Abstract
The rising number of deaths caused by fentanyl overdosing in the US due to the overwhelming illicit use of this synthetic opioid has started a global campaign to develop efficient ways to control its production and distribution as well as discovering efficient antidotes to mitigate its lethal effects. Another important vein of focused research established by various agencies lies in the development of efficient and practical protocols for the detection of this opioid and analogs thereof in various matrices, whether environmental or biological in nature, particularly in the field of gas chromatography-mass spectrometry (GC-MS). The following review will cover the literature dealing with the detection and identification of synthetic opioids belonging to the fentanyl class by GC-MS means and hyphenated versions of the technique. Detailed descriptions will be given for the GC-MS methods employed for the analysis of the opioid, starting with the nature of the extraction protocol employed prior to analysis to the actual findings presented by the cited reports. Great effort has gone into describing the methods involved in each paper in a detailed manner and these have been compiled by year in tables at the end of each section for the reader’s convenience. Lastly, the review will end with concluding remarks about the state of GC-MS analysis with regards to these powerful opioids and what lies ahead for this analytical field.
Introduction
Within the past 20 years, lethal overdosing cases involving the illicit use of fentanyl and other congeners belonging to this class of synthetic opioids have caused fear and raised the level of awareness among medical as well as government agencies globally. Originally synthesized with the ultimate goal of improving the effects of anesthesia during perioperative procedures in the clinic,[Citation1,Citation2] these compounds have made their way into the illicit drug market due to their ability to cause euphoria on end users in similar fashion to other well-known drugs like cocaine and heroin. Furthermore, well-known fentanyls as well as newer, more potent analogs are being commonly used as adulterants in heroin and cocaine batches in order to further enhance their euphoric effects, thus expanding their reach and with regards to the new analogs, increasing the difficulty in accurately identifying them.[Citation3] The flagship compound for this class of synthetic opioids, and the first one to break in the medical field of anesthesiology as a major, more powerful alternative to morphine, is fentanyl (). Fentanyl was synthesized by Paul Adriaan Janssen in 1960 as part of a medicinal chemistry program aimed at developing stronger pain-relieving drugs largely based on the pethidine chemical structure ().[Citation4,Citation5] Fentanyl proved to be a blockbuster discovery and found its way into the United States market in 1968 under the trade name Sublimaze®.[Citation6] Since its synthesis and realization of its powerful pain-relieving effects, fentanyl analogs with superior pharmacokinetic properties, onset time, and effective dosage have been successfully produced with a large number of these arising from Janssen’s group structure-activity relationship (SAR) chemistry efforts.[Citation6–9] Currently, a large and constantly expanding array of fentanyl analogs exists possessing a large range of physicochemical properties, which strictly determines their ultimate clinical application. Some of these compounds, along with their trade names and potency relative to morphine, the gold standard, pain-eliminating drug in the clinical setting, are given in .
Figure 1. Structure of fentanyl and the analogs discussed in this review along with the morphine and the pethidine scaffolds. A detailed and large medicinal chemistry approach based on the pethidine nucleus gave birth to the fentanyl class of opioids. In addition, the structures of the two most common, current antidotes to treat fentanyl overdose, Naloxone and Naltrexone, are given. Where applicable for the fentanyls, other common names as well as tradenames are provided along with their individual potencies relative to morphine are given in brackets.
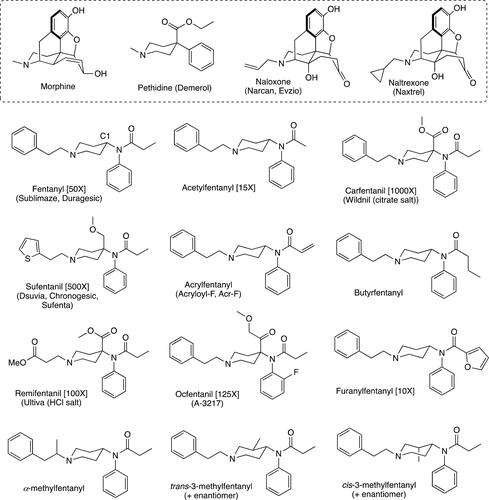
The main biological target of the fentanyls is the µ-opioid receptor, a transmembrane protein present in the central nervous system (CNS) that is responsible for the relaying of signals from the nervous system directly to muscle tissues for their proper functioning.[Citation10–12] Blockage of these receptors by the fentanyl molecule results in an altered state of the protein eventually leading to complete muscle relaxation. The opioid’s lethality originates from making this inhibitory event directly affect muscle tissues involved in respiration, thus causing the affected individuals’ death by respiratory depression and subsequent arrest. Interestingly, the therapeutic window (TW), defined as the difference between a drug’s effective and cytotoxic doses, of some fentanyls is so wide that a lethal concentration of the synthetic opioid is likely not achievable at a given time, but an effective one in causing the paralysis of an individual without causing his/her death. Due to their powerful inhibitory effect on the CNS and given their large TW, these synthetic opioids have found wide applications in the field of chemical warfare agents (CWAs) not as lethal weapons but as incapacitating agents.[Citation13,Citation14] Presently, there are limited antidotes available to the medical community for the treatment of an overdose incident involving these synthetic opioids. One of the most widely employed and effective to a limited extent is naloxone, a drug that is a structural analog of morphine (). Naloxone, known commercially by the tradename Narcan® or Evzio®, acts as an antagonist, competitive binder to the µ-opioid receptor. Approved by the U. S. Food and Drug Administration (FDA) in 1971, it is naloxone’s competitive binding event that keeps the fentanyl from tightly binding to the receptor, leaving enough time for its forced clearance from the system during its unbound state. Unfortunately, naloxone features a short circulation half-life (t1/2 ∼ 2 hours), thus requiring repeated injections of the affected individual to avoid a second overdosing episode by the remaining, longer-acting fentanyl (half-life for fentanyl is t1/2 ∼ 8.5 hours).[Citation15,Citation16] Therefore, it is common practice nowadays that confirmed opioid-overdose patients undergo close monitoring after naloxone treatment for at least 8 hours before their discharge is authorized (CDC, 2013; FDA, 2016).[Citation17,Citation18] Another structurally related antidote, approved by the FDA in 1984, and that has found wide application in this area is naltrexone (tradename: Naxtrel®) that although represents an alternative to naloxone, it still lacks the long-acting effect needed to safely provide prolonged protection against fentanyl due to its still short circulation half-life (t1/2 ∼ 4 hours).[Citation19–21] To this end, several approaches are currently under development to counteract the effects of this class of lethal compounds, these medical countermeasures seek to provide an additional preventive layer of protection to the affected individual and removing the heavy reliability on the limited efficacy of naloxone, naltrexone and other forms of treatment currently used in emergency situations.[Citation22–27]
It has been briefly noted above that one of the most notorious facets of synthetic opioids, specifically those belonging to the fentanyl family, is their use as incapacitating agents. A modern example of this use is highlighted by the Moscow Dubrovka Theater siege in 2002. During this event, Russian military forces employed an aerosolized cocktail of fentanyls to subdue Chechen rebels causing the death of 170 people in the process, 127 of them hostages.[Citation28–30] Although initially carfentanil was suspected as the only opioid employed in aerosolized form in combination with halothane, it was later found by careful analytical work at the Defence Science and Technology Laboratory (DSTL) in the United Kingdom that remifentanil was also a component of the mixture.[Citation31] Even though fentanyl use as a means of incapacitating a threat can have lethal consequences, these cases are sporadic and not often encountered. A more serious side of these opioids and one that continues to have a tremendous impact in society, is their increasing role as a key player in illicit drug trafficking and distribution. For example, within the last ten years fentanyl itself was initially detected in confiscated batches of heroin in the US and Australia, making the overall combined effect a lethal one.[Citation32,Citation33] This type of adulteration of known drug samples has not involved fentanyl itself but other, newer and relatively unknown analogs such as ocfentanil, which was detected in heroin samples purchased from the hidden web.[Citation34] Aside from their involvement in these alarming scenarios, a more preoccupying component of the pandemic is the simplicity of the chemical processes employed for their production, particularly in the case of fentanyl and structurally-related analogs. Most of the methods are largely based on the original synthetic route invented by Janssen but have been modified and improved providing the drug in better yields and reduced number of steps.[Citation35–37] As a result of this, various research and government agencies along with law enforcement have significant advances in the overall fight against these lethal substances that range from controlling their illicit production/distribution pathways to the development of efficient antidotes for use in the medical field.[Citation38,Citation39] In parallel fashion and assuming a crucial supporting role, analytical chemistry in the form of liquid chromatography-mass spectrometry (LC-MS)[Citation40,Citation41] and gas chromatography-mass spectrometry (GC-MS)[Citation42–44] have a provided powerful means for the rapid and effective detection of these agents. This review will focus on several cases where the detection and analysis of fentanyl has been accomplished using solely GC-MS. While LC-MS methods for fentanyl analysis are known to be highly sensitive and unequivocally identify the compound, it still suffers from drawbacks such as operational cost and maintenance and their lack of field deployability (i.e., detection in a CWA-based scenario). The latter in particular becomes an attractive and crucial feature where there is the urgent necessity of an immediate detection and identification of these substances after their release whether it is during a military conflict or a civilian-related scenario. In contrast, GC-MS instrumentation can be found virtually in every analytical chemistry laboratory, in big part due to its much lower cost for purchase, maintenance and operation, while portable versions of the instrument are becoming more powerful and robust for field deployment scenarios.[Citation45–47] For a great review in the detection of synthetic opioids as well as others not related to the fentanyls using various analytical approaches, the reader is invited to examine the article by Gerace et al., which provides a general overview of LC-MS and GC-MS methods that have proven useful in the field[Citation42] as well as one published by Professor Olaf Drummer at Monash University in Australia that has nicely collected reports dealing with fatalities involving these substances and analytical methods involved in their analyses.[Citation44] Lastly, for a detailed, lengthy yet thorough overview on the fentanyl class of compounds, that includes their history, organic and medicinal chemistry, biology, as well as their central role in cases involving their illicit use and distribution, the reader is invited to read an outstanding book by the RAND Corporation.[Citation48]
GC-MS offers an attractive alternative to LC-MS as an analytical method for the analysis and detection of this class of compounds regardless of the matrix they are in (environmental or biological). As alluded to previously, one of such advantages is the cost associated not only with the acquisition of but also operational and maintenance of GC-MS instrumentation, leading to its more frequent prevalence in most analytical laboratories over LC-MS instrumentation. A second one is that GC-MS, operated in electron ionization mode at 70 eV, produce mass spectra that are comparable and reproducible when collected by instruments from different vendors, allowing for the development of mass spectral libraries and structure determination for new and unknown compounds. A third one is the capability of using a portable unit that can be deployed in various areas where an exposure to these toxic substances has occurred. The GC-MS instrument structural components and operational demands allow for engineered miniaturization of these systems, an important quality still lacking in current LC-MS technologies.
One of the advantages of applying LC-MS for the detection of these synthetic opioids, and one that cannot be exploited by GC-MS methods, is the unparalleled use of the basic nitrogen in their structure to generate a protonated form of the molecule (in the case of fentanyl, a hydrochloride, citric or oxalate salt) which itself enhances the detection of the opioid by various LC-based methods. In looking at the structures of established alkaloids for which highly sensitive LC-MS methods exist (e.g., morphine, heroin), one can see that these compounds, just like the fentanyls, share a tertiary nitrogen that can be easily protonated by the acid (e.g., formic acid) used in the mobile phase. Unfortunately for GC-MS methods, the great majority of fentanyl samples found in drug seizures and in overdose victims are encountered in this form (i.e., salt form), meaning that a direct analysis is not initially possible. Therefore, conversion of the salt into the fentanyl free base is necessary when seeking the detection of the opioid by GC-MS. Employing protocols that involve this conversion of the fentanyl compound into its free base has allowed for GC-MS techniques to successfully detect the opioid at levels relevant to fatal overdose cases featuring values between 0.2–45 ng.L−1 for blood and 2.2–450 ng.L−1 for urine, two of the most common tissues collected in all the cases discussed in this review. provides a scheme with the goal of capturing the important parts of a sample preparation steps when dealing with the analysis of fentanyl and related opioids using GC-MS. The roadmap presented in was created using the common techniques used for fentanyl analyses described on the papers that are covered in this review. For more in depth description of each, the reader is invited to read the relevant tables for each fentanyl in the sections that follow. Thus, starts out with the nature of the sample to be analyzed, the encountered cases involve biological fluids such as blood (from different tissues, but in most cases femoral and heart blood), plasma and urine. The aqueous matrix samples noted in the box refer to samples that originate from the cleaning of a given object found during a drug seizure or associated with a lethal overdose (e.g., needles, syringes, fentanyl dermal patch or capsules). At this point, the samples may be screened for other common drugs of abuse such as cocaine, heroin or amphetamines employing radioimmunological assays (RIA) or Enzyme-linked Immunosorbent Assays (ELISA) that make use of antibodies to positively identify these drugs including fentanyls themselves.[Citation49,Citation50] With regards to these biochemical assays the reader must be aware that despite their use in detecting fentanyl their specificity for this opioid is not optimal and often provide false positive results (vide infra).[Citation51,Citation52] For example, LSD is well-known to show cross-reactivity with fentanyl and closely related analogs of this opioid.[Citation53] Continuing with the sample preparation scheme, the sample is initially treated with an internal standard which often is a deuterated version of fentanyl such as fentanyl-D3 or -D5, or an analog of the opioid with similar structure and GC response such as N-phenyl-N-[1-(3-phenylpropyl)-4-piperidinyl]propanamide. It is at this point that the sample is basified and taken through a solid-phase extraction (SPE) path (, Path A) or standard extraction (, Path B). Regarding Path A, the SPE cleaning step is highly useful when dealing with very complex matrices such as blood and plasma and provides after the extraction a sample that is suitable for direct analysis by GC-MS after proper solvent exchange. Path B is the most commonly encountered one in the literature and it is very efficient at extracting fentanyl and congeners from less complex matrices like urine or aqueous samples obtained from objects contaminated with the opioid. In this path, the basified sample is treated with 1-chlorobutane as originally described by Foerster and Mason in their landmark fentanyl extraction paper in 1974[Citation54] or treatment with another organic solvent like 2-methylbutane. Once the extraction is carried out and the organic phase isolated, this one is acidified (usually with sulfuric acid) to convert the opioid into its salt form. Once the salt formation has been accomplished, a second extraction is carried out and the aqueous phase is collected. This is done presumably to add another layer of sample clean-up in the protocol specially when dealing with matrices like blood and plasma that often contain innumerable compounds that can obfuscate the opioid’s signal during the GC analysis. After the acid extraction step, the aqueous phase is once again basified and extracted with an organic solvent that after evaporation and reconstitution in another organic solvent will now contain the fentanyl free base ready for injection in the GC instrument.
Figure 2. General protocol for sample preparation for the GC-MS analysis of fentanyl and related opioids in biological and environmental* samples. *By environmental it is meant that the sample originated from the environment where the fentanyl was collected and this may include wipe samples from surfaces or washed samples from objects associated with an overdose case. EtOAc = ethyl acetate; MeOH = methanol.
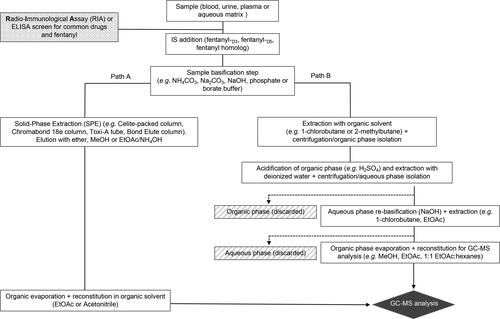
An additional part of some protocols that will be described in this review, and ones that have involved the analysis of these nitrogenous opioids, is the use of SurfasilTM siliconizing fluid. The purpose of using SurfasilTM is to block polar groups exposed on glass surfaces such as those on glass autosampler vials used during the preparation and analysis of samples that results in nonspecific binding of the opioid and therefore loss of analyte in the solution for proper detection.[Citation55] What follows in the next set of section of this review is the discussion of research work that has mainly involved the use of GC-MS for the analysis of fentanyls and fentanyl-related synthetic opioids.
