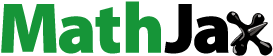
Abstract
Boswellia resin is an exudate from the cut bark of Boswellia trees. The main constituents of pharmacological interest are boswellic acids (pentacyclic triterpenoids), namely α-boswellic acid, β-boswellic acid, 3-O-acetyl-α-boswellic acid, 3-O-acetyl-β-boswellic acid, 11-keto-β-boswellic acid, and 3-O-acetyl-11-keto-β-boswellic acid. Nowadays, dietary supplements with Boswellia serrata extract are used in the treatment of inflammatory joint diseases. Additionally, the constituents of Boswellia resin have shown potential for the treatment of other chronic inflammatory diseases and various types of cancer. Separation methods including ultra/high-performance liquid chromatography, gas chromatography, thin layer chromatography, supercritical fluid chromatography, and capillary electrochromatography coupled with UV or MS detection have been used for the determination of boswellic acids in various matrices (mostly plant material and biological samples). This review aims to provide a comprehensive summary of these separation methods, offering a critical discussion of their strengths and limitations in the analysis of boswellic acids. The knowledge of various separation methods plays a pivotal role in the quality control of herbal dietary supplements and the monitoring of the metabolism and pharmacokinetics of their constituents. The approaches based on metabolomics and network pharmacology represent new ways of fingerprinting secondary metabolites in Boswellia resin increasing the comprehensiveness of the output of these methods resulting in safer dietary supplements.
Introduction
Boswellia species are trees or shrubs of the Burseraceae family, which are native to North and East Africa, India, and the Middle East.[Citation1,Citation2] Boswellia plants exude the resin when their bark is wounded. This resin, also known as frankincense or olibanum, is harvested from the trees by tapping. Historically, Boswellia resin has been used as incense in religious ceremonies, in cultural traditions, as an important component in cosmetic products, in adhesives, or as a coating material.[Citation1,Citation3] The medicinal properties of Boswellia (Indian Frankincense) have been recognized for centuries. Ayurveda, a traditional Indian system of medicine, describes the use of Boswellia for the treatment of inflammatory diseases, gastrointestinal disorders, and respiratory diseases.[Citation1,Citation4] Nowadays, frankincense has been shown to be effective in the treatment of several chronic diseases, including asthma, Crohn’s disease, rheumatoid arthritis, and osteoarthritis.[Citation4–7] Moreover, the anti-neoplastic activity of Boswellia has also been reported.[Citation2,Citation6,Citation8]
Composition of Boswellia oleo-gum resin
The qualitative and quantitative composition of oleo-gum resin depends on the parent plant, climate, geographic location, and harvesting conditions.[Citation9] Generally, the resin of Boswellia sp. is composed of essential oil (5–9%), mucinous fraction (6–20%), and resinous fraction (50–60%).[Citation10] The Boswellia serrata oleogum contains 30–60% resin, 5-10% essential oils, and the rest is made up of polysaccharides (∼ 65% arabinose, galactose, and xylose).[Citation1,Citation11] According to the review paper written by Shah and coworkers,[Citation12] the B. serrata gum resin is composed of 25–35% of higher terpenoids, 8–12% of essential oils, 45–60% of polysaccharides, and small amounts of proteins and inorganic compounds.[Citation12] The resinous part of Boswellia resin contains a variety of terpenoid compounds, including monoterpenes, diterpenes (incensole, incensole acetate, incensole oxide, isoincensole oxide), triterpenes (α-amyrin, β-amyrin), tetracyclic triterpenic acids (3-oxo-tirucallic acid, 3-acetoxy-tirucallic acid, 3-hydroxy-tirucallic acid), and pentacyclic triterpenic acids (boswellic acids, lupeolic acid, acetyl-lupeolic acid).[Citation1,Citation11,Citation13,Citation14] Many of them contribute to anti-inflammatory [Citation2,Citation14,Citation15] and anti-tumor effects [Citation2,Citation8] of the resin or have their own medicinal effects, such as incensole and incensole acetate, which have been reported to have antidepressant activity.[Citation15] The mixture of 3α,24-dihydroxyurs-12-ene and 3α,24-dihydroxyolean-12-ene known as pentacyclic triterpenediol, another constituent of crude Boswellia extracts, has a significant potential in the treatment of various types of cancer.[Citation16,Citation17] The insight into the biosynthetic pathways of triterpenes in the genus Boswellia has been reviewed by Al-Harrasi et al.[Citation10]
Chemistry of boswellic acids
Boswellic acids (BA) represent some of the major active components of Boswellia resins. Structurally they belong to the group of pentacyclic triterpenoids. Six major BAs present in varying quantities in all Boswellia species (see and Table S1) are α-boswellic acid (αBA), β-boswellic acid (βBA), their acetylated forms 3-O-acetyl-α-boswellic acid (AαBA) and 3-O-acetyl-β-boswellic acid (AβBA), and the keto-derivatives 11-keto-β-boswellic acid (KBA) and 3-O-acetyl-11-keto-β-boswellic acid (AKBA).[Citation1,Citation11,Citation12,Citation19] The ursane type (β-boswellic acids) and the oleane type (α-boswellic acids) isomers differ in the position of two methyl groups at C-19/C-20. The basic skeleton comprises a hydroxy group at C-3, carboxylic acid moiety at C-4, and one double bond (C12-C13). KBA and AKBA contain a keto group at position 11. This structural feature represents a strong chromophore that allows their UV detection at 250 nm, while the other BAs absorb at 208 nm.[Citation1] The hydroxy group at C-3 can be acetylated, giving the naturally occurring acetylated forms. The chemical structures are shown in and the physico-chemical properties of six major BAs are summarized in . Other BAs identified in gum resin of Boswellia species are 9,11-dehydro-α-BA, 9,11-dehydro-β-BA, and their acetylated forms.[Citation13] The synthetic modifications of BAs yielding a variety of structural analogs or derivatives and their biological activities are discussed in previously published reviews.[Citation12,Citation22]
Figure 1. Content of major boswellic acids in Boswellia oleo-gum resins expressed in % (w/w). This figure was prepared from data presented in Table 2 in ref.[Citation18]
![Figure 1. Content of major boswellic acids in Boswellia oleo-gum resins expressed in % (w/w). This figure was prepared from data presented in Table 2 in ref.[Citation18]](/cms/asset/68ab1ad6-06f1-4bc5-bc91-e32c3d6fbd81/batc_a_2312502_f0001_c.jpg)
Figure 2. Chemical structures and IUPAC names of six BAs.[Citation20]
![Figure 2. Chemical structures and IUPAC names of six BAs.[Citation20]](/cms/asset/6133a96b-16c4-4b3c-9d80-3db9ce827e01/batc_a_2312502_f0002_b.jpg)
Table 1. Physico-chemical properties of six BAs; pKa, log P, and MW from SciFinder, [Citation21] monoisotopic MW from ChemSpider, [Citation20] λmax from ref. [Citation1].
Requirements of pharmacopeias related to pharmacological activity
The 11th Edition of the European Pharmacopeia (Ph. Eur.) contains a monograph on Indian frankincense (Olibanum indicum),[Citation23] while the Dietary Supplements section of the United States Pharmacopeia 39 (USP) involves two monographs on Boswellia serrata and Boswellia serrata extract.[Citation24] Both Ph. Eur. and USP use thin-layer chromatography for the identification of keto derivates of β-boswellic acids (KBA and AKBA),[Citation23,Citation24] while the USP requires also the identification of zones of β-boswellic acid and 3-O-acetyl-β-boswellic acid.[Citation24] The assay of KBA and AKBA in Indian frankincense is carried out by high-performance liquid chromatography according to both pharmacopeias.[Citation23,Citation24] In Ph. Eur. and USP,[Citation23,Citation24] as well as in the Indian Pharmacopeia,[Citation25] Boswellia serrata is the only official species of frankincense, in contrast to the Chinese Pharmacopeia, where Boswellia carterii and Boswellia sacra are the official species.[Citation26] It must be critically pointed out that the methods prescribed by the Ph. Eur. and USP do not follow the current knowledge regarding the pharmacological activity and bioavailability of BAs. One of the first hypotheses on the anti-inflammatory effect of BAs was based on the reduced formation of leukotriene (LT) due to the inhibition of 5-lipooxygenase (5-LO) by KBA and AKBA.[Citation1] However, this is only valid for in vitro test models, whereas the experiment with whole blood that mimics in vivo activity showed the loss of the 5-LO inhibition. This loss of activity may be caused by the low bioavailability [Citation19] of KBA and AKBA accompanied by the strong plasma protein binding of KBA and AKBA resulting in insufficient plasma concentrations and consequently low 5-LO inhibition.[Citation27] Later, microsomal prostaglandin E2 synthase-1 (mPGES1) was described as another molecular basis for the anti-inflammatory activity of BAs. βBA was identified as the most active BA in the reduction of mPGES1 in cell-based assay as well as in vivo in a mouse model. Both KBA and AKBA failed to suppress mPGES1 due to the low bioavailability and strong plasma protein binding.[Citation28] Human cathepsin G (catG) has been described as another molecular target for BAs. Also, βBA and AβBA were found to inhibit catG with similar IC50 values (0.8 µM βBA, 1.2 µM AβBA) as for KBA (3.7 µM) and AKBA (0.6 µM). Since the determined plasma concentrations (n = 3) were significantly higher for the BAs lacking the keto group (6.35 ± 1.0 µM for βBA and 4.90 ± 0.5 µM for AβBA) than for KBA (0.33 ± 0.1 µM) and AKBA (0.04 ± 0.01 µM), the non-keto derivatives of BAs can be regarded as anti-inflammatory secondary metabolites that should also be covered by official methods for the characterization of frankincense.[Citation29] Therefore, the pharmacopeial analytical methods used for the quality control of frankincense should be adapted to detect and quantify other BAs, such as βBA, as well. Moreover, other minor constituents of frankincense such as tirucallic, lupeolic, and roburic acids were found to be highly potent in inhibiting mPGES1 indicating that other constituents of the resin may also play a role in its anti-inflammatory activity.[Citation30]
In an in vivo study involving mice with streptozotocin-induced diabetes, Shehata et al.[Citation31] demonstrated that Boswellia serrata extract effectively mitigated the elevated levels of pro-inflammatory cytokines IL-1α, IL-1β, IL-2, IL-6, IFN-γ, and TNF-α induced by streptozotocin treatment. The authors hypothesized that the mechanism behind this effect may involve the reduction of the stimulating effect of colony-stimulating factors on granulocytes or the inhibition of NFκB release from these cells. However, the exact components of the extract contributing to that effect were not identified. Nevertheless, this study shows the potential of Boswellia serrata extract to reduce hyperglycemia in diabetes type 1.[Citation31]
The objective of this review
The importance of developing analytical methods for the analysis of dietary supplements containing Boswellia extracts is emphasized by Meins et al.,[Citation32] who surveyed the quality of such products sold in European and American markets. Of the six US and eleven European dietary supplements tested, seven did not comply with the label claims. One European dietary supplement did not contain any of the six major boswellic acids, and one American product contained them only in trace amounts. The ratios of the individual boswellic acids in some products indicated the use of extracts from different Boswellia species instead of Boswellia serrata. The undeclared use of AKBA-enriched resin was suspected in some dietary supplements.[Citation32] In another study, Börner and coworkers confirmed various qualities of three dietary supplements that were tested for both the content of BAs and in vitro activity. Two products showed typical distributions of BAs profile and higher amounts of non-acetylated/non-ketylated BAs that was in agreement with the typical composition of Boswellia serrata extract. The last product showed a significantly lower amount of boswellic acids (around two orders of magnitude lower calculated as the sum of BAs) with another distribution of individual components. Finally, the dietary supplement with the low amount of boswellic acids was much less efficient against 5-LO and mPGES-1 compared to high-quality products.[Citation33]
These two above-mentioned examples [Citation32,Citation33] clearly demonstrate the need for high-performance separation methods for (i) the state-of-the-art quality control of the olibanum-based dietary supplements, (ii) fingerprinting of terpenoids in frankincense of different origins, and (iii) monitoring the pharmacokinetics and metabolism of its active components. So far, Xu and coworkers [Citation34] summarized the literature describing the methods for the analysis of pentacyclic triterpenoids in medicinal plants, but the methods for BAs analysis were only marginally described. Other reviews dealing with the phytochemistry and/or pharmacology of boswellic acids summarize only a few selected analytical methods for the determination of boswellic acids,[Citation12,Citation35] including pharmacokinetic studies.[Citation7,Citation19] A review of current trends in the analysis of bioactive terpenoids was recently published by Das et al.[Citation36] However, only five analytical methods for the determination of triterpenoids were mentioned, none of which were used for the analysis of boswellic acids. Thus, our work aims to systematically and critically summarize the separation methods used for the analysis of boswellic acids in various matrices to fill the gap in the scientific literature.
