Abstract
Meat and fish chemical composition and sensory attributes are markers of quality that require innovative assessment methods as existing ones are rather technical, laborious, and expensive. Emerging trends of advanced technology instruments have been lauded in the pharmaceutical, cosmetic and food industries for their high sensitivity, customizability, rapidness and affordability. Common among these, are the electronic tongue (e-tongue) and electronic nose (e-nose) but their use for meat and fish quality, remains scanty and scattered. This paper aims to systematically discuss the developing trends, principles and the recent use of e-tongue and e-nose for quality measurements in fish and meat. From over 90 research papers, it was observed that an arsenal of chemometric tools have been pivotal in applying these instruments for rapid quantitative, qualitative and predictive analysis of some physical properties, chemical properties, storability and the authentication of meat and fish. Both instruments require no reagent (waste free analytical procedure) and have been lauded for precision and*accuracy but e-nose may be better suited for meat and fish assessments. Unlike the e-tongue, e-nose requires no liquid sample preparation and portable versions are promising for rapid remote analysis of meat and fish samples that can save cost on transferring carcass to laboratories.
Introduction
The meat and fish industry in recent times have been regarded as one of the largest sections of the food industry with a soaring demand. Meat and fish are considered as staples in certain countries but they are also essential for human survival and consumed for both pleasure and nutrition; a good source of protein, vitamins and minerals (Henchion et al. Citation2014).
Fish, the inclusive term for aquatic animals. With the continuous development of living standards and the relative change of dietary structure, there is a rising and persistent increase in demand for safe and good quality meat and fish. The value chain for meat and fish is highly variable, difficult to control and requires constant measures to guarantee quality control. Quality assurance and control are major tasks in production and processing of meat and meat products and is often related to sensory analysis. Important meat quality parameters are not limited but the most important ones to the consumer include compositional quality (lean to fat ratio, protein, pH) and the palatability factors such as visual appearance, smell, firmness, juiciness, tenderness, and flavor (Haddi et al. Citation2015).
Chemical procedures and instrumental methods used for monitoring such parameters include the Kjeldahl method (for crude protein), Soxhlet Extraction Method (for crude fat), pH meter (acidity/alkalinity), colorimeter (for color) (Pugliese and Sirtori Citation2012). Some of these methods are however, tedious, destructive, time consuming and in some cases expensive and often do not give a complete overview of meat quality. Another trend in the food industry for quality assurance is the adoption of sensory evaluations using trained panelist. Sensory analysis is a well-established method for determining the sensorial qualities of food based on the receptors in human organs (consumer perception), but it can also be time consuming and subjective or biased (dependent on the panelist results). Details about recent designs and implementation of sensory evaluations have been discussed by Yu, Low, and Zhou (Citation2018).
Assessing food quality is complex because it translates into assessing all the molecular composition of the food based on biological receptors. Receptors in engineering can be said to be equivalent with sensors except that sensors are man-made and can be modified to suit whatever purpose they are desired for. Gaining grounds in the field of sensors are the acoustic, chemical, electronic, optical mechanical and thermal sensors etc (Stroble, Stone, and Watkins Citation2009) but this review focuses on the electronic sensors, specifically the tongue (e-tongue) and the electronic nose (e-nose). Thus, for the context of this review electronic sensor devices refers to the e-tongue and e-nose. Currently, these devices have gained fame in the pharmaceutical, cosmetics, environmental control, engineering (petroleum), agriculture and beverage industries but their applications for meat processing and quality management is not clearly defined. The amount of meat the world eats is expected to rise by over 55 per cent in the next 20 years (Woodvine Citation2009), thus, stringent methods are required for quality control. It is against this backdrop that this review aims to assess and discuss the recent applications of electronic sensor devices in the meat and fish industry, their future prospects and their pros and cons.
Meat classification
Animal food sources can be classified under three main groups: as meat, poultry, or fish (McWilliams Citation2012). For meat scientist, this grouping depends on a number of factors such as the concentration of myoglobin in the flesh, the lipid profile of the carcass and the general physiology of the animal flesh during after slaughtering (Keeton and Dikeman Citation2017). Red meat in the broad sense, may refer to red fleshed carcass, among which the most patronized are pork, lamb/mutton, beef, and veal. Red meat has been credited with good levels of biological protein, minerals (iron, iron, thiamin, riboflavin, zinc) and vitamins (B6 and B12) (Wyness Citation2016). They however (allegedly), contribute to heart diseases, a controversial subject under discussion and debate in the food industry. Poultry meat is the general term used to represent chicken, geese, turkey, duck and other fowls and their products. Poultry is often recommended for athletes because it is ideal for maintaining good cholesterol levels due to their high contents of polyunsaturated fatty acids (Farrel Citation2008). They are however poor in fiber, complex carbohydrates, nor vitamin C (Woodvine Citation2009). Fish is the inclusive term used to refer to aquatic animals. They are abundant in iodine, vitamin D, calcium, poly-unsaturated and mono-unsaturated fatty acids like Omega 3 and Omega 6 fatty acids (Keeton and Dikeman Citation2017) and is sometimes classified under white meat.
The use of concise lexicons in meat and fish classification is essential for accuracy in scientific studies. The classification of meat and fish discussed above (red meat, poultry and fish) will be used in this study.
Electronic sensors
According to Thevenot et al. (Citation1999), “a biosensor is an independently integrated receptor transducer device, which is capable of providing selective quantitative or semi-quantitative analytical information using a biological recognition element”. Other types of sensors exist and can be constituted from diverse components to serve specific purposes. Essentially, sensors are meant to be incorporated into analytical instruments to provide rapid, real-time, accurate and reliable data about various samples understudy. Advanced research and technological developments over the past decade, have led to the development and optimization of biosensors. There have been improvements in the sensitivity, selectivity, and multiplexing capacity of modern biosensors (Perumal and Hashim Citation2014). Ideally, they now have the potential to respond continuously and reversibly without necessarily damaging the sample. Several types of biosensors exist depending on the basic components (Cesar Paixão and Subrayal Citation2017): the biological signaling method or the type of signal transduction. The major types can be seen in but the focus of this review is on the ones that employ the electrochemical mechanism of signal transduction because of their potentials for reliable evaluations in meat and fish.
Figure 1. Main types of biosensors. Concepts were adapted from Perumal and Hashim (Citation2014).
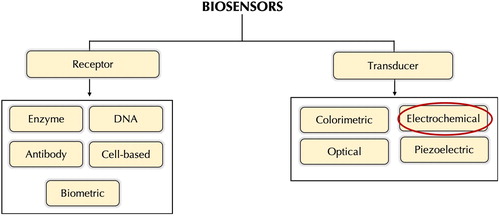
Electrochemical devices mainly monitor the current at a fixed or programed modulated voltage (amperometry/pulse voltammetry) or the voltage at zero current (potentiometry), or assess conductivity or impedance changes. Optical devices on the other hand, employ varying principles such as the effect of biological structures on light absorption, fluorescence, refractive index, or other optical parameters (Tothill and Turner Citation2015). Impedance is the total electrical resistance to the flow of an alternating current being passed through a given medium. Typically, impedance decreases while conductivity and capacitance increases during measurement (Lee, Kim, and Kim Citation2009). Voltammetric devices rely on the relationship between the current and the applied potential. There are often two main approaches, firstly to measure the current response as a function of applied potential and secondly, to monitor the potential response as a function of applied current (Cesar Paixão and Subrayal Citation2017). This review focuses on the electronic tongue (potentiometric and voltammetric) and the electronic nose because, their sensor specificity and selectivity give them superior advantages for research and technical purposes. shows the general advantages of the instruments that could be explored for red meat, poultry and fish quality control. Application of sensor-based instruments involves more than one variable thus multivariate analyses is required to interpret such results.
Common multivariate methods associated with sensor-based instruments
Multivariate analysis is simply a simultaneous observation and analysis of more than one outcome variable. This is contrary to univariate and bivariate analysis which involves just one and two outcomes respectively. The data captured from sensor-based instruments can be explored using different pattern recognition and multivariate statistical methods () of which, the principal component analysis (PCA) statistical method is the most common for data visualization purposes. PCA is favorable because it is a way of identifying patterns in data and it expresses the data in such a way that it highlights their differences and similarities. It is an outlier sensitive statistical method that reduces the amount of data to a smaller number of newly derived variables which represent the original data adequately (Shlens Citation2005). Detailed concepts of PCA application have been reported by Joliffe and Morgan (Citation1992).
