Abstract
Phytosterols and phytostanols are natural products present in vegetable oils, nuts, and seeds, or added to consumer food products whose intake is inversely associated with incidence and prognosis of several cancers. Randomized cancer prevention trials in humans are unfeasible due to time and cost yet the cellular processes and signaling cascades that underpin anti-cancer effects of these phytochemicals have been explored extensively in vitro and in preclinical in vivo models. Here we have performed an original systematic review, meta-analysis, and qualitative interpretation of literature published up to June 2020. MEDLINE, Scopus, and hand-searching identified 408 unique records that were screened leading to 32 original articles that had investigated the effects of phytosterols or phytostanols on cancer biology in preclinical models. Data was extracted from 22 publications for meta-analysis. Phytosterols were most commonly studied and found to reduce primary and metastatic tumor burden in all cancer sites evaluated. Expression of pAKT, and markers of metastasis (alkaline phosphatase, matrix metalloproteases, epithelial to mesenchymal transcription factors, lung and brain colonization), angiogenesis (vascular endothelial growth factor, CD31), and proliferation (Ki67, proliferating cell nuclear antigen) were consistently reduced by phytosterol treatment in breast and colorectal cancer. Very high dose treatment (equivalent to 0.2–1 g/kg body weight not easily achievable through diet or supplementation in humans) was associated with adverse events including poor gut health and intestinal adenoma development. Phytosterols and phytostanols are already clinically recommended for cardiovascular disease risk reduction, and represent promising anti-cancer agents that could be delivered in clinic and to the general population at low cost, with a well understood safety profile, and now with a robust understanding of mechanism-of-action.
Graphical Abstract
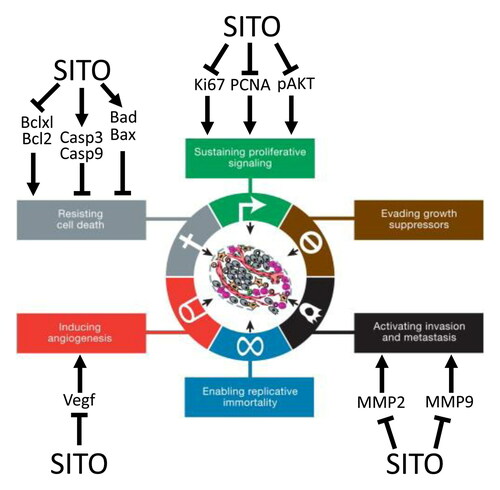
Introduction
Dietary intake of fruits and vegetables, and of grains and seeds, is inversely associated with cancer risk and directly associated with cancer patient survival (WCRF/AICR Citation2018). Research that provides mechanistic explanations for these epidemiological and clinical trial observations is incomplete, which limits translation for public health. One group of phytochemicals with proposed anti-cancer activity are phytosterols and their saturated counterparts, phytostanols. Case-control studies indicated that high dietary phytosterol/stanol (PSS) intake has been associated with reduced odds of several cancers including lung (odds ratio (OR) 0.29, 95% CI 0.14–0.63) (Mendilaharsu et al. Citation1998), stomach (OR 0.33, 95% CI 0.17–0.65) (De Stefani et al. Citation2000), colorectum (OR 0.50, 95% CI 0.41–0.61) (Huang et al. Citation2017), and ovary (OR 0.42, 95% CI 0.20–0.87) (McCann et al. Citation2003). A recent meta-analysis indicated PSS intake imparts a non-linear reduction in pan-cancer relative risk (RR 0.63, 95% CI 0.49–0.81) with peak reduction achieved with approximately 0.5 g/day or 6–7 mg/kg (Jiang et al. Citation2019).
Phytosterols, structurally and functionally related to cholesterol (), are present in relatively large amounts in vegetable oils, nuts and seeds (Phillips et al. Citation2005) with the total phytosterol content of some vegetable oils reaching values as high as 19 g/kg (Yang et al. Citation2019). The richest sources are commercial products supplemented with PSS (approx. 2–3 g PSS per portion) that are marketed for lowering low density lipoprotein cholesterol (LDL-C), and do so by 10–15% in addition to what is achievable by statins (EFSA Panel on Dietetic Products, Nutrition and Allergies Citation2013; Han et al. Citation2016). Large scale manufacturing of PSS has standardized production methods which has ameliorated many problems commonly associated with studying natural products, where isolation, extraction, or synthesis methodology may vary, and final compounds applied in studies may be variable.
Figure 1. Molecular structure of cholesterol and common dietary plant sterols and stanols. Reproduced from (Hutchinson et al. Citation2019) with permission.
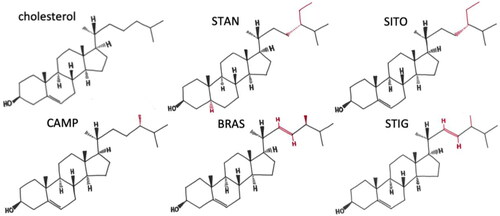
In 2000, Hanahan and Weinberg published ‘The Hallmarks of Cancer’ highlighting key characteristics of the developing tumor (Hanahan and Weinberg Citation2000). Here, we use the updated categorization (Hanahan and Weinberg Citation2011) to map how PSS may be interacting with cancerous and pre-cancerous cells in pre-clinical cancer models. This systematic review addresses a critical gap in the literature by collating all available information regarding the cellular and molecular response of tumors to PSS in vivo. Consistent evidence of a robust molecular mechanism (or lack thereof) would allow clinical research to build on the epidemiological evidence and begin evaluation of PSS as supplements that could contribute to reduced global cancer burden in the prevention setting, or as treatment adjuncts that could improve prognosis for cancer patients.
Methods
Search strategy
A comprehensive search of online databases MEDLINE and Scopus was carried out throughout June 2020. Search terms are available in supplementary materials. The intention to review was submitted to PROSPERO on 9th June 2020 and was approved and published on 11th June 2020 with reference number CRD42020191337 (Thorne et al. Citation2020) and can be accessed at crd.york.ac.uk/PROSPERO.
Study selection
Titles and abstracts were screened for inclusion criteria; i) original data papers (e.g. reviews were excluded at this point); ii) conducted in whole organism animal models; iii) evaluated cancer (other diseases excluded); iv) phytosterol, phytostanol, a derivative/metabolite, or a mixture; v) English language publications; vi) not published in a predatory journal, listed on the website predatoryjournals.com. Screening was performed in duplicate by independent reviewers. Discrepancies were evaluated, discussed, and agreed by all members of the research team.
Data extraction
All data were extracted in duplicate into Microsoft Excel by independent contributors. Any disagreements were resolved by discussion with full research team. Extracted data included information and measures on animal models; study design; intervention and control treatments, duration, and dose; cancer type; and outcome assessment. Effect sizes were extracted as means with measures of variance. Where effect sizes were only presented in figures, WebPlotDigitizer (v4.2) was used to extract data (Rohatgi Citation2019). Where more than 1 treatment group was compared to the same control, the effect size of the highest dose was extracted, and/or the parent PSS molecule was chosen rather than derivatives.