The literature survey was conducted on Web of Science using the following key words individually and/or in combination: Boswellia, boswellic, TLC, GC, LC, CE, SFC, and chromatography. Matches found were sorted by title and abstract for relevance to analytical chemistry. The full texts of relevant hits were downloaded and key information on the analytical methods used were listed in the tabulated forms. The general workflow and current trends in the analysis of BAs by various separation methods are described and discussed in our review. The findings of the scientifically most significant articles are presented in the context of the state-of-the-art knowledge of BAs chemistry and pharmacology. Literature from 1989 up to May 2023 was covered.
Liquid chromatography-based methods in the analysis of boswellic acids
High-performance liquid chromatography (HPLC) and ultra high-performance liquid chromatography (UHPLC) are the most widely used separation methods in the analysis of BAs. The separation conditions and the main attributes of the reviewed works are listed in .[Citation18,Citation23,Citation24,Citation29,Citation33,Citation37–77]
Table 2. LC-based techniques used in the analysis of BAs.
Separation selectivity in HPLC and UHPLC
The HPLC separation of BAs is usually carried out using C18 or C8 reversed-phase columns. A binary mixture of acidified water and organic solvents such as acetonitrile or methanol has typically been used as the mobile phase for the HPLC analysis of BAs. However, Tausch et al.[Citation29] used a water-acetonitrile mobile phase with the addition of a small amount of ammonia to separate four boswellic acids: KBA, AKBA, βBA, and AβBA. The alkaline pH of the mobile phase increases the fraction of deprotonated BA molecules, which reduces their retention on C18 columns resulting in retention times of 3.70 min (KBA), 4.13 min (AKBA), 4.19 min (βBA), and 4.34 min (AβBA), respectively.[Citation29] However, the higher fraction of ionized boswellic acid molecules should facilitate their ionization in the negative electrospray mode, which would have a positive effect on the sensitivity of the method. Zhang et al.[Citation76] also used an alkaline water: acetonitrile mobile phase for the analysis of β-elemonic acid (also known as 3-oxo-tirucallic acid).
Most of the HPLC and UHPLC methods utilized gradient elution to improve the separation of various BAs among other monitored compounds, such as tirucallic and lupeolic acids, amyrins, and flavonoids. The typical elution order on C8 or C18 columns using a binary mixture of water and acetonitrile or methanol at acidic pH was as follows: KBA, AKBA, αBA, βBA, AαBA, AβBA which corresponds to the log P values given in (see Introduction). Under these conditions, baseline separation of these analytes is generally achieved. However, Singh et al.[Citation42] described a different elution order under similar conditions, where acetylated forms of boswellic acids were eluted before the non-acetylated forms: AKBA, KBA, AαBA, AβBA, αBA, βBA. Most HPLC separations took longer than 20 min, depending on the purpose of the method. Using the UHPLC technique, much shorter retention times, higher separation efficiency, and improved separation selectivity could be achieved. For example, Ai et al.[Citation75] achieved a baseline separation of 5 boswellic acids (AKBA was not included) with a 9.5 min gradient method, with the retention time of the last peak of only 5.94 min. As discussed above, Tausch et al.[Citation29] described the method that allows rapid analysis of boswellic acids using conventional HPLC.
The fluorinated stationary phase was applied for the separation of isomeric acetyl-11-keto-α-boswellic acid (AKαBA) and AKBA from the chick chorioallantoic membrane.[Citation70] Compared to the commonly used C18 columns, the elution order of AKαBA and AKBA was reversed when using the fluorinated stationary phase. The obtained results also show an improved selectivity for these analytes on the fluorinated stationary phase compared to conventionally used C18 columns ().[Citation70]
Figure 3. Separation of the AKBA (1) solution with a 5% (w/w) impurity of AKαBA (2) on two stationary phases: (a) HS F5 (fluorinated stationary phase, 150 × 4 mm, 5 μm) (b) Reprosil PUR ODS-3 (C18 stationary phase, 250 × 3 mm, 5 μm), detection at 250 nm. Figure reproduced from [Citation70] with permission from Elsevier.
![Figure 3. Separation of the AKBA (1) solution with a 5% (w/w) impurity of AKαBA (2) on two stationary phases: (a) HS F5 (fluorinated stationary phase, 150 × 4 mm, 5 μm) (b) Reprosil PUR ODS-3 (C18 stationary phase, 250 × 3 mm, 5 μm), detection at 250 nm. Figure reproduced from [Citation70] with permission from Elsevier.](/cms/asset/3ada12c5-433c-4aac-bef7-41b9597dfe92/batc_a_2312502_f0003_c.jpg)
A more complex approach based on the off-line 2D-LC method was used for the qualitative and quantitative analysis of 17 triterpenoids in Boswellia papyrifera.[Citation43] The analysis in the first dimension was carried out on two C18 columns (250 × 4.6 mm, 5 μm) connected in series using a binary mixture of methanol and water with 0.1% trifluoroacetic acid (TFA) as the mobile phase under gradient elution. This allowed the quantification of 13 triterpenic acids. The peak corresponding to β-boswellic acid showed signs of inhomogeneity, so the authors applied the second LC dimension for its complete separation from co-eluting compounds. Fractions corresponding to peaks of β-boswellic acid, α-boswellic acid, and 3-O-acetyl-11-methoxy-β-boswellic acid (the latter two were used as control standards) were collected and later analyzed on the same C18 column (250 × 4.6 mm, 5 μm) with pure acetonitrile as mobile phase in the second dimension. The two compounds that coeluted with βBA in the first dimension were successfully separated in the second dimension. After their isolation and spectroscopic analysis, these coeluting compounds were identified as 3-α-O-acetyl-7,24-dien-tirucallic acid and 3-β-O-acetyl-8,24-dien-tirucallic acid. This off-line 2D-LC method allowed the accurate quantification of βBA in the acidic fraction of Boswellia papyrifera.[Citation43]
Detection in HPLC and UHPLC assays
HPLC with UV detection has been widely applied for the analysis of crude herbal extracts and commercial formulations. Due to the presence of a strong chromophore – a keto group – KBA and AKBA have their absorption maximum at roughly 250 nm, which is the most commonly used wavelength for the detection of these two compounds. Boswellic acids that do not possess a keto group have a lower overall absorbance and their absorption maximum is shifted to about 200 nm [Citation62] which reduces the selectivity of the detection. The use of alternative wavelengths for the detection of certain BAs has also been described, specifically the detection of αBA at 280 nm [Citation51] and AKBA at 420 nm.[Citation60] However, these values significantly differ from the reported λmax of these compounds,[Citation1] therefore, the feasibility of UV detection under these conditions is questionable. The lower sensitivity and selectivity of UV detection may be a limiting factor in the more advanced analyses, such as fingerprinting of Boswellia species, and in the analysis of more complex matrices such as plasma samples.
Therefore, LC-ESI-MS also represents a standard tool in the analysis of BAs,[Citation18,Citation29,Citation33,Citation37,Citation42,Citation44–46,Citation49,Citation52–54,Citation58,Citation64,Citation66,Citation72–77] while atmospheric pressure chemical ionization that is considered more suitable for the LC-MS analysis of hydrophobic compounds has only been applied in two studies.[Citation69,Citation71] Surprisingly, Liu et al.[Citation45] claim that ESI+ provides better sensitivity for all boswellic acids which is not the expected MS behavior for acidic compounds. This phenomenon is explained by the formation of intramolecular hydrogen bonds between the 3-OH and 4-COOH groups resulting in low production of [M-H]− ions. The authors used acetonitrile and water, each containing 0.01% acetic acid, as the mobile phase.[Citation45] However, these results contradict the previous research by Frank et al.,[Citation64] who used a mobile phase based on 10 mM ammonium acetate/0.05% acetic acid in water and acetonitrile. According to the results of Frank et al.,[Citation64] only KBA and AKBA were detected in ESI+ with acceptable sensitivity, whereas all six major boswellic acids were detected with similarly high sensitivity in ESI− (see ).[Citation64] Both papers state that ESI+ allows to achieve better sensitivity for keto-boswellic acids,[Citation45,Citation64] which was also confirmed by Wang et al.[Citation73] In ESI+ mode, keto-boswellic acids tend to produce [M + H]+ ions because of the protophilic properties of the 11-keto groups. The sodium adducts are more typical for BAs lacking an 11-keto group.[Citation45] The deprotonated molecules [M − H]− are typical for ESI-,[Citation52,Citation72] but the formation of [M + CH3COO]− has also been described.[Citation42] Selected ion monitoring (SIM) or selected reaction monitoring (SRM) modes have been used for the quantification of BAs. Depending on the ESI polarity, the m/z corresponding to either protonated or deprotonated molecules were used for SIM quantification.[Citation33,Citation72,Citation73]
Figure 4. Total ion current chromatogram obtained after the SPE-LC/ESI-MS analysis of a mixture of the boswellic acid standards in the SIM ESI- mode (A) and the SIM ESI+ mode (B). Elution order: 1-KBA, 2-AKBA, 3-αBA, 4-βBA, 5-AαBA, 6-AβBA (25 µmol/L each). Figure reproduced from [Citation64] with permission from Elsevier.
![Figure 4. Total ion current chromatogram obtained after the SPE-LC/ESI-MS analysis of a mixture of the boswellic acid standards in the SIM ESI- mode (A) and the SIM ESI+ mode (B). Elution order: 1-KBA, 2-AKBA, 3-αBA, 4-βBA, 5-AαBA, 6-AβBA (25 µmol/L each). Figure reproduced from [Citation64] with permission from Elsevier.](/cms/asset/835e5d76-7b18-4fdb-9140-59ea73fb799a/batc_a_2312502_f0004_b.jpg)
MS/MS was applied in almost all cases with either QqQ or Q-ToF mass analyzers. The combination of U/HPLC with MS detection provides high selectivity, which is not only advantageous for the analysis of complex biological samples but also for the analysis of Boswellia resins and resin extracts, which may contain other isomeric terpenoids that cannot be distinguished without selective separation. For example, αBA, βBA, lupeolic acid, 3-β-hydroxy-7,24-dien-tirucallic acid, 3-α-hydroxy-7,24-dien-tirucallic acid, and 3-α-hydroxy-8,24-dien-tirucallic acid have the same exact mass of 456.3603. Keto-ursolic acid is isomeric with KBA (m = 470.3396), while 3-O-acetyl-oleanolic and 3-O-acetyl-ursolic acids have the same exact mass as AαBA and AβBA (m = 498.3709).[Citation53]
The typical fragmentation path of boswellic acids () includes a loss of water (−18 Da), loss of CO2 (−44), and the retro-Diels-Alder cleavage.[Citation37] Both AKBA and KBA produce a characteristic ion of m/z = 407, which is formed by the loss of CO2 and H2O in the case of KBA, and by the loss of CO2 and AcOH in the case of AKBA.[Citation37] The intensity of particular fragments strongly depends on selected MS/MS parameters.
