Abstract
Previous ecological research on social dynamics has largely been conducted on human dyads; however, to provide support for Hume's touchstone, ecological psychologists must also investigate the perception-action and collective action capabilities of non-humans. This approach allows us to circumvent possible cognitivist interpretations of complex social phenomena by exploring social dynamics across organisms with a range of abilities. Here, we outline how the integration of ecology and ecological psychology will benefit ecological theory (i.e., by informing large scale human collective coordination) and the way we engineer collaborative human groups. To demonstrate the benefits of this avenue of research, we detail the well-studied communication system of the western honey bee, Apis mellifera, and highlight the context-sensitivity and specificity of their communicative signals. We discuss two collective social phenomena in bees—swarming and task allocation—and how their study can inform human interpersonal dynamics. In order to use insects as a basis for improving human group coordination, we suggest that we must not only identify a suitable insect but also a particular phenomenon that shares the same values along critical dimensions of variability. We end by providing potential real-world implications of this disciplinary cross-pollination.
Social insects are well known for their miraculous creations: Macrotermes michaelseni, a species of termite, build closed-chimney mound structures up to three meters in height that perform gas exchange (Turner, Citation2011), honey bees create thousands of seemingly perfect hexagonal cells for the storage of food and larvae (Camazine, Citation1991; Camazine et al., Citation1990), and fire ants coordinate and link together to form hydrophobic rafts during times of flooding (Mlot et al., Citation2011). All of these macro-level emergent phenomena are the result of individual dynamic interactions and self-organization of group members. The environmental alterations resulting from these events further constrain and guide micro-macro interactions. This complex perception-action cycle succeeds not by some explicit plan or top-down governance but by social coordination and task dynamics of individual members engaged in a shared physical environment (). As such, social insects have much to teach the fields of ecology and ecological psychology alike.
Figure 1. A visual guide of complex adaptive behavior as the result of individual self-organized relationships, interactions with a shared environment, and mutual constraints. Reprinted with permission under a CC 3.0 BY-SA license (Megan Chiovaro and Alexandra Paxton, https://osf.io/zqrf2/).
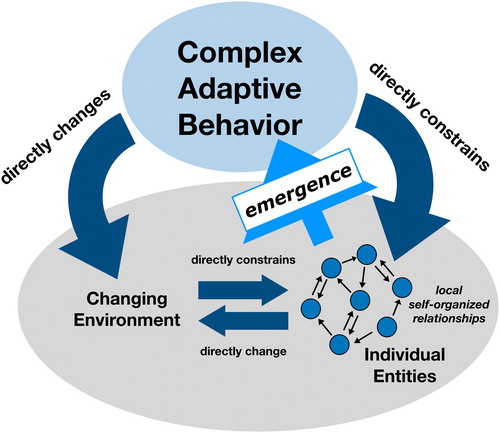
One of the most vital and commonly studied of all social insects is the western honey bee (Apis mellifera). Often mistaken by the public for wasps, these flower-feeding invertebrates are members of the Apoidea family (Rajasekhar et al., Citation2017) and are generally very docile. As social insects, they engage in continuous dynamic interactions with one another and their environment and are capable of translating information vital for the colony’s survival to other bees through their repertoire of communicative behaviors. With the ability to fly over 6 kilometers in a single trip (Visscher & Seeley, Citation1982), they are responsible for a significant portion of plant pollination: Over 75% of the human food supply is attributable to insect pollination, largely thanks to honey bees (Klein et al., Citation2007).
Contributing to the historical work in the fields of ecology and entomology, previous psychological research on honey bees has largely focused on visual perception, exploring hypotheses on pattern recognition, problem solving, object orientation, optic flow, and operant conditioning (e.g., Avarguès-Weber et al., Citation2012; Lehrer, Citation1994). Fundamental questions regarding honey bees’ perception-action capabilities, use of communicative information, methods of collective coordination, and task dynamics—topics well suited for an ecological approach—have been left unanswered.
Ecologists and ethologists have long investigated the use of information by local agents in social collectives and the subsequent emergence of self-organization (i.e., global patterns resulting from local rules in the absence of a central executive; Nicolis & Prigogine, Citation1977; Camazine et al., Citation2003; Sumpter, Citation2006). Many have even begun to draw the bridge between non-human collective action and psychological phenomena. For example, Couzin (Citation2009) highlighted that schools of fish and flocks of birds share many decision-making principles with that of a neural network, noting similarities like decentralized, local interactions determining global structure, multiple stable states existing for any set of local interactions, speed-accuracy tradeoffs, and amplification and damping of information. In fish schools, change of direction occurs without any kind of signaling, any awareness of which other individuals have knowledge, or any indication about whether their viewpoint is within the majority or minority, yet schools can make consensus decisions (Couzin et al., Citation2005). Similarly, foraging behavior of insects (e.g., ants, bees) has been likened to cognitive search processes (Hills et al., Citation2015). Even mammals have been found to act collectively by following simple rules without top-down governance (e.g., herds of buffalo; Sinclair, Citation1977).
These phenomena are considered examples of collective intelligence or swarm intelligence (SI), a rapidly growing area of research. Krause et al. (Citation2010) proposed a definition of SI to unite phenomena exhibited by all organisms:
two or more individuals independently, or at least partially independently, acquire information and these different packages of information are combined and processed through social interaction, which provides a solution to a cognitive problem in a way that cannot be implemented by isolated individuals. (p. 29)
Under this definition, both humans and social insects can be directly compared, and the work of ecologists studying collective behavior and task dynamics can be used as a model for enhancing human social behavior. The utilization of SI principles has gained popularity for its widespread applicability, even in applied fields like market predictions, crowd control, and company management (Krause et al., Citation2010).
Building on previous insect work from ecology and psychology, researchers today have begun to exploit social insect collective dynamics for the benefit of human systems, arguing that simple behavioral rules followed by individuals, relative states of individuals, alterations to the surrounding environment, and exchange, amplification, and decay of information all intermesh to guide and constrain collective behavior (e.g., Sumpter, Citation2006; Couzin, Citation2009). Some have begun to use honey bee social dynamics specifically (e.g., with applications to engineering; Rajasekhar et al., Citation2017). Moving into the realm of self-organization and dynamical systems theories has thus proven fruitful, but the benefits of jointly studying humans and social insects, particularly those for human factors engineering, have not yet been fully realized.
Here, we consider honey bees—as individuals and collectives—from the perspective of ecological psychology (a field dedicated to the discovery of lawful animal-environment relationships; cf. Gibson, Citation1979). Specifically, we propose that honey bees’ communicative behaviors and social structure can inform the use of strategic constraints on human collective dynamics and organizational systems to increase efficiency. Although honey bees have piqued the interest of many ecological psychologists and are used as case study examples for ecological concepts, seldom has ecology directly met ecological psychology in the literature of either field (but see Srinivasan, Citation1998). No one—to our knowledge—has yet explored the implications of honey bee collectives for human factors engineering from an ecological perspective.
Transitioning to an explicit focus on honey bee collectives would make for an important and timely addition not only to understanding these important social invertebrates in their proper context but also to our understanding of and ability to influence human behavior. Recent debate on the existence of a collective intelligence factor among human groups (e.g., see criticism by Credé et al., Citation2017, and response by Woolley et al., Citation2018)—specifically about the extent, variability, and limitations of collective intelligence—has left the study of large human group coordination of action wide open. This sparks multiple questions: What is it about the social dynamics of honey bee colonies that makes them so consistently successful in their group tasks, while human groups are so variable? Why might some applications of insect phenomena to human group coordination be successful while others fail?
Despite dramatic differences in the scope and scale of the collaborative dynamics of these two types of organisms, we believe that studying honey bee communicative behaviors and task dynamics may provide insights that can be applied to boost the success of human collectives. We propose that—in order to use insects as a basis for improving human group coordination—we must identify both a suitable insect and a particular phenomenon which shares the same values along critical dimensions of variability (adapted from Goldstone & Gureckis, Citation2009). In order to do so, we consider the honey bee and begin with a discussion of its context-sensitive communicative repertoire. We then detail several honey bee collective phenomena and outline questions where ecological psychology may prove insightful. Finally, we end with a discussion of the real-world implications of this endeavor and outline a framework for consistently applying insect task dynamics to human groups to enhance collective success.
Context-sensitive communication
While humans have a broad range of communicative behaviors available to coordinate their actions with one another, honey bees employ a more limited but nevertheless impressive set of communicative signals to indicate locations of resources, advertise new nests-sites, and call for redistribution of the workforce. Here, we outline the most studied signals and their purposes for colony regulation. From an ecological perspective, these signals appear to be a context-sensitive form of communication that still exhibits specificity in the information they each convey, a tenet of perception-action for which ecological psychology has advocated since its inception.