Statistical analysis
Meta-analyses were performed in RevMan version 5.3 (The Nordic Cochrane Centre, T. C. C Citation2014). Heterogeneity was anticipated between studies due to variation in animal models and treatments, so random-effects models were used if ≥ 3 studies were available. Where fewer than three studies were available, a fixed effects model was applied to preserve power and minimize risk of type-1 errors (Jackson and Turner Citation2017). In analysis where effect sizes were calculated using different metrics that could not be harmonized, standardized mean difference (SMD) was used (Borenstein et al. Citation2009). Effect sizes of SMD are interpreted as mean difference in units of standard deviation (versus control) following exposure to the intervention. Degree of heterogeneity of meta-analyses was quantified using I2. We anticipated that meta-analyses of animal studies would reflect higher levels of heterogeneity than human clinical trials (Vesterinen et al. Citation2014) as clinical trials aim to minimise inter-population variables, whereas animal studies generally aim to minimize intra-study variation through the use of inbred strains, strict protocol, and controlled environments. This in turn, makes the collective assessment of animal studies susceptible to high inter-study variation because each study has adapted its own protocol. We applied I2 > 75% as a marker of high heterogeneity for meta-analysis of animal studies (Peter et al. Citation2020). In meta-analyses with ≥10 comparisons per outcome and I2 > 75%, sources of heterogeneity were explored and discussed (Deeks et al. Citation2019; Peter et al. Citation2020). Assessment of publication bias was performed by visual inspection where ≥10 studies were assessed for a single outcome.
Risk of bias
Risk of bias (ROB) was performed for experimental design and adherence to BJP and PROPSERO guidelines for animal experiments (SF1A); adherence to BJP guidelines for natural products (SF1B); adherence to BJP guidelines for immunoblotting adapted to include immunohistochemistry (SF1C).
Results
Systematic search
Three hundred and thirty-six records identified in Scopus were combined with 281 from Medline and with 22 identified through other routes (e.g. preliminary literature reviews) resulting in 408 unique records after removal of duplicates. After screening for inclusion and exclusion criteria full text of 46 records was analyzed. Thirty-two were found suitable for inclusion in qualitative synthesis (summarized in ) and of these 22 were appropriate for data extraction and meta-analysis. This information is summarized in the PRISMA diagram ().
Table 1. Summary of all extracted data.
Animal models
Mice or rats were used in all studies except for one that used zebrafish. Of the 32 studies using mammalian models, 18 employed xenograft assays, 10 induced tumors through mutagen, and 4 were spontaneous genetic cancer models. A total of nine studies evaluated colorectal cancer (CRC), 9 breast cancer (BCa), 4 skin/melanoma, 2 lung (LCa). Gastric cancer (GCa), Ehrlich-Lettre ascites carcinoma (ECa), hepatoma (HEP), cholangiocarcinoma (CCA), ovarian cancer (OCa), pancreatic cancer (PaCa), renal cancer (RCa), and prostate cancer (PCa) were each studied once. Metastasis was evaluated in three studies. Twice in the context of skin evaluating metastasis to the brain and lung, and once in breast cancer evaluating metastasis to the lung.
PSS administration
The most commonly studied PSS was sitosterol ([SITO] n = 11) and its derivatives (n = 7). Stigmasterol ([STIG] n = 4), fucosterol ([FUCO] n = 2) and PSS mixtures (n = 6) were next most common, with peniocerol (PENI) and Z-guggulsterone (ZGUG) reported once each. Campesterol (CAMP), a relatively common PSS was only studied as part of PSS mixtures (n = 5). Phytostanols were only assessed as mixtures. PSS were administered via three main routes, either integrated into chow per oral (PO) (n = 15), oral gavage (OG) (n = 5), or injection intravenously (IV) (n = 1), or intraperitoneally (IP) (n = 10) or as microinjection (n = 1). The concentration of PSS to which animals were exposed varied by several orders of magnitude. Doses, normalized for a typical 65–75 kg human, ranged from the equivalent of 3 mg per person per week up to 75 g per person per day.
Sustaining proliferative signaling
A range of growth factors and signaling pathways regulate the cell cycle machinery. Typically, tumor proliferative index in humans can be measured by expression of cell cycle machinery proteins such as Ki67, PCNA, and CDKs. These proteins can be measured in tumor tissue by immunohistochemistry or immunoblotting, and tumor growth can be tracked non-invasively with calipers or measuring expression of light producing transgenes. Cells can also be isolated from tumors and flow sorted based on DNA content providing a measure of cell cycle kinetics in the tumor.
In our meta-analysis of 14 studies (16 comparisons; n = 199) across PSS treatments we report that PSS mitigates tumor growth volume in breast (MD = −827.17 mm3; 95% CI: −1297.26, −357.07; I2 = 100%; p < 0.001), colon (MD = −1,298.56 mm3; 95% CI: −2,156.76, −440.35; I2 = 96%; p = 0.003), other cancers (MD = −864.21 mm3; 95% CI: −1199.11, −215.36; I2 = 99%; p = 0.005), and overall cancer (MD = −864.21 mm3; 95% CI: −1137.55, −590.88; I2 = 99%; p < 0.001), compared to controls (). Similarly, tumor mass was much smaller across PSS treatments in 11 studies (15 comparisons; n = 222) reporting on breast (MD = −0.61 g; 95% CI: −0.93, −0.29; I2 = 93%; p < 0.001), colon (MD = −3.40 g; 95% CI: −3.68, −3.12; I2 = 13%; p < 0.001), other cancers (MD = −0.76 g; 95% CI: −1.10, −0.42; I2 = 97%; p < 0.001), and overall cancer (MD = −1.18; 95% CI: −1.49, −0.87; I2 = 98%; p < 0.001), compared to controls (). For overall total cancer mass () and volume (), we observed very high heterogeneity (I2>75%) which is likely attributed to differences in effect sizes between cancer models. No evidence of publication bias was observed in funnel plots for either analyses (data not shown). Tumor growth was also assessed in several studies via plasma markers CEA, CA125, and CA153. All markers were significantly reduced in PSS groups compared to controls ().
Figure 3. Forest plot of tumor size and proliferation markers after plant phytosterols and stanols administration. (A) Mean difference in change between PSS treatment and control of tumor volume (mm3) according to cancer type. (B) Mean difference in change between PSS treatment and control of tumor weight (g) according to cancer type. (C) Standard mean difference in change between PSS treatment and control of PCNA proliferation marker. (D) Standard mean difference in change between PSS treatment and control of Ki67 proliferation marker.
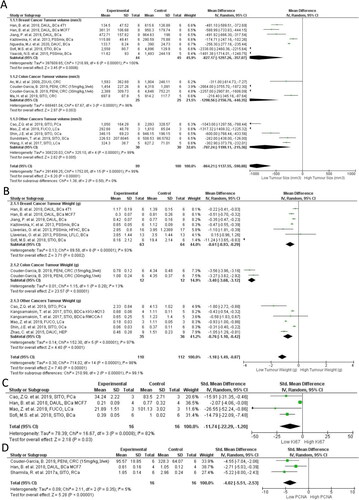
Figure 4. Forest plot of cancer serum biomarkers after plant phytosterols and stanols administration. (A) Standard mean difference in change between PSS treatment and control of CEA. (B) Standard mean difference in change between PSS treatment and control of CA125. (C) Mean difference in change between PSS treatment and control of CA153.