Methods
For the assembly of this review, each fentanyl discussed herein was searched in conjunction with GC-MS techniques from June-October 2020. Systematic searches on the Internet were conducted in English, using “fentanyl” and “GC-MS and/or GCMS” in the Google search engine. In addition the same search terms were used when collecting research work in PubMed and ISI Web of Knowledge (ISI-WK). For the “fentanyl” and “GC-MS” combination yielded the most literature hits (184 in PubMed and 105 in ISI-WK) that included work with other fentanyls covered and not covered in this review. Lastly, the same search criteria was applied to SciFinderTM, a Chemical Abstracts database, using the Research Topic option and this yielded a total of 256 references, most of which matched the ones found previously by PubMed and ISI-WK. However, some of the hits offered by the SciFinder search include articles where GC-MS is mentioned in the document but not necessarily employed as one or main technique for the analysis of the given fentanyl, thus eliminating these from consideration.
Fentanyl: The flagship synthetic opioid
The parent molecule fentanyl, N-(1-phenylethylpiperidin-4-yl)-N-phenylpropionamide, is the flagship compound of this class of synthetic opioids exhibiting a potency of 50 times more of that showcased by the gold standard in the management of pain in the clinic, morphine. Fentanyl, synthesized in 1960 by Paul Adriaan Janssen in Belgium, was one of the first powerful narcotics belonging to this class of compounds featuring a phenylamide moiety in a 1,4-relationship to the ring nitrogen in the biologically functional piperidine moiety previously found in the pethidine nucleus (). Early testing of fentanyl confirmed its ability to cause rapid anesthetic effects while more importantly causing minimal effects on the cardiovascular system of the patient.[Citation56,Citation57] Due to its potency and ease of manufacture, fentanyl has been involved in a number of deaths involving its illicit trafficking and use in Australia[Citation33] and within the United States[Citation58,Citation59] in Michigan,[Citation32] New Mexico,[Citation60] Florida and Ohio,[Citation61,Citation62] New York[Citation63] and Rhode Island[Citation64] just to name a few. The original Janssen synthesis of fentanyl featured five steps and it would provide a blueprint to be followed by subsequent synthetic efforts that methodically refined or improved existing yields in this route, making use of the commercial availability of new intermediates or more efficient methodologies that appeared in the literature over time. Currently one of the most visible ones, as it is openly published online, is what has become known as the Siegfried method that is applicable to the synthesis of fentanyl and analogs thereof (). The Siegfried method reduces the number of steps from five to four as it proposes the use of the N-phenyethyl-4-piperidone as the starting material instead of the N-benzyl-4-piperidone, employed originally by Janssen.[Citation65] This benzyl → phenylethyl group conversion basically eliminates two steps from the original synthesis, the first one being the removal of the benzyl group using hydrogenolysis and installation of the phenylethyl side chain via alkylation (). Other synthetic approaches to fentanyl have appeared, including one from our own laboratory that involves a total of three steps ().[Citation36] It is important for the reader to be generally aware of the different synthetic pathways to this class of opioids, industrially, academically as well as clandestine-based ones, as their analysis by various analytical methods has opened the new sub-division of chemical attribution signature analysis in the field of chemical forensics. In this field, a deep analysis by various technologies, mainly comprising GC-MS and LC-MS, is done on a given fentanyl sample and particular attention is given to by-products and unreacted intermediates that, when considered together, can provide a glimpse of the overall synthetic route employed for its production. Therefore, the ability to attribute a specific fentanyl sample to a given synthetic route provides important intelligence information to law enforcement and drug-enforcement agencies particularly with samples that have a clandestine origin.[Citation66] To this end, the development of algorithms geared toward machine-learning for the establishment of analyses capable of linking a sample to its synthetic pathway have been foci of intense research efforts.[Citation67–73] This emerging field, rapidly developing in analytical chemistry, has found significant application particularly in the forensic analysis of samples associated not only with fentanyls but other chemical warfare agents.[Citation74–78] Throughout this review, great effort has been placed in providing a brief introduction of the synthetic methods used to construct the discussed fentanyls as well as clear schematics that describe these pathways.
Figure 3. Some of the synthetic pathways to fentanyl including the original one published by Janssen. Each arrow in the scheme represents a step in the synthetic route. Janssen’s route makes use of the benzylated piperidone material and its presence adds two additional steps, debenzylation followed by alkylation with (2-chloroethyl)benzene, relative to the Siegfried method[Citation65] and the one published by Valdez et al.[Citation36]
![Figure 3. Some of the synthetic pathways to fentanyl including the original one published by Janssen. Each arrow in the scheme represents a step in the synthetic route. Janssen’s route makes use of the benzylated piperidone material and its presence adds two additional steps, debenzylation followed by alkylation with (2-chloroethyl)benzene, relative to the Siegfried method[Citation65] and the one published by Valdez et al.[Citation36]](/cms/asset/d4e3a183-add6-4916-a22e-f0f663325a86/batc_a_1927668_f0003_b.jpg)
Fentanyl is a schedule II compound that exists as a salt (hydrochloride or citric acid as it is commonly employed in the clinic) and akin to the other members of this family, all GC-MS analyses discussed in this review will involve a basic work-up step () in order to generate the free base of the opioid. One of the first reports involving the use of GC-MS as a means for the detection and identification of fentanyl appeared in 1981 from the Department of Anesthesiology at the University of Texas Medical School[Citation79] (). In this paper, Lin et al. describe the detection of the opioid in fentanyl-treated mongrel dogs. The analysis of the plasma extracted from the animals initially involved the addition of the internal standard (IS) fentanyl-D3 that was synthesized in their laboratory. After the IS addition, the spiked plasma was basified using ammonium carbonate and extracted with ethyl acetate. After the extracts were combined and evaporated to dryness, they were reconstituted in ethyl acetate and directly analyzed by GC-MS using single-ion monitoring (SIM) mode. Early reports such as this one on the analysis of fentanyl by GC-MS in biological fluids like blood, plasma and urine featured a basic extraction step as an important piece in the overall sample preparation. This is because as its free base, fentanyl can be detected by GC-MS using a number of hyphenated methods such as GC-NPD (nitrogen-phosphorus detection) that favorably exploits the presence of two nitrogen atoms in the molecule for specific detection. Another report in 1986 by Moore et al.[Citation80] from the Special Testing Laboratory at the Drug Enforcement Administration in Virginia, describes the analysis of fentanyl in sugar-adulterated samples using GC-MS using electron capture detection (ECD) (). Their developed method involved the chemical modification of fentanyl using heptafluorobutyric anhydride (HFBA) and 4-dimethylaminopyridine (DMAP) at 75 °C to presumably give two neutral, fluorovinyl-derivatized products that were extracted using isooctane after a basic work-up using 1 N Na2CO3 and solid-phase extraction (SPE) clean up over Celite® with diethyl ether. No limits of detection (LOD) were reported for this approach, but a minimum detectable quantity (MDQ) of 20–40 pg of the derivatized fentanyl from an original 100 µg of fentanyl starting quantity was provided. It is interesting to note that although the authors mention that the fluorovinyl products form during the derivatization reaction prior to GC-MS analysis, it is very likely that these species form in the instrument’s injection port at much higher temperatures than 75 °C.[Citation90] In another paper, this hyphenated GC method was successfully applied in the initial screening of fentanyl in blood samples by the Office of the Medical Examiner in Baltimore, MD.[Citation81] After the detection of nitrogen-containing species in the preliminary GC-MS run, the nature of the opioid was confirmed employing GC-MS using SIM. In this paper, the authors use an interesting internal standard known as R38527 which is an alfentanil analog. Briefly, the authors spiked a blood sample with R38527 and partitioned the mixture between buffer and 1-chlorobutane. After extracting the fentanyl into the 1-chlorobutane layer, this one is acidified and re-extracted with sulfuric acid. As mentioned above, an additional round of acidification followed by re-basification step is often added to a protocol to further aid in the removal of various interferences present in blood and plasma samples and thus enhancing the extraction of the opioid in every step. After re-basification of the aqueous phase and extraction with 1-chlorobutane, the sample was ready for GC-MS analysis. In 1993, Laganière et al. from the Therapeutic Products Directorate in Canada published a method for the detection of fentanyl in human plasma that involved the extraction of the opioid using 1-chlorobutane as the organic phase after basification of the aqueous phase with a 1 M NaOH solution.[Citation82] Subsequent solvent exchange for a 1:1 hexane/ethanol mixture was then used for the GC injections. A 71% recovery of the spiked fentanyl is reported equating to a 3.5 µg.L−1 concentration for the method’s performance (). Two years later, an interesting report by Kingsbury et al. from the University of Connecticut School of Medicine was published in 1995 and it involved the use of Toxi-A tubes for the SPE extraction of fentanyl citrate from used syringes.[Citation83] The Toxi-A tube SPE extraction provides fentanyl as the free base via basic neutralization and thus it can be directly analyzed by GC-MS. The authors report a method’s LOD for fentanyl of 0.4 mg.L−1. Another interesting report appeared the following year by Sachs et al. at the University of Munich, dealing with the analysis and detection of fentanyl in hair. The hair samples were collected from patients who have been exposed to the opioid in a controlled manner, thus providing the authors with the total amount of the drug administered to the patient before the analysis was performed.[Citation84] Their protocol involved the milling of the patient’s hair and extraction with a basic buffer under mild heating. The combined aqueous washes were then taken through a SPE method using a 3:1 dichloromethane:methanol (DCM:MeOH) gradient that was collected, evaporated and reconstituted in methanol for analysis by GC-MS (). The following year, a paper by the Kronstand group at the Department of Forensic Chemistry at University Hospital in Linköping, Sweden reported their analysis of fentanyl in blood and urine samples from nine drug-addict fatalities.[Citation85] Using fentanyl-D5 as an internal standard and employing isooctane as the organic extracting solvent from the biological matrices, the mean fentanyl concentrations for blood and urine were found to be 9.97 and 2.08 ng g−1, respectively, values that typically found in overdose victims. In 2000, the Los Angeles County Department of Coroner published their studies on the detection of fentanyl in postmortem samples arising from the use of the Duragesic® transdermal patch.[Citation86] In this report, various tissues samples that included: blood (femoral and heart), vitreous humor, bile, spleen, kidney and lungs were analyzed by GC-MS using the SIM mode (m/z = 245, 146, 189). The analysts found fentanyl concentration values in all examined tissues from different decedents that ranged from 1.8 to 895 µg L−1 in tissues like blood, vitreous humor, bile and urine, while it ranged from 3.5 to 613 µg kg−1 in tissues like liver, spleen, kidney and lung. In this case, the use of another organic solvent extract for the biological sample preparation step is worth mentioning and that was 2-methylbutane. Another report involving the GC-MS analysis of fentanyl appeared in 2003 from the Virginia Division of Forensic Science and the Department of Justice Sciences at the University of Alabama at Birmingham.[Citation87] In this report, the authors analyze blood among other biological tissues collected from 23 overdose fatalities with a sample preparation protocol that included precipitation with acetonitrile and SPE extraction with ethyl acetate:ammonium hydroxide (98:2). The protocol’s lowest limit of quantitation (LOQ) was reported at 1.0 µg L−1 and the fentanyl extraction recovery was reported as 88%. Thus, in 2008, Malkawi et al. from the College of Pharmacy at the University of Kentucky similarly accomplished the detection of fentanyl in rabbit plasma samples after their extraction using a 1:1 acetontrile:1-chlorobutane mixture.[Citation88] No need for basification of the mixture was reported in this protocol, due to the fact that fentanyl free base was employed in the studies from the start. After evaporation of the extracted aliquot the residue was reconstituted in methanol and analyzed by GC-MS. The reported LOD of fentanyl for this protocol was determined to be 1 ng.mL−1. An interesting component of the protocol is that it is one of the only ones that uses SurfasilTM to block any absorption of fentanyl onto the surfaces of the glass vials involved in the analysis besides the work described by Laganière et al.[Citation82] In 2011 the Laboratorio Antidoping in Rome, Italy published a GC-MS method for the rapid screening of fentanyl among other opiates such as morphine and cocaine. The method describes the detection and quantification of these substances after a liquid-liquid extraction under alkaline conditions (pH 9) using Na2CO3. Their total chromatographic separation run is conveniently short (6 minutes) which makes the protocol highly appealing for its rapidity.[Citation89] The authors report the LOD for fentanyl to be 5 ng.mL−1 (). In the same year, the laboratory published reported the detection of fentanyl by GC-MS along with its metabolic product norfentanyl in urine samples.[Citation91] The protocol involves the liquid-liquid extraction of fentanyl and norfentanyl from urine using 2 M NaOH using tert-butyl methyl ether (TBME) as the organic extractant. For the specific detection of norfentanyl, a derivatization using pentafluoropropionic anhydride (PFPA) was performed to improve the detection of this primary metabolic product of fentanyl. The determined LOD values for fentanyl and its metabolite (as its pentafluorobutyryl derivative) were reported to be 0.08 ng.mL−1 (). Furthermore, the method was tested for its effectiveness in real samples dealing with individuals who have received therapeutic doses of the opioid as well as an individual whose death was attributed to fentanyl overdose. Another report surfaced in 2011 by Bravo et al. from the Universidad Arturo Prat in Chile, describing their work on the analysis of fentanyl in blood and plasma samples.[Citation92] Their protocol involved the use of a slightly acidic (pH 6) buffer for the homogenization of the samples and their SPE elution with a basic solvent mixture composed of DCM:IPA:NH4OH (78:20:2) where IPA is isopropyl alcohol. After evaporation of the volatiles, the residue was taken up in ethyl acetate and analyzed by GC-MS (). More recent reports dealing with the analysis of fentanyls in biological samples have utilized the method of basification followed by organic extraction successfully in many scenarios and case reports. In 2014, McIntyre et al. published their work on ante-mortem and postmortem analysis of fentanyl using a SIM mode GC-MS.[Citation93] Briefly, the method involved the use of ammonium hydroxide to render the diluted samples basic and the use of 1-chlorobutane to extract the fentanyl free base. After collecting the 1-chlorobutane layer, an acidic extraction step (using HCl) was included to regenerate the fentanyl hydrochloride salt and transfer the opioid into the aqueous layer. The additional acidification step was done to remove as much as possible any interferences in the blood matrix that might have been extracted along with the fentanyl into the organic phase. Finally, the acidic aqueous phase was neutralized with ammonium hydroxide and a second extraction with 1-chlorobutane was performed to yield a much cleaner sample. After evaporation and reconstitution in ethyl acetate, the samples were analyzed by GC-MS (). In 2015, an effort involving several laboratories that included the University of Colorado, Colorado Springs, the Beth-El College in Colorado Springs and the El Paso County Coroner’s Office in Colorado Springs published their study on the stability of fentanyl in synthetic urine making use of a dispersed liquid-liquid microextraction (DLLM) approach.[Citation94] In their work the authors compare the extraction efficiencies of DLLM to be comparable to those obtained when using traditional liquid-liquid (LLE) and SPE extraction protocols. Their stability studies on fentanyl involved a 12-week period of assessment, and the results show the high efficiency of the approach with recovery values for an initial fentanyl concentration of 1000 ng.mL−1, as well as the internal standard, fentanyl-D5, of 836 ng.mL−1 (LLE method), 870 ng.mL−1 (SPE method) and 841 ng.mL−1 for their highlighted DLLE method (). It will become evident in the upcoming sections of this review that given fentanyl’s chemical stability toward acids and bases and the structural similarities shared by members of this opioid family that the sample preparation steps strongly resemble the ones described on this section. Nevertheless, one must be cautious when deciding the pH of the solution employed for basic work-up in certain cases where the fentanyl analog might possess labile functionalities such as methyl esters (i.e., carfentanil and remifentanil) and also the pH of the solution employed for the acid work-up step that might cause the degradation of acid-sensitive moieties in the opioid like methyl ethers (i.e., alfentanil and sufentanil) and the furan ring (i.e., furanylfentanyl).
Table 1. Summary of discussed GC-MS analyses for fentanyl.
