Abstract
Cancer is a major cause of death worldwide. MET tyrosine kinase receptor [MET, c-MET, hepatocyte growth factor (HGF) receptor] pathway activation is associated with the appearance of several hallmarks of cancer. The HGF/MET pathway has emerged as an important actionable target across many solid tumors; therefore, biomarker discovery becomes essential in order to guide clinical intervention and patient stratification with the aim of moving towards personalized medicine. The focus of this review is on how the aberrant activation of the HGF/MET pathway in tumor tissue or the circulation can provide diagnostic and prognostic biomarkers and predictive biomarkers of drug response. Many meta-analyses have shown that aberrant activation of the MET pathway in tumor tissue, including MET gene overexpression, gene amplification, exon 14 skipping and other activating mutations, is almost invariably associated with shorter survival and poor prognosis. Most meta-analyses have been performed in non-small cell lung cancer (NSCLC), breast, head and neck cancers as well as colorectal, gastric, pancreatic and other gastrointestinal cancers. Furthermore, several studies have shown the predictive value of MET biomarkers in the identification of patients who gain the most benefit from HGF/MET targeted therapies administered as single or combination therapies. The highest predictive values have been observed for response to foretinib and savolitinib in renal cancer, as well as tivantinib in NSCLC and colorectal cancer. However, some studies, especially those based on MET expression, have failed to show much value in these stratifications. This may be rooted in lack of standardization of methodologies, in particular in scoring systems applied in immunohistochemistry determinations or absence of oncogenic addiction of cancer cells to the MET pathway, despite detection of overexpression. Measurements of amplification and mutation aberrations are less likely to suffer from these pitfalls. Increased levels of MET soluble ectodomain (sMET) in circulation have also been associated with poor prognosis; however, the evidence is not as strong as it is with tissue-based biomarkers. As a diagnostic biomarker, sMET has shown its value in distinguishing cancer patients from healthy individuals in prostate and bladder cancers and in melanoma. On the other hand, increased circulating HGF has also been presented as a valuable prognostic and diagnostic biomarker in many cancers; however, there is controversy on the predictive value of HGF as a biomarker. Other biomarkers such as circulating tumor DNA (ctDNA) and tumor HGF levels have also been briefly covered. In conclusion, HGF/MET aberrations can provide valuable diagnostic, prognostic and predictive biomarkers and represent vital assets for personalized cancer therapy.
I- Introduction
The incidence and mortality of cancer are rapidly growing throughout the world. The latest GLOBOCAN report from the World Health Organization shows that cancer is the first or second most common cause of death before the age of 70 years in 91 out of 172 countries [Citation1]. An estimated 75 million people will be living with cancer by 2030, while 21 million new cancer cases and 13 million deaths currently occur every year worldwide [Citation2–4].
Mesenchymal-epithelial transition tyrosine kinase receptor (MET, c-MET) belongs to the family of receptor tyrosine kinases (RTKs) that is encoded by MET proto-oncogene located on human chromosome 7 (7q21-31) [Citation5,Citation6].
Since the discovery of MET and its native ligand, hepatocyte growth factor (HGF) in the mid-1980s, aberrant activation or dysregulation of this proto-oncogene has been suspected to be associated with the pathophysiology of several cancers [Citation7]. Starting from the initial findings on the oncogenic role of MET mutations in papillary renal carcinoma [Citation8], and followed by discoveries of other mutations in different human cancers [Citation9], several lines of research in the past few decades have provided strong evidence for an important role of MET in human cancers [Citation10,Citation11] including gastrointestinal [Citation12,Citation13], lung [Citation14,Citation15], breast [Citation16], cervical [Citation17] and thyroid cancer [Citation18]. As a result, some therapeutic agents against this pathway have found their way to routine cancer management protocols, and several others are under pre-clinical and clinical investigation [Citation11,Citation13,Citation19,Citation20].
MET is synthesized as a 170 kD single-chain precursor protein (pro-MET) which undergoes proteolytic cleavage, generating a 50 kD extracellular α-subunit and a 145 kD transmembrane β-subunit. The α-subunit (N-terminal peptide) is linked to the β-subunit by a disulfide bridge [Citation21–24]. The β-subunit consists of an extracellular ligand-binding domain, a single-pass transmembrane domain, and an intracellular segment. The cytoplasmic domain includes a juxtamembrane (JM) domain involved in MET post translational regulation and a catalytic kinase domain that is responsible for tyrosine kinase activity and that modulates multiple downstream signaling pathways () [Citation10,Citation25–28].
Figure 1. HGF/MET signaling pathway. The binding of hepatocyte growth factor (HGF), the natural ligand of MET tyrosine kinase receptor, induces receptor dimerization. Upon dimerization, transphosphorylation of tyrosine 1234 and 1235 residues in the kinase domain activates the kinase activity of the receptor. This is followed by further phosphorylation of Tyr1349 and Tyr1356 residues in the multifunctional docking site. This provides functional recognition sites for a variety of adaptor/effector proteins and subsequent activation of many downstream signaling pathways including MAPK, PI3K/AKT, FAK and STAT3 among others. Downstream signaling basically instigates cellular proliferation, survival, motility, migration, and invasion. Downregulation of MET receptor is initiated by recruiting CBL and ubiquitin mediated degradation as well as extracellular shedding. MET is proteolytically cleaved by ADAM-10 and ADAM-17 resulting in the formation of soluble MET ectodomain (sMET), which is secreted in the extracellular space and can be ultimately found in the circulation. The crosstalk between MET and other membrane receptors such as plexins, integrins, and EGFR and other RTKs promotes metastasis, invasion, and drug resistance. ADAM: a disintegrin and metalloproteinase family; CBL: casitas B lineage lymphoma proto-oncogene; STAT3: signal transducer and activator of transcription 3; FAK: focal adhesion kinase; MAPK: mitogen-activated protein kinase; PI3K: phosphoinositide 3-kinase; RTK: receptor tyrosine kinase.
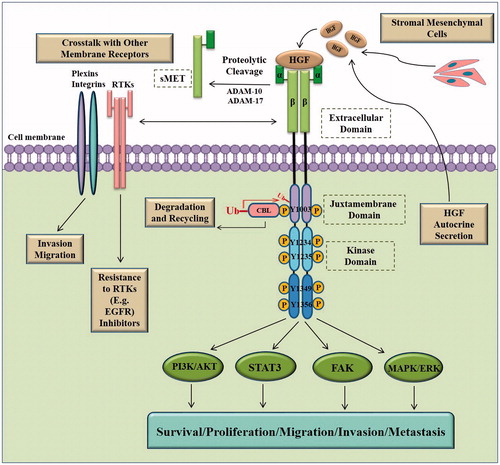
Hepatocyte growth factor (HGF), also known as scatter factor, is the natural endogenous ligand of the MET receptor and is secreted predominantly by mesenchymal cells as an inactive precursor (pro-HGF). For HGF to be activated, pro-HGF is proteolytically cleaved at the Arg494-Val495 bond by enzymes such as serum HGF activator and cellular type II transmembrane serine proteinases to generate mature HGF [Citation29]. The mature bioactive form of HGF is a disulfide-linked heterodimer composed of α-chain (69 kD) and β-chain subunits (34 kD) [Citation5,Citation28].
MET signaling, which is normally activated by the binding of its natural ligand, HGF, results in receptor dimerization and phosphorylation of two tyrosine residues, Tyr1234 and Tyr1235 in the kinase catalytic domain [Citation30,Citation31]. Subsequent phosphorylation of the docking site residues, Tyr1349 and Tyr1356, leads to the recruitment of a network of intracellular adapter and effector proteins such as phosphoinositide 3-kinase (PI3K), phospholipase Cγ 1 (PLCγ1), growth factor receptor- bound protein 2 (GrB2), GrB2-associated binding protein 1 (GaB1), and signal transducer and activator of transcription 3 (STAT3). Consequently, MET mediated activation of several intracellular signaling pathways, including the PI3K)/Akt, STAT3, SRC/FAK (FAK, focal adhesion kinase) and mitogen-activated protein kinase (MAPK)/ERK pathways, occurs [Citation7,Citation25,Citation28,Citation32–34]. Activation of these pathways results in the emergence of diverse cellular hallmarks of cancer including cell proliferation, survival, inhibition of apoptosis, migration, invasion and metastasis () [Citation6,Citation25,Citation28,Citation31,Citation35].
HGF/MET signaling is tightly regulated by various control mechanisms that attenuate or terminate the activated pathways. One such mechanism, which negatively regulates receptor signaling, is the internalization and degradation/recycling of the MET receptor via the recruitment of casitas B lineage lymphoma proto-oncogene (CBL), a ubiquitin-protein ligase. The ubiquitination of phosphorylated MET can occur through the direct interaction of CBL with Tyr1003 in the JM domain or indirectly by its binding to Tyr1356 via the Grb2 adaptor protein [Citation10,Citation36,Citation37]. Another mechanism of MET downregulation is provided by the activity of tyrosine-specific phosphatases including PP2A, DEP-1, SHP2 and PTP1B [Citation11,Citation36].
Ectodomain shedding and regulated proteolysis also lead to downregulation of MET activity. The MET receptor is proteolytically cleaved by sheddase enzymes including the members of “a disintegrin and metalloproteinase” (ADAM) family, such as ADAM-10 and ADAM-17, as well as γ-secretase, and results in the formation of a soluble extracellular ectodomain and a intracellular MET fragment that is rapidly degraded by the proteasome [Citation38,Citation39]. The soluble extracellular MET fragment (sMET) can bind to HGF, sequestering it from MET receptor binding and thus antagonizing MET signaling. Moreover, the interaction of sMET with full-length MET may also lead to impaired receptor dimerization [Citation7,Citation40–42].
Ectodomain shedding of MET and some other transmembrane receptors may have important impacts on the pathophysiology and drug response in different types of cancer [Citation42]. In this context, several studies have reported that expression of sMET correlates with cancer progression [Citation40].
I-A- The importance of HGF/MET aberrations as clinical biomarkers
HGF/MET targeted therapies have had inconsistent outcomes in different tumor types. While the outcome has been promising in several clinical trials [Citation12,Citation43–45], others have not been able to provide enough evidence for the clinical benefit of MET targeted small molecule inhibitors or antibodies [Citation46–50].
The study of the correlation of MET aberrations in tumor tissue with disease prognosis helps to distinguish the tumor types in which MET signaling has the highest level of biological relevance and a significant impact on tumor behavior. Hence, in addition to MET being a prognostic biomarker, the study of MET aberrations in tumor tissue helps to select cancer patients who could potentially benefit from HGF/MET targeted therapies.
The ultimate goals of studies that examine MET pathway activation in different types of cancer is to identify a predictive biomarker that enables stratification of patients with one cancer type into subpopulations and to identify those patients who are most likely to draw benefit from HGF/MET targeted therapies. This biomarker-guided approach, which is the cornerstone of precision medicine trials, could significantly increase the efficacy of targeted therapies [Citation51].
Several clinical studies have addressed these issues and reported MET aberrations as indicators of short survival and clinicopathological features of advanced disease in diverse types of cancer, such as gastric [Citation52], colorectal [Citation53], breast [Citation54], hepatocellular [Citation55], pancreatic [Citation56], and lung cancer [Citation57]. In addition, the predictive role of MET dysregulation has been explored in different settings, and some results indicate improved response to multiple MET targeted agents in MET-positive compared with MET-negative populations [Citation58–60]. These topics are covered in the next sections.
Alterations that may lead to HGF/MET signaling pathway activation in different tumors include gene overexpression, increased gene copy number gain (CNG), mutations in the kinase or non-kinase regions of the MET receptor as well as aberrant autocrine or paracrine HGF secretion, which have been thoroughly investigated in several different cancers () [Citation6,Citation12,Citation61,Citation62]. These alterations and the importance of each one as a biomarker are the subject of this review and are fully discussed in the following sections (summarized in ).
Figure 2. Aberrations in HGF/MET signaling pathway in cancer. Aberration of HGF/MET pathway may be caused by different molecular mechanisms including MET amplification, MET point mutations, exon 14 skipping mutations and excessive autocrine/paracrine HGF secretion. MET overexpression at the transcription level can be caused through transcription factors (e.g. Ets and Sp1) activation, hypoxia-inducible factor (HIF) activation and downregulation of repressor microRNAs. HGF overexpression can occur by transcriptional up-regulation due to mutations such as those in deoxyadenosine tract element (DATE) in the HGF gene promoter.
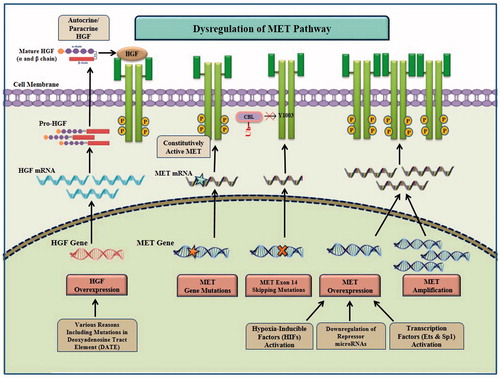
II- MET aberrant activation in tumor tissue as a prognostic biomarker
MET dysregulated signaling in tumor tissue can result from various genetic alterations in cancer cells including transcriptional dysregulation, MET gene amplification and mutational activations such as those resulting in exon 14 skipping ( and ) [Citation63]. Understanding how HGF/MET functions as a prognostic biomarker in different types of cancer may guide the identification of tumor types and subtypes that draw the maximum benefit from HGF/MET targeted therapies. summarizes the meta-analyses of studies on aberrant MET activation in various tumor tissues.
Table 1. Overview of meta-analysis studies on aberrant MET activation in tumor tissue as a prognostic biomarker in different types of cancer.