The waggle dance
Honey bees are well known in the psychological community for their unique waggle dances, first discovered by Karl von Frisch (von Frisch, Citation1967). Pollen- and nectar-foraging worker bees use this dance to communicate the location of profitable food sources with high sucrose concentration (Raveret-Richter & Waddington, Citation1993; Seeley, Citation1995; von Frisch, Citation1965; Waddington, Citation1997). Upon returning to the hive from such a source, foragers move onto the “dance floor” area of the comb and begin rapidly shaking their abdomen, creating vibrations that carry across the comb. They will proceed to make “waggle runs” in a straight trajectory, followed by an alternating semi-circle shape, returning to their original location and completing a figure-eight pattern (). Dancing foragers will make return trips to their profitable location, interspersed with periods of dancing. Cessation of the dance occurs when a source is no longer profitable (Seeley, Citation1995).
Figure 2. A graphical depiction of the honey bee waggle dance. From a top-down view, the difference in angle between the location of the food source and the azimuth of the sun relative to the location of the hive is denoted alpha [a]. This information is used by forager bees, determining the angle of their waggle dances in the hive relative to the direction of gravity. Reprinted with permission under a CC 3.0 BY-SA license (Megan Chiovaro and Alexandra Paxton, https://osf.io/s7zau/).
![Figure 2. A graphical depiction of the honey bee waggle dance. From a top-down view, the difference in angle between the location of the food source and the azimuth of the sun relative to the location of the hive is denoted alpha [a]. This information is used by forager bees, determining the angle of their waggle dances in the hive relative to the direction of gravity. Reprinted with permission under a CC 3.0 BY-SA license (Megan Chiovaro and Alexandra Paxton, https://osf.io/s7zau/).](/cms/asset/243dff8b-2f15-47b7-90f1-db838656341a/heco_a_1836966_f0002_c.jpg)
The structure of the dance directly conveys information about the food source. The angle of the waggle run with respect to the direction of gravity corresponds to the angle (alpha [a] in ) at which the food source is located relative to the azimuth of the sun, and the run duration conveys its distance from the hive as determined by a visual odometer (Dacke & Srinivasan, Citation2007; Esch & Burns, Citation1996; Gould & Gould, Citation1988; Seeley, Citation1995; von Frisch, Citation1967). With no reference to the sun inside the dark hive, the dancers will adjust their waggle run angle over time using their circadian cycle, known as Zeitgedächtnis (Beier, Citation1968; Beier & Lindauer, Citation1970; Bennett & Renner, Citation1963; Renner, Citation1955, Citation1957). Profitability of a source is also conveyed through the dance by the number of waggle runs per foraging trip, often referred to as the “liveliness” of the dance (Seeley et al., Citation2000). Information from the dance is perceived fairly well by onlooker bees, only requiring observations of 5–6 runs (Esch & Bastian, Citation1970; Mautz, Citation1971).
This systematic, reliable method of communication has helped honey bees flourish, particularly in areas where food sources are clustered or scarce (Dornhaus & Chittka, Citation2004; Primack, Citation1985; Schneider, Citation1989; Sherman & Visscher, Citation2002). However, as compelling as this social signal may seem, the rate of acceptance of directional information is shockingly low: While only 12–25% of onlooker bees will take new navigation attempts using information from witnessed dances, 75–88% of onlooker bees—typically comprising experienced foragers—will be “re-engaged” (i.e., observe relatively few waggle runs and then return to a previously visited source, rather than the source indicated by the dance; Biesmeijer & Seeley, Citation2005). To explain this phenomenon, some researchers have suggested that merely witnessing a dance motivates bees to continue foraging (Grüter et al., Citation2008). Other research has indicated that the scent of the dancing bee leads experienced foragers to return to a previously visited site of the same flower species (Biesmeijer & Seeley, Citation2005; Grüter et al., Citation2008; Wenner et al., Citation1969). Simply encountering a common foraging scent without witnessing a dance also increases the proportion of bees leaving the hive to forage (Grüter et al., Citation2008; Thom et al., Citation2007). Conceptually, this shortfall of information utilization is similar to that seen in humans. Functional fixedness is the inability of individuals to recognize multiple functions of a given object (Duncker, Citation1945; German & Defeyter, Citation2000)—or, in other words, to perceive the full affordances of an object. It may be that the expert foragers have a sort of functional fixedness in their observation of these dances, with the scent of the source on the dancer providing a greater pull than the directional information provided by the dancer.
The divergence in behavior between novice foragers and experienced foragers has left a critical question: At what point is a bee an “experienced” forager, in that they will no longer use the dance information to the fullest? Currently, the apparent disregard for detailed information by experienced foragers is poorly understood. However, an ecological approach to foraging behavior could help uncover the relationship between an individual organism’s history, environmental information, and communication, as well as other factors underlying the acceptance and use of the information. If the tradeoff between directional and olfactory information is determined to be a function of age, beekeepers might benefit from strategic flower-planting. For example, established hives would thrive in fields where different species of flowers were planted in distinct locations. This would help minimize the ambiguity of locations, leading older bees to reliably visit intended locations even when guided entirely by scent. The association between scent and location could be conceptualized as an invariant, a structured relationship that guides behavior. By altering the environment in this way, beekeepers could help realize invariant relationships between scent and location and thus increase the productivity of their older foragers. Some may argue that strategic planting is a lost effort, for honey bees will fly kilometers away from the hive to forage. Though this is true, a profitable flower patch nearby will attract more foragers given its inherent decreased energy requirements. Additionally, flowers of the same species share the same flowering periods; thus, even if a waggle dance indicates a farther location, the closer scent-familiar patch will likely be similar in profitability and frequented by older foragers, leading to decreased foraging time by making initial scent-based trips successful.
The tremble dance
In addition to the waggle dance, honey bees use several other signals to convey information. The tremble dance (von Frisch, Citation1923) involves the same rapid shaking used in the waggle dance but is instead followed by angular readjustments (of approximately 50 degrees; Seeley, Citation1992) rather than rotations. It encourages in-hive foraging tasks, such as nectar and pollen storage. If a forager bee returns to the hive and waits a “long” period of time before unloading her collection to an in-hive worker, she will climb between her worker sisters while performing this dance to communicate the need for more “receiver” bees (Biesmeijer, Citation2003; Kirchner, Citation1993; Seeley, Citation1992). Most foragers who wait 50 seconds or more to unload their collection will perform the tremble dance, while foragers who wait 20 seconds or less are most likely to perform waggle dances (Seeley, Citation1992).
Ecological psychology’s focus on identifying grounded, organism-environment variables could help—shifting focus to identify one or more variables (e.g., the amount of pollen/nectar load, quality of pollen/nectar load) that shape how long a forager bee waits, rather than absolute time spent waiting. For example, Richardson, Marsh, & Baron. (Citation2007) investigated the transition from solo to joint action in a plank-moving task. The shift in approach was explained by an action-scaled ratio (i.e., the average plank length at which they switched to joint action divided by arm span). In this case, it was not the absolute length of the plank that determined their actions but rather the relationship to the situation and the state of the environment. The change in behavior of a forager bee from patience to utilization of the tremble dance could similarly be due to more complex, scaled relationships (e.g., a combination of wait time, foraging urgency, pollen load weight, current foraging conditions, time of day), rather than absolute waiting time.
The stop signal
The stop signal is another method of communication but an inhibitory one; it is used to reduce foraging activities, such as waggle dance frequency (Kirchner, Citation1993; Nieh, Citation1993; Pastor & Seeley, Citation2005). Forager bees will approach other foragers performing waggle dances advertising sites of lesser profitability and head-butt them while making a high-pitched chirping noise. Receiving one of these signals typically results in the targeted forager ceasing their dance and leaving the dance floor (Nieh, Citation1993). They are primarily used to target same-site foragers when a source has become dangerous to visit, which is why this signal is also known as a “danger signal” (Nieh, Citation2010). Stop signals may also be used to slow the rate of recruitment during times of highly profitable foraging, allowing the various jobs in the workforce to balance and reestablish smooth transfer of labor (Nieh, Citation1993). Given that the signal is displayed by tremble dancers, dance followers, and waggle dancers and is targeted at bees undertaking those same roles as well as food exchange, the meaning of this signal is highly context-dependent. The signal specifies different potential actions depending on the role of the signaler and that of the listener.