Acetylfentanil
Also known chemically as N-(1-phenylethylpiperidin-4-yl)-N-phenylacetamide and structurally simpler than fentanyl itself, acetylfentanyl is one carbon away removed from fentanyl (). Making its appearance in 2013 acetylfentanyl quickly became identified as the main causative agent in ten overdosing fatalities occurring between March 7 and April 11, 2013 in Rhode Island.[Citation52,Citation95] In the medical field, acetylfentanyl is not used as an analgesic despite exhibiting a potency 15 times more than that one of morphine.[Citation9] It was not until 2015, that the Drug Enforcement Agency (DEA) officially designated acetylfentanyl as a Schedule I substance. The synthesis of acetylfentanyl follows the same process as for fentanyl and simple analogs[Citation9,Citation96] with the replacement of a the propionyl chloride (or propionic anhydride) by acetyl chloride (or acetic anhydride) as the acylating agent for 4-ANPP (). Although not evaluated at the time, Janssen published the synthesis of acetylfentanyl in a patent in 1965 when his team was deeply involved in the production of fentanyl analogs.[Citation97] It is believed that due to the lack of monitoring for acetylfentanyl in lethal cases before 2013 by clinical laboratories the number of actual fatalities stemming from its use might be underappreciated.[Citation9] Acetylfentanyl’s potency coupled to its ease of production has turned into a potential threat as an emerging opioid as more deaths became associated with it in Sweden[Citation98] and the US.[Citation99] For an acetylfentanyl-specific review describing its synthesis, pharmacological activity, prevalence in the illicit market, and its legal status, the reader is invited to examine an excellent review by Katselou et al.[Citation100]
Protocols involving the analysis and detection of acetylfentanyl by GC-MS means can expected to be similar in structure to those employed for fentanyl. In 2016, a case report by McIntyre et al from the County of San Diego Medical Coroner’s Office described their work involving the distribution of acetylfentanyl in a postmortem setting.[Citation101] Collection points for the postmortem analysis included peripheral (iliac veins) and central (heart) blood, liver tissue, vitreous humor (eye) and urine. Thus, the collected samples were diluted with water and basified using concentrated ammonium hydroxide. Following the pH adjustment, the aqueous mixture was extracted with 1-chlorobutane. The organic layer (top layer) was then removed, mixed with 1 N hydrochloric acid, discarded and the aqueous phase re-basified and extracted once again with 1-chlorobutane to obtain the free base of the opioid. Once the 1-chlorobutane was removed with a stream of nitrogen, the residue was reconstituted in ethyl acetate and analyzed by GC-MS using the SIM (specific ion monitoring) mode (). The following year, the same group reported their findings on the analysis of postmortem tissues from a 40-year old victim of overdose by a combination of butyrylfentanyl, acetylfentanyl and cocaine.[Citation106] The details of this specific case report, including the sample preparation and detection of acetylfentanyl are presented in (vide infra). The same year, Melent’ev et al. reported their findings on the analysis of acetylfentanyl in urine samples by GC-MS. In addition to the direct detection of the acetylfentanyl, the authors found acylated versions of the opioid when the derivatization of the samples were done using acetic anhydride, propionic anhydride among others.[Citation118] Detection of the modified acetylfentanyl itself along with the metabolic products (that do need acylation for GC-MS detection) was accomplished. Due to the low likelihood that the parent opioid would undergo acylation at the aromatic region of the phenylethyl side chain even at elevated temperatures (80–90 °C), it is presumed that the observed acylation of the actual opioid occurred at the GC instrument’s port. In 2016, a report from the chief medical examiner in Oklahoma by Fort et al. described two case reports involving acetylfentanyl.[Citation102] In this report, the forensic chemists employ a two stage screening, the first one involves the use of an ELISA test to screen eight common, different drug classes in the victim’s blood while the second one involves the use the alkaline drug screen where the use of GC-MS becomes central for opioid detection. The postmortem collection points from the two individuals included heart blood, femoral blood, liver, brain, vitreous humor, and urine. The alkaline screen involved the extraction of the acetylfentanyl from aqueous solutions of these collection points using 1-chlorobutane and concentrated ammonium hydroxide.[Citation102] Again, it is this basic work up that results in the overall production of the acetylfentanyl in its free base form that is detectable by GC-MS using SIM mode (). Another report appearing the same year was by Cunningham et al. in which the authors presented their detailed analysis of acetylfentanyl in one fatal intoxication case. Although their work and the results presented on the various tissue concentrations of acetylfentanyl were obtained using LC-MS/MS methods (), the confirmation of acetylfentanyl in these samples was initially established by GC-MS by using an initial SPE employing Toxitube A cartridges followed by the alkaline screening protocol to obtain the opioid in its free base form.[Citation103] One last report that appeared in 2016 originated out of Kumamoto University in Japan by Yonemitsu et al. In their report, the authors utilize GC-MS to identify acetylfentanyl in a fatal intoxication case along with contaminated paraphernalia that included a plastic bag and a used syringe.[Citation104] The extraction protocol for the analyzed tissues employed in this lab involved the use of an EXtrelut NT3 column for the SPE cleaning of the opioid from the aqueous mixture, eluting it with ethyl acetate. After solvent exchange with methanol, the sample was directly analyzed by GC-MS (). In 2017, a paper by Takase et al. from the Department of Legal Medicine at the Yamaguchi University in Japan reported the use of GC-MS for detecting acetylfentanyl in blood from the heart of an insufflation overdose victim.[Citation105] The sample preparation included the direct elution of the sample through a hydrophilic-lipophilic balanced (HLB) column, which is a reverse-phased column and retains polar compounds well, with 5% MeOH:H2O then only MeOH to obtain a fraction (in MeOH) that was then injected in the GC instrument. Another report that appeared in this year was the one by Dwyer et al. from the Allegheny County Office of the Medical Examiner in Pennsylvania.[Citation99] In this study, the authors initially employ ELISA as a means of screening drugs in blood samples from 41 overdose cases in Pennsylvania from 2015 to 2016. The authors use alkaline extractions of the blood samples and these were concentrated and prepared for GC-MS analysis using the SIM mode. The authors report the range, median, mean, and standard deviation of blood acetylfentanyl concentrations over all 41 cases to be: 0.13–2100 ng mL−1, 11 ng mL−1, 169.3 ng mL−1, and 405.3 ng mL−1, respectively.
Table 2. Summary of discussed GC-MS analyses for acetylfentanyl.
Table 3. Highlighted list of GC-MS analyses of other fentanyls discussed in this review.
GC-MS analysis of other commonly encountered fentanyl-related opioids
Carfentanil
Synthesized by Janssen pharmaceuticals in 1976, carfentanil (methyl 1-(2-phenylethyl)-4-(N-propanylanilino)piperidine-4-carboxylate) became an analog that exceeded the power of the parent drug by 200-fold and 1000 times more of that showcased by morphine ().[Citation119] Although commonly associated with its usage as a tranquilizer for large wildlife in the veterinary sciences,[Citation120–123] carfentanil is more infamous for its confirmed use during the Moscow Dubrovka Theater crisis in 2002[Citation28–30] and its growing potential as a weapon of mass destruction.[Citation124] Moreover, this powerful opioid has recently become involved in various lethal overdose cases worldwide indicating it spread into the illicit drug market.[Citation116,Citation125,Citation126] The opioid’s potency make it a much more feared threat than fentanyl itself since larger number of pills can be produced with it than with fentanyl to produce the same psychoactive or lethal effects.[Citation127–129] Added to this is the well-founded conjecture that illicit distributors of the drug do not have the time and resources to make sure the correct aliquot of carfentanil is in each pill to produce the psychoactive effects in an individual without causing any of the toxic side effects. As the appearance of this more powerful fentanyl provided a quick awareness on this issue, methods for its detection and proper identification by analytical means started to develop quite rapidly. Structurally, carfentanil is an achiral molecule due to a plane of symmetry running through the middle of the piperidine ring. The only difference between fentanyl and carfentanil is the presence of a methyl ester moiety at the C1 position that effectively raises the difficulty and length of the synthetic route for its production (). The synthesis of carfentanil was initially published by the Janssen group in 1976 and made use of N-phenylethyl-4-piperidone as the starting material (). Reaction of the ketone moiety with aniline and cyanide in the presence of an acid, usually acetic acid, install a quaternary carbon center bearing a cyanoamino substituent in what is known as the Strecker reaction.[Citation130] Aside from yielding Carfentanil in an overall 1% yield, the method introduced the Strecker reaction as a viable way of constructing the opioid skeleton that was later refined by the Taber group at the University of Delaware in their synthesis of the benzylated analog of Carfentanil with an overall yield of 60%[Citation131] and later used by the Edgewood Research Center in their own synthesis of Carfentanil ().[Citation132] Another important route that appeared in 1990 was one involving an hydantoin intermediate from the α-amino nitrile described by the Glaxo Research Laboratory in North Carolina ().[Citation133] The synthesis of the benzylated analog of Carfentanil using the hydantoin route was accomplished in 39% yield. Further modifications of the resulting molecule produced the carfentanil molecule along with the possibility of using the route to gain access to other analogs not only of carfetanil but opioids sharing this common structural motif. An interesting and approach to the carfentanil nucleus was developed by the Deprez and Laconde groups at the Université Lille Nord de France, and their work included the synthesis of remifentanil which is discussed more in detail in the sections below.[Citation134]
Protocols involving the analysis and detection of carfentanil by GC-MS means can expected to be similar in anatomy to those employed for fentanyl and acetylfentanyl. Thus, back in 2012 when the Defence Science and Technology Laboratory (DSTL) in England published their analysis of the clothing and plasma samples belonging to three survivors (two males and a female) of the Moscow Dubrovka Theater siege in 2002, they made use of GC-MS as part of the overall analysis of the sample that also included LC-MS.[Citation31] More specifically, pieces of clothing from the victims were copiously washed with deionized water and the collected fractions basified with sodium hydroxide (1 M concentration, pH ∼ 13). Extraction of the basic, aqueous layer was done using dichloromethane and directly analyzed by GC-MS. For the plasma samples obtained, these were initially treated with sodium hydroxide and extracted with 1-chlorobutane. Interestingly in this case, the researchers opted for evaporating the sample to dryness and solvent reuptake the residue in toluene for direct injection and analysis by tandem mass spectrometry (GC-MS-MS) without an additional acidification/rebasification step. Using GC-MS, scientists at DSTL were able to determine unambiguously that a mixture of carfentanil and remifentanil was employed during the crisis by the Russian special forces that eventually eliminated the threat but unfortunately resulted in the deaths of innocent civilian by-standers.[Citation28] Another paper describing the use of GC-MS for the detection and identification of carfentanil was published by Swanson et al. from the Medical Examiner Department in Tampa, Florida in 2017.[Citation116] The work describes the analysis of tissues collected postmortem from overdosing decedents using GC-MS-SIM. The tissues included heart blood, vitreous humor and urine. The sample preparation differs significantly from the one published by DSTL in 2012, beginning with the basification of the biological sample with saturated borate buffer (pH is not reported but presumably is >10 for this process) and extraction with a mixture of organic solvents (78:20:2 toluene/hexanes/isoamyl alcohol (THIA)) that serves to trap the opioid in its basic form. A second step is added at this point where the mixture is acidified with sulfuric acid and back-extracted into an aqueous layer. After the water fraction is re-basified and extracted with ethyl acetate, the organic is evaporated and dissolved in ethyl acetate for GC-MS analysis. The authors reported concentrations of carfentanil in the blood samples down to 0.12 ng.mL−1 in one of the decedents. In addition to carfentanil, the authors also report finding furanylfentanyl in one of the victims’ blood samples (0.34 ng.mL−1).[Citation116]
Sufentanil
Synthesized by Janssen pharmaceuticals in 1976[Citation135,Citation136], sufentanil became an analog that exceeded the power of the parent drug by 10-fold and 500 times more of that showcased by morphine[Citation137] () due to its larger binding affinity to the µ-opioid receptor.[Citation138] Structurally, sufentanil just like fentanyl and carfentanil is an achiral molecule due to the plane of symmetry running through the middle of the piperidine ring. Its synthesis may be accomplished using a similar route to the one outlined for carfentanil above where the initial benzyl-protected 4-piperidone starting material undergoes a Strecker reaction to yield the crucial phenylaminecyano-containing intermediate (). Synthetic manipulations on this intermediate involve the conversion of the cyano moiety into the methoxymethyl unit in Sufentanil. As it was in the case for the fentanyl synthesis, Janssen carried the piperidine nitrogen protected with the benzyl group throughout the whole sequence, removing it at the very end to install the unique thiophene-containing unit. Another synthetic pathway, disclosed in 1999, involved the intermediacy of an epoxide intermediate that can be opened with aniline to install the key methoxymethyl and amine moieties in Sufentanil in one step ().[Citation139] The authors use trimethylsulfonium iodide (Me3S+.I-) to convert the 4-substituted piperidone into their key epoxide[Citation140] that can then be opened with aniline to give a 1,2-aminoalcohol intermediate that can then be elaborated further to give sufentanil. However, the overall yield for sufentanil is ∼2%, and the main reason for this is that the key epoxide opening step is not efficient and provides a mixture of isomers as the regiospecificity of this step is poor, yielding the right intermediate that can be carried forward toward sufentanil obtained in 6% yield.
As we have seen in previous report (vide supra), sufentanil can be detected by GC-MS means and some of the previous papers have conducted studies that included additional fentanyls. As sufentanil is a neutral molecule like fentanyl on its free base state, most of the sample preparation protocols for fentanyl are applicable to the analysis of sufentanil in biological matrices. One of the first reports appeared in 1985 by Weldon et al. from the Department of Anesthesiology at the University of Arizona, Tucson and it dealt with the analysis of sufentanil in the human serum of an individual who had been injected with the opioid at a 1.5 µg.kg−1 concentration.[Citation107] The serum was obtained after centrifugation of the blood samples (5 mL) that were collected over a day. In this report, the authors used a modified version of an extraction method for fentanyl previously reported.[Citation141] Briefly, the serum was treated with fentanyl as an internal standard, basified with 2 N NaOH and partitioned between hexanes:MeOH (19:1). After centrifugation, the top layer was acidified with 0.1 N HCl, followed by another round of extraction with hexanes:MeOH (19:1). The resulting organic phase from this protocol was evaporated and taken up in toluene for analysis by GC-MS. The detection limit for the developed method was in the range of 30–50 pg.mL−1. Another report surfaced four years later by Ferslew et al. at East Tennessee University that involved the determination of sufentanil in postmortem analyses of tissues that included blood, urine, vitreous humor, liver and kidney from a self-injection of sufentanil and midazolam.[Citation142] In this work, the basification of the tissues homogenates is accomplished with 1 N NaOH and the exact organic extraction protocol as the one described by Weldon et al. is followed using hexanes:MeOH (19:1), however, no acidification intermediate step is mentioned and after the organic phase is collected and evaporated, it is taken up in ethyl acetate for GC-MS analysis. This method, using fentanyl as an internal standard, was able to detect sufentanil levels down in the range between 1.1 and 5.5 ng.mL−1. Another important report dealing with the GC-MS analysis of sufentanil emerged in 1993 when the synthetic opioid was detected in the blood and the cerebrospinal fluid of sufentanil-treated dogs.[Citation108] The extraction protocol described by Stevens et al. at the Medical College of Wisconsin is identical to the one established by Weldon et al.[Citation107] and Gillespie et al.,[Citation141] including the acidification step which was done presumably to obtain cleaner samples for the analysis. The study involved a time course evaluation of the levels of sufentanil in the fluids described above, with the rapid, maximum concentration of sufentanil (t = 6 min.) found in the lumbar region of the spinal cord (at 57 ng.mL−1). The work focused on these specific tissues that are normally protected by the blood brain barrier (BBB) that severely limits the ability of xenobiotics to access the central nervous system (CNS) unless they possess the right balance of hydrophobicity in their structural surface as well as avoiding export proteins like P-glycoprotein.[Citation143] It is well-known that sufentanil is a more hydrophobic than fentanyl and thus able to cross and even reside within lipid membranes including those of the BBB.[Citation144] Thus, when it comes to targeting the CNS, the effects of sufentanil are heightened relative to those of morphine and fentanyl. As a side note to the reader, it is interesting to note that morphine does not cross the BBB due to its hydrophilicity under physiological conditions as well as serving as a substrate for the P-glycoprotein export protein whose main function is to expel xenobiotics once they are lodged in the lipid bilayer membrane comprising the BBB, whereas sufentanil has high lipophilicity and is not a known substrate for the P-glycoprotein export protein.[Citation145] Two additional reports surfaced in 2004 from the van Nimmen group at the Department of Occupational, Environmental and Insurance Medicine at the Katholieke Universiteit in Leuven, Belgium. Their first report involved the analysis of urine collected from opioid-exposed workers and the panel of analytes under investigation included fentanyl, sufentanil and alfentanil.[Citation110] In this work, the urine samples, after basification with NaOH, underwent SPE clean-up using am EXtrelut NT1 column. After the extracts were evaporated, they were reconstituted in MeOH and analyzed by GC-MS using the penta-deuterated analogs for each opioid as internal standards. The method’s LOD was determined to be 2.5 pg.mL−1 for fentanyl, 2.5 pg.mL−1 for sufentanil and 7.5 pg mL−1 for alfentanil. Furthermore, the nor-fentanyl metabolic products were also detected (via the perfluorinated derivatives) with a LOD of < 50 pg.mL−1 of urine. The group’s second paper dealt with the detection of the same three opioids (fentanyl, sufentanil and alfentanil) in air and surface contamination wipes.[Citation111] For these experiments, the authors use Institute of Occupational Medicine (IOM) personal inhalable samplers for the air collection. For the air sampling method developed, the authors reported LOD values of 0.4 ng for fentanyl and sufentanil while 1.6 ng for alfentanil, values that were found to be identical to those associated with their wipe sampling method.
