Abstract
A large number of herb-drying studies have been conducted in recent decades and several herb-drying techniques have been introduced. However, the quality of commercial dried herbs is still lower than that of fresh herbs. In this paper, studies regarding the effect of drying techniques and pre-drying treatments on the aroma and color of dried herbs are reviewed with the aim of providing an overview of different technological strategies developed for improving the quality of aromatic herbs for their industrial drying.
Introduction
Herbs are “any plant with leaves, seeds, or flowers used for flavoring, food, medicine, or perfume” (2019). Herbs are considered to be highly perishable foods due to their high moisture content and most herbs are chill-sensitive (Pirbalouti, Mahdad, and Craker Citation2013). They are therefore processed by drying to create shelf-stable products (Orphanides, Goulas, and Gekas Citation2016). Drying preserves the quality of herbs by reducing the moisture content, which inhibits the growth of microorganisms and chemical alterations during dried storage (Diaz-Maroto, Perez-Coello, and Cabezudo Citation2002b). In the culinary sense, dried herbs are generally used as “flavoring” agents to add their characteristic aromas to the foods. Apart from the culinary usages of herbs, their essential oil can be used as an antimicrobial agent that is effective against bacteria, yeast, and molds (Bor et al. Citation2016). Dried herbs also have many applications in other fields, such as in medical and toiletry products and in perfume manufacturing. Herbs are known to be an excellent source of antioxidants (Embuscado Citation2015). The quality characteristics considered to be the most important for dried herbs may depend on their usage. For instance, the quality of medical dried herbs is defined by the content of bioactive compounds (Ebadi et al. Citation2015), while the quality of culinary dried herbs is usually defined by their color and fresh-like characteristic aroma (Rahimmalek and Goli Citation2013). The focus of dried-herb quality in this review will be on the color and aroma.
A large number of herb-drying studies have been conducted in recent decades and several herb-drying techniques have been introduced. Studies on herb-drying methods have received increased attention in the past 20 years. For example, when using the Web of Science with “herb” and the name of drying method as topics and “drying” as a title, an increasing trend of studies can be seen in different drying methods (). Drying techniques have been developed that aim to improve quality as well as provide new possibilities to increase the efficiency of the drying process. Several drying techniques have been introduced in recent years, namely supercritical carbon dioxide drying (Busic et al. Citation2014) and heat-pump-assisted drying (Artnaseaw, Theerakulpisut, and Benjapiyaporn Citation2010). Besides the development of those drying techniques, the development of pre-drying treatments has also received considerable attention. A number of pretreatments for the drying of herbs have been studied during the past decades, such as ultrasound (de la Fuente-Blanco et al. Citation2006) and pulsed electric field (Kwao et al. Citation2016). Along with the developed drying methods and pretreatments, innovations have been introduced in solar-powered drying systems. Innovative integrated solar drying systems have been developed, such as heat-pump integrated solar dryers (Tham et al. Citation2017) and fluidized bed solar dryers (Ceylan and Gurel Citation2016). Hybrid drying, which combines two or more drying techniques have also been tested. Jin et al. (Citation2018) reviewed several hybrid drying technologies such as solar-hot air drying, microwave-vacuum drying, and hot air- low humidity drying. Most of these developments aimed to decrease the drying time or lowering the drying temperature (Jin et al. Citation2018). The aim of this paper is to systematically review drying and pre-drying methods used for improving the quality of dried culinary herbs.
Quality characteristics of dried herbs
Dried culinary herbs are usually high in value, thus, the expectation of consumers regarding the quality of the product are generally high (Schaarschmidt Citation2016). The quality specifications of dried herbs have been listed mostly to ensure the chemical and microbiological safety of the products, such as, moisture content, bulk density, foreign matter, the content of excreta, aflatoxins and heavy metals. reports the drying technologies that have been used for various types of herbs as well as the quality properties that were analyzed. Among these quality properties, color and aroma are probably the most important quality characteristics affecting consumer acceptance (Schaarschmidt Citation2016). In this section, important aspects of aroma and color properties of the dried herbs will be reviewed.
Table 1. Analyzed properties for different herbs and drying methods.
Aroma compounds
Essential oil is the main contribution of herb aroma although it is present in small amounts (Rao et al. Citation1998). The International Organization for Standardization (ISO) has defined the meaning of the term “essential oil” as a “product obtained from a natural raw material of plant origin, by steam distillation, by mechanical processes from the epicarp of citrus fruits, or by dry distillation, after separation of the aqueous phase — if any — by physical processes” (ISO 9235:2013). Essential oils can be used in many types of applications, such as pharmaceutics, cosmetics, and the medical and food industries (Orphanides, Goulas, and Gekas Citation2016). In fresh herbs, essential oils are stored on the surface of the leaves in specialized structures called trichomes, which are uni- or multicellular appendages in the epidermal cells that develop outwards from the surface of plant organs such as leaves, roots or barks (Werker Citation2000). Upon drying, the retention of essential oils in the dried leaves depends on the integrity of the oil glands in the dried product (Ebadi et al. Citation2015). Therefore, preserving trichome integrity or minimizing the damage to trichomes during drying could improve the yield of essential oils and the aroma quality of dried herbs. Volatile compounds in herbs can be also found in glycosidically-bound forms as they are water soluble and can be accumulated in the plant tissues (Winterhalter and Skouroumounis Citation1997).
Chemical composition of essential oil and its alterations during drying
Essential oils are composed of a few or many chemical compounds, with some types of herbs containing more than a hundred chemical compounds (Antal et al. Citation2011). The chemical composition of the essential oils varies depending on the type of herb, harvesting season, postharvest practices, age of the plant and storage conditions (Dokhani et al. Citation2005). Each chemical compound contributes its specific flavor to the essential oil. This contribution relies on their specific odor threshold, which can be determined by the structure and volatility of the compound (Turek and Stintzing Citation2013). The changes in the concentration of the essential oil chemical components (either by chemical reactions or degradation), even with minor components, may result in drastic changes in the essential oil flavor (Grosch Citation2001).
Essential oil can be divided into 2 fractions: (1) the volatile fraction that yields about 90-95% of the total oil. This fraction is mainly composed of monoterpenes, sesquiterpenes, aldehydes, alcohols, and esters; and (2) the nonvolatile fraction, which contains hydrocarbons, sterols, and other large molecular weight molecules such as triterpenes, squalenes and saponins (Humphrey and Beale Citation2006; Orphanides, Goulas, and Gekas Citation2016). Some major chemical compounds of herbal essential oils have been reported, such as 1,8-cineole in bay leaves (Diaz-Maroto, Perez-Coello, and Cabezudo Citation2002b), p-mentha-1,3,8-triene, β-phellandrene, and isopropenyl 4-methylbenzene in parsley (Diaz-Maroto, Perez-Coello, and Cabezudo Citation2002a); α-pinene, camphene, 1,8-cineole, camphor, bornyl acetate and borneol in rosemary (Rao et al. Citation1998); and α-pinene, β-pinene, 1,8-cineole, camphor, camphene, α-terpineol, caryophyllene, ascaridole and bornyl acetate in Iranian achillea species (Dokhani et al. Citation2005).
Many studies have been conducted to investigate the chemical profiles of essential oils. However, it should be noted that the methods for extraction and analysis methods of essential oils could influence the results (Diaz-Maroto, Perez-Coello, and Cabezudo Citation2002b). For example, the extracted essential oil from dried bay leaves using simultaneous distillation extraction (SDE) contained α-thujene, camphene, β-pinene and elemicin, while the essential oil obtained from direct thermal desorption and solid-phase micro extraction (SPME) did not contain such compounds (Diaz-Maroto, Perez-Coello, and Cabezudo Citation2002b). Some essential oil chemical components could be only artifacts of the extraction and analysis methods but not present in the fresh plants (Kubeczka Citation2009). Therefore, the extraction and analysis methods used need to be taken into consideration when comparing the amount or chemical compounds of essential oils. In addition, optimum sample preparation methods should be conducted to prevent the transformation of the analyzed components (Chen, Poon, and Lam Citation1998). Several essential oil extraction and analysis methods have been used, including hydro-distillation, solvent extraction or simultaneous distillation–extraction (SDE), and headspace methods (Lucchesi, Chemat, and Smadja Citation2004). Out of these methods, SDE and SPME are the most widely used (Diaz-Maroto, Perez-Coello, and Cabezudo Citation2002b).
The chemical constituents of essential oils are unstable substances. They can be easily converted into other types of compounds though various chemical reactions such as oxidation, isomerization, cyclization, or dehydrogenation reactions. These chemical reactions can be triggered either enzymatically or chemically (Turek and Stintzing Citation2013). One of the most important chemical alterations of the essential oil constituents is autoxidation. The autoxidation reaction affects the deterioration process of terpenoids, which is the largest class of natural volatiles in plants (Başer and Demirci Citation2011). During the autoxidation of terpenoids, secondary products such as hydroperoxides can be formed and then decomposed in the presence of light, heat, and increased acidity in advanced stages of the oxidation process (Turek and Stintzing Citation2013). These chemical alterations of the essential oil constituents could occur during either the drying process or the storage period of the dried products. The utilization of heat during drying process could accelerate these chemical reactions (Lee, Lee, and Choe Citation2007). During the drying process, heat promotes the initial formation of free radicals, which catalyze the autoxidation process of the essential oil (Choe and Min Citation2006). Therefore, increasing drying temperature will lead to greater loss of aroma compounds and, consequently, more aroma quality degradation in the dried herbs.
The presence of light is another important aspect affecting the degradation of essential oils, especially during the sun-drying process, where herbs are exposed to direct sunlight, or during the storage of dried herbs without light protection packages. The presence of light, either ultraviolet or visible, accelerates the autoxidation process by triggering hydrogen abstraction, which leads to the formation of lipid alkyl radicals (Choe and Min Citation2006). Two types of oxygen molecules are responsible for the autoxidation of oil: the singlet oxygen (1O2) and the triplet oxygen (3O2). While 1O2 is suggested to be mainly involved with the initial phase of the oil oxidation process (Lee and Min Citation1988), the 3O2 is likely to react with the alkyl radicals at normal oxygen pressure and form lipid peroxyl radicals. These lipid peroxyl radicals are likely to abstract hydrogen from other molecules and catalyze the oxidation process, leading to the degradation of the aroma compounds. In addition, there are other aspects affecting the formation and the decomposition of hydroperoxides such as the presence of oxygen, antioxidants, water content, metal contaminants and chemical structure of the compounds (Turek and Stintzing Citation2013).
Drying can cause a great reduction in the amount of essential oil in many types of herbs, reportedly 36–45% in basil, 23–33% in marjoram, and 6-17% in oregano even when the herbs were air-dried at room temperature (Nykanen and Nykanen Citation1987), as cited in (Diaz-Maroto, Perez-Coello, and Cabezudo Citation2002b). During the drying process, the volatile profile of the essential oil could change substantially due to the formation of secondary aroma compounds such as alcohols, aldehydes, peroxides, and ketones (Turek and Stintzing Citation2013). These secondary products may constitute a high percentage of the total volatile content in dried products. (Huopalahti, Kesalahti, and Linko Citation1985), reported that secondary aroma compounds might account for over 50% of the total volatile content in air-dried dill leaves (dried at 25, 40, and 50 °C). The changes in the essential oil compounds of herbs during the drying process might be a result of the release of compounds from the rupture of cell walls, oxidation reactions, or the hydrolysis of glycosylated volatile compounds (Xing et al. Citation2018).
The reduction or changes of the volatile compounds in dried herbs during the drying process depend on drying parameters including drying method, temperature, vacuum level (in case of processes such as vacuum drying or freeze drying), drying time, and amount of water evaporated during drying (Antal Citation2010; Figiel and Michalska Citation2016). In general, drying of herbs results in the reduction of volatile compounds and some drying methods might enable better preservation of the volatile compounds than others (Chua et al. Citation2019).
The drying temperature plays an important role on the preservation of volatile compounds of dried herbs after the drying process. Applying high drying temperature is commonly lead to the loss of volatile compounds content. At high drying temperature, trichomes may risk rupture which leads to the loss of volatile compounds through evaporation. In addition, high drying temperatures could promote the degradation of heat-labile compounds in the essential oil (Argyropoulos and Muller Citation2014). However, some contradictory results have been observed. In the case of hot-air dried lemon-myrtle leaves, drying temperature of 50 °C resulted in higher citral content compared to drying temperature of 30 and 40 °C. This better preservation effect might be caused by the crust layer which was formed on the leaves surface limiting the diffusion of high molecular weight volatile compounds from the tissues (Buchaillot, Caffin, and Bhandari Citation2009).
The vacuum level is one of the most important factors affecting the essential oil yield (Chua et al. Citation2019). In the case of freeze-dried spearmint, although decreasing the chamber pressure resulted in the decrease of drying time, it also caused a significant loss of volatile compounds (Antal et al. Citation2011). In the case of vacuum-microwave drying, increasing vacuum levels decreased the quality of volatile compounds of dried rosemary (Calín-Sánchez et al. Citation2011). The effects of these drying methods on the quality of dried herbs are reviewed in sections “Freeze drying” and “Microwave-vacuum drying” in this paper.
The amount of moisture evaporated from the tissues is another factor affecting the volatile compounds in dried herbs. In air-dried oregano, the amount of water evaporated was strongly correlated to the reduction of volatile compounds, as during the drying process water vapor might act as a carrier allowing the diffusion of volatile compounds from the tissues to the surroundings (Figiel et al. Citation2010). In addition, volatile compounds with high water affinity are more likely to be lost during the drying process (Sellami et al. Citation2011).
The changes in volatile compounds during the drying process also depend on the biological factors of the herbs, including initial moisture content, the age of the plant, growth conditions and harvesting time (Ascrizzi, Fraternale, and Flamini Citation2018). Storage conditions also affect the content of volatiles of the dried products, especially in the presence of light and oxygen (Baritaux et al. Citation1992). The reduction of some essential oil components can be considered to be a benefit, such as the reduction of pulegone, a hepatotoxin in Hedeoma pulegioides and Mentha pulegium (Asekun, Grierson, and Afolayan Citation2007; Chen, Lebetkin, and Burka Citation2001) reported that pulegone content in dried wild mint (Mentha longifolia L. subsp. capensis) was significantly reduced by hot-air drying at 40 °C. It has therefore been suggested that this type of mint be consumed in dried form rather than as fresh.