II-A- Increased MET expression
Increased expression of MET measured at both the protein and mRNA levels has been reported in several different types of tumors ( and ) [Citation14,Citation84–88]. MET overexpression can be a result of transcriptional up-regulation due to hypoxia-inducible factor (HIF) activation or alteration in other transcription factors including Ets and Sp1 [Citation89,Citation90]. It can also be caused by downregulation of repressor microRNAs, such as miR-1, miR-34, and miR-449a, that target MET [Citation90]. An abundance of MET receptor monomers on the cell membrane can induce spontaneous dimerization, phosphorylation and subsequent activation of the receptor in a ligand-independent manner, and hence result in the activation of downstream signaling pathways that ultimately lead to tumorigenesis [Citation91].
II-A-1- Non-small cell lung cancer (NSCLC)
Data from several meta-analyses have suggested that high MET expression is a negative prognostic biomarker in different cancers ( and ). A meta-analysis of hazard ratios (HRs) from 18 retrospective studies that included 5516 cases with non-small cell lung cancer (NSCLC) showed that high MET expression significantly increased the risk of mortality, even when studies responsible for heterogeneity (HR 1.52, 95% confidence interval (CI) 1.08–2.15) were excluded [Citation67]. Similarly, a meta-analysis by Pyo et al., with 4454 NSCLC cases from 22 studies, confirmed that MET positivity was significantly correlated with shorter overall survival (OS) (HR 1.551, 95% CI 1.101–2.184) [Citation66]. They observed that MET expression was significantly higher in non-squamous cell carcinomas and in patients with higher clinical stages [Citation66].
II-A-2- Breast cancer
In breast cancer patients, studies have shown that high levels of MET expression are associated with favorable prognosis [Citation92,Citation93], have no significant association [Citation94,Citation95], or report statistically significant association between MET overexpression and poor prognosis [Citation96–99]. The conflicting results of multiple trials have been evaluated in recent meta-analyses [Citation68,Citation69,Citation72]. An earlier meta-analysis of HRs from 21 studies that included 6010 breast cancer patients showed that MET overexpression was related to shorter OS (HR 1.52, 95% CI 1.15–2.01) in 17 studies that included 4228 patients as well as to shorter relapse-free survival (RFS) (HR 1.60, 95% CI 1.27–2.00) in 12 studies with 3570 patients [Citation70]. Pooled data in the fixed-effects model showed that MET was significantly correlated with poor OS in lymph node negative breast cancer (HR 2.04, 95% CI 1.48–2.80) and with poor RFS in hormone-receptor positive (HR 1.41, 95% CI 1.11–1.79) and triple negative breast cancer (HR 2.31, 95% CI 1.53–3.48), but did not correlate with prognosis in human epidermal growth factor receptor (EGFR)-2 positive breast cancer (HR 1.20, 95% CI 0.91–1.59) [Citation70]. Zhao et al. gathered 32 studies with 8281 patients; among these, 18 reports with 4751 cases were suitable for OS data analysis and 12 other studies with 3598 cases were available for disease free survival (DFS) data assessment. The data showed that MET overexpression was significantly associated with poor OS (HR 1.65, 95% CI 1.328–2.051) and poor DFS (HR 1.53, 95% CI 1.20–1.95). The results of subgroup analysis by immunohistochemistry (IHC) indicated a significantly poor prognosis in the patients with higher MET expression level [Citation68].
The differences in the characteristics of breast cancer between Asian and Western populations have been discussed in various reports [Citation100–102]. Results of subgroup analysis according to ethnicity has suggested that MET is a predictor of poor prognosis (both RFS and OS) in Western patients but not in the Asian patients [Citation70]. These findings agreed with the results of a recent meta-analysis that showed that MET oncogenic alterations were not associated with poor prognosis in Asian patients [Citation68].
II-A-3- Colorectal cancer
The value of MET overexpression as a prognostic biomarker in colorectal cancer was shown in two systematic reviews in 2015 [Citation71,Citation72]. Data from a meta-analysis of 11 studies that included 1563 patients and used the fixed-effects model demonstrated that patients with high MET expression had a significantly shorter OS (HR 1.33, 95% CI 1.06–1.59) and progression free survival (PFS) (HR 1.47, 95% CI 1.03–1.91) [Citation72]. The second meta-analysis, with a smaller number of samples, showed a significantly shorter OS and DFS in patients with high MET expression; the data related to the subgroup examined by IHC indicated a significantly shorter DFS in the patients with MET overexpression, and suggested that patients diagnosed with stage III–IV cancer had higher MET expression level compared to those diagnosed with stage I–II [Citation71].
II-A-4- Gastric cancer
Two meta-analyses have been performed on published articles in patients diagnosed with gastric cancer [Citation73,Citation74]; some of the same studies were included and analyzed in both these meta-analyses. The results of both studies revealed that higher MET expression was an indicator of poor prognosis in both early and advanced gastric cancer patients. Data from subgroup analysis related to method, race, tumor stage and type of aberration (amplification or expression) suggested that elevated MET expression had a significant negative impact on survival [Citation73,Citation74]. More recent published reports have confirmed these earlier findings [Citation52,Citation86,Citation103,Citation104].
II-A-5- Head and neck cancer
Several meta-analyses involving head and neck cancer patients have suggested the validity of MET overexpression as a negative prognostic biomarker [Citation75,Citation76]. Szturz et al. conducted a meta-analysis to explore the prognostic value of different cutoff levels of MET expression. They classified MET expression, measured by IHC, into three levels (I, II, and III) with an increasing order of positivity. Results showed that a high MET level, above cutoff level II, was associated with worse survival outcomes and higher disease stage [Citation76]. From 16 studies, 1948 patients were included in the meta-analysis by Kim et al. [Citation75], who found that patients with overexpression of MET had significantly inferior DFS (HR 1.49, 95% CI 1.04–2.14) and OS (HR 1.83, 95% CI 1.29–2.60) compared to those with low MET expression. Finally, another meta-analysis demonstrated that MET overexpression in the Asian subgroup had a significant association with poor OS but not with DFS [Citation77].
II-A-6- Other cancers
The value of MET expression in tumor tissue as a prognostic biomarker has been studied in pancreatic cancer [Citation78], hepatocellular carcinoma [Citation79], and biliary tract [Citation80], esophageal [Citation81], renal [Citation75], and cervical cancers [Citation83]. The details of these meta-analyses are shown in . In addition, several studies confirm that high expression of MET is associated with poor survival in other less prevalent cancers. For instance, Mao et al. have shown that in cholangiocarcinoma, patients with MET overexpression had significantly shorter OS and DFS compared to those with low MET expression (OS, p = .003 and PFS, p = .009). The expression of MET in patients with tumor tissues was significantly higher than that in adjacent tissues. Based on multivariate COX regression analysis, the high expression of MET was an independent risk factor for DFS and OS for patient with cholangiocarcinoma [Citation105]. Another study reported similar data in patients with glottic laryngeal squamous cell carcinoma [Citation106].
II-B- MET gene amplification
Amplification of the MET protooncogene or gene CNG, which causes protein overexpression and constitutive activation of the MET receptor (), has been detected in NSCLC [Citation107] and breast [Citation16], gastric [Citation52,Citation108] and renal cancers [Citation109]. Several methods, including fluorescence in situ hybridization (FISH) (the most widely used technique), silver in situ hybridization, quantitative polymerase chain reaction and Southern blotting, have been applied to detect MET gene amplification [Citation14,Citation85,Citation110]. Because different techniques and criteria have been used to detect gene amplification, the prevalence of MET amplification in cancer patients varies greatly in the literature. For instance, the rate of MET amplification based on FISH analysis ranged from 1.5 to 11% among those with gastric cancer [Citation52,Citation111,Citation112] and 2.4 to 4.1% for NSCLC patients [Citation113,Citation114], whereas the prevalence of high MET copy number was observed in up to ∼20% of NSCLC [Citation115] and ∼30% of gastric cancer [Citation116] patients by polymerase chain reaction (PCR)-based assays.
In NSCLC, the prognostic value of MET CNG and its association with poor overall survival was first shown by Okuda et al. [Citation117].
There are a few meta-analyses in the literature on the prognostic role of MET CNG in NSCLC [Citation64,Citation67,Citation118]. A recent meta-analysis combined the results from 21 studies that involved 7647 patients and showed the association between MET CNG and inferior OS (HR 1.45, 95% CI 1.16–1.80) [Citation64]. Subgroup analyses based on histology and ethnicity indicated that MET CNG significantly correlated with shorter survival, especially in patients with adenocarcinoma (HR 1.41, 95% CI 1.11–1.79) and in Asian populations (HR 1.58, 95% CI 1.32–1.88). However, the number of studies that reported data regarding DFS seemed to be insufficient to determine a significant association of high MET CNG with DFS (HR 1.37, 95% CI 0.88–2.12).
The prognostic value of MET CNG in NSCLC was consistent with the results of two previous meta-analyses published in 2014 [Citation67,Citation118]. Some patient populations from these studies shared the same patient origin. It has also been noted that FISH, followed by reverse transcriptase-PCR (RT-PCR), was the most widely used method for detection of CNG [Citation67,Citation118].
II-C-Exon 14 skipping mutations
Exon 14 of MET encodes the JM domain of the receptor tyrosine kinase, which contains an important tyrosine residue (Tyr1003) and is the binding site for CBL, an E3 ubiquitin-protein ligase. Mutations that result in exon 14 splicing alterations result in the loss of this important regulatory region in the MET receptor and lead to decreased ubiquitination and hence reduced lysosomal degradation and prolonged MET signaling [Citation85,Citation119]. This post translational dysregulation activates MET signaling in cancer cells and promotes oncogenesis [Citation6,Citation120].
Exon 14-skipping mutations have been amply reported in lung cancer, with a prevalence of approximately 3–5%, and in other tumor types, with probably lower frequencies [Citation121–123]. These mutations are very likely to confer sensitivity to MET targeted therapies [Citation65,Citation124,Citation125].
The association between the MET mutation and the clinico-pathological features and prognosis of NSCLC has been investigated in a meta-analysis of 11 retrospective studies [Citation65]. Data from only two studies reporting the HR on overall survival in patients with MET exon 14 mutations could be pooled [Citation126,Citation127]. The pooled results indicated that the presence of MET exon 14 mutations in NSCLC patients was correlated with a significantly poor prognosis (HR 1.82, CI 1.04–3.19). Of note, based on the histologic subtypes, the incidence of this mutation was detected mostly in pulmonary sarcomatoid carcinoma [Citation65].
II-D-MET mutations
MET germline and somatic mutations have been identified across different receptor domains including the kinase, JM, and extracellular domains in several tumor types (hereditary and sporadic papillary renal cell, gastric, head and neck, breast, and ovarian cancers) [Citation8,Citation9,Citation128]. However, there are few publications on the correlation between activating mutations and prognosis.
Kinase domain mutations such as Thr1191Ile (detected in hepatocellular carcinoma), Tyr1248Cys/Asp/His (sporadic and hereditary papillary renal cell carcinoma), Tyr1230Cys/Tyr1235Asp (head and neck squamous cell cancers), Asp1228Val (NSCLC), and Ala1108Ser (gastric cancer) prompt ligand-dependent or ligand-independent constitutive MET activation [Citation9,Citation11,Citation129]. In the JM domain, missense mutations such as Tyr1010Ile, Arg988Cys and Pro1009Ser have been reported in lung cancer, gastric, breast, ovarian and colorectal cancer [Citation9]. Extracellular Sema domain mutations (i.e. Glu168Asp, Leu299Phe, Ser323Gly, and Asn375Ser) have not yet been carefully examined, but likely affect the structure of the HGF-binding region and MET receptor dimerization [Citation12,Citation130,Citation131].
III- MET aberrant activation in tumor tissue as a predictive biomarker
III-A- MET activation biomarkers as a guide to stratify patients for MET-targeted therapies
The HGF/MET pathway has become an attractive therapeutic target because of its critical roles in regulating multiple processes involved in tumorigenesis and numerous pathways related to hallmarks of cancer. Several studies have addressed the important issue of using MET alterations in tumor tissue as predictive biomarkers for making clinical decisions on the administration of targeted therapies [Citation60,Citation132–134]. For example, in NSCLC patients, in addition to using predictive biomarkers such as EGFR mutations, PD-L1 expression and ALK/ROS1 rearrangements, which are already part of routine practice to guide therapeutic decision making, MET alterations are being considered as the next candidate to add to the list of predictive biomarkers [Citation135].
HGF/MET targeted therapies can be divided into the following groups: (1) selective type I inhibitors that bind to the active (phosphorylated) conformation of the receptor; (2) nonselective MET kinase inhibitors, such as type II and III inhibitors, that bind to the non-active un-phosphorylated conformation and allosteric site of the receptor, respectively; (3) anti-MET monoclonal antibodies; and (4) HGF-directed antibodies [Citation136–138] ().
Figure 4. HGF/MET-targeted therapies for management of cancer. Several strategies to hamper the activity of the HGF/MET pathway are available to patients or are currently under clinical investigation. These include small molecule tyrosine kinase inhibitors (TKIs) and monoclonal antibodies (mAb) against MET or HGF. Small molecule inhibitors can be mainly divided into three classes, labeled as types I, II, and III. Both type I and II inhibitors bind to the ATP binding site. Type I inhibitors more efficiently bind to the active protein kinase conformation (Asp-Phe-Gly (DFG)-in), while Type II inhibitors generally bind to a DFG out inactive conformation. Type I inhibitors may also bind to the inactive conformation. Type III inhibitors are non-ATP competitive and bind to a site distinct from the ATP binding pocket. Antibodies could be directed against the MET receptor itself or its ligand HGF.
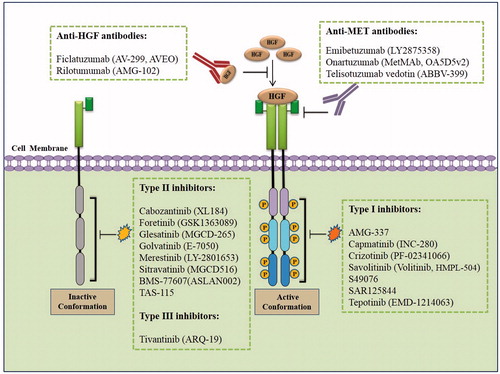
HGF/MET inhibitors have been assessed as monotherapy or in combination with other anticancer targeted therapies or cytotoxic agents [Citation136,Citation138]. The value of MET-related biomarkers such as MET overexpression, CNG and exon 14 splicing mutations to predict the response to MET targeted therapy has been documented in several clinical trials. Here, we provide an update on the most relevant data on the potential applications of MET as a predictive biomarker to identify patients most likely to benefit from therapy ().