Figure 5. Fragmentation path of KBA. Reprint with permission from Ref.[Citation37]
![Figure 5. Fragmentation path of KBA. Reprint with permission from Ref.[Citation37]](/cms/asset/fa6fc9d9-3c2a-4cdf-ad40-a2936dd2dcda/batc_a_2312502_f0005_b.jpg)
The SRM transitions in positive and negative ion modes that were described as optimal are listed in . While most research papers use the same SRM transitions for α- and β-isomers, Xie et al.[Citation58] describe the use of different transitions to distinguish the isomers that were not completely separated by means of LC. However, it is important to note that in this study, the masses of the precursor ions selected for αBA, βBA, and AαBA correspond to the monoisotopic molecular weights of these analytes, rather than to the expected [M + H]+. In addition, a mass difference of 1 Da can be observed between the precursor ions of isomeric AαBA and AβBA (see ). Therefore, the correctness of such an approach is questionable.
Table 3. SRM transitions used in the analysis of 6 main boswellic acids. −, negative ion mode; +, positive ion mode.
Thanks to the lower LOD and LOQ of MS detection compared to UV detection (see Table S2), MS detection can be used to study biological samples with low levels of BAs, such as in pharmacokinetic studies that require selective and sensitive techniques. MS detection provides reliable identification and structural information about BAs. It can be used to determine BAs in complex samples, even when baseline separation is not achieved. In general, this last statement is not true for isomeric compounds unless different transitions can be obtained.
A somewhat limiting factor in LC-MS quantitative analysis of boswellic acids is the lack of commercially available stable isotopically labeled standards (SIL-IS). Some research papers describe the use of non-isotopically labeled IS, for example, glycyrrhetinic acid,[Citation58] 2,5-dimethyl-celecoxib,[Citation29] fluoxymesterone,[Citation72] or ursolic acid,[Citation77] but these can only be considered as IS from an LC perspective.
The HPLC method was also used to confirm the results of quantification of KBA obtained by near-infrared spectroscopy (NIRS) in the range of 700–2500 nm coupled with partial least squares (PLS) regression analysis.[Citation50] The online coupling of HPLC with NMR in the analysis of BAs was rare and only described in one paper.[Citation45] The other BA-focused papers that discuss both LC and NMR utilize these techniques separately or coupled off-line.[Citation55,Citation56]
The fluorescence detection was used in one HPLC method for the analysis of archaeological samples. The samples were derivatized with dansyl chloride prior to analysis and detected using the excitation and emission wavelengths of 347 nm and 530 nm, respectively. For the derivatization of archaeological samples available in small quantities, the use of fluorometric detection is a good alternative to GC analysis.[Citation59]
Types of samples and sample preparation for LC assays
The analyzed material was in most cases plant material from different Boswellia species or commercial products containing BAs. In this case, sample preparation consisted mainly of nonselective extraction in a polar organic solvent such as MeOH followed by filtration and dilution,[Citation45,Citation46,Citation52] often with a centrifugation step implemented.[Citation18] Paul et al.[Citation43] used the procedure of pH-dependent liquid-liquid extraction (LLE) to separate the acidic fraction from interfering matrix components in two-dimensional (2D) HPLC method developed for the separation of boswellic, lupeolic, and tirucallic acids, all of which may be present in detectable amounts in Boswellia extracts.
Another category of samples analyzed for BAs content were biological tissues and fluids of human, rat, and mouse origin, which require a different approach in terms of sample preparation.[Citation69,Citation71,Citation74] For the extraction of BAs from biological samples after oral administration, solid phase extraction (SPE),[Citation67,Citation74] LLE,[Citation75] and supported liquid extraction (SLE, referred to as matrix-assisted LLE by the authors [Citation69,Citation70,Citation72]) were used more frequently compared to their use in the analysis of herbal extracts. The average extraction recoveries of these methods are listed in Table S3.
In one case, SLE on diatomaceous earth was combined with solid phase extraction on a graphitized carbon black surface, which allowed effective purification of the human plasma samples prior to HPLC analysis. The mean extraction efficiency of this method was 66%.[Citation68] The method was later applied for the preparation of in vivo samples in a study investigating the metabolic stability and identification of metabolites of BAs in biological samples.[Citation71]
The LC-MS analysis after automated on-line SPE extraction (in that work referred to as LC/LC/ESI-MS) was presented by Frank et al.[Citation64] for fingerprint analyses of characteristic BAs in different frankincense samples. A C8 extraction column with a particle size of 5 µm was used in this study.[Citation64]
Challenges in the characterization of Boswellia extracts
The most challenging task in the characterization of Boswellia species is to develop a comprehensive tool combining UHPLC-HRMS analysis followed by chemometric analysis to differentiate resin material of different botanical origin by its complex chemical composition, that is, to distinguish Boswellia serrata resin not only by the difference in the amount of major secondary plant metabolites such as the 6 main boswellic acids but also to use the occurrence of minor constituents to differentiate resins from various Boswellia species in a more detailed and robust way as described by Zhang et al.[Citation46] In this case, 12 diagnostic peaks including AKBA, βBA, αBA, and AβBA were used to categorize the samples originating from B. serrata, B. sacra, and B. papyrifera collected from Sudan and Ethiopia. The chemometric evaluation using principal component analysis (PCA) and hierarchical cluster analysis (HCA) heatmap is a reliable tool for the differentiation of various samples. The differentiation based on the determination of 6 major BAs was found to be insufficient. This metabolomic-based approach may also be of interest for the characterization and differentiation of other products of herbal origin. In this context, UHPLC-HRMS[Citation46] is a more suitable analytical tool than UHPLC-QqQ[Citation18] due to the higher number of diagnostic peaks identified (12 vs. 8). In the latter work, the samples were sorted into 4 clusters after the PCA analysis. However, each cluster contained samples from different Boswellia species (see ), demonstrating the need for more sophisticated methods to differentiate the individual species, as previously shown by Zhang et al.[Citation46] Moreover, Schmiech et al.[Citation18] used three marker compounds to calculate the so-called Boswellia index (Bosi), which helped to differentiate Boswellia species:
where [AKBA] is the concentration of AKBA in resin (μg/mg), [ABA] is the concentration of AβBA in resin (μg/mg), and [BA] is the concentration of βBA in resin (μg/mg).
Figure 6. Multivariate statistical analysis of pentacyclic triterpenic acid concentrations in Boswellia oleo-gum resin. Samples # 1-11 (B. sacra, red), samples # 12-14 (B. dalzielii, violet), samples # 15-17 (B. papyrifera, pink), samples # 18-24 (B. serrata, blue), samples # 25-29 (B. carterii, green), samples # 30-36 (B. neglecta, yellow), sample # 37 (B. rivae, grey), and samples # 38-40 (B. frereana, grey). (a) Dendogram of cluster analysis. The samples were assigned to four different clusters: cluster A (red), cluster B (blue), cluster C (grey), and cluster D (yellow). (b) Biplot of PCA with clusters from cluster analysis and subgroup (violet) for B. dalzielii. Sample B.o. (‘X’ B.o., B. occulta) was added subsequently. Figure reproduced from [Citation18] under the terms of the Creative Commons Attribution License (CC BY) of MDPI publisher.
![Figure 6. Multivariate statistical analysis of pentacyclic triterpenic acid concentrations in Boswellia oleo-gum resin. Samples # 1-11 (B. sacra, red), samples # 12-14 (B. dalzielii, violet), samples # 15-17 (B. papyrifera, pink), samples # 18-24 (B. serrata, blue), samples # 25-29 (B. carterii, green), samples # 30-36 (B. neglecta, yellow), sample # 37 (B. rivae, grey), and samples # 38-40 (B. frereana, grey). (a) Dendogram of cluster analysis. The samples were assigned to four different clusters: cluster A (red), cluster B (blue), cluster C (grey), and cluster D (yellow). (b) Biplot of PCA with clusters from cluster analysis and subgroup (violet) for B. dalzielii. Sample B.o. (‘X’ B.o., B. occulta) was added subsequently. Figure reproduced from [Citation18] under the terms of the Creative Commons Attribution License (CC BY) of MDPI publisher.](/cms/asset/ec1d16df-f2a2-4b0b-baa5-3a9976746a98/batc_a_2312502_f0006_c.jpg)
Since the samples in each cluster show very large variations in Bosi (see Table S1), more accurate classification using untargeted methods is more appropriate to distinguish different samples. However, this untargeted approach could also be quite challenging for some species such as B. carterii, which shows a very large variability in their patterns [Citation18] (see ). Therefore, further investigation in the metabolomic-based profiling of various Boswellia resins and products derived from them is desirable.
Another key aspect, which is also a challenge in phytochemistry, is the identification of secondary plant metabolites, especially those with low abundance in the plant material. Therefore, the comprehensive identification of plant metabolites in frankincense samples using a coupling strategy combining preliminary screening by HPLC-DAD/ESI-MSn, HPLC-HRMS, and HPLC-NMR followed by preparative HPLC to obtain a limited number of subfractions that were subsequently characterized by MS and NMR represents a holistic approach to identify secondary plant metabolites of potential pharmacological interest.[Citation45] This approach is not as tedious and time-consuming as typically used isolation procedures and may therefore be a source of inspiration for the characterization of secondary metabolites in other plants.
Another interesting workflow [Citation52] combines untargeted analysis of plant secondary metabolites with network pharmacology to predict absorption and pharmacological activity. This approach was followed by experimental validation of the absorption and pharmacological activity of frankincense and processed frankincense against various molecular targets (see ). This work represents a holistic approach to identifying different biomarkers to describe the differences in the chemical composition of frankincense and processed frankincense. In total, 153 compounds were detected by UHPLC-Q-ToF analysis, of which 81 compounds could be used to discriminate between frankincense and processed frankincense. In total, 54 compounds were identified and 18 of them were used for quantification. Some general rules were drawn: (i) 9,11-dehydro compounds were more abundant, (ii) the amount of 11-keto structures was decreased, (iii) the amount of α/βBAs was decreased, (iv) the amount of Aα/βBA was increased, (v) lupeol and lupeol acetate were less abundant, and (vi) the amount of six tirucallane type compounds was increased in the processed frankincense. The combination of separation methods with experimentally validated network pharmacology allowed a more comprehensive and logical study of the difference in medicinal activity between frankincense and processed frankincense. This approach may also be of interest to explore the medicinal potential of other medicinal plants in a more detailed and systematic manner.
Figure 7. The workflow that combines UHPLC-Q-ToF-based metabolomics to identify the markers to differentiate between frankincense and processed frankincense (step 1), network pharmacology to predict the absorption and pharmacological activity (step 2), and the validation of the predictive strength of network pharmacology toward the absorption and pharmacological activity (step 3), confirming the positive effect of frankincense processing on the better absorption and modification of the pharmacological activity. Figure reproduced from [Citation52] under the terms of the Creative Commons Attribution License (CC BY) of Frontiers publisher.
![Figure 7. The workflow that combines UHPLC-Q-ToF-based metabolomics to identify the markers to differentiate between frankincense and processed frankincense (step 1), network pharmacology to predict the absorption and pharmacological activity (step 2), and the validation of the predictive strength of network pharmacology toward the absorption and pharmacological activity (step 3), confirming the positive effect of frankincense processing on the better absorption and modification of the pharmacological activity. Figure reproduced from [Citation52] under the terms of the Creative Commons Attribution License (CC BY) of Frontiers publisher.](/cms/asset/9252b1fb-2c4a-4339-b2bf-375bcb6c4e8e/batc_a_2312502_f0007_c.jpg)
Gas chromatography
Gas chromatography (GC) methods are less represented in the analysis of boswellic acids compared to LC and TLC. An overview of the GC methods used in the analysis of boswellic acids is summarized in .[Citation39,Citation79–88] Many of the methods listed () were developed for the analysis of the archaeological samples, exploiting the potential of GC-MS for the characterization of a wide variety of organic compounds from a small amount of sample.[Citation82–87] GC-MS provides information on the molecular composition of abundant compounds or minor components of archaeological resinous substances involved in funerary rituals and religious ceremonies, including the presence of frankincense. Typically, the GC-MS was performed using electron ionization (EI) at 70 eV. The EI-GC mass spectra and fragmentation patterns can be found in ref.[Citation82,Citation85,Citation86] Due to the complex composition of archaeological samples (10-37 identified compounds), their analysis takes up to 60 min,[Citation82–84,Citation87] while methods focusing solely on triterpenoid compounds in Boswellia plant material take less time (up to 25 min).[Citation79,Citation81]
Table 4. GC methods used in the analysis of BAs.