Color of dried herbs
The main objective of many herb-drying studies has been to improve the color of the dried products or reduce the color changes during drying and during storage (Baritaux et al. Citation1992). The color degradation in dried herbs is provoked by the degradation of pigments such as chlorophyll and anthocyanin. For green herbs, chlorophylls degradation is the most common change that may occur during the drying process (Rayaguru and Routray Citation2010). Lafeuille et al. (Citation2014) analyzed chlorophylls and its colored derivatives in culinary herbs influenced by various drying processes. In the paper, a chlorophyll degradation ladder was designed to assess the dried herbs color after the drying process. The ladder was separated into four categories by the amount of green pigments preserved after the drying process: (1) no significant impact (> 90% preserved), (2) low impact (65–90% preserved). (3) medium impact (35–65% preserved), and (4) important impact (< 35% preserved). According to these criteria, freeze drying can be categorized into the first ladder as it showed no significant impact on the content of green chlorophyll derivatives. The most popular drying method, hot-air drying, was categorized into the second ladder. Sun drying falls into the forth category due to its significant impact on the preservation of green chlorophyll derivatives. Heat degradation pathways of chlorophyll have been described (Di Cesare et al. Citation2003).Two major types of chlorophylls are responsible for the changes in herb color during the drying process: chlorophylls a and b. The chemical structures of the two chlorophylls are very similar, with the only difference being that chlorophyll b has an aldehyde group at the C7 position of its porphyrin ring. The color of chlorophyll a is blue green, while chlorophyll b is yellow-green. Due to the asymmetry at carbon C13, chlorophylls a and b might turn into their epimers chlorophyll a′ and b′ under mild process conditions. These epimers have almost exact visible spectrum to their non-prime forms and does not affect the color of the dried products. However, the prime (′) epimers are slightly less stable than their original form (Scheer Citation1991), therefore, chemical reactions might occur easier than non-prime epimers. The changes or removal of chlorophyll molecule periphery might create derivatives with the same chlorophyll visible spectrum. The most common changes in this group is the loss of phytol group at C17 due to hydrolytic reaction catalyzed by enzymes in plants such as chlorophyllase (Lafeuille, Lefevre, and Lebuhotel Citation2014). Chlorophyllide which is a derivative from the loss of phytol from the chlorophyll molecule has the same visible spectrum as chlorophyll, however, it has higher water solubility and could be lost easily during heating processes such as blanching, which is one of the most common pre-drying treatment. shows the degradation pathways of chlorophyll during the drying process. The loss of chelated Mg2+ from chlorophyll structure creates olive-brown pheophytin. Process steps which damage the cell membrane, such as harvesting, heating, or drying could allow sap acidic compounds to react with chlorophyll molecules and promotes the loss of chelated Mg2+. Chelated Mg2+ could be lost by both dry and moist heat and also occurred with external acid conditions (Scheer Citation1991). The loss of chelated Mg2+ is one of the most common color degradations of herb during drying process. In addition, chlorophyllide is more heat sensitive than chlorophyll in terms of losing its Mg2+, the loss of chelated Mg2+ from chlorophyllide molecules creates olive-brown pheophorbide. The loss of phytol group from the chlorophyll a structure by heat occurs easier than the loss of Mg2+ (Di Cesare et al. Citation2003; Eskin Citation1990). As chlorophyll a is more sensitive to heat than chlorophyll b, the degradation of chlorophyll a result in a change in the chlorophyll a/b ratio, which changes the color of the dried products from green-blue to green-yellow.
Figure 2. Chlorophyll degradation pathways during the drying process as described in Di Cesare et al. (Citation2003). Chemical structures were taken from PubChems’s database (Kim et al. Citation2019) and the structures were recreated using ChemDraw Cloud (version. 18.1.0-14 + eea6052, PerkinElmer, Waltham, MA, USA).
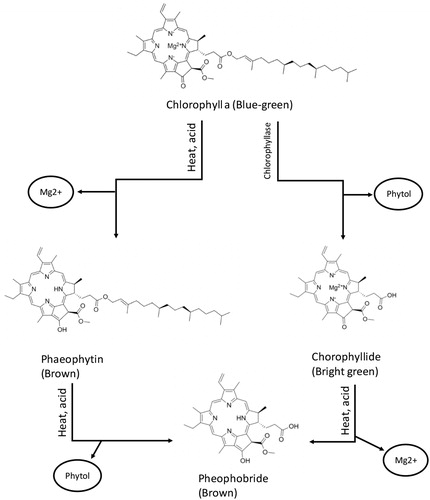
Collapsing during the drying process of the plant tissues could lead to the release of chlorophyll molecules from the protein complex, which could promote the transformation of chlorophylls into pheophytins due to greater exposure of the chlorophylls’ structure to heat. This event could also lead to the releasing of substrates for enzymatic browning reactions to the surrounding areas.
The degradation of chlorophylls a and b also depends on the type of plant. It has been shown that dried lovage and parsley, which are herbs of the Apiaceae family, showed higher retention of chlorophylls a and b in comparison with basil, mint and oregano, which are herbs of the Lamiaceae family (Sledz and Witrowa-Rajchert Citation2012). The changes in color could be reduced by optimizing the drying process parameters such as drying temperature, time, and air velocity. Pretreatments prior to drying, such as blanching (Di Cesare et al. Citation2003) and pulsed electric field (Kwao et al. Citation2016), were reported to improve the color of the dried herbs.
Pretreatments for drying of herbs
Pretreatments prior to drying are processing strategies aimed at achieving high-quality dried herbs, shorten the drying time, and reducing the energy consumption (Deng et al. Citation2019). Good pretreatments implementation should create only minimal modification to the drying process settings to reduce the follow-up costs from the modification (Rooy Citation2012). summarizes studies reviewed in this section on the effect of pretreatments on the quality of dried herbs. Several pretreatments have been reported to provide benefits for drying of herbs, such as blanching, pulsed electric field, and ultrasonic treatment. In this section, the effect of pretreatments on the quality of the dried herbs prior to various drying methods will be reviewed.
Table 2. Effects of pretreatments on the quality of dried herbs.
Blanching
Blanching provides benefits to the drying of many types of herbs. The major benefit of blanching is the reduction of color degradation. It was reported that blanching reduced the drying time of basil. Steam blanching for 15 s increased the drying rate by a factor of 10 compared to untreated leaves (Rocha, Lebert, and Martyaudouin 1993). The steam-blanched dried basil leaves also showed better color retention and increased chlorophyll a/b ratio. Similar results were observed in parsley leaves; the steam-blanched parsley showed a 30% faster drying rate and energy consumption was reduced by 72% in comparison with the drying of untreated leaves. Also, the blanched dried parsley showed good lutein content retention and better color retention (Sledz et al. Citation2016). A similar result was obtained in dill leaves blanched in hot water for 1 min prior to drying with several drying methods, including through-flow drying (45 °C), cross-flow drying (40 °C), vacuum drying (45 °C), and freeze drying (Raghavan et al. Citation1994). Blanching decreased the drying time for all drying methods tested. However, it was reported that blanching caused higher loss of the total essential oil content in the dried products. The opposite results were observed in blanched coriander leaves (80 °C in water), where the drying rate was slower in comparison with untreated leaves (Ahmed, Shivhare, and Singh Citation2001). Nevertheless, blanching resulted in better chlorophyll retention and higher rehydration capacity of the dried products compared to un-blanched dried products. Similar results were observed in basil (Nani et al. Citation2001). Using blanching in combination with chemical agents could provide benefits to the dried products; adding potassium metabisulfite to the blanching solution improved the retention of ascorbic acid, beta-carotene, and chlorophyll of dried amaranth and fenugreek leaves (Negi and Roy Citation2000).
There are different blanching techniques for herbs, such as water blanching, steam blanching, and microwave blanching (Singh, Raghavan, and Abraham Citation1996). The blanched dried marjoram and rosemary treated with all these blanching techniques showed better color retention in comparison with un-blanched dried products. In addition, water blanching showed the best color retention, followed by microwave and steam blanching. However, microwave blanching showed higher ascorbic acid content and better textural properties. Vacuum blanching, where the herbs are packed in a vacuum bag and then blanched in hot water, provided better retention of bioactive compounds in the dried product. The effect of water blanching and vacuum blanching (at 100 °C) on the quality of dried java tea prior to convective drying, heat pump dehumidify drying, mixed-mode solar drying, and freeze drying has been reported (Klungboonkrong, Phoungchandang, and Lamsal Citation2018). The results showed that vacuum blanching resulted in higher contents of sinensetin and eupatorin in the dried product in comparison with water blanching. Also, the vacuum-blanched dried leaves (dried using heat pump dehumidify dryer) showed better cell wall integrity than un-blanched samples.
Blanching can also be detrimental to herb quality. It has been reported to cause significant loss of the antioxidant properties in some types of herbs such as clove basil, Basella alba, Corchorus olitorius, and Solanum macrocarpon (Oboh Citation2005). Moreover, blanching was also reported to cause the degradation of aroma in some types of herbs such as basil, where the destruction of oil glands was observed. Blanching caused higher loss of aroma compounds in samples dried with several drying methods including air drying (50 °C), microwave drying, and freeze drying. The un-blanched-freeze dried sample was the only sample that showed no reduction in aroma compounds.
Pulsed electric field (PEF)
Pulsed electric field (PEF) is a non-thermal processing method that applies an external electric field to cells or tissues, provoking poration of the cell membrane. PEF has gained extensive attention due to its wide application range in food processing, such as extraction, drying, and microbial inactivation (Khan et al. Citation2018). Several studies on the effect of PEF on the drying of several types of plant raw materials have been conducted (Huang et al. Citation2018; Kwao et al. Citation2016; Ostermeier et al. Citation2018; Parniakov et al. Citation2016; Telfser and Galindo Citation2019). Most of these studies focused on the use of irreversible permeabilization (cells do not survive the application of PEF) of plant tissues, provoking permanent damage to the cell membrane and resulting in increased moisture diffusion coefficient and drastic reduction in drying time. This reduction in drying time could provide favorable results in the drying of heat-sensitive foods such as herbs (Orphanides, Goulas, and Gekas Citation2016). To the best of our knowledge, only two studies (Kwao et al. Citation2016; Telfser and Galindo Citation2019) have investigated the effect of reversible permeabilization (cells survive the application of PEF) as a pretreatment for drying on the quality of herbs. Both reversible and irreversible electroporation were able to electroporate guard cells of the stomata of basil leaves (Kwao et al. Citation2016). The electroporation of guard cells provoked sustained stomatal opening during the hot-air drying process, which increased the drying rate and improved color, aroma, and rehydration capacity of treated samples. Telfser and Galindo (Citation2019) studied the effect of reversible permeabilization in combination with different drying processes (hot-air drying, vacuum drying, and freeze drying) on the quality of basil. This study showed that the reversible permeabilization treatment of the tissues reduced the drying time for every tested drying method (57% for hot-air drying, 33% for vacuum drying, and 25% for freeze drying). Moreover, reversibly PEF-treated leaves showed better preservation of trichome integrity with both hot-air and vacuum drying in comparison with untreated leaves. However, the trichomes of freeze-dried samples were damaged in both PEF-treated and untreated leaves.
Ultrasound
Ultrasound is a non-thermal pretreatment for drying of food materials. The process is conducted by applying high-power ultrasound with low frequencies (20–100 kHz) and high intensities (10–1000 W/cm) to the food material, resulting in increased mass transfer without heating or with only very subtle heating (Tiwari and Mason Citation2012). Ultrasound induces the formation of micropores on the surface of the materials, which results in a lower case-hardening effect at the top of the material surfaces during drying, which would inhibit water removal (Fernandes and Rodrigues Citation2007). This treatment has been reported to improve the drying rate of the convective drying of plant-based foods (Kowalski and Rybicki Citation2017). The studies of ultrasound as a pretreatment for drying processes have been reported in many types of foods (de la Fuente-Blanco et al. Citation2006; Gamboa-Santos et al. Citation2014; Garcia-Perez et al. Citation2007; Santacatalina et al. Citation2015; Schossler, Jager, and Knorr Citation2012). In herbs, ultrasonic-treated dried parsley showed higher total phenolic, chlorophyll, and lutein content in comparison with non-treated leaves (Dadan et al. Citation2018). However, it was reported that the best method for pretreating parsley was steam blanching, considering the content of polyphenols, antioxidant activity, chlorophyll a, chlorophyll b and lutein. The effect of high-power ultrasound (HPU) as a pretreatment for the supercritical carbon dioxide drying process (scCO2) of coriander leaves was reported (Michelino et al. Citation2018). The HPU pretreatment was used to improve the drying time and provided better microorganism inactivation effect in comparison with the non-treated dried product. Similar results were observed in the drying of thyme leaves (Rodriguez, Mulet, and Bon Citation2014), where the ultrasound treatment resulted in the reduction of drying time by 30% in comparison with untreated leaves. However, the decreasing drying time effect of ultrasound pretreatment was only observed at a drying temperature below 70 °C (drying temperatures of 40, 50, 60, 70 and 80 °C were investigated).
Herbs drying methods
Drying method is one of the main factors affecting the quality of dried herbs (Diaz-Maroto, Perez-Coello, and Cabezudo Citation2002b) and its influence has been extensively studied. summarizes studies reviewed in this section on the effect of drying methods on the quality of dried herbs. Drying methods applying high temperature would significantly decrease the amount of aroma compounds, since aroma compounds are heat-sensitive substances and can be evaporated from plant tissues easily during drying (Khangholil and Rezaeinodehi Citation2008). In contrast, the essential oil content in some types of herbs has been reported to be unaffected by the drying method tested, namely Mexican oregano (shade, sun, and 40 °C were compared) (Calvo-Irabien et al. Citation2009) and bay leaf (convective drying at 40, 50, and 60 °C, sun drying, and shade drying were compared) (Demir et al. Citation2004).