Table 2. Overview of clinical trials examining the value of MET aberrations in tumor tissue as a predictive biomarker of drug response to guide stratification of cancer patients for HGF/MET targeted therapies.
An important biomarker-based phase II trial of savolitinib identified MET positive papillary renal cell carcinoma patients (patients with MET CNG, tumor HGF, MET overexpression or MET mutations). The authors observed that 40% of the cancers were MET driven, 46% were MET independent, and the status of the rest was unknown [Citation134].
The efficacy of onartuzumab, an anti-MET antibody (), has been investigated in patients with MET IHC-positive NSCLC in phase I, II and III clinical trials. These studies have reported different findings ( and ) [Citation46,Citation49,Citation133,Citation142,Citation154]. A phase II trial compared erlotinib, an EGFR inhibitor, plus onartuzumab in one group and erlotinib plus placebo in the second group of NSCLC patients who were tested for MET expression by IHC. This study showed increased PFS (HR 0.53, p = .04), OS (HR 0.37, p = .002), and overall response rate (ORR 3.2% vs. 8.6%) in MET-positive patients in the erlotinib plus onartuzumab arm of the study compared to patients receiving erlotinib and placebo. Of note, the MET-negative patients treated with onartuzumab plus erlotinib experienced worse outcomes in terms of PFS (2.01 vs. 3.02) as well as OS (8.1 vs. 15.3) [Citation142]. Similarly, in another study with patients with advanced NSCLC, the predictive role of several biomarkers including MET/EGFR amplification (FISH), MET overexpression (IHC), MET/EGFR mRNA expression, and high-plasma HGF levels were evaluated. The authors suggested that MET-IHC overexpression was the best predictor of patient benefit from onartuzumab [Citation133]. Despite the promising phase II data, a larger, double-blind, phase III study of onartuzumab plus erlotinib (vs. erlotinib plus placebo) that included 499 patients did not show clinical benefit in MET positive (2+/3+) metastatic NSCLC patients [Citation49]. This was consistent with a more recent report by Wakelee et al., which evaluated onartuzumab in combination with platinum/paclitaxel/bevacizumab or platinum/pemetrexed and failed to detect any benefit of combination therapy in either the intent-to-treat population or the patients with MET-positive tumors [Citation46].
Figure 5. Potential role of tumor tissue MET aberrations to predict the response to HGF/MET targeted therapies in different cancers. The prediction of the effectiveness of MET targeted therapies based on MET biomarkers in tumor tissue are shown in a summarizing scheme. Higher predictive value indicates that MET-positive patients have benefited from treatment, while lower predictive value shows that the drug has not been effective in MET-high patients. YYY: Biomarker value was proved by directly comparing MET-positive and MET-negative patients. YY: Biomarker value was independently shown in MET-positive patients and MET-negative patients. Y: Biomarker value was shown in MET-positive patients. N: No biomarker value was shown for MET aberration. The details of the studies are reported in . Reference numbers are shown in brackets.
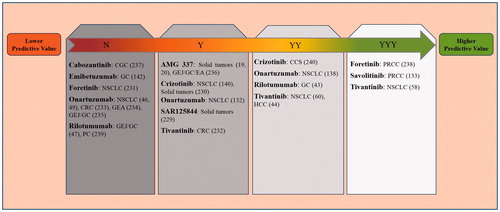
Unlike these disappointing results, MET small molecule inhibitors, including crizotinib [Citation125,Citation140] and tivantinib [Citation58,Citation60] have shown better antitumor activities in NSCLC patients with MET exon 14 deletions and MET overexpression, respectively.
In gastrointestinal tumors, several clinical studies have addressed the predictive role of MET dysregulation as an actionable target. These investigations have tested HGF/MET pathway inhibitors as single agents or in combination with other therapies, and have reported different outcomes [Citation20,Citation47,Citation155]. For instance, when 71 patients with advanced hepatocellular carcinoma were enrolled in a placebo-controlled double-blind phase II study, the results showed that tivantinib was associated with a trend towards improved time to progression (2.7 vs. 1.7 months, p = .03), PFS (2.2 vs. 1.4 months, p = .02), and OS (7.2 vs. 3.8 months, p = .01) in the MET-high patients [Citation44]. Moreover, a recent study evaluating the effect of rilotumumab administration, an anti-HGF antibody, on clinical outcome has shown a survival benefit in MET-positive patients, [Citation43]. However, Sakai et al. described unfavorable clinical outcomes in a non-randomized phase II trial assessing emibetuzumab, an anti-MET antibody, in Asian patients with advanced gastric adenocarcinoma with clinical predictors of MET status based on IHC and/or FISH [Citation147]. However, in this report, out of 65 patients, only 15 patients were diagnosed as MET-positive and could be enrolled in the study.
AMG 337, a selective MET inhibitor tested in a phase II clinical trial, has shown significant antitumor effect in gastric/gastro-esophageal junction/esophageal adenocarcinoma patients with MET amplification, but not in MET-amplified NSCLC [Citation20]. AMG 337 also showed promising results in a phase I study in patients with MET-amplified solid tumors [Citation19]. The above reports are summarized in .
As mentioned above, dysregulation of the MET pathway can arise from different mechanisms (). Our knowledge of the nature of these aberrations may have useful clinical implications. For example, in patients with MET amplification, receptor activation occurs mainly via ligand-independent mechanisms; therefore, small molecule inhibitors targeting the kinase domain could be more advantageous than anti-MET/HGF monoclonal antibodies [Citation15].
Moreover, an important challenge is to identify the patients in which tumor growth and invasion are critically dependent on MET alterations. Although MET overexpression is used frequently as a predictive factor to detect MET activation, it may not necessarily lead to oncogenic addiction in cancer cells [Citation14,Citation156]. Therefore, it has been argued that the use of IHC may have limitations in the selection of patients for anti-MET therapy.
Finally, methodologies that are applied to evaluate biomarkers need to be optimized. Scoring methods for the assessment of MET aberrations have not been validated extensively. This critical pitfall is demonstrated by the high variability in the percentage of patients who are designated as MET-high or MET-low. For example, high MET expression detected by IHC in gastric cancer ranged from 20–80% [Citation73]. Similarly, MET amplification detected by FISH in NSCLC varied from 1–40% [Citation64].
III-B- MET activation biomarkers as a guide to stratify patients with resistance to EGFR-TKIs
Functional interactions between MET and other cell surface receptors have been widely characterized [Citation32]. MET receptors not only form homodimers but also contribute to hetero interactions with other RTKs that result in fully activated downstream signaling, just as is seen following homodimerization [Citation32,Citation157]. The MET signaling pathway can be activated by a variety of MET interacting molecules, for example, membrane proteins or receptors such as plexins, integrins and other RTKs such as vascular endothelial growth factor receptor (VEGFR), insulin-like growth factor 1 receptor (IGF1R), EGFR, RET and AXL () [Citation157–159]. These processes may play an important role in cancer progression and specially in resistance to targeted therapies.
Among RTKs, the most studied interaction is between MET and EGFR. This communication is believed to induce MET activation in the absence of HGF after stimulation of cells with the EGFR ligands, EGF or transforming growth factor-α (TGF-α) [Citation160].
These biological processes have important clinical implications, because EGFR-TKIs provide an important asset for management of certain subsets of NSCLC patients [Citation161]. However, despite impressive initial responses, acquired resistance eventually occurred in a considerable number of patients. Different molecular events underlie this drug resistance, the main mechanism being the occurrence of secondary EGFR point mutations such as Tre790Met [Citation162–165]. However, activation of the MET pathway has also been consistently observed as another main driver of resistance, which leads to increased downstream oncogenic signaling in the presence of continuous treatment with anti-EGFR agents [Citation166–168].
A study that further supports these observations demonstrated that overexpression of TGF-α in colorectal cancer cells contributed to EGFR inhibition resistance by increasing EGFR/MET interaction and MET phosphorylation [Citation169]. However, resistance could be overcome by combined inhibition of EGFR and MET, as indicated in human lung, pancreatic and breast tumor xenografts [Citation7,Citation170].
Some studies have addressed the important issue of the influence of the MET pathway on resistance to EGFR targeted therapies using MET aberrations in tumor tissue as predictive biomarkers [Citation142]. MET inhibitors may be effective in overcoming this resistance and are currently being studied in several randomized clinical trials. As reported by van Veggel et al., MET amplification could mediate EGFR-TKI resistance in patients with EGFR mutation positive NSCLC. It was indeed shown that 50% of patients receiving crizotinib as monotherapy or in combination with an EGFR-TKI experienced partial response; however, responses were typically not lasting, and the median PFS was only 3.5 months (95% CI 1.4–5.2) [Citation161]. In a multicenter retrospective study, the clinical response to MET inhibitors, mostly crizotinib, was investigated in patients with metastatic EGFR-mutated NSCLC with MET amplification or overexpression as evaluated on a post-progression re-biopsy. Among patients receiving a MET inhibitor as a single agent or in combination with anti-EGFR agents, an objective response was reported in only 2 out of 19 evaluable patients [Citation171]. Another phase II trial evaluated whether acquired resistance to erlotinib in patients who harbored MET overexpression could be overcome by emibetuzumab, a monoclonal anti-MET antibody. The ORR was increased in both the monotherapy and combination arms in MET-high patients (≥60% of cells ≥2+ by IHC) compared with MET positive cases (≥10% of cells ≥2+) [Citation172]. Moreover, a combination of capmatinib (INC280) and gefitinib was assessed in a phase II study in EGFR-mutated NSCLC patients with acquired resistance to EGFR-TKI. Of the 65 evaluable patients with high MET expression, the ORR was 18% and the disease control rate (DCR) was 80%. A higher response rate was observed in MET-amplified patients (7 out of 23 patients with CNG ≥ 6 had partial responses (OS 30%) [Citation173]. Moreover, a few case reports have documented complete response to crizotinib treatment in patients with MET-overexpressing NSCLC after developing EGFR-TKI resistance [Citation174].
IV- Circulating MET levels as diagnostic and prognostic biomarkers
Several studies have investigated the potential utility of the soluble truncated ectodomain of MET protein (sMET) as a biomarker in different types of cancer and the correlation between sMET and tissue MET protein expression (). Most of these studies have reported that circulating MET correlates with tumor tissue expression levels [Citation57,Citation175,Citation177,Citation179,Citation180], although this idea was rejected by one report [Citation55]. In a study of 198 patients with NSCLC, Gao et al. observed that the plasma sMET levels were significantly correlated with tissue MET protein expression levels (p < .001). The OS was 9.5 vs. 22.2 months for patients with high sMet levels (>766 ng/mL) compared with patients having low levels of sMet (<766 ng/mL, respectively, p < .001). The average plasma sMET concentration was significantly higher in tissue MET-positive patients compared to subjects with MET-negative tumors or healthy individuals [Citation57]. The results of multivariate analysis in another study suggested that the sMet concentration was the strongest prognostic factor for PFS after EGFR-TKI therapy (HR 3.583, 95% CI 1.379–9.312) [Citation181].
Table 3. Overview of studies on the value of circulating soluble MET (sMET) levels as diagnostic or prognostic biomarkers in patients with different types of cancer.
A study by Barisione et al. evaluated the prognostic value of the serum sMET level in patients with metastatic uveal melanoma; the survival analysis revealed that cases exhibiting lower MET expression had a higher median survival time compared with patients expressing high levels [Citation179]. In addition, this study investigated the diagnostic role of sMet by discriminating metastatic uveal melanoma from nonmetastatic uveal melanoma and healthy subjects using receiver operating characteristic (ROC) curves with area under the curve (AUC) of 0.82 (95% CI 0.68–0.95, p < .001) and 0.83 (95% CI 0.71–0.95, p < .001), respectively [Citation179].
Kaye et al. [Citation177] investigated the association of plasma sMET with prostate cancer. Remarkably, they identified higher levels of sMET in plasma samples of groups of patients with benign (n = 109) and malignant (n = 236) diseases when compared to 80 healthy controls (p < .0001). sMET could also differentiate between malignant cases and healthy individuals with an AUC value of 0.8309 (sensitivity 79%, specificity 94%, p < .0001). However, the median sMET level was found not to correlate with invasive disease, metastasis, pathological grade and tumor stage [Citation177]. In contrast to these results, a study of 156 patients with localized and metastatic prostate cancer reported that soluble urinary sMET had a significant correlation with tumor metastasis. Urinary sMET showed an AUC value of 0.90 (95% CI 0.84–0.95) in discriminating localized from metastatic disease [Citation62]. In addition, another study reported that urinary MET levels could distinguish between patients with invasive vs. not invasive bladder cancer (AUC 0.7008, p < .0001) [Citation178].
In contrast to the above studies, in a case-control set of 290 subjects, the sMET level was significantly decreased among gastric cancer patients compared to controls (p < .0001) [Citation176]. Intriguingly, this longitudinal cohort study showed that soluble MET levels appeared to decrease before the onset of gastric cancer [Citation176].
Independent studies have suggested that CagA, the H. pylori effector protein, stimulates cancer-associated signal transduction by forming a complex with MET [Citation182–184]. Although there was no significant genotype–phenotype interaction between soluble MET protein and single nucleotide polymorphism (SNPs) of the CagA-related genes, after adding the genetic counts of these SNPs, the diagnostic value of MET protein to distinguish gastric cancer patients from normal individuals improved significantly [Citation176].