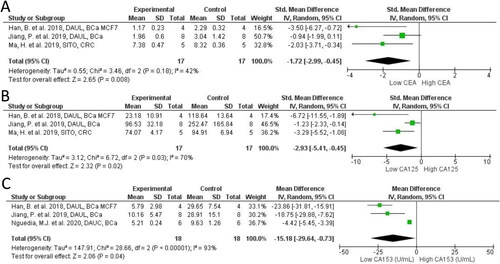
Deregulation of signaling pathways can lead to structural changes in epithelial cell organization leading to formation of aberrant crypt foci (ACF), an early marker of CRC risk (Alrawi et al. Citation2006). SITO at a range of doses between 5 and 20 mg/kg per day (Baskar et al. Citation2010), and at 0.2% dw PO (Deschner et al. Citation1982) reduced colonic epithelial cell proliferation, ACF and crypt multiplicity, as well as tumor growth in xenograft CRC. In a DMBA mutagen model of skin cancer, STIG (0.2–0.4 g/kg PO) resulted in fewer and smaller skin papillomas, which were preceded by significantly longer latency period (Ali et al. Citation2015). STIG (50 mg/kg IP) also slowed tumor cell doubling time in a melanoma xenograft model (Iyer and Patil Citation2012). Yaccob and colleagues found a striking reduction in an NMU mutagen model of breast cancer, where tumor number was reduced 10-fold suggesting that induced tumors actually regressed in the treatment group (Yaacob et al. Citation2015).
Expression of cell proliferation markers such as Ki67, PCNA, components of the cell cycle machinery, and proliferation promoting oncogenes were evaluated in several studies. When amalgamated we found a significant reduction in Ki67 (SMD = −11.74; 95% CI: −22.29, −1.20; p = 0.03; ) and PCNA (SMD = −4.02; 95% CI: −5.51, −2.53; p < 0.0001; ). Ki67 was evaluated by western Blot (WB) or immunohistochemistry (IHC) in four studies, investigating 20, 40, 60, 80, 100 mg/kg treatments. Ki67 and PCNA were significantly reduced across all studies. Interestingly, Ki67 was reduced by FUCO in LCa in a convincing dose-dependent manner (Mao et al. Citation2019) as was PCNA by PENI dose (15 mg or 30 mg/kg weekly) and frequency (15 mg/kg once/week or three/week) in CRC xenograft HCT116 (Couder-García et al. Citation2019). Aside from Ki67 and PCNA, Cyclin D1, a proliferation control protein, was reduced by 50% in the tumors of SITO treated KCa models (Sharmila and Sindhu Citation2017a), but was unchanged in another study where very high PSS containing chow was provided to Apcmin mice (Marttinen et al. Citation2014) ().
A range of studies have considered tumor cell proliferation after exposing models to PSS mixtures, which are arguably more representative of typical human exposure. In physiological doses PSS mixtures exerted their inhibitory effects on tumor growth in different cancer types like cholangiocarcinoma and breast cancer (Kangsamaksin et al. Citation2017; Kazłowska et al. Citation2013). In an N-Nitroso-N-methylurea (MNU) carcinogen induced model of CRC, mice were fed with different doses of PSS in feed (0.3%, 1% and 2% dw) of a PSS mixture (60% SITO, 30% CAMP, 5% STIG) preneoplastic lesion formation was reduced (Janezic and Rao Citation1992). Ju et al. evaluated exposure to very high doses of a PSS mixture (9.8 g/kg SITO+ 0.2 g/kg STIG) in chow and observed reduced tumor area in BCa xenograft mouse (Ju et al. Citation2004), and Yaacob who applied somewhat lower doses (40 mg/kg: 53% SITO, 16% CAMP, 26% STIG) also found reduced tumor volume and number in an MNU mutagen model of BCa (Yaacob et al. Citation2015).
At cancer sites where exposure to dietary compounds is considered highest, such as the GI tract, some studies found PSS mixtures to not be so effective. Rats fed with 24 mg/rat/day of a PSS mixture (55% SITO, 41% CAMP, 4% STIG) developed a similar tumor burden to their controls and were likely to suffer complications from poor gut bacterial health (Quilliot et al. Citation2001). Notably, CAMP made up a large proportion of this PSS mixture. A phytosterol mixture again containing high CAMP, provided at exceptionally high levels via chow (20 mg/mouse/day, which when calculated by weight by weight, is equivalent to 70 g/person/day), was found to promote tumor formation in the Apcmin mouse model (Marttinen et al. Citation2014). In an experimentally matched study by the same group, the same dose of phytostanols was also found to promote tumor formation in the same model (Marttinen et al. Citation2013).
Combined, the broad consensus in the published data indicates that PSS is anti-proliferative in vivo, and reduced tumor growth is associated with lower expression of proliferation markers such as Ki67 and PCNA. However, in some studies performed at very high doses, especially in mixtures containing high CAMP or CAMS concentrations, PSS appeared to be either ineffective, led to gut health complications, or in two cases promoted tumor growth and activation of oncogene expression and activity. The route of administration for high doses appeared to be an important determinant. The GI tract is exposed to highest doses of orally administered compounds, which may explain why dose equivalent to 70 g/person/day of plant stanols, or plant sterols, was associated with intestinal tumor formation. We are unaware of studies that have directly compared different routes of PSS administration to explore this hypothesis further. Globally, these data indicate that PSS dose, frequency and route of administration are likely to be important variables to consider in human studies, especially if pharmacological approaches with maximum tolerable doses are considered.
Resisting cell death
Programmed cell death is important for managing malignant and pre-malignant cells. Cancer cells become resistant to death signals by increasing the expression of anti-apoptotic proteins, and reducing expression of pro-apoptotic proteins. Bcl2 and Bclxl for example maintain mitochondrial membrane stability (thus preventing the release of cytochrome c into the cytoplasm, a very early event in apoptosis) and Bad and Bax oppose this mechanism by destabilizing mitochondrial membrane integrity. Caspases are then activated and enact orchestrated cellular destruction. In vivo, cell death can be assessed longitudinally by tumor growth assays, on flow sorted tumor cells labeled for various stages of apoptosis, or by measuring expression of proteins regulating apoptosis.
Across all studies we identified, tumors of PSS treated animals had significantly greater expression of pro-apoptotic proteins and reduced expression of anti-apoptotic signals (). There was a significant decrease in expression of Bcl2 (SMD = −3.14; 95% CI: −5.40, −0.89; p = 0.006; ) and BclxL (MD = −0.43 DU; 95% CI: −0.51, −0.35; p < 0.0001; ) in the treated animals relative to controls. Both of these proteins function to maintain mitochondrial membrane integrity and resist apoptosis. For the pro-apoptotic proteins, Bax was strongly induced (SMD = 7.86; 95% CI: 2.69, 13.03; p = 0.003; ), whilst Bad was also induced (MD = 0.14 DU; 95% CI: 0.07, 0.21; p < 0.0001 ).