Acrylfentanyl
Acrylfentanyl, N-phenyl-N-[1-(2-phenylethyl)-4-piperidinyl]-2-propenamide or acryloylfentanyl, is a fentanyl analogs that can be considered an unsaturated version of the flagship opioid, featuring an olefinic amide rather than a fully saturated ethylamide side chain (). Acrylfentanyl was first reported in May 2016 when it was detected and identified as a nonscheduled component in a powder seized at a psychiatric ward in Denmark,[Citation115,Citation146] even though its synthetic origins can be traced as far back as 1982.[Citation147] Its preparation follows the same sequence used for fentanyl with the installation of its signature unsaturated amide accomplished with the treatment of the 1-phenethyl-N-phenylpiperidin-4-amine intermediate (4-ANPP in ) with acryloyl chloride. Another route to the opioid emerged in 1999, where the intermediate 4-ANPP is treated with the chemical 3-chloropropanoyl chloride to give an intermediate chlorinated precursor that then undergoes an in situ spontaneous elimination to yield acrylfentanyl ().[Citation148] In the Danish study by Breindahl et al., acrylfentanyl was tentatively identified in the seized powder from the confiscated capsules using GC-MS with electron-impact ionization (EI). During this first analysis of the powder by GC-MS, its EI spectrum could not be confidently identified by use of library searches that resulted in the initial theory that this could be a close relative of fentanyl. This is an initial facet of identifying an unknown analyte using the instrument’s library matching program which can be extremely valuable but need to be considered with caution when assigning a chemical structure to an unknown analyte.[Citation149–151] Thus, subsequent analyses involving quadrupole time-of-flight high resolution mass spectrometry (QTOF-MS) couped to an ultra-high performance liquid chromatography (UHPLC) unit, nuclear magnetic resonance (NMR) and infrared spectroscopy (IR) were needed in order to fully identify acrylfentanyl as the opioid. The amount of the acrylfentanyl hydrochloride was eventually determined using high performance liquid chromatography and triple quadrupole spectrometry (LC-MS-MS). Although the authors do not mention the sample preparation for the GC-MS analysis, it can be anticipated that the powder was taken through a basic extraction step to convert the acrylfentanyl hydrochloride to its free base. In the end, the nature of the unknown fentanyl at the time was confirmed through the synthesis of a standard of acrylfentanyl. Acrylfentanyl was determined to be the main causative agent in the death of 40 intoxications in Sweden during the period between April and October of 2016.[Citation152] In this report, the authors specifically focused on five out of the forty cases that presented acrylfentanyl as the main causative agent for the respiratory depression of the decedents. Thus, these cases involved only acrylfentanyl and in three out of the five the mode of intoxication involved a nasal spray. For this mode of intoxication, the blood concentrations of the opioid in the victims for each case was found to be 0.02, 0.78, and 2.9 ng.g−1. The remaining two cases involved the use of tablets and the toxicology report showed concentrations of acrylfentanyl in the victim’s blood of 0.01 and 0.31 ng.g−1. The quantitation of acrylfentanyl was done using an internal standard of the same drug.
Butyrylfentanyl
Butyrylfentanyl, N-(1-phenethylpiperidin-4-yl)-N-phenylbutyramide or butyrfentanyl, is a designer fentanyl was first reported in Poland in the summer of 2013[Citation153] and then in Sweden in 2015.[Citation154] In Sweden, the cases involved patients with suspected acute exposure to synthetic opioids and requiring hospitalization in 2014; two of the four intoxications exhibited serum concentrations of 0.9 and 0.6 ng.mL−1 of butyrylfentanyl while the others featured fentanyl as the more prominent opioid component. An intoxication with butyrylfentanyl in the United States involved a teenager who survived an overdose but who subsequently suffered from numerous conditions brought upon by snorting butyrylfentanyl such as hypoxic respiratory failure and diffuse alveolar hemorrhage. The detection and identification of butyrylfentanyl in this case was accomplished using GC-MS.[Citation113] Butyrylfentanyl may be synthesized using the original Janssen route to fentanyl as the only difference between this opioid and the parent fentanyl one lies in the nature of the amide portion of the molecule. Alternatively, it can be made by the reaction between 4-ANPP, the last intermediate before fentanyl in the Siegfried and Valdez routes, with butanoyl chloride.[Citation36,Citation65] Due to its great striking similarity to fentanyl, GC-MS analyses of butyrylfentanyl involve the same sample preparation steps. In 2016, a report appeared where butyrylfentanyl was identified in autopsy findings for two fatal intoxications involving this opioid.[Citation113] In this work the overall analysis and eventual identification of butyrylfentanyl was accomplished using UHPLC-MS-MS, but the initial, preliminary identification step involved the use of headspace EI-GC-MS in addition to the established immunoassays for common drugs of abuse. Aside from butyrylfentanyl, acetylfentanyl was also identified in the analysis of the blood, vitreous humor and urine specimens using the exact sample preparation described by Swanson et al., in their analysis of carfentanil and furanylfentanyl (). A report emerged in 2016 from the San Diego Medical Examiner’s Office describing the detection of butyrylfentanyl, along with acetylfentanyl, during the postmortem analyses of tissues from a 40-year victim who had a history of intravenous drug use.[Citation106] The work solely focuses on a GC-MS-based analysis of the samples, and the sample preparation steps are similar to those employed for the analysis of fentanyl. Briefly, the samples were partitioned in deionized water:1-chlorobutane and basified using ammonium hydroxide followed by centrifugation and re-acidification using HCl of the organic layer followed by an aqueous extraction. Re-basification with ammonium hydroxide and extraction with 1-chlorobutane yielded and organic phase that was then concentrated and taken up in ethyl acetate for GC-MS analysis using SIM ().[Citation106] The cause of death was deemed an accidental ingestion (gastric contents of the opioid where found) of a mixture of butyrylfentanyl and acetylfentanyl in addition to cocaine. The concentrations of butyrylfentanyl found in the collected peripheral and central blood were 58 and 97 ng.mL−1 respectively, while those for acetylfentanyl were 38 and 32 ng.mL−1 respectively (). In another interesting paper in 2016, Staeheli et al. at the Zurich Institute of Forensic Medicine showed that butyrylfentanyl can undergo postmortem tissue redistribution.[Citation114] In this work, the authors demonstrate a marked difference in the opioid concentrations in the tissues studied over time in a postmortem scenario, thus they found that butyrylfentanyl’s concentration in femoral blood increased by 120% and heart blood by 55% over the time span of ∼10 hours. Although the authors make use of LC-MS-MS for the final determination of the opioid concentrations in the collected tissues, they do make use of GC-MS in the preliminary stages of the analysis. During this analysis the authors could not pinpoint the exact nature of the structure as there were no high confidence library matches available, however a peak with a m/z = 145 was indicative enough to suggest a fentanyl analog was present. This, coupled to the earlier finding that the immunoassay results yielded a potentially positive hit for a fentanyl-like compound strengthened the hypothesis for a possible fentanyl analog ().
Remifentanil
Remifentanil, methyl 1-(3-methoxy-3-oxopropyl)-4-(N-phenylpropionamido)piperidine-4-carboxylate, is a Schedule I fentanyl analog that has been found to be ∼100 times more potent than morphine and roughly twice as potent as fentanyl itself. Remifentanil is an interesting member of this class of synthetic opioids because it does not exhibit a phenylethyl side chain or another aromatic ring-containing side chain off the piperidine ring nitrogen. Instead, remifentanil contains a propanoic acid methyl ester moiety, in contrast to the other members of the family that to some extent feature an aromatic ring at the end of this side group, like the phenyl group in fentanyl, the thiophene moiety in sufentanil or the unique tetrazolone ring system exhibited by alfentanil. It has been widely employed as a general anesthetic in medicine under the tradename Ultiva and it is highly preferred over other related analogs for its rapid onset and fast recovery time in patients who have undergone.[Citation155–157] It is these two specific qualities that have made remifentanil not one of the major players in the ambit of illicit drugs although there are some cases of drug abuse that where the opioid has been found to be the responsible culprit.[Citation158] The synthesis of remifentanil follows the same route as for the synthesis of the carfentanil nucleus, in fact published works involving its synthesis often have their process applied to the synthesis of carfentanil itself. Some notable syntheses that require mentioning are outlined in . Thus, a report from Glaxo at Research Triangle Park in 1991 described the synthesis of remifentanil starting with the key, benzylated piperidine intermediate employed in the original Janssen route ().[Citation159] Hydrogenolysis of the benzyl group to liberate the piperidine ring nitrogen followed by a Michael addition reaction with acrylic acid t-butyl ester to generate a remifentanil analog at this point. Exchange of the t-butyl ester to the methyl ester provided the final opioid. In 2010, Mallinckrodt LLC published their synthesis of remifentanil in a patent that was centered around the use of a fully unprotected piperidine that was reacted regioselectively in stepwise fashion to yield remifentanil ().[Citation160] In the same year, the groups of Deprez and Laconde at the Université Lille Nord de France published their synthesis of remifentanil and carfentanil using the Ugi Multicomponent reaction.[Citation134] In this work, the authors make use of the four component reaction to rapidly assemble the remifentanil, and carfentanil, skeleton in one pot, followed by an additional acid hydrolysis step to yield remifentanil in 67% yield (70% for carfentanil).
Analysis of remifentanil by GC-MS involves the same sample preparation protocol, however it is noteworthy to mention that during the basification step, one must be careful not to use a highly basic work-up as this may cause hydrolysis of the methyl ester moiety in the opioid generating a carboxylate salt that may remain in the aqueous phase and may not be readily detected by GC-MS means if it partially dissolves in the organic solvent. To this end, some additional sample manipulations may be added to the original sample preparation outlined initially in this review, such as avoiding a basification step or placing an additive, like citric acid, to keep the pH from becoming too basic and causing the hydrolysis of the ester moiety in remifentanil.[Citation161,Citation162] Thus, a report in 2002 was published by Bjorksten et al. as a collaborative effort between the Royal Melbourne Hospital and the University of Melbourne in Australia, describing the development of a protocol for remifentanil analysis in human blood by GC-MS.[Citation109] In this work, the authors spiked citric acid-treated blood with different concentrations of remifentanil, fentanyl as an internal standard and proceed to carry out extractions of the opioid. The protocol involved the use of 1-chlorobutane as an extraction organic phase and the acidification step was carried out with 10 mM HCl. Interestingly, the basification step does not exist in this protocol and is supplanted by the use of a 10 mM KH2PO4 buffer solution (pH 4.2). Extraction of this aqueous phase with 1-chlorobutane followed by evaporation and reconstitution in methanol readied the sample for GC-MS analysis (). The developed method was found to have a lower limit of quantitation of 0.2 ng.mL−1 for the opioid and very importantly a between-day coefficients of variation of less than 15%.
Ocfentanil
Ocfentanil, N-(2-fluorophenyl)-N-(4-(2-methoxyacetyl)-1-phenethylpiperidin-4-yl)propionamide, is an analog displaying keen similarity to fentanyl on its short-acting opioid-like effects and features a similar potency profile. However, structurally ocfentanil features two distinctive features that make it different from fentanyl. One of these is the presence of a fluorine substituent in the amide moiety phenyl ring while the other one is an α-methoxycarbonyl unit off the amide nitrogen (). Its synthesis appeared for the first time in 1986[Citation162] and was evaluated in humans in 1989[Citation109] in search of an analgesic analog of fentanyl with better therapeutic index and fewer cardiovascular and respiratory effects but was never commercialized. Ocfentanil has been the main causative synthetic opioid in fatal overdose cases in Belgium[Citation163], Switzerland[Citation164] and Italy.[Citation165] Analysis in all cases above involving fatalities were done using LC-MS and hyphenated methods (e.g., UHPLC-MS-MS). Analysis by GC-MS cannot be found except for a report from the Hospital del Mar Medical Research Institute in Barcelona, Spain surfacing in 2017 by Quintana et al. describing the identification of ocfentanil in heroin samples purchased in the hidden web. Due to the low concentrations of this fentanyl in the samples it was concluded that it was been used as an adulterant by online sellers of heroin.[Citation34] Although no exact description on the sample preparation is given by the authors, the ocfentanil was initially detected by EI-GC-MS and further confirmation using LC-MS-MS.
Furanylfentanyl
Furanylfentanyl, N-(1-phenethylpiperidin-4-yl)-N-phenylfuran-2-carboxamide, is a fentanyl analog that made its entrance into the opioid crisis stage in Sweden in 2015.[Citation98] Although the Sweden 14 cases during this time were confirmed to involve other fentanyl analogs like acetylfentanyl and 4-methoxybutyrfentanyl, furanylfentanyl was detected in large serum concentrations in these individuals ranging from 4.4 to 148 ng.mL−1. It is accepted that these individuals were able to survive as a result of their immediate hospitalization, where 12 were placed in intensive care units while 2 required intubation/mechanical respiration, since these large furanylfentanyl concentrations have been associated in other lethal cases. Since then, only few cases have involved furanylfentanyl as the main causative agent in the overdose, like the one reported in British Columbia, Canada in 2016. In this case, the overdose cases involved the use of crack cocaine that was contaminated with furanylfentanyl.[Citation166] Furanylfentanyl became labeled Schedule I by the DEA in 2016 and was first synthesized in 1986 using the same sequence of steps involved in the Janssen route as well of that what will become known as the Siegfried method for fentanyl. The only difference lying obviously in the final acylation step of 4-ANPP where 2-furoyl chloride was used instead of propanoyl chloride.[Citation167] Regarding its detection by GC-MS, furanylfentanyl was found using this technique in individuals as reported by the Medical Examiner Department in Tampa, Florida.[Citation116] In this report, the authors examined two fatal cases involving furanylfentanyl and carfentanil separately. Initial toxicology screening of the initially extracted postmortem tissues that included blood, vitreous humor and urine, did not identify the common drugs of abuse but both furanylfentanyl and carfentanil were detected in the alkaline extractions of the tissues by GC-MS. In this work, the use of the unique solvent combination known as THIA (78:20:2 toluene:hexanes:isoamyl alcohol) is employed during the sample preparation steps for GC analysis (). Further confirmation of both opioids was done using a more targeted method involving LC-MS-MS, and even after this attempt in one of the cases it was not possible to confidently assign one of the unknown opioids to carfentanil, thus additional analysis by another reference laboratory finally revealed the presence of this opioid in extremely low concentrations (0.12 ng.mL−1 in blood). In 2019, a report by Misailidi et al. from the Department of Forensic Medicine and Toxicology at the National and Kapodistrian University on the analysis of spiked furanylfentanyl, among others, in blood.[Citation117] Utilizing a deuterated version of methadone (methadone-D3) the authors describe a sample preparation protocol that includes the universal acidification/basification steps that get the sample ready for GC-MS-SIM analysis. The method’s LOD were found to be in the sub-nanogram ranges for the studied opioids: furanylfentanyl (0.3 ng.mL−1), ocfentanil (1.0 ng.mL−1), acetylfentanyl (0.15 ng.mL−1) and butyrylfentanyl (0.50 ng.mL−1).