Sample preparation for GC-MS is more complicated than for other chromatographic methods as it involves solvent extraction followed by derivatization of the analytes. Older publications described the methylation of BAs with highly toxic and explosive diazomethane.[Citation79,Citation88] However, this obsolete method has been replaced by silylation with trimethylsilyl chloride (TMSCl, TMCS),[Citation81,Citation82,Citation84–86] bis(trimethylsilyl)amine (HDMS),[Citation82,Citation84] and N,O-bis(trimethylsilyl)trifluoroacetamide (BSTFA)[Citation80,Citation81,Citation83,Citation85–87] based on the conversion of –OH and –COOH groups of BAs to volatile trimethylsilylated derivatives.
For the analysis of resinous materials in archaeological finds, Modugno et al.[Citation83] used direct-exposure electron ionization mass spectrometry (DE-MS) as an alternative method that avoided laborious sample pretreatment followed by derivatization. Samples were analyzed directly after dissolution in dichloromethane. DE-MS is a fast tool that allows the characterization of the main components of the sample within a few minutes. On the other hand, it failed to provide detailed molecular composition and identification of minor components in complex mixed samples.[Citation83]
GC enables the separation of various compounds present in frankincense including the degradation products often identified in archeological samples.[Citation82,Citation85,Citation86] The separation selectivity is competitive with U/HPLC methods, enabling the separation of oleanane (αBAs) structural types, which eluted earlier than the ursane structural types (βBAs).[Citation82,Citation85] Thus, a complete separation of all major BAs can be achieved within one run including the separation of other diterpenoids (e.g., serratol and incensole) and triterpenoids such as tirucallic acids.[Citation85] The elution order is also affected by the molecular weight of the derivatized molecules resulting in the following elution order: αBA, βBA, AαBA, AβBA, KBA, and AKBA.[Citation85] The same elution order was also described in other works.[Citation80,Citation86] 5% phenyl-95% methylpolysiloxane was the stationary phase in all these works.
Jemmali et al.[Citation81] developed a GC-MS method for the separation of triterpenic compounds in plant material including Boswellia resin. In their study, a chemometrically driven approach based on PCA and HCA followed by a deeper evaluation of pre-selected columns using Derringer's desirability function to select the most appropriate stationary phase was applied. The columns used in the study ranged from highly apolar with a polarity index of 194 (100% polydimethylsiloxane) up to highly polar columns with a polarity index of 921 (50% phenyl-50% methylpolysiloxane). HP-5ms followed by DB-5ms were found to be the most suitable columns for the separation of triterpenoids, which is in good agreement with most works listed in that use the columns based on 5% phenyl-95% methylpolysiloxane chemistry. Since both columns are based on the same chemistry, their polarity expressed by the McReynolds constant is very similar, that is, 307 and 338, respectively. It should be pointed out here that the authors refer to HP-5ms and DB-5ms columns as if they had different substituents to dimethylpolysiloxane, that is, 5% diphenyl and 5% phenyl, respectively. According to the manufacturer, however, both of these columns contain the identical stationary phase, which is equivalent to USP phase 27.[Citation24] The only difference should be in production processes. The elution order of the boswellic acids was also described rather vaguely. According to Table 3 in ref.[Citation81], the elution order was βBA, αBA, AβBA, and AαBA, while another elution order, that is, αBA, βBA, AαBA, and AβBA was described in the section 3.7.1. in ref.[Citation81] The latter is in accordance with the data in other works using the same column chemistry (see ).
Many published GC-MS methods are also suitable for triterpenoid fingerprinting to distinguish different Boswellia resins as shown by Hanus et al.[Citation80] for Boswellia serrata and carterii. The comparison of the relative abundances of di- and triterpenoids in Boswellia carterii, sacra, serrata, and papyrifera was shown in the work of Baeten et al.[Citation85] The highest percentage of total BAs in relation to total di- and triterpenoid fraction was found in Boswellia serrata (60% abundance) with a ratio of AKBA/KBA of 1.1 (Table S4 and ). The other species were found to contain between 42.0 and 47.8% of BAs but with a higher ratio of AKBA/KBA (4.2-6.9). When compared to the AKBA/KBA ratios listed in Table S1, which was adapted from a paper by Schmiech et al.,[Citation18] there is a significant discrepancy in the AKBA/KBA ratios determined for Boswellia sacra (6.4 vs 17.9) and Boswellia papyrifera (4.2 vs 8.07). The reason for this may be the extreme values of the standard deviations reported by Schmiech et al.,[Citation18] which in turn may be caused by the inconsistency of the composition of the plant material, which strongly depends on the origin and growth conditions of the plants. The keto derivatives are less abundant BAs compared to the non-keto derivatives. The ursane type (βBAs) was found to be more abundant than the oleanane type (αBAs). Tirucallanes were found in low amounts in Boswellia sacra (0.4%) and Boswellia carterii (5.0%), while much higher amounts were found in Boswellia serrata (12.7%) and Boswellia papyrifera (22.1%) (Table S4).
Figure 8. Relative abundances (%) of boswellic acids related to the total di- and triterpene fractions identified in commercial samples of Boswellia resins. This figure was prepared from the data presented in Table 2 in ref.[Citation85]
![Figure 8. Relative abundances (%) of boswellic acids related to the total di- and triterpene fractions identified in commercial samples of Boswellia resins. This figure was prepared from the data presented in Table 2 in ref.[Citation85]](/cms/asset/1f9f649d-6a3d-41da-a98b-4b787030542c/batc_a_2312502_f0008_c.jpg)
To our knowledge, the studies comparing the data from GC and LC determination of BAs of the same Boswellia sample have not yet been published. Such studies would provide useful information on the agreement of quantitative data obtained by these two separation methods.
Another stationary phase based on poly[(3,3,3-trifluoropropyl)]methylsiloxane with a high polarity of 850 was used for the determination of KBA in human plasma.[Citation88] The method was fully validated using 18α-glycyrrhetinic acid as an IS. The retention times of IS and KBA were 3.68 and 4.51 min, respectively, allowing relatively rapid determination of an analyte in plasma samples. Sample preparation included protein precipitation (PP), LLE, derivatization by methylation with diazomethane solution in ethylether, and reconstitution in toluene prior to GC-MS analysis. Negative ion chemical ionization with ammonia as reaction gas was used to detect the analyte and IS at m/z 483 in SIM. The entire analysis took 8 min. The method with a LOQ of 10 ng/mL was validated in terms of selectivity, inter-day/intra-day precision and accuracy, linearity, sensitivity, stability, and recovery.[Citation88]
Thin-layer chromatography
Planar chromatography is an indispensable separation method in plant analysis because of its simplicity and speed, although it has some limitations in terms of specificity and sensitivity. While thin-layer chromatography (TLC) is primarily considered a technique for qualitative analysis, it can also provide precise and accurate quantitative data. The latter is true for high-performance thin-layer chromatography (HPTLC), which is typically combined with densitometric detection. It is also cost-effective because it uses less mobile phase than HPLC and can analyze many samples simultaneously. However, due to the use of harmful solvents such as chloroform and toluene, the environmental impact of TLC may be higher than that of other separation techniques.
An overview of the experimental settings of TLC methods for the qualitative and/or quantitative analysis of BAs, including the conditions prescribed by the Ph. Eur. and USP,[Citation23,Citation24] is presented in .[Citation38,Citation78,Citation79,Citation89–102] TLC and HPTLC have been used for the characterization of herbal extracts,[Citation38,Citation78,Citation79,Citation90–98] the determination of major constituents in different dosage forms,[Citation98–102] and in one case for the analysis of plasma samples.[Citation102]
Table 5. TLC methods used in the analysis of BAs.
Silica gel 60 F254 on aluminum or glass plates was used as the stationary phase in all studies, with the exception of the utilization of silica gel G for the separation of methyl esters of βBA and AβBA.[Citation79] Various solvent combinations were used as a mobile phase; in most cases, the composition included hexane or another non-polar solvent. The addition of a small amount of acetic acid (or formic acid in Ph. Eur.[Citation23]) as an additive to the mobile phase was quite common. Under these conditions, the retardation of BAs by silica can be reduced and the elution can be achieved in narrower zones.
Direct densitometric determination of KBA and AKBA was possible without derivatization at 250 or 254 nm, whereas densitometric evaluation at 540, 560, or 366 nm after derivatization with anisaldehyde-sulfuric acid reagent was necessary for the detection/quantification of other boswellic acids such as βBA and AβBA.
In most cases, sample preparation for a TLC/HPTLC analysis was simple and involved dissolution and subsequent dilution of the analyzed sample in MeOH. This was not the case in only one work where HPTLC was used to analyze plasma.[Citation102] Here, sample preparation involved the addition of hydrochloric acid, LLE with the mixture of hexane and methyl-tert-butyl ether (2:3), drying of the supernatant with anhydrous sodium sulfate at elevated temperature (60–70 °C), and reconstitution in chloroform.[Citation102] For the stability testing, the sample was exposed to alkaline and acidic environments or stressed by heat.[Citation102]
Most of the separation methods were partially or fully validated.[Citation38,Citation89,Citation91,Citation96–102] Shah et al.[Citation102] reported limits of detection at 8.75 ng/ml for KBA in plasma while LOD at 11.38 and 14.58 ng/spot were estimated for AKBA and KBA in the assay of tablets. Goel and coworkers[Citation98] reported a LOD of 3.06 ng/band for AKBA.
TLC is usually used to resolve KBA and AKBA. Some methods allow the separation of 4 BAs, i.e., KBA, AKBA, βBA, and AβBA.[Citation89,Citation91] On the other hand, Paul et al.[Citation94] reported the TLC method for the differentiation of Boswellia serrata, sacra, papyrifera, and carterii. Besides boswellic acids, other triterpenoids of pharmacological interest such as incensole, incensole acetate, 3-oxo-8,24-dien-tirucallic acid, serratol, and β-caryophyllene oxide were separated from each other. It should be noted that TLC has limited separation selectivity toward the individual isomers of boswellic acids, i.e., α-/β- isomers were not reported to be separated (see also where αBA eluted together with βBA and AαBA coeluted with AβBA).
Figure 9. The image of the TLC plate showing the separation of diethyl ether extracts of resins from different Boswellia species. The plate was treated with anisaldehyde reagent after the development (left). The model plate is also shown (right). Identification of spots: KBA (Rf 0.10), AKBA (Rf 0.16), α + βBA (Rf 0.19), 3-oxo-8,24-dien-tirucallic acid (Rf 0.22), AαBA + AβBA (Rf 0.25), incensole (Rf 0.27), serratol (Rf 0,46), β-caryophyllene oxide (Rf 0.50), incensole acetate (Rf 0.68), terpenes without functional group (Rf 0.80). Samples (from left to right): 1: B. papyrifera; 2: B. serrata; 3: B. carterii, 4: B. sacra. Figure reproduced from [Citation94] with permission from John Wiley & Sons.
![Figure 9. The image of the TLC plate showing the separation of diethyl ether extracts of resins from different Boswellia species. The plate was treated with anisaldehyde reagent after the development (left). The model plate is also shown (right). Identification of spots: KBA (Rf 0.10), AKBA (Rf 0.16), α + βBA (Rf 0.19), 3-oxo-8,24-dien-tirucallic acid (Rf 0.22), AαBA + AβBA (Rf 0.25), incensole (Rf 0.27), serratol (Rf 0,46), β-caryophyllene oxide (Rf 0.50), incensole acetate (Rf 0.68), terpenes without functional group (Rf 0.80). Samples (from left to right): 1: B. papyrifera; 2: B. serrata; 3: B. carterii, 4: B. sacra. Figure reproduced from [Citation94] with permission from John Wiley & Sons.](/cms/asset/b0be0af7-6ddd-4d41-981a-eefcbed2e87d/batc_a_2312502_f0009_c.jpg)
Goel et al.[Citation98] reported stability indicating method that allowed the separation of AKBA next to its degradation products under forced conditions. In another study, two-dimensional TLC was used to monitor the conversion of 3-O-acetyl-11-hydroxy-β-BA, an intermediate of AKBA, to 3-O-acetyl-9,11-dehydro-β-BA.[Citation90] These two reports demonstrate the potential of TLC for the rapid and inexpensive monitoring of olibanum stability.