Table 3. Effects of drying methods on the quality of dried herbs.
There are several well-known herb-drying methods such as sun drying, shade drying, freeze drying and hot-air drying. Among these drying methods, hot-air oven drying in the temperature range of 40–60 °C is the most common drying method used in herb drying studies in lab scale experiments (Shaw et al. 2016). Due to undesirable effects of high drying temperature on the quality of dried products, many studies have focused on the development of alternative drying methods, which could provide advantages over conventional methods. Some of these methods, such as solar-assisted drying (Ceylan, and Gurel Citation2016), microwave drying (Arslan and Özcan Citation2012), microwave-vacuum drying (Giri and Prasad Citation2007), infrared-assisted drying (Łechtańska, Szadzińska, and Kowalski Citation2015), heat-pump drying (Fatouh et al. Citation2006), and contact drying (Tarhan et al. Citation2011) are already being used in the industry. In the next sections, the effect of both conventional and newly developed drying methods on the quality of dried herbs will be reviewed.
Sun drying
Sun or solar drying is the oldest drying method that has been and is still used to dry many types of agricultural products, such as medical plants and aromatic herbs in most tropical or sub-tropical countries (Orphanides, Goulas, and Gekas Citation2016). During the process, fresh herbs are placed on well-ventilated drying racks and are exposed directly to the sunlight (Janjai, and Bala Citation2012). Sun drying may not be a suitable drying method for some types of herbs due to lower product quality. Sun drying causes a substantial color and aroma degradation in dried herbs. In the case of roman chamomile, the amount of major volatile components such as isobutyl isobutyrate, 3-methylbutyl isobutyrate and propyl tiglate of sun-dried roman chamomile was lower than that of hot-air-dried samples (dried at 40 °C) (Omidbaigi, Sefidkon, and Kazemi Citation2004). In the case of lemon grass, the sun-dried lemon grass was found to contain lower amounts of total essential oil in comparison with dried lemon grass obtained from hot-air drying (Hanaa et al. Citation2012). In basil (Ocimum basilicum L.), sun drying caused a greater reduction of essential oil content compared to shade drying and hot-air drying at 40 °C (Hassanpouraghdam et al. Citation2010). Sun drying also caused higher damage to the epidermal surface, shrinkage of the glandular trichomes and higher reduction of mineral content in Vernonia amygdalina leaves in comparison with shade drying (Alara et al. Citation2018).
Shade drying
Shade drying is another herb drying method that utilizes solar energy as a heating source. The process is conducted in almost the same way as sun drying, except that the herbs are placed under the shade in a room with good ventilation, low humidity (e.g. 22–27% for Lippia citriodora (Ebadi et al. Citation2015) and with no direct exposure to sunlight. During the shade-drying process, the ventilated air is heated up using solar energy before passing through the herbs (Sharma, Chen, and Lan Citation2009). This drying method could provide advantages over sun drying due to its ability to preserve light-sensitive substances and minimize light-induced chemical reactions such as oxidation. However, the drying time of shade drying is longer than sun drying, which is already considered to be an excessively long time process (Pirbalouti, Mahdad, and Craker Citation2013). Studies using this drying method have shown that shade drying is a better drying method in terms of preserving essential oil content and color of the dried products in comparison with other drying methods such as hot-air drying, sun drying, microwave drying and freeze drying for many types of herbs, namely rosemary (compared to oven drying at 45 °C and sun drying) (Khorshidi et al. Citation2009), Tanacetum parthenium (compared to oven drying at 40 °C and sun drying) (CitationOmidbaigi, Kabudani, and Tabibzadeh 2007), thyme (compared to freeze drying) (Sárosi et al. Citation2013), basil (compared to oven drying at 40 and 60 °C and sun drying) (Hassanpouraghdam et al. Citation2010), mint (compared to convective drying at 40 °C) (Rababah et al. Citation2015), lemon balm (compared to convective drying at 40 °C) (Rababah et al. Citation2015), and sage (compared to convective drying at 40 °C) (Rababah et al. Citation2015). Also, shade drying is a better herb-drying process in terms of preserving the integrity of the trichomes. It was found that shade drying caused less damage to trichomes on dried Lippia Citriodora leaves in comparison with oven drying at 60 °C and vacuum drying at 40 °C (Ebadi et al. Citation2015).
In terms of bioactive compound content, shade drying also showed good retention of bioactive compounds in dried herbs such as misai kucing (Orthosiphon aristatus) (Abdullah, Shaari, and Azimi Citation2012). When shade drying, sun drying and air drying of misai kucing (40 °C) were compared, it was found that the shade-dried product showed the highest total phenolic content. In addition, shade drying was the only drying method that could maintain the rosmarinic acid content close to the fresh herbs. However, shade drying caused significant loss of the functional properties in some types of herbs, for example, the total antioxidant activity (TAA) of peppermint and lemon balm decreased significantly after shade drying (with a drying temperature of 25–32 °C for 10 days) and the loss of ascorbic acid and carotenoids in the dried samples was observed (Capecka, Mareczek, and Leja Citation2005). In addition, lower contents of aroma compounds of some shade-dried herbs were reported in comparison with other drying methods. In the case of thyme, shade-dried thyme showed lower essential oil content in the dried product compared to hot-air drying at 50 and 70 °C, sun drying and freeze drying (Rahimmalek and Goli Citation2013). Nevertheless, like sun drying, shade drying is still popular in rural areas or in small businesses due to its low investment cost and high-quality dried products (Janjai and Bala Citation2012).
Solar-assisted drying
Solar-assisted drying is a development of a well-known drying method, sun drying. Since solar energy is costless, the development of new solar-assisted drying techniques has gained considerable attention from researchers. This development is aimed at increasing the energy efficiency of the drying process and overcoming the major problems of traditional sun drying. Solar drying can be categorized into three main groups, (1) direct sunlight drying (which is the same as sun drying in this review), (2) indirect solar drying or convective solar drying, (3) mixed-mode or hybrid solar drying (Rabha, Muthukumar, and Somayaji Citation2017). Several studies on the development of solar-assisted dryers of herbs have been conducted in recent years, namely forced convection solar tunnel dryers (Rabha, Muthukumar, and Somayaji Citation2017), forced convection solar greenhouse dryers (Morad et al. Citation2017), solar-assisted fluidized bed dryers (Ceylan and Gurel Citation2016), and solar collector dryers (Sevik Citation2014). Many types of herbs dried using solar-assisted dryers have been studied, for example thyme and mint (indirect mode forced convection solar dryer) (El-Sebaii, and Shalaby Citation2013), peppermint (using solar tunnel greenhouse dryer) (Morad et al. Citation2017), java tea (solar greenhouse dryer with integrated heat pump) (Tham et al. Citation2017), parsley (solar-heat pump dryer) (Sevik Citation2014), rosemary (solar collector with auxiliary heater, at 50–80 °C) (Mghazli et al. Citation2017), saffron (heat-pump-assisted hybrid photovoltaic-thermal solar dryer) (Mortezapour et al. Citation2012), and misai kucing (solar-assisted heat pump dryer) (Gan et al. Citation2017). The solar tunnel greenhouse dryer for peppermint leaves has shown a reduction in drying time of 23–25% in comparison with a regular greenhouse dryer (Morad et al. Citation2017). The solar-assisted dryer using the combination of the solar collector and heat pump system, which can be used to create a nonstop working solar dryer, was used to obtain dried mint leaves with good quality (considering thermal damage, shrinkage, and taste), similar to regular sun-dried products (Sevik Citation2014). With the combination of solar collector, heat exchanger, reflector, main and secondary drying chambers, and supplementary water heater, the solar dryer for the drying of chamomile showed reduced drying time by 50% compared to direct sun drying. Additionally, the product had higher volatile oil content (Amer, Gottschalk, and Hossain Citation2018). The bin-type solar dryer integrated with a solar collector produced better-quality dried rosella flower and lemongrass compared to a regular solar dryer (Janjai, and Tung Citation2005). The integration of solar-assisted dryer and dehumidification system provided better color of dried pegaga leaves due to the lower drying temperature and relative humidity of the solar-assisted dehumidification drying system in comparison with a regular solar dryer (Yahya et al. Citation2004). Many of these new developments in solar drying showed considerable improvement in comparison to the traditional sun drying, especially in the energy efficiency of the process and quality of the dried products. However, the studies on the effect of these processes on aroma and color of dried culinary herbs is still lacking.
Hot-air drying
As mentioned above, solar-powered drying methods have the major drawback of excessively long drying times. In the industry, the most common and popular herb-drying method is oven drying (also called “convective drying” or “hot-air drying”), especially in non-tropical countries where sunlight is not sufficient for sun and shade drying (Orphanides, Goulas, and Gekas Citation2016). The major advantage of hot-air drying is the controllability of the process, in which food producers have full control over the process parameters such as drying temperature, drying time, and air velocity. These parameters can be adjusted to achieve the desired product properties (Orphanides, Goulas, and Gekas Citation2016). The process parameters for many types of herbs have been investigated and optimized for better quality of dried products (Orphanides, Goulas, and Gekas Citation2016). However, after hot-air drying, low content of total volatile compounds is obtained (Chua et al. Citation2019). Hot-air drying could lead to major degradation of herb aroma and high drying temperature could lead to the degradation of pigments (Fennell et al. Citation2004). Therefore, low drying temperatures (35–50 °C) have been suggested for the preservation of heat-sensitive compounds in the dried products (Müller et al. Citation1989). During the drying process, the hot air flow through the materials promotes the evaporation of moisture and volatile compounds (Orphanides, Goulas, and Gekas Citation2016) and creates a suitable environment for oxidation reactions (Antal Citation2010). Other major drawbacks of hot-air drying are high shrinkage of the products and high energy consumption (Orphanides, Goulas, and Gekas Citation2016). In addition, as hot-air drying is one of the most energy-intensive food processing methods, efforts have focused on reducing the energy consumption, increasing the process efficiency, and reducing the drying time (Won, Min, and Lee Citation2015). In the section below, the effects of hot-air drying parameters on quality degradation of dried herbs will be reviewed.
Effect of air temperature and humidity on the quality of dried herbs
Drying of herbs is recommended to be conducted by hot-air drying at 40–60 °C (Shaw et al. 2016). However, these drying temperatures lead to undesirable changes in aroma of the culinary dried herbs (Antal et al. Citation2011). It has been reported that increasing the drying temperature from 40 to 60 °C resulted in lower content of total volatiles, less fresh-like aroma and increase in spiciness, hay-like, sweet, earthy, and woody flavors in dried basil leaves (Calin-Sanchez et al. Citation2012). Similar results were observed in many types of herbs, such as peppermint (increasing the drying temperatures from 30 to 70 °C) (Rohloff et al. Citation2005), kaffir lime leaves (from 50 to 70 °C) (Jirapakkul, Tinchan, and Chaiseri Citation2013), Achillea fragrantissima (from 35 to 45 °C) (Abaas, Hamzah, and Majeed Citation2013), and sage (from 30 to 60 °C) (Venskutonis Citation1997). Drying temperatures higher than 60 °C result in the loss of most volatile compounds in the dried products in many types of herbs (Allium schoenoprasum L., Anethum graveolens L., Anthriscus cerefolium (L.) Hoffm., Artemisia dracunculus L., Coriandrum sativum L., Levisticum officinale Koch. Mentha spicata L., Origanum majorana L., Petroselinum crispum (Mill.) Nym. ex A. W. Hill, Salvia officinalis L., Satureja hortensis L., and Thymus vulgaris L.) (Deans, Svoboda, and Bartlett Citation1991).
Additionally, increasing the hot-air drying temperature induces many other undesirable changes in the dried products, such as collapse of tissues (Prothon, Ahrne, and Sjoholm Citation2003), loss of bioactive compounds (Tambunan and Yudistira Citation2001), and increased color alteration (Calín-Sánchez et al. Citation2013). In the case of Moringa Oleifera, the color of leaves dried at 40 °C was better preserved in comparison with that from leaves dried at 50 and 60 °C (Ali et al. Citation2014). Structurally, in the case of Vernonia amygdalina, drying the leaves at 60 °C caused significantly higher damage to the epidermal surfaces of the leaves, shrinkage of the trichomes and higher degree of cell wall deformation than drying at 40 and 50 °C (Alara, Abdurahman, and Olalere Citation2019). Increasing the drying temperature also reduced the antioxidant capacity in many types of herbs, namely rosemary (Rosmarinus officinalis), motherwort (Leonurus cardiaca), and peppermint (Mentha piperita) (drying temperatures of 40 and 70 °C were compared) (Yi and Wetzstein Citation2011), meadowsweet (Filipendula ulmaria) and willow (Salix alba) (total phenols, salicylates, and quercetin content were compared at the drying temperatures of 30 and 70 °C) (Harbourne et al. Citation2009). In contrast, some studies report that increasing the drying temperature resulted in higher amounts of certain aroma compounds. This was the case for lemon verbena, in which a higher concentration of the volatile compounds was obtained at the drying temperature of 50 °C in comparison with drying at 30 and 40 °C (Shahhoseini et al. Citation2013). A similar result was observed in thyme leaves dried with hot-air drying at 30, 38 and 45 °C (Piga et al. Citation2007). The positive effect of increasing the drying temperature was also observed for the phytochemical content in the drying of herbal tea (containing several types of herbs including Centella asiatica, Mentha arvensis, and Polygonum minus), showing that the phytochemical content (including chlorophyll, ascorbic acid, niacin, riboflavin, and carotenoids) of the dried tea obtained at 70 °C was higher than that of tea dried at 50 °C (Mahanom, Azizah, and Dzulkifly Citation1999).