Methylfentanyl
Methylfentanyl as the name implies is a synthetic opioid related to fentanyl and containing an extra methyl unit in its structure relative to the parent compound. However, it is important to mention that when initial reports started to show up about this “new” analog of fentanyl whose effect were still reversed via naloxone treatment.[Citation168,Citation169] After analyses that involved infrared (IR) and nuclear magnetic resonance (NMR) spectroscopy in addition to EI-GC-MS it was found that some of these can be attributed to 3-methylfentanyl while others (15 cases in total) identified α-methylfentanyl as the culprit.[Citation170] During their initial appearance in the field, the term methylfentanyl was used as a general term and it actually encompasses two very distinct compounds and their associated isomers (enantiomers and diastereomers) collectively becoming known with various names including “china white,” “china girl,” “Persian white,” “egg white,” and “999.” Thus, I will refer to α-methylfentanyl (N-phenyl-N-(1-(1-phenylpropan-2-yl)piperidin-4-yl)propionamide), when discussing the methylfentanyl where the methyl group resides on the phenylethyl side chain, while we will refer to 3-methylfentanyl (N-(3-methyl-1-phenethylpiperidin-4-yl)-N-phenylpropionamide), to the isomer bearing the methyl group on the 3-position of the piperidine ring (). α-Methylfentanyl and 3-methylfentanyl, have both been found to be more potent than fentanyl by a factor of ∼1 and ∼16 respectively.[Citation171,Citation172] Both designer fentanyls made their entrance into the illicit drug market in California, with α-methylfentanyl appearing in 1979 while 3-methylfentanyl did so in 1984 when it was involved in a burst of epidemic overdoses.[Citation173] After these episodes, the abundance of reports involving these methylated analogs of fentanyl started to appear, with the identification of 3-methylfentanyl in a seized sample in St. Petersburg in 1990.[Citation112] Two more recent cases where this class of fentanyl analogs were involved in fatal overdosing are in Estonia back during the time period between 2005 and 2006[Citation174] and the three lethal cases in involving strictly 3-methylfentanyl in Finland back in 2002.[Citation175] It was found that the concentration of methylfentanyls, plainly dubbed as a mixture of cis and trans isomers and very likely a combination of all four isomers, was 1.9 ng.mL−1 in the victims’ blood, which represents a value that is about ten-fold lower than the one usually associated with fentanyl concentrations involved in fatal cases. The second case originated in Summit and Cuyahoga Counties in Ohio, US and it involved the death of 125 individuals and were attributed mainly to mixtures of carfentanil and other fentanyl members where 3-methylfentanyl was found to be one of the key players.[Citation176]
Figure 8. Chemical structures of ?-methylfentanyl and 3-methylfentanyl. The asterisks in each structure denote a chiral carbon center. In ?-methylfentanyl the presence of one chiral center gives rise to the existence of two stereoisomers, while the presence of two chiral centers in 3-methylfentanyl gives rise to the existence of four isomers (a pair of enantiomers and a pair of diastereomers).
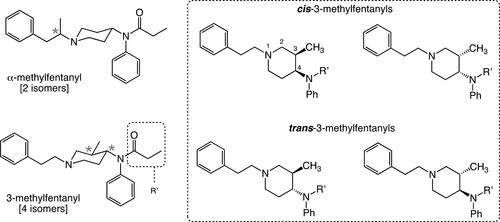
The synthesis of the methylfentanyls follows the same pathway as for the synthesis of fentanyl. The only difference between these and the parent fentanyl molecule is the presence of that chiral methyl group, which can have significant, negative consequences in the yields of the final product. The synthesis can be accomplished using 1-benzyl-3-methyl-4-piperidone, a starting material that has the methyl group already in place and can be taken through the synthetic steps originally described by Janssen to yield 3-methylfentanyl as a mixture of isomers. Another route begins with an N-protected 4-piperidone, where N could be the benzyl or the phenylethyl group, which can be alkylated at its C3 position using standard enolate chemistry (). Several ways of generating the enolate from this material can be sought and the actual alkylation goes through in good yields, the only side product arising in minor quantities from this step being the bis-alkylated product (). An interesting note about the first route is that the performance of the reductive amination step of the imine is severely hampered by the steric bulk imposed nearby by the methyl group thus not allowing for an efficient delivery of the reductive hydride species to the activated imine.[Citation68] Synthesis of the α-methylfentanyl structural isomer is simpler and generally possesses the same yields as for the synthesis of fentanyl. As expected, the synthesis of α-methylfentanyl follows the same route and the only difference lies in the use of 2-bromo-3-phenylpropane for the alkylation of the piperidine nitrogen in 4-piperidone. After this alkylation is accomplished, the same set of synthetic steps can be carried out to yield α-methylfentanyl generally as a mixture of enantiomers.
GCMS analysis of 3-methylfentanyls follow the same protocol for fentanyl as the addition of a methyl group does not create any issues with chemical instability of the fentanyl nucleus. However, addition of this group results in the creation of two, vicinal stereocenters that now give rise to the existence of four isomers for a given 3-methylfentanyl. In the case of α-methylfentanyl, only a mixture of enantiomers can be encountered as there is only one stereocenter present in the molecule. As a result of these stereogenic centers in both compounds, it is not uncommon to observe two closely eluting peaks during GC-MS analysis bearing strikingly similar mass spectra down to the fragmentation patterns. Complete resolution of all the isomers for both methylfentanyls can be accomplished with the use of a chiral GC column where the interactions of each isomer will differ significantly from the others thus giving rise to fairly different retention times. The nature and chromatographic behavior of isomers arising from 3-methylfentanyl was studied by GC-MS and LC-MS and reported by Kanamori et al. from the National Research Institute of Police Science in Japan in 2017.[Citation177] The authors successfully synthesized both cis and trans isomers of 3-methylfentanyl and were able to observe their clear separation and resolution by GC-MS. In addition to the chromatographic methods above, the authors also present a thorough Nuclear Magnetic Resonance (NMR) analysis of both sets of diastereomers which possess unique 1H NMR spectra. Another report dealing with the analysis of the cis and trans isomers derived from 3-methylfentanyl appeared in 2018 by Fogarty et al. at the Center for Forensic Science Research and Education and NMS Labs in Pennsylvania.[Citation178] Although their method does not involve GC-MS but LC-TOF-MS, it is noteworthy for its ability to detect both isomers at ultra-low levels ranging from 0.46 and 0.67 ng.mL−1 for the cis and the trans isomers respectively during their analysis of 25 postmortem cases. The analysis of blood and urine samples arising from the Estonia lethal episodes and that were conducted in the Department of Forensic Medicine at the University of Helsinki, involved their liquid-liquid extraction with butyl acetate and disodium hydrogen phosphate buffer (pH 9). After evaporation of the organic layer the residue was analyzed by GC-MS for a cursory screening of fentanyls in the tissues, the rest was taken up in ammonium acetate for the quantitative analysis by LC-MS-MS ().
Conclusions and outlook
The global pandemic involving synthetic opioids, particularly those belonging to the fentanyl class, has had a powerful worldwide impact on various facets of society ranging from healthcare to law enforcement to basic and applied research ventures in academic as well as private sectors. Aside from the enormous and expensive efforts devoted to control the opioid pandemic spearheaded by law enforcement and federal agencies like the Drug Enforcement Agency (DEA) and the Federal Bureau of Investigation (FBI), it is globally recognized that substantial research efforts are still needed in the development of medical countermeasures to mitigate the toxic effects of these toxic substances. To this end, research groups spanning academic as well as government laboratories have begun establishing programs aimed at the development of more effective and longer-lasting antidotes to treat and save the life of an overdose victim. Another line of research that goes hand in hand with antidote development is the discovery of effective medical countermeasures that can preemptively provide protection to medical personnel responding to an emergency or to military personnel that could be at a risk of exposure to the opioids during a conflict. It is important to know that although these are very important avenues to certainly pursue and accelerate the discovery phase for solid antidotal candidates, one also has to recognize that of equal importance are investigative efforts that lead to innovative ways of analyzing old and newly emerging synthetic opioids reliably and rapidly. If there is an analytical technique that has the potential of achieving both of the above paramount requirements, reliability and speed of analysis, it is GC-MS. As demonstrated by the literature reviewed herein, one can see that the sample preparation protocol still represents the most crucial step of any analysis involving GC-MS as its overall success will determine the ability of the technique to detect the opioid. The swift detection and identification of these substances in a clinically admitted individual, whether it is from an overdose scenario or an intended exposure as highlighted by the Moscow theater hostage crisis, is of paramount importance as it quickly aids the medical practitioner in determining the right medical treatment for the victim. Furthermore, the importance of building a spectral library can not stressed enough, as due to the fairly straightforward synthetic means by which these opioids are constructed, the need for better and constantly expanding mass spectral libraries containing the newly emerging analogs will be needed to aid in their identification. The construction of large libraries is of utmost importance as these tremendously aid in the rapid identification of the opioid and a potentially new analog, and thus moving away from a dependence on targeted detections which a priori can be used to generally assess the toxic substance landscape in a given sample. GC-MS is a popular analytical technique due to its ease of execution, relatively inexpensive maintenance and simplicity in its operation when compared to LC-MS units. In addition to this, the attained portability of these devices, which are becoming closer in sensitivity and power to their benchtop counterparts with every year that passes by, have catapulted this analytical technique at the forefront on field deployment applications.
Acknowledgements
Disclaimer: This document (LLNL-JRNL-818063) was prepared as an account of work sponsored by an agency of the United States government. Neither the United States government nor Lawrence Livermore National Security, LLC, nor any of their employees makes any warranty, expressed or implied, or assumes any legal liability or responsibility for the accuracy, completeness, or usefulness of any information, apparatus, product, or process disclosed, or represents that its use would not infringe privately owned rights. Reference herein to any specific commercial product, process, or service by trade name, trademark, manufacturer, or otherwise does not necessarily constitute or imply its endorsement, recommendation, or favoring by the United States government or Lawrence Livermore National Security, LLC. The views and opinions of authors expressed herein do not necessarily state or reflect those of the United States government or Lawrence Livermore National Security, LLC, and shall not be used for advertising or product endorsement purposes.
Additional information
Funding
References
- Grass, J. A. Sufentanil: Clinical Use as Postoperative Analgesic: Epidural/Intrathecal Route. J. Pain Symptom Manag. 1992, 7, 271–286. DOI: 10.1016/0885-3924(92)90061-L.
- Grass, J. A. Fentanyl: Clinical Use as Postoperative Analgesic: Epidural/Intrathecal Route. J. Pain Symptom Manag. 1992, 7, 419–430. DOI: 10.1016/0885-3924(92)90022-A.
- Buchanan, J. F.; Brown, C. R. ‘Designer Drugs’—A Problem in Clinical Toxicology. Med. Toxicol. Adverse Drug Exp. 1988, 3, 1–17. DOI: 10.1007/BF03259928.
- Janssen, P. A. J.; Eddy, N. B. Compounds Related to Pethidine-IV. New General Chemical Methods of Increasing the Analgesic Activity of Pethidine. J. Med. Chem. 1960, 2, 31–45. DOI: 10.1021/jm50008a003.
- Janssen, P. A. J.; Gardocki, J. F. Method for Producing Analgesia. US Patent 3141823, 1964.
- Stanley, T. H. The History and Development of the Fentanyl Series. J. Pain Symp. Manag. 1992, 7, S3–S7. DOI: 10.1016/0885-3924(92)90047-L.
- Janssen, P. A. J. A Review of the Chemical Features Associated with Strong Morphine-Like Activity. Br. J. Anaesth. 1962, 34, 260–268. DOI: 10.1093/bja/34.4.260.
- Vardanyan, R. S.; Hruby, V. J. Fentanyl-Related Compounds and Derivatives: Current Status and Future Prospects for Pharmaceutical Applications. Future Med. Chem. 2014, 6, 385–412. DOI: 10.4155/fmc.13.215.
- Armenian, P.; Vo, K. T.; Barr-Walker, J.; Lynch, K. L. Fentanyl, Fentanyl Analogs and Novel Synthetic Opioids: A Comprehensive Review. Neuropharmacology 2018, 134, 121–132. DOI: 10.1016/j.neuropharm.2017.10.016.
- Chen, J.-C.; Smith, E. R.; Cahill, M.; Cohen, R.; Fishman, J. B. The Opioid Receptor Binding of Dezocine, Morphine, Fentanyl, Butorphanol and Nalbuphine. Life Sci. 1993, 52, 389–396. DOI: 10.1016/0024-3205(93)90152-s.
- Pathan, H.; Williams, J. Basic Opioid Pharmacology: An Update. Br. J. Pain 2012, 6, 11–16. DOI: 10.1177/2049463712438493.
- Shim, J.; Coop, A.; MacKerell, A. D. Jr. Molecular Details of the Activation of the m Opioid Receptor. J. Phys. Chem. B. 2013, 117, 7907–7917. DOI: 10.1021/jp404238n.
- Pearson, A. Incapacitating Biochemical Weapons. Nonproliferation Rev. 2006, 13, 151–188. DOI: 10.1080/10736700601012029.
- Ganesan, K.; Raza, S. K.; Vijayaraghavan, R. Chemical Warfare Agents. J. Pharm. Bioall. Sci. 2010, 2, 166–178. DOI: 10.4103/0975-7406.68498.
- Duthie, D. J. R.; McLaren, A. D.; Nimmo, W. S. Pharmacokinetics of Fentanyl during Constant Rate i.v. infusion for the Relief of Pain after Surgery. Br. J. Anaesth. 1986, 58, 950–956. DOI: 10.1093/bja/58.9.950.
- Kharasch, E. D. Opioid Half-Lives and Hemlines: The Long and Short of Fashion. Anesthesiology 2015, 122, 969–970. DOI: 10.1097/ALN.0000000000000634.
- Centers for Disease Control and Prevention. Recommendations for Laboratory Testing for Acetyl Fentanyl and Patient Evaluation and Treatment for Overdose with Synthetic Opioid. CDC Health Alert Advisory, June 20, 2013. Health Alert Network, HAN00350.
- Food and Drug Administration. FDA Advisory Committee on the Most Appropriate Dose or Doses of Naloxone to Reverse the Effects of Life-Threatening Opioid Overdose in the Community Settings. 2016. https://www.fda.gov/media/100409/download (accessed Oct 13, 2020).
- Crabtree, B. L. Review of Naltrexone, a Long-Acting Opioid Antagonist. Clin. Pharm. 1984, 3, 273–280.
- Gonzalez, J. P.; Brogden, R. N. N. A Review of Its Pharmacodynamic and Pharmacokinetic Properties and Therapeutic Efficacy in the Management of Opioid Dependence. Drugs 1988, 35, 192–213. DOI: 10.2165/00003495-198835030-00002.
- Kjome, K. L.; Moeller, F. G. Long-Acting Injectable Naltrexone for the Management of Patients with Opioid Dependence. Subst. Abuse 2011, 5, 1–9. DOI: 10.4137/SART.S5452.
- Mayer, B. P.; Lau, E. Y.; Kennedy, D. J.; Valdez, C. A. Solution-State Structure and Affinities of Cyclodextrin:Fentanyl Complexes by Nuclear Magnetic Resonance Spectroscopy and Molecular Dynamics Simulation. J. Phys. Chem. B. 2016, 120, 2423–2433. DOI: 10.1021/acs.jpcb.5b12333.