Figure 3. Main multivariate data analysis methods involved in the application of electronic sensors; MLR (Multiple linear regression), PCR (Principal component regression), PLSR (Partial least squares regression), ANN (Artificial neural network), LDA (Linear discriminant analysis), PLS-DA (Partial Least Squares Discriminant Analysis), KNN (k-nearest neighbor’s algorithm), SVM (support vector machine), PCA (Principal component analysis), K-means (clustering). Concepts were adapted from Palit et al. (Citation2010), Granato et al. (Citation2018) and Medina et al. (Citation2019).
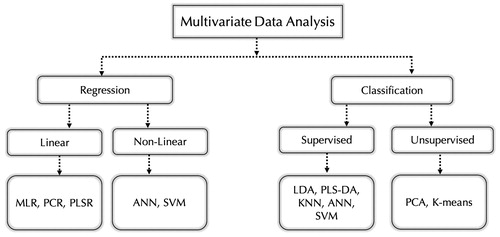
In classification, KNN can be used for speedy evaluations as it does not require training with the data, however, data standardization and normalization is a requirement. KNN is not recommend for large datasets or data sets with high dimensions. The Support vector machine (SVM) may be a better option when working with high dimensions but is very sensitive to outliers and noisy data and sometimes underperforms when large datasets are involved (Granato et al. Citation2018). Artificial neural networking (ANN) has also been acknowledged for the ability to deliver an output even when incomplete information was used during training. The reliability of its performance heavily depends on how important the missing values are (Medina et al. Citation2019).
For quantitative purposes, latent variables of PCA may be applied in principal component regression (PCR) models. PCR is optimized to describe the largest amount of variation of the sensor data, not taking into account the reference variable that we calibrate on. In contrast, partial least squares (PLS) regression generates the new latent variables by optimizing the model for describing the largest amount of variance of both the measured sensor data and reference values. These can ultimately be used to build strong models that can predict certain parameters based on the variables being investigated. According to Simões Da Costa, Delgadillo, and Rudnitskaya (Citation2014), there are two main optimization parameters when using the partial least squares (PLS) approach: Root Mean Square Error (RMSE) and the adjusted R2. The adjusted R2 is a R2 corrected for the number of explanatory terms in the model and thus, penalizes models with high number of parameters. While R2 usually increases when a new term is added to the model, the adjusted R2 increases only if the added term improves the model more than would be expected. This property is particularly useful when comparing performance of multivariate analysis. The data set largely influences modeling and prediction (Wang, Xu, and Yu Citation2017). Detailed explanations of these methods have been reported by Palit et al. (Citation2010). The purpose of statistical methods is to calibrate the signal responses of the electronic sensors and permit fitting of the multidimensional output of the sensor set (Wei et al. Citation2018). Adequate data processing is an essential step when using biosensor instruments for reliable results.
The electronic tongue
Food quality assurance is a domain dominated by conventional methods and chemical analysis to assure consumer safety. Some of these methods however, are slow and require relatively sophisticated processes, making their implementation at industrial level rather limited as they are not suitable for rapid monitoring. In addition to their cost issues, they also require highly skilled operators and time-consuming processes (Gil et al. Citation2011). With this gap, was the need to explore alternative but equally advanced rapid analytical instruments capable of performing similar tasks with better sensitivities. Several trials and studies led to the development of such instruments one of which, is commonly referred to as the electronic tongue (e-tongue). Earlier versions of this instrument were designed with biosensors that employed electrochemical transducers to function, hence the name “The electronic tongue”. Several models of chemical sensors (based on taste patterns) have also been used to design the electronic tongue’s sensor array: electrochemical (potentiometric, voltammetric, amperometric, impedimetric, conductimetric), optical, mass, and enzymatic biosensors (Valle, Mimendia, and Gutie Citation2010). Ideally, the e-tongue can be defined as an advanced analytical instrument made up of an array of sensors and pattern recognition technologies capable of performing quantitative and qualitative analysis (Winquist Citation2008). shows the general sequential application of the e-tongue. The e-tongue has been appraised and acknowledged in food industries for liquid food analysis but its applications for meat and fish quality remains scanty. A full discussion about the different architectures of e-tongue have been reported by Banerjee et al. (Citation2016) but the most frequently used are the Potentiometric and Voltammetric e-tongue.
The potentiometric e-tongue versus the voltammetric e-tongue
Potentiometry represents a direct application of the Nernst equation through the measurement of potentials of non-polarized electrodes lacking current flow (Magdalena and Wardencki, Citation2015). In voltammetry, a potential is applied on the working electrode, the measurement of the resulting current between the working electrode and the reference electrode is evaluated (Winquist Citation2008).
Arguably, potentiometric e-tongues are more famous but voltammetric e-tongues are more adaptable with more resistance to electrical imbalances and have better signal to noise ratio (Campos et al. Citation2012). The voltammetric e-tongues are however, limited to redox-active substances (Escuder-Gilabert and Peris Citation2010) and are sometimes associated with low detection limits. Detailed principles about the diverse types of e-tongue have been reported (Tuczek Citation2010). The potentiometric e-tongue has gained fame in the area of quality assurance. It is made up of an array of nonselective chemical sensors () with partial specificity to different constituents in solution. Signals from the sensors are transduced by a pattern recognition technology with a potential to recognize quantitative and qualitative compositions of simple and complex solutions (Ciosek and Wróblewski Citation2011).
Figure 5. Reference electrode and Partially selective sensors of the Alpha Astree potentiometric electronic tongue.
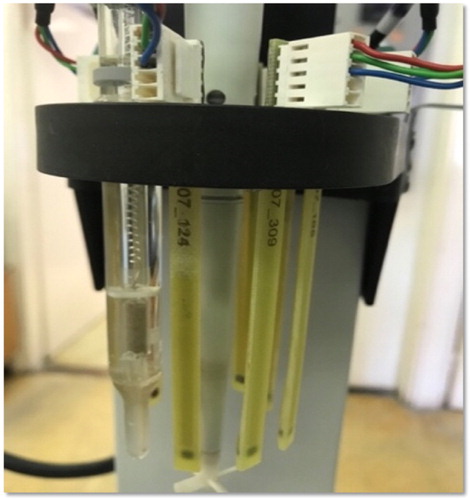
The type of materials required for the sensing units remains of utmost importance, particularly if a high performance is desired. This is because, although e-tongues do not require special interactions with the analyte, the sensing-unit is still required to respond electrically to small changes in the liquid under analysis. More so, depending on the method of detection, a level of electrical conductivity and/or electroactivity may be required (Tuczek Citation2010).
One of the first class of materials used for e-tongues were lipid membranes, in an attempt to mimic the materials of the human tongue (lipid bilayers provides the framework for a cell membrane). Chalcogenide glasses in electrochemical measurements have also been explored and presented main advantages such as the ease of electrode preparation and cross selectivity (Rudnitskaya et al. Citation2017). Also gaining grounds, are the ion sensitive field effect transistors (ISFET), novel integrated devices in the micro electrochemical lab. These are very similar to the metal oxide semiconductor field effect transistor (MOSFET) but their sensitive area represents a transistor gate and incorporates the means of transduction from an ion concentration to a voltage (Rita et al. Citation2017). Detailed reviews about e-tongue sensors have been published (Podrazka et al. Citation2017)
Challenges and progress associated with e-tongue application for red meat, poultry and fish quality control
Accurate signal transfer from e-tongue sensors is crucial for using the instrument but issues of signal drift have remained a challenge. Sensor drift refers to the changes in sensor sensitivity over time and may be as result to aging (for biosensors) or poisoning. Sensors, are also susceptible to diverse environment conditions such as oxidation and temperature fluctuations that weaken their sensitivity (Holmin et al. Citation2001). They can also be prone to adsorbing certain components after signal acquisition that can interfere with the surface interactions of sensors in subsequent analysis. Pre-conditioning and post-conditioning of sensors after analysis are among the recommend methods for boosting sensor strength but mathematical approaches in the form of chemometrics are also being explored (Rudnitskaya Citation2018), in combination with good calibration routines.
Also, samples in liquid can be freely analyzed using the e-tongue but those in solid form may require transformations that preferably should not affect the biochemical components of the sample. Before analyzing meat, poultry or fish for instance, certain protocols are needed to transform the samples into liquid state but without any major alteration or change in the chemical composition. This has so far been a challenge in the meat and fish industries but recently, several approaches have been explored.