TLC can be considered as an alternative method for simple drug formulation assays including the determination of BAs (typically eluting in the single zone) together with the other active ingredients in multicomponent antiarthritic formulations.[Citation96,Citation99–101] The major limitation of such methods is poor selectivity, which in most cases does not allow simultaneous quantification of individual BAs.[Citation96,Citation100,Citation101] The only exception was the determination of KBA and AKBA next to E- and Z-guggulsterone.[Citation99]
Miscellaneous separation techniques
Supercritical fluid chromatography (SFC) is a chromatography method that uses supercritical or subcritical fluid as the mobile phase. Supercritical fluid has properties of both liquid and gas. Carbon dioxide, an environmentally friendly fluid, is typically used as the mobile phase. Furthermore, the flexibility of the SFC technique can be improved by mixing some organic modifiers, such as methanol, in the mobile phase, which allows for an increased solubility of most analytes. Combined with short analysis times and high separation efficiency, this makes SFC a very promising technique. A single SFC-UV/SFC-MS method was developed for the analysis of boswellic acids in dietary supplements (). A high-strength C18 silica stationary phase with C18 chemically bonded to specially prepared high-purity silica microspheres (StableBond) was used to enable the complete resolution of six boswellic acids. The optimized method allowed the baseline separation of six major BAs in less than 6 min. The elution order on a C18 column was AKBA, AαBA, AβBA, KBA, αBA, βBA,[Citation103] which differs from that described in the HPLC and UHPLC papers discussed above. The LOD and LOQ of this method were in the range of 4.6-5.5 μg/mL and 13.9-16.7 μg/mL respectively, which is higher compared to the average LOD and LOQ obtained in LC-UV (Table S2). The SFC/MS methods enabled the identification of other compounds such as 3α-acetyl-9,11-dehydro-β-BA and tirucallic acids (see ) [103]. This confirms the potential of using SFC/MS for rapid fingerprinting of resins from different Boswellia species.
Figure 10. SFC separation of a mixture of BA standards (A) and a sample of a dietary supplement containing Boswellia serrata extract (B). 1 – AKBA, 2 – AαBA, 3 – AβBA, 4 – KBA, 5 – αBA, 6 – βBA. Compounds a and b are estimated to be 3α-acetyl-9,11-dehydro-β-boswellic acid and an isomer of 3-oxo-tirucallic acid, respectively. Figure reproduced from [Citation103] under the terms of the Creative Commons Attribution License (CC BY) of Elsevier.
![Figure 10. SFC separation of a mixture of BA standards (A) and a sample of a dietary supplement containing Boswellia serrata extract (B). 1 – AKBA, 2 – AαBA, 3 – AβBA, 4 – KBA, 5 – αBA, 6 – βBA. Compounds a and b are estimated to be 3α-acetyl-9,11-dehydro-β-boswellic acid and an isomer of 3-oxo-tirucallic acid, respectively. Figure reproduced from [Citation103] under the terms of the Creative Commons Attribution License (CC BY) of Elsevier.](/cms/asset/aecc7868-2d4b-4fa8-a7ff-02c35aed542d/batc_a_2312502_f0010_b.jpg)
Table 6. Miscellaneous methods used in the analysis of BAs.
Capillary electrochromatography (CEC) is a separation technique that combines electrodriven flow with the chromatographic separation mechanism by packing a separation capillary with an HPLC-type stationary phase. Just like CZE, this method has the flat flow velocity profile of electroosmotic flow. To achieve a baseline separation of six boswellic acids, Ganzera et al.[Citation104] used a fused silica capillary with an effective length of 25 cm filled with C18 stationary phase (particle size 3 µm). The mobile phase was a 9:1 mixture of acetonitrile and aqueous 20 mmol/L ammonium formate solution (pH 6.5). The analysis took less than 20 min, and the migration/elution order was the same as for the typical HPLC separations (). The determination of Boswellia serrata extract by this CEC method and reference HPLC methods were in good agreement.[Citation104]
Conclusions and future perspectives
Liquid chromatography, GC, and TLC represent the most frequently used separation methods in the analysis of boswellic acids. While LC and GC enabled the baseline separation of the main boswellic acids, TLC showed limited selectivity, especially in the separation of α- and β-isomers. The selectivity and sensitivity of conventional LC and GC methods can be improved by using MS detectors. Therefore, LC-MS can be applied in the characterization and fingerprinting of various Boswellia resins, dietary supplements, and pharmacokinetic studies. GC-MS was employed mostly for the analysis of BAs in archaeological samples after the mandatory sample derivatization which limits the widespread application of this technique. Since TLC offers only limited selectivity and higher LOQs, this technique may be only used for rapid and cost-effective qualitative and quantitative analysis of various extracts of dietary supplements. Although only one SFC method has been developed to date, this method also shows good potential for the analysis of active components in Boswellia resins due to its excellent selectivity within a short analysis time.[Citation103] Electromigration methods play a very limited role in the analysis of BAs. Only one CEC method with fused silica capillaries packed with C-18 stationary phase has been developed so far. This method provided the same selectivity for boswellic acids as LC. Considering the lower robustness of CEC in general, the need for sample derivatization in GC, and the limited selectivity and sensitivity of TLC, liquid-chromatography-based methods (HPLC and UHPLC) represent the techniques of the first choice in the analysis of BAs.
While targeted LC or LC-MS methods enable to distinguish different Boswellia species [Citation18] or to evaluate the quality of dietary supplements,[Citation32,Citation33] they do not represent the state-of-the-art tool for comprehensive fingerprinting. As the USP and Ph. Eur. require the determination of KBA and AKBA, it is necessary to reevaluate these requirements of the Pharmacopeias in order to comply with the current knowledge on the mechanism of action, i.e., the determination of other BAs such as βBA should also be involved. The development of untargeted LC-MS methods for the unambiguous identification and classification of resins from different Boswellia species is still a current issue. Only a limited number of papers focused on this topic so far. For example, Zhang et al.[Citation46] analyzed and compared the resin from 3 different species. The main advantage of these sophisticated methods lies in obtaining more comprehensive information about the qualitative and quantitative composition of different Boswellia resins, which facilitates the detection of possible adulteration of Boswellia serrata dietary supplements. Moreover, these methods may be useful to monitor the metabolism of BAs. Therefore, the LC-MS and especially untargeted methods with HRMS will play an important role in fingerprinting resins of different origins and unraveling the metabolism of their constituents. In this respect, the utilization of ion-mobility mass spectrometry or 2D separation techniques could bring a further degree of selectivity, especially toward minor components.
The fact that other components of Boswellia resin also show various pharmacological activities (including the anti-neoplastic ones) makes these compounds interesting and attractive for further research and investigation. Modern analytical methods may help in the analysis of BAs and their metabolites during the clinical trials that need to be carried out to validate the clinical usefulness of Boswellia serrata products, i.e., to move from the experience-based product to evidence-based medicine.[Citation33] Thus, our review brings a critical overview that might help the researchers in further exploring of medicinal utilization of Boswellia extract and its thorough characterization.
Abbreviations | ||
ACN | = | acetonitrile |
AKBA | = | 3-O-acetyl-11-keto-β-boswellic acid |
AαBA | = | acetyl-α-boswellic acid |
AβBA | = | acetyl-β-boswellic acid |
αBA | = | α-boswellic acid |
BA | = | boswellic acid |
βBA | = | β-boswellic acid |
BSTFA | = | N,O-bis(trimethylsilyl)trifluoroacetamide |
CEC | = | capillary electrochromatography |
CI | = | chemical ionization |
DE-MS | = | direct-exposure electron ionization mass spectrometry |
EI | = | electron ionization |
ESI | = | electrospray ionization |
FID | = | flame ionization detector |
GC | = | gas chromatography |
HCA | = | hierarchical cluster analysis |
HDMS | = | bis(trimethylsilyl)amine (or hexamethyldisilazane) |
HPLC | = | high-performance liquid chromatography |
HPTLC | = | high-performance thin-layer chromatography |
HRMS | = | high resolution mass spectrometry |
HSCCC | = | high-speed countercurrent chromatography |
IFN | = | interferon |
IL | = | interleukin |
IS | = | internal standard |
IT | = | ion trap |
KBA | = | 11-keto-β-boswellic acid |
LC | = | liquid chromatography |
LOD | = | limit of detection |
LOQ | = | limit of quantification |
LLE | = | liquid-liquid extraction |
LT | = | leukotriene |
5-LO | = | 5-lipooxygenase |
MeOH | = | methanol |
mPGES1 | = | microsomal prostaglandin E2 synthase-1 |
MS | = | mass spectrometry |
NFκB | = | nuclear factor kappa B |
NICI | = | negative ion chemical ionization |
NIRS | = | near-infrared spectroscopy |
NMR | = | nuclear magnetic resonance |
PCA | = | principal component analysis |
Ph. Eur. | = | European Pharmacopeia |
PLS | = | partial least squares regression analysis |
PP | = | protein precipitation |
Py-GC | = | pyrolysis gas chromatography |
Q | = | quadrupole |
QqQ | = | triple quadrupole |
Q-ToF | = | quadrupole time-of-flight |
SFC | = | supercritical fluid chromatography |
SIM | = | selected ion monitoring |
SIL-IS | = | stable isotopically labeled standards |
SLE | = | supported liquid extraction |
SPE | = | solid phase extraction |
SRM | = | selected reaction monitoring |
TFA | = | trifluoroacetic acid |
TLC | = | thin-layer chromatography |
TMSCl (or TMCS) | = | trimethylsilyl chloride |
TNF | = | tumor necrosis factor |
UHPLC | = | ultra high-performance liquid chromatography |
USP | = | United States Pharmacopeia. |
Supplemental Material
Download PDF (406 KB)Disclosure statement
No potential conflict of interest was reported by the author(s).
Correction Statement
This article has been corrected with minor changes. These changes do not impact the academic content of the article.
Additional information
Funding
References
- Siddiqui, M. Z. Boswellia Serrata, a Potential Antiinflammatory Agent: An Overview. Indian J. Pharm. Sci. 2011, 73, 255–261. DOI: 10.4103/0250-474X.93507.
- Zhang, Y. X.; Ning, Z. C.; Lu, C.; Zhao, S. Y.; Wang, J. F.; Liu, B. Q.; Xu, X. G.; Liu, Y. Y. Triterpenoid Resinous Metabolites from the Genus Boswellia: Pharmacological Activities and Potential Species-Identifying Properties. Chem. Cent. J. 2013, 7, 153. DOI: 10.1186/1752-153x-7-153.
- Michie, C. A.; Cooper, E. Frankincense and Myrrh as Remedies in Children. J. R Soc. Med. 1991, 84, 602–605. DOI: 10.1177/014107689108401011.
- Ammon, H. P. T. Boswellic Acids and Their Role in Chronic Inflammatory Diseases. Adv. Exp. Med. Biol. 2016, 928, 291–327. DOI: 10.1007/978-3-319-41334-1_13.
- Houssen, M. E.; Ragab, A.; Mesbah, A.; El-Samanoudy, A. Z.; Othman, G.; Moustafa, A. F.; Badria, F. A. Natural anti-Inflammatory Products and Leukotriene Inhibitors as Complementary Therapy for Bronchial Asthma. Clin. Biochem. 2010, 43, 887–890. DOI: 10.1016/j.clinbiochem.2010.04.061.
- Roy, N. K.; Parama, D.; Banik, K.; Bordoloi, D.; Devi, A. K.; Thakur, K. K.; Padmavathi, G.; Shakibaei, M.; Fan, L.; Sethi, G.; Kunnumakkara, A. B. An Update on Pharmacological Potential of Boswellic Acids against Chronic Diseases. Int. J. Mol. Sci. 2019, 20, 4101. DOI: 10.3390/ijms20174101.