- Lynn, R. R.; Galinkin, J. L. Naloxone Dosage for Opioid Reversal: Current Evidence and Clinical Implications. Ther. Adv. Drug Saf. 2018, 9, 63–88. DOI: 10.1177/2042098617744161.
- Chimbar, L.; Moleta, Y. Naloxone Effectiveness: A Systematic Review. J Addict. Nurs. 2018, 29, 167–171. DOI: 10.1097/JAN.0000000000000230.
- Han, Y.; Yan, W.; Zheng, Y.; Khan, M. Z.; Yuan, K.; Li, L. The Rising Crisis of Illicit Fentanyl Use, Overdose, and Potential Therapeutic Strategies. Transl. Psychiat. 2019, 9, 282. DOI: 10.1038/s41398-019-0625-0.
- Pardo, B.; Taylor, J.; Caulkins, J.; Reuter, P.; Kilmer, B. The Dawn of a New Synthetic Opioid Era: The Need for Innovative Interventions. Addiction 2020. DOI: 10.1111/add.15222.
- France, C. P.; Ahern, G.; Averick, S.; Enright, H. A.; Esmaeli-Azad, B.; Federico, A.; Gerak, L. R.; Kolber, B.; Lau, E. Y.; Lao, V.; et al. Countermeasures for Preventing and Treating Opioid Overdose. Clin. Pharmacol. Ther. 2021, 109, 578–590. DOI: 10.1002/cpt.2098.
- Wax, P. M.; Becker, C. E.; Curry, S. C. Unexpected “Gas” Casualties in Moscow: A Medical Toxicology Perspective. Ann. Emerg. Med. 2003, 41, 700–705. DOI: 10.1067/mem.2003.148.
- Coupland, R. M. Incapacitating Chemical Weapons: A Year after the Moscow Theatre Siege. Lancet 2003, 362, 1346. DOI: 10.1016/S0140-6736(03)14684-3.
- Stone, R. Weapons in Waiting. Science 2018, 359, 24–24. DOI: 10.1126/science.359.6371.24.
- Riches, J. R.; Read, R. W.; Black, R. M.; Cooper, N. J.; Timperley, C. M. Analysis of Clothing and Urine from Moscow Theatre Siege Casual-Ties Reveals Carfentanil and Remifentanil Use. J. Anal. Toxicol. 2012, 36, 647–656. DOI: 10.1093/jat/bks07.
- Algren, D. A.; Monteilh, C. P.; Punja, M.; Schier, J. G.; Belson, M.; Hepler, B. R.; Schmidt, C. J.; Miller, C. E.; Patel, M.; Paulozzi, L. J.; et al. Fentanyl-Associated Fatalities among Illicit Drug Users in Wayne County, Michigan (July 2005–May 2006). J. Med. Toxicol. 2013, 9, 106–115. DOI: 10.1007/s13181-012-0285-4.
- Rodda, L. N.; Pilgrim, J. L.; Di Rago, M.; Crump, K.; Gerostamoulos, D.; Drummer, O. H. A Cluster of Fentanyl-Laced Heroin Deaths in 2015 in Melbourne. Australia. J. Anal. Toxicol. 2017, 41, 318–324. DOI: 10.1093/jat/bkx013.
- Quintana, P.; Ventura, M.; Grifell, M.; Palma, A.; Galindo, L.; Fornís, I.; Gil, C.; Carbón, X.; Caudevilla, F.; Farré, M.; Torrens, M. The Hidden Web and the Fentanyl Problem: Detection of Ocfentanil as an Adulterant in Heroin. Int. J. Drug Policy 2017, 40, 78–83. DOI: 10.1016/j.drugpo.2016.10.006.
- Gupta, P. K.; Ganesan, K.; Pande, A.; Malhotra, R. C. A Convenient One Pot Synthesis of Fentanyl. J. Chem. Res. 2005, 7, 452–453. DOI: 10.3184/030823405774309078.
- Valdez, C. A.; Leif, R. N.; Mayer, B. P. An Efficient, Optimized Synthesis of Fentanyl and Related Analogs. PLoS One 2014, 9, e108250. DOI: 10.1371/journal.pone.0108250.
- Walz, A. J.; Hsu, F.-L. An Operationally Simple Synthesis of Fentanyl Citrate. Org. Prep. Proced. Int 2017, 49, 467–470. DOI: 10.1080/00304948.2017.1374129.
- Moss, R. B.; Carlo, D. J. Higher Doses of Naloxone Are Needed in the Synthetic Opioid Era. Subst. Abuse Treat. Prev. Policy 2019, 14, 1–6.
- Yeung, D. T.; Bough, K. J.; Harper, J. R.; Platoff, G. E. Jr., National Institutes of Health (NIH) Executive Meeting Summary: Developing Medical Countermeasures to Rescue Opioid-Induced Respiratory Depression (a Trans-Agency Scientific Meeting)—August 6/7, 2019. J. Med. Toxicol. 2020, 16, 87–105. DOI: 10.1007/s13181-019-00750-x.
- Kumar, K.; Morgan, D. J.; Crankshaw, D. P. Determination of Fentanyl and Alfentanil in Plasma by High-Performance Liquid Chromatography with Ultraviolet Detection. J Chromatogr 1987, 419, 464–468. DOI: 10.1016/0378-4347(87)80317-1.
- Cooreman, S.; Deprez, C.; Martens, F.; Van Bocxlaer, J.; Croes, K. A Comprehensive LC-MS-Based Quantitative Analysis of Fentanyl-Like Drugs in Plasma and Urine. J. Sep. Sci. 2010, 33, 2654–2662. DOI: 10.1002/jssc.201000330.
- Gerace, E.; Salomone, A.; Vincenti, M. Analytical Approaches in Fatal Intoxication Cases Involving New Synthetic Opioids. CPB 2018, 19, 113–123. DOI: 10.2174/1389201019666180405162734.
- Valdez, C. A.; Leif, R. N.; Hok, S.; Hart, B. R. Analysis of Chemical War-Fare Agents by Gas Chromatography-Mass Spectrometry: Methods for Their Direct Detection and Derivatization Approaches for the Analysis of Their Degradation Products. Rev. Anal. Chem. 2018, 37, 1–26. DOI: 10.1515/revac-2017-0007.
- Drummer, O. H. Fatalities Caused by Novel Opioids: A Review. Forensic Sci. Res. 2019, 4, 95–110. DOI: 10.1080/20961790.2018.1460063.
- Schott, M.; Wehrenfennig, C.; Gasch, T.; Düring, R.-A.; Vilcinskas, A. A Portable Gas Chromatograph with Simultaneous Detection by Mass Spectrometry and Electroantennography for the Highly Sensitive in Situ Measurement of Volatiles. Anal. Bioanal. Chem. 2013, 405, 7457–7467. DOI: 10.1007/s00216-013-7196-3.
- Leary, P. E.; Dobson, G. S.; Reffner, J. A. Development and Applications of Portable Gas Chromatography-Mass Spectrometry for Emergency Responders, the Military, and Law-Enforcement Organizations. Appl. Spectrosc. 2016, 70, 888–896. DOI: 10.1177/0003702816638294.
- Leary, P. E.; Kammrath, B. W.; Lattman, K. J.; Beals, G. L. Deploying Portable Gas Chromatography-Mass Spectrometry (GC-MS) to Military Users for the Identification of Toxic Chemical Agents in Theater. Appl. Spectrosc. 2019, 73, 841–858. DOI: 10.1177/0003702819849499.
- Pardo, B.; Taylor, J.; Caulkins, J. C.; Kilmer, B.; Reuter, P.; Stein, B. D. The Future of Fentanyl and Other Synthetic Opioids. Santa Monica, CA: RAND Corporation, 2019. DOI: 10.7249/RR3117.
- Ruangyuttikarn, W.; Law, M. Y.; Rollins, D. E.; Moody, D. E. Detection of Fentanyl and Its Analogs by Enzyme-Linked Immunosorbent Assay. J. Anal. Toxicol. 1990, 14, 160–164. DOI: 10.1093/jat/14.3.160.
- Alburges, M. E.; Hanson, G. R.; Gibb, J. W.; Sakashita, C. O.; Rollins, D. E. Fentanyl Receptor Assay. II. Utilization of a Radioreceptor Assay for the Analysis of Fentanyl Analogues in Urine. J. Anal. Toxicol. 1992, 16, 36–41. DOI: 10.1093/jat/16.1.36.
- Wang, B. T.; Colby, J. M.; Wu, A. H. B.; Lynch, K. L. Cross-Reactivity of Acetylfentanyl and Risperidone with Fentanyl Immunoassay. J. Anal. Toxicol. 2014, 38, 672–675. DOI: 10.1093/jat/bku103.
- Ogilvie, L.; Stanley, C.; Lewis, L.; Boyd, M.; Lozier, M. Notes from the Field: Acetyl Fentanyl Overdose fatalities-Rhode Island, March-May 2013. Morb. Mortal Wkly. Rep. 2013, 62, 703–704.
- Schütz, H.; Paine, A.; Erdmann, F.; Weiler, G.; Verhoff, M. A. Immunoassays for Drug Screening in Urine: Chances, Challenges, and Pitfalls. Forensic Sci. Med. Pathol. 2006, 2, 75–83. DOI: 10.1385/FSMP:2:2:75.
- Foerster, E. H.; Mason, M. F. Preliminary Studies on the Use of n-Butyl Chloride as an Extractant in a Drug Screening Procedure. J. Forensic Sci. 1974, 19, 10084J. DOI: 10.1520/JFS10084J.
- Varvel, J. R.; Shafer, S. L.; Hwang, S. S.; Coen, P. A.; Stanski, D. R. Absorption Characteristics of Transdermally Administered Fentanyl. Anesthesiology 1989, 70, 928–934. DOI: 10.1097/00000542-198906000-00008.
- Gardocki, J. F.; Yelnosky, J. A. Study of Some of the Pharmacological Activity of Fentanyl Citrate. Toxicol. Appl. Pharmacol. 1964, 6, 48–62. DOI: 10.1016/0041-008X(64)90021-3.
- Janssen, P. A. J. Potent, New Analgesics, Tailor-Made for Different Purposes. Acta Anaesthesiol. Scand. 1982, 26, 262–268. DOI: 10.1111/j.1399-6576.1982.tb01765.x.
- Gladden, R. M.; Martinez, P.; Seth, P. Fentanyl Law Enforcement Submissions and Increases in Synthetic Opioid-Involved Overdose Deaths—27 States, 2013-2014. MMWR Morb. Mortal. Wkly. Rep. 2016, 65, 837–843. DOI: 10.15585/mmwr.mm6533a2.
- Marinetti, L. J.; Ehlers, B. J. A Series of Forensic Toxicology and Drug Seizure Cases Involving Illicit Fentanyl Alone and in Combination with Heroin, Cocaine or Heroin and Cocaine. J. Anal. Toxicol. 2014, 38, 592–598. DOI: 10.1093/jat/bku086.
- Krinsky, C. S.; Lathrop, S. L.; Crossey, M.; Baker, G.; Zumwalt, R. A Toxicology-Based Review of Fentanyl-Related Deaths in New Mexico (1986-2007). Am. J. Forensic Med. Pathol. 2011, 32, 347–351. DOI: 10.1097/PAF.0b013e31822ad269.
- Lee, D.; Chronister, C. W.; Broussard, W. A.; Utley-Bobak, S. R.; Schultz, D. L.; Vega, R. S.; Goldberger, B. A. Illicit Fentanyl-Related Fatalities in Florida: Toxicological Findings. J. Anal. Toxicol. 2016, 40, 588–594. DOI: 10.1093/jat/bkw087.
- Peterson, A. B.; Gladden, R. M.; Delcher, C.; Spies, E.; Garcia-Williams, A.; Wang, Y.; Halpin, J.; Zibbell, J.; McCarty, C. L.; DeFiore-Hyrmer, J.; et al. Increases in Fentanyl-Related Overdose Deaths—Florida and Ohio, 2013-2015. MMWR Morb. Mortal. Wkly. Rep. 2016, 65, 844–849. DOI: 10.15585/mmwr.mm6533a3.
- Blachman-Forshay, J.; Nolan, M. L.; McAteer, J. M.; Paone, D. Estimating the Risk of Exposure to Fentanyl in New York City: Testing Drug Residue in Used Syringes. Am. J. Public Health 2018, 108, 1666–1668. DOI: 10.2105/AJPH.2018.304694.
- Mercado-Crespo, M. C.; Sumner, S. A.; Bridget Spelke, M.; Sugerman, D. E.; Stanley, C. Notes from the Field: Increase in Fentanyl-Related Overdose Deaths—Rhode Island, November 2013-March 2014. MMWR Morb. Mortal. Wkly. Rep. 2014, 63, 531.
- Siegfried. Synthesis of Fentanyl. 2014. https://erowid.org/archive/rhodium/chemistry/fentanyl.html (accessed Oct 7, 2020).
- Norman, K.; Ciesielski, A. L.; Wagner, J. R. Identification and Associated Hazards of Clandestine Drug Laboratories. WIREs Forensic Sci. 2021, 3, e1393. DOI: 10.1002/wfs2.1393.
- Mayer, B. P.; DeHope, A. J.; Mew, D. A.; Spackman, P. E.; Williams, A. M. Chemical Attribution of Fentanyl Using Multivariate Statistical Analysis of Orthogonal Mass Spectral Data. Anal. Chem. 2016, 88, 4303–4310. DOI: 10.1021/acs.analchem.5b04434.
- Mayer, B. P.; Valdez, C. A.; DeHope, A. J.; Spackman, P. E.; Williams, A. M. Statistical Analysis of the Chemical Attribution Signatures of 3-Methylfentanyl and Its Methods of Production. Talanta 2018, 186, 645–654. DOI: 10.1016/j.talanta.2018.02.026.
- Mörén, L.; Qvarnström, J.; Engqvist, M.; Afshin-Sander, R.; Wu, X.; Dahlén, J.; Löfberg, C.; Larsson, A.; Östin, A. Attribution of Fentanyl Analogue Synthesis Route by Multivariable Data Analysis of Orthogonal Mass Spectral Data. Talanta 2019, 203, 122–130. DOI: 10.1016/j.talanta.2019.05.025.
- Casale, J. F.; Hays, P.; Toske, S. G.; Mallette, J. R. Unique Bipiperidinyl Impurities Produced from the “One-Pot” Synthesis of Fentanyl. Forensic Chem. 2020, 17, 100203. DOI: 10.1016/j.forc.2019.100203.
- Vaughan, S. R.; DeGreeff, L. E.; Forte, L.; Holness, H. K.; Furton, K. G. Identification of Volatile Components in the Headspace of Pharmaceutical-Grade Fentanyl. Forensic Chem. 2021, 24, 100331. DOI: 10.1016/j.forc.2021.100331.
- Ovenden, S. P. B.; McDowall, L. J.; McKeown, H. E.; McGill, N. W.; Jones, O. A. H.; Pearson, J. R.; Petricevic, M.; Rogers, M. L.; Rook, T. J.; Williams, J.; et al. Investigating the Chemical Impurity Profiles of Fentanyl Preparations and Precursors to Identify Chemical Attribution Signatures for Synthetic Method Attribution. Forensic Sci. Int. 2021, 321, 110742. DOI: 10.1016/j.forsciint.2021.110742.
- Vandeputte, M. M.; Krotulski, A. J.; Hulpia, F.; Calenbergh, V. S.; Stove, C. P. Phenethyl-4-ANPP: A Marginally Active Byproduct Suggesting a Switch in Illicit Fentanyl Synthesis Routes. J. Anal. Toxicol. 2021. DOI: 10.1093/jat/bkab032.
- Fraga, C. G.; Bronk, K.; Dockendorff, B. P.; Heredia-Langner, A. Organic Chemical Attribution Signatures for the Sourcing of a Mustard Agent and Its Starting Materials. Anal. Chem. 2016, 88, 5406–5413. DOI: 10.1021/acs.analchem.6b00766.
- Höjer Holmgren, K.; Valdez, C. A.; Magnusson, R.; Vu, A. K.; Lindberg, S.; Williams, A. M.; Alcaraz, A.; Åstot, C.; Hok, S.; Norlin, R. Part 1: Tracing Russian VX to Its Synthetic Routes by Multivariate Statistics of Chemical Attribution Signatures. Talanta 2018, 186, 586–596. DOI: 10.1016/j.talanta.2018.02.104.