Figure 5. Forest plot of apoptosis markers after plant phytosterols and stanols administration. (A) Standard mean difference in change between PSS treatment and control of Bcl-2. (B) Mean difference in change between PSS treatment and control of Bclxl. (C) Standard mean difference in change between PSS treatment and control of Bax. (D) Mean difference in change between PSS treatment and control of Bad. (E) Standard mean difference in change between PSS treatment and control of Caspase-3. (F) Standard mean difference in change between PSS treatment and control of Caspase-9.
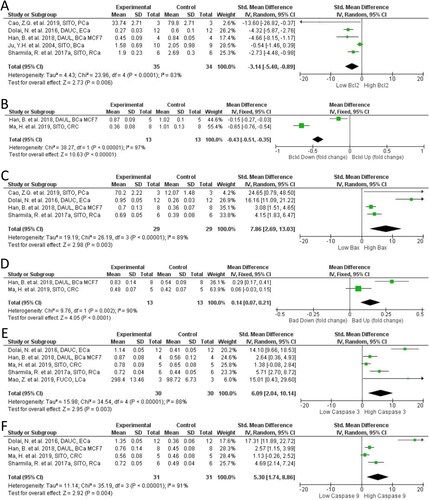
Downstream of mitochondrial membrane integrity regulation, caspase expression also regulates apoptosis. In PSS treated models Casp3 (SMD = 6.09; 95% CI: 2.04, 10.14; p = 0.003; ) and Casp9 (SMD = 5.30; 95% CI: 1.74, 8.86; p = 0.004; ) were both significantly higher than in controls. PSS derivatives appeared important in the magnitude of pro-apoptotic effect observed. Dolai et al., was the only study included using a PSS derivative, DAUC, and evaluated its effect on apoptosis proteins at 50 mg and 100 mg/kg per day IP. A clear dose dependant effect was seen with greater increase in cleaved Casp3 and Casp9, and Bax expression and decrease in anti-apoptotic Bcl2 in the highest treatment group (). Ma et al., established that either DAUC or DAUL at 60 mg/kg OG resulted in a greater Casp3 and Casp9 activation than SITO compared to the control untreated model, which was accompanied by significant decreases in PI3K/Akt signaling that were not present in the SITO group (Ma et al. Citation2019).
Other hallmarks
Proliferation and cell death were the most heavily studied hallmarks identified during the systematic search. Other hallmarks were less extensively studied, yet important discoveries have been made. Four studies measured markers of metastasis or direct metastatic colonization, and three evaluated markers of angiogenesis. PSS treated animals had significantly reduced metastatic colonization (SMD = −1.34; 95% CI: −1.91, −0.77; p < 0.0001; ) from models of PCa (Awad et al. Citation2001), BCa (Han et al. Citation2018), and melanoma (Imanaka et al. Citation2008; Sundstrom et al. Citation2019). Matrix metalloproteinases (MMPs) enzymatic activity facilitate tumor invasion and metastatic process degrading the extracellular matrix (ECM) components, modulating cell adhesion and interfering with the biological activity of ECM components and other proteins. At the molecular level matrix metalloproteinase-2 (MMP2) and matrix metalloproteinase-9 (MMP9) were found significantly reduced in BCa xenograft models (p < 0.00001 for both; ). Expression of Snail, a transcription factor that drives epithelial-mesenchymal transformation (EMT), was significantly reduced by SITO in PaCa BXPC3 xenografts, as were markers of EMT such as vimentin (Cao et al. Citation2018) (). ERK activation is associated with tumor cell angiogenesis and the metastatic EMT and Sharmila and Sindhu (Citation2017b) found SITO reduced pERK. Sundstrom et al. (2019) also observed pERK reduction in brain metastases by SITO which was accompanied by fewer brain metastases in the PSS treated group (Sundstrom et al. Citation2019). Cao and colleagues evaluated GSK3β signaling in the context of metastasis formation and EMT in a PaCa xenograft model (Cao et al. 2018). SITO given daily by IP at 80 mg/kg significantly reduced E-cadherin and increased vimentin. The angiogenesis factor VEGF was reduced in the tumors of PSS treated mice, compared to controls, as observed in two separate BCa models (4T1 and MCF7), treated PO with 50 mg/kg and 100 mg/kg of DAUL (Han et al. Citation2018), and in a RCa model, treated with 20 mg/kg PO of SITO (Sharmila and Sindhu Citation2017a) (p < 0.0001; ). Furthermore, STIG was shown to reduce CCA CD31+ vessels, suggesting a disruption in tumor blood vessel formation (Kangsamaksin et al. Citation2017) but not by a SITO:STIG:CAMP mixture in MMTV-PyMT Tg mice (Llaverias et al. Citation2013) (). Furthermore, only two studies were focused on tumor-promoting inflammation hallmark. Lipid peroxidation (LPO) is a mechanism that induces onset and progression of carcinogenesis through the production of reactive compounds like malondialdehyde (MDA). Ali et al. (Citation2015) and Nguedia et al. (Citation2020) showed that DMBA induced LPO, measured through MDA levels, is significantly reduced by STIG or DAUC relative to controls in mutagenic models of skin papilloma and breast cancer, respectively. However, while STIG increased the levels of superoxide dismutase (SOD), catalase (CAT) and the reduced form of glutathione (GSH), which are essential for reactive oxygen species (ROS) catalysis, DAUC increased only CAT activity (Nguedia et al. Citation2020), suggesting a weaker ability to induce endogenous antioxidant mechanisms compared to STIG.
Figure 6. Forest plot of metastasis and metastasis markers after plant phytosterols and stanols administration. (A) Mean difference in change between PSS treatment and control of metastasis number. (B) Mean difference in change between PSS treatment and control of MMP2. (C) Mean difference in change between PSS treatment and control of MMP9. (D) Standard mean difference in change between PSS treatment and control of VEGF.
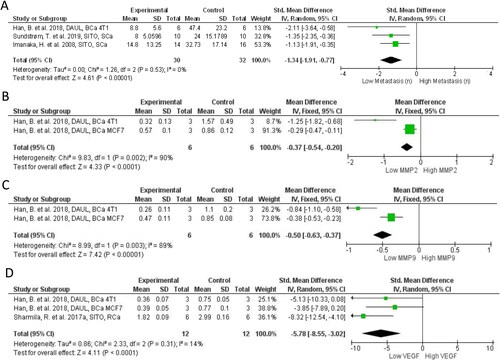
Broad impact oncogenes
We found a number of oncogenes and tumor suppressor genes were evaluated as secondary endpoints in numerous studies. In vitro, PSS have been found to supress activity of AKT and NFκB pathways, and to act as PARP inhibitors, and these roles are evaluated here.