V- Circulating HGF levels as diagnostic, prognostic and predictive biomarkers
HGF gene expression has been shown to be upregulated by cytokines and growth factors including TNF-α, IL-1, EGF, fibroblast growth factor, platelet derived growth factor and prostaglandins as well as by interactions with other RTKs such as EGFR () [Citation185–187]. In addition, HGF is frequently co-expressed with MET in cancer cells and generates an autocrine receptor activation loop [Citation11]. In the human HGF gene promoter, a repeat of 30 deoxy adenosines, called the deoxyadenosine tract element (DATE), acts as a transcriptional repressor. Truncation mutations within DATE result in constitutive activation of the HGF promoter and subsequent aberrant HGF expression [Citation186]. In breast cancer patients, 51% of African Americans and 15% of individuals of mixed European ethnic background harbored a mutant DATE variant (25 As or fewer) in their tumor cells [Citation186].
The prognostic value of circulating HGF levels has been reported by several investigators ( and ). Most of these studies observed a negative correlation between HGF levels and survival of patients with different types of cancer; however, this association was not confirmed in all studies [Citation196].
Table 4. Overview of studies on the value of circulating HGF levels as diagnostic and prognostic biomarkers in patients with different types of cancer.
In addition to serving as a prognostic biomarker, HGF has the potential to serve as a diagnostic biomarker by distinguishing between cancer patients and healthy individuals, as well as to function as a predictive factor of response to therapy (). The role of HGF as a diagnostic, prognostic and predictive biomarker in different types of cancer is discussed below.
V-A- NSCLC
The assessment of sensitivity and specificity of plasma HGF levels in the study by Fang et al. suggested that HGF was not sensitive enough to detect early stage NSCLC (stage I–II) reliably; this finding may be due to the small sample size [Citation206]. Similar observations regarding the diagnostic value of HGF were reported in a later study that included a larger number of patients [Citation207]. The sensitivity of plasma HGF level was significantly higher in lung squamous cell cancer patients (stage III–IV) [Citation206].
The relationship between increased HGF and clinical outcome and drug response has been explored in several studies in lung cancer patients [Citation189,Citation190,Citation208,Citation209]. In a recent study performed in 81 patients receiving anticancer treatment (53 and 48 patients received first-line and second-line therapy, respectively), high serum HGF concentration after first-line chemotherapy predicted a shorter PFS in second-line treatment compared with low serum HGF [Citation188].
V-B- Breast cancer
The possible prognostic value of increased serum HGF levels in breast cancer was suggested for the first time by Toi et al. [Citation210] in a Japanese cohort of patients. Serum levels of HGF were analyzed by enzyme-linked immunosorbent assay in 200 primary breast cancer patients. Increased serum HGF levels were associated with a statistically significant worse prognosis in terms of DFS (p = .0001). Other investigators also demonstrated that higher serum levels of soluble HGF were associated with more lymph node involvement, higher frequency of poorly differentiated tumors, more advanced cancer stages and distant metastases [Citation211–214]. In a ROC analysis, the AUC for HGF was 0.695, which indicated that HGF could diagnose the estrogen receptor positive from the negative tumors in primary breast cancer patients [Citation211].
However, a study by Kim et al. reported conflicting data regarding the prognostic value of HGF; when patients were divided into four groups based on their HGF levels, only those with the highest HGF levels showed a trend towards a longer DFS (p = .008) [Citation191].
V-C- Colorectal cancer
A recent meta-analysis by Huang et al. combined results from nine studies that investigated the correlation between HGF and the prognosis and survival of colorectal cancer patients. The results indicated that overexpression of HGF was associated with a worse prognosis, considering both OS (HR 2.50, 95% CI 2.12–2.96) and DFS (HR 1.99, 95% CI 1.59–2.50) [Citation53]. Toiyama et al. observed that in patients undergoing colorectal carcinoma resection, elevated serum HGF levels correlated with tumor size, lymph node metastasis, and distant metastasis [Citation215].
In addition, the relationship between serum HGF and therapeutic responses was studied in colorectal cancer patients receiving bevacizumab and other therapies. High levels of HGF were associated with shorter PFS and OS, regardless of the type of treatment. Also, patients with lower pretreatment plasma levels of HGF showed remarkably larger benefit from bevacizumab treatment in terms of PFS and OS compared with those with high HGF concentration [Citation192].
V-D- Esophageal cancer
In esophageal squamous cell carcinoma, Ren et al. were the first to demonstrate that serum levels of HGF were elevated in patients compared to the control group (600 vs. 214 pg/mL, respectively, p < .001). Higher serum levels of HGF also showed significant correlation with the stage of disease and survival [Citation216].
Similarly, in another study, increased serum levels of HGF were found to be correlated with tumor stage (p = .002) and metastasis (p < .001) [Citation217]. Conversely, conflicting results emerged from a study concerning the prognostic value of HGF in esophageal squamous cell carcinoma patients. No association between circulating HGF and survival or response to therapy was found in patient receiving neoadjuvant chemo(radio)therapy. These conflicting results may be explained by the long-term effect of treatment, because all samples were taken after neoadjuvant therapy [Citation196].
V-E- Gastric cancer
Studies in gastric cancer patients have widely reported elevated serum HGF levels and their relationship with clinico-pathological features [Citation194,Citation218–220].
In addition, the role of serum HGF in predicting the response to treatment was evaluated by Takahashi et al. in gastric cancer patients [Citation193]. Among 46 patients treated with trastuzumab, those with high levels of serum HGF had shorter OS (HR 3.857, 95% CI 1.309–11.361) and had a higher risk of progression compared to patients with low levels. Evidence was also provided that the serum HGF level was significantly inferior in responders compared with non-responders (p = .014) [Citation193].
V-F- Head and neck cancer
Le et al. evaluated the prognostic and predictive roles of plasma HGF in 498 patients with stage III–IV head and neck cancer who received radiotherapy with cisplatin or cisplatin plus tirapazamine, a hypoxic cell cytotoxin [Citation221]. Since HGF gene expression was upregulated by hypoxic conditions [Citation221], they hypothesized that the concentration of HGF may detect a population that benefited from tirapazamine. High pretreatment HGF levels were a prognostic factor for shorter OS in patients receiving cisplatin, but not in those receiving tirapazamine/cisplatin. They also suggested that the combination of tirapazamine and cisplatin may be beneficial in patients with high HGF, but not in those with low HGF; however, these differences did not reach statistical significance [Citation221].
It should be noted that, although circulating HGF levels could be a good biomarker to predict the response to HGF/MET targeted therapy, the situation differs with other targeted therapies or chemotherapy.
V-G- Hepatocellular carcinoma
Similar findings have demonstrated the diagnostic value of serum HGF in hepatocellular cancer [Citation198,Citation222,Citation223]. However, several studies have reported conflicting results concerning the prognostic relevance of increased serum HGF levels. Rimassa et al. [Citation55] reported that patients with higher baseline HGF had a significantly shorter survival regardless of the therapy. In another study on HCC patients, no prognostic value was reported [Citation198].
In a cohort study, the effects of plasma HGF levels on survival and liver function were assessed in patients after radiotherapy and surgery for unresectable and resectable liver cancers, respectively. Increased plasma HGF levels significantly correlated with the CTP and MELD scores, indicators of the severity of liver disease. The authors suggested that pretreatment plasma HGF may be a useful biomarker to predict the susceptibility to radiation-induced liver dysfunction and patient survival after radiotherapy and liver transplantation [Citation224].
V-H- Pancreatic cancer
The diagnostic value of HGF in pancreatic cancer was reported in a study by Barakat et al. who evaluated patients with periampullary cancer, benign pancreatic tumor, and chronic pancreatitis [Citation225]. Plasma HGF levels were increased in patients with pancreatic cancer compared to normal controls, as well as in patients with benign pancreatic tumor and chronic pancreatitis. As shown by ROC curve analysis, HGF distinguished pancreatic cancer patients from subjects with benign conditions (sensitivity 84%, specificity 90%, AUC 0.919). Of note, 10 days after pancreaticoduodenectomy, the plasma HGF levels in patient were significantly higher than preoperative levels (p = .0009), despite removal of the tumor. However, HGF returned to preoperative concentrations by one month after surgery. In addition, patients with early tumor recurrence had higher preoperative HGF levels than patients without tumor recurrence. Similarly, in another study, after hepatopancreatic surgery, serum HGF levels were elevated compared to preoperative levels [Citation226].
V-I- Cervical cancer
In cervical cancer, the serum HGF level showed the highest diagnostic value in comparison to other factors for distinguishing cervical squamous cell carcinoma from the cervical intraepithelial neoplasia patients and healthy controls (AUC 0.99, sensitivity 77%, specificity 54%) [Citation199]. This study also demonstrated that serum HGF concentrations in HPV-positive patients were higher than HPV-negative individuals. These data were consistent with a previous study that reported a strong association between HGF overexpression and cervical HPV and HIV infections [Citation227]. In terms of the prognostic value of HGF, the study of Zhang et al. showed that cervical cancer patients with low serum HGF levels had significantly longer OS and PFS than those with high levels [Citation199].
V-J- Multiple myeloma
The prognostic and predictive value of HGF included in serum or plasma cytokine and angiogenic factor profile analysis has been explored in different tumor types [Citation205,Citation228–231]. In a recent study, Saltarella et al. evaluated the panel of angiogenic factors including HGF in multiple myeloma patients; they suggested that increased plasma HGF was associated with shorter relative PFS and predicted the benefit from therapeutic regimens [Citation205]. However, contrary to the findings of most studies evaluating angiogenic markers that have supported the role of HGF in treatment response prediction, in the study of Minarik et al., HGF was not proved to be a potential predictive factor of response to treatment in multiple myeloma patients [Citation232].
VI- Other biomarkers
VI-A- HGF level in tumor tissue
Tumor tissue levels of HGF have also been found to correlate with poor prognosis and have been proposed as potential biomarkers [Citation233,Citation234]. Higher HGF expression in esophageal tumor tissue was associated with a shorter OS [Citation196]. HGF overexpression was also observed in 67.1% of tonsillar squamous cell carcinoma patients, and patients with HGF overexpression experienced a shorter OS (49.1 vs. 93.8 months, p = .001) than those with a low MET expression; similar trends were observed with PFS (46.0 vs. 85.5 months, p = .004) [Citation235]. Moreover, in endometrial cancer, patients with HGF-positive, fibroblast growth factor-positive tumors had an increased risk of recurrence compared with cases with negative expression of both markers (HR 9.88, 95% CI 2.63–37.16) [Citation236].
VI-B- Circulating tumor DNA
In addition to the above-mentioned use of MET dysregulation for biomarker discovery in tumor tissue, recently a promising noninvasive method based on the detection of circulating tumor DNA (ctDNA) in blood samples has also been suggested as a cancer biomarker [Citation237–243]. One study comparing the MET amplification rate in different tissue-based and blood-based analysis methods showed that the frequency of MET amplification using ctDNA in metastatic colorectal cancer patients after exposure to anti-EGFR antibody therapy was significantly increased compared with antibody-naïve patients (p < .001) [Citation237]. Similarly, a case report of a treatment refractory patient with metastatic colorectal cancer showed that MET amplification was detected in ctDNA using next-generation sequencing, but not in tissue biopsy samples. The significant response that the patient experienced after treatment with the combination of cabozantinib plus panitumumab led to the suggestion that MET amplification in ctDNA may be a predictive biomarker for response [Citation244]. Finally, in NSCLC, a recent study demonstrated the potential clinical utility of ctDNA as a guide for therapy when tissue DNA was insufficient or unavailable [Citation240].
VI-C- Circulating mRNA
Circulating tumor-related genes that can be easily detected by RT-quantitative PCR in the serum or plasma of cancer patients may be reliable diagnostic and prognostic biomarkers in several types of cancer [Citation245]. One study comparing the expression of five mRNA species, MET, CEA, GalNAc-T, hTERT and MUC-1, in peripheral blood in patients with gastric cancer showed that the MET mRNA level was associated with the T stage (p = .025), lymph node metastasis (p = .036), distant metastasis (p = .031) and disease stage (p = .023) [Citation246]. Similar results were reported in a later study which showed that 41.2% of patients had serum MET mRNA overexpression [Citation247].
Conclusions
Dysregulation of HGF/MET pathways in cancer cells may occur by MET gene overexpression, gene amplification or gene CNG, and several activating mutations including those causing exon 14 skipping. sMET and HGF levels in circulation could also be altered aberrantly.
MET aberrations in tumor tissue serve as unequivocal prognostic biomarkers in several types of cancer including NSCLC, breast, head and neck cancers as well as colorectal, gastric, pancreatic and other gastrointestinal tumors. On the other hand, the predictive value of MET activation biomarkers in tumor tissue has not always been consistent. Most studies have suggested that selection of patients based on MET biomarkers may have clinical utility by showing that MET-positive patients, in particular NSCLC subjects, carrying any of the aberrations drew the most benefit from HGF/MET targeted therapies. However, some studies have failed to show such straight-forward associations. The root of these discrepancies may lie in the methods used for assessment of pathway dysregulations in tumor tissue.
Similar to tissue MET aberrations, increased levels of sMET in the plasma or serum in cancer patients have been mostly associated with poor prognosis; however, the evidence is not as strong as for tissue MET. As a diagnostic biomarker, sMET levels have shown their value in prostate and bladder cancer as well as in melanoma, but this role has not been solidly established yet. On the other hand, HGF aberrant signaling, mostly detected in the circulation and sometimes also in tumor tissue, has been presented as a valuable prognostic and diagnostic biomarker; however, controversies exist on its predictive value.
A frequently-encountered problem is the high variation in the prevalence of MET/HGF alterations in different reports. In this context, the main challenge is the standardization and unification of measurement techniques and scoring systems, especially those used in the determination of expression levels in tumor tissue. Another important challenge is to identify cancer types and subtypes that involve MET oncogenic addiction. This is of vital importance, because only these tumor types may benefit from HGF/MET targeted therapies.
While the prognostic value of HGF/MET biomarkers has been largely accepted, the diagnostic value of HGF/MET biomarkers is a new field with promising possibilities, and many questions remain to be answered regarding their role as biomarkers to predict the response to targeted therapies.
The HGF/MET pathway has emerged as an important actionable target across many solid tumors, and biomarker discovery has become essential to guide clinical interventions in this field. Moving along this path should pave the way towards personalized medicine.