- Jansson, D.; Wiklund Lindström, S.; Norlin, R.; Hok, S.; Valdez, C. A.; Williams, A. M.; Alcaraz, A.; Nilsson, C.; Åstot, C. Part 2: Forensic Attribution Profiling of Russian VX in Food Using Liquid Chromatography-Mass Spectrometry. Talanta 2018, 186, 597–606. DOI: 10.1016/j.talanta.2018.02.103.
- Williams, A. M.; Vu, A. K.; Jansson, D.; Mayer, B. P.; Hok, S.; Norlin, R.; Valdez, C. A.; Nilsson, C.; Åstot, C.; Alcaraz, A. Part 3: Solid Phase Extraction of Russian VX and Its Chemical Attribution Signatures in Food Matrices and Their Detection by GC-MS and LC-MS. Talanta 2018, 186, 607–614. DOI: 10.1016/j.talanta.2018.03.044.
- Ovenden, S. P. B.; Webster, R. L.; Micich, E.; McDowall, L. J.; McGill, N. W.; Williams, J.; Zanatta, S. D. The Identification of Chemical Attribution Signatures of Stored VX Nerve Agents Using NMR, GC-MS and LC-HRMS. Talanta 2020, 211, 120753. DOI: 10.1016/j.talanta.2020.120753.
- Lin, S. N.; Wang, T. F.; Caprioli, R. M.; Mo, B. P. Determination of Plasma Fentanyl by GC-Mass Spectrometry and Pharmacokinetic Analysis. J. Pharm. Sci. 1981, 70, 1276–1279. DOI: 10.1002/jps.2600701124.
- Moore, J. M.; Allen, A. C.; Cooper, D. A.; Carr, S. M. Determination of Fentanyl and Related Compounds by Capillary Gas Chromatography with Electron Capture Detection. Anal. Chem. 1986, 58, 1656–1660. DOI: 10.1021/ac00121a013.
- Watts, V.; Caplan, Y. Determination of Fentanyl in Whole Blood at Subnanogram Concentrations by Dual Capillary Column Gas Chromatography with Nitrogen Sensitive Detectors and Gas Chromatography/Mass Spectrometry. J. Anal. Toxicol. 1988, 12, 246–254. DOI: 10.1093/jat/12.5.246.
- Laganière, S.; Goernert, L.; Gallicano, K.; Otson, R. Improved Extraction, Sensitivity, Specificity, and Stability for Measuring Fentanyl in Plasma by Gas Chromatography with Nitrogen Detection. Clin. Chem. 1993, 39, 2206–2207. DOI:10.1093/clinchem/39.10.2206.
- Kingsbury, D. P.; Makowski, G. S.; Stone, J. A. Quantitative Analysis of Fentanyl in Pharmaceutical Preparations by Gas Chromatography-Mass Spectrometry. J. Anal. Toxicol. 1995, 19, 27–30. DOI: 10.1093/jat/19.1.27.
- Sachs, H.; Uhl, M.; Hege-Scheuing, G.; Schneider, E. Analysis of Fentanyl and Sufentanil in Hair by GC/MS/MS. Int. J. Leg. Med. 1996, 109, 213–215. DOI: 10.1007/BF01225521.
- Kronstrand, R.; Druid, H.; Holmgren, P.; Rajs, J. A Cluster of Fentanyl-Related Deaths among Drug Addicts in Sweden. Forensic Sci. Int. 1997, 88, 185–193. DOI: 10.1016/s0379-0738(97)00068-6.
- Anderson, D. T.; Muto, J. J. Duragesic Transdermal Patch: postmortem Tissue Distribution of Fentanyl in 25 Cases. J. Anal. Toxicol. 2000, 24, 627–634. DOI: 10.1093/jat/24.7.627.
- Kuhlman, J. J.; McCaulley, R.; Valouch, T. J.; Behonick, G. S. Fentanyl Use, Misuse, and Abuse: A Summary of 23 Post-Mortem Cases. J. Anal. Toxicol. 2003, 27, 499–504. DOI: 10.1093/jat/27.7.499.
- Malkawi, A. H.; Al-Ghananeem, A. M.; Crooks, P. A. Development of a GC-MS Assay for the Determination of Fentanyl Pharmacokinetics in Rabbit Plasma after Sublingual Spray Delivery. AAPS J. 2008, 10, 261–267. DOI: 10.1208/s12248-008-9031-x.
- Strano-Rossi, S.; Bermejo, A. M.; de la Torre, X.; Botrè, F. Fast GC-MS Method for the Simultaneous Screening of THC-COOH, Cocaine, Opiates and Analogues Including Buprenorphine and Fentanyl, and Their Metabolites in Urine. Anal. Bioanal. Chem. 2011, 399, 1623–1630. DOI: 10.1007/s00216-010-4471-4.
- Moore, J. M.; Allen, A. C.; Cooper, D. A. Determination of Manufacturing Impurities in Heroin by Capillary Gas Chromatography with Electron Capture Detection after Derivatization with Heptafluorobutyric Anhydride. Anal. Chem. 1984, 56, 642–646. DOI: 10.1021/ac00268a012.
- Strano-Rossi, S.; Alvarez, I.; Tabernero, M. J.; Cabarcos, P.; Fernández, P.; Bermejo, A. M. Determination of Fentanyl, Metabolite and Analogs in Urine by GC/MS. J. Appl. Toxicol. 2011, 31, 649–654. DOI: 10.1002/jat.1613.
- Bravo, F.; González, D.; Benites, J. Development and Validation of a Solid-Phase Extraction Gas Chromatography-Mass Spectrometry Method for the Simultaneous Quantification of Opioid Drugs in Human Whole Blood and Plasma. J. Chil. Chem. Soc. 2011, 56, 799–802. DOI: 10.4067/S0717-97072011000300017.
- McIntyre, I. M.; Gary, R. D.; Estrada, J.; Nelson, C. L. Antemortem and Postmortem Fentanyl Concentrations: A Case Report. Int. J. Legal Med. 2014, 128, 65–67. DOI: 10.1007/s00414-013-0897-5.
- Gardner, M. A.; Sampsel, S.; Jenkins, W. W.; Owens, J. E. Analysis of Fentanyl in Urine by DLLME-GC-MS. J. Anal. Toxicol. 2015, 39, 118–125. DOI: 10.1093/jat/bku136.
- Lozier, M. J.; Boyd, M.; Stanley, C.; Ogilvie, L.; King, E.; Martin, C.; Lewis, L. Acetyl Fentanyl, a Novel Fentanyl Analog, Causes 14 Overdose Deaths in Rhode Island, March–May 2013. J. Med. Toxicol. 2015, 11, 208–217. DOI: 10.1007/s13181-015-0477-9.
- Higashikawa, Y.; Suzuki, S. Studies on 1-(2-Phenthyl)-4- (N-Propionylanilino)Piperidine (Fentanyl) and Its Related Compounds: structure-Analgesic Activity Relationship for Fentanyl, Methyl-Substituted Fentanyls and Other Analogues. Forensic Toxicol. 2008, 26, 1–5. DOI: 10.4155/fmc.13.215.
- Janssen, P. A. J. 1-Aralkyl-4-(n-Aryl-Carbonyl Amino)-Piperidines and Related Compounds. US Patent 3164600, 1965.
- Helander, A.; Bäckberg, M.; Beck, O. Intoxications Involving the Fentanyl Analogs Acetylfentanyl, 4-Methoxybutyrfentanyl and Furanylfentanyl: Results from the Swedish STRIDA Project. Clin. Toxicol. 2016, 54, 324–332. DOI: 10.3109/15563650.2016.1139715.
- Dwyer, J. B.; Janssen, J.; Luckasevic, T. M.; Williams, K. E. Report of Increasing Overdose Deaths That Include Acetyl Fentanyl in Multiple Countries of the Southwestern Regions of Pennsylvania in 2015-2016. J. Forensic Sci. 2018, 63, 195–200. DOI: 10.1111/1556-4029.13517.
- Katselou, M.; Papoutsis, I.; Nikolaou, P.; Spiliopoulou, C.; Athanaselis, S. Old Opioids, New Concerns: The Case of Acetylfentanyl. Forensic Toxicol. 2016, 34, 201–212. DOI: 10.1007/s11419-016-0310-4.
- McIntyre, I. M.; Trochta, A.; Gary, R. D.; Malamatos, M.; Lucas, J. R. An Acute Acetyl Fentanyl Fatality: A Case Report with Postmortem Concentrations. J. Anal. Toxicol. 2015, 39, 490–494. DOI: 10.1093/jat/bkv043.
- Fort, C.; Curtis, B.; Nichols, C.; Niblo, C. Acetyl Fentanyl Toxicity: Two Case Reports. J. Anal. Toxicol. 2016, 40, 754–757.
- Cunningham, S. M.; Haikal, N. A.; Kraner, J. C. Fatal Intoxication with Acetyl Fentanyl. J. Forensic Sci. 2016, 61, S276–S280. DOI: 10.1111/1556-4029.12953.
- Yonemitsu, K.; Sasao, A.; Mishima, S.; Ohtsu, Y.; Nishitani, Y. A Fatal Poisoning Case by Intravenous Injection of "Bath Salts" Containing Acetyl Fentanyl and 4-Methoxy PV8. Forensic Sci. Int. 2016, 267, e6–e9. DOI: 10.1016/j.forsciint.2016.08.025.
- Takase, I.; Koizumi, T.; Fujimoto, I.; Yanai, A.; Fujimiya, T. An Autopsy Case of Acetyl Fentanyl Intoxication Caused by Insufflation of ‘Designer Drugs. Legal Med. 2016, 21, 38–44. DOI: 10.1016/j.legalmed.2016.05.006.
- McIntyre, I. M.; Trochta, A.; Gary, R. D.; Wright, J.; Mena, O. An Acute Butyr-Fentanyl Fatality: A Case Report with Postmortem Concentrations. J. Anal. Toxicol. 2016, 40, 162–166. DOI: 10.1093/jat/bkv043.
- Weldon, S. T.; Perry, D. F.; Cork, R. C.; Gandolfi, A. J. Detection of Picogram Levels of Sufentanil by Capillary Gas Chromatography. Anesthesiology 1985, 63, 684–687. DOI: 10.1097/00000542-198512000-00021.
- Stevens, R. A.; Petty, R. H.; Hill, H. F.; Kao, T. C.; Schaffer, R.; Hahn, M. B.; Harris, P. Redistribution of Sufentanil to Cerebrospinal Fluid and Systemic Circulation after Epidural Administration in Dogs. Anesth. Analg. 1993, 76, 323–327.
- Bjorksten, A. R.; Chan, C.; Crankshaw, D. P. Determination of Remifentanil in Human Blood by Capillary Gas Chromatography with Nitrogen-Selective Detection. J. Chromatogr. B Analyt. Technol. Biomed. Life Sci. 2002, 775, 97–101. DOI: 10.1016/S1570-0232(02)00178-2.
- Van Nimmen, N. F. J.; Poels, K. L. C.; Veulemans, H. A. F. Highly Sensitive Gas Chromatographic-Mass Spectrometric Screening Method for the Determination of Picogram Levels of Fentanyl, Sufentanil and Alfentanil and Their Major Metabolites in Urine of Opioid Exposed Workers. J. Chromatogr. B 2004, 804, 375–387. DOI: 10.1016/j.jchromb.2004.01.044.
- Van Nimmen, N. F. J.; Veulemans, H. A. F. Development and Validation of a Highly Sensitive Gas Chromatographic-Mass Spectrometric Screening Method for the Simultaneous Determination of Nanogram Levels of Fentanyl, Sufentanil and Alfentanil in Air and Surface Contamination Wipes. J. Chromatogr. A 2004, 1035, 249–259. DOI: 10.1016/j.chroma.2004.02.074.
- Sorokin, V. I.; Semkin, E. P.; Savilov, A. P. Expert Examination of 3-Methylfentanyl. Microgram 1994, 27, 221–226.
- Poklis, J.; Poklis, A.; Wolf, C.; Hathaway, C.; Arbefeville, E.; Chrostowski, L.; Devers, K.; Hair, L.; Mainland, M.; Merves, M.; Pearson, J. Two Fatal Intoxications Involving Butyryl Fentanyl. J. Anal. Toxicol. 2016, 40, 703–708. DOI: 10.1093/jat/bkw048.
- Staeheli, S. N.; Baumgartner, M. R.; Gauthier, S.; Gascho, D.; Jarmer, J.; Kraemer, T.; Steuer, A. E. Time-Dependent Postmortem Redistribution of Butyrfentanyl and Its Metabolites in Blood and Alternative Matrices in a Case of Butyrfentanyl Intoxication. Forensic Sci. Int. 2016, 266, 170–177. DOI: 10.1016/j.forsciint.2016.05.034.
- Breindahl, T.; Kimergård, A.; Andreasen, M. F.; Pedersen, D. S. Identification of a New Psychoactive Substance in Seized Material: The Synthetic Opioid N-Phenyl-N-[1-(2-Phenethyl)Piperidin-4-yl]Prop-2-Enamide (Acrylfentanyl). Drug Test. Analysis 2017, 9, 415–422. DOI: 10.1002/dta.2046.
- Swanson, D. M.; Hair, L. S.; Strauch Rivers, S. R.; Smyth, B. C.; Brogan, S. C.; Ventoso, A. D.; Vaccaro, S. L.; Pearson, J. M. Fatalities Involving Carfentanil and Furanyl Fentanyl: Two Case Reports. J. Anal. Toxicol. 2017, 41, 498–502. DOI: 10.1093/jat/bkx037.
- Misailidi, N.; Athanaselis, S.; Nikolaou, P.; Katselou, M.; Dotsikas, Y.; Spiliopoulou, C.; Papoutsis, I. A GC–MS Method for the Determination of Furanylfentanyl and Ocfentanil in Whole Blood with Full Validation. Forensic Toxicol. 2019, 37, 238–244. DOI: 10.1007/s11419-018-0449-2.
- Melent’ev, A. B.; Kataev, S. S.; Dvorskaya, O. N. Identification and Analytical Properties of Acetyl Fentanyl Metabolites. J. Anal. Toxicol. 2015, 70, 240–248.
- George, A. V.; Lu, J. J.; Pisano, M. V.; Metz, J.; Erickson, T. B. Carfentanil—An Ultra Potent Opioid. Am. J. Emerg. Med. 2010, 28, 530–532. DOI: 10.1016/j.ajem.2010.03.003.
- Haigh, J. C.; Lee, L. J.; Schweinsburg, R. E. Immobilization of Polar Bears with Carfentanil. J. Wildl. Dis. 1983, 19, 140–144. DOI: 10.7589/0090-3558-19.2.140.
- Neumann, G.; Erhardt, W.; Oberhuber, B.; Fritsch, R.; Blumel, G. A New Highly Potent and Short-Acting Analgesic, Carfentanyl (R33799), in Combination with the Hypnotic Agent, Etomidat (r26490) as a Method of Anaesthesia in guinea Pigs. Res. Exp. Med. 1980, 177, 135–143. DOI: 10.1007/BF01851842.
- Baker, J. R.; Gatesman, T. J. Use of Carfentanil and Ketamine-Xylazine Mixture to Immobilize Wild Great Seals (Halichoerus grypus). Vet. Rec. 1985, 116, 208–210. DOI: 10.1136/vr.116.8.208.
- Weckmann, T. J.; Tai, C. L.; Woods, W. E.; Tai, H. H.; Blake, J. W.; Tobin, T. Pharmacological Effects and Detection Methods of Methylated Analogs of Fentanyl in Horses. Am. J. Vet. Res. 1989, 50, 502–507.
- Shafer, S. L. Carfentanil: A Weapon of Mass Destruction. Can. J. Anesth. 2019, 66, 351–355. DOI: 10.1007/s12630-019-01295-x.
- Waite, K.; Deeken, A.; Perch, S.; Kohler, L. J. Current Opioid Trends in Summit County, Ohio. Acad. Forensic Pathol. 2017, 7, 632–639. DOI: 10.23907/2017.053.