Excessive pAKT leads to enhanced tumor growth, resistance to death signals, metastasis, and angiogenesis (Revathidevi and Munirajan Citation2019). Our meta-analysis of 3 pAKT studies (n = 42 animals) indicated that pAKT levels were 45% lower in PSS treated groups (95% CI: −67%, −23%; p < 0.0001; ). In our qualitive assessment, we noted that PI3K (an oncogene on the same pathway as AKT) was also downregulated (Han et al. Citation2018) and hypophosphorylated in PSS treated animals (Ma et al. Citation2019) (). Phosphorylation of NFκB’s regulatory and transcription factor subunits was reduced by PSS in BCa and PaCa models. In BCa xenograft DAUL (100 mg/kg day; 7.5 g equ. human dose) reduced phosphorylation of IKKα/β, IkBa, and p65 (Han et al. Citation2018). In mammary gland extracts of the genetic BCa PyMT Tg model PSS treatment significantly impaired NFκB activity (Llaverias et al. Citation2013) under high fat diet conditions (). In the PaCa model, signaling by Nrf2-ARE was not altered by SITO, but NFκB activity was reduced. However, as reported earlier for proliferation, the CAMP rich high dose PSS mixture indicated that phytosterols given at high doses (20 mg per mouse per day) did not lead to suppression of oncogene expression or function (EGFR, b-catenin, cycline D1 or pERK) (Marttinen et al. Citation2014). Furthermore, the sister paper published the year before indicated that high phytostanol (8 g/kg dw; 20 mg/day/mouse; 92% CAMS, 8% STAN) led to significant increases in pro-proliferative proteins including EGFR and Cyclin-D1 (Marttinen et al. Citation2013).
Figure 7. Forest plot of pAKT and PARP expression after plant phytosterols and stanols administration. (A) Standard mean difference in change between PSS treatment and control of pAKT. (B) Standard mean difference in change between PSS treatment and control of nuclear PARP. (C) Mean difference in change between PSS treatment and control cleaved PARP.
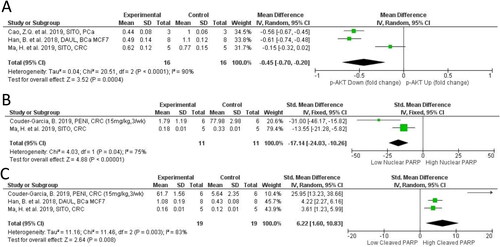
PARP is over expressed in many cancers and is a therapeutic target in the treatment of several cancer types with the use of PARP inhibitors. Our meta-analysis of 2 studies found nuclear PARP to be significantly reduced by PSS (SMD = −17.14; 95% CI: −24.03, −10.26; p < 0.0001 ) (Couder-García et al. Citation2019; Ma et al. Citation2019) and cytoplasmic/cleaved PARP significantly increased (SMD = 6.22; 95% CI: 1.60, 10.83; p = 0.008 ) (Han et al. Citation2018; Couder-García et al. Citation2019; Ma et al. Citation2019). HCT116 cells are Ataxia-Telangiectasia Mutated (ATM)-deficient indicating a sensitivity to DNA repair inhibiting drugs. Couder-Garcia and colleagues provided the only study that considered both dose and frequency of administration of PENI in mice xenograft model using HCT116 (). Interestingly PARP cleavage was strongly induced, and to a similar extent in both the frequent administration group (15 mg/kg 3 per week: 10.9-fold) and the high dose group (30 mg/kg once per week: 10.6-fold) (Han et al. Citation2018; Couder-García et al. Citation2019; Ma et al. Citation2019). As this study varied the frequency and dose of PENI administration it provided valuable information on how administration regimen should be considered in translational first-in-human studies.
The data described here suggests that PSS, particularly the glucoside derivatives of SITO, may be natural PARP inhibitors useful in the treatment of cancers characterized by mutations in DNA repair genes such as BRCA1/2. SITO, DAUC, and DAUL all influenced PARP activity by promoting significant decreases in nuclear (active) PARP, and increases in its cleaved (inactive) form.
Mechanistic insights
Several mechanistic insights into how PSS may alter proliferation of tumor cells were provided during the systematic review and details are reported in . Proliferation of estrogen receptor positive breast cancer MCF7 xenografts was inhibited by SITO provided in chow at 9.8 g/kg dw. Interestingly, SITO treatment led to 35% lower circulating levels of exogenously introduced estradiol, suggesting the antiproliferative actions of PSS could have been indirect via promoting estradiol clearance (Ju et al. Citation2004). Mitochondrial function was also found significantly impaired by SITO in a model of melanoma brain metastasis. In this study the authors discovered that mitochondrial membrane integrity was impaired by SITO and this led to oxidative stress mediated apoptosis (Sundstrom et al. Citation2019). The high PSS dose studies performed in the Apcmin mouse found increases in activity (phosphorylation) and expression of pro-proliferative oncogenes including EGFR, and ERK1/2. Activation of the same proteins, and others (c-jun, c-fos, JNK, and p38) in a KCa model was significantly reduced by prolonged exposure (44-weeks) to SITO (20 mg/kg PO 3 times/week) (Sharmila and Sindhu Citation2017b). These dose dependent differences in oncogene activation indicate a potential non-linear relationship, and activity and expression of such tumor markers should be assessed in clinical trials.
Evaluation of methodology
Heterogeneity
As expected, high levels of heterogeneity (I2>75%) were observed in the majority of our meta-analyses—all of which contained < 10 studies for each outcome. However, we also demonstrate consistent directionality of effects between studies within each meta-analysis. This suggests that despite inter-study differences in experimental design that underlie the high levels of heterogeneity, the administration of PSS consistently confers protective effects against the hallmarks of cancer. Although the present study was not powered to investigate sub-groups across most analyses, future studies may be adequately powered to identify key experimental features that drive heterogeneity.
Risk of bias and adherence to guidelines for reporting on natural products, animal research, and immunoblotting
According to BJP and PROSPERO guidelines for declaration of transparency and scientific rigor (BJP Citation2018a), animal research (BJP Citation2018b), use of natural products (BJP Citation2020), and use of immunoblotting and immunohistochemistry (BJP Citation2018c) we developed a 57 point survey () that was completed in duplicate by two independent researchers. Four papers did not report ethical approval for their research and corresponding authors did not respond via contact details provided in the manuscripts. Using our selection criteria, we had low risk of bias in terms of reporting study design (). Low ROB was also found for PSS origin, purity and measurement methods as these criteria were used to exclude manuscripts that did not report these characteristics, and/or evaluated effects of plant/food extracts rather than pure PSS. Few records evaluated PSS toxicity, pharmacokinetics, dosage rationale, or compared to clinically effective drug (). However, given the long-term use of PSS in the cardiovascular disease setting, these characteristics have been reported extensively elsewhere. Moreover, antibody validation was not reported in any study, immunoblots were always cropped (). We do not see this as a particular limitation here as antibodies against common oncogenes such as pAKT and VEGF have been extensively published previously.