The shaking signal
Perhaps most intriguing of the excitatory signals is the shaking signal, also referred to as the vibration dance (e.g., Schneider et al., Citation1986a, Citation1986b), vibration signal (e.g., Lewis et al., Citation2002), and jerking dance (von Frisch, Citation1967). To produce a shaking signal, a worker bee will grasp another worker with her front legs and shake her own body dorsoventrally at approximately 16 Hz for about 1–2 seconds (Biesmeijer, Citation2003). Some work has suggested that the meaning of this signal is context-dependent (Biesmeijer, Citation2003; Hyland et al., Citation2007; Schneider et al., Citation2001; Schneider & Lewis, Citation2004). It can be used, as in the tremble dance, to promote an increase in the number of receiver bees, but it can also be used to promote an increase in the number of forager bees after a period of unprofitable foraging (Seeley et al., Citation2010). Exposure to these dances also leads to increases in non-foraging related tasks, including comb building and repairing, honey processing, and brood care (Schneider, Citation2010; Schneider & McNally, Citation1991). Worker honey bees who are inactive (i.e., not currently engaged in a task) tend to be more frequent targets of the dances (Lewis et al., Citation2002), and this individualistic targeting does not exclude inactive virgin and laying queen bees (Fletcher, Citation1978; Schneider, Citation1990).
Thus the meaning of the signal is dependent not only on the context of the work environment (i.e., what jobs are in need of more workers) but also on the particular targeted individual’s role (i.e., a queen vs. a worker bee) and history (e.g., encouraging mating flights for virgin queens, prompting more consistent egg-laying for laying queens, leading younger workers to begin caring for brood or tending the queen, urging older workers to leave the hive to forage). In an account of human ecological linguistics, Van Lier (Citation2002) suggested that “[l]anguage does not arise from input that is processed, but from affordances that are brought forth by active engagement, and which enable further action and interaction” (p. 146, emphasis in original). In a similar vein, the meaning of the shaking signal is not derived purely from the stimulus input to surrounding workers; rather, the meaning of the shaking signal arises from the specific interaction, in which the specific receiving bee has a specific set of capabilities and a specific history that, together, determine the opportunities for action that arise from the shaking signal. To a young worker, the signal may mean they must resume brood tending duties, but to a forager, it could mean there is a need for more pollen collectors. Just as how a word obtains its meaning through an interaction, the meaning of the signal is determined through the interaction between two specific bees. The intentional, embedded nature of the shaking signal can be conceptualized as an interaction affordance, an opportunity for action that is only possible given interaction with another individual (Worgan & Moore, Citation2010). Here, the speaker (or dancer) is an intentional participant in the interaction who “exploits his or her knowledge of the listener and the wider context to fulfill those intentions, manipulating the set of interaction affordances and adjusting his or her signal to this end” (Worgan & Moore, Citation2010, p. 333). In humans and honey bees, the abilities of the listener directly constrain the meaning of information exchanged during an interaction.
Interim discussion
While previous research has carefully identified various characteristics of honey bees’ excitatory and inhibitory communicative behaviors, further investigation is necessary to gain a full understanding of the relationships between workers and their communication methods. Grüter and Farina (Citation2009) posed interesting questions left largely unexplored: How can we explain the variation of dance-following behavior? What determines the extent to which a forager will use directional information from the dance? They suggest future research consider the communication process to be the result of multiple forms of information resulting in a complex system—a direction of inquiry that is well-suited to the dynamical and ecological perspectives in psychology. Taking an ecological approach to bees and invertebrates alike could significantly advance our knowledge of their dynamic, highly effective, context-sensitive social system.
Human communication—though it contains many more dimensions—is context sensitive as well. Ecological and social scientists are well equipped to begin applying lessons learned from honey bee dynamics to human collectives. Constraining communication in similar ways may increase specificity and thus decrease ambiguity, in turn reducing the amount of information lost in communication. If achievable, perhaps human groups engaged in collaborative joint tasks could become as efficient as social insect collectives. Although this may not be feasible for all human groups, those whose actions and tasks can be sufficiently constrained may experience improvements that—while perhaps only slight at the individual level—can have large impacts on group outcomes (see Discussion section for more detail).
Honey bee collective phenomena
The study of interpersonal dynamics—for example, in coordination of movement and communication (e.g., Abney et al., Citation2014; Marsh et al., Citation2009; Paxton & Dale, Citation2013; Richardson, Marsh, Isenhower, et al., Citation2007)—has been a staple in the ecological psychology literature. Although mentions of honey bees can be found sprinkled in Gibsonian-flavored works (e.g., Shaw & Turvey, Citation1981; Srinivasan, Citation1998; Turvey, Citation2018), no roadmap for an ecological study of honey bees—and the benefits such an approach might bring—has yet been articulated. In this section, we outline potential pathways of investigation that may benefit and promote the ecological approach.
Identifying suitable nest-sites as perceiving nested group affordances
The waggle dance is used in other colony processes beyond foraging related communication. Swarming is a method of colony growth and advancement (Lewis & Schneider, Citation2008). In late spring, the queen bee resumes her normal laying patterns, propagating many new bees (Seeley, Citation1982b). After these preparation measures, approximately half of the worker bees and the existing queen uproot from the original hive and move to a new site, leaving behind the remaining half of the workers, who construct 6–12 large peanut-looking comb cells and rear daughter queens (Painter-Kurt & Schneider, Citation2010; Pierce et al., Citation2007; Seeley, Citation1982b; Winston, Citation1987). The strongest daughter queen, determined by a stinging duel, will assume power of the hive (Seeley, Citation1982b). This reproductive process allows for the expansion of one hive into two independent colonies.
When a colony is ready to swarm, they temporarily relocate to a branch near the original colony, and foragers leave the swarm cluster to scout for potential new homes. Only an estimated 5% of foragers participate in the search for a new home, but those who do may fly up to 10 kilometers away (Seeley, Citation1982b; Seeley et al., Citation1979), using the waggle dance upon their return to communicate viable locations. Previous research has demonstrated that a number of factors influence a scout’s evaluation of a new home, including the volume of the cavity, entrance height relative to the ground, entrance height relative to the cavity floor, and overall entrance size (Seeley, Citation1977, Citation1982b; Seeley & Morse, Citation1978; Visscher et al., Citation1985).
Despite our ability to identify what these important variables seem to be, it is currently unclear how a bee decides what would make a suitable nest-site given the vast difference in size between a single bee and a potential nest-site that can accommodate thousands of bees. However, as a problem of body-scaled affordance perception (e.g., Wagman, Langley, et al., Citation2017), nested affordances (e.g., Wagman et al., Citation2016), and perception of affordances for others (e.g., Wagman, Stoffregen, et al., Citation2017), ecological psychology is well-suited to help explain this phenomenon and to use this phenomenon as a model for studying nested affordances for a collective.
Although dynamical systems perspectives point to insect structures as canonical examples of self-organization and emergence (e.g., termite mounds, honeycomb formation; Camazine et al., Citation2003), these models are often largely concerned with identifying the simple rules by which individual insects’ local interactions with one another and their environments lead to complex macro-level structures. By contrast, nest-site identification provides an interesting test-case in which an individual insect’s perception has dramatic implications for the collective. From an ecological perspective, the question of potential nest-site identification allows for novel investigation of nested affordances and affordance perception beyond a two-animal system (cf. Wagman, Stoffregen, et al., Citation2017) and into a substantially larger group system, given that a single bee must be able to perceive the affordances for a yet-to-be-built emergent structure that no bee could construct alone.
Scout bees may be tuning to higher-order variables—that is, patterns of stimulation that specify information about the environment. Indirect theories of perception argue for lower-order stimulation such as points of light that must be processed and enriched by a perceiver. By contrast, higher-order variables—such as optic flow—directly specify an animal's relationship to its environment and do not require enrichment. All necessary information exists within the pattern of stimulation. For example, when scouts are traversing the interior of a potential nest-site, indirect theories might argue that perception of volume is based on sensation to the retina and internal calculations. From a direct perception approach, the duration of optic flow could be used as a higher-order variable specifying volume. The longer it takes to visually inspect every inch of the cavity, the larger it is. Identification of higher-order variables that specify the viability of cavities as hive sites can lead to interesting new insights into this selection process. Alternatively, mechanoreceptors (a key component for haptic perception across many species; Turvey & Fonseca, Citation2014; e.g., as used by walking spiders; Zill & Seyfarth, Citation1996) used during the exploration process could directly specify interior cavity size.