Reported sample preparation methods for analysis of meat and fish with e-tongue
A major advantage of e-tongue analysis is that it is rapid and does not require reagents, therefore issues of waste management are limited. In this regard certain extraction techniques have been explored and have proved to be effective.
In the extraction and ultrafiltration of beef according to Zhang et al. (Citation2012), de-ionized water of temperature 100˚C was used to homogenize the meat. The homogenized meat was then filtered using a Whatman paper (54 membranes pore size), and centrifuged at 11,000 g for 20 min. The supernatant was then collected for e-tongue analysis. It is important to note that the temperature of 100˚C used in this extraction procedure has the potential to alter the state of certain meat components and as such needs to be optimized. Liu et al. (Citation2017) and Tian et al. (Citation2018) used the method to preprocess chicken for e-tongue analysis.
Gil et al. (Citation2007) also used the extraction and ultrafiltration method for studies on fish but with a slight modification. The fish fillet was gutted and preserved in sample containers at 4˚C for 7 days before analysis. Inosine 5′-monophophate (IMP), inosine (Ino), hypoxanthine (Hx) and potentiometric values (silver and gold electrodes) were determined in each sample every day with the e-tongue. Extraction and ultrafiltration was also used by Han et al. (Citation2015) to preprocess crucian fish and by García et al. (Citation2017) to preprocess cod fish for e-tongue analysis.
Generally, the extraction and ultrafiltration method are the most effective in terms of cost and reliable results. Other types of extraction procedures also exist but remain unexplored for e-tongue analyses. They have nonetheless proved useful with other analytical instruments.
Unexplored methods of meat and fish sample preparation for e-tongue analysis
Microwave-assisted extraction (MAE) and accelerated solvent extraction (ASE) used by Tan et al. (Citation2019) for polycyclic aromatic hydrocarbons evaluation in fish with GC-MS (gas chromatography- mass spectrometry) and by Ojemaye and Petrik (Citation2019) to determine conjugated or metabolic active compounds in fish with LC-MS (liquid chromatography).
Pressurized liquid extraction used by Chu and Metcalfe (Citation2007) to determine Pharmaceutically active compounds in three varieties of fish.
QuEChERS (Quick, Easy, Cheap, Effective, Rugged and Safe) method used by Ragnar, Christiansen, and Deribe (Citation2011) to determine organic pollutants in fish with GC-MS.
These extraction methods have proved effective in the respective studies and the nature of their extract (less dense liquid extract) implies that; they could be adopted for e-tongue analysis but it is important to consider the following factors first:
It is essential to factor in the cost and the technicalities associated with these extraction methods. For instance, ASE increases diffusion and solubility rate but involves a rather complex cleanup protocol.
The final volume of the extract is crucial in determining the required sample size and dilution factors that would be used. More studies need to be conducted to develop an ideal protocol through a cost-benefit analysis.
It is important to note that some food products such as wines have a reported dilution level of 50% (Soós et al. Citation2015) for the best qualitative and quantitative measurements with the e-tongue. There is however no standard dilution level in regards to meat and fish and as such, could be explored.
Applications of e-tongue red meat, poultry and fish quality
In detecting substances undetectable by their natural counterparts, the e-tongue has exhibited acceptable correlations with organoleptic results of human panelists and have showed better sensitivity than the human tongue (Campbell et al. Citation2012). According to Valle, Mimendia, and Gutie (Citation2010) six major categories exist for e-tongue meat analysis: process monitoring, freshness evaluation and shelf-life investigation, authenticity assessment, foodstuff recognition, quantitative analysis, and other quality control studies.
From , the e-tongue has successfully been used to investigate diverse quality parameters in red meat: pork, beef, goat and lamb. The potentiometric e-tongue was used to assess freshness (Feng et al. Citation2018), flavor and shelf-life of beef (Zhang et al. Citation2015). It has also been used to monitor storage of pork loin stored for over 10 days and to discriminate between goat, beef and lamb meat in the fight against food fraud. Different types of meat, irradiated at varying levels using an electron beam accelerator resulted in higher 2-thiobarbituric acid reactive substances (TBARS) than the non-irradiated counterpart regardless of animal species, but beef was the most susceptible to oxidation (Feng et al. Citation2017). Based on this, further studies was performed by Feng et al. (Citation2018) to determine the changes of taste profiles under different irradiation doses using the electronic tongue
Table 1. Some recent trends of e-tongue application for red meat quality.
The voltammetric e-tongue, based on carbon modified by bisphthalocyanines and polypyrrole doped with various dopants was used for the detection and quantification of putrescine and ammonia in beef (Apetrei and Apetrei Citation2016). Biogenic amines are formed in foods with rich protein contents because of the hydrolysis processes associated with amino-acids decarboxylation. The formation of biogenic amines is a drawback in the meat industry that is facilitated by the action of microorganisms in foods with a rich protein content.
E-tongue has also been used as a correlative technique with e-nose to classify goat, sheep and beef meat (Haddi et al. Citation2015)
Generally, the potentiometric e-tongue (with Ag/AgCl reference electrode) was the most frequently used. This may be attributed to its partially selective sensors, easy maintenance and less technical requirements.
Applications of e-tongue for poultry quality
From this review, there was a paucity of information with regards to the application of e-tongue for chicken, turkey or duck freshness. There were however studies of processed duck and chicken with the e-tongue as shown in .
Table 2. Some recent trends of e-tongue application in poultry.
Taste patterns from sugar-based chicken seasonings were investigated and predicted with the e-tongue (Tian et al. Citation2018). The e-tongue could discriminate fructose, glucose, sucrose and umami taste profiles in the chicken samples. Sugar is an important flavor enhancer that can compensate for saltiness and spicy taste. Studies with the e-tongue have also been reported for monitoring the effect of different cooking methods in meat. Changes in the taste profile of Dezhou-braised chicken during frying was assessed by Liu et al. (Citation2017). Frying showed little influence on umami taste but greatly influenced saltiness and bitterness when measured with the e-tongue.
Similar conclusions were made for duck meat cured with salt (2%, w/w), sodium nitrite (0.01%, w/w), sodium erythorbate and brine solution (Zhao Shuangjuan et al. Citation2018). According to their study, e-tongue confirmed that the different treatments led to the increase of free amino acids, oligopeptides and inosine monophosphate. The dry-cure processing technique have been reported to produce umami related taste compounds such as free amino acids and oligopeptides (Vidal et al. Citation2019). E-tongue has also been used a correlative technique with computer vision to characterize chicken meat qualities (Rita et al. Citation2017).
Applications of e-tongue for fish quality
Fish freshness is a relatively complex concept, which includes different microbiological, physicochemical and biochemical attributes. It is therefore of essence to carefully consider the robustness of any method or instruments that can be used to evaluate these parameters. shows the recent applications of the potentiometric and voltammetric e-tongue for quality assurance in fish.
Table 3. Some recent trends of e-tongue application for fish quality.
The potentiometric e-tongue could determine changes in volatile compounds and predict freshness of tilapia (Shi et al. Citation2018) stored at temperatures of 0 to 10 °C. It was used to validate sensory results of grass carp fish by discriminating grass carp fishes stored at on different says for 15 days (Pattarapon et al. Citation2018). The voltammetric e-tongue could also discriminate cod fish freshness and predict microbial spoilage of the fish (Ruiz-Rico et al. Citation2013). This is important for rapid prediction of tilapia spoilage. Total viable count (TVC) on fish was also determine and predicted with the potentiometric e-tongue (Han et al. Citation2015). TVC is a microbiological representation of the presence of microbes in a product. E-tongue can be used as a correlative technique for rapid monitoring or prediction of fish freshness and quality. Generally, the potentiometric e-tongue has been used to investigate fish quality more than the voltammetric e-tongue. E-tongue has been used a correlative technique with the electronic eye to characterize fish sauce (Nakano et al. Citation2018) and with the electronic nose to monitor tilapia freshness (Shi et al. Citation2018).