Abbreviations | ||
ADAM | = | a disintegrin and metalloproteinase |
AUC | = | area under the curve |
CBL | = | casitas B lineage lymphoma proto-oncogene |
CI | = | confidence interval |
CNG | = | copy number gain |
DFS | = | disease free survival |
EGFR | = | epithelial growth factor receptor |
FAK | = | focal adhesion kinase |
FISH | = | fluorescence in situ hybridization |
GaB1 | = | GrB2-associated binding protein 1 |
GrB2 | = | growth factor receptor-bound protein 2 |
HGF | = | hepatocyte growth factor |
HIF | = | hypoxia-inducible factor |
HR | = | hazard ratio |
IGF1R | = | insulin-like growth factor 1 receptor |
IHC | = | immunohistochemistry |
JM | = | juxtamembrane |
MET | = | mesenchymal-epithelial transition tyrosine kinase receptor, c-MET |
NSCLC | = | non-small cell lung cancer |
ORR | = | overall response rate |
OS | = | overall survival |
PCR | = | polymerase chain reaction |
PFS | = | progression free survival |
PI3K | = | phosphoinositide 3-kinase |
PLC-γ | = | phospholipase Cγ |
RFS | = | relapse free survival |
ROC | = | receiver operating characteristic |
RTK | = | receptor tyrosine kinase |
RT-PCR | = | reverse transcriptase- polymerase chain reaction |
sMET | = | soluble MET, soluble truncated ectodomain of MET protein |
STAT3 | = | signal transducer and activator of transcription 3 |
TGF-α | = | transforming growth factor-α |
TKI | = | tyrosine kinase inhibitor |
VEGFR | = | vascular endothelial growth factor receptor |
Supplementary_Figure_1B_-_DFS_FOREST_PLOT_JPEG2_-_ed_LCA.jpg
Download JPEG Image (80.7 KB)Supplementary_Figure_1A_-_OS_FOREST_PLOT_JPEG_-_ed_LCA.jpg
Download JPEG Image (101.7 KB)Supplementary_Table_1__circulating__HGF__-_ed_LCA.docx
Download MS Word (38.3 KB)Disclosure statement
The authors declare no conflict of interest.
Additional information
Funding
References
- Bray F, Ferlay J, Soerjomataram I. Global cancer statistics 2018: GLOBOCAN estimates of incidence and mortality worldwide for 36 cancers in 185 countries. CA Cancer J Clin. 2018;68:394–424.
- Fitzmaurice C, Allen C, Barber RM, et al. Global, regional, and national cancer incidence, mortality, years of life lost, years lived with disability, and disability-adjusted life-years for 32 cancer groups, 1990 to 2015: a systematic analysis for the global burden of disease study. JAMA Oncol. 2017;3:524–548.
- Torre LA, Bray F, Siegel RL, et al. Global cancer statistics, 2012. CA Cancer J Clin. 2015;65:87–108.
- Allemani C, Matsuda T, Di Carlo V, et al. Global surveillance of trends in cancer survival 2000-14 (CONCORD-3): analysis of individual records for 37,513,025 patients diagnosed with one of 18 cancers from 322 population-based registries in 71 countries. Lancet. 2018;391:1023–1075.
- Zhang Y, Xia M, Jin K, et al. Function of the c-Met receptor tyrosine kinase in carcinogenesis and associated therapeutic opportunities. Mol Cancer. 2018;17:45–58.
- Van Der Steen N, Giovannetti E, Pauwels P, et al. cMET exon 14 skipping: from the structure to the clinic. J Thorac Oncol. 2016;11:1423–1432.
- Gherardi E, Birchmeier W, Birchmeier C, et al. Targeting MET in cancer: rationale and progress. Nat Rev Cancer. 2012;12:89–103.
- Schmidt L, Duh FM, Chen F, et al. Germline and somatic mutations in the tyrosine kinase domain of the MET proto-oncogene in papillary renal carcinomas. Nat Genet. 1997;16:68–73.
- Tovar EA, Graveel CR. MET in human cancer: germline and somatic mutations. Ann Transl Med. 2017;5:205–210.
- Matsumoto K, Umitsu M, De Silva DM, et al. Hepatocyte growth factor/MET in cancer progression and biomarker discovery. Cancer Sci. 2017;108:296–307.
- Maroun CR, Rowlands T. The Met receptor tyrosine kinase: a key player in oncogenesis and drug resistance. Pharmacol Ther. 2014;142:316–338.
- Bradley CA, Salto-Tellez M, Laurent-Puig P, et al. Targeting c-MET in gastrointestinal tumours: rationale, opportunities and challenges. Nat Rev Clin Oncol. 2017;14:562–576.
- Hack SP, Bruey JM, Koeppen H. HGF/MET-directed therapeutics in gastroesophageal cancer: a review of clinical and biomarker development. Oncotarget. 2014;5:2866–2880.
- Finocchiaro G, Toschi L, Gianoncelli L, et al. Prognostic and predictive value of MET deregulation in non-small cell lung cancer. Ann Transl Med. 2015;3:83–94.
- Salgia R. MET in lung cancer: biomarker selection based on scientific rationale. Mol Cancer Ther. 2017;16:555–565.
- Ho-Yen CM, Jones JL, Kermorgant S. The clinical and functional significance of c-Met in breast cancer: a review. Breast Cancer Res. 2015;17:52–62.
- Boromand N, Hasanzadeh M, ShahidSales S, et al. Clinical and prognostic value of the C-Met/HGF signaling pathway in cervical cancer. J Cell Physiol. 2018;233:4490–4496.
- Trovato M, Campenni A, Giovinazzo S, et al. Hepatocyte growth factor/C-Met axis in thyroid cancer: from diagnostic biomarker to therapeutic target. Biomark Insights. 2017;12:11–18.
- Hong DS, LoRusso P, Hamid O, et al. Phase I study of AMG 337, a highly selective small-molecule met inhibitor, in patients with advanced solid tumors. Clin Cancer Res. 2019;25:2403–2413.
- Van Cutsem E, Karaszewska B, Kang YK, et al. A multicenter phase II study of AMG 337 in patients with MET-amplified gastric/gastroesophageal junction/esophageal adenocarcinoma and other MET-amplified solid tumors. Clin Cancer Res. 2019;25:2414–2423.
- Thewke DP, Kou J, Fulmer ML, et al. The HGF/MET signaling and therapeutics in cancer. In: Matsuo H, Kataoka H, Xie Q, editors. Regulation of signal transduction in human cell research. Singapore: Springer; 2018. p. 155–181.
- Giordano S, Ponzetto C, Di Renzo MF, et al. Tyrosine kinase receptor indistinguishable from the c-met protein. Nature. 1989;339:155–156.
- Bottaro DP, Rubin JS, Faletto DL, et al. Identification of the hepatocyte growth factor receptor as the c-met proto-oncogene product. Science. 1991;251:802–804.
- Baldanzi G, Graziani A. Physiological signaling and structure of the HGF receptor MET. Biomedicines. 2014;3:1–31.
- Yamaoka T, Kusumoto S, Ando K, et al. Receptor tyrosine kinase-targeted cancer therapy. Int J Mol Sci. 2018;19:3491–3526.
- Schiering N, Knapp S, Marconi M, et al. Crystal structure of the tyrosine kinase domain of the hepatocyte growth factor receptor c-Met and its complex with the microbial alkaloid K-252a. Proc Natl Acad Sci USA. 2003;100:12654–12659.
- Gherardi E, Sandin S, Petoukhov MV, et al. Structural basis of hepatocyte growth factor/scatter factor and MET signalling. Proc Natl Acad Sci USA. 2006;103:4046–4051.
- Organ SL, Tsao M-S. An overview of the c-MET signaling pathway. Ther Adv Med Oncol. 2011;3:S7–S19.
- Fukushima T, Uchiyama S, Tanaka H, et al. Hepatocyte growth factor activator: a proteinase linking tissue injury with repair. Int J Mol Sci. 2018;19:3435–3445.
- Duplaquet L, Kherrouche Z, Baldacci S, et al. The multiple paths towards MET receptor addiction in cancer. Oncogene. 2018;37:3200–3215.
- Comoglio PM, Trusolino L, Boccaccio C. Known and novel roles of the MET oncogene in cancer: a coherent approach to targeted therapy. Nat Rev Cancer. 2018;18:341–358.
- Viticchiè G, Muller P. c-Met and other cell surface molecules: interaction, activation and functional consequences. Biomedicines. 2015;3:46–70.
- Ponzetto C, Bardelli A, Zhen Z, et al. A multifunctional docking site mediates signaling and transformation by the hepatocyte growth factor/scatter factor receptor family. Cell. 1994;77:261–271.
- Weidner KM, Di Cesare S, Sachs M, et al. Interaction between Gab1 and the c-Met receptor tyrosine kinase is responsible for epithelial morphogenesis. Nature. 1996;384:173–176.
- Giovannetti E, van der Borden CL, Frampton AE, et al. Never let it go: stopping key mechanisms underlying metastasis to fight pancreatic cancer. Semin Cancer Biol. 2017;44:43–59.
- Zhang J, Babic A. Regulation of the MET oncogene: molecular mechanisms. Carcinogenesis. 2016;37:345–355.
- Mohapatra B, Ahmad G, Nadeau S, et al. Protein tyrosine kinase regulation by ubiquitination: critical roles of Cbl-family ubiquitin ligases. Biochim Biophys Acta. 2013;1833:122–139.
- Chalupsky K, Kanchev I, Zbodakova O, et al. ADAM10/17-dependent release of soluble c-Met correlates with hepatocellular damage. Folia Biol (Praha). 2013;59:76–86.
- Merilahti JAM, Elenius K. Gamma-secretase-dependent signaling of receptor tyrosine kinases. Oncogene. 2019;38:151–163.
- Hass R, Jennek S, Yang Y, et al. c-Met expression and activity in urogenital cancers–novel aspects of signal transduction and medical implications. Cell Commun Signal. 2017;15:10–18.
- Prat M, Oltolina F, Basilico C. Monoclonal antibodies against the MET/HGF receptor and its ligand: multitask tools with applications from basic research to therapy. Biomedicines. 2014;2:359–383.
- Miller MA, Sullivan RJ, Lauffenburger DA. Molecular pathways: receptor ectodomain shedding in treatment, resistance, and monitoring of cancer. Clin Cancer Res. 2017;23:623–629.
- Zhu M, Tang R, Doshi S, et al. Exposure-response analysis of rilotumumab in gastric cancer: the role of tumour MET expression. Br J Cancer. 2015;112:429–437.
- Santoro A, Rimassa L, Borbath I, et al. Tivantinib for second-line treatment of advanced hepatocellular carcinoma: a randomised, placebo-controlled phase 2 study. Lancet Oncol. 2013;14:55–63.
- Iveson T, Donehower RC, Davidenko I, et al. Rilotumumab in combination with epirubicin, cisplatin, and capecitabine as first-line treatment for gastric or oesophagogastric junction adenocarcinoma: an open-label, dose de-escalation phase 1b study and a double-blind, randomised phase 2 study. Lancet Oncol. 2014;15:1007–1018.
- Wakelee H, Zvirbule Z, De Braud F, et al. Efficacy and safety of onartuzumab in combination with first-line bevacizumab- or pemetrexed-based chemotherapy regimens in advanced non-squamous non-small-cell lung cancer. Clin Lung Cancer. 2017;18:50–59.
- Catenacci DV, Tebbutt NC, Davidenko I, et al. Rilotumumab plus epirubicin, cisplatin, and capecitabine as first-line therapy in advanced MET-positive gastric or gastro-oesophageal junction cancer (RILOMET-1): a randomised, double-blind, placebo-controlled, phase 3 trial. Lancet Oncol. 2017;18:1467–1482.
- Rimassa L, Assenat E, Peck-Radosavljevic M, et al. Second-line tivantinib (ARQ 197) vs. placebo in patients (Pts) with MET-high hepatocellular carcinoma (HCC): results of the METIV-HCC phase III trial [abstract]. J Clin Oncol. 2017;35: 4000.
- Spigel DR, Edelman MJ, O'Byrne K, et al. Onartuzumab plus erlotinib versus erlotinib in previously treated stage IIIb or IV NSCLC: results from the pivotal phase III randomized, multicenter, placebo-controlled METLung (OAM4971g) global trial [abstract]. J Clin Oncol. 2014;32:8000.
- Spigel DR, Edelman MJ, O’Byrne K, et al. Results from the phase III randomized trial of onartuzumab plus erlotinib versus erlotinib in previously treated stage IIIB or IV non-small-cell lung cancer: METLung. J Clin Oncol. 2017;35:412–420.
- Rashed WM. C-MET as a potential target therapy toward personalized therapy in some pediatric tumors: an overview. Crit Rev Oncol Hematol. 2018;131:7–15.
- Kim HS, Chon HJ, Kim H, et al. MET in gastric cancer with liver metastasis: the relationship between MET amplification and Met overexpression in primary stomach tumors and liver metastasis. J Surg Oncol. 2018;117:1679–1686.
- Huang CY, Zhou QY, Hu Y, et al. Hepatocyte growth factor is a prognostic marker in patients with colorectal cancer: a meta-analysis. Oncotarget. 2017;8:23459–23469.
- Wang M, Liang L, Lei X, et al. Evaluation of cMET aberration by immunohistochemistry and fluorescence in situ hybridization (FISH) in triple negative breast cancers. Ann Diagn Pathol. 2018;35:69–76.
- Rimassa L, Abbadessa G, Personeni N, et al. Tumor and circulating biomarkers in patients with second-line hepatocellular carcinoma from the randomized phase II study with tivantinib. Oncotarget. 2016;7:72622–72633.
- Neuzillet C, Couvelard A, Tijeras-Raballand A, et al. High c-Met expression in stage I-II pancreatic adenocarcinoma: proposal for an immunostaining scoring method and correlation with poor prognosis. Histopathology. 2015;67:664–676.
- Gao H-F, Li A-N, Yang J-J, et al. Soluble c-Met levels correlated with tissue c-Met protein expression in patients with advanced non-small-cell lung cancer. Clin Lung Cancer. 2017;18:85–91.