Earlier theories of human perception suggested that our perceptual and cognitive capabilities were special and unparalleled by that of other organisms. Now, by bridging accounts of human perception with that of insects—such as mechanoreceptors used in haptic perception—we can support the theory that ecological constraints, specific to the species at hand, give rise to higher-order relations that guide perception-action. This venture—and others like it—would promote the efficacy of an ecological approach to perception-action that spans all species (i.e., providing support for Hume’s touchstone; see Hume, Citation1983; Turvey & Shaw, Citation1999).
Nest-site selection as collective decision-making
When one honey bee has thoroughly inspected and settled upon a particular nest-site location, they will return to the swarm cluster and run around on the surface of the swarm while waggle dancing vigorously (Lindauer, Citation1955, Citation1961; Seeley & Buhrman, Citation2001). Approximately one-third of scouts visiting a particular site will actually advertise it, and those who do will perform dances that are nearly twice as long as dances intended for foraging purposes (Camazine et al., Citation1999). It remains unclear what factors play a role in the decision to advertise or refrain from doing so.
Upon returning, these scouts will also observe dances advertising competing locations (Visscher & Camazine, Citation1999a). Only about 3–10% of scouts will visit both potential sites in a bilateral decision process, with the majority performing multiple visits when their first choice of site was inferior to the other or when the other had a large majority of dance advertisement (Camazine et al., Citation1999; Visscher & Camazine, Citation1999b). Onlookers differentiate between the value of the sites through the vigor of the dance, similar to the increased number of waggle runs for more profitable food sources (Lindauer, Citation1955, Citation1961; Seeley & Buhrman, Citation2001).
After visiting the potential locations, the swarm cluster must come to a kind of consensus. Some researchers have suggested that absolute unanimity is needed (Lindauer, Citation1955; Seeley & Buhrman, Citation1999; Visscher & Camazine, Citation1999b), but this is contradicted by other researchers’ observations of a handful of scouts still advertising the inferior site when the swarm lifts off (Camazine et al., Citation1999; Seeley & Buhrman, Citation1999). Camazine et al. (Citation1999) suggested that the decision is only made after the scouts feel that there has been considerable debate between the locations, determined by the number of scouts found at the preferred location, in turn giving the appearance of unanimity. However, this hypothesis has not yet reached empirical consensus. Camazine et al. (Citation1999) echo Gibsonian and dynamical systems approaches in their work, noting that reaching a decision on a single site
resembles not a committee where individuals compare and choose nest sites and persuade others to their choice, but rather a self-organized system where simple rules for individual behavior lead to growing recruitment from some sites and dropout at others until a clear collective decision is reached. (italics added, p. 379).
While Camazine and colleagues were not (to our knowledge) explicitly Gibsonian, their conceptualization of this process highlights the congruence between the ecological perspective and the thinking of many ecologists. To better understand this decision-making process, Seeley and Visscher (Citation2003) sought to evaluate two hypothesized decision thresholds: quorum-sensing and consensus-sensing. Consensus-sensing requires all scouts to perform the same waggle dance, indicating the same potential nest-site, while quorum-sensing requires only a majority of scouts to advertise one site over another. Seeley and Visscher (Citation2003) found that honey bees more closely followed the quorum-sensing hypothesis when there were 10–15 scouts at the actual preferred nest-site. However, the question of how the bees confirm the number of scouts present at the site—whether by physical contact or vision when in close proximity—is still unsolved, given that both visual, tactile, and chemical signals play important roles in bee communication. These decision-making processes in honey bees have been fruitfully simulated in the past using models of human behavior, like cognitive reaction-time models (cf. Passino et al., Citation2008) or information flow in human groups (cf. Britton et al., Citation2002), providing support for a more explicit connection between the study of human and honey bee social processes.
We believe that ecological psychology could benefit from using this phenomenon as a model of collective decision-making. Most research to date has taken a more traditional, cognitive approach to modeling and understanding honey bee cognition (cf. Britton et al., Citation2002; Passino et al., Citation2008); in other work, neuroscientists have found striking parallels to human neural architecture (e.g., Passino et al., Citation2008; Visscher, Citation2007) and brain dynamics during choice tasks (Marshall et al., Citation2009). However, honey bees—like other animals—can serve as a convenient model for understanding basic perception and action without the conflation of other cognitive complexities that can come with studying humans. By studying how individual agents that lack complex neural architectures come to agreement, we may be able to develop a more ecological explanation to decision-making—a relatively understudied area in ecological psychology (but not completely unexplored; e.g., decision-making in sports and games; see Araujo et al., Citation2006; Araujo et al., Citation2009; Benerink et al., Citation2016; Nalepka et al., Citation2017).
Task allocation as a complex adaptive system
One of the most noteworthy accomplishments of honey bees is their seamless worker organization across essential hive duties, otherwise known as task allocation. Individual members all participate in group coordination of action to achieve a sole purpose, the survival and growth of the colony (Seeley, Citation1998). They do this through task partitioning, in which the large tasks such as foraging and food storage are divided into many smaller, individually achievable subtasks (Anderson et al., Citation2001; Burd & Howard, Citation2008; Ratnieks & Anderson, Citation1999), such as collecting pollen, collecting nectar, receiving the loads from foragers, storing the loads, and processing the nectar until it is honey (Seeley, Citation1995).
Honey bees adopt new tasks as a function of their age, a process known as age polyethism. Workers transition to new sets of jobs as time passes, with each set of jobs being spatially related within the hive (Seeley, Citation1982a). Seeley (Citation1982a) identified five castes, including a single reproductive caste (the queen bee) and four worker castes (the female bees): the cell cleaning caste, brood nest caste, food storage caste, and forager caste. The assembly-line-like process of task allocation in conjunction with age polyethism requires the smooth transition of labor supply among various tasks, successive promotion to new tasks as individual workers age, and ability for workers to change jobs in times of need (Seeley, Citation1995).
For example, as described in the “Context-Sensitive Communication” section above, foragers will use the shaking signal to encourage higher levels of activity and an increase in receiver bees. They will also use the signal in the early morning to stimulate other foragers to begin collecting, with a positive correlation between morning vibration signals and successful multi-day foraging (Nieh, Citation1998), later waggle dance production (Schneider et al., Citation1986b), future foraging, and the synchronization of foraging with other non-foraging related tasks (Schneider & Lewis, Citation2004). These signals indicate various task needs and help regulate the in-hive productivity of foraging and non-foraging functions of the hive. Synchronization of tasks is essential for the effective tradeoff of materials and continual work rotation. Extensive algorithms have been developed—such as the well-known Artificial Bee Colony (ABC) algorithm (for review, see Ab Wahab et al., Citation2015)—in an attempt to broaden knowledge on task allocation in social insect collectives and explore other applications for real-world issues.
In times of highly profitable foraging, the colony must regulate the allocation of their in-hive workers to maximize hive benefit. Doing so allows the success of the collective, rather than any particular individual, and occurs without an explicit leader or top-down organization. These macro-level adjustments to activity that rely on micro-level dynamics pose interesting avenues for ecological psychology. Disengaged worker bees have been observed patrolling the hive, inspecting clusters of cells and gathering information from all work areas (Johnson, Citation2008), but it remains unclear how patrolling workers ultimately decide which task they will adopt. Investigation of the intentional information-seeking behavior of these workers could lead to insights into the task allocation process.
Interim discussion
In studying the perception-action capabilities of honey bee collectives, we hope to inform the literature of higher-order colony regulation as a result of lower-order adjustments (see ). This holistic approach to inter-organism coordination of action allows ecological psychology to explore the world of social insects, as well as artificial collective intelligence. Exploration of synchronization and pattern formation has already led to discoveries of motion coordination in non-living dissipative structures (Davis et al., Citation2016). Further investigation of these principles in living, collective social insects will allow us to divulge how coordination of action leads to collective success, and inform the field of potential behaviors that may be used for human group improvement.
Real-world implications
Many parallels have already been drawn between honey bees and humans, and multidisciplinary researchers have worked to apply models of their behavior to human society (e.g., Nayar et al., Citation2019; Reina et al., Citation2018) and robotics (for review of tasks, see Bayındır, Citation2016). Applying a dynamical systems perspective could help identify underlying dynamics of honey bees’ complicated social system and pinpoint laws of inter-organism coordination of action—just as dynamical systems perspectives have improved our understanding of interpersonal human systems (e.g., Richardson, Marsh, Isenhower, et al., Citation2007, Citation2014; Schmidt & Richardson, Citation2008).