The future of e-tongue for red meat, poultry and fish analysis
Application of the e-tongue in the meat industry is a welcome advantage because numerous samples can be analyzed at a shorter period compared to other analytical methods that are expensive and time-consuming. In addition, sensors can be specifically developed for special sensitivities to certain meat components of interest especially in the application of the voltammetric e-tongue. Reagents are not required; therefore, issues of waste management are very limited and makes them cost effective. Experiments can also be conducted with small sample sizes for quantification and qualification purposes with low sophistication. The have also been recognized for precision and accuracy when used as a correlative technique with other analytical instruments. On the other hand, the sensor drift in the e-tongue is an existing concern because it can influence the experimental results (Wojnowski et al. Citation2017) but several interventions have been established to reduce its excessive effects on data. They include good experimental design and mathematical approaches that involve multivariate data analysis. Multivariate statistics applies optimal mathematical and statistical methods to process data. shows a quick overview of e-tongue application for meat and fish quality. More focus is required for longevity of the sensors and transforming the meat and fish products into liquid state for e-tongue analysis.
The electronic nose (E-nose)
Smell is an important parameter and indicator of freshness in the food industry. The freshness of food degrades due to environmental factors and spoilage from biochemical reactions during storage (Pennacchia, Ercolini, and Villani Citation2011). The main ingredients of food like protein, fat and carbohydrates will be decomposed by enzymes and bacteria, producing odor. The presence of these bacteria is the determining factor in shelf- life evaluation, since changes in aroma profile often precedes changes in product's appearance: discoloration, sliminess and softness (Papadopoulou et al. Citation2013). Spoilage/freshness is a sensory quality and is usually detected by examining for off-odors and discoloration (Gliszczy Citation2017). Metabolites such as trimethylamine, aldehydes, ketones and esters, as well as other low molecular weight compounds responsible for off-flavors and sensory product rejections are produced (Zhang et al. Citation2017).
Sensory analysis is often cumbersome and expensive, especially when it is performed by highly-trained specialists, who cannot work for extended periods of time due to olfactory fatigue. The meat and fish production and processing industry is in need of novel approaches for the classification and spoilage evaluation of its products (Wojnowski et al. Citation2017). They require an instrument that would provide a rapid result both at the processing plants and at retail level as the quality evaluation of meat products is necessary in order to ensure the consumer's safety. Consumption of spoiled meat or fish can lead to serious health hazards such as nausea, vomiting, and diarrhea. This and consumer perception of smell in several food products has resulted in a steady interest in the electronic nose (e-nose), an advanced technology with better sensitivity to the human nose for the determination of odor. A single, volatile molecular compound may commonly have referred to as an odorant (e.g., geosmin, pentadecalactone, ethyl acetate, or dimethysulfide), whereas volatile compounds perceived by an organism (usually a complex mixture of many odorants: e.g. caramel, apple, lavender, and chocolate) are defined as an odor. In some reports the device has been referred to as, the ‘gas sensor array’ or ‘multisensorial system’ instead but nonetheless, it can detect and recognize items on the basis of their odor signature with the help of electrochemical sensors and an appropriate pattern classification algorithm (Tarassenko and Denham Citation2006).
Development of the e-nose technique is however emphasized by the continuous emergence of novel types of sensors. In early applications, where classical chemical sensors were used, it was difficult to analyze complex matrices like food products (Wojnowski et al. Citation2017). These difficulties were often attributed to insufficient stability of measurement, the need for frequent calibration and high-power consumption. Another challenge was the miniaturization of electronic noses, with an integration with consumer electronics in mind (Rateni, Dario, and Cavallo Citation2017). Focus is also placed on the development of units intended for real-time monitoring of odors.
Principle of operation for the E-nose
The value of all sensing systems is to convert various types of sensory information from exposure (visual, auditory, mechanical, chemical) into information encoded by the biological system of neurons (circuits) in the brain or through electronic circuits of the biomimetic system (Chaudhary and Gupta Citation2009). In the brain, this transformation usually takes the form of a transduction (conversion) of sensory information into tones of neuronal impulses initiated in the biological circuits of a neural pathway (Persaud and Dodd Citation1982). In engineered devices, this takes place as interpretation of odorant signals into electrical charges existing within analytical computer circuits. For electronic odor detectors, the needed information upon exposure, is the vapor phase molecules emitted from odorous substances that have been volatilized to a degree dependent on their vapor pressures (Jiang, Chen, and Liu Citation2014). In essence, the odorant encoding task is to convert the chemical and physical information about these gas phase molecules (structure, charge, size, molecular weight, polarity, solubility, volatility, concentration, and so on) with the help of advanced technologies onto computer systems (Persaud and Dodd Citation1982).
The e-nose is a scientific tool equipped with an array of gas sensors and an artificial intelligence algorithm system capable of discriminating such volatiles. A comprehensive review on the history of the electronic nose was discussed by Loutfi et al. (Citation2015). E-nose sensors may also be developed from tin oxide films impregnated with different precious metals or from chemically modified metalloporphyrins. The degree to which polymer, tin oxide, or metalloporphyrin-based sensors interact with odorant compounds depend on their relative affinity for the vapor compound, which is also dependent on solvation, polarity, charge affinity, and other chemical and physical properties of the sensing materials (Tarassenko and Denham Citation2006). In most e-nose systems, the component materials are mainly of hydrocarbon polymers chosen from catalogs of commercially available polymeric materials or purposely synthesized for detecting specified vapor (Cesar Paixão and Subrayal Citation2017). shows the major sensor types in e-nose systems and their respective advantages. E-nose gas sensors consist of sensing layers for interacting with oxidizing or reducing gases. Changes in the sensing layers as a result of resistance leads to an increase or decrease in charge carrier concentration with the gas to be detected (Timsorn et al. Citation2016)
Figure 7. Gas sensors capable of sensing diverse types of gases, concepts were adapted from (Baldwin et al. Citation2011; Sanaeifar et al. Citation2017; Berna Citation2010).
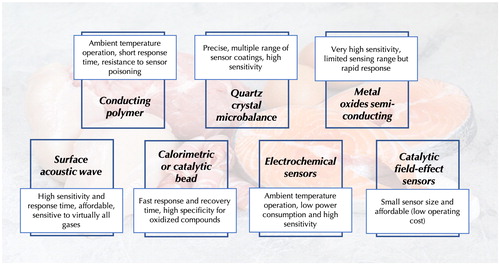
According to Smolander and Ahvenainen (Citation2006), the semiconductor of metal oxides is one of the most acknowledged e-nose sensors with a low cost, long life, high sensitivity, simple circuit requirement and has been used to assess meat and fish freshness. They absorb chemical molecules in gas phase and convert them into electrical signals. Sanaeifar et al. (Citation2017) gave detailed reports about the advantages and disadvantages of different types of detectors in the e-nose. It is also worth mentioning that, the exact mechanisms that impart the sensitivity to different odorant vapors are not certainly understood to the degree that one can clearly predict how a particular material will respond to a particular odorant vapor (Cesar Paixão and Subrayal Citation2017).
Challenges and progress associated with e-nose application for red meat, poultry and fish quality control
The e-nose has been pivotal in the mimicking of the human nose functions. This is an outcome that has been widely exploited by researchers in the food industries, often for preliminary quality control purposes. The prevalence of pathogenic microbes in food products is a significant problem for food preservation and storage (Gębicki and Szulczyński Citation2018). Microbiological methods have often been used to monitor these conditions, but recent applications of the e-nose to detect gaseous compounds emitted by specific microorganisms so as to detect their presence has been successful.
There have however been concerns about the sensitivity of e-nose gas sensors. Some sensors for instance, have varying sensitivities for different types of gases (Berna Citation2010). The sensitivity of the emitted gas has been reported to dependent on the type of gas sensor being used, it’s sensing range, recovery time and specificity. Some sensors are also reportedly more sensitive to oxidizing compounds (Wilson and Baietto Citation2011).
When investigating diverse forms of adulteration, accurate homogenization of the samples under study is crucial and is a major factor in the outcome of the experiment. In e-nose analysis, headspace generation time increases the concentration of volatile compounds from the sample and is often encouraged when using the e-nose. The speed at which the volatile compounds are brought to the e-nose sensor chamber should be taken into consideration in its application as it influences the signal strength (González-Martín et al. Citation2000).
In addition, the design of most e-nose’s often makes it possible to be used in open environments with varying environmental conditions such as humidity, temperature and the presence of diverse gases. Although most e-noses are capable of working under such conditions, their effectiveness can be influenced.
