Vojta, and J. Klánová, “Outdoor Passive Monitoring of Semi Volatile Organic Compounds (SVOCs): a Critical Evaluation of Performance and Limitations of Polyurethane Foam (PUF) Disks,” Environmental Science Processes & Impact 16, no. 3 (2014): 433–44.
- R. Bramming Jorgensen, B. Strandberg, A. K. Sjaastad, A. Johansen, and K. Svendsen, “Simulated Restaurant Cook Exposure to Emissions of PAHs, Mutagenic Aldehydes, and Particles from Frying Bacon,” Journal of Occupational and Environmental Hygiene 10 (2013): 122–31.
- B. Strandberg, P. Gustafson, H. Söderström, L. Barregard, P.-A. Bergqvist, and G. Sällsten, “The Use of Semipermeable Membrane Devices as Passive Samplers to Determine Persistent Organic Compounds in Indoor Air,” Journal of Environmental Monitoring 8, no. 2 (2006): 257–62.
- J. Klingberg, M. Broberg, B. Strandberg, P. Thorsson, and H. Pleijel, “Urban Vegetation as a Mitigator of Air Pollution and Noise Exposure - a Case Study in Gothenburg, Sweden,” Science of the Total Environment 599–600 (2017): 1728–39.
- M. Lewné, S. Johannesson, B. Strandberg, and C. Bigert, “Exposure to Particles, Polycyclic Aromatic Hydrocarbons and Nitrogen Dioxide in Swedish Restaurant Kitchen Workers,” Annals of Occupational Hygiene 61 (2017): 152–63.
- M. Sjöström, A. Julander, B. Strandberg, M. Lewné, and C. Bigert, “Airborne and Dermal Exposure to Polycyclic Aromatic Hydrocarbons, Volatile Organic Compounds, and Particles among Firefighters and Police Investigators,” Annals of Work Exposures and Health 63, no. 5 (2019): 533–45.
- SINTEF report. “Analysis of PAH and alkylated PAH in oils”. . SINTEF Ocean AS. Environmental Technology, Laboratory Services, Trondheim, Norway, 2017.
- B. Strandberg, K. Bergemalm-Rynell, and G. Sallsten, “Evaluation of Three Types of Passive Samplers for Measuring 1,3-Butadiene and Benzene at Workplaces,” Environmental Science: Processes & Impacts 16, no. 5 (2014): 1008–14.
- E. Wild, J. Dent, T. O. Gareth, and K. C. Jones, “Real-Time Visualization and Quantification of PAH Photodegradation on and within Plant Leaves,” Environmental Science & Technology 39, no. 1 (2005): 268–73.
- L. Tuduri, T. Harner, and H. Hung, “Polyurethane Foam (PUF) Disks Passive Air Samplers: Wind Effect on Sampling Rates,” Environmental Pollution 144, no. 2 (2006): 377–83.
- T. Harner, N. J. Farrar, M. Shoeib, K. C. Jones, and F. A. Gobas, “Characterization of Polymer-Coated Glass as a Passive Air Sampler for Persistent Organic Pollutants,” Environmental Science & Technology 37, no. 11 (2003): 2486–93.
- X. L. Cao, and C. N. Hewitt, “Application of Passive Samplers to the Monitoring of Low Concentration Organic Vapors in Indoor and Ambient Air – a Review,” Environmental Technology 12, no. 11 (1991): 1055–62.
- M. Z. Markovic, S. Prokop, R. M. Staebler, J. Liggio, and T. Harner, “Evaluation of the Particle Infiltration Efficiency of Three Passive Samplers and the PS-1 Active Air Sampler,” Atmospheric Environment 112 (2015): 289–93.
- L. Melymuk, P. Bohlin, O. Sáňka, K. Pozo, and J. Klánová, “Current Challenges in Air Sampling of Semivolatile Organic Contaminants: Sampling Artifacts and Their Influence on Data Comparability,” Environmental Science & Technology 48, no. 24 (2014): 14077–91.
- C. Moeckel, T. Harner, L. Nizzetto, B. Strandberg, A. Lindroth, and K. C. Jones, “Use of Depuration Compounds in Passive Air Samplers: Results from Active Sampling-Supported Field Deployment, Potential Uses, and Recommendations,” Environmental Science & Technology 43, no. 9 (2009): 3227–32.
- I. T. K. Nisbet, and P. K. LaGoy, “Toxic Equivalency Factors (TEFs) for Polycyclic Aromatic Hydrocarbons (PAHs),” Regulatory Toxicology and Pharmacology 16, no. 3 (1992): 290–300.
- C. E. Bostrom, P. Gerde, A. Hanberg, B. Jemstrom, C. Johansson, T. Kyrklund, A. Rannug, M. Tornqvist, K. Victorin, and R. Westerholm, “Cancer Risk Assessment, Indicators, and Guidelines for Polycyclic Aromatic Hydrocarbons in the Ambient Air,” Environmental Health Perspectives 110 (2002): 451–88.
- For Nickel, Cadmium, Mercury, and PAHs in Ambient Air; European Union (EU) Directive 2004/107/CE; EU: Brussels, Belgium, 2004.
- F. Marino, A. Cecinato, and P. A. Siskos, “Nitro-PAH in Ambient Particulate Matter in the Atmosphere of Athens,” Chemosphere 40, no. 5 (2000): 533–7.
- K. Cwiklak, J. S. Pastuszka, and R. Rogula-Kozlowska, “Influence of Traffic on Particulate-Matter Polycyclic Aromatic Hydrocarbons in Urban Atmosphere of Zabrze, Poland,” Polish Journal of Environmental Studies 18 (2009): 579–85.
- J. Cheng, T. Yuan, Q. Wu, W. C. Zhao, H. Y. Xie, Y. Ma, J. Ma, and W. H. Wang, “PM10-Bound Polycyclic Aromatic Hydrocarbons (PAHs) and Cancer Risk Estimation in the Atmosphere Surrounding an Industrial Area of Shanghai, China,” Water, Air, and Soil Pollution 183, no. 1–4 (2007): 437–46.
- V. Mugica, S. Hernandez, M. Torres, and R. Garcia, “Seasonal Variation of Polycyclic Aromatic Hydrocarbon Exposure Levels in Mexico City,” Journal of the Air and Waste Management Association 60, no. 5 (2010): 548–55.