To date, ecological psychology has tended to avoid the study of more social phenomena, given the difficulty of grounding social phenomena outside of cognitivist models (for overview, see Paxton et al., Citation2019). Exploration of honey bees’ efficacious task allocation and hive regulation methods could inform ecological psychology by improving our understanding of the underlying dynamics of micro-level activity coordination in the regulation of macro-level dynamics across multiple species. In doing so, we can begin to approach ecological models for understanding human cognitive and social phenomena like blind insight, confirmation bias, and groupthink as embedded individual and collective processes.
While the literature surrounding the construct of human collective intelligence has come under debate (e.g., Credé et al., Citation2017; Woolley et al., Citation2018), Goldstone and Gureckis (Citation2009) have suggested four critical dimensions of variation for classifying human groups: primary motivation of individuals (i.e., selfish or for the group benefit), interactions among individuals (i.e., cooperative or competitive), typical dynamic of individuals (i.e., imitation of others or differentiation from them), and characteristic outcome of the collective behavior. We believe that these categories may also be useful when considering differences between human and social insect groups; therefore, it is along these dimensions that we suggest using social insects as a model for enhancing human groups. The debate over a collective intelligence construct may be in part due to fundamental differences along these four spectra. If we wish to use insect collectives as a model for human groups, it is essential that we identify not only a particular insect but a particular phenomenon that lies within the same values on these dimensions.
When considering the dynamics of honey bee colonies, parallels can be drawn with aspects of human society, from political action to work teams. Honey bee interactions are related to that of humans across several dimensions. Members of both groups communicate locally, the quality of various opinions or options does not necessarily need to be compared by individuals (although humans often do), and no single individual must have a global view of all potential options and opinions, but all the pieces of information held by various individuals does contribute to the final group outcome (Goldstone & Gureckis, Citation2009). For example, the internet allows for targeted communication, acquiring of information but not the full collection of diverse opinions, and people do not need to consider (and likely cannot collect) all possible opinions. Yet when decisions are made (e.g., during democratic elections), all information disseminated on the internet plays some role in the eventual outcome. Thomas Seeley (Citation1995) likened the nest-site selection of a colony to a New England town meeting, where individuals communicate locally, share and accept information at their own will, and all information across all individuals at the meeting weigh into the final decision.
Finding aligned phenomena
It may be helpful to readers for us to consider in detail one specific example of how a particular social insect phenomenon might align well with a particular human collective phenomenon. To do so, we examine the parallels between honey bees and accountants, both in structure and along the dimensions of variability outlined by Goldstone and Gureckis (Citation2009). We use this as an opportunity to further introduce a novel dimension of collective dynamics—and, more specifically, collective communication—that may be particularly useful when drawing lessons for human groups from social insect collectives.
The honey bee task allocation process of acquisition, transfer, and storage of food aligns with the typical structure of a large accounting firm. Here, young accountants are assigned the menial tasks. They take less intellectual effort and carry less risk on behalf of the worker’s standing with the company and on the quality of the final product (e.g., an audit report). As they progress to more complex roles, the associated risk increases, similar to how forager bees carry the greatest risk being out in the wild subject to adverse weather and predators and lack of productivity could contribute to starvation of the colony. The promotion schedules of both collectives run on regular, incremental timelines: Accountants typically have regular, staggered promotion schedules, and honey bees switch to new roles with little variation in promotion timeline (Seeley, Citation1982a). During their time in each job, individuals perform repetitive tasks until mastery. Both learn new roles mainly through observation: Accountants use documents from previous years completed by those formerly in their role for guidance, and honey bees learn the roles of others through observation without any kind of explicit instruction.
Along the dimensions of variation outlined by Goldstone and Gureckis (Citation2009), these two collective groups share their primary motivation (the success of the group), the individual interactions (cooperative; though this drastically differs in other business fields such as finance), and their typical dynamic (imitation). The dimension along which they vary is their characteristic outcome. Honey bee colonies coordinate their foraging roles seamlessly, continually storing away food for the winter. Human accounting teams are similarly productive, but their productivity is more variable than that of a honey bee colony. We believe this is due to another aspect of the individual interaction dimension, one not discussed by Goldstone and Gureckis: the communication toolkit (or the number and variety of communication modalities available).
The human toolkit for communication is rich and multimodal, leaving room for error by the communicator and recipient. Honey bee signals embrace specificity, but there may be multiple ways of conveying the same idea in human language. Accounting teams that rely on virtual collaboration use instant messaging and emails for short communications and requests, while larger requests and client interactions are handled via phone calls. Given the limitations of the messaging platforms, possibilities for miscommunication on behalf of the informer, and room for interpretation on behalf of the receiver, information is not always reliably transmitted. As the working world becomes increasingly virtual, efforts to disambiguate and assure reliable transfer of information are even more important. Thus it is along the communication toolkit dimension that foraging honey bees may inspire changes to work groups that are similar in structure to accounting firms. If we can constrain and guide communication in ways that result in higher specificity of information, these teams may become more successful.
Work to empirically investigate this idea is currently underway. We are studying how different constraints on dyadic human communication impact team productivity on a joint action task inspired by the processing pipeline of foraging honey bees. We are also extending the paradigm to online interactive experiments, to explore these impacts in larger groups—from a handful to dozens of individuals.
Through this example, we hope to inspire a two-fold method for reliably using insect collectives as a model for engineering human collectives. First, a suitable insect collective must be identified. This has been increasingly done in many fields, but success has been variable—partly due, we believe, to overlooking critical dimensions of variability. We propose that a second criteria must also be met in order to effectively use insect dynamics: a suitable collective phenomenon. Any insect collective demonstrates a number of goals and group behaviors, each with their own values along the dimensions of variation, and only some of those will be similar enough to provide an effective analogy for human groups. It is along these dimensions that a suitable phenomenon must be identified so that differences in dynamics and structure can be used to guide recommendations for improvement.
While some argue that models of collective behavior are too unconstrained, their wide applicability helps reveal similar dynamics across organisms. As Goldstone and Gureckis (Citation2009) note, integrated endeavors and investigation of shared principles across seemingly unrelated phenomena have lead to some of the most noteworthy scientific feats, including the unification of electromagnetic and gravitational acceleration by Einstein (Citation1945), and the unification of evolution principles of humans and snails by Darwin (Citation1859). It is by this argument that we promote the unified investigation of honey bee colonies and human groups.
Conclusion
Social invertebrate collectives exhibit many phenomena of interest to ecological psychologists, particularly at the group level. While the success of blending research on social insects and human psychology to understand collective dynamics has been mixed to date, we propose that productive integration of these fields requires identifying both the right social insect and the right phenomenon. Once identified, fundamental differences along other spectra—such as communication toolkits—can be leveraged to constrain human group behavior (at individual and interpersonal levels) to create more productive collectives. Although the extent to which these alterations can be made is variable, even slight improvement at the individual-level can result in system-level improvement. Through their task allocation and nest-site selection, the western honey bee, Apis mellifera, demonstrates remarkable parallels to human collective dynamics and offers a new avenue for ecological psychology and human factors to explore together in pursuit of improving human coordination of action.
Acknowledgements
We would like to thank the attendees of the 20th International Conference on Perception and Action for their feedback on an earlier version of this work (presented as part of the symposium, “Action Coordination in Non-Human Self-Organizing Collectives: Multidisciplinary Lessons from Living and Nonliving Systems”). We would also like to thank two anonymous reviewers for their thoughtful suggestions on the paper’s structure and narrative flow. Lastly, our thanks go out to Salvatore Vancio for his feedback on the manuscript and helping us ground the analogies between honey bee collective phenomena and accounting firms.
References
- Ab Wahab, M. N., Nefti-Meziani, S., & Atyabi, A. (2015). A comprehensive review of swarm optimization algorithms. PloS One, 10(5), e0122827. https://doi.org/10.1371/journal.pone.0122827
- Abney, D. H., Paxton, A., Dale, R., & Kello, C. T. (2014). Complexity matching in dyadic conversation. Journal of Experimental Psychology: General, 143(6), 2304–2315. https://doi.org/10.1037/xge0000021
- Anderson, C., Franks, N. R., & McShea, D. W. (2001). The complexity and hierarchical structure of tasks in insect societies. Animal Behaviour, 62(4), 643–651. https://doi.org/10.1006/anbe.2001.1795
- Araujo, D., Davids, K. W., & Hristovski, R. (2006). The ecological dynamics of decision-making in sport. Psychology of Sport and Exercise, 7(6), 653–676.
- Araujo, D., Davids, K. W., Yi Chow, J., Passos, P., & Raab, M. (2009). The development of decision making skill in sport: An ecological dynamics perspective. In D. Araujo, H. Ripoll, & M. Raab (Eds.), Perspectives on cognition and action in sport (pp. 157–169). Nova Science Publishers, Inc.