According to Schaller, Bosset, and Escher (Citation1998), a good sensor “should have highest sensitivity to the target group of chemical compound(s) intended for detection and with a threshold of detection similar to that of the human nose (10-12 g mL-1)”. It is therefore crucial to have an idea about the kind of gas to be expected from the food item investigated in order to select an appropriate gas sensor for optimum sensitivity. It is also important that the selected gas sensor should have a relatively low sensitivity to variable environmental conditions. Wojnowski et al. (Citation2019) developed electrochemical sensors with good longevity (up to 10 years lifespan) and could withstand changes in relative humidity but the complex headspace of meat samples limits their qualitative and quantitative applications in meat. Comprehensive details (design, applications, advantages and disadvantages) about major types of e-nose gas sensors and their sensitivities to odors, have been reported by Berna (Citation2010).
Applications of e-nose for red meat quality
shows the trend of e-nose application for red meat quality. E-nose based on metal oxide sensors was effectively applied for rapid prediction of ochratoxin A-producing strains and Penicillium strains during the seasoning process of dry-cured pork meat products (Lippolis et al. Citation2016).
Table 4. Some recent trends of e-nose application for red meat quality.
The e-nose has been established as a faster approach for discrimination compared to the traditional microbial plating which often takes several hours or days to get results, but there is a paucity of information in correlating specific aroma compounds of spoiled meat to the presence of a specific microbial species. This suggests that, although the e-nose could be used for quick preliminary analysis of meat spoilage, microbial plating may still be required for details about the microbes’ present. This makes the e-nose a viable tool for corelative analysis.
In meat authentication, mutton was blended with pork at adulteration levels of 0%, 20%, 40%, 60%, 80% and 100% and sealed with plastic, in a beaker for a headspace generation time of 30 min (Tian, Wang, and Cui Citation2013). It has been reported that some sensors have no reaction to the fresh beef but have intense reaction to the decayed beef (Najam et al. Citation2012). This is acceptable as the smell of a fresh product is often less intense compared to when it is decayed.
Haddi et al. (Citation2015) evaluated the performance of an e-nose and e-tongue system for the distinction of beef, goat and sheep among a mixed variety of meat. It was proven that PCA analysis of the e-nose data could not differentiate correctly between the 3rd and 6th day of storage of beef, goat and sheep meats but e-tongue results showed a good separation between storage days for the three types of meats. This is particularly relevant because e-nose is believed to be better suited for solid samples than the e-tongue. It is also an indication that, although all the gases go into the head space, not all changes can be detected. Nonetheless, the e-nose was still successful in discriminating red meat coming from different animal origins.
Applications of e-nose for poultry quality
shows the trend of e-nose application for poultry and white meat quality. Indirect determination of chicken fat oxidation was performed with an e-nose equipped with 18 MOSFET gas sensors where, e-nose proved to be a viable tool for predicting chicken fat oxidation. Chicken meat is composed of high amounts fatty acids that are susceptible to oxidative reactions that compromise the quality of the meat (Henchion et al. Citation2014). E-nose provides a means for monitoring such deterioration.
Table 5. Some recent trends of e-nose application in poultry.
Unmarinated broiler chicken cuts were stored differently under modified atmosphere packaging and assessed for shelf-life qualities with an e-nose equipped with MOSFET sensors. Chicken breast stored under different temperatures for different days were also accurately classified with the e-nose (Smolander and Ahvenainen Citation2006). Some gas sensors were more sensitive to reducing gases at varying temperatures of chicken storage.
E-nose could also predict biogenic amines in poultry with a good accuracy. Biogenic amines are nonvolatile compounds produced by interactions between decarboxylase- positive microorganisms and free amino acids (Henchion et al. Citation2014). It is therefore an indication of microbial presence and its index is a good reference method for evaluating meat freshness based on headspace analysis and fingerprinting with e-nose.
Egg freshness could also be predicted with 95% accuracy in PLSR using a MOSFET equipped e-nose. Portable e-noses can provide on-site monitoring of egg quality in poultry farms.
Applications of e-nose for fish quality
shows the trend of e-nose application for fish quality. An 18 MOS sensor was used for an accelerated but comprehensive chemotaxonomic discrimination of four species of marine fish surimi. The sensors were located in three different chambers controlled at different temperature because the sensors of e-nose were temperature sensitive. This is very essential for quality assurance of fish, particularly for consumers with allergies or preference for different types of fishes. The study was done in combination with chemical analysis proving the efficacy of e-nose as vital complimentary tool for the fish characterization.
Table 6. Some recent trends of e-nose application for fish quality.
Pattarapon et al. (Citation2018) recently, demonstrated the capability of e-nose in discriminating different aromas from grass carp fish after 14 days of storage in vacuum conditions. In their study, sensor 1 (for aromatic compounds), sensor 2 (for sulfides, mercaptan, thioether), sensor 7 (for fatty hydrocarbon derivatives), sensor 8 (helium), 10 (hydrocarbon), and sensor 12 (sulphide) proved to be very effective. Vacuum packaging effectively extends the shelf-life of fishery products by maintaining their odors and flavor, e-nose thus can be very instrumental in its quality assurance process.
The future of e-nose for red meat, poultry and fish analysis
There is a potential of the e-nose for rapid authentication and quality assurance in meat and fish but the future prospects () of the instruments’ application in this field also needs to be addressed for consistency and reliability. The semiconductor of metal oxide (MOS-FET) gas sensor array was the most prevalent e-nose gas sensor. Small size, low power consumption, short response time, wide operating temperature and high efficiency are some of the advantages presented by this sensor type. MOSFET gas sensors offer excellent discrimination and lead the way for a new generation of “smart sensors” with a great influence on the future commercial markets for gas sensors. The e-nose poses no harm to the user nor the food sample, nor for the nature, thus, its application in the meat industry should therefore be encouraged. Unlike the e-tongue, the e-nose requires no sample transformation from solid to liquid state and is better suited for sensing meat and fish volatile compounds as observations can be made both in the liquid and solid states. Quality control is of great importance within the meat and fish industry and with these advantages of e-nose, it would be possible to monitor meat and fish as raw materials or throughout the processing chain. More studies are however required for optimum sensitivity to the different types of gases that can be emitted from the sample and also the headspace waiting time per sample. Different statistical approaches have been used for diverse studies in fish and meat but PCA, LDA and PLSR were the most dominant. According to Tian, Wang, and Cui (Citation2013) stepwise discriminant analysis (Step-LDA) is a very effective method for the pretreatment of multidimensional signals of the e-nose. Portable e-noses have been used for both meat and fish analysis (Güney and Atasoy Citation2015; O’Connell et al. Citation2001; Timsorn et al. Citation2016; Wojnowski et al. Citation2019) and, provides potentials for in-situ analysis in commercial environments.
Conclusion
Electronic sensor devices are gaining prominence in qualitative and quantitative evaluation of red meat, poultry and fish. The electronic tongue and electronic nose have been used for meat authentication, classification and shelf-life-storage. Depending on the sensor installed in the device, the electronic tongue determines the classes of fat in meat and even biogenic amines in fish. This means the device cannot just perform sensory assessment but can be used for toxicological purposes as well. This function could also be explored to determine naturally occurring toxins in other foods. Among the different types of e-tongue sensors, the ion sensitive field effect transistors (ISFET), a subgroup of the potentiometric e-tongues, appeared to be of emerging interest to researchers for its cross-selectivity functions. The potentiometric e-tongue in general also appeared to be the most frequently used but the potential of the voltammetric e-tongue is not limited (except in instances of redox experiments). Sensor variation also proved essential in e-nose with MOSFET sensor type as the most explored. The e-nose was effective in predicting freshness and adulteration in pork. Contrary to popular notion that adulteration is mainly focused on liquid and powders, it has recently reared its ugly head in the meat industry as well. The rapid non-destructive nature of e-nose analysis therefore puts it in the right position to control this canker. In spite of the acknowledged potentials of the e-nose in this review, developing specific sensors for specific meat/fish attributes and assessing the factors that decrease their sensitivity will undoubtedly improve performance for sensory and quality control of meat/fish. Both devices have advantages of no waste (no reagents), cost efficiency, high sensitivity and compatibility with chemometric tools for reliable data analysis and monitoring of meat and fish quality. The instruments can also be used as correlative techniques with other analytical instruments for precision and accuracy. Further study into the longevity of sensors is recommended.
Disclosure statement
The authors declare no conflict of interest.
Additional information
Funding
References
- Apetrei, I. M., and C. Apetrei. 2016. Application of voltammetric e-tongue for the detection of ammonia and putrescine in beef products. Sensors and Actuators B: Chemical 234:371–9. doi: 10.1016/j.snb.2016.05.005.