- Solanki, N.; Gupta, G.; Chellappan, D. K.; Singh, S. K.; Gulati, M.; Paudel, K. R.; Hansbro, P. M.; Dua, K.; Bhan, S.; Saini, M.; Dureja, H. Boswellic Acids: A Critical Appraisal of Their Therapeutic and Nutritional Benefits in Chronic Inflammatory Diseases. Endocr. Metab. Immune Disord. Drug Targets. 2024, 24, 116–129. DOI: 10.2174/1871530323666230512154634.
- Sharma, T.; Jana, S. Boswellic Acids as Natural Anticancer Medicine: Precious Gift to Humankind. J. Herb Med. 2020, 20, 100313. DOI: 10.1016/j.hermed.2019.100313.
- Poeckel, D.; Werz, O. Boswellic Acids: Biological Actions and Molecular Targets. Curr. Med. Chem. 2006, 13, 3359–3369. DOI: 10.2174/092986706779010333.
- Al-Harrasi, A.; Khan, A. L.; Rehman, N. U.; Csuk, R. Biosynthetic Diversity in Triterpene Cyclization within the Boswellia Genus. Phytochemistry 2021, 184, 112660. DOI: 10.1016/j.phytochem.2021.112660.
- Vuddanda, P. R.; Singh, S.; Velaga, S. Boswellic acid - Medicinal Use of an Ancient Herbal Remedy. J Herb Med 2016, 6, 163–170. DOI: 10.1016/j.hermed.2016.08.002.
- Shah, B. A.; Qazi, G. N.; Taneja, S. C. Boswellic Acids: A Group of Medicinally Important Compounds. Nat. Prod. Rep. 2009, 26, 72–89. DOI: 10.1039/b809437n.
- Iram, F.; Khan, S. A.; Husain, A. Phytochemistry and Potential Therapeutic Actions of Boswellic Acids: A Mini-Review. Asian Pac J Trop Bio 2017, 7, 513–523. DOI: 10.1016/j.apjtb.2017.05.001.
- Koeberle, A.; Werz, O. Natural Products as Inhibitors of Prostaglandin E-2 and Pro-Inflammatory 5-Lipoxygenase-Derived Lipid Mediator Biosynthesis. Biotechnol. Adv. 2018, 36, 1709–1723. DOI: 10.1016/j.biotechadv.2018.02.010.
- Al-Harrasi, A.; Csuk, R.; Khan, A.; Hussain, J. Distribution of the anti-Inflammatory and anti-Depressant Compounds: Incensole and Incensole Acetate in Genus Boswellia. Phytochemistry 2019, 161, 28–40. DOI: 10.1016/j.phytochem.2019.01.007.
- Bhushan, S.; Malik, F.; Kumar, A.; Isher, H. K.; Kaur, I. P.; Taneja, S. C.; Singh, J. Activation of p53/p21/PUMA Alliance and Disruption of PI-3/Akt in Multimodal Targeting of Apoptotic Signaling Cascades in Cervical Cancer Cells by a Pentacyclic Triterpenediol From Boswellia Serrata. Mol. Carcinog. 2009, 48, 1093–1108. DOI: 10.1002/mc.20559.
- Bhushan, S.; Kakkar, V.; Pal, H. C.; Guru, S. K.; Kumar, A.; Mondhe, D. M.; Sharma, P. R.; Taneja, S. C.; Kaur, I. P.; Singh, J.; Saxena, A. K. Enhanced Anticancer Potential of Encapsulated Solid Lipid Nanoparticles of TPD: A Novel Triterpenediol from Boswellia Serrata. Mol. Pharm. 2013, 10, 225–235. DOI: 10.1021/mp300385m.
- Schmiech, M.; Lang, S. J.; Werner, K.; Rashan, L. J.; Syrovets, T.; Simmet, T. Comparative Analysis of Pentacyclic Triterpenic Acid Compositions in Oleogum Resins of Different Boswellia Species and Their In Vitro Cytotoxicity against Treatment-Resistant Human Breast Cancer Cells. Molecules 2019, 24, 2153. DOI: 10.3390/molecules24112153.
- Du, Z. Y.; Liu, Z. L.; Ning, Z. C.; Liu, Y. Y.; Song, Z. Q.; Wang, C.; Lu, A. P. Prospects of Boswellic Acids as Potential Pharmaceutics. Planta Med. 2015, 81, 259–271. DOI: 10.1055/s-0034-1396313.
- ChemSpider. ChemSpider ID 552872, 147762, 28536207, 9561371, 9343298, 8023261. https://www.chemspider.com/. (accessed July 7 2023).
- SciFinder. CAS registry numbers 471-66-9, 631-69-6, 89913-60-0, 5968-70-7, 17019-92-0, 67416-61-9; calculated using ACD/Labs software. https://scifinder.cas.org. (accessed July 7 2023).
- Hussain, H.; Ali, I.; Wang, D. J.; Hakkim, F. L.; Westermann, B.; Rashan, L.; Ahmed, I.; Green, I. R. Boswellic Acids: Privileged Structures to Develop Lead Compounds for Anticancer Drug Discovery. Expert Opin. Drug Discov. 2021, 16, 851–867. DOI: 10.1080/17460441.2021.1892640.
- Council of Europe. European Pharmacopoeia. 11th Ed. Council of Europe: Strasbourg, France, 2023.
- The United States Pharmacopeial Convention. The United States Pharmacopeia - National Formulary; The United States Pharmacopeial Convention: Rockville, Maryland, USA, 2023.
- Indian Pharmacopoeia Commission. Indian Pharmacopoeia 6th Edition; Indian Pharmacopoeia Commission: Ghaziabad, India, 2010.
- Chinese Pharmacopoeia Commission. Chinese Pharmacopoeia; China Medical Science and Technology Press: Beijing, China, 2020.
- Siemoneit, U.; Pergola, C.; Jazzar, B.; Northoff, H.; Skarke, C.; Jauch, J.; Werz, O. On the Interference of Boswellic Acids with 5-Lipoxygenase: Mechanistic Studies in Vitro and Pharmacological Relevance. Eur. J. Pharmacol. 2009, 606, 246–254. DOI: 10.1016/j.ejphar.2009.01.044.
- Siemoneit, U.; Koeberle, A.; Rossi, A.; Dehm, F.; Verhoff, M.; Reckel, S.; Maier, T. J.; Jauch, J.; Northoff, H.; Bernhard, F.; et al. Inhibition of Microsomal Prostaglandin E-2 Synthase-1 as a Molecular Basis for the anti-Inflammatory Actions of Boswellic Acids from Frankincense. Br. J. Pharmacol. 2011, 162, 147–162. DOI: 10.1111/j.1476-5381.2010.01020.x.
- Tausch, L.; Henkel, A.; Siemoneit, U.; Poeckel, D.; Kather, N.; Franke, L.; Hofmann, B.; Schneider, G.; Angioni, C.; Geisslinger, G.; et al. Identification of Human Cathepsin G As a Functional Target of Boswellic Acids from the Anti-Inflammatory Remedy Frankincense. J. Immunol. 2009, 183, 3433–3442. DOI: 10.4049/jimmunol.0803574.
- Verhoff, M.; Seitz, S.; Paul, M.; Noha, S. M.; Jauch, J.; Schuster, D.; Werz, O. Tetra- and Pentacyclic Triterpene Acids from the Ancient Anti-Inflammatory Remedy Frankincense as Inhibitors of Microsomal Prostaglandin E-2 Synthase-1. J. Nat. Prod. 2014, 77, 1445–1451. DOI: 10.1021/np500198g.
- Shehata, A. M.; Quintanilla-Fend, L.; Bettio, S.; Singh, C. B.; Ammon, H. P. T. Prevention of Multiple Low-Dose Streptozotocin (MLD-STZ) Diabetes in Mice by an Extract from Gum Resin of Boswellia Serrata (BE). Phytomedicine 2011, 18, 1037–1044. DOI: 10.1016/j.phymed.2011.06.035.
- Meins, J.; Artaria, C.; Riva, A.; Morazzoni, P.; Schubert-Zsilavecz, M.; Abdel-Tawab, M. Survey on the Quality of the Top-Selling European and American Botanical Dietary Supplements Containing Boswellic Acids. Planta Med. 2016, 82, 573–579. DOI: 10.1055/s-0042-103497.
- Börner, F.; Werner, M.; Ertelt, J.; Meins, J.; Abdel-Tawab, M.; Werz, O. Analysis of Boswellic Acid Contents and Related Pharmacological Activities of Frankincense-Based Remedies That Modulate Inflammation. Pharmaceuticals (Basel) 2021, 14, 660. DOI: 10.3390/ph14070660.
- Xu, C. C.; Wang, B.; Pu, Y. Q.; Tao, J. S.; Zhang, T. Techniques for the Analysis of Pentacyclic Triterpenoids in Medicinal Plants. J. Sep. Sci. 2018, 41, 6–19. DOI: 10.1002/jssc.201700201.
- Huang, K.; Chen, Y. R.; Liang, K. Y.; Xu, X. Y.; Jiang, J.; Liu, M. H.; Zhou, F. H. Review of the Chemical Composition, Pharmacological Effects, Pharmacokinetics, and Quality Control of Boswellia Carterii. Evid. Based Complement. Alternat. Med. 2022, 2022, 6627104. DOI: 10.1155/2022/6627104.
- Das, D. D.; Sharma, N.; Chawla, V.; Chawla, P. A. Current Trends of Analytical Techniques for Bioactive Terpenoids: A Review. Crit. Rev. Anal. Chem. 2023. DOI: 10.1080/10408347.2023.2219757.
- Katragunta, K.; Siva, B.; Kondepudi, N.; Vadaparthi, P. R. R.; Rao, N. R.; Tiwari, A. K.; Babu, K. S. Estimation of Boswellic Acids in Herbal Formulations Containing Boswellia Serrata Extract and Comprehensive Characterization of Secondary Metabolites Using UPLC-Q-Tof-MSe. J. Pharm. Anal. 2019, 9, 414–422. DOI: 10.1016/j.jpha.2019.09.007.
- Mehta, M.; Satija, S.; Garg, M. Comparison Between HPLC and HPTLC Densitometry for the Determination of 11-Keto-Beta-Boswellic Acid and 3-Acetyl-11-Keto-Beta-Boswellic Acid from Boswellia Serrata Extract. IJPER. 2016, 50, 418–423. DOI: 10.5530/ijper.50.3.15.
- Suhail, M. M.; Wu, W. J.; Cao, A.; Mondalek, F. G.; Fung, K. M.; Shih, P. T.; Fang, Y. T.; Woolley, C.; Young, G.; Lin, H. K. Boswellia Sacra Essential Oil Induces Tumor Cell-Specific Apoptosis and Suppresses Tumor Aggressiveness in Cultured Human Breast Cancer Cells. BMC Complement. Altern. Med. 2011, 11, 129. DOI: 10.1186/1472-6882-11-129.
- Subbaraju, G. V.; Sridhar, P.; Ramakrishna, S.; Sreemannarayana, A.; Vanisree, M.; Babu, S. K. Isolation and HPLC Estimation of Six Boswellic Acids from Boswellia Serrata Extract. Asian J. Chem. 2004, 16, 1824–1832.
- Mathe, C.; Culioli, G.; Archier, P.; Vieillescazes, C. High-Performance Liquid Chromatographic Analysis of Triterpenoids in Commercial Frankincense. Chromatographia 2004, 60, 493–499. DOI: 10.1365/s10337-004-0417-3.
- Singh, S.; Khajuria, A.; Taneja, S. C.; Johri, R. K.; Singh, J.; Qazi, G. N. Boswellic Acids: A Leukotriene Inhibitor Also Effective through Topical Application in Inflammatory Disorders. Phytomedicine 2008, 15, 400–407. DOI: 10.1016/j.phymed.2007.11.019.