- Avarguès-Weber, A., Mota, T., & Giurfa, M. (2012). New vistas on honey bee vision. Apidologie, 43(3), 244–268. https://doi.org/10.1007/s13592-012-0124-2
- Bayındır, L. (2016). A review of swarm robotics tasks. Neurocomputing, 172, 292–321. https://doi.org/10.1016/j.neucom.2015.05.116
- Beier, W. (1968). Beeinflussung der inneren Uhr der Bienen durch Phasenverschiebung des Licht- Dunkel-Zeitgebers. Zeitschrift Fur Bienenforschung, 9, 356–378.
- Beier, W., & Lindauer, M. (1970). Der Sonnenstand als Zeitgeber für die Biene. Apidologie, 1(1), 5–28. https://doi.org/10.1051/apido:19700101
- Benerink, N. H., Zaal, F. T., Casanova, R., Bonnardel, N., & Bootsma, R. J. (2016). Playing 'Pong' together: Emergent coordination in a doubles interception task. Frontiers in Psychology, 7, 1910. https://doi.org/10.3389/fpsyg.2016.01910
- Bennett, M. F., & Renner, M. (1963). The collecting performance of honeybees under laboratory conditions. The Biological Bulletin, 125(3), 416–430. https://doi.org/10.2307/1539356
- Biesmeijer, J. C. (2003). The occurrence and context of the shaking signal in honey bees (Apis mellifera) exploiting natural food sources. Ethology, 109(12), 1009–1020. https://doi.org/10.1046/j.0179-1613.2003.00939.x
- Biesmeijer, J. C., & Seeley, T. D. (2005). The use of waggle dance information by honey bees throughout their foraging careers. Behavioral Ecology and Sociobiology, 59(1), 133–142. https://doi.org/10.1007/s00265-005-0019-6
- Britton, N. F., Franks, N. R., Pratt, S. C., & Seeley, T. D. (2002). Deciding on a new home: How do honeybees agree? Proceedings of the Royal Society B: Biological Sciences, 269(1498), 1383–1388. https://doi.org/10.1098/rspb.2002.2001
- Burd, M., & Howard, J. J. (2008). Optimality in a partitioned task performed by social insects. Biology Letters, 4(6), 627–629. https://doi.org/10.1098/rsbl.2008.0398
- Camazine, S. (1991). Self-organizing pattern formation on the combs of honey bee colonies. Behavioral Ecology and Sociobiology, 28(1), 61–76. https://doi.org/10.1007/BF00172140
- Camazine, S., Deneubourg, J. L., Franks, N. R., Sneyd, J., Bonabeau, E., & Theraula, G. (2003). Self-organization in biological systems (Vol. 7). Princeton university press.
- Camazine, S., Sneyd, J., Jenkins, M. J., & Murray, J. D. (1990). A mathematical model of self-organized pattern formation on the combs of honeybee colonies. Journal of Theoretical Biology, 147(4), 553–571. https://doi.org/10.1016/S0022-5193(05)80264-4
- Camazine, S., Visscher, K. P., Finley, J., & Vetter, R. S. (1999). House-hunting by honey bee swarms: Collective decisions and individual behaviors. Insectes Sociaux, 46(4), 348–360. https://doi.org/10.1007/s000400050156
- Couzin, I. D., Krause, J., Franks, N. R., & Levin, S. A.(2005). Effective leadership and decision-making in animal groups on the move. Nature, 433(7025), 513-516.
- Couzin, I. D. (2009). Collective cognition in animal groups. Trends in Cognitive Sciences, 13(1), 36–43. https://doi.org/10.1016/j.tics.2008.10.002
- Credé, M., & Howardson, G. (2017). The structure of group task performance-A second look at “collective intelligence”: Comment on Woolley et al. (2010). The Journal of Applied Psychology, 102(10), 1483–1492. https://doi.org/10.1037/apl0000176
- Dacke, M., & Srinivasan, M. V. (2007). Honeybee navigation: distance estimation in the third dimension. The Journal of Experimental Biology, 210(Pt 5), 845–853. https://doi.org/10.1242/jeb.002089
- Darwin, C. (1859). On the origin of species by means of natural selection. John Murray.
- Davis, T. J., Kay, B. A., Kondepudi, D., & Dixon, J. A. (2016). Ecological psychology spontaneous inter entity coordination in a dissipative structure. Ecological Psychology, 28(1), 23–36. https://doi.org/10.1080/10407413.2016.1121737
- Dornhaus, A., & Chittka, L. (2004). Why do honey bees dance? Behavioral Ecology and Sociobiology, 55(4), 395–401. https://doi.org/10.1007/s00265-003-0726-9
- Duncker, K. (1945). On problem-solving. Psychological Monographs, 58(5), i–113. https://doi.org/10.1037/h0093599
- Einstein, A. (1945). The meaning of relativity. Princeton University Press.
- Esch, H., & Bastian, J. A. (1970). How do newly recruited honey bees approach a food site? Zeitschrift fuer vergleichende Physiologie, 68(2), 175–181.
- Esch, H., & Burns, J. (1996). Distance estimation by foraging honeybees. The Journal of Experimental Biology, 199(Pt 1), 155–162.
- Fletcher, D. J. C. (1978). The influence of vibratory dances by worker honeybees on the activity of virgin queens. Journal of Apicultural Research, 17(1), 3–13. (https://doi.org/10.1080/00218839.1978.11099893
- German, T. P., & Defeyter, M. A. (2000). Immunity to functional fixedness in young children. Psychonomic Bulletin & Review, 7(4), 707–712. https://doi.org/10.3758/bf03213010
- Gibson, J. J. (1979). The ecological approach to visual perception. Houghton Mifflin.
- Goldstone, R. L., & Gureckis, T. M. (2009). Collective behavior. Topics in Cognitive Science, 1(3), 412–438. https://doi.org/10.1111/j.1756-8765.2009.01038.x
- Gould, J. M., & Gould, C. G. (1988). The honey bee. Scientific American Library.
- Grüter, C., Balbuena, M. S., & Farina, W. M. (2008). Informational conflicts created by the waggle dance. Proceedings of the Royal Society B: Biological Sciences, 275(1640), 1321–1327. https://doi.org/10.1098/rspb.2008.0186
- Grüter, C., & Farina, W. M. (2009). The honeybee waggle dance: Can we follow the steps? Trends in Ecology & Evolution, 24(5), 242–247. https://doi.org/10.1016/j.tree.2008.12.007
- Hills, T. T., Todd, P. M., Lazer, D., Redish, A. D., Couzin, I. D., & Cognitive Search Research Group. (2015). Exploration Versusexploitation in Space, Mind, and Society. Trends in Cognitive Sciences, 19(1), 46–54.
- Hume, D. (1983). A treatise of human nature. Clarendone Press.
- Hyland, K. M., Cao, T. T., Malechuk, A. M., Lewis, L. A., & Schneider, S. S. (2007). Vibration signal behaviour and the use of modulatory communication in established and newly founded honey bee colonies. Animal Behaviour, 73(3), 541–551. https://doi.org/10.1016/j.anbehav.2006.10.006
- Johnson, B. R. (2008). Global information sampling in the honey bee. Die Naturwissenschaften, 95(6), 523–530. https://doi.org/10.1007/s00114-008-0354-3
- Kirchner, W. H. (1993). Vibrational signals in the tremble dance of the honeybee, Apis mellifera. Behavioral Ecology and Sociobiology, 33(3), 169–172. https://doi.org/10.1007/BF00216597
- Klein, A.-M., Vaissière, B. E., Cane, J. H., Steffan-Dewenter, I., Cunningham, S. A., Kremen, C., & Tscharntke, T. (2007). Importance of pollinators in changing landscapes for world crops. Proceedings of the Royal Society B: Biological Sciences, 274(1608), 303–313. https://doi.org/10.1098/rspb.2006.3721
- Krause, J., Ruxton, G. D., & Krause, S. (2010). Swarm intelligence in animals and humans. Trends in Ecology & Evolution, 25(1), 28–34. https://doi.org/10.1016/j.tree.2009.06.016
- Lehrer, M. (1994). Spatial vision in the honeybee: The use of different cues in different tasks. Vision Research, 34(18), 2363–2385. https://doi.org/10.1016/0042-6989(94)90282-8
- Lewis, L. A., & Schneider, S. S. (2008). “Migration dances” in swarming colonies of the honey bee, Apis mellifera. Apidologie, 39(3), 354–361. https://doi.org/10.1051/apido:2008018
- Lewis, L. A., Schneider, S. S., & Degrandi-Hoffman, G. (2002). Factors influencing the selection of recipients by workers performing vibration signals in colonies of the honey bee. Animal Behaviour, 63(2), 361–367. https://doi.org/10.1006/anbe.2001.1894
- Lindauer, M. (1955). Schwarmbienen auf Wohnungssuche. Zeitschrift für Vergleichende Physiologie, 37(4), 263–324. https://doi.org/10.1007/BF00303153
- Lindauer, M. (1961). Communication among social bees. Harvard University Press.