Freeze drying
Freeze drying has been suggested by several studies as a suitable drying method for preserving the fresh-like aroma of herbs due to its low operating temperature (Antal Citation2010). This drying process has been extensively reported to produce dried herbs with better aroma compared to other drying methods in many types of herbs such as spearmint, which showed less aroma compound reduction compared to hot-air dried leaves (Antal et al. Citation2011). Similar results were reported in basil leaves when freeze drying was compared to air drying at 50 °C (Di Cesare et al. Citation2003). Freeze drying showed better preservation of the yield and the chemical composition of the essential oil of purple and green basil leaves in comparison with sun drying, shade drying, hot-air drying at 40 and 60 °C, and microwave drying at 500 and 700 W (Pirbalouti, Mahdad, and Craker Citation2013). Similar results were shown for Iranian coriander (Pirbalouti, Salehi, and Craker Citation2017). Freeze-dried thyme resulted in only a 1–3% reduction in the total volatiles content (Venskutonis, Poll, and Larsen Citation1996). Freeze-dried oregano showed better color retention in comparison with air and vacuum-microwave drying (Yousif et al. Citation2000), and freeze-dried Andrographis paniculata leaves showed less shrinkage and higher porosity in comparison with hot-air drying (Tummanichanont, Phoungchandang, and Srzednicki Citation2017). However, comparing to microwave drying, freeze drying was reported to produce lower-quality dried products. In the case of garden thyme (Thymus daenensis), the freeze-dried leaves contained high amounts of essential oils and had good color, yet presented less intense aroma than leaves dried using microwave drying (Rahimmalek and Goli Citation2013). Similar results were observed in basil, for which freeze-dried leaves had lower contents of characteristic volatile compounds than microwave-dried leaves (eucalyptol, linalool, eugenol, and methyl eugenol content were compared). It was also found in the same study that freeze drying caused a greater reduction in chlorophyll pigments of the dried products in comparison with microwave drying (Di Cesare et al. Citation2003). Freeze drying could cause a major loss in the aroma compounds of dried herbs (Calín-Sánchez et al. Citation2013). It has been reported that freeze drying of parsley caused the loss of major volatile components such as p-mentha-1,3,8-triene and apiole (Diaz-Maroto, Perez-Coello, and Cabezudo Citation2002a). Loss of aroma was also observed in freeze-dried sweet basil (Ocimum basilicum L.) using a sensorial panel and, considering the high investment cost of the freeze-drying process, hot-air drying was suggested as the best drying method for drying of sweet basil (Díaz-Maroto et al. Citation2004). Similar results were observed in bay leaf (Diaz-Maroto, Perez-Coello, and Cabezudo Citation2002b).
Freeze drying produces high-quality dried herbs in terms of bioactive compounds in many types of herbs. When freeze-dried and hot-air-dried thyme leaves (Thymus vulgaris) were compared, freeze-dried thyme leaves showed higher yields of thymol compared to those obtained using oven drying at 30-50 °C and shade drying (Sárosi et al. Citation2013). Similar results were observed in freeze-dried rosemary leaves in terms of antioxidants compared to hot-air drying at 45 °C (Ibanez et al. Citation1999) and in freeze-dried spearmint leaves compared to sun drying, shade drying, convective drying and microwave drying (Orphanides, Goulas, and Gekas Citation2013). In contrast, freeze drying caused higher losses of bioactive compounds in Lamiaceae herbs including rosemary, oregano, marjoram, sage, basil, and thyme (hot-air, vacuum, and freeze drying were compared). In the study, freeze-dried samples had lower total contents of phenolic compounds, rosmarinic acid, and antioxidant capacity compared to the other drying methods (Hossain et al. Citation2010).
Microwave drying
Microwave drying is a drying technique which is currently available in herb processing industry (Moses et al. Citation2014; Wray and Ramaswamy Citation2015). It allows rapid evaporation of water from food, providing relatively shorter drying times compared to many drying methods (convective drying, shade and sun drying, freeze drying) (Chi et al. Citation2003) and decreased energy consumption in the drying process (Di Cesare et al. Citation2003). Microwave-dried products showed less shrinkage, better color and rehydration capacity compared to hot-air drying (Kathirvel et al. Citation2006). The quality of microwave-dried products is influenced by drying parameters such as microwave power (W), drying time, the initial moisture content of the product, and the dielectric properties of the materials (Moses et al. Citation2014). Increasing the microwave power from 360 to 900 W reduced the drying time of parsley by 64% and microwave-dried parsley showed good color retention with only slightly darker color than fresh parsley (Soysal Citation2004). Similar results were observed in coriander (Sarimeseli Citation2011), where increasing the microwave power from 180 to 360 W resulted in an increasing diffusivity coefficient while the rehydration capacity of the dried coriander leaves decreased.
In comparative studies, the quality of microwave-dried herbs was higher than that obtained with other drying methods. Microwave-dried basil leaves showed higher retention of volatiles compared to the dried products from convective drying (50 °C) and freeze drying. In the study, the microwave-dried leaves showed fewer color changes in the dried product compared to the convective-dried product. The lower color alteration could be the result of the shorter drying time of microwave drying (Di Cesare et al. Citation2003). A comparison study of microwave drying (with the microwave power of 700 W, 2450 MHz), sun drying, and hot-air drying (at 50 °C) of rosemary leaves showed that the color of microwave-dried rosemary was better than that of hot-air-dried products (Arslan and Özcan Citation2008). A similar result was observed in microwave-dried coriander foliage dried at the microwave power of 295 W, which showed better color retention in comparison with the convective dried sample at 50 °C.
Microwave drying provides high-quality dried products in terms of preserving or enhancing the content of bioactive compounds. Applying microwave drying at 850 W resulted in higher intactness of trans-β-carotene and higher extractability of pigments in dried coriander leaves compared to convective drying at 45 °C (Divya, Puthusseri, and Neelwarne Citation2012). Similar results were observed in microwave-dried sage leaves at the microwave power of 850 W (Hamrouni-Sellami et al. Citation2013), in which the microwave-dried product showed higher retention of total phenolic compounds, flavonoid content and antioxidant activity in comparison with the dried products dried using convective drying at 45 °C. Similar results were observed in Gynura pseudochina leaves (Sukadeetad et al. Citation2018).
Microwaves can be used in combination with other drying methods such as hot-air drying either as a pre-drying stage to reduce the initial moisture content of the materials or as the final stage of drying (Orphanides, Goulas, and Gekas Citation2016). However, the major drawback of microwave drying is the non-uniformity in heating, which results in the formation of temperature gradients in the product, especially in the large-size products, during the drying process. This non-uniform heating could lead to non-uniform dehydration of the product, overheating, and quality degradation (Ozkan, Akbudak, and Akbudak Citation2007). Nevertheless, the interest in microwave drying of herbs has increased in recent years. This is likely because herbs are usually smaller and thinner in size than most other solid foods, and thus non-uniform heating might not be a major drawback for microwave drying of herbs. However, microwave drying for some types of herbs, such as marjoram (Raghavan et al. Citation1997) and rosemary (Rao et al. Citation1998), were reported to cause a greater reduction of aroma compounds in comparison with many drying methods, including convective drying, shade drying, and sun drying. Microwave drying time is much faster than all the compared conventional drying methods. However, for some types of herbs, further studies of microwave drying parameters are needed to optimize the process and improve the quality of the dried products (Moses et al. Citation2014).
Microwave-vacuum drying
The combination of microwave and vacuum drying has recently gained attention (Orphanides, Goulas, and Gekas Citation2016). The process is conducted by using microwave irradiation as the heating source to increase the temperature of the food materials in the sub-atmospheric pressure drying chamber. The vacuum creates the driving force of water evaporation, resulting in faster drying rates in comparison with convective drying and microwave drying (Soysal Citation2004). Compared to hot-air drying, microwave-vacuum drying could reduce the drying time by 70–90% and also produce better-quality products (Giri and Prasad Citation2007). The level of thymol in vacuum-microwave dried L. berlandieri was 1.3 times higher than those dried using air drying (Yousif et al. Citation2000). The microwave-vacuum drying of mint leaves resulted in better color retention of dried products compared to hot-air drying. SEM images of these microwave-vacuum dried products showed more porosity and less collapse compared to hot-air-dried samples (Therdthai and Zhou Citation2009). However, the major limitation of the vacuum-drying process is the capacity of the vacuum pump. With the high load of initial moisture from food materials, the vacuum pump may exceed its capacity quickly, resulting in a less efficient process.
In contrast, microwave-vacuum drying has been reported to reduce the dried product quality in some types of herbs, such as rosemary. Microwave-vacuum drying resulted in a higher loss of volatile compounds of dried rosemary in comparison with hot-air drying, and with the combination of hot-air drying and microwave-vacuum drying (Szumny et al. Citation2010). The microwave-vacuum-dried rosemary contained fewer volatile compounds and lower sensory quality in comparison with hot-air drying at 60 °C. In their study, the authors suggested that microwave-vacuum drying was a “not suitable” drying method for rosemary. However, in the same study, the combination of hot-air drying and microwave-vacuum drying (called convective pre-drying and vacuum-microwave finish-drying (CPD-VMFD) was reported to provide the highest concentration of volatile compounds in the dried products. To sum up, microwave-vacuum drying is a promising combined drying method with good potential to become a suitable method for drying herbs. However, further optimization studies need to be done.
Heat-pump-assisted drying
Heat-pump drying is another drying technique development aimed at increasing the efficiency of traditional convective drying. A heat pump is usually coupled with another air-drying unit to increase the initial input air temperature. The system could be called “heat pump dryer or heat pump-assisted dryer” (Fatouh et al. Citation2006). The heat pump dryer is suitable for industrial herb drying as it can be operated in wide ranges of air velocity and drying temperatures (Fatouh et al. Citation2006). Another major benefit of heat pump dryers is their ability to dehumidify the outlet air of the drying unit. The dehumidifying effect occurs when the temperature of the evaporator is lower than the dew point of the air at the evaporator inlet (Fatouh et al. Citation2006). Heat-pump drying could provide better-quality dried products due to its ability to control the properties of the air during the process. Heat-pump solar drying of java tea (Orthosiphon aristatus) showed better controllability of the relative humidity of the drying room in comparison with regular solar greenhouse dryers, especially during the nighttime. The dehumidifying system reduced the relative humidity of the drying room by 10–15% and was able to maintain the maximum relative humidity of 65%. Moreover, the drying rate of the heat-pump-integrated solar greenhouse was 3-4 times better than that of a regular greenhouse dryer (Tham et al. Citation2017).
The focus of recent studies has been the heat-pump drying of medical herbs, in which the content of bioactive compounds in the dried products has been investigated (Gan et al. Citation2017; Klungboonkrong, Phoungchandang, and Lamsal Citation2018; Tummanichanont, Phoungchandang, and Srzednicki Citation2017). Most of the studies reported that heat-pump dryers produced better-quality dried products in terms of preserving bioactive compounds in comparison with other drying methods, such as in the case of misai kucing (compared with solar drying) (Torki-Harchegani et al. Citation2017) and Andrographis paniculata (compared with hot-air drying, microwave drying and freeze drying) (Tummanichanont, Phoungchandang, and Srzednicki Citation2017). When the effect of heat-pump drying on the quality of Andrographis paniculata was investigated, it was found that the heat-pump drying (with dehumidifier function, called heat pump dehumidifier dryer (HPD)) at 40, 50 and 60 °C resulted in higher amounts of bioactive compounds, including andrographolide, neoandrographolide and total phenolics, in comparison with hot-air-dried samples at the same drying temperatures. In the same study, heat-pump drying was shown to be better at maintaining the original shape of parenchyma cell structures of the dried products compared to air drying. A comparison study on the effect of heat-pump drying (using heat pump dehumidify dryer; HPD), convective drying and freeze drying on the quality of java tea, found that heat-pump-dried java tea at the drying temperature of 60 °C showed good retention of total phenolic content and antioxidant activity of the dried products similar to freeze-dried products (Klungboonkrong, Phoungchandang, and Lamsal Citation2018). Additionally, the HPD system reduced the drying time by 44.8% compared to convective drying at the same drying temperature. Moreover, the microstructure of the products dried using HPD showed fuller and more regular cell structure than the convective dried product. Overall, heat-pump drying provided promising results for improving the content of bioactive compounds and the structural properties of the dried products. However, further studies of this drying technique in culinary herbs are needed to test the effect of the process on their aroma.
Infrared drying
The major advantages of this drying process are the adaptability, simplicity, fast heating rate, and fast drying rate (Ashtiani, Salarikia, and Golzarian Citation2017). During the process, the electromagnetic energy from infrared wavelength radiation is transmitted and absorbed by the material generating heat from inside of the materials due to the changes of molecular vibrational state (Krishnamurthy et al. Citation2008). Infrared drying has higher energy efficiency compared to hot-air drying. However, only a few studies of herb drying using infrared have been conducted in recent years. When drying mint leaves, the energy efficiency and drying rate of infrared drying were higher than during convective drying (drying temperatures of 30, 40, 50 °C were compared) (Ashtiani, Salarikia, and Golzarian Citation2017). Increasing the infrared drying temperature resulted in higher crocin and safranal content of dried saffron (Torki-Harchegani et al. Citation2017). These compounds are the main chemical compounds contributing to dried saffron quality. Infrared irradiation is suitable for thin-layer drying due to its short traveling distance in the materials and the dependency of the contacted area on the materials. Moreover, the fast drying rate of infrared drying (compared to hot-air drying) (Ashtiani, Salarikia, and Golzarian Citation2017; Torki-Harchegani et al. Citation2017) and the ability to maintain high drying rate at lower moisture content (Pääkkönen, Havento, and Galambosi Citation1999) would make infrared drying a promising alternative drying method for herbs. However, Chua et al. (Citation2019) reported that the non-uniform heating of infrared leads to the degradation of the aroma quality in dried herbs.
Fluidized bed drying
Fluidized bed drying has been implemented in the food industry for many types of agricultural products, including herbal leaves (Gangopadhyay and Chaudhuri Citation1979). The process is carried out by passing high-velocity hot air (high enough to create fluidization of the products) to the drying bed where the products are placed. The drying rate of this method is much higher than traditional convective drying due to the higher heating rate of the fluidization heating. For fluidized bed drying of lemon myrtle leaves, increasing the drying temperature (drying temperatures of 30, 40, and 50 °C were compared) resulted in higher retention of citral content (which contributes the “citrus” aroma) of the dried product (Buchaillot, Caffin, and Bhandari Citation2009). However, the lowest tested drying temperature (30 °C) showed better color retention, and the highest drying temperature (50 °C) showed unacceptable color quality degradation.