- Paul, M.; Brüning, G.; Weihrather, J.; Jauch, J. Qualitative and Quantitative Analysis of 17 Different Types of Tetra- and Pentacyclic Triterpenic Acids in Boswellia Papyrifera by a Semi-Automatic Homomodal 2D HPLC Method. Chromatographia 2011, 74, 29–40. DOI: 10.1007/s10337-011-2041-3.
- Su, S. L.; Hua, Y. Q.; Wang, Y. Y.; Gu, W.; Zhou, W.; Duan, J. A.; Jiang, H. F.; Chen, T.; Tang, Y. P. Evaluation of the anti-Inflammatory and Analgesic Properties of Individual and Combined Extracts from Commiphora Myrrha, and Boswellia Carterii. J. Ethnopharmacol. 2012, 139, 649–656. DOI: 10.1016/j.jep.2011.12.013.
- Liu, Y. Y.; Liu, Z. L.; Lu, C.; Li, J. B.; Ning, Z. C.; Song, Z. Q.; Wang, C.; Du, Z. Y.; Lu, X. M.; Zhao, S. Y.; Lu, A. P. Comprehensive Identification of Active Triterpenoid Metabolites in Frankincense Using a Coupling Strategy. J. Chromatogr. B Analyt. Technol. Biomed. Life Sci. 2014, 963, 90–98. DOI: 10.1016/j.jchromb.2014.05.054.
- Zhang, C.; Sun, L.; Tian, R. T.; Jin, H. Y.; Ma, S. C.; Gu, B. R. Combination of Quantitative Analysis and Chemometric Analysis for the Quality Evaluation of Three Different Frankincenses by Ultra High Performance Liquid Chromatography and Quadrupole Time of Flight Mass Spectrometry. J. Sep. Sci. 2015, 38, 3324–3330. DOI: 10.1002/jssc.201500326.
- Ho, T. J.; Jiang, S. J.; Lin, G. H.; Li, T. S.; Yiin, L. M.; Yang, J. S.; Hsieh, M. C.; Wu, C. C.; Lin, J. G.; Chen, H. P. The In Vitro and In Vivo Wound Healing Properties of the Chinese Herbal Medicine "Jinchuang Ointment. Evid. Based Complement. Alternat. Med. 2016, 2016, 1654056. DOI: 10.1155/2016/1654056.
- Sharma, N.; Bhardwaj, V.; Singh, S.; Ali, S. A.; Gupta, D. K.; Paul, S.; Satti, N. K.; Chandra, S.; Verma, M. K. Simultaneous Quantification of Triterpenoic Acids by High Performance Liquid Chromatography Method in the Extracts of Gum Resin of Boswellia Serrata Obtained by Different Extraction Techniques. Chem. Cent. J. 2016, 10, 49. DOI: 10.1186/s13065-016-0194-8.
- Mannino, G.; Occhipinti, A.; Maffei, M. E. Quantitative Determination of 3-O-Acetyl-11-Keto-beta-Boswellic Acid (AKBA) and Other Boswellic Acids in Boswellia Sacra Flueck (Syn. B. carteri Birdw) and Boswellia Serrata Roxb. Molecules 2016, 21, 1329. DOI: 10.3390/molecules21101329.
- Al-Harrasi, A.; Rehman, N. U.; Mabood, F.; Albroumi, M.; Ali, L.; Hussain, J.; Hussain, H.; Csuk, R.; Khan, A. L.; Alam, T.; Alameri, S. Application of NIRS Coupled with PLS Regression as a Rapid, Non-Destructive Alternative Method for Quantification of KBA in Boswellia Sacra. Spectrochim. Acta. A Mol. Biomol. Spectrosc. 2017, 184, 277–285. DOI: 10.1016/j.saa.2017.05.018.
- Yoon, J. H.; Kim, J. H.; Ham, S. S.; Gang, B. Y.; Lee, S. H.; Choi, G.; Kim, Y. S.; Lee, G.; Ju, Y. S. Optimal Processing Conditions of Boswellia Carteri Birdw. Using Response Surface Methodology. Pharmacogn. Mag. 2018, 14, 235–241. DOI: 10.4103/pm.pm_140_17.
- Ning, Z. C.; Wang, C.; Liu, Y. Y.; Song, Z. Q.; Ma, X. L.; Liang, D. R.; Liu, Z. L.; Lu, A. P. Integrating Strategies of Herbal Metabolomics, Network Pharmacology, and Experiment Validation to Investigate Frankincense Processing Effects. Front. Pharmacol. 2018, 9, 1482. DOI: 10.3389/fphar.2018.01482.
- Yoo, Y. J.; Huh, S. E.; Kim, Y.; Jang, H. J. Anti-Cancer Activity of Boswellia Carterii Extract Alters the Stress Functional Gene Expression in the Pancreatic Cancer Cell. BioChip J. 2019, 13, 191–201. DOI: 10.1007/s13206-019-3210-y.
- Tambe, A.; Mokashi, P.; Pandita, N. Ex-Vivo Intestinal Absorption Study of Boswellic Acid, Cyclodextrin Complexes and Poloxamer Solid Dispersions Using Everted Gut Sac Technique. J. Pharm. Biomed. Anal. 2019, 167, 66–73. DOI: 10.1016/j.jpba.2018.12.018.
- Yu, J. Q.; Zhao, H. W.; Wang, D. J.; Song, X. Y.; Zhao, L.; Wang, X. Extraction and Purification of Five Terpenoids from Olibanum by Ultrahigh Pressure Technique and High-Speed Countercurrent Chromatography. J. Sep. Sci. 2017, 40, 2732–2740. DOI: 10.1002/jssc.201700215.
- Zhao, H. W.; Geng, Y. L.; Zhu, H.; Yang, P.; Yu, J. Q. Preparative Separation of Flavanones and Terpenoids from Olibanum by High-Speed Counter-Current Chromatography. Acta Chromatogr. 2019, 31, 28–32. DOI: 10.1556/1326.2017.00323.
- Mostafa, D. M.; Ammar, N. M.; Basha, M.; Hussein, R. A.; El Awdan, S.; Awad, G. Transdermal Microemulsions of Boswellia Carterii Bird: Formulation, Characterization and in Vivo Evaluation of anti-Inflammatory Activity. Drug Deliv. 2015, 22, 748–756. DOI: 10.3109/10717544.2014.898347.
- Xie, X. Y.; Liu, Q.; Zhu, F.; Zhang, T. J.; Xu, X. W.; Tao, Y. Tracing Analgesic Constituents from Crude and Vinegar-Processed Resin of Boswellia Carterii by Integrating Ultra-Performance Liquid Chromatography Tandem Mass Spectrometry-Based Determination, Analgesic Evaluation in Mice, and Gray Relationship Analysis. Biomed. Chromatogr. 2022, 36, e5430. DOI: 10.1002/bmc.5430.
- Aksamija, A.; Mathe, C.; Vieillescazes, C. Liquid Chromatography of Triterpenic Resins after Derivatization with Dansyl Chloride. J. Liq. Chromatogr. 2012, 35, 1222–1237. DOI: 10.1080/10826076.2011.619032.
- Rajabian, A.; Boroushaki, M. T.; Hayatdavoudi, P.; Sadeghnia, H. R. Boswellia Serrata Protects Against Glutamate-Induced Oxidative Stress and Apoptosis in PC12 and N2a Cells. DNA Cell Biol. 2016, 35, 666–679. DOI: 10.1089/dna.2016.3332.
- Shah, S. A.; Rathod, I. S.; Suhagia, B. N.; Pandya, S. S.; Parmar, V. K. A Simple High-Performance Liquid Chromatographic Method for the Estimation of Boswellic Acids from the Market Formulations Containing Boswellia Serrata Extract. J. Chromatogr. Sci. 2008, 46, 735–738. DOI: 10.1093/chromsci/46.8.735.
- Büchele, B.; Zugmaier, W.; Simmet, T. Analysis of Pentacyclic Triterpenic Acids from Frankincense Gum Resins and Related Phytopharmaceuticals by High-Performance Liquid Chromatography. Identification of Lupeolic Acid, a Novel Pentacyclic Triterpene. J. Chromatogr. B Analyt. Technol. Biomed. Life Sci. 2003, 791, 21–30. DOI: 10.1016/S1570-0232(03)00160-0.
- Ganzera, M.; Khan, I. A. A Reversed Phase High Performance Liquid Chromatography Method for the Analysis of Boswellic Acids in Boswellia Serrata. Planta Med. 2001, 67, 778–780. DOI: 10.1055/s-2001-18346.
- Frank, A.; Unger, M. Analysis of Frankincense from Various Boswellia Species with Inhibitory Activity on Human Drug Metabolising Cytochrome P450 Enzymes Using Liquid Chromatography Mass Spectrometry after Automated on-Line Extraction. J. Chromatogr. A 2006, 1112, 255–262. DOI: 10.1016/j.chroma.2005.11.116.
- Miscioscia, E.; Shmalberg, J.; Scott, K. C. Measurement of 3-Acetyl-11-Keto-Beta-Boswellic Acid and 11-Keto-Beta-Boswellic Acid in Boswellia Serrata Supplements Administered to Dogs. BMC Vet. Res. 2019, 15, 270. DOI: 10.1186/s12917-019-2021-7.
- Wang, X. Y.; Lei, H. B.; Qi, X. P.; Guo, X.; Xu, X. K.; Zu, X. P.; Ye, J. Simultaneous Determination of Five Bioactive Components of XiaoJin Capsule in Normal and Mammary Gland Hyperplasia Rat Plasma Using LC-MS/MS and Its Application to a Pharmacokinetic Study. Biomed. Chromatogr. 2021, 35, e5000. DOI: 10.1002/bmc.5000.
- Tawab, M. A.; Kaunzinger, A.; Bahr, U.; Karas, M.; Wurglics, M.; Schubert-Zsilavecz, M. Development of a High-Performance Liquid Chromatographic Method for the Determination of 11-Keto-Beta-Boswellic Acid in Human Plasma. J. Chromatogr. B Biomed. Sci. Appl. 2001, 761, 221–227. DOI: 10.1016/S0378-4347(01)00335-8.
- Büchele, B.; Simmet, T. Analysis of 12 Different Pentacyclic Triterpenic Acids from Frankincense in Human Plasma by High-Performance Liquid Chromatography and Photodiode Array Detection. J. Chromatogr. B Analyt. Technol. Biomed. Life Sci. 2003, 795, 355–362. DOI: 10.1016/S1570-0232(03)00555-5.
- Reising, K.; Meins, J.; Bastian, B.; Eckert, G.; Mueller, W. E.; Schubert-Zsilavecz, M.; Abdel-Tawab, M. Determination of Boswellic Acids in Brain and Plasma by High-Performance Liquid Chromatography/Tandem Mass Spectrometry. Anal. Chem. 2005, 77, 6640–6645. DOI: 10.1021/ac0506478.
- Büchele, B.; Zugmaier, W.; Genze, F.; Simmet, T. High-Performance Liquid Chromatographic Determination of Acetyl-11-Keto-Alpha-Boswellic Acid, a Novel Pentacyclic Triterpenoid, in Plasma Using a Fluorinated Stationary Phase and Photodiode Array Detection: Application in Pharmacokinetic Studies. J. Chromatogr. B Analyt. Technol. Biomed. Life Sci. 2005, 829, 144–148. DOI: 10.1016/j.jchromb.2005.09.043.
- Krüger, P.; Daneshfar, R.; Eckert, G. P.; Klein, J.; Volmer, D. A.; Bahr, U.; Müller, W. E.; Karas, M.; Schubert-Zsilavecz, M.; Abdel-Tawab, M. Metabolism of Boswellic Acids in Vitro and in Vivo. Drug Metab. Dispos. 2008, 36, 1135–1142. DOI: 10.1124/dmd.107.018424.
- Gerbeth, K.; Meins, J.; Kirste, S.; Momm, F.; Schubert-Zsilavecz, M.; Abdel-Tawab, M. Determination of Major Boswellic Acids in Plasma by High-Pressure Liquid Chromatography/Mass Spectrometry. J. Pharm. Biomed. Anal. 2011, 56, 998–1005. DOI: 10.1016/j.jpba.2011.07.026.