- Marsh, K. L., Richardson, M. J., & Schmidt, R. C. (2009). Social connection through joint action and interpersonal coordination. Topics in Cognitive Science, 1(2), 320–339. https://doi.org/10.1111/j.1756-8765.2009.01022.x
- Marshall, J. A. R., Bogacz, R., Dornhaus, A., Planqué, R., Kovacs, T., & Franks, N. R. (2009). On optimal decision-making in brains and social insect colonies. Journal of the Royal Society, Interface, 6(40), 1065–1074. https://doi.org/10.1098/rsif.2008.0511
- Mautz, D. (1971). Der Kommunikationseffekt der Schwänzeltänze bei Apis mellifera carnica (Pollm). Zeitschrift für Vergleichende Physiologie, 72(2), 197–220. https://doi.org/10.1007/BF00297822
- Mlot, N. J., Tovey, C. A., & Hu, D. L. (2011). Fire ants self-assemble into waterproof rafts to survive floods. Proceedings of the National Academy of Sciences, 108(19), 7669–7673. https://doi.org/10.1073/pnas.1016658108
- Nalepka, P., Kallen, R. W., Chemero, A., Saltzman, E., & Richardson, M. J. (2017). Herd those sheep: Emergent multiagent coordination and behavioral-mode switching. Psychological Science, 28(5), 630–650. https://doi.org/10.1177/0956797617692107
- Nayar, N., Ahuja, S., & Jain, S. (2019). Swarm intelligence for feature selection: A review of literature and reflection on future challenges. In Advances in Data and Information Sciences (pp. 211–221). Springer.
- Nicolis, G., & Prigogine, I. (1977). Self-organization in nonequilibrium systems. Wiley.
- Nieh, J. C. (1993). The stop signal of honey bees: Reconsidering its message. Behavioral Ecology and Sociobiology, 33(1), 51–56. https://doi.org/10.1007/BF00164346
- Nieh, J. C. (1998). The honey bee shaking signal: Function and design of a modulatory communication signal. Behavioral Ecology and Sociobiology, 42(1), 23–36. https://doi.org/10.1007/s002650050408
- Nieh, J. C. (2010). A negative feedback signal that is triggered by peril curbs honey bee recruitment. Current Biology: CB, 20(4), 310–315. https://doi.org/10.1016/j.cub.2009.12.060
- Painter-Kurt, S., & Schneider, S. S. (2010). Age and behavior of honey bees, Apis mellifera (Hymenoptera: Apidae), that perform vibration signals on queens and queen cells. Ethology, 104(6), 475–485. https://doi.org/10.1111/j.1439-0310.1998.tb00084.x
- Passino, K. M., Seeley, T. D., & Visscher, K. P. (2008). Swarm cognition in honey bees. Behavioral Ecology and Sociobiology, 62(3), 401–414. https://doi.org/10.1007/s00265-007-0468-l
- Pastor, K. A., & Seeley, T. D. (2005). The brief piping signal of the honey bee: Begging call or stop signal? Ethology, 111(8), 775–784. https://doi.org/10.1111/j.1439-0310.2005.01116.x
- Paxton, A., Blau, J. J. C., & Weston, M. L. (2019). The case for intersectionality in ecological psychology. Studies in Perception and Action XV: Proceedings from the Twentieth International Conference on Perception and Action.
- Paxton, A., & Dale, R. (2013). Argument disrupts interpersonal synchrony. Quarterly Journal of Experimental Psychology (2006), 66(11), 2092–2102. https://doi.org/10.1080/17470218.2013.853089
- Pierce, A. L., Lewis, L. A., & Schneider, S. S. (2007). The use of the vibration signal and worker piping to influence queen behavior during swarming in honey bees, Apis mellifera. Ethology, 113(3), 267–275. https://doi.org/10.1111/j.1439-0310.2006.01314.x
- Primack, R. B. (1985). Longevity of individual flowers. Annual Review of Ecology and Systematics, 16(1), 15–37. https://doi.org/10.1146/annurev.es.16.110185.000311
- Rajasekhar, A., Lynn, N., Das, S., & Suganthan, P. N. (2017). Computing with the collective intelligence of honey bees – A survey. Swarm and Evolutionary Computation, 32, 25–48. https://doi.org/10.1016/j.swevo.2016.06.001
- Ratnieks, F. L. W., & Anderson, C. (1999). Task partitioning in insect societies. II. Use of queueing delay information in recruitment. The American Naturalist, 154(5), 536–548. https://doi.org/10.1086/303256
- Raveret-Richter, M., & Waddington, K. D. (1993). Past foraging experience influences honey bee dance behaviour. Animal Behaviour, 46(1), 123–128. https://doi.org/10.1006/anbe.1993.1167
- Reina, A., Bose, T., Trianni, V., & Marshall, J. A. R. (2018). Psychophysical laws and the superorganism. Scientific Reports, 8(1), 4387. https://doi.org/10.1038/s41598-018-22616-y
- Renner, M. (1955). Über die Haltung von Bienen in geschlossenen, künstlich beleuchteten Räumen. Die Naturwissenschaften, 42(19), 539–540. https://doi.org/10.1007/BF00630155
- Renner, M. (1957). Neue versuche über den zeitsinn der honigbiene. Zeitschrift für vergleichende. Zeitschrift für Vergleichende Physiologie, 40(1), 85–118. https://doi.org/10.1007/BF00298152
- Richardson, M. J., Dale, R., & Marsh, K. L. (2014). Complex dynamical systems in social and personality psychology: Theory, modeling, and analysis. In H. T. Reis and C. M. Judd (Eds.), Handbook of research methods in social and personality psychology: Theory, modeling, and analysis. Cambridge University Press.
- Richardson, M. J., Marsh, K. L., & Baron, R. M. (2007). Judging and actualizing intrapersonal and interpersonal affordances. Journal of Experimental Psychology: Human Perception and Performance, 33(4), 845–859. https://doi.org/10.1037/0096-1523.33.4.845
- Richardson, M. J., Marsh, K. L., Isenhower, R. W., Goodman, J. R., & Schmidt, R. C. (2007). Rocking together: Dynamics of intentional and unintentional interpersonal coordination. Human Movement Science, 26(6), 867–891. https://doi.org/10.1016/j.humov.2007.07.002
- Schmidt, R. C., & Richardson, M. J. (2008). Dynamics of interpersonal coordination. In Coordination: Neural, behavioral and social dynamics (pp. 281–308). Springer.
- Schneider, S. S. (1989). Spatial foraging patterns of the African honey bee Apis mellifera scutellata. Journal of Insect Behavior, 2(4), 505–521. https://doi.org/10.1007/BF01053351
- Schneider, S. S. (1990). Queen behavior and worker-queen interactions in absconding and swarming colonies of the African honey bee, Apis mellifera scutellata (Hymenoptera: Apidae). Journal of the Kansas Entomological Society, 63(1), 179–186.