Conclusions
SITO is the most common phytosterol found in foods and we found SITO was strongly associated with the inhibition of several cancer hallmarks including: resisting cell death, sustaining proliferative signaling, inducing angiogenesis, and activating invasion and metastasis (Graphical Abstract). SITO is abundant in healthy diets, especially in many vegetable oils (500 mg sterol per 100 g oil), cereals (50 mg/100g), and nuts (200 mg/100g) (Yang et al. Citation2019). However, given the wide range of PSS available in nature, over 200 different PSS have now been identified and classified (Moreau et al. Citation2018) it may be important to determine whether any of these PSS that are as yet untested as anti-tumor agents, could be better suited. The evidence provided here that SITO at physiologically achievable concentrations significantly impairs tumor development supports the argument that clinical trials should be evaluating its efficacy as an adjunct to existing treatment. If translatable to humans, the evidence presented here suggests that relatively modest (>200 mg) daily PSS intake could impair oncogenic signaling and suppression of multiple cancer hallmarks leading to reduced cancer risk. Indeed a distinction should be made between cancer prevention using dietary advice, and cancer treatment using nutraceutical approaches.
We also have found convincing evidence that PSS may synergise with several existing therapies such as PARP inhibitors (Couder-García et al. Citation2019), gemcitabine (Cao et al. Citation2018), and vemurafenib (Sundstrom et al. Citation2019). However, the cellular receptors for PSS have not been clearly identified in the context of cancer cell biology. Previously, PSS was shown to dampen the effect of oxysterols in breast cancer (Hutchinson et al. Citation2019), and oxysterols are now considered strong mediators of the pathophysiology of several cancers (Baek et al. Citation2017; Segala et al. 2017; He et al. Citation2019). Further mechanistic evidence could be provided by applying emerging technologies such as phage display high throughput screens (Dilly et al. Citation2017) to panels of PSS to identify cellular protein receptors to which PSS directly bind. An alternative mechanism of action is integration into cellular membranes. Disruption of the plasma membrane would impair signaling by oncogenic signaling pathways including AKT (Fakih et al. Citation2018), and disruption of mitochondrial membrane integrity promotes oxidative stress and tumor cell specific apoptosis (Sundstrom et al. Citation2019). Given the well understood toxicity profile of PSS, combined with their now >20-year use in clinic to reduce cardio-vascular disease risk, and the plethora of preclinical in vivo evidence we have summarized here, it is timely to consider PSS as adjuncts to cancer therapies.
Competing interests
No potential conflict of interest was reported by the authors.
Abbreviations | ||
ACF | = | Aberrant Crypt Foci; |
AWH | = | Average weight human (70kg); |
BCa | = | Breast Cancer; |
BRAS | = | Dihydrobrassicasterol; |
CA125 | = | Cancer antigen 125; |
CA153 | = | Cancer antigen 153; |
CA199 | = | Cancer antigen 199; |
CA242 | = | Cancer Antigen 242; |
Cav1 | = | Caveolin 1; |
CEA | = | Carcinoembryonic antigen; |
CCA | = | Cholangiocarcinoma; |
CAT | = | Catalase; |
CGLF | = | Colonic Glands in area of lymphoid follicle; |
CAMP | = | Campesterol; |
CAMS | = | Campestanol; |
CI | = | confidence interval; |
CRC | = | Colorectal Cancer; |
DAUC | = | Daucosterol a.k.a β-Sitosterol glucoside; |
DAUL | = | Daucosterol Linoleate or β-Sitosterol-Glucoside Linoleate; |
DAUN | = | Daucosterol linolenate; |
DAUP | = | Daucosterol Palmitate; |
DU | = | Densitometry Unit; |
dw | = | dry weight; |
EFSA | = | European Food Standards Agency; |
EGFR | = | Epidermal growth factor receptor; |
EMT | = | Epithelial Mesenchymal Transition; |
ER | = | Estrogen Receptor; |
ERK | = | Extracellular-signal-regulated kinase; |
E2 | = | Estradiol; |
ECa | = | Ehrlich-Lettre ascites carcinoma; |
EMT | = | epithelial-mesenchymal transformation; |
FUCO | = | Fucosterol; |
GGT | = | Gamma glutamyl transferase; |
GSH | = | glutathione; |
HFHC | = | High Fat High Cholesterol; |
HEP | = | Hepatoma; |
IP | = | intraperitoneal; |
IV | = | intravenous; |
LCa | = | Lung Cancer; |
LDH | = | Lactate dehydrogenase; |
LDL-C | = | low density lipoprotein cholesterol; |
LFLC | = | Low Fat Low Cholesterol; |
MAPK | = | Mitogen-activated protein kinase; |
MNU | = | N-methyl-N-nitrosourea; |
MDA | = | malondialdehyde; |
MDSC | = | Myeloid-Derived Suppressor Cells; |
n | = | number; |
MMP2 | = | matrix metalloproteinase-2; |
MMP9 | = | matrix metalloproteinase-9; |
NR | = | Not Reported; |
NF-κB | = | Nuclear Factor Kappa Beta; |
NOAEL | = | No Observed Adverse Effect Level; |
OCa | = | Ovarian Cancer; |
OG | = | Oral gavage; |
PaCa | = | Pancreatic Cancer; |
PCa | = | Prostate Cancer; |
PARP | = | Poly (ADP-ribose) polymerase; |
PCNA | = | Proliferating Cell Nuclear Antigen; |
PENI | = | Peniocerol; |
PO | = | per oral; |
PSS | = | Phytosterols and stanols; |
PyMT antigen | = | Polyoma Virus Middle T antigen; |
PSTE | = | Plant Sterol Esters; |
P4 | = | Progesterone; |
RCa | = | Renal Cancer = ROS = Reactive Oxygen Species; |
SCa | = | Skin Cancer or Melanoma; |
SCFA | = | Short Chain Fatty Acids; |
SITO | = | β-Sitosterol; |
STAN | = | Sitostanol; |
STIG | = | Stigmasterol; |
SI | = | Subcutaneous injection; |
SOD | = | Superoxide dismutase; |
TSP-1 | = | Thrombospondin-1; |
VEGF | = | Vascular Endothelial Growth Factor; |
ZGUG | = | Z-Guggulsterone; |
Wei | = | Weight. |
Supplemental Material
Download MS Word (15.2 KB)Additional information
Funding
References
- Ali, H., S. Dixit, D. Ali, S. M. Alqahtani, S. Alkahtani, and S. Alarifi. 2015. Isolation and evaluation of anticancer efficacy of stigmasterol in a mouse model of DMBA-induced skin carcinoma. Drug Design, Development and Therapy 9:2793–800.
- Alrawi, S. J., M. Schiff, R. E. Carroll, M. Dayton, J. F. Gibbs, M. Kulavlat, D. Tan, K. Berman, D. L. Stoler, and G. R. Anderson. 2006. Aberrant crypt foci. Anticancer Research 26 (1A):107–19.
- An, M. J.,J. H. Cheon,S. W. Kim,E. S. Kim,T. I. Kim, andW. H. Kim. 2009. Guggulsterone induces apoptosis in colon cancer cells and inhibits tumor growth in murine colorectal cancer xenografts. Cancer Letters 279 (1):93–100. doi:10.1016/j.canlet.2009.01.026.