Herbs may not be suitable for fluidization drying due to their high moisture content, large surface area to volume ratio, and rough surfaces, which could lead to poor air percolation. To overcome this problem, vibrofluidized bed drying has been developed (de Aquino Brito Lima-Corrêa et al. Citation2017). The vibrofluidized drying process is a type of fluidized bed dryer, which is attached with a vibrator module to enhance the performance of the fluidized bed dryer. Vibrofluidized bed drying achieved the requirement of moisture reduction and moisture homogeneity of dried basil leaves while conventional fluidized bed drying did not. However, the loss of eugenol content of the dried product was observed with drying temperatures of 45 and 60 °C.
Supercritical CO2 drying (scCO2)
This process uses supercritical carbon dioxide as a drying medium. The major advantages of this drying technique are mild operating temperature (usually close to ambient temperature), low or non-presence of oxygen, low product shrinkage, and better rehydration capacity of dried products. Only a few studies of scCO2 drying of herbs have been conducted. CO2 drying of basil was reported (Busic et al. Citation2014) in comparison with other drying techniques including convective drying (40 °C for 26 h) and freeze drying (−20 °C at 0.005 bar for 4 days). The results showed that the best quality of dried basil was achieved by freeze drying, followed by scCO2 drying, while convective drying showed the worst dried product quality considering the preservation of color, bioactive compounds, and the fresh-like characteristic properties. However, it was suggested that scCO2 drying was the most suitable drying process among the three studied drying methods due to the acceptable quality of the dried herbs, and drastically shorter drying time (2–3 h) compared to freeze drying (4 days) and air drying (26 h). Another study of scCO2 drying of herbs was conducted in combination with ultrasound pretreatment in coriander leaves (Michelino et al. Citation2018). The results showed that scCO2 drying provided good inactivation of microorganisms. According to the results, yeast, molds and mesophilic bacteria were reduced by 4 Log during the drying process. However, the analysis of sensory and chemical properties of dried products was not reported in the study.
Radio-frequency drying
Radio-frequency (RF) drying combines the utilization of radio frequency heating and convective drying. Radio frequency heating relies on the dielectric properties of the food materials, similar to microwave heating, but with differences in wave frequencies (Nijhuis et al. Citation1998). Radio-frequency heating could help increase the drying rate, especially during the falling rate period where the conventional convective drying encounters its limitation (Thomas Citation1996). To the best of our knowledge, there is only one RF drying study of herbs. The effect of RF drying with infrared was compared with convective drying on the quality of dill greens (Naidu et al. Citation2016). RF drying showed faster drying rates than convective drying at 50 °C. However, the RF-dried dill greens showed the lowest bioactive compound content (including chlorophylls a and b, carotenoids and ascorbic acid) in comparison with the dried products from convective drying (50 °C, with 58–63% RH and 28–30% RH) and infrared drying. According to the results, RF drying might not be a suitable drying method for herbs considering the degradation of chlorophyll and resulting color changes.
Hybrid drying methods
Hybrid drying methods are the combination of two or more drying techniques to overcome the problem of single stage drying. In this paper, we have reviewed heat pump drying, solar assisted drying, microwave-vacuum drying, and radio-frequency drying. These drying techniques have recently gained attention from researchers due to their ability to shorten processing time, minimize quality degradation and maintain the process efficiency (Chou, and Chua Citation2001). Currently, the three methods that have received the most attention are probably solar-assisted drying, microwave-assisted drying, and heat pump-assisted drying (Chou, and Chua Citation2001; Jin et al. Citation2018). However, the information on the effects of these hybrid technologies on the quality of dried herbs is limited.
Conclusions
Improving the quality characteristics of dried herbs has been the main subject of many studies on drying and pre-drying methods for the past 20 years. A number of pre-drying treatments and drying methods, investigated in different herbs, have been developed, showing an improvement in quality, better energy conservation, and better process efficiency. Hybrid-drying techniques have shown promising results on the improvement of dried herbs quality including both color and aroma. In spite of these technological developments, obtaining high-quality dried herbs is still an issue as herbs are highly sensitive to different pre-drying and drying process conditions, mainly in regard to color and aroma. Moreover, the quality of dried herbs is very sensitive to the type of herb, harvesting season, postharvest practices, age of the plant and storage conditions. Therefore, optimization of quality requires studying each specific pre-drying and drying method for each type of herb.
Author contributions
G. Thamkaew wrote the manuscript, created figures and tables. I. Sjöholm and F. Gómez Galindo supervised and revised the manuscript.
Acknowledgements
This study was supported by grants from the Royal Thai Government, Ministry of Science and Technology of Thailand.
Disclosure statement
No potential conflict of interest was reported by the authors.
References
- Abaas, I., M. Hamzah, and A. Majeed. 2013. Analysis with evaluation of drying temperature on essential oil content of achillea frayrantissima l. And artemisia herb-alba l. International Journal of Pharmacy and Pharmaceutical Sciences 5 (3):913–4.
- Abdullah, S., A.R. Shaari and A. Azimi. 2012. Effect of drying methods on metabolites composition of misai kucing (Orthosiphon stamineus) leaves. 3rd International Conference on Biotechnology and Food Science (Icbfs) 2:178–82. doi: 10.1016/j.apcbee.2012.06.032.
- Ahmed, J., U. S. Shivhare, and G. Singh. 2001. Drying characteristics and product quality of coriander leaves. Food and Bioproducts Processing 79 (2):103–6. doi: 10.1205/096030801750286258.
- Aktaş, M., A. Khanlari, B. Aktekeli, and A. Amini. 2017. Analysis of a new drying chamber for heat pump mint leaves dryer. International Journal of Hydrogen Energy 42 (28):18034–44. doi: 10.1016/j.ijhydene.2017.03.007.
- Alara, O. R., N. H. Abdurahman, S. K. Abdul Mudalip, and O. A. Olalere. 2018. Mathematical modeling of thin layer drying using open sun and shade of Vernonia amygdalina leaves. Agriculture and Natural Resources 52 (1):53–8. doi: 10.1016/j.anres.2018.05.013.
- Alara, O. R., N. H. Abdurahman, and O. A. Olalere. 2019. Mathematical modelling and morphological properties of thin layer oven drying of Vernonia amygdalina leaves. Journal of the Saudi Society of Agricultural Sciences 18 (3):309–15. doi: 10.1016/j.jssas.2017.09.003.
- Ali, M. A., Y. A. Yusof, N. L. Chin, M. N. Ibrahim, and S. M. A. Basra. 2014. Drying kinetics and colour analysis of Moringa oleifera leaves. Agriculture and Agricultural Science Procedia. 2nd International Conference on Agricultural and Food Engineering (Cafe 2014) - New Trends Forward 2:394–400. doi: 10.1016/j.aaspro.2014.11.055.
- Alibas, I. 2007. Energy consumption and colour characteristics of nettle leaves during microwave, vacuum and convective drying. Biosystems Engineering 96 (4):495–502. doi: 10.1016/j.biosystemseng.2006.12.011.
- Amer, B. M. A., K. Gottschalk, and M. A. Hossain. 2018. Integrated hybrid solar drying system and its drying kinetics of chamomile. Renewable Energy 121:539–47. doi: 10.1016/j.renene.2018.01.055.
- Antal, T. 2010. Inspection of the technological characteristics influencing the quality of dried fruits and vegetables. PhD diss., University of Debrecen, Debrecen, Hungary.
- Antal, T., A. Figiel, B. Kerekes, and L. Sikolya. 2011. Effect of drying methods on the quality of the essential oil of spearmint leaves (Mentha spicatal). Drying Technology 29 (15):1836–44. doi: 10.1080/07373937.2011.606519.
- Arabhosseini, A., S. Padhye, T. A. Van Beek, A. J. B. Van Boxtel, W. Huisman, M. A. Posthumus, and J. Muller. 2006. Loss of essential oil of tarragon (Artemisia dracunculus l.) due to drying. Journal of the Science of Food and Agriculture 86 (15):2543–50. doi: 10.1002/jsfa.2641.
- Argyropoulos, D., and J. Muller. 2014. Changes of essential oil content and composition during convective drying of lemon balm (Melissa officinalis l.). Industrial Crops and Products 52:118–24. doi: 10.1016/j.indcrop.2013.10.020.
- Arslan, D., and M. M. Özcan. 2008. Evaluation of drying methods with respect to drying kinetics, mineral content and colour characteristics of rosemary leaves. Energy Conversion and Management 49 (5):1258–64. doi: 10.1016/j.enconman.2007.08.005.
- Arslan, D., and M. M. Özcan. 2012. Evaluation of drying methods with respect to drying kinetics, mineral content, and color characteristics of savory leaves. Food and Bioprocess Technology 5 (3):983–91. doi: 10.1007/s11947-010-0498-y.
- Arslan, D., M. M. Özcan, and H. O. Mengeş. 2010. Evaluation of drying methods with respect to drying parameters, some nutritional and colour characteristics of peppermint (Mentha x piperita l). Energy Conversion and Management 51 (12):2769–75. doi: 10.1016/j.enconman.2010.06.013.
- Arslan, D., M. M. Özcan, and A. Unver. 2005. Effect of drying methods on the mineral content of basil (Ocimum basilicum l.). Journal of Food Engineering 69 (3):375–9. doi: 10.1016/j.jfoodeng.2004.08.030.
- Artnaseaw, A., S. Theerakulpisut, and C. Benjapiyaporn. 2010. Thin layer modeling of tom yum herbs in vacuum heat pump dryer. Food Science and Technology International = Ciencia y tecnologia de los alimentos internacional 16 (2):135–46. doi: 10.1177/1082013209353090.
- Ascrizzi, R., D. Fraternale, and G. Flamini. 2018. Photochemical response of parsley (Petroselinum crispum (mill.) fuss) grown under red light: The effect on the essential oil composition and yield. Journal of Photochemistry and Photobiology B: Biology 185:185–91. doi: 10.1016/j.jphotobiol.2018.06.006.
- Asekun, O. T., D. S. Grierson, and A. J. Afolayan. 2007. Effects of drying methods on the quality and quantity of the essential oil of Mentha longifolia l. Subsp capensis. Food Chemistry 101 (3):995–8. doi: 10.1016/j.foodchem.2006.02.052.
- Ashtiani, S.-H. M., A. Salarikia, and M. R. Golzarian. 2017. Analyzing drying characteristics and modeling of thin layers of peppermint leaves under hot-air and infrared treatments. Information Processing in Agriculture 4 (2):128–39. doi: 10.1016/j.inpa.2017.03.001.
- Baritaux, O., H. Richard, J. Touche, and M. Derbesy. 1992. Effects of drying and storage of herbs and spices on the essential oil. Part i. Basil, Ocimum basilicum l. Flavour and Fragrance Journal 7 (5):267–71. doi: 10.1002/ffj.2730070507.
- Başer, K. H. C., and F. Demirci. 2011. Essential oils. In Kirk-othmer Encyclopedia of Chemical Technology (1–37). https://onlinelibrary.wiley.com/doi/abs/10.1002/0471238961.1509121913151511.a01.pub2.
- Boggia, R., R. Leardi, P. Zunin, A. Bottino, and G. Capannelli. 2013. Dehydration of pdo genovese basil leaves (Ocimum basilicum maximum l. Cv genovese gigante) by direct osmosis. Journal of Food Processing and Preservation 37 (5):621–9. doi: 10.1111/j.1745-4549.2012.00682.x.
- Bor, T., S. O. Aljaloud, R. Gyawali, and S. A. Ibrahim. 2016. Antimicrobials from herbs, spices, and plants. In Fruits, vegetables, and herbs, ed. R. R. Watson and V. R. Preedy, 551–78. London, UK: Academic Press.
- Buchaillot, A., N. Caffin, and B. Bhandari. 2009. Drying of lemon myrtle (Backhousia citriodora) leaves: Retention of volatiles and color. Drying Technology 27 (3):445–50. doi: 10.1080/07373930802683740.
- Busic, A., A. Vojvodic, D. Komes, C. Akkermans, A. Belscak-Cvitanovic, M. Stolk, and G. Hofland. 2014. Comparative evaluation of Co2 drying as an alternative drying technique of basil (Ocimum basilicum l.) – The effect on bioactive and sensory properties. Food Research International 64:34–42. doi: 10.1016/j.foodres.2014.06.013.
- Calín-Sánchez, Á., A. Figiel, K. Lech, A. Szumny, and Á. A. Carbonell-Barrachina. 2013. Effects of drying methods on the composition of thyme (Thymus vulgarisl.) essential oil. Drying Technology 31 (2):224–35. doi: 10.1080/07373937.2012.725686.
- Calin-Sanchez, A., K. Lech, A. Szumny, A. Figiel, and A. A. Carbonell-Barrachina. 2012. Volatile composition of sweet basil essential oil (Ocimum basilicum l.) as affected by drying method. Food Research International 48 (1):217–25. doi: 10.1016/j.foodres.2012.03.015.
- Calín-Sánchez, Á., A. Szumny, A. Figiel, K. Jałoszyński, M. Adamski, and Á. A. Carbonell-Barrachina. 2011. Effects of vacuum level and microwave power on rosemary volatile composition during vacuum–microwave drying. Journal of Food Engineering 103 (2):219–27. doi: 10.1016/j.jfoodeng.2010.10.018.
- Calvo-Irabien, L. M., J. A. Yam-Puc, G. Dzib, F. Escalante-Erosa, and L. M. Peña-Rodriguez. 2009. Effect of postharvest drying on the composition of Mexican oregano (Lippia graveolens) essential oil. Journal of Herbs, Spices & Medicinal Plants 15 (3):281–7. doi: 10.1080/10496470903379001.