- Schneider, S. S. (2010). The modulation of worker activity by the vibration dance of the honey bee. Ethology, 74(3), 211–218. https://doi.org/10.1111/j.1439-0310.1987.tb00934.x
- Schneider, S. S., & Lewis, L. A. (2004). The vibration signal, modulatory communication and the organization of labor in honey bees, Apis mellifera. Apidologie, 35(2), 117–131. https://doi.org/10.1051/apido
- Schneider, S. S., & McNally, L. C. (1991). The vibration dance behavior of queenless workers of the honey bee, Apis mellifera (Hymenoptera: Apidae). Journal of Insect Behavior, 4(3), 319–322. https://doi.org/10.1007/BF01048281
- Schneider, S. S., Stamps, J. A., & Gary, N. E. (1986a). The vibration dance of the honey bee. I. Communication regulating foraging on two time scales. Animal Behaviour, 34(2), 377–385. https://doi.org/10.1016/S0003-3472(86)80105-1
- Schneider, S. S., Stamps, J. A., & Gary, N. E. (1986b). The vibration dance of the honey bee. 2. The effects of foraging success on daily patterns of vibration activity. Animal Behaviour, 34(2), 386–391. https://doi.org/10.1016/S0003-3472(86)80106-3
- Schneider, S. S., Painter-Kurt, S., & Degrandi-Hoffman, G. (2001). The role of the vibration signal during queen competition in colonies of the honey bee, Apis mellifera. Animal Behaviour, 61(6), 1173–1180. https://doi.org/10.1006/anbe.2000.1689
- Seeley, T. (1977). Measurement of nest cavity volume by the honey bee (Apis mellifera). Behavioral Ecology and Sociobiology, 2(2), 201–227. https://doi.org/10.1007/BF00361902
- Seeley, T. D. (1982a). Adaptive significance of the age polyethism schedule in honeybee colonies. Behavioral Ecology and Sociobiology, 11(4), 287–293. https://doi.org/10.1007/BF00299306
- Seeley, T. D. (1982b). How honeybees find a home. Scientific American, 247(4), 158–169. https://doi.org/10.2307/24966709
- Seeley, T. D. (1992). The tremble dance of the honey bee: Message and meanings. Behavioral Ecology and Sociobiology, 31(6), 375–383. https://doi.org/10.1007/BF00170604
- Seeley, T. D. (1995). The wisdom of the hive: The social physiology of honey bee colonies. Harvard University Press.
- Seeley, T. D. (1998). Thoughts on information and integration in honey bee colonies. Apidologie, 29(1–2), 67–80. https://doi.org/10.1051/apido:19980104
- Seeley, T. D., Mikheyev, A. S., & Pagano, G. J. (2000). Dancing bees tune both duration and rate of waggle-run production in relation to nectar-source profitability. Journal of Comparative Physiology. A, Sensory, Neural, and Behavioral Physiology, 186(9), 813–819. https://doi.org/10.1007/s003590000134
- Seeley, T., Morse, R., & Visscher, P. K. (1979). The natural history of the flight of honey bee swarms. Psyche: A Journal of Entomology, 86(2–3), 103–113. https://doi.org/10.1155/1979/80869
- Seeley, T. D., Weidenmüller, A., & Kühnholz, S. (2010). The shaking signal of the honey bee informs workers to prepare for greater activity. Ethology, 104(1), 10–26. https://doi.org/10.1111/j.1439-0310.1998.tb00026.x
- Seeley, T. D., & Buhrman, S. C. (1999). Group decision making in swarms of honey bees. Behavioral Ecology and Sociobiology, 45(1), 19–31. https://doi.org/10.1007/s002650050536
- Seeley, T. D., & Buhrman, S. C. (2001). Nest-site selection in honey bees: How well do swarms implement the “Best-of-N” decision rule? Behavioral Ecology and Sociobiology, 49(5), 416–427. https://doi.org/10.1007/s002650000299
- Seeley, T., & Morse, R. (1978). Nest site selection by the honey bee, Apis mellifera. Insectes Sociaux, 25(4), 323–337. https://doi.org/10.1007/BF02224297
- Seeley, T. D., & Visscher, K. P. (2003). Choosing a home: How the scouts in a honey bee swarm perceive the completion of their group decision making. Behavioral Ecology and Sociobiology, 54(5), 511–520. https://doi.org/10.1007/s00265-003-0664-6
- Shaw, R. E., & Turvey, M. T. (1981). Coalitions as models for ecosystems: A realistic perspective on perceptual organization. In M. Kubovy & J. R. Pomerantz (Eds.), Perceptual Organization (pp. 343–415). Lawrence Erlbaum Associates, Inc.
- Sherman, G., & Visscher, K. P. (2002). Honeybee colonies achieve fitness through dancing. Nature, 419(6910), 920–922. https://doi.org/10.1038/nature01127
- Sinclair, A. R. E.(1977). The African Buffalo: a Study of Resource Limitation of Population. Chicago: University Press of Chicago.
- Srinivasan, M. V. (1998). Insects as Gibsonian animals. Ecological Psychology, 10(3), 251–270. https://doi.org/10.1207/s15326969eco103&4_5
- Sumpter, D. (2006). The principles of collective animal behaviour. Philosophical Transactions of the Royal Society B: Biological Sciences, 361(1465), 5–22. https://doi.org/10.1098/rstb.2005.1733
- Thom, C., Gilley, D. C., Hooper, J., & Esch, H. E. (2007). The scent of the waggle dance. PLoS Biology, 5(9), e228. https://doi.org/10.1371/journal.pbio.0050228
- Turner, S. J. (2011). Termites as models of swarm cognition. Swarm Intelligence, 5(1), 19–43. https://doi.org/10.1007/s11721-010-0049-1
- Turvey, M. T. (2018). Lectures on perception: An ecological perspective. Routledge.
- Turvey, M. T., & Fonseca, S. T. (2014). The medium of haptic perception: A tensegrity hypothesis. Journal of Motor Behavior, 46(3), 143–187. https://doi.org/10.1080/00222895.2013.798252
- Turvey, M. T., & Shaw, R. E. (1999). Ecological foundations of cognition. I: Symmetry and specificity of animal-environment systems. Journal of Consciousness Studies, 6(11–12), 95–110.
- Van Lier, L. (2002). An ecological-semiotic perspective on language and linguistics. In Language acquisition and language socialization: Ecological perspectives (pp. 140–164). Continuum.
- Visscher, K. P., & Seeley, T. D. (1982). Foraging strategy of honeybee colonies in a temperate deciduous forest. Ecology, 63(6), 1790–1801. https://doi.org/10.2307/1940121
- Visscher, K. P. (2007). Group decision making in nest-site selection among social insects. Annual Review of Entomology, 52, 255–275. https://doi.org/10.1146/annurev.ento.51.110104.151025
- Visscher, K. P., & Camazine, S. (1999a). Collective decisions and cognition in bees. Nature, 397(6718), 400. https://doi.org/10.1038/17047
- Visscher, K. P., & Camazine, S. (1999b). The mystery of swarming honeybees: From individual behaviors to collective decisions. In Information processing in social insects (pp. 355–378). Birkhäuser.
- Visscher, K. P., Morse, R. A., & Seeley, T. D. (1985). Honey bees choosing a home prefer previously occupied cavities. Insectes Sociaux, 32(2), 217–220. https://doi.org/10.1007/BF02224236
- von Frisch, K. (1923). Uber die “Sprache” der Bienen, eine tier-psychologische Untersuchung. Zoologisches Jahrbuch Physiol, 40, 1–186.
- von Frisch, K. (1965). Tanzsprache und Orientierung der Bienen. Springer.
- von Frisch, K. (1967). The dance language and orientation of bees. Harvard University Press.
- Waddington, K. D. (1997). Foraging behavior of nectarivores and pollen collectors. Acta Horticulturae, 437(437), 175–192. https://doi.org/10.17660/ActaHortic.1997.437.18
- Wagman, J. B., Langley, M. D., & Farmer-Dougan, V. (2017). Doggone affordances: Canine perception of affordances for reaching. Psychonomic Bulletin & Review, 24(4), 1097–1103. https://doi.org/10.3758/s13423-016-1183-6
- Wagman, J. B., Stoffregen, T. A., Bai, J., & Schloesser, D. S. (2017). Perceiving nested affordances for another person’s actions. Quarterly Journal of Experimental Psychology, 71(3), 790–799. https://doi.org/10.1080/17470218.2016.1277249
- Wagman, J. B., Caputo, S. E., & Stoffregen, T. A. (2016). Hierarchical nesting of affordances in a tool use task. Journal of Experimental Psychology: Human Perception and Performance, 42(10), 1627–1642. https://doi.org/10.1037/xhp0
- Wenner, A. M., Wells, P. H., & Johnson, D. L. (1969). Honey bee recruitment to food sources: Olfaction or language? Science (New York, N.Y.), 164(3875), 84–86. https://doi.org/10.1126/science.164.3875.84
- Winston, M. L. (1987). The biology of the honey bee. Harvard University Press.
- Woolley, A. W., Kim, Y., & Malone, T. W. (2018). Measuring collective intelligence in groups: a reply to Credé and Howardson. June, 1, 5431–5418.
- Worgan, S. F., & Moore, R. K. (2010). Speech as the perception of affordances. Ecological Psychology, 22(4), 327–343. https://doi.org/10.1080/10407413.2010.517125
- Zill, S. N., & Seyfarth, E. A. (1996). Exoskeletal sensors for walking. Scientific American, 275(1), 86–90. https://doi.org/10.1038/scientificamerican0796-86