- Baldwin, E. A., J. Bai, A. Plotto, S. Dea, U. Citrus, S. N. W. Ave, and W. Haven. 2011. Electronic noses and tongues: Applications for the food and pharmaceutical industries. Sensors 11 (5):4744–66. doi: 10.3390/s110504744.
- Banerjee, R., B. Tudu, R. Bandyopadhyay, and N. Bhattacharyya. 2016. A review on combined odor and taste sensor systems. Journal of Food Engineering 190:10–21. doi: 10.1016/j.jfoodeng.2016.06.001.
- Berna, A. 2010. Metal oxide sensors for electronic noses and their application to food analysis. Sensors 10 (4):3882–910. doi: 10.3390/s100403882.
- Campbell, G. A., J. A. Charles, K. Roberts-Skilton, M. Tsundupalli, C. K. Oh, A. Weinecke, R. Wagner, and D. Franz. 2012. Evaluating the taste masking effectiveness of various flavors in a stable formulated pediatric suspension and solution using the AstreeTM electronic tongue. Powder Technology 224:109–23. doi: 10.1016/j.powtec.2012.02.038.
- Campos, I., M. Alcañiz, D. Aguado, R. Barat, J. Ferrer, L. Gil, M. Marrakchi, R. Martínez-Mañez, J. Soto, and J.-L. Vivancos. 2012. A voltammetric electronic tongue as tool for water quality monitoring in wastewater treatment plants. Water Research 46 (8):2605–14. doi: 10.1016/j.watres.2012.02.029.
- Cesar Paixão, T. R. L., and M. R. Subrayal. 2017. Materials for Chemical Sensing. Lancashire: Springer. http://link.springer.com/10.1007/978-3-319-47835-7.
- Chaudhary, M., and A. Gupta. 2009. Microcantilever-based sensors. Defence Science Journal 59 (6):634. Elsevier Inc. doi: 10.14429/dsj.59.1569.
- Chu, S., and C. D. Metcalfe. 2007. Analysis of paroxetine, fluoxetine and norfluoxetine in fish tissues using pressurized liquid extraction, mixed mode solid phase extraction cleanup and liquid chromatography-tandem mass spectrometry. Journal of Chromatography A 1163 (1–2):112–8. doi: 10.1016/j.chroma.2007.06.014.
- Ciosek, P., and W. Wróblewski. 2011. Potentiometric electronic tongues for foodstuff and biosample recognition — An overview. Sensors 11 (5):4688–701. doi: 10.3390/s110504688.
- Dutta, R., E. L. Hines, J. W. Gardner, D. D. Udrea, and P. Boilot. 2003. Non-destructive egg freshness determination : An electronic nose based approach. Measurement Science and Technology 14 (2003):190–8. doi: 10.1088/0957-0233/14/2/306.
- Escuder-Gilabert, L., and M. Peris. 2010. Review : Highlights in recent applications of electronic tongues in food analysis. Analytica Chimica Acta 665 (1):15–25. doi: 10.1016/j.aca.2010.03.017.
- Farrel, D. 2008. The role of poultry in human nutrition. Food and Agriculture Organization of the United Nations (FAO), 4072. doi:10.1016/j.jmaa.2004.03.027.
- Feng, X., S. H. Moon, H. Y. Lee, and D. U. Ahn. 2017. Effect of irradiation on the parameters that influence quality characteristics of raw turkey breast meat. Radiation Physics and Chemistry 130:40–6. doi: 10.1016/j.radphyschem.2016.07.015.
- Feng, X., C. Jo, K. C. Nam, and D. U. Ahn. 2018. Effect of Irradiation on the Parameters That Influence Quality Characteristics of Raw Beef Round Eye. Innovative Food Science and Emerging Technologies 45 (September 2017):115–21.
- García, M. R., M. L. Cabo, J. R. Herrera, G. Ramilo-Fernández, A. A. Alonso, and E. Balsa-Canto. 2017. Smart sensor to predict retail fresh fish quality under ice storage. Journal of Food Engineering 197:87–97. doi: 10.1016/j.jfoodeng.2016.11.006.
- Gębicki, J., and B. Szulczyński. 2018. Discrimination of selected fungi species based on their odour profile using prototypes of electronic nose instruments. Measurement 116 (August 2015):307–13. doi: 10.1016/j.measurement.2017.11.029.
- Gil, L., J. M. Barat, D. Baigts, R. Martínez-Máñez, J. Soto, E. Garcia-Breijo, M.-C. Aristoy, F. Toldrá, and E. Llobet. 2011. Monitoring of physical – chemical and microbiological changes in fresh pork meat under cold storage by means of a potentiometric electronic tongue. Food Chemistry 126 (3):1261–8. doi: 10.1016/j.foodchem.2010.11.054.
- Gil, L., E. Garcia-Breijo, J. M. Barat, R. Martínez-Máñez, J. Soto, F. Baena, J. Ibanez, E. Llobet, and J. Brezmes. 2007. Analysis of fish freshness by using metallic potentiometric electrodes. IEEE International Symposium on Industrial Electronics 131:1485–90. doi:10.1109/ISIE.2007.4374822.
- Gil, L., J. Soto, I. Campos, R. Masot, M. Alca, and E. García-Breijo. 2010. Chemical Accurate concentration determination of anions nitrate, nitrite and chloride in minced meat using a voltammetric electronic tongue. Sensors and Actuators B: Chemical 149:71–8. doi: 10.1016/j.snb.2010.06.028.
- Gliszczy, A. 2017. Electronic nose as a tool for monitoring the authenticity of food. A Review. Food Anal. Methods 10:1800–16. doi:10.1007/s12161-016-0739-4.
- Gong, H., Z. Yang, M. Liu, Z. Shi, J. Li, W. Chen, and X. Qiao. 2017. Time-dependent categorization of volatile aroma compound formation in stewed Chinese spicy beef using electron nose profile coupled with thermal desorption GC – MS detection. Food Science and Human Wellness 6 (3):137. doi: 10.1016/j.fshw.2017.07.001.
- González-Martín, I., J. L. Pérez-Pavón, C. González-Pérez, J. Hernández-Méndez, and N. Álvarez-García. 2000. Differentiation of products derived from Iberian breed swine by electronic olfactometry (electronic nose). Analytica Chimica Acta 424 (2):279–87. doi: 10.1016/S0003-2670(00)01106-5.
- Granato, D., P. Putnik, D. B. Kovačević, J. S. Santos, V. Calado, R. S. Rocha, A. G. D. Cruz, B. Jarvis, O. Y. Rodionova, and A. Pomerantsev. 2018. Trends in chemometrics: Food authentication, microbiology, and effects of processing. Comprehensive Reviews in Food Science and Food Safety 17 (3):663–77. doi: 10.1111/1541-4337.12341.
- Güney, S., and A. Atasoy. 2015. Study of fish species discrimination via electronic nose. Computers and Electronics in Agriculture 119:83–91. doi: 10.1016/j.compag.2015.10.005.
- Haddi, Z., N. E. Barbri, K. Tahri, M. Bougrini, N. E. Bari, E. Llobet, and B. Bouchikhi. 2015. Analytical Methods Instrumental assessment of red meat origins and their storage time using electronic sensing systems. Analytical Methods 7 (12):5193–203. doi: 10.1039/C5AY00572H.
- Han, F., X. Huang, E. Teye, and G. Haiyang. 2015. Quantitative analysis of fish microbiological quality using electronic tongue coupled with nonlinear pattern recognition algorithms. Journal of Food Safety 35 (3):336–44. doi: 10.1111/jfs.12180.
- Henchion, M., M. Mccarthy, V. C. Resconi, and D. Troy. 2014. Meat consumption : Trends and quality matters. Meat Science 98 (3):561–8. doi: 10.1016/j.meatsci.2014.06.007.
- Holmin, S., C. Krantz-Rülcker, I. Lundström, and F. Winquist. 2001. Drift correction of electronic tongue responses. Measurement Science and Technology 12 (8):1348–54. doi: 10.1088/0957-0233/12/8/350.
- Jiang, H., Q. Chen, and G. Liu. 2014. Monitoring of solid-state fermentation of protein feed by electronic nose and chemometric analysis. Process Biochemistry 49 (4):583–8. doi: 10.1016/j.procbio.2014.01.006.