- Capecka, E., A. Mareczek, and M. Leja. 2005. Antioxidant activity of fresh and dry herbs of some lamiaceae species. Food Chemistry 93 (2):223–6. doi: 10.1016/j.foodchem.2004.09.020.
- Ceylan, I., and A. E. Gurel. 2016. Solar-assisted fluidized bed dryer integrated with a heat pump for mint leaves. Applied Thermal Engineering 106:899–905. doi: 10.1016/j.applthermaleng.2016.06.077.
- Chen, L. J., E. H. Lebetkin, and L. T. Burka. 2001. Metabolism of (r)-(+)-pulegone in f344 rats. Drug Metabolism and Disposition 29 (12):1567–77. doi: 10.1124/dmd.31.7.892.
- Chen, W. Q., K. F. Poon, and M. H. W. Lam. 1998. The application of solid phase microextraction in the analysis of organophosphorus pesticides in a food plant. Environmental Science & Technology 32 (23):3816–20. doi: 10.1021/es980294c.
- Chi, J.-W., Z.-C. Wei, Z.-H. Xu, and Y. Zhang. 2003. Application and development of microwave techniques in food processing. Storage and Process 1:003.
- Choe, E., and D. B. Min. 2006. Mechanisms and factors for edible oil oxidation. Comprehensive Reviews in Food Science and Food Safety 5 (4):169–86. doi: 10.1111/j.1541-4337.2006.00009.x.
- Chou, S. K., and K. J. Chua. 2001. New hybrid drying technologies for heat sensitive foodstuffs. Trends in Food Science & Technology 12 (10):359–69. doi: 10.1016/S0924-2244(01)00102-9.
- Chua, L. Y. W., C. H. Chong, B. L. Chua, and A. Figiel. 2019. Influence of drying methods on the antibacterial, antioxidant and essential oil volatile composition of herbs: A review. Food and Bioprocess Technology 12 (3):450–76. doi: 10.1007/s11947-018-2227-x.
- Dadan, M., K. Rybak, A. Wiktor, M. Nowacka, J. Zubernik, and D. Witrowa-Rajchert. 2018. Selected chemical composition changes in microwave-convective dried parsley leaves affected by ultrasound and steaming pre-treatments - An optimization approach. Food Chemistry 239:242–51. doi: 10.1016/j.foodchem.2017.06.061.
- De Aquino Brito Lima-Corrêa, R., M. Dos Santos Andrade, M. F. D. G. F. Da Silva, J. T. Freire, and M. D. C. Ferreira. 2017. Thin-layer and vibrofluidized drying of basil leaves (Ocimum basilicum l.): Analysis of drying homogeneity and influence of drying conditions on the composition of essential oil and leaf colour. Journal of Applied Research on Medicinal and Aromatic Plants 7:54–63. doi: 10.1016/j.jarmap.2017.05.001.
- De La Fuente-Blanco, S., E. Riera-Franco De Sarabia, V. M. Acosta-Aparicio, A. Blanco-Blanco, and J. A. Gallego-Juarez. 2006. Food drying process by power ultrasound. Ultrasonics 44:e523–7. doi: 10.1016/j.ultras.2006.05.181.
- Deans, S. G., K. P. Svoboda, and M. C. Bartlett. 1991. Effect of microwave oven and warm-air drying on the microflora and volatile oil profile of culinary herbs. Journal of Essential Oil Research 3 (5):341–7. doi: 10.1080/10412905.1991.9697954.
- Demir, V., T. Gunhan, A. K. Yagcioglu, and A. Degirmencioglu. 2004. Mathematical modelling and the determination of some quality parameters of air-dried bay leaves. Biosystems Engineering 88 (3):325–35. doi: 10.1016/j.biosystemseng.2004.04.005.
- Deng, L.-Z., A. S. Mujumdar, Q. Zhang, X.-H. Yang, J. Wang, Z.-A. Zheng, Z.-J. Gao, and H.-W. Xiao. 2019. Chemical and physical pretreatments of fruits and vegetables: Effects on drying characteristics and quality attributes – A comprehensive review. Critical Reviews in Food Science and Nutrition 59 (9):1408–32. doi: 10.1080/10408398.2017.1409192.
- Di Cesare, L. F., E. Forni, D. Viscardi, and R. C. Nani. 2003. Changes in the chemical composition of basil caused by different drying procedures. Journal of Agricultural and Food Chemistry 51 (12):3575–81. doi: 10.1021/jf021080o.
- Di Cesare, L. F., E. Forni, D. Viscardi, and R. C. Nani. 2004. Influence of drying techniques on the volatile phenolic compounds, chlorophyll and colour of oregano (Origanum vulgare l.). Ssp. Prismaticum gaudin). Italian Journal of Food Science 16 (2):165–175.
- Diaz-Maroto, M. C., M. S. Perez-Coello, and M. D. Cabezudo. 2002a. Effect of different drying methods on the volatile components of parsley (Petroselinum crispum l.). European Food Research and Technology 215 (3):227–30. doi: 10.1007/s00217-002-0529-7.
- Diaz-Maroto, M. C., M. S. Perez-Coello, and M. D. Cabezudo. 2002b. Effect of drying method on the volatiles in bay leaf (Laurus nobilis l.). Journal of Agricultural and Food Chemistry 50 (16):4520–4. doi: 10.1021/jf011573d.
- Díaz-Maroto, M. C., E. Sánchez Palomo, L. Castro, M. A. González Viñas, and M. S. Pérez-Coello. 2004. Changes produced in the aroma compounds and structural integrity of basil (Ocimum basilicuml) during drying. Journal of the Science of Food and Agriculture 84 (15):2070–6. doi: 10.1002/jsfa.1921.
- Divya, P., B. Puthusseri, and B. Neelwarne. 2012. Carotenoid content, its stability during drying and the antioxidant activity of commercial coriander (Coriandrum sativum l.) varieties. Food Research International 45 (1):342–50. doi: 10.1016/j.foodres.2011.09.021.
- Dokhani, S., T. Cottrell, J. Khajeddin, and G. Mazza. 2005. Analysis of aroma and phenolic components of selected achillea species. Plant Foods for Human Nutrition 60 (2):55–62. doi: 10.1007/s11130-005-5100-9.
- Doymaz, İ. 2006. Thin-layer drying behaviour of mint leaves. Journal of Food Engineering 74 (3):370–5. İ. doi: 10.1016/j.jfoodeng.2005.03.009.
- Doymaz, İ. 2014. Thin-layer drying of bay laurel leaves (Laurus nobilis l.). Journal of Food Processing and Preservation 38 (1):449–56. doi: 10.1111/j.1745-4549.2012.00793.x.
- Doymaz, İ., N. Tugrul, and M. Pala. 2006. Drying characteristics of dill and parsley leaves. Journal of Food Engineering 77 (3):559–65. doi: 10.1016/j.jfoodeng.2005.06.070.
- Ebadi, M. T., M. Azizi, F. Sefidkon, and N. Ahmadi. 2015. Influence of different drying methods on drying period, essential oil content and composition of Lippia citriodora kunth. Journal of Applied Research on Medicinal and Aromatic Plants 2 (4):182–7. doi: 10.1016/j.jarmap.2015.06.001.
- El-Sebaii, A. A., and S. M. Shalaby. 2013. Experimental investigation of an indirect-mode forced convection solar dryer for drying thymus and mint. Energy Conversion and Management 74:109–16. doi: 10.1016/j.enconman.2013.05.006.
- Embuscado, M. E. 2015. Herbs and spices as antioxidants for food preservation. In Handbook of antioxidants for food preservation, ed. F. Shahidi, 251–83. Cambridge, UK: Woodhead Publishing.
- Eskin, M. N. A. 1990. Biochemical changes in raw foods: fruits and vegetables. Biochemistry of foods, 70–78. Toronto, ON: Academic Press.
- Fatouh, M., M. N. Metwally, A. B. Helali, and M. H. Shedid. 2006. Herbs drying using a heat pump dryer. Energy Conversion and Management 47 (15-16):2629–43. doi: 10.1016/j.enconman.2005.10.022.
- Fennell, C. W., M. E. Light, S. G. Sparg, G. I. Stafford, and J. Van Staden. 2004. Assessing African medicinal plants for efficacy and safety: Agricultural and storage practices. Journal of Ethnopharmacology 95 (2-3):113–21. doi: 10.1016/j.jep.2004.05.025.
- Fernandes, FaN, and S. Rodrigues. 2007. Ultrasound as pre-treatment for drying of fruits: Dehydration of banana. Journal of Food Engineering 82 (2):261–7. doi: 10.1016/j.jfoodeng.2007.02.032.
- Figiel, A., and A. Michalska. 2016. Overall quality of fruits and vegetables products affected by the drying processes with the assistance of vacuum-microwaves. International Journal of Molecular Sciences 18 (1).71. doi: 10.3390/ijms180100:.
- Figiel, A., A. Szumny, A. Gutierrez-Ortiz, and A. A. Carbonell-Barrachina. 2010. Composition of oregano essential oil (Origanum vulgare) as affected by drying method. Journal of Food Engineering 98 (2):240–7. doi: 10.1016/j.jfoodeng.2010.01.002.
- Gamboa-Santos, J., A. Montilla, J. A. Carcel, M. Villamiel, and J. V. Garcia-Perez. 2014. Air-borne ultrasound application in the convective drying of strawberry. Journal of Food Engineering 128:132–9. doi: 10.1016/j.jfoodeng.2013.12.021.
- Gan, S. H., M. X. Ng, T. C. Tham, L. S. Chua, R. Aziz, M. R. Baba, L. C. Abdullah, S. P. Ong, and C. L. Law. 2017. Drying characteristics of Orthosiphon stamineus benth by solar-assisted heat pump drying. Drying Technology 35 (14):1755–64. doi: 10.1080/07373937.2016.1275673.
- Gangopadhyay, H., and D. R. Chaudhuri. 1979. Comparative studies on dehydration of peas in fluidized-bed and conventional tray drier. Journal of Food Science and Technology-Mysore 16 (5):206–7.
- Garcia-Perez, J. V., J. A. Carcel, J. Benedito, and A. Mulet. 2007. Power ultrasound mass transfer enhancement in food drying. Food and Bioproducts Processing 85 (3):247–54. doi: 10.1205/fbp07010.
- Gardeli, C., V. Evageliou, C. Poulos, S. Yanniotis, and M. Komaitis. 2010. Drying of fennel plants: Oven, freeze drying, effect of freeze-drying time, and use of biopolymers. Drying Technology 28 (4):542–9. doi: 10.1080/07373931003622321.
- Giri, S. K., and S. Prasad. 2007. Drying kinetics and rehydration characteristics of microwave-vacuum and convective hot-air dried mushrooms. Journal of Food Engineering 78 (2):512–21. doi: 10.1016/j.jfoodeng.2005.10.021.
- Grosch, W. 2001. Evaluation of the key odorants of foods by dilution experiments, aroma models and omission. Chemical Senses 26 (5):533–45. doi: 10.1093/chemse/26.5.533.
- Hamrouni-Sellami, I., F. Z. Rahali, I. B. Rebey, S. Bourgou, F. Limam, and B. Marzouk. 2013. Total phenolics, flavonoids, and antioxidant activity of sage (Salvia officinalis l.) plants as affected by different drying methods. Food and Bioprocess Technology 6 (3):806–17. doi: 10.1007/s11947-012-0877-7.
- Hanaa, A. M., Y. Sallam, A. El-Leithy, and S. E. Aly. 2012. Lemongrass (Cymbopogon citratus) essential oil as affected by drying methods. Annals of Agricultural Sciences 57 (2):113–6. doi: 10.1016/j.aoas.2012.08.004.
- Harbourne, N., E. Marete, J. C. Jacquier, and D. O'Riordan. 2009. Effect of drying methods on the phenolic constituents of meadowsweet (Filipendula ulmaria) and willow (Salix alba). Lwt - Food Science and Technology 42 (9):1468–73. doi: 10.1016/j.lwt.2009.05.005.
- Hassanpouraghdam, M. B., A. Hassani, L. Vojodi, and N. Farsad-Akhtar. 2010. Drying method affects essential oil content and composition of basil (Ocimum basilicuml.). Journal of Essential Oil Bearing Plants 13 (6):759–66. doi: 10.1080/0972060X.2010.10643892.
- Heindl, A. G., and J. Müller. 2007. Microwave drying of medicinal and aromatic plants. Stewart Postharvest Review 4 (3):1–6.
- Hossain, M. B., C. Barry-Ryan, A. B. Martin-Diana, and N. P. Brunton. 2010. Effect of drying method on the antioxidant capacity of six lamiaceae herbs. Food Chemistry 123 (1):85–91. doi: 10.1016/j.foodchem.2010.04.003.
- Huang, H., Q. Wang, T. Du, C. Lin, Y. Lai, D. Zhu, W. Wu, X. Ma, S. Bai, Z. Li, et al. 2018. Matrine inhibits the progression of prostate cancer by promoting expression of gadd45b. The Prostate 78 (5):327–35. doi: 10.1002/pros.23469.
- Hui Gan, S., T. Chai Tham, M. Xiang Ng, L. Suan Chua, R. Aziz, M. Redza Baba, L. Chuah Abdullah, S. Pheng Ong, and C. L. Law. 2017. Study on retention of metabolites composition in misai kucing (Orthosiphon stamineus) by heat pump assisted solar drying. Journal of Food Processing and Preservation 41 (6):e13262. doi: 10.1111/jfpp.13262.
- Humphrey, A. J., and M. H. Beale. 2006. Terpenes. In Plant secondary metabolites: Occurrence, structure and role in the human diet, 47–101. Oxford, UK: Blackwell Publishing.
- Huopalahti, R., E. Kesalahti, and R. Linko. 1985. Effect of hot air and freeze-drying on the volatile compounds of dill (Anethum-graveolens l) herb. Agricultural and Food Science 57 (2):133–8. doi: 10.23986/afsci.72194.