- Azuma K, Hirashima T, Yamamoto N, et al. Phase II study of erlotinib plus tivantinib (ARQ 197) in patients with locally advanced or metastatic EGFR mutation-positive non-small-cell lung cancer just after progression on EGFR-TKI, gefitinib or erlotinib. ESMO Open. 2016;1:e000063–e000070.
- Puzanov I, Sosman J, Santoro A, et al. Phase 1 trial of tivantinib in combination with sorafenib in adult patients with advanced solid tumors. Invest New Drugs. 2015;33:159–168.
- Scagliotti GV, Von Pawel J, Novello S, et al. Phase III multinational, randomized, double-blind, placebo-controlled study of tivantinib (ARQ 197) plus erlotinib versus erlotinib alone in previously treated patients with locally advanced or metastatic nonsquamous non-small-cell lung cancer. J Clin Oncol. 2015;33:2667–2674.
- Kentsis A, Reed C, Rice KL, et al. Autocrine activation of the MET receptor tyrosine kinase in acute myeloid leukemia. Nat Med. 2012;18:1118–1122.
- Russo AL, Jedlicka K, Wernick M, et al. Urine analysis and protein networking identify met as a marker of metastatic prostate cancer. Clin Cancer Res. 2009;15:4292–4298.
- Ma PC, Tretiakova MS, MacKinnon AC, et al. Expression and mutational analysis of MET in human solid cancers. Genes Chromosomes Cancer. 2008;47:1025–1037.
- Kim JH, Kim HS, Kim BJ. Prognostic value of MET copy number gain in non-small-cell lung cancer: an updated meta-analysis. J Cancer. 2018;9:1836–1845.
- Vuong HG, Ho ATN, Altibi AM, et al. Clinicopathological implications of MET exon 14 mutations in non-small cell lung cancer – a systematic review and meta-analysis. Lung Cancer. 2018;123:76–82.
- Pyo J-S, Kang G, Cho WJ, et al. Clinicopathological significance and concordance analysis of c-MET immunohistochemistry in non-small cell lung cancers: a meta-analysis. Pathol Res Pract. 2016;212:710–716.
- Guo B, Cen H, Tan X, et al. Prognostic value of MET gene copy number and protein expression in patients with surgically resected non-small cell lung cancer: a meta-analysis of published literatures. PLoS One. 2014;9:e99399–e99410.
- Zhao X, Qu J, Hui Y, et al. Clinicopathological and prognostic significance of c-Met overexpression in breast cancer. Oncotarget. 2017;8:56758–56767.
- Wang F, Li S, Zhao Y, et al. Predictive role of the overexpression for CXCR4, C-Met, and VEGF-C among breast cancer patients: a meta-analysis. Breast. 2016;28:45–53.
- Yan S, Jiao X, Zou H, et al. Prognostic significance of c-Met in breast cancer: a meta-analysis of 6010 cases. Diagn Pathol. 2015;10:62–71.
- Gao H, Guan M, Sun Z, et al. High c-Met expression is a negative prognostic marker for colorectal cancer: a meta-analysis. Tumor Biol. 2015;36:515–520.
- Liu Y, Yu X-F, Zou J, et al. Prognostic value of c-Met in colorectal cancer: a meta-analysis. World J Gastroenterol. 2015;21:3706–3710.
- Peng Z, Zhu Y, Wang Q, et al. Prognostic significance of MET amplification and expression in gastric cancer: a systematic review with meta-analysis. PLoS One. 2014;9:e84502–e84508.
- Yu S, Yu Y, Zhao N, et al. C-Met as a prognostic marker in gastric cancer: a systematic review and meta-analysis. PLoS One. 2013;8:e79137–79146.
- Kim JH, Kim BJ, Kim HS. Clinicopathological impacts of high c-Met expression in head and neck squamous cell carcinoma: a meta-analysis and review. Oncotarget. 2017;8:113120–113128.
- Szturz P, Budíková M, Vermorken JB, et al. Prognostic value of c-MET in head and neck cancer: a systematic review and meta-analysis of aggregate data. Oral Oncol. 2017;74:68–76.
- Vsiansky V, Gumulec J, Raudenska M, et al. Prognostic role of c-Met in head and neck squamous cell cancer tissues: a meta-analysis. Sci Rep. 2018;8:10370–10376.
- Kim JH, Kim HS, Kim BJ, et al. Prognostic value of c-Met overexpression in pancreatic adenocarcinoma: a meta-analysis. Oncotarget. 2017;8:73098–73104.
- Kim JH, Kim HS, Kim BJ, et al. Prognostic value of c-Met overexpression in hepatocellular carcinoma: a meta-analysis and review. Oncotarget. 2017;8:90351–90357.
- Zhou W, Jiang C, Zhan N, et al. Human epidermal growth factor receptor 2, epidermal growth factor receptor, and c-MET overexpression and survival in biliary tract cancer: a meta-analysis. J Can Res Ther. 2018;14:S28–S35.
- Ren J-L, Wu H-F, Wang W-J, et al. C-Met as a potential novel prognostic marker in squamous cell carcinoma and adenocarcinoma of esophagus: evidence from a meta-analysis. Panminerva Med. 2017;59:97–106.
- Kim JH, Kim BJ, Kim HS. Clinicopathological impacts of high c-Met expression in renal cell carcinoma: a meta-analysis and review. Oncotarget. 2017;8:75478–75487.
- Peng J, Qi S, Wang P, et al. Diagnosis and prognostic significance of c-Met in cervical cancer: a meta-analysis. Dis Markers. 2016;2016:6594016.
- Gambella M, Palumbo A, Rocci A. MET/HGF pathway in multiple myeloma: from diagnosis to targeted therapy? Expert Rev Mol Diagn. 2015;15:881–893.
- Zhang Y, Du Z, Zhang M. Biomarker development in MET-targeted therapy. Oncotarget. 2016;7:37370–37389.
- Choi J, Lee HE, Lee HS, et al. Evaluation of intratumoral and intertumoral heterogeneity of MET protein expression in gastric cancer. Appl Immunohistochem Mol Morphol. 2018;26:445–453.
- Kim I-H, Lee IH, Lee JE, et al. Prognostic impact of multiple clinicopathologic risk factors and c-MET overexpression in patients who have undergone resection of stage IB non–small-cell lung cancer. Clin Lung Cancer. 2016;17:e31–e43.
- Puvanenthiran S, Essapen S, Haagsma B, et al. Co-expression and prognostic significance of the HER family members, EGFRvIII, c-MET, CD44 in patients with ovarian cancer. Oncotarget. 2018;9:19662–19674.
- Pennacchietti S, Michieli P, Galluzzo M, et al. Hypoxia promotes invasive growth by transcriptional activation of the met protooncogene. Cancer Cell. 2003;3:347–361.
- Gambarotta G, Boccaccio C, Giordano S, et al. Ets up-regulates MET transcription. Oncogene. 1996;13:1911–1917.
- Ghiso E, Giordano S. Targeting MET: why, where and how? Curr Opin Pharmacol. 2013;13:511–518.
- Nakopoulou L, Gakiopoulou H, Keramopoulos A, et al. c-met tyrosine kinase receptor expression is associated with abnormal beta-catenin expression and favourable prognostic factors in invasive breast carcinoma. Histopathology. 2000;36:313–325.
- Koh YW, Lee HJ, Ahn J-H, et al. MET expression is associated with disease-specific survival in breast cancer patients in the neoadjuvant setting. Pathol Res Pract. 2014;210:494–500.
- Inanc M, Ozkan M, Karaca H, et al. Cytokeratin 5/6, c-Met expressions, and PTEN loss prognostic indicators in triple-negative breast cancer. Med Oncol. 2014;31:801–808.
- Zagouri F, Brandstetter A, Moussiolis D, et al. Low protein expression of MET in ER-positive and HER2-positive breast cancer. Anticancer Res. 2014;34:1227–1231.
- Lengyel E, Prechtel D, Resau JH, et al. C‐Met overexpression in node‐positive breast cancer identifies patients with poor clinical outcome independent of Her2/neu. Int J Cancer. 2005;113:678–682.
- Ocal IT, Dolled‐Filhart M, Thomas G, et al. Tissue microarray‐based studies of patients with lymph node negative breast carcinoma show that met expression is associated with worse outcome but is not correlated with epidermal growth factor family receptors. Cancer. 2003;97:1841–1848.
- Ghoussoub RA, Dillon DA, D'Aquila T, et al. Expression of c‐met is a strong independent prognostic factor in breast carcinoma. Cancer. 1998;82:1513–1520.
- Kim YJ, Choi JS, Seo J, et al. MET is a potential target for use in combination therapy with EGFR inhibition in triple‐negative/basal‐like breast cancer. Int J Cancer. 2014;134:2424–2436.
- Kim Y, Yoo K-Y, Goodman MT. Differences in incidence, mortality and survival of breast cancer by regions and countries in Asia and contributing factors. Asian Pac J Cancer Prev. 2015;16:2857–2870.
- Fan L, Goss PE, Strasser-Weippl K. Current status and future projections of breast cancer in Asia. Breast Care (Basel). 2015;10:372–378.
- Leong SP, Shen Z-Z, Liu T-J, et al. Is breast cancer the same disease in Asian and Western countries? World J Surg. 2010;34:2308–2324.
- Huang K-H, Sung I, Fang W-L, et al. Correlation between HGF/c-Met and Notch1 signaling pathways in human gastric cancer cells. Oncol Rep. 2018;40:294–302.
- Fuse N, Kuboki Y, Kuwata T, et al. Prognostic impact of HER2, EGFR, and c-MET status on overall survival of advanced gastric cancer patients. Gastric Cancer. 2016;19:183–191.
- Mao Z-Y, Zhu G-Q, Ren L, et al. Prognostic value of C-met expression in cholangiocarcinoma. Technol Cancer Res Treat. 2016;15:227–233.
- Jiang M, Zhang H, Xiao H, et al. High expression of c-Met and EGFR is associated with poor survival of patients with glottic laryngeal squamous cell carcinoma. Oncol Lett. 2018;15:931–939.
- Park C-K, Oh I-J, Choi Y-D, et al. A prospective observational study evaluating the correlation of c-MET expression and EGFR gene mutation with response to erlotinib as second-line treatment for patients with advanced/metastatic non-small-cell lung cancer. Oncology. 2018;94:373–382.
- Catenacci DV, Ang A, Liao WL, et al. MET tyrosine kinase receptor expression and amplification as prognostic biomarkers of survival in gastroesophageal adenocarcinoma. Cancer. 2017;123:1061–1070.
- Schiefer AI, Mesteri I, Berghoff AS, et al. Evaluation of tyrosine kinase receptors in brain metastases of clear cell renal cell carcinoma reveals cM et as a negative prognostic factor. Histopathology. 2015;67:799–805.
- Kawakami H, Okamoto I, Okamoto W, et al. Targeting MET amplification as a new oncogenic driver. Cancers (Basel). 2014;6:1540–1552.
- Kawakami H, Okamoto I, Arao T, et al. MET amplification as a potential therapeutic target in gastric cancer. Oncotarget. 2013;4:9–17.
- Liu Y, Shen D, Yin X, et al. HER2, MET and FGFR2 oncogenic driver alterations define distinct molecular segments for targeted therapies in gastric carcinoma. Br J Cancer. 2014;110:1169–1178.
- Sterlacci W, Fiegl M, Gugger M, et al. MET overexpression and gene amplification: prevalence, clinico-pathological characteristics and prognostic significance in a large cohort of patients with surgically resected NSCLC. Virchows Arch. 2017;471:49–55.
- Cappuzzo F, Marchetti A, Skokan M, et al. Increased MET gene copy number negatively affects survival of surgically resected non–small-cell lung cancer patients. J Clin Oncol. 2009;27:1667–1674.
- Beau-Faller M, Ruppert A-M, Voegeli A-C, et al. MET gene copy number in non-small cell lung cancer: molecular analysis in a targeted tyrosine kinase inhibitor naive cohort. J Thorac Oncol. 2008;3:331–339.
- Shi J, Yao D, Liu W, et al. Frequent gene amplification predicts poor prognosis in gastric cancer. Int J Mol Sci. 2012;13:4714–4726.
- Okuda K, Sasaki H, Yukiue H, et al. Met gene copy number predicts the prognosis for completely resected non-small cell lung cancer. Cancer Sci. 2008;99:2280–2285.
- Dimou A, Non L, Chae YK, et al. MET gene copy number predicts worse overall survival in patients with non-small cell lung cancer (NSCLC); a systematic review and meta-analysis. PLoS One 2014;9:e107677.
- Trusolino L, Bertotti A, Comoglio PM. MET signalling: principles and functions in development, organ regeneration and cancer. Nat Rev Mol Cell Biol. 2010;11:834–848.
- Kong-Beltran M, Seshagiri S, Zha J, et al. Somatic mutations lead to an oncogenic deletion of met in lung cancer. Cancer Res. 2006;66:283–289.
- Kim EK, Kim KA, Lee CY, et al. Molecular diagnostic assays and clinicopathologic implications of MET exon 14 skipping mutation in non–small-cell lung cancer. Clin Lung Cancer. 2019;20:e123–e132.
- Paik P, Sakai H, Bruns R, et al. 546TiP Tepotinib in non-small cell lung cancer (NSCLC) with MET-exon 14 skipping mutations (METex14+) and MET amplification (METamp); a phase II trial in progress [abstract]. Ann Oncol. 2018;29:ix150–ix169.
- Heist RS, Shim HS, Gingipally S, et al. MET exon 14 skipping in non-small cell lung cancer. Oncologist. 2016;21:481–486.
- Frampton GM, Ali SM, Rosenzweig M, et al. Activation of MET via diverse exon 14 splicing alterations occurs in multiple tumor types and confers clinical sensitivity to MET inhibitors. Cancer Discov. 2015;5:850–859.
- Paik PK, Drilon A, Fan PD, et al. Response to MET inhibitors in patients with stage IV lung adenocarcinomas harboring MET mutations causing exon 14 skipping. Cancer Discov. 2015;5:842–849.