- Joliffe, I. T., and B. Morgan. 1992. Principal component analysis and exploratory factor analysis. Statistical Methods in Medical Research 1 (1):69–95. doi: 10.1177/096228029200100105.
- Keeton, J. T., and M. E. Dikeman. 2017. Red’ and ‘white’ meats—terms that lead to confusion. Animal Frontiers 7 (4):29–33. doi: 10.2527/af.2017.0440.
- Lee, C.-S., S. Kim, and M. Kim. 2009. Ion-sensitive field-effect transistor for biological sensing. Sensors 9 (9):7111–31. doi: 10.3390/s90907111.
- Lippolis, V., M. Ferrara, S. Cervellieri, A. Damascelli, F. Epifani, M. Pascale, and G. Perrone. 2016. Rapid prediction of ochratoxin A-producing strains of Penicillium on dry-cured meat by MOS-based electronic nose. International Journal of Food Microbiology 218 (November):71–7. doi: 10.1016/j.ijfoodmicro.2015.11.011.
- Liu, D., S. Li, N. Wang, Y. Deng, L. Sha, S. Gai, H. Liu, and X. Xu. 2017. Evolution of taste compounds of Dezhou-braised chicken during cooking evaluated by chemical analysis and an electronic tongue system. Journal of Food Science 82 (5):1076. doi: 10.1111/1750-3841.13693.
- Loutfi, A., S. Coradeschi, G. K. Mani, P. Shankar, and J. B. B. Rayappan. 2015. Electronic noses for food quality : A review. Journal of Food Engineering 144 (August 2014):103–11. doi: 10.1016/j.jfoodeng.2014.07.019.
- Magdalena, S., and W. Waldemar. 2015. Food Analysis Using Arti Fi Cial Senses'. Journal of agricultural and food chemistry 6 (5):80–233.
- McWilliams, M. 2012. Meats, poultry, and fish. In Foods: Experimental Perspectives, 253–85. Los Angeles: California State University. https://www.pearson.com/us/higher-education/product/Mc-Williams-Foods-Experimental-Perspectives-7th-Edition/9780137079292.html.
- Medina, S., R. Perestrelo, P. Silva, J. A. M. Pereira, and J. S. Câmara. 2019. Current trends and recent advances on food authenticity technologies and chemometric approaches. Trends in Food Science & Technology 85 (December 2018):163–76. doi: 10.1016/j.tifs.2019.01.017.
- Najam, H., E. Naveed, E. Waleed, and S. K. Hyung. 2012. Meat and fish freshness inspection system based on odor sensing. Sensors 12:15542–57. doi:10.3390/s121115542.
- Nakano, M., Y. Sagane, R. Koizumi, Y. Nakazawa, M. Yamazaki, K. Ikehama, K. Yoshida, T. Watanabe, K. Takano, and H. Sato. 2018. Clustering of commercial fish sauce products based on an e-panel technique. Data in Brief 16:515–20. doi: 10.1016/j.dib.2017.11.083.
- O’Connell, M., G. Valdora, G. Peltzer, and R. Martín Negri. 2001. A practical approach for fish freshness determinations using a portable electronic nose. Sensors and Actuators B: Chemical 80 (2):149–54. doi: 10.1016/S0925-4005(01)00904-2.
- Ojemaye, C. Y., and L. Petrik. 2019. Occurrences, levels and risk assessment studies of emerging pollutants (pharmaceuticals, perfluoroalkyl and endocrine disrupting compounds) in fish samples from Kalk Bay Harbour, South Africa. Environmental Pollution 252:562–72. doi: 10.1016/j.envpol.2019.05.091.
- Palit, M., B. Tudu, N. Bhattacharyya, A. Dutta, P. K. Dutta, A. Jana, R. Bandyopadhyay, and A. Chatterjee. 2010. Comparison of multivariate preprocessing techniques as applied to electronic tongue based pattern classification for black tea. Analytica Chimica Acta 675 (1):8–15. doi: 10.1016/j.aca.2010.06.036.
- Papadopoulou, O. S., E. Z. Panagou, F. R. Mohareb, and G. E. Nychas. 2013. Sensory and microbiological quality assessment of beef fillets using a portable electronic nose in tandem with support vector machine analysis. Food Research International 50 (1):241–9. doi: 10.1016/j.foodres.2012.10.020.
- Pattarapon, P.,. M. Zhang, B. Bhandari, and Z. Gao. 2018. Effect of vacuum storage on the freshness of grass carp (Ctenopharyngodon idella) fillet based on normal and electronic sensory measurement. Journal of Food Processing and Preservation 42 (2):e13418–8. doi: 10.1111/jfpp.13418.
- Pennacchia, C., D. Ercolini, and F. Villani. 2011. Spoilage-related microbiota associated with chilled beef stored in air or vacuum pack. Food Microbiology 28 (1):84–93. doi: 10.1016/j.fm.2010.08.010.
- Persaud, K., and G. Dodd. 1982. Electronic Nose. In Nature:352–55. https://www.nature.com/articles/299352a0.
- Perumal, V., and U. Hashim. 2014. Advances in biosensors: Principle, architecture and applications. Journal of Applied Biomedicine 12 (1):1–15. doi: 10.1016/j.jab.2013.02.001.
- Podrazka, M., E. Báczyńska, M. Kundys, P. S. Jeleń, and E. W. Nery. 2017. Electronic tongue-A tool for all tastes? Biosensors 8 (1):1–24. doi: 10.3390/bios8010003.
- Pugliese, C., and F. Sirtori. 2012. Review: Quality of meat and meat products produced from southern European pig breeds. Meat Science 90 (3):511–8. doi: 10.1016/j.meatsci.2011.09.019.
- Ragnar, H., A. Christiansen, and E. Deribe. 2011. Application of QuEChERS method for extraction of selected persistent organic pollutants in fish tissue and analysis by gas chromatography mass spectrometry. Journal of Chromatography A 1218 (41):7234–41. doi: 10.1016/j.chroma.2011.08.050.
- Rateni, G., P. Dario, and F. Cavallo. 2017. Smartphone-based food diagnostic technologies: A review. Sensors 17 (6):1453. doi: 10.3390/s17061453.
- Rita, A., D. Rosa, F. Leone, F. Cheli, and V. Chiofalo. 2017. Fusion of electronic nose, electronic tongue and computer vision for animal source food authentication and quality assessment e A review. Journal of Food Engineering 210:62–75. doi: 10.1016/j.jfoodeng.2017.04.024.
- Rodríguez-Méndez, M. L., M. Gay, C. Apetrei, and J. A. De Saja. 2009. Biogenic amines and fish freshness assessment using a multisensor system based on voltammetric electrodes. Comparison between CPE and screen-printed electrodes. Electrochimica Acta 54 (27):7033–41. doi: 10.1016/j.electacta.2009.07.024.
- Rudnitskaya, A. 2018. Calibration update and drift correction for electronic noses and tongues. Frontiers in Chemistry 6 (September):433. doi: 10.3389/fchem.2018.00433.
- Rudnitskaya, A., A. Maria, S. Costa, and I. Delgadillo. 2017. Sensors and Actuators B : Chemical Calibration update strategies for an array of potentiometric chemical sensors. Sensors and Actuators B: Chemical 238:1181–9. doi: 10.1016/j.snb.2016.06.075.
- Ruiz-Rico, M., A. Fuentes, R. Masot, M. Alcañiz, I. Fernández-Segovia, and J. Manuel. 2013. Use of the voltammetric tongue in fresh cod (Gadus morhua) quality assessment. Innovative Food Science & Emerging Technologies 18:256–63. doi: 10.1016/j.ifset.2012.12.010.
- Sanaeifar, A., H. ZakiDizaji, A. Jafari, and M. d l Guardia. 2017. Early detection of contamination and defect in foodstuffs by electronic nose: A review. TrAC Trends in Analytical Chemistry 97:257–71. doi: 10.1016/j.trac.2017.09.014.
- Schaller, E., J. O. Bosset, and F. Escher. 1998. Electronic noses” and their application to food. LWT - Food Science and Technology 31 (4):305–16. doi: 10.1006/fstl.1998.0376.
- Shi, C., X. Yang, S. Han, B. Fan, Z. Zhao, X. Wu, and J. Qian. 2018. Nondestructive prediction of tilapia fillet freshness during storage at different temperatures by integrating an electronic nose and tongue with radial basis function neural networks. Food and Bioprocess Technology 11 (10):1840–52.