- Awad, A. B., C. S. Fink, H. Williams, and U. Kim. 2001. In vitro and in vivo (SCID mice) effects of phytosterols on the growth and dissemination of human prostate cancer PC-3 cells. European Journal of Cancer Prevention 10 (6):507–13.
- Bae, H.,J.-Y. Lee,G. Song, andW. Lim. 2020. Fucosterol Suppresses the Progression of Human Ovarian Cancer by Inducing Mitochondrial Dysfunction and Endoplasmic Reticulum Stress. Marine Drugs 18 (5):261 doi:10.3390/md18050261.
- Baek, A. E., Y. A. Yu, S. He, S. E. Wardell, C. Y. Chang, S. Kwon, R. V. Pillai, H. B. McDowell, J. W. Thompson, L. G. Dubois, et al. 2017. The cholesterol metabolite 27 hydroxycholesterol facilitates breast cancer metastasis through its actions on immune cells. Nature Communications 8 (1):864. doi: 10.1038/s41467-017-00910-z.
- Baskar A. A., S. Ignacimuthu, G. M. Paulraj, and K. S. Al Numair. 2010. Chemopreventive potential of β-Sitosterol in experimental colon cancer model - an In vitro and In vivo study. BMC Complementary and Alternative Medicine. doi:10.1186/1472-6882-10-24. PMID: 20525330.
- BJP. 2018a. Declaration of transparency and scientific rigour: Checklist for design & analysis. British Journal of Pharmacology 175 (13):2709.
- BJP. 2018b. Declaration of transparency and scientific rigour: Checklist for animal experimentation. British Journal of Pharmacology 175 (13):2711.
- BJP. 2018c. Declaration of transparency and scientific rigour: Checklist for immunoblotting and immunohistochemistry. British Journal of Pharmacology 175 (13):2710.
- BJP. 2020. Declaration of transparency and scientific rigour: Checklist for reproducibility of natural product research. British Journal of Pharmacology. 177 (10):2179.
- Borenstein, M., L. V. Hedges, J. P. T. Higgins, and H. R. Rothstein. 2009. Effect sizes based on means. In Introduction to meta‐analysis, 21–32.
- Cao, Z. Q., X. X. Wang, L. Lu, J. W. Xu, X. B. Li, G. R. Zhang, Z. J. Ma, A. C. Shi, Y. Wang, and Y. J. Song. 2018. β-sitosterol and gemcitabine exhibit synergistic anti-pancreatic cancer activity by modulating apoptosis and inhibiting epithelial-mesenchymal transition by deactivating Akt/GSK-3β signaling. Frontiers in Pharmacology 9:1525. doi: 10.3389/fphar.2018.01525.
- Couder-García, B. D. C., N. J. Jacobo-Herrera, A. Zentella-Dehesa, L. Rocha-Zavaleta, Z. Tavarez-Santamaría, and M. Martínez-Vázquez. 2019. The phytosterol peniocerol inhibits cell proliferation and tumor growth in a colon cancer xenograft model. Frontiers in Oncology 9:1341.
- De Stefani, E., P. Boffetta, A. L. Ronco, P. Brennan, H. Deneo-Pellegrini, J. C. Carzoglio, and M. Mendilaharsu. 2000. Plant sterols and risk of stomach cancer: A case-control study in Uruguay. Nutrition and Cancer 37 (2):140–4. doi: 10.1207/S15327914NC372_4.
- Deeks, J. J., J. P. T. Higgins, and D. G. Altman. 2019. Cochrane handbook for systematic reviews of interventions. John Wiley & Sons.
- Deschner, E. E.,B. I. Cohen, andR. F. Raicht. 1982. The kinetics of the protective effect of?-sitosterol against MNU-induced colonic neoplasia. Journal of Cancer Research and Clinical Oncology 103 (1):49–54. doi:10.1007/BF00410305.
- Dilly, S. J., A. J. Clark, A. Marsh, D. A. Mitchell, R. Cain, C. W. G. Fishwick, and P. C. Taylor. 2017. A chemical genomics approach to drug reprofiling in oncology: Antipsychotic drug risperidone as a potential adenocarcinoma treatment. Cancer Letters 393:16–21. doi: 10.1016/j.canlet.2017.01.042.
- Dolai, N.,A. Kumar,A. Islam, andP. K. Haldar. 2016. Apoptogenic effects of β-sitosterol glucoside from Castanopsis indica leaves. Natural Product Research 30 (4):482–5. doi:10.1080/14786419.2015.1023201.
- EFSA Panel on Dietetic Products, Nutrition and Allergies. 2013. Scientific Opinion on the substantiation of a health claim related to the consumption of 2 g/day of plant stanols (as plant stanol esters) as part of a diet low in saturated fat and a two‐fold greater reduction in blood LDL‐cholesterol concentrations compared to the consumption of a diet low in saturated fat alone pursuant to Article 14 of Regulation (EC) No 1924/2006. EFSA Journal 11 (4).
- Fakih, O., D. Sanver, D. Kane, and J. L. Thorne. 2018. Exploring the biophysical properties of phytosterols in the plasma membrane for novel cancer prevention strategies. Biochimie 153:150–61. doi: 10.1016/j.biochi.2018.04.028.
- Han, B., P. Jiang, W. Liu, H. Xu, Y. Li, Z. Li, H. Ma, Y. Yu, X. Li, and X. Ye. 2018. Role of daucosterol linoleate on breast cancer: Studies on apoptosis and metastasis. Journal of Agricultural and Food Chemistry 66 (24):6031–41. doi: 10.1021/acs.jafc.8b01387.
- Han, S., J. Jiao, J. Xu, D. Zimmermann, L. Actis-Goretta, L. Guan, Y. Zhao, and L. Qin. 2016. Effects of plant stanol or sterol-enriched diets on lipid profiles in patients treated with statins: Systematic review and meta-analysis. Scientific Reports 6:31337. doi: 10.1038/srep31337.
- Hanahan, D., and R. A. Weinberg. 2000. The hallmarks of cancer. Cell 100 (1):57–70. doi: 10.1016/S0092-8674(00)81683-9.
- Hanahan, D., and R. A. Weinberg. 2011. Hallmarks of cancer: The next generation. Cell 144 (5):646–74. doi: 10.1016/j.cell.2011.02.013.
- He, S., L. Ma, A. E. Baek, A. Vardanyan, V. Vembar, J. J. Chen, A. T. Nelson, J. E. Burdette, and E. R. Nelson. 2019. Host CYP27A1 expression is essential for ovarian cancer progression. Endocrine-Related Cancer 26 (7):659–75. doi: 10.1530/ERC-18-0572.
- Huang, J., M. Xu, Y. J. Fang, M. S. Lu, Z. Z. Pan, W. Q. Huang, Y. M. Chen, and C. X. Zhang. 2017. Association between phytosterol intake and colorectal cancer risk: A case-control study. British Journal of Nutrition 117 (6):839–50. doi: 10.1017/S0007114517000617.