- Tong JH, Yeung SF, Chan AW, et al. MET amplification and exon 14 splice site mutation define unique molecular subgroups of non–small cell lung carcinoma with poor prognosis. Clin Cancer Res. 2016;22:3048–3056.
- Ludovini V, Bianconi F, Pistola L, et al. Optimization of patient selection for EGFR-TKIs in advanced non-small cell lung cancer by combined analysis of KRAS, PIK3CA, MET, and non-sensitizing EGFR R mutations. Cancer Chemother Pharmacol. 2012;69:1289–1299.
- Peters S, Adjei AA. MET: a promising anticancer therapeutic target. Nat Rev Clin Oncol. 2012;9:314–326.
- Bahcall M, Sim T, Paweletz CP, et al. Acquired METD1228V mutation and resistance to MET inhibition in lung cancer. Cancer Discov. 2016;6:1334–1341.
- Krishnaswamy S, Kanteti R, Duke-Cohan JS, et al. Ethnic differences and functional analysis of MET mutations in lung cancer. Clin Cancer Res. 2009;15:5714–5723.
- Kong-Beltran M, Stamos J, Wickramasinghe D. The Sema domain of Met is necessary for receptor dimerization and activation. Cancer Cell. 2004;6:75–84.
- Camidge DR, Davies KD. MET copy number as a secondary driver of epidermal growth factor receptor tyrosine kinase inhibitor resistance in EGFR-mutant non-small-cell lung cancer. J Clin Oncol. 2019;37:855–857.
- Koeppen H, Yu W, Zha J, et al. Biomarker analyses from a placebo-controlled phase II study evaluating erlotinib ± onartuzumab in advanced non–small cell lung cancer: MET expression levels are predictive of patient benefit. Clin Lung Cancer. 2014;20:4488–4498.
- Choueiri TK, Plimack E, Arkenau H-T, et al. Biomarker-based phase II trial of savolitinib in patients with advanced papillary renal cell cancer. J Clin Oncol. 2017;35:2993–3001.
- Sholl L. Molecular diagnostics of lung cancer in the clinic. Transl Lung Cancer Res. 2017;6:560–569.
- Kim K-H, Kim H. Progress of antibody-based inhibitors of the HGF–cMET axis in cancer therapy. Exp Mol Med. 2017;49:e307–e315.
- Roskoski R. Classification of small molecule protein kinase inhibitors based upon the structures of their drug-enzyme complexes. Pharmacol Res. 2016;103:26–48.
- Mo H-N, Liu P. Targeting MET in cancer therapy. Chronic Dis Transl Med. 2017;3:148–153.
- Angevin E, Spitaleri G, Rodon J, et al. A first-in-human phase I study of SAR125844, a selective MET tyrosine kinase inhibitor, in patients with advanced solid tumours with MET amplification. Eur J Cancer. 2017;87:131–139.
- Drilon AE, Camidge DR, Ou S-H, et al. Efficacy and safety of crizotinib in patients (pts) with advanced MET exon 14-altered non-small cell lung cancer (NSCLC) [abstract]. J Clin Oncol. 2016;34:108.
- Vassal G, Ledeley M-C, Tournigand C, et al. Activity of crizotinib in relapsed MET amplified malignancies: results of the French AcSé Program. J Clin Oncol. 2015;33:2595–2595.
- Spigel DR, Ervin TJ, Ramlau RA, et al. Randomized phase II trial of onartuzumab in combination with erlotinib in patients with advanced non-small-cell lung cancer. J Clin Oncol. 2013;31:4105–4114.
- Rayson D, Lupichuk S, Potvin K, et al. Canadian Cancer Trials Group IND197: a phase II study of foretinib in patients with estrogen receptor, progesterone receptor, and human epidermal growth factor receptor 2-negative recurrent or metastatic breast cancer. Breast Cancer Res Treat. 2016;157:109–116.
- Rimassa L, Bozzarelli S, Pietrantonio F, et al. Phase II study of tivantinib and cetuximab in patients with KRAS wild-type metastatic colorectal cancer with acquired resistance to EGFR inhibitors and emergence of MET overexpression: lesson learned for future trials with EGFR/MET dual inhibition. Clin Colorectal Cancer. 2019;18:125–132.
- Bendell JC, Hochster H, Hart LL, et al. A Phase II randomized trial (GO27827) of first-line FOLFOX plus bevacizumab with or without the met inhibitor Onartuzumab in patients with metastatic colorectal cancer. Oncologist. 2017;22:264–271.
- Shah MA, Bang Y-J, Lordick F, et al. Effect of fluorouracil, leucovorin, and oxaliplatin with or without onartuzumab in HER2-negative, MET-positive gastroesophageal adenocarcinoma: the METGastric randomized clinical trial. JAMA Oncol. 2017;3:620–627.
- Sakai D, Chung HC, Oh D-Y, et al. A non-randomized, open-label, single-arm, phase 2 study of emibetuzumab in Asian patients with MET diagnostic positive, advanced gastric cancer. Cancer Chemother Pharmacol. 2017;80:1197–1207.
- Shah MA, Cho J-Y, Tan IB, et al. A randomized phase II study of FOLFOX with or without the MET inhibitor onartuzumab in advanced adenocarcinoma of the stomach and gastroesophageal junction. Oncologist. 2016;21:1085–1090.
- Kwak EL, LoRusso P, Hamid O, et al. Clinical activity of AMG 337, an oral MET kinase inhibitor, in adult patients (pts) with MET-amplified gastroesophageal junction (GEJ), gastric (G), or esophageal (E) cancer [abstract]. J Clin Oncol. 2015;33. DOI:10.1200/jco.2015.33.3_suppl.1
- Goyal L, Zheng H, Yurgelun MB, et al. A phase 2 and biomarker study of cabozantinib in patients with advanced cholangiocarcinoma. Cancer. 2017;123:1979–1988.
- Choueiri TK, Vaishampayan U, Rosenberg JE, et al. Phase II and biomarker study of the dual MET/VEGFR2 inhibitor foretinib in patients with papillary renal cell carcinoma. J Clin Oncol. 2013;31:181–186.
- Ryan CJ, Rosenthal M, Ng S, et al. Targeted MET inhibition in castration-resistant prostate cancer: a randomized phase II study and biomarker analysis with rilotumumab plus mitoxantrone and prednisone. Clin Cancer Res. 2013;19:215–224.
- Schöffski P, Wozniak A, Stacchiotti S, et al. Activity and safety of crizotinib in patients with advanced clear-cell sarcoma with MET alterations: European Organization for Research and Treatment of Cancer phase II trial 90101 ‘CREATE”. Ann Oncol. 2017;28:3000–3008.
- Nishio M, Horiike A, Nokihara H, et al. Phase I study of the anti-MET antibody onartuzumab in patients with solid tumors and MET-positive lung cancer. Invest New Drugs. 2015;33:632–640.
- Shitara K, Kim TM, Yokota T, et al. Phase I dose-escalation study of the c-Met tyrosine kinase inhibitor SAR125844 in Asian patients with advanced solid tumors, including patients with MET-amplified gastric cancer. Oncotarget. 2017;8:79546–79555.
- Falchook GS, Kurzrock R, Amin HM, et al. Efficacy, safety, biomarkers, and phase II dose modeling in a phase I trial of the oral selective c-Met inhibitor tepotinib (MSC2156119J) [abstract]. J Clin Oncol. 2015;33:2591.
- Tanizaki J, Okamoto I, Sakai K, et al. Differential roles of trans-phosphorylated EGFR, HER2, HER3, and RET as heterodimerisation partners of MET in lung cancer with MET amplification. Br J Cancer. 2011;105:807–813.
- Conrotto P, Corso S, Gamberini S, et al. Interplay between scatter factor receptors and B plexins controls invasive growth. Oncogene. 2004;23:5131–5137.
- Yoshioka T, Otero J, Chen Y, et al. β4 Integrin signaling induces expansion of prostate tumor progenitors. J Clin Invest. 2013;123:682–699.
- Jo M, Stolz DB, Esplen JE, et al. Cross-talk between epidermal growth factor receptor and c-Met signal pathways in transformed cells. J Biol Chem. 2000;275:8806–8811.
- van Veggel B, de Langen AJ, Hashemi S, et al. Crizotinib treatment for patients with EGFR mutation positive NSCLC that acquire cMET amplification after EGFR TKI therapy results in short-lived and heterogeneous responses. Lung Cancer. 2018;124:130–134.
- Galli G, Corrao G, Imbimbo M, et al. Uncommon mutations in epidermal growth factor receptor and response to first and second generation tyrosine kinase inhibitors: a case series and literature review. Lung Cancer. 2018;115:135–142.
- Wang Z-F, Ren S-X, Li W, et al. Frequency of the acquired resistant mutation T790 M in non-small cell lung cancer patients with active exon 19Del and exon 21 L858R: a systematic review and meta-analysis . BMC Cancer. 2018;18:148–154.
- Westover D, Zugazagoitia J, Cho B, et al. Mechanisms of acquired resistance to first-and second-generation EGFR tyrosine kinase inhibitors. Ann Oncol. 2018;29:i10–i19.
- Leonetti A, Assaraf YG, Veltsista PD, et al. MicroRNAs as a drug resistance mechanism to targeted therapies in EGFR-mutated NSCLC: current implications and future directions. Drug Resist Update. 2019;42:1–11.
- Yang Z, Tam KY. Combination strategies using EGFR-TKi in NSCLC therapy: learning from the gap between pre-clinical results and clinical outcomes. Int J Biol Sci. 2018;14:204–216.
- Xu J, Wang J, Zhang S. Mechanisms of resistance to irreversible epidermal growth factor receptor tyrosine kinase inhibitors and therapeutic strategies in non-small cell lung cancer. Oncotarget. 2017;8:90557–90578.
- Drilon A, Cappuzzo F, Ou S-H, et al. Targeting MET in lung cancer: will expectations finally be MET? J Thorac Oncol. 2017;12:15–26.
- Troiani T, Martinelli E, Napolitano S, et al. Increased TGF-α as a mechanism of acquired resistance to the anti-EGFR inhibitor cetuximab through EGFR–MET interaction and activation of MET signaling in colon cancer cells. Clin Cancer Res. 2013;19:6751–6765.
- Lan S-H, Wu S-Y, Raghavaraju G, et al. The crosstalk of c-MET with related receptor tyrosine kinases in urothelial bladder cancer. In: Persad R, Ranasinghe w, editors. Advances in the scientific evaluation of bladder cancer and molecular basis for diagnosis and treatment. Taiwan: IntechOpen; 2013. p. 21–39.
- Baldacci S, Mazieres J, Tomasini P, et al. Outcome of EGFR-mutated NSCLC patients with MET-driven resistance to EGFR tyrosine kinase inhibitors. Oncotarget. 2017;8:105103–105114.
- Camidge DR, Moran T, Demedts I, et al. A randomized, open-label, phase 2 study of emibetuzumab plus erlotinib (LY + E) and emibetuzumab monotherapy (LY) in patients with acquired resistance to erlotinib and MET diagnostic positive (MET Dx+) metastatic NSCLC [abstract]. J Clin Oncol. 2016;34:9070.
- Wu Y-L, Kim D-W, Felip E, et al. Phase (Ph) II safety and efficacy results of a single-arm ph ib/II study of capmatinib (INC280)+ gefitinib in patients (pts) with EGFR-mutated (mut), cMET-positive (cMET+) non-small cell lung cancer (NSCLC) [abstract]. J Clin Oncol. 2016;34:9020.
- Xu Y, Fan Y. Responses to crizotinib can occur in c-MET overexpressing nonsmall cell lung cancer after developing EGFR-TKI resistance. Cancer Biol Ther. 2019;20:145–149.
- Lv H, Shan B, Tian Z, et al. Soluble c-Met is a reliable and sensitive marker to detect c-Met expression level in lung cancer. Biomed Res Int. 2015;2015:626578–662686.
- Yang JJ, Yang JH, Kim J, et al. Soluble c-Met protein as a susceptible biomarker for gastric cancer risk: a nested case-control study within the Korean Multicenter Cancer Cohort. Int J Cancer. 2013;132:2148–2156.
- Kaye DR, Pinto PA, Cecchi F, et al. Tumor and plasma met levels in non-metastatic prostate cancer. PLoS One. 2016;11:e0157130–157138.
- McNeil BK, Sorbellini M, Grubb RL, et al. Preliminary evaluation of urinary soluble Met as a biomarker for urothelial carcinoma of the bladder. J Transl Med. 2014;12:199–204.
- Barisione G, Fabbi M, Gino A, et al. Potential role of soluble c-Met as a new candidate biomarker of metastatic uveal melanoma. JAMA Ophthalmol. 2015;133:1013–1021.
- Wader KF, Fagerli UM, Holt RU, et al. Soluble c-Met in serum of patients with multiple myeloma: correlation with clinical parameters. Eur J Haematol. 2011;87:394–399.
- Gao H-F, Yang J-J, Chen Z-H, et al. Plasma dynamic monitoring of soluble c-Met level for EGFR-TKI treatment in advanced non-small cell lung cancer. Oncotarget. 2016;7:39535–39543.
- Huang X, Wang C, Sun J, et al. Clinical value of CagA, c-Met, PI3K and Beclin-1 expressed in gastric cancer and their association with prognosis. Oncol Lett. 2018;15:947–955.
- Churin Y, Al-Ghoul L, Kepp O, et al. Helicobacter pylori CagA protein targets the c-Met receptor and enhances the motogenic response. J Cell Biol. 2003;161:249–255.
- Xie C, Yang Z, Hu Y, et al. Expression of c-Met and hepatocyte growth factor in various gastric pathologies and its association with Helicobacter pylori infection. Oncol Lett. 2017;14:6151–6155.
- Weng J, Mohan RR, Li Q, et al. IL-1 upregulates keratinocyte growth factor and hepatocyte growth factor mRNA and protein production by cultured stromal fibroblast cells: interleukin-1 beta expression in the cornea. Cornea. 1997;16:465–471.