- Shlens, J. 2005. A Tutorial on Principal Component Analysis. International Journal of Remote Sensing 51 (2):1–13. https://www.cs.cmu.edu/∼elaw/papers/pca.pdf.
- Simões Da Costa, A. M., I. Delgadillo, and A. Rudnitskaya. 2014. Detection of copper, lead, cadmium and iron in wine using electronic tongue sensor system. Talanta 129:63–71. doi: 10.1016/j.talanta.2014.04.030.
- Smolander, M., and R. Ahvenainen. 2006. Application of an electronic nose for quality assessment of modified atmosphere packaged poultry meat. Food Control 17 (August):5–13. doi: 10.1016/j.foodcont.2004.08.002.
- Song, S.,. L. Yuan, X. Zhang, K. Hayat, H. Chen, F. Liu, Z. Xiao, and Y. Niu. 2013. Rapid measuring and modelling flavour quality changes of oxidised chicken fat by electronic nose profiles through the partial least squares regression analysis. Food Chemistry 141 (4):4278–88. doi: 10.1016/j.foodchem.2013.07.009.
- Soós, J., E. Várvölgyi, G. Bázár, J. Felföldi, I. Magyar, and Z. Kovács. 2015. Electronic tongue in botrytized wine authentication. 16th International Symposium on Olfaction and Electronic Nose, 75–6.
- Stroble, J., R. Stone, and S. Watkins. 2009. General overview and article picture –An overview of biomimetic sensor technology. Sensor Review 29 (2):112–9. doi: 10.1108/02602280910936219.
- Tan, J., X. Lu, L. Fu, G. Yang, and J. Chen. 2019. Quantification of Cl-PAHs and their parent compounds in fish by improved ASE method and stable isotope dilution GC-MS. Ecotoxicology and Environmental Safety 186 (October):109775. doi: 10.1016/j.ecoenv.2019.109775.
- Tarassenko, L., and M. Denham. 2006. Cognitive Systems - Information Processing Meets Brain Science Sensory Processing. 4th ed. Elsevier Ltd. http://dx.doi.org/10.1016/B978-0-12-088566-4.50008-8.
- Thevenot, D., K. Tóth, R. A. Durst, and G. S. Wilson. 1999. Electrochemical Biosensors: Recommended Definitions and Classification. Pure and Applied Chemistry 71 (12):2333–48.
- Tian, H. X., Y. J. Zhang, L. Qin, C. Chen, Y. Liu, and H. Y. Yu. 2018. Evaluating taste contribution of brown sugar in chicken seasoning using taste compounds, sensory evaluation, and electronic tongue. International Journal of Food Properties 21 (1):471–83. doi: 10.1080/10942912.2018.1424721.
- Tian, X., J. Wang, and S. Cui. 2013. Analysis of pork adulteration in minced mutton using electronic nose of metal oxide sensors. Journal of Food Engineering 119 (4):744–9. doi: 10.1016/j.jfoodeng.2013.07.004.
- Tikk, K., J. E. Haugen, H. J. Andersen, and M. D. Aaslyng. 2008. Monitoring of warmed-over flavour in pork using the electronic nose - correlation to sensory attributes and secondary lipid oxidation products. Meat Science 80 (4):1254–63. doi: 10.1016/j.meatsci.2008.05.040.
- Timsorn, K., T. Thoopboochagorn, N. Lertwattanasakul, and C. Wongchoosuk. 2016. Evaluation of bacterial population on chicken meats using a briefcase electronic nose. Biosystems Engineering 151 (June):116–25. doi: 10.1016/j.biosystemseng.2016.09.005.
- Tothill, I. E., and A. P. F. Turner. 2015. Encyclopedia of Psychopharmacology Biogenic Amines 2nd ed. Silsoe: Springer-Verlag Berlin Heidelberg. http://link.springer.com/10.1007/978-3-642-36172-2_200474.
- Tuczek, F. 2010. Recent advances in electronic absorption spectroscopy. The Royal Society of Chemistry 135:2481–2495. doi: 10.1021/bk-1998-0692.ch005.
- Valle, M., A. Mimendia, and J. M. Gutie. 2010. A review of the use of the potentiometric electronic tongue in the monitoring of environmental systems. Environmental Modelling & Software 25:1023–1030. doi: 10.1016/j.envsoft.2009.12.003.
- Vidal, N. P., C. Manful, T. H. Pham, E. Wheeler, P. Stewart, D. Keough, and R. Thomas. 2019. Dataset of the volatile compounds detected in unmarinated and marinated grilled ruminant meats with novel unfiltered beer-based marinades to improve their nutritional quality, safety, and sensory perception. Data in Brief 27:104622. doi: 10.1016/j.dib.2019.104622.
- Wang, Q., B. Xu, and H. Yu. 2017. Electronic nose and electronic tongue combined with fuzzy mathematics sensory evaluation to optimize bacon smoking procedure. Scientia Agricultura Sinica 50 (1):3864. doi: 10.3864/j.issn.0578-1752.2017.01.014.
- Wei, Z., Y. Yang, J. Wang, W. Zhang, and Q. Ren. 2018. The measurement principles, working parameters and configurations of voltammetric electronic tongues and its applications for foodstuff analysis. Journal of Food Engineering 217:75–92. doi: 10.1016/j.jfoodeng.2017.08.005.
- Wilson, A. D., and M. Baietto. 2011. Advances in electronic-nose technologies developed for biomedical applications. Sensors 11 (1):1105–1176. doi: 10.3390/s110101105.
- Winquist, F. 2008. Voltammetric electronic tongues – Basic principles and applications. Microchimica Acta 163:3–10.
- Wojnowski, W., K. Kalinowska, T. Majchrzak, J. Płotka-Wasylka, and J. Namieśnik. 2019. Prediction of the biogenic amines index of poultry meat using an electronic nose. Sensors 19 (7):1580. doi: 10.3390/s19071580.
- Wojnowski, W.,. T. Majchrzak, T. Dymerski, J. Gębicki, and J. Namieśnik. 2017. Electronic noses : Powerful tools in meat quality assessment. Meat Science 131 (April):119–131. doi: 10.1016/j.meatsci.2017.04.240.
- Woodvine, A. 2009. The health consequences of consuming chicken, turkey, duck and goose meat The health consequences of consuming chicken, turkey, duck and goose meat. York Court, Wilder Street, Bristol BS2 8QH.
- Wyness, L. 2016. The role of red meat in the diet: Nutrition and health benefits. Proceedings of the Nutrition Society 75 (3):227–232. doi: 10.1017/S0029665115004267.
- Yu, P., M. Y. Low, and W. Zhou. 2018. Design of experiments and regression modelling in food flavour and sensory analysis: A review. Trends in Food Science & Technology 71 (November 2017):202–215. doi: 10.1016/j.tifs.2017.11.013.
- Zhang, M., X. Wang, Y. Liu, X. Xu, and G. Zhou. 2012. Isolation and identification of flavour peptides from Puffer fish (Takifugu obscurus) muscle using an electronic tongue and MALDI-TOF/TOF MS/MS. Food Chemistry 135 (3):1463–1470. doi: 10.1016/j.foodchem.2012.06.026.
- Zhang, W., B. M. Naveena, C. Jo, R. Sakata, G. Zhou, R. Banerjee, and T. Nishiumi. 2017. Technological demands of meat processing – An Asian perspective. Meat Science 132 (April):35–44. doi: 10.1016/j.meatsci.2017.05.008.
- Zhang, X., Y. Zhang, Q. Meng, N. Li, and L. Ren. 2015. Evaluation of beef by electronic tongue system TS-5000Z : Flavor assessment, recognition and chemical compositions according to its correlation with flavor. PloS ONE 10 (September):1–10. doi: 10.1371/journal.pone.0137807.
- Zhang, Z., J. Tong, D. Chen, and Y. Lan. 2008. Electronic nose with an air sensor matrix for detecting beef freshness. Journal of Bionic Engineering 5 (1):67–73. doi: 10.1016/S1672-6529(08)60008-6.
- Shuangjuan, Z., F. Xi, H. Zhicheng, D. Changsheng, T. Jixia, L. Xiaoyu, … H. Wen. 2018. Effect of cooking methods on the chemical compounds associated with umami taste in duck breast meat. Journal of Food Science and Engineering 8 (2):65–73. doi: 10.17265/2159-5828/2018.02.002.