- Ibanez, E., A. Oca, G. De Murga, S. Lopez-Sebastian, J. Tabera, and G. Reglero. 1999. Supercritical fluid extraction and fractionation of different preprocessed rosemary plants. Journal of Agricultural and Food Chemistry 47 (4):1400–4. doi: 10.1021/jf980982f.
- Janjai, S., and B. K. Bala. 2012. Solar drying technology. Food Engineering Reviews 4 (1):16–54. doi: 10.1007/s12393-011-9044-6.
- Janjai, S., and P. Tung. 2005. Performance of a solar dryer using hot air from roof-integrated solar collectors for drying herbs and spices. Renewable Energy 30 (14):2085–95. doi: 10.1016/j.renene.2005.02.006.
- Jin, W., A. S. Mujumdar, M. Zhang, and W. Shi. 2018. Novel drying techniques for spices and herbs: A review. Food Engineering Reviews 10 (1):34–45. doi: 10.1007/s12393-017-9165-7.
- Jirapakkul, W., P. Tinchan, and S. Chaiseri. 2013. Effect of drying temperature on key odourants in kaffir lime (Citrus hystrixd C., rutaceae) leaves. International Journal of Food Science & Technology 48 (1):143–9. doi: 10.1111/j.1365-2621.2012.03170.x.
- Kathirvel, K.,. K. R. Naik, Y. Gariepy, V. Orsat, and G. Raghavan. 2006. Microwave drying-a promising alternative for the herb processing industry. In 2006 ASAE Annual Meeting, 1: American Society of Agricultural and Biological Engineers.
- Khan, M. K., K. Ahmad, S. Hassan, M. Imran, N. Ahmad, and C. M. Xu. 2018. Effect of novel technologies on polyphenols during food processing. Innovative Food Science & Emerging Technologies 45:361–81. doi: 10.1016/j.ifset.2017.12.006.
- Khangholil, S., and A. Rezaeinodehi. 2008. Effect of drying temperature on essential oil content and composition of sweet wormwood (Artemisia annua) growing wild in iran. Pakistan Journal of Biological Sciences: PJBS 11 (6):934 7.7. doi: 10.3923/pjbs.2008.934.
- Khorshidi, J., R. Mohammadi, T. Fakhr, and H. Nourbakhsh. 2009. Influence of drying methods, extraction time, and organ type on essential oil content of rosemary (Rosmarinus officinalis l.). Natural Science 7 (11):42–4.
- Kim, S., J. Chen, T. Cheng, A. Gindulyte, J. He, S. He, Q. Li, B. A. Shoemaker, P. A. Thiessen, B. Yu, et al. 2019. Pubchem 2019 update: Improved access to chemical data. Nucleic Acids Research 47 (D1):D1102–D1109. D09. doi: 10.1093/nar/gky1033.
- Klungboonkrong, V., S. Phoungchandang, and B. Lamsal. 2018. Drying of Orthosiphon aristatus leaves: Mathematical modeling, drying characteristics, and quality aspects. Chemical Engineering Communications 205 (9):1239–51. doi: 10.1080/00986445.2018.1443080.
- Kowalski, S. J., and A. Rybicki. 2017. Ultrasound in wet biological materials subjected to drying. Journal of Food Engineering 212:271–82. doi: 10.1016/j.jfoodeng.2017.05.032.
- Krishnamurthy, K., H. K. Khurana, S. Jun, J. Irudayaraj, and A. Demirci. 2008. Infrared heating in food processing: An overview. Comprehensive Reviews in Food Science and Food Safety 7 (1):2–13. doi: 10.1111/j.1541-4337.2007.00024.x.
- Kubeczka, K.-H. 2009. History and sources of essential oil research. In Handbook of essential oils, 12–47. Boca Raton, FL: CRC Press.
- Kumar, R., S. Sharma, S. Sharma, and N. Kumar. 2016. Drying methods and distillation time affects essential oil content and chemical compositions of Acorus calamus l. In the Western Himalayas. Journal of Applied Research on Medicinal and Aromatic Plants 3 (3):136–41. doi: 10.1016/j.jarmap.2016.06.001.
- Kwao, S., S. Al-Hamimi, M. E. V. Damas, A. G. Rasmusson, and F. Gómez Galindo. 2016. Effect of guard cells electroporation on drying kinetics and aroma compounds of genovese basil (Ocimum basilicum l.) leaves. Innovative Food Science & Emerging Technologies 38:15–23. doi: 10.1016/j.ifset.2016.09.011.
- Rooy, L., and G. 2012. Commercial imperatives. In Handbook of food process design, 2 volume set, ed. J. Ahmed and M. S. Rahman, 1436–70. Chichester, UK: Wiley-Blackwell.
- Lafeuille, J. L., S. Lefevre, and J. Lebuhotel. 2014. Quantitation of chlorophylls and 22 of their colored degradation products in culinary aromatic herbs by HPLC-DAD-MS and correlation with color changes during the dehydration process. Journal of Agricultural and Food Chemistry 62 (8):1926–35. doi: 10.1021/jf4054947.
- Łechtańska, J. M., J. Szadzińska, and S. J. Kowalski. 2015. Microwave- and infrared-assisted convective drying of green pepper: Quality and energy considerations. Chemical Engineering and Processing: Process Intensification 98:155–64. doi: 10.1016/j.cep.2015.10.001.
- Lee, E. C., and D. B. Min. 1988. Quenching mechanism of beta-carotene on the chlorophyll sensitized photooxidation of soybean oil. Journal of Food Science 53 (6):1894–5. doi: 10.1111/j.1365-2621.1988.tb07868.x.
- Lee, J., Y. Lee, and E. Choe. 2007. Temperature dependence of the autoxidation and antioxidants of soybean, sunflower, and olive oil. European Food Research and Technology 226 (1-2):239–46. doi: 10.1007/s00217-006-0532-5.
- Lucchesi, M. E., F. Chemat, and J. Smadja. 2004. An original solvent free microwave extraction of essential oils from spices. Flavour and Fragrance Journal 19 (2):134–8. doi: 10.1002/ffj.1274.
- Mahanom, H., A. Azizah, and M. Dzulkifly. 1999. Effect of different drying methods on concentrations of several phytochemicals in herbal preparation of 8 medicinal plants leaves. Malaysian Journal of Science 5:47–54.
- Mghazli, S., M. Ouhammou, N. Hidar, L. Lahnine, A. Idlimam, and M. Mahrouz. 2017. Drying characteristics and kinetics solar drying of Moroccan rosemary leaves. Renewable Energy 108:303–10. doi: 10.1016/j.renene.2017.02.022.
- Michelino, F., A. Zambon, M. T. Vizzotto, S. Cozzi, and S. Spilimbergo. 2018. High power ultrasound combined with supercritical carbon dioxide for the drying and microbial inactivation of coriander. Journal of Co2 Utilization 24:516–21. doi: 10.1016/j.jcou.2018.02.010.
- Morad, M. M., M. A. El-Shazly, K. I. Wasfy, and HaM. El-Maghawry. 2017. Thermal analysis and performance evaluation of a solar tunnel greenhouse dryer for drying peppermint plants. Renewable Energy 101:992–1004. doi: 10.1016/j.renene.2016.09.042.
- Mortezapour, H., B. Ghobadian, S. Minaei, and M. H. Khoshtaghaza. 2012. Saffron drying with a heat pump–assisted hybrid photovoltaic–thermal solar dryer. Drying Technology 30 (6):560–6. doi: 10.1080/07373937.2011.645261.
- Moses, J. A., T. Norton, K. Alagusundaram, and B. K. Tiwari. 2014. Novel drying techniques for the food industry. Food Engineering Reviews 6 (3):43–55. doi: 10.1007/s12393-014-9078-7.
- Mujaffar, S., and S. John. 2018. Thin-layer drying behavior of West Indian lemongrass (Cymbopogan citratus) leaves. Food Science & Nutrition 6 (4):1085–99. doi: 10.1002/fsn3.642.
- Müller, J., G. Reisinger, J. Kisgeci, E. Kotta, M. Tesic, and W. Mühlbauer. 1989. Development of a greenhouse-type solar dryer for medicinal plants and herbs. Solar & Wind Technology 6 (5):523–30. doi: 10.1016/0741-983X(89)90086-6.
- Naidu, M. M., M. Vedashree, P. Satapathy, H. Khanum, R. Ramsamy, and H. U. Hebbar. 2016. Effect of drying methods on the quality characteristics of dill (Anethum graveolens) greens. Food Chemistry 192:849–56. doi: 10.1016/j.foodchem.2015.07.076.
- Nani, R. C., L. F. Di Cesare, D. Viscardi, A. Brambilla, and G. Bertolo. 2001. Effect of blanching and drying methods on the quality of dried basil (O. basilicum l.) and sage (Salvia officinalis l.). Proceedings of ICEF 8.
- Negi, P. S., and S. K. Roy. 2000. Effect of blanching and drying methods on beta-carotene, ascorbic acid and chlorophyll retention of leafy vegetables. Lwt - Food Science and Technology 33 (4):295–8. doi: 10.1006/fstl.2000.0659.
- Nijhuis, H. H., H. M. Torringa, S. Muresan, D. Yuksel, C. Leguijt, and W. Kloek. 1998. Approaches to improving the quality of dried fruit and vegetables. Trends in Food Science & Technology 9 (1):13–20. doi: 10.1016/S0924-2244(97)00007-1.
- Nykanen, L., and I. Nykanen. 1987. Effect of drying on the composition of the essential oil of some labiatae herbs cultivated in Finland. In Flavour science and technology: Proceedings of the 5th Weurman Flavour Research Symposium. Chichester, West Sussex: Wiley.
- Oboh, G. 2005. Effect of blanching on the antioxidant properties of some tropical green leafy vegetables. Lwt - Food Science and Technology 38 (5):513–7. doi: 10.1016/j.lwt.2004.07.007.
- Omidbaigi, R., M. Kabudani, and Z. Tabibzadeh. 2007. Effect of drying methods on the essential oil content and composition Oftanacetum parthenium(l.) schultz bip cv. Journal of Essential Oil Bearing Plants 10 (1):26–30. doi: 10.1080/0972060X.2007.10643514.
- Omidbaigi, R., F. Sefidkon, and F. Kazemi. 2004. Influence of drying methods on the essential oil content and composition of roman chamomile. Flavour and Fragrance Journal 19 (3):196–8. doi: 10.1002/ffj.1340.
- Orphanides, A., V. Goulas, and V. Gekas. 2013. Effect of drying method on the phenolic content and antioxidant capacity of spearmint. Czech Journal of Food Sciences 31 (No. 5):509–13. doi: 10.17221/526/2012-CJFS.
- Orphanides, A., V. Goulas, and V. Gekas. 2016. Drying technologies: Vehicle to high-quality herbs. Food Engineering Reviews 8 (2):164–80. doi: 10.1007/s12393-015-9128-9.
- Ostermeier, R., P. Giersemehl, C. Siemer, S. Topfl, and H. Jager. 2018. Influence of pulsed electric field (pef) pre-treatment on the convective drying kinetics of onions. Journal of Food Engineering 237:110–7. doi: 10.1016/j.jfoodeng.2018.05.010.
- Oxford. 2019. Definition of herb in English. In Oxford English Dictionary, ed. O. E. Dictionary: @OxfordWords. Oxford, UK: Oxford University Press.
- Ozkan, I. A., B. Akbudak, and N. Akbudak. 2007. Microwave drying characteristics of spinach. Journal of Food Engineering 78 (2):577–83. doi: 10.1016/j.jfoodeng.2005.10.026.
- Pääkkönen, K., J. Havento, and B. Galambosi. 1999. Infrared drying of herbs (research note). Agricultural and Food Science 8 (1):19–27. doi: 10.23986/afsci.5622.
- Pääkkönen, K., T. Malmsten, and L. Hyvonen. 1989. Effects of drying method, packaging, and storage-temperature and time on the quality of dill (Anethum-graveolens l). Journal of Food Science 54 (6):1485–7. &. doi: 10.1111/j.1365-2621.1989.tb05141.x.
- Parniakov, O., O. Bals, N. Lebovka, and E. Vorobiev. 2016. Pulsed electric field assisted vacuum freeze-drying of apple tissue. Innovative Food Science & Emerging Technologies 35:52–7. doi: 10.1016/j.ifset.2016.04.002.
- Piga, A., M. Usai, M. Marchetti, M. Foddai, A. Del Caro, P. Meier, V. Onorati, and F. Vinci. 2007. Influence of different drying parameters on the composition of volatile compounds of thyme and rosemary cultivated in sardinia. Paper presentat at the 3rd CIGR. Section VI International Symposium on Food and Agricultural Products: Processing and Innovations, in Naples, Italy.
- Pin, K. Y., T. G. Chuah, A. A. Rashih, C. L. Law, M. A. Rasadah, and T. S. Y. Choong. 2009. Drying of betel leaves (Piper betlel): Quality and drying kinetics. Drying Technology 27 (1):149–55. doi: 10.1080/07373930802566077.
- Pirbalouti, A. G., E. Mahdad, and L. Craker. 2013. Effects of drying methods on qualitative and quantitative properties of essential oil of two basil landraces. Food Chemistry 141 (3):2440–9. doi: 10.1016/j.foodchem.2013.05.098.
- Pirbalouti, A. G., S. Salehi, and L. Craker. 2017. Effect of drying methods on qualitative and quantitative properties of essential oil from the aerial parts of coriander. Journal of Applied Research on Medicinal and Aromatic Plants 4 (1):35–40. doi: 10.1016/j.jarmap.2016.07.006.
- Prothon, F., L. Ahrne, and I. Sjoholm. 2003. Mechanisms and prevention of plant tissue collapse during dehydration: A critical review. Critical Reviews in Food Science and Nutrition 43 (4):447–79. doi: 10.1080/10408690390826581.
- Rababah, T. M., M. Al-U’datt, M. Alhamad, M. Al-Mahasneh, K. Ereifej, J. Andrade, B. Altarifi, A. Almajwal, and W. Yang. 2015. Effects of drying process on total phenolics, antioxidant activity and flavonoid contents of common Mediterranean herbs. International Journal of Agricultural and Biological Engineering 8 (2):145–50. doi: 10.3965/j.ijabe.20150802.1496.