- Wheeler DL, Yarden Y, editors. The MET receptor family. In: Receptor tyrosine kinases: family and subfamilies. Switzerland: Springer; 2015. p. 321–358.
- Garnett J, Chumbalkar V, Vaillant B, et al. Regulation of HGF expression by ΔEGFR-mediated c-Met activation in glioblastoma cells. Neoplasia. 2013;15:73–84.
- Tsuji T, Sakamori Y, Ozasa H, et al. Clinical impact of high serum hepatocyte growth factor in advanced non-small cell lung cancer. Oncotarget. 2017;8:71805–71816.
- Naumnik W, Naumnik B, Niklińska W, et al. Clinical implications of hepatocyte growth factor, interleukin-20, and interleukin-22 in serum and bronchoalveolar fluid of patients with non-small cell lung cancer. Adv Exp Med Biol. 2016;952:41–49.
- Umeguchi H, Sueoka-Aragane N, Kobayashi N, et al. Usefulness of plasma HGF level for monitoring acquired resistance to EGFR tyrosine kinase inhibitors in non-small cell lung cancer. Oncol Rep. 2015;33:391–396.
- Kim H, Youk J, Yang Y, et al. Prognostic implication of serum hepatocyte growth factor in stage II/III breast cancer patients who received neoadjuvant chemotherapy. J Cancer Res Clin Oncol. 2016;142:707–714.
- Bai L, Wang F, Zhang D-S, et al. A plasma cytokine and angiogenic factor (CAF) analysis for selection of bevacizumab therapy in patients with metastatic colorectal cancer. Sci Rep. 2015;5:17717–17729.
- Takahashi N, Furuta K, Taniguchi H, et al. Serum level of hepatocyte growth factor is a novel marker of predicting the outcome and resistance to the treatment with trastuzumab in HER2-positive patients with metastatic gastric cancer. Oncotarget. 2016;7:4925–4938.
- Noguchi E, Saito N, Kobayashi M, et al. Clinical significance of hepatocyte growth factor/c-Met expression in the assessment of gastric cancer progression. Mol Med Rep. 2015;11:3423–3431.
- Park DJ, Yoon C, Thomas N, et al. Prognostic significance of targetable angiogenic and growth factors in patients undergoing resection for gastric and gastroesophageal junction cancers. Ann Surg Oncol. 2014;21:1130–1137.
- Dreikhausen L, Blank S, Sisic L, et al. Association of angiogenic factors with prognosis in esophageal cancer. BMC Cancer. 2015;15:121–131.
- Unić A, Derek L, Duvnjak M, et al. Diagnostic specificity and sensitivity of PIVKAII, GP3, CSTB, SCCA1 and HGF for the diagnosis of hepatocellular carcinoma in patients with alcoholic liver cirrhosis. Ann Clin Biochem. 2018;55:355–362.
- Karabulut S, Tas F, Akyüz F, et al. Clinical significance of serum hepatocyte growth factor (HGF) levels in hepatocellular carcinoma. Tumor Biol. 2014;35:2327–2333.
- Zhang Y, Wu JZ, Yang YQ, et al. Expression of growth-regulated oncogene-1, hepatocyte growth factor, platelet-derived growth factor-AA and soluble E-selectin and their association with high-risk human papillomavirus infection in squamous cell carcinoma of the uterine cervix. Mol Med Rep. 2014;10:1013–1024.
- Mo Z-y, Wang P, Li X, et al. The clinical value of serum hepatocyte growth factor levels in patients undergoing primary radiotherapy for glioma: effect on progression-free survival. Med Oncol. 2014;31:122–127.
- Horala A, Swiatly A, Matysiak J, et al. Diagnostic value of serum angiogenesis markers in ovarian cancer using multiplex immunoassay. Int J Mol Sci. 2017;18:123–137.
- Dayyani F, Zurita AJ, Nogueras-González GM, et al. The combination of serum insulin, osteopontin, and hepatocyte growth factor predicts time to castration-resistant progression in androgen dependent metastatic prostate cancer-an exploratory study. BMC Cancer. 2016;16:721–730.
- Hügel R, Muendlein A, Volbeding L, et al. Serum levels of hepatocyte growth factor as a potential tumor marker in patients with malignant melanoma. Melanoma Res. 2016;26:354–360.
- Kubo Y, Fukushima S, Inamori Y, et al. Serum concentrations of HGF are correlated with response to anti-PD-1 antibody therapy in patients with metastatic melanoma. J Dermatol Sci. 2019;93:33–40.
- Saltarella I, Morabito F, Giuliani N, et al. Prognostic or predictive value of circulating cytokines and angiogenic factors for initial treatment of multiple myeloma in the GIMEMA MM0305 randomized controlled trial. J Hematol Oncol. 2019;12:4–13.
- Fang M, Wang S, Zheng Y, et al. Prognostic and predictive significance of plasma hepatocyte growth factor and carcinoembryonic antigen in non-small lung cancer after surgery. Eur Rev Med Pharmacol Sci. 2014;18:398–403.
- Ma R, Xu H, Wu J, et al. Identification of serum proteins and multivariate models for diagnosis and therapeutic monitoring of lung cancer. Oncotarget. 2017;8:18901–18913.
- Hosoda H, Izumi H, Tukada Y, et al. Plasma hepatocyte growth factor elevation may be associated with early metastatic disease in primary lung cancer patients. Ann Thorac Cardiovasc Surg. 2012;18:1–7.
- Tanaka H, Kimura T, Kudoh S, et al. Reaction of plasma hepatocyte growth factor levels in non‐small cell lung cancer patients treated with EGFR‐TKIs. Int J Cancer. 2011;129:1410–1416.
- Toi M, Taniguchi T, Ueno T, et al. Significance of circulating hepatocyte growth factor level as a prognostic indicator in primary breast cancer. Clin Cancer Res. 1998;4:659–664.
- Ahmed H, Metwally F, Mahdy E, et al. Clinical value of serum hepatocyte growth factor, B-cell lymphoma-2 and nitric oxide in primary breast cancer patients. Eur Rev Med Pharmacol Sci. 2012;16:958–965.
- Sheen-Chen S-M, Liu Y-W, Eng H-L, et al. Serum levels of hepatocyte growth factor in patients with breast cancer. Cancer Epidemiol Biomarkers Prev. 2005;14:715–717.
- Taniguchi T, Toi M, Inada K, et al. Serum concentrations of hepatocyte growth factor in breast cancer patients. Clin Cancer Res. 1995;1:1031–1034.
- El-Attar HA, Sheta MI. Hepatocyte growth factor profile with breast cancer. Indian J Pathol Microbiol. 2011;54:509–513.
- Toiyama Y, Miki C, Inoue Y, et al. Serum hepatocyte growth factor as a prognostic marker for stage II or III colorectal cancer patients. Int J Cancer. 2009;125:1657–1662.
- Ren Y, Cao B, Law S, et al. Hepatocyte growth factor promotes cancer cell migration and angiogenic factors expression: a prognostic marker of human esophageal squamous cell carcinomas. Clin Cancer Res. 2005;11:6190–6197.
- Kilic-Baygutalp N, Ozturk N, Orsal-Ibisoglu E, et al. Evaluation of serum HGF and CK18 levels in patients with esophageal cancer. Genet Mol Res. 2016;15. DOI:10.4238/gmr.15038583
- Tanaka K, Miki C, Wakuda R, et al. Circulating level of hepatocyte growth factor as a useful tumor marker in patients with early-stage gastric carcinoma . Scand J Gastroenterol. 2004;39:754–760.
- Wu C, Chi C, Su T, et al. Serum hepatocyte growth factor level associate with gastric cancer progression. Anticancer Res. 1998;18:3657–3659.
- Taniguchi T, Kitamura M, Arai K, et al. Increase in the circulating level of hepatocyte growth factor in gastric cancer patients. Br J Cancer. 1997;75:673–677.
- Le Q-T, Fisher R, Oliner KS, et al. Prognostic and predictive significance of plasma HGF and IL-8 in a phase III trial of chemoradiation with or without tirapazamine in locoregionally advanced head and neck cancer. Clin Cancer Res. 2012;18:1798–1807.
- Alkozai EM, Porte RJ, Adelmeijer J, et al. Levels of angiogenic proteins in plasma and platelets are not different between patients with hepatitis B/C-related cirrhosis and patients with cirrhosis and hepatocellular carcinoma. Platelets. 2015;26:577–582.
- Yamagamim H, Moriyama M, Matsumura H, et al. Serum concentrations of human hepatocyte growth factor is a useful indicator for predicting the occurrence of hepatocellular carcinomas in C-viral chronic liver diseases. Cancer. 2002;95:824–834.
- Hong TS, Grassberger C, Yeap BY, et al. Pretreatment plasma HGF as potential biomarker for susceptibility to radiation-induced liver dysfunction after radiotherapy. NPJ Precis Oncol. 2018;2:22–24.
- Barakat O, Rodriguez GC, Raijman I, et al. Clinical value of plasma hepatocyte growth factor measurement for the diagnosis of periampullary cancer and prognosis after pancreaticoduodenectomy. J Surg Oncol. 2010;102:816–820.
- Justinger C, Schlüter C, Oliviera-Frick V, et al. Increased growth factor expression after hepatic and pancreatic resection. Oncol Rep. 2008;20:1527–1531.
- Walker F, Kermorgant S, Daraï E, et al. Hepatocyte growth factor and c-Met in cervical intraepithelial neoplasia: overexpression of proteins associated with oncogenic human papillomavirus and human immunodeficiency virus. Clin Cancer Res. 2003;9:273–284.
- Zurita A, Gagnon R, Liu Y, et al. Integrating cytokines and angiogenic factors and tumour bulk with selected clinical criteria improves determination of prognosis in advanced renal cell carcinoma. Br J Cancer. 2017;117:478–484.
- Koufos N, Syrios J, Michailidou D, et al. Distinct patterns of angiogenic factor expression as a predictive factor of response to chemotherapy in stage IIIA non-small-cell lung cancer patients. Mol Clin Oncol. 2016;5:440–446.
- Tran HT, Liu Y, Zurita AJ, et al. Prognostic or predictive plasma cytokines and angiogenic factors for patients treated with pazopanib for metastatic renal-cell cancer: a retrospective analysis of phase 2 and phase 3 trials. Lancet Oncol. 2012;13:827–837.
- Borgia JA, Pithadia R, Ibrahem Z, et al. Potential predictive value of hepatocyte growth factor (HGF) in advanced non-small cell lung cancer (NSCLC) treated with a platinum doublet and bevacizumab [abstract]. J Clin Oncol. 2014;32(Suppl15). DOI:10.1200/jco.2014.32.15_suppl.e22000
- Minarik J, Pika T, Bacovsky J, et al. Prognostic value of hepatocyte growth factor, syndecan-1, and osteopontin in multiple myeloma and monoclonal gammopathy of undetermined significance. Sci World J. 2012;2012:1–356133.
- Bharti A, Ma PC, Maulik G, et al. Haptoglobin α-subunit and hepatocyte growth factor can potentially serve as serum tumor biomarkers in small cell lung cancer. Anticancer Res. 2004;24:1031–1038.
- Siegfried JM, Weissfeld LA, Luketich JD, et al. The clinical significance of hepatocyte growth factor for non-small cell lung cancer. Ann Thorac Surg. 1998;66:1915–1918.
- Kwon MJ, Kim DH, Park H-R, et al. Frequent hepatocyte growth factor overexpression and low frequency of c-Met gene amplification in human papillomavirus-negative tonsillar squamous cell carcinoma and their prognostic significances. Hum Pathol. 2014;45:1327–1338.
- Felix A, Edwards R, Stone R, et al. Associations between hepatocyte growth factor, c-Met, and basic fibroblast growth factor and survival in endometrial cancer patients. Br J Cancer. 2012;106:2004–2009.
- Raghav K, Morris V, Tang C, et al. MET amplification in metastatic colorectal cancer: an acquired response to EGFR inhibition, not a de novo phenomenon. Oncotarget 2016;7:54627–54631.
- Kim ST, Banks KC, Lee S-H, et al. Prospective feasibility study for using cell-free circulating tumor DNA–guided therapy in refractory metastatic solid cancers: an interim analysis. JCO Precis Oncol. 2017;1:1–15.
- Rozenblum AB, Ilouze M, Dudnik E, et al. Clinical impact of hybrid capture–based next-generation sequencing on changes in treatment decisions in lung cancer. J Thorac Oncol. 2017;12:258–268.
- Aggarwal C, Thompson JC, Black TA, et al. Clinical implications of plasma-based genotyping with the delivery of personalized therapy in metastatic non–small cell lung cancer. JAMA Oncol. 2019;5:173–180.
- Li B, Janku F, Jung B, et al. Ultra-deep next-generation sequencing of plasma cell-free DNA in patients with advanced lung cancers: results from the Actionable Genome Consortium. Ann Oncol. 2019;30:597–603.
- Li D, Li F, Wu Y, et al. Quantification of serum MET in non-small-cell lung cancer and its clinical significance. Clin Biochem. 2015;48:110–114.
- Oellerich M, Schutz E, Beck J, et al. Using circulating cell-free DNA to monitor personalized cancer therapy. Crit Rev Clin Lab Sci. 2017;54:205–218.
- Jia J, Morse MA, Nagy RJ, et al. Cell-free DNA profiling to discover mechanisms of exceptional response to cabozantinib plus panitumumab in a patient with treatment refractory metastatic colorectal cancer. Front Oncol. 2018;8:305–311.
- Han X, Wang J, Sun Y. Circulating tumor DNA as biomarkers for cancer detection. Genom Proteom Bioinf. 2017;15:59–72.
- Shin JH, Chung J, Kim HO, et al. Detection of cancer cells in peripheral blood of stomach cancer patients using RT–PCR amplification of tumour‐specific mRNAs. Aliment Pharmacol Ther. 2002;16:137–144.
- Wang J-Y, Hsieh J-S, Chen C-C, et al. Alterations of APC, c-met, and p53 genes in tumor tissue and serum of patients with gastric cancers. J Surg Res. 2004;120:242–248.