- Wang, H.; Zhang, C. N.; Wu, Y.; Ai, Y.; Lee, D. Y. W.; Dai, R. H. Comparative Pharmacokinetic Study of Two Boswellic Acids in Normal and Arthritic Rat Plasma after Oral Administration of Boswellia Serrata Extract or Huo Luo Xiao Ling Dan by LC-MS. Biomed. Chromatogr. 2014, 28, 1402–1408. DOI: 10.1002/bmc.3182.
- Zheng, L.; Cong, H. J.; Wu, B.; Xue, M.; Xiang, T.; Yao, Z. Q.; Lin, W. H. HPLC-Q-TOF-MS/MS Analysis of the Constituents in the Rat Biological Fluids After Oral Administration of Qing Ru Xiao Granules. J. Chromatogr. Sci. 2015, 53, 1562–1569. DOI: 10.1093/chromsci/bmv058.
- Ai, Y.; Wang, F. R.; Wu, Y.; Ma, W.; Bian, Q. X.; Lee, D. Y. W.; Dai, R. H. Simultaneous Determination of Two Flavonoids and Six Pentacyclic Triterpenes by UPLC-MS/MS: Application to a Comparative Pharmacokinetic Study in Normal and Arthritic Rats after Oral Administration of Huo Luo Xiao Ling Dan or Its Single Herb Extract. Anal. Methods 2015, 7, 8402–8411. DOI: 10.1039/C5AY01409C.
- Zhang, Y.; Huo, X. X.; Cheng, L. Y.; Chen, M. Y.; Song, L.; Yu, Y. L.; Zhou, K. Development and Validation of a Sensitive UHPLC-MS/MS Method for the Measurement of 13-Elemonic Acid in Rat Plasma and Tissues and Its Application to Pharmacokinetics and Tissue Distribution Study. J. Chromatogr. B Analyt. Technol. Biomed. Life Sci. 2021, 1167, 122566. DOI: 10.1016/j.jchromb.2021.122566.
- Sharma, T.; Jana, S. A Validated LC-MS/MS Method for Simultaneous Determination of 3-O-Acetyl-11-Keto-beta-Boswellic Acid (AKBA) and Its Active Metabolite Acetyl-11-Hydroxy-beta-Boswellic Acid (Ac-11-OH-BA) in Rat Plasma: Application to a Pharmacokinetic Study. J. Chromatogr. Sci. 2020, 58, 485–493. DOI: 10.1093/chromsci/bmaa010.
- Mukadam, S.; Ghule, C.; Girme, A.; Shinde, V. M.; Hingorani, L.; Mahadik, K. R. A Simple HPTLC Approach of Quantification of Serratol and Tirucallic Acid with Boswellic Acids in Boswellia Serrata by Validated Densitometric Method with MS/MS Characterization. J. Chromatogr. Sci. 2023, 61, 953–962. DOI: 10.1093/chromsci/bmad012.
- Hairfield, E. M.; Hairfield, H. H.; Mcnair, H. M. Gc, Gc/Ms, and Tlc of Beta-Boswellic Acid and O-Acetyl-Beta-Boswellic Acid from B. Serrata, B. Carteii, and B. Papyrifera. J. Chromatogr. Sci. 1989, 27, 127–133. DOI: 10.1093/chromsci/27.3.127.
- Hanus, L. O.; Moussaieff, A.; Rezanka, T.; Abu-Lafi, S.; Dembitsky, V. M. Phytochemical Analysis and Comparison for Differentiation of Boswellia Carterii and B. serrata. Nat. Prod. Commun. 2007, 2, 139–142. DOI: 10.1177/1934578X0700200206.
- Jemmali, Z.; Chartier, A.; Elfakir, C. Development of a Gas Chromatography-Mass Spectrometry Method to Monitor in a Single Run, Mono- to Triterpenoid Compounds Distribution in Resinous Plant Materials. J. Chromatogr. A 2016, 1443, 241–253. DOI: 10.1016/j.chroma.2016.03.031.
- Mathe, C.; Culioli, G.; Archier, P.; Vieillescazes, C. Characterization of Archaeological Frankincense by Gas Chromatography-Mass Spectrometry. J. Chromatogr. A 2004, 1023, 277–285. DOI: 10.1016/j.chroma.2003.10.016.
- Modugno, F.; Ribechini, E.; Colombini, M. P. Chemical Study of triterpenoid resinous materials in Archaeological Findings by Means of Direct Exposure Electron Ionisation Mass Spectrometry and Gas Chromatography/Mass Spectrometry. Rapid Commun. Mass Spectrom. 2006, 20, 1787–1800. DOI: 10.1002/rcm.2507.
- Mathe, C.; Connan, J.; Archier, P.; Mouton, M.; Vieillescazes, C. Analysis of Frankincense in Archaeological Samples by Gas Chromatography-Mass Spectrometry. Ann. Chim. 2007, 97, 433–445. DOI: 10.1002/adic.200790029.
- Baeten, J.; Deforce, K.; Challe, S.; De Vos, D.; Degryse, P. Holy Smoke in Medieval Funerary Rites: Chemical Fingerprints of Frankincense in Southern Belgian Incense Burners. PLoS One. 2014, 9, e113142. DOI: 10.1371/journal.pone.0113142.
- Brettell, R. C.; Schotsmans, E. M. J.; Rogers, P. W.; Reifarth, N.; Redfern, R. C.; Stern, B.; Heron, C. P. Choicest Unguents’: Molecular Evidence for the Use of Resinous Plant Exudates in Late Roman Mortuary Rites in Britain. J. Archaeol. Sci. 2015, 53, 639–648. DOI: 10.1016/j.jas.2014.11.006.
- Devièse, T.; Ribechini, E.; Castex, D.; Stuart, B.; Regert, M.; Colombini, M. P. A Multi-Analytical Approach Using FTIR, GC/MS and Py-GC/MS Revealed Early Evidence of Embalming Practices in Roman Catacombs. Microchem. J. 2017, 133, 49–59. DOI: 10.1016/j.microc.2017.03.012.
- Kaunzinger, A.; Baumeister, A.; Cuda, K.; Häring, N.; Schug, B.; Blume, H. H.; Raddatz, K.; Fischer, G.; Schubert-Zsilavecz, M. Determination of 11-Keto-Boswellic Acid in Human Plasma. J. Pharm. Biomed. Anal. 2002, 28, 729–739. DOI: 10.1016/S0731-7085(01)00674-4.
- Krohn, K.; Rao, M. S.; Raman, N. V.; Khalilullah, M. High-Performance Thin Layer Chromatographic Analysis of anti-Inflammatory Triterpenoids from Boswellia Serrata Roxb. Phytochem. Anal. 2001, 12, 374–376. DOI: 10.1002/pca.606.
- Basar, S.; Koch, A. Test of the Stability of Olibanum Resins and Extracts. Jpc-J. Planar Chromat. 2004, 17, 479–482. DOI: 10.1556/JPC.17.2004.6.16.
- Pozharitskaya, O. N.; Ivanova, S. A.; Shikov, A. N.; Makarov, V. G. Separation and Quantification of Terpenoids of Boswellia Serrata Roxb. extract by Planar Chromatography Techniques (TLC and AMD). J. Sep. Sci. 2006, 29, 2245–2250. DOI: 10.1002/jssc.200600078.
- Sahoo, N. K.; Sarkar, S.; Patwardhan, B. Stability Study on Boswellia serrata (Hydro-Alcoholic) Extract. Asian J. Chem. 2009, 21, 3529–3534.
- Sharma, A.; Gupta, N. K.; Dixit, V. K. Complexation with Phosphatidyl Choline as a Strategy for Absorption Enhancement of Boswellic Acid. Drug Deliv. 2010, 17, 587–595. DOI: 10.3109/10717544.2010.501461.
- Paul, M.; Brüning, G.; Bergmann, J.; Jauch, J. A Thin-Layer Chromatography Method for the Identification of Three Different Olibanum Resins (Boswellia serrata, Boswellia papyrifera and Boswellia carterii, Respectively, Boswellia sacra). Phytochem. Anal. 2012, 23, 184–189. DOI: 10.1002/pca.1341.
- Al-Harrasi, A.; Ali, L.; Ceniviva, E.; Al-Rawahi, A.; Hussain, J.; Hussain, H.; Rehman, N. U.; Abbas, G.; Al-Harrasi, R. Antiglycation and Antioxidant Activities and HPTLC Analysis of Boswellia sacra Oleogum Resin: The Sacred Frankincense. Trop. J. Pharm. Res. 2013, 12, 597–602. DOI: 10.4314/tjpr.v12i4.23.
- Gohel, K.; Patel, K.; Shah, P.; Hingorani, L.; Gandhi, T. Box-Behnken Design-Assisted Optimization for Simultaneous Estimation of Quercetin, Kaempferol, and Keto-beta-Boswellic Acid by High-Performance Thin-Layer Chromatography Method. J. Planar Chromat. 2018, 31, 318–326. DOI: 10.1556/1006.2018.31.4.7.
- Mehta, M.; Garg, M.; Dua, K.; Satija, S. Simultaneous HPTLC Densitometric Estimation of KBA and AKBA from Boswellia serrata. CAC. 2018, 15, 84–91. DOI: 10.2174/1573411014666180704123521.
- Goel, A.; Goel, R.; Jain, G. K.; Singh, R. M.; Ahmad, F. J.; Singh, G. N. Development and Validation of a Stability-Indicating HPTLC Method for Analysis of 3-Acetyl-11-Keto-Beta-Boswellic Acid in a Herbal Extract and a Nanoparticles Formulation. Acta Chromatogr. 2008, 20, 497–511. DOI: 10.1556/AChrom.20.2008.3.15.
- Shelke, N. N.; Kuber, V. V.; Bhope, S. G.; Jadhav, R. B. Simultaneous HPTLC Analysis of E-Guggulsterone, Z-Guggulsterone, 11-Keto-beta-Boswellic Acid, and 3-Acetyl-11-Keto-beta-Boswellic Acid in an Anti-Arthritic Formulation. J. Planar Chromat. 2011, 24, 242–247. DOI: 10.1556/JPC.24.2011.3.13.
- Vaykole, A. M.; Nirmal, S. A.; Jadhav, R. S.; Pattan, S. R. Development and Validation of Hptlc Method to Detect Curcumin, Piperine, and Boswellic Acid in Polyherbal Transdermal Patch. J. Liq. Chromatogr. R. T. 2014, 37, 367–378. DOI: 10.1080/10826076.2012.745141.
- Parmar, R. P.; Kanaki, N. S. Simultaneous Estimation of Boswellic Acid and Diosgenin in Polyherbal Transdermal Gel Using Hptlc. Indo Am. J. Pharm. Sci. 2017, 4, 2109–2113. DOI: 10.5281/zenodo.835840.
- Shah, S. A.; Rathod, I. S.; Suhagia, B. N.; Patel, D. A.; Parmar, V. K.; Shah, B. K.; Vaishnavi, V. M. Estimation of Boswellic Acids from Market Formulations of Boswellia serrata Extract and 11-Keto Beta-Boswellic Acid in Human Plasma by High-Performance Thin-Layer Chromatography. J. Chromatogr. B Analyt. Technol. Biomed. Life Sci. 2007, 848, 232–238. DOI: 10.1016/j.jchromb.2006.10.026.
- Zwerger, M.; Ganzera, M. Analysis of Boswellic Acids in Dietary Supplements Containing Indian Frankincense (Boswellia serrata) by Supercritical Fluid Chromatography. J. Pharm. Biomed. Anal. 2021, 201, 114106. DOI: 10.1016/j.jpba.2021.114106.
- Ganzera, M.; Stöggl, W. M.; Bonn, G. K.; Khan, I. A.; Stuppner, H. Capillary Electrochromatography of Boswellic Acids in Boswellia serrata Roxb. J. Sep. Sci. 2003, 26, 1383–1388. DOI: 10.1002/jssc.200301562.