- Rabha, D. K., P. Muthukumar, and C. Somayaji. 2017. Experimental investigation of thin layer drying kinetics of ghost chilli pepper (Capsicum chinense jacq.) dried in a forced convection solar tunnel dryer. Renewable Energy 105:583–9. doi: 10.1016/j.renene.2016.12.091.
- Raghavan, B., K. O. Abraham, M. L. Shankaranarayana, and W. D. Koller. 1994. Studies on flavor changes during drying of dill (Anethum-sowa roxb) leaves. Journal of Food Quality 17 (6):457–66. doi: 10.1111/j.1745-4557.1994.tb00166.x.
- Raghavan, B., L. J. Rao, M. Singh, and K. O. Abraham. 1997. Effect of drying methods on the flavour quality of marjoram (Oreganum majorana l). Food / Nahrung 41 (3):159–61. doi: 10.1002/food.19970410309.
- Rahimmalek, M., and S. H. Goli. 2013. Evaluation of six drying treatments with respect to essential oil yield, composition and color characteristics of Thymys daenensis subsp daenensis. Celak leaves. Industrial Crops and Products 42:613–9. doi: 10.1016/j.indcrop.2012.06.012.
- Rao, L. J., M. Singh, B. Raghavan, and K. O. Abraham. 1998. Rosemary (Rosmarinus officinalis l.): Impact of drying on its flavor quality. Journal of Food Quality 21 (2):107–15. doi: 10.1111/j.1745-4557.1998.tb00508.x.
- Rayaguru, K., and W. Routray. 2010. Effect of drying conditions on drying kinetics and quality of aromatic Pandanus amaryllifolius leaves. Journal of Food Science and Technology 47 (6):668–73. doi: 10.1007/s13197-010-0114-1.
- Rocha, T., A. Lebert, and C. Marty-Audouin. 1993. Effect of pretreatments and drying conditions on drying rate and color retention of basil (Ocimum-basilicum). Lwt - Food Science and Technology 26 (5):456–63. doi: 10.1006/fstl.1993.1090.
- Rodriguez, J., A. Mulet, and J. Bon. 2014. Influence of high-intensity ultrasound on drying kinetics in fixed beds of high porosity. Journal of Food Engineering 127:93–102. doi: 10.1016/j.jfoodeng.2013.12.002.
- Rohloff, J., S. Dragland, R. Mordal, and T. H. Iversen. 2005. Effect of harvest time and drying method on biomass production, essential oil yield, and quality of peppermint (mentha x piperita l.). Journal of Agricultural and Food Chemistry 53 (10):4143–8. doi: 10.1021/jf047998s.
- Rubinskiene, M., P. Viskelis, E. Dambrauskiene, J. Viskelis, and R. Karkleliene. 2015. Effect of drying methods on the chemical composition and colour of peppermint (mentha x piperita l.) leaves. Zemdirbyste-Agriculture 102 (2):223–8. doi: 10.13080/z-a.2015.102.029.
- Santacatalina, J. V., D. Fissore, J. A. Carcel, A. Mulet, and J. V. Garcia-Perez. 2015. Model-based investigation into atmospheric freeze drying assisted by power ultrasound. Journal of Food Engineering 151:7–15. doi: 10.1016/j.jfoodeng.2014.11.013.
- Sarimeseli, A. 2011. Microwave drying characteristics of coriander (Coriandrum sativum l.) leaves. Energy Conversion and Management 52 (2):1449–53. doi: 10.1016/j.enconman.2010.10.007.
- Sárosi, S.,. L. Sipos, Z. Kókai, Z. Pluhár, B. Szilvássy, and I. Novák. 2013. Effect of different drying techniques on the aroma profile of Thymus vulgaris analyzed by GC–MS and sensory profile methods. Industrial Crops and Products 46:210–6. doi: 10.1016/j.indcrop.2013.01.028.
- Schaarschmidt, S. 2016. Public and private standards for dried culinary herbs and spices—part i: Standards defining the physical and chemical product quality and safety. Food Control 70:339–49. doi: 10.1016/j.foodcont.2016.06.004.
- Scheer, H. 1991. Structure and occurence of chlorophylls. In Chlorophylls, ed. H. Scheer, 3–30. Boca Raton, FL: CRC Press.
- Schossler, K., H. Jager, and D. Knorr. 2012. Effect of continuous and intermittent ultrasound on drying time and effective diffusivity during convective drying of apple and red bell pepper. Journal of Food Engineering 108 (1):103–10. doi: 10.1016/j.jfoodeng.2011.07.018.
- Sellami, I. H., W. A. Wannes, I. Bettaieb, S. Berrima, T. Chahed, B. Marzouk, and F. Limam. 2011. Qualitative and quantitative changes in the essential oil of Laurus nobilis l. Leaves as affected by different drying methods. Food Chemistry 126 (2):691–7. doi: 10.1016/j.foodchem.2010.11.022.
- Sevik, S. 2014. Experimental investigation of a new design solar-heat pump dryer under the different climatic conditions and drying behavior of selected products. Solar Energy 105:190–205. doi: 10.1016/j.solener.2014.03.037.
- Shahhoseini, R., H. Ghorbani, S. R. Karimi, A. Estaji, and M. Moghaddam. 2013. Qualitative and quantitative changes in the essential oil of lemon verbena (Lippia citriodora) as affected by drying condition. Drying Technology 31 (9):1020–8. doi: 10.1080/07373937.2013.771649.
- Sharma, A., C. R. Chen, and N. V. Lan. 2009. Solar-energy drying systems: A review. Renewable and Sustainable Energy Reviews 13 (6-7):1185–210. doi: 10.1016/j.rser.2008.08.015.
- Shaw, M., V. Meda, L. Tabil, and A. Opoku. 2016. 2006. Drying and color characteristics of coriander foliage using convective thin-layer and microwave drying. Journal of Microwave Power and Electromagnetic Energy 41 (2):56–65. doi: 10.1080/0832782311688559.
- Singh, M., B. Raghavan, and K. O. Abraham. 1996. Processing of marjoram (Majorana hortensis moench) and rosemary (Rosmarinus officinalis l). Effect of blanching methods on quality. Food / Nahrung 40 (5):264–6. doi: 10.1002/food.19960400507.
- Sledz, M., A. Wiktor, K. Rybak, M. Nowacka, and D. Witrowa-Rajchert. 2016. The impact of ultrasound and steam blanching pre-treatments on the drying kinetics, energy consumption and selected properties of parsley leaves. Applied Acoustics 103:148–56. doi: 10.1016/j.apacoust.2015.05.006.
- Sledz, M., and D. Witrowa-Rajchert. 2012. Influence of microwave-convective drying of chlorophyll content and colour of herbs. Acta Agrophysica 19 (4):865–876.
- Soysal, Y. 2004. Microwave drying characteristics of parsley. Biosystems Engineering 89 (2):167–73. doi: 10.1016/j.biosystemseng.2004.07.008.
- Sukadeetad, K., W. Nakbanpote, M. Heinrich, and N. Nuengchamnong. 2018. Effect of drying methods and solvent extraction on the phenolic compounds of Gynura pseudochina (l.) dc. Leaf extracts and their anti-psoriatic property. Industrial Crops and Products 120:34–46. doi: 10.1016/j.indcrop.2018.04.020.
- Szumny, A., A. Figiel, A. Gutierrez-Ortiz, and A. A. Carbonell-Barrachina. 2010. Composition of rosemary essential oil (Rosmarinus officinalis) as affected by drying method. Journal of Food Engineering 97 (2):253–60. doi: 10.1016/j.jfoodeng.2009.10.019.
- Tambunan, A. H., and K. Yudistira. 2001. Freeze drying characteristics of medicinal herbs. Drying Technology 19 (2):325–31. doi: 10.1081/DRT-100102907.
- Tarakemeh, A., and A. Abutalebi. 2012. Effect of drying method on the essential oil quantity of basil (Ocimum basilicuml.). Journal of Essential Oil Bearing Plants 15 (3):503–5. doi: 10.1080/0972060X.2012.10644079.
- Tarhan, S., I. Telci, M. T. Tuncay, and H. Polatci. 2010. Product quality and energy consumption when drying peppermint by rotary drum dryer. Industrial Crops and Products 32 (3):420–7. doi: 10.1016/j.indcrop.2010.06.003.
- Tarhan, S., I. Telci, M. T. Tuncay, and H. Polatci. 2011. Peppermint drying performance of contact dryer in terms of product quality, energy consumption, and drying duration. Drying Technology 29 (6):642–51. doi: 10.1080/07373937.2010.520421.
- Telfser, A., and F. G. Galindo. 2019. Effect of reversible permeabilization in combination with different drying methods on the structure and sensorial quality of dried basil (Ocimum basilicum l.) leaves. LWT-Food Science and Technology 99:148–55. doi: 10.1016/j.lwt.2018.09.062.
- Tham, T. C., M. X. Ng, S. H. Gan, L. S. Chua, R. Aziz, L. A. Chuah, C. L. Hii, S. P. Ong, N. L. Chin, and C. L. Law. 2017. Effect of ambient conditions on drying of herbs in solar greenhouse dryer with integrated heat pump. Drying Technology 35 (14):1721–32. doi: 10.1080/07373937.2016.1271984.
- Therdthai, N., and W. B. Zhou. 2009. Characterization of microwave vacuum drying and hot air drying of mint leaves (Mentha cordifolia opiz ex fresen). Journal of Food Engineering 91 (3):482–9. doi: 10.1016/j.jfoodeng.2008.09.031.
- Thomas, W. 1996. Rf drying provides process savings: New systems optimize radio frequency drying for the ceramic and glass fiber industries. Ceramic Industry Magazine 146 (4):30–4.
- Tiwari, B. K., and T. J. Mason. 2012. Ultrasound processing of fluid foods. In Novel thermal and non-thermal technologies for fluid foods, ed. P. J. Cullen, B. K. Tiwari and V. P. Valdramidis, 135–65. San Diego: Academic Press.
- Torki-Harchegani, M., D. Ghanbarian, V. Maghsoodi, and A. Moheb. 2017. Infrared thin layer drying of saffron (Crocus sativus l.) stigmas: Mass transfer parameters and quality assessment. Chinese Journal of Chemical Engineering 25 (4):426–32. doi: 10.1016/j.cjche.2016.09.005.
- Tummanichanont, C., S. Phoungchandang, and G. Srzednicki. 2017. Effects of pretreatment and drying methods on drying characteristics and quality attributes of Andrographis paniculata. Journal of Food Processing and Preservation 41 (6):e13310. doi: 10.1111/jfpp.13310.
- Turek, C., and F. C. Stintzing. 2013. Stability of essential oils: A review. Comprehensive Reviews in Food Science and Food Safety 12 (1):40–53. doi: 10.1111/1541-4337.12006.
- Venskutonis, P. R. 1997. Effect of drying on the volatile constituents of thyme (Thymus vulgaris l) and sage (Salvia officinalis l). Food Chemistry 59 (2):219–27. doi: 10.1016/S0308-8146(96)00242-7.
- Venskutonis, P. R., L. Poll, and M. Larsen. 1996. Influence of drying and irradiation on the composition of volatile compounds of thyme (Thymus vulgaris l.). Flavour and Fragrance Journal 11 (2):123–8. doi: 10.1002/(Sici)1099-1026(199603)11:2 < 123::Aid-Ffj555 > 3.0.Co;2-1.
- Werker, E. 2000. Trichome diversity and development. In Advances in botanical research, vol. 31, 1–35. New York, US: Academic Press.
- Winterhalter, P. and G.K. Skouroumounis. 1997. Glycoconjugated aroma compounds: Occurrence, role and biotechnological transformation. In Biotechnology of aroma compounds, ed. R. G. Berger, W. Babel, H. W. Blanch, C. L. Cooney, S. O. Enfors, K. E. L. Eriksson, A. Fiechter, A. M. Klibanov, B. Mattiasson, S. B. Primrose, H. J. Rehm, P. L. Rogers, H. Sahm, K. Schügerl, G. T. Tsao, K. Venkat, J. Villadsen, U. Von Stockar and C. Wandrey, 73–105. Berlin, Heidelberg: Springer.
- Won, Y.-C., S. C. Min, and D.-U. Lee. 2015. Accelerated drying and improved color properties of red pepper by pretreatment of pulsed electric fields. Drying Technology 33 (8):926–32. doi: 10.1080/07373937.2014.999371.
- Wray, D., and H. S. Ramaswamy. 2015. Novel concepts in microwave drying of foods. Drying Technology 33 (7):769–83. doi: 10.1080/07373937.2014.985793.
- Xing, Y., H. Lei, J. Wang, Y. Wang, J. Wang, and H. Xu. 2018. Effects of different drying methods on the total phenolic, rosmarinic acid and essential oil of purple perilla leaves. Journal of Essential Oil Bearing Plants 20 (6):1594–606. doi: 10.1080/0972060x1413957.
- Yahya, M., M. Othman, K. Sopian, W. Daud, and B. Yatim. 2004. Quality of pegaga leaf dried in a solar assisted dehumidification drying system. Proceedings of the 14th IDS, 1049–54.
- Yi, W. G., and H. Y. Wetzstein. 2011. Effects of drying and extraction conditions on the biochemical activity of selected herbs. HortScience 46 (1):70–3. doi: 10.21273/HORTSCI.46.1.70.
- Yousif, A. N., T. D. Durance, C. H. Scaman, and B. Girard. 2000. Headspace volatiles and physical characteristics of vacuum-microwave, air, and freeze-dried oregano (Lippia berlandieri schauer). Journal of Food Science 65 (6):926–30. doi: 10.1111/j.1365-2621.2000.tb09394.x.
- Yousif, A. N., C. H. Scaman, T. D. Durance, and B. Girard. 1999. Flavor volatiles and physical properties of vacuum-microwave- and air-dried sweet basil (Ocimum basilicum l.). Journal of Agricultural and Food Chemistry 47 (11):4777–81. doi: 10.1021/jf990484m.