- Zibbell, J. E.; Aldridge, A. P.; Cauchon, D.; DeFiore-Hyrmer, J.; Conway, K. P. Association of Law Enforcement Seizures of Heroin, Fentanyl and Carfentanil with Opioid Overdose Deaths in Ohio, 2014-2017. JAMA Netw. Open 2019, 2, e1914666. DOI: 10.1001/jamanetworkopen.2019.14666.
- Cowles, C. E.; Jr., Mitchell, J.; Stepp, J. E.; Bewley, B. Z. Carfentanil: A New and Often Unrecognized Threat. J. Spec. Oper. Med. 2017, 17, 120–122.
- Cole, J. B.; Nelson, L. S. Controversies and Carfentanil: We Have Much to Learn about the Present State of Opioid Poisoning. Am. J. Emerg. Med. 2017, 35, 1743–1745. DOI: 10.1016/j.ajem.2017.08.045.
- Misailidi, N.; Papoutsis, I.; Nikolaou, P.; Dona, A.; Spiliopoulou, C.; Athanaselis, S. Fentanyls Continue to Replace Heroin in the Drug Arena: The Cases of Ocfentanil and Carfentanil. Forensic Toxicol. 2018, 36, 12–32. DOI: 10.1007/s11419-017-0379-4.
- Enders, D.; Shilvock, J. P. Some Recent Applications of α-Amino Nitrile Chemistry. Chem. Soc. Rev. 2000, 29, 359–373. DOI: 10.1039/a908290e.
- Taber, D. F.; Rahimizadeh, M. Amide to Ester Conversion: A Practical Route to the Carfentanil Class of Analgetics. J. Org. Chem. 1992, 57, 4037–4038. DOI: 10.1021/jo00040a061.
- McClure, C. K.; Kiessling, A. J.; Banks, H. D. Synthesis of N-(1-benzyl)-4-Methoxycarbonyl-4-Piperidinyl-N-Phenylpropanamide. Edgewood Research, Development & Engineering Center, Aberdeen Proving Ground, MD. ERDEC-TR-070, May 1993.
- Feldman, P. L.; Brackeen, M. F. A Novel Route to the 4-Anilido-4-(Methoxycarbonyl)Piperidine Class of Analgetics. J. Org. Chem. 1990, 55, 4207–4209. DOI: 10.1021/jo00300a047.
- Malaquin, S.; Jida, M.; Gesquiere, J.-C.; Deprez-Poulain, R.; Deprez, B.; Laconde, G. Ugi Reaction for the Synthesis of 4-Aminopiperidine-4-Carboxylic Acid Derivatives. Application to the Synthesis of Carfentanil and Remifentanil. Tetrahedron Lett. 2010, 51, 2983–2985. DOI: 10.1016/j.tetlet.2010.03.120.
- van Daele, P. G. H.; De Bruyn, M. F. L.; Boey, J. M.; Sanczuk, S.; Agten, J. T. M.; Janssen, P. A. J. Synthetic Analgesics: N-(1-[2-Arylethyl]-4-Substituted 4-Piperidinyl)-N-Arylalkanamides. Arznei-Forschung 1976, 26, 1521–1531. DOI: 10.1002/chin.197646236.
- Niemegeers, C. J.; Schellekens, K. H.; van Bever, W. F.; Janssen, P. A. Sufentanil, a Very Potent and Extremely Safe Intravenous Morphine-Like Compound in Mice, Rats and Dogs. Arznei-Forschung 1976, 26, 1551–1556.
- Monk, J. P.; Beresford, R.; Ward, A. S. A Review of Its Pharmacological Properties and Therapeutic Use. Drugs 1988, 36, 286–313. DOI: 10.2165/00003495-198836030-00003.
- Leysen, J. E.; Gommeren, W.; Niemegeers, C. J. [3H]Sufentanil, a Superior Ligand for mu-Opiate Receptors: Binding Properties and Regional Distribution in Rat Brain and Spinal Cord. Eur. J. Pharmacol. 1983, 87, 209–225. DOI: 10.1016/0014-2999(83)90331-X.
- Shin, D.-Y.; Ryu, J.-S.; Hyun, S.-S.; Park, H.-J.; Jeon, R.-O.; Suh, Y.-G. Total Synthesis of Sufentanil. Arch. Pharm. Res. 1999, 22, 398–400. DOI: 10.1007/BF02979064.
- Corey, E. J.; Chaykovsky, M. Dimethyloxosulfonium Methylide ((CH3)2SOCH2) and Dimethylsulfonium Methylide ((CH3)2SCH2). Formation and Application to Organic Synthesis. J. Am. Chem. Soc. 1965, 87, 1353–1364. DOI: 10.1021/ja01084a03.
- Gillespie, T. J.; Gandolfi, A. J.; Maiorino, R. M.; Vaughan, R. W. Gas Chromatographic Determination of Fentanyl and Its Analogues in Human Plasma. J. Anal. Toxicol. 1981, 5, 133–137. DOI: 10.1093/jat/5.3.133.
- Ferslew, K. E.; Hagardorn, A. N.; McCormick, W. F. Postmortem Determination of the Biological Distribution of Sufentanil and Midazolam after an Acute Intoxication. J. Forensic Sci. 1989, 34, 12630J. DOI: 10.1520/JFS12630J.
- Bennion, B. J.; Be, N. A.; McNerney, M. W.; Lao, V.; Carlson, E. M.; Valdez, C. A.; Malfatti, M. A.; Enright, H. A.; Nguyen, T. H.; Lightstone, F. C.; Carpenter, T. S. Predicting a Drug’s Membrane Permeability: A Computational Model Validated with in Vitro Permeability Assay Data. J. Phys. Chem. B. 2017, 121, 5228–5237. DOI: 10.1021/acs.jpcb.7b02914.
- Koyyalagunta, D. Chapter 113—Opioid Analgesics. Pain Manag. 2007, 2, 939–964. DOI: 10.1016/B978-0-7216-0334-6.50117-5.
- Wandel, C.; Kim, R.; Wood, M.; Wood, A. Interaction of Morphine, Fentanyl, Sufentanil, Alfentanil, and Loperamide with the Efflux Drug Transporter P-Glycoprotein. Anesthesiology 2002, 96, 913–920. DOI: 10.1097/00000542-200204000-00019.
- Ujváry, I.; Jorge, R.; Christie, R.; Le Ruez, T.; Danielsson, H. V.; Kronstrand, R.; Elliott, S.; Gallegos, A.; Sedefov, R.; Evans-Brown, M. Acryloylfentanyl, a Recently Emerged New Psychoactive Substance: A Comprehensive Review. Forensic Toxicol. 2017, 35, 232–243. DOI: 10.1007/s11419-017-0367-8.
- Maryanoff, B. E.; Simon, E. J.; Gioannini, T.; Gorissen, H. Potential Affinity Labels for the Opiate Receptor Based on Fentanyl and Related Compounds. J. Med. Chem. 1982, 25, 913–919. DOI: 10.1021/jm00350a006.
- Pierzynski, H. G.; Neubauer, L.; Choi, C.; Franckowski, R.; Augustin, S. D.; Iula, D. M. Tips for Interpreting GC-MS Fragmentation of Unknown Substituted Fentanyls. Cayman Curr. 2017, 28, 1–3.
- Valdez, C. A.; Leif, R. N.; Hok, S.; Alcaraz, A. Assessing the Reliability of the NIST Library during Routine GC-MS Analyses: Structure and Spectral Data Corroboration for 5,5-Diphenyl-1,3-Dioxolan-4-One during a Recent OPCW Proficiency Test. J. Mass Spectrom. 2018, 53, 419–422. DOI: 10.1002/jms.4073.
- Moorthy, A. S.; Kearsley, A. J.; Mallard, W. G.; Wallace, W. E. Mass Spectral Similarity Mapping Applied to Fentanyl Analogs. Forensic Chem. 2020, 19, 100237. DOI: 10.1016/j.forc.2020.100237.
- Guerrieri, D.; Rapp, E.; Roman, M.; Thelander, G.; Kronstrand, R. Acrylfentanyl: another New Psychoactive Drug with Fatal Consequences. Forensic Sci. Int 2017, 277, e21–e29. DOI: 10.1016/j.forsciint.2017.05.010.
- UNODC. Recommended Methods for the Identification and Analysis of Fentanyl and Its Analogues in Biological Specimens. United Nations Office on Drugs and Crime, Vienna, 2017. DOI: 10.18356/aca7aca5-en.
- Bäckberg, M.; Beck, O.; Jönsson, K.-H.; Helander, A. Opioid Intoxications Involving Butyrfentanyl, 4-Fluorobutyrfentanyl, and Fentanyl from the Swedish STRIDA Project. Clin. Toxicol. 2015, 53, 609–615. DOI: 10.3109/15563650.2015.1054505.
- Cole, J. B.; Dunbar, J. F.; McIntire, S. A.; Regelmann, W. E.; Slusher, T. M. Butyrfentanyl Overdose Resulting in Diffuse Alveolar Hemorrhage. Pediatrics 2015, 135, e740–e743. DOI: 10.1542/peds.2014-2878.
- Bürkle, H.; Dunbar, S.; Van Aken, H. Remifentanil: A Novel, Short-Acting, Mu-Opioid. Anesth. Analg. 1996, 83, 646–651. DOI: 10.1097/00000539-199609000-00038.
- Patel, S. S.; Spencer, C. M. Remifentanil. Drugs 1996, 52, 417–427. DOI: 10.2165/00003495-199652030-00009.
- Rosow, C. E. An Overview of Remifentanil. Anesth. Analg. 1999, 89, S1–S3. DOI: 10.1097/00000539-199910001-00001.
- Levine, A. I.; Bryson, E. O. Intranasal Self-Administration of Remifentanil as the Foray into Opioid Abuse by an Anesthesia Resident. Anesth. Analg. 2010, 110, 524–525. DOI: 10.1213/ANE.0b013e3181c5f069.
- Feldman, P. L.; James, M. K.; Brackeen, M. F.; Johnson, M. R.; Leighton, H. J. US Patent. 5019583, 1991.
- Cheng, B. K.-M.; Halvachs, R. E. Process for Remifentanil Synthesis. US Patent 2010048908, 2010.
- Selinger, K.; Lanzo, C.; Sekut, A. Determination of Remifentanil in Human and Dog Blood by HPLC with UV Detection. J. Pharm. Biomed. Anal. 1994, 12, 243–248. DOI: 10.1016/0731-7085(94)90035-3.
- Haidar, S. H.; Liang, Z.; Selinger, K.; Hamlett, L.; Eddington, N. D. Determination of Remifentanil, an Ultra-Short-Acting Opioid Anesthetic, in Rat Blood by High Performance Liquid Chromatography with Ultraviolet Detection. J. Pharm. Biomed. Anal. 1996, 14, 1727–1732. DOI: 10.1016/0731-7085(96)01831-6.
- Coopman, V.; Cordonnier, J.; De Leeuw, M.; Cirimele, V. Ocfentanil Overdose Fatality in the Recreational Drug Scene. Forensic Sci. Int. 2016, 266, 469–473. DOI: 10.1016/j.forsciint.2016.07.005.
- Dussy, F. E.; Hangartner, S.; Hamberg, C.; Berchtold, C.; Scherer, U.; Schlotterbeck, G.; Wyler, D.; Briellmann, T. A. An Acute Ocfentanil Fatality: A Case Report with Postmortem Concentrations. J. Anal. Toxicol. 2016, 40, 761–766. DOI: 10.1093/jat/bkw096.
- Casati, S.; Minoli, M.; Angeli, I.; Ravelli, A.; Crudele, G. D. L.; Orioli, M. An Ocfentanil‐Related Death Case: UHPLC–MS/MS Analysis of the Drug. Drug Test. Anal. 2019, 11, 173–177. DOI: 10.1002/dta.2473.
- Klar, S. A.; Brodkin, E.; Gibson, E.; Padhi, S.; Predy, C.; Green, C.; Lee, V. Notes from the Field: Furanyl-Fentanyl Overdose Events Caused by Smoking Contaminated Crack Cocaine—British Columbia, Canada, July 15–18, 2016. Mmwr. Morb. Mortal. Wkly. Rep. 2016, 65, 1015–1016. DOI: 10.15585/mmwr.mm6537a6.
- Huang, B.-S.; Terrell, R. C.; Deutsche, K. H.; Kudzma, L. V.; Lalinde, N. L. N-Aryl-N-(4-Piperidinyl)Amides and Pharmaceutical Compositions and Method Employing Such Compounds. US Patent 4584303A 1986.
- Martin, M.; Hecker, J.; Clark, R.; Frye, J.; Jehle, D.; Lucid, E. J.; Harcheiroad, F. China White Epidemic: An Eastern United States Emergency Department Experience. Ann. Emerg. Med. 1991, 70, 158–164. DOI: 10.1016/S0196-0644(05)81216-8.
- Hibbs, J.; Perper, J.; Winek, C. L. An Outbreak of Designer Drug-Related Deaths in Pennsylvania. JAMA 1991, 265, 1011–1013. DOI: 10.1001/jama.1991.03460080081037.
- Kram, T. C.; Cooper, D. A.; Allen, A. C. Behind the Identification of China White. Anal. Chem. 1981, 53, 1379A–1386A. DOI: 10.1021/ac00235a790.
- Henderson, G. L. Fentanyl-Related Deaths: Demographics, Circumstances, and Toxicology of 112 Cases. J. Forensic Sci. 1991, 36, 13045J. DOI: 10.1520/JFS13045J.
- Vuckovic, S.; Prostran, M.; Ivanovic, M.; Dosen-Micovic, L.; Todorovic, Z.; Nesic, Z.; Stojanovic, R.; Divac, N.; Mikovic, Z. Fentanyl Analogs: Structure-Activity-Relationship Study. CMC. 2009, 16, 2468–2474. DOI: 10.2174/092986709788682074.
- Henderson, G. L. Designer Drugs: Past History and Future Prospects. J. Forensic Sci. 1988, 33, 11976J. DOI: 10.1520/JFS11976J.
- Ojanperä, I.; Gergov, M.; Liiv, M.; Riikoja, A.; Vuori, E. An Epidemic of Fatal 3-Methylfentanyl Poisoning in Estonia. Int. J. Legal Med. 2008, 122, 395–400. DOI: 10.1007/s00414-008-0230-x.
- Ojanperä, I.; Gergov, M.; Rasanen, I.; Lunetta, P.; Toivonen, S.; Tiainen, E.; Vuori, E. Blood Levels of 3-Methylfentanyl in 3 Fatal Poisoning Cases. Am. J. Forensic Med. Pathol. 2006, 27, 328–331. DOI: 10.1097/01.paf.0000188097.78132.e5.
- Sofalvi, S.; Schueler, H. E.; Lavins, E. S.; Kaspar, C. K.; Brooker, I. T.; Mazzola, C. D.; Dolinak, D.; Gilson, T. P.; Perch, S. An LC–MS-MS Method for the Analysis of Carfentanil, 3-Methylfentanyl, 2-Furanyl Fentanyl, Acetyl Fentanyl, Fentanyl and Norfentanyl in Postmortem and Impaired-Driving Cases. J. Anal. Toxicol. 2017, 41, 473–483. DOI: 10.1093/jat/bkx052.
- Kanamori, T.; Iwata, Y. T.; Segawa, H.; Yamamuro, T.; Kuwayama, K.; Tsujikawa, K.; Inoue, H. Characterization and Differentiation of Geometric Isomers of 3-Methylfentanyl Analogs by Gas Chromatography/Mass Spectrometry, Liquid Chromatography/Mass Spectrometry, and Nuclear Magnetic Resonance Spectroscopy. J. Forensic Sci. 2017, 62, 1472–1478. DOI: 10.1111/1556-4029.13395.
- Fogarty, M. F.; Papsun, D. M.; Logan, B. K. Analysis of Cis and Trans 3-Methylfentanyl by Liquid Chromatography-High Resolution Mass Spectrometry and Findings in Forensic Toxicology Casework. Drug Test. Anal. 2018, 10, 1474–1482. DOI: 10.1002/dta.2414.