- Hutchinson, S. A., P. Lianto, J. B. Moore, T. A. Hughes, andJ. L. Thorne. 2019. Phytosterols inhibit side-chain oxysterol mediated activation of LXR in breast cancer cells. International Journal of Molecular Sciences 20 (13):3241 doi:10.3390/ijms20133241.
- Imanaka, H., H. Koide, K. Shimizu, T. Asai, N. Kinouchi Shimizu, A. Ishikado, T. Makino, and N. Oku. 2008. Chemoprevention of tumor metastasis by liposomal beta-sitosterol intake. Biological & Pharmaceutical Bulletin 31 (3):400–4. doi: 10.1248/bpb.31.400.
- Iyer, D., and U. K. Patil. 2012. Efficacy of stigmast-5-en-3β-ol isolated from Salvadora persica L. as antihyperlipidemic and anti-tumor agent: Evidence from animal studies. Asian Pacific Journal of Tropical Disease 2 (Suppl 2):S849–S855. doi: 10.1016/S2222-1808(12)60278-3.
- Jackson, D., and R. Turner. 2017. Power analysis for random-effects meta-analysis. Research Synthesis Methods 8 (3):290–302. doi: 10.1002/jrsm.1240.
- Janezic, S. A., and A. V. Rao. 1992. Dose-dependent effects of dietary phytosterol on epithelial cell proliferation of the murine colon. Food and Chemical Toxicology 30 (7):611–6. doi: 10.1016/0278-6915(92)90195-Q.
- Jiang, P.,B. Han,L. Jiang,Y. Li,Y. Yu,H. Xu,Z. Li,D. Zhou,X. Jia,X. Li, et al. 2019. Simultaneous separation and quantitation of three phytosterols from the sweet potato, and determination of their anti-breast cancer activity. Journal of Pharmaceutical and Biomedical Analysis 174:718–27. doi:10.1016/j.jpba.2019.06.048.
- Jiang, L., X. Zhao, J. Xu, C. Li, Y. Yu, W. Wang, and L. Zhu. 2019. The protective effect of dietary phytosterols on cancer risk: A systematic meta-analysis. Journal of Oncology 2019:1–11. doi: 10.1155/2019/7479518.
- Ju, Y. H., L. M. Clausen, K. F. Allred, A. L. Almada, and W. G. Helferich. 2004. β-sitosterol, β-sitosterol glucoside, and a mixture of β-sitosterol and β-sitosterol glucoside modulate the growth of estrogen-responsive breast cancer cells in vitro and in ovariectomized athymic mice. The Journal of Nutrition 134 (5):1145–51. doi: 10.1093/jn/134.5.1145.
- Kangsamaksin, T., S. Chaithongyot, C. Wootthichairangsan, R. Hanchaina, C. Tangshewinsirikul, and J. Svasti. 2017. Lupeol and stigmasterol suppress tumor angiogenesis and inhibit cholangiocarcinoma growth in mice via downregulation of tumor necrosis factor-α. PLoS One 12 (12):e0189628. doi: 10.1371/journal.pone.0189628.
- Kazłowska, K., H. T. V. Lin, S. H. Chang, and G. J. Tsai. 2013. In vitro and in vivo anticancer effects of sterol fraction from red algae porphyra dentata. Evidence-Based Complementary and Alternative Medicine 2013:1–10. doi: 10.1155/2013/493869.
- Llaverias, G., J. C. Escolà-Gil, E. Lerma, J. Julve, C. Pons, A. Cabré, M. Cofán, E. Ros, J. L. Sánchez-Quesada, and F. Blanco-Vaca. 2013. Phytosterols inhibit the tumor growth and lipoprotein oxidizability induced by a high-fat diet in mice with inherited breast cancer. The Journal of Nutritional Biochemistry 24 (1):39–48. doi: 10.1016/j.jnutbio.2012.01.007.
- Ma, H., Y. Yu, M. Wang, Z. Li, H. Xu, C. Tian, J. Zhang, X. Ye, and X. Li. 2019. Correlation between microbes and colorectal cancer: Tumor apoptosis is induced by sitosterols through promoting gut microbiota to produce short-chain fatty acids. Apoptosis 24 (1-2):168–83. doi: 10.1007/s10495-018-1500-9.
- Mao, Z., X. Shen, P. Dong, G. Liu, S. Pan, X. Sun, H. Hu, L. Pan, and J. Huang. 2019. Fucosterol exerts antiproliferative effects on human lung cancer cells by inducing apoptosis, cell cycle arrest and targeting of Raf/MEK/ERK signalling pathway. Phytomedicine. doi: 10.1016/j.phymed.2018.12.032.
- Marttinen, M., E. Päivärinta, M. Storvik, L. Huikko, H. Luoma-Halkola, V. Piironen, A. M. Pajari, and M. Mutanen. 2013. Plant stanols induce intestinal tumor formation by up-regulating Wnt and EGFR signaling in Apc Min mice. Journal of Nutritional Biochemistry 24 (1):343–52. doi: 10.1016/j.jnutbio.2012.07.002.
- Marttinen, M., A. M. Pajari, E. Päivärinta, M. Storvik, P. Marttinen, T. Nurmi, M. Niku, V. Piironen, and M. Mutanen. 2014. Plant sterol feeding induces tumor formation and alters sterol metabolism in the intestine of Apc(Min) mice. Nutrition and Cancer 66 (2):259–69. doi: 10.1080/01635581.2014.865244.
- McCann, S. E., J. L. Freudenheim, J. R. Marshall, and S. Graham. 2003. Risk of human ovarian cancer is related to dietary intake of selected nutrients, phytochemicals and food groups. The Journal of Nutrition 133 (6):1937–42. doi: 10.1093/jn/133.6.1937.
- Mendilaharsu, M., E. De Stefani, H. Deneo-Pellegrini, J. Carzoglio, and A. Ronco. 1998. Phytosterols and risk of lung cancer: A case-control study in Uruguay. Lung Cancer 21 (1):37–45. doi: 10.1016/S0169-5002(98)00044-0.
- Moreau, R. A., L. Nystrom, B. D. Whitaker, J. K. Winkler-Moser, D. J. Baer, S. K. Gebauer, and K. B. Hicks. 2018. Phytosterols and their derivatives: Structural diversity, distribution, metabolism, analysis, and health-promoting uses. Progress in Lipid Research 70:35–61. doi: 10.1016/j.plipres.2018.04.001.
- Nguedia, M. Y., A. B. Tueche, A. J. G. Yaya, V. Yadji, D. T. Ndinteh, D. Njamen, and S. Zingue. 2020. Daucosterol from Crateva adansonii DC (Capparaceae) reduces 7,12-dimethylbenz(a)anthracene-induced mammary tumors in Wistar rats. Environmental Toxicology 35 (10):1125–36. doi: 10.1002/tox.22948.
- Peter, E. L., A. G. Mtewa, P. B. Nagendrappa, A. Kaligirwa, and C. D. Sesaazi. 2020. Systematic review and meta-analysis protocol for efficacy and safety of Momordica charantia L. on animal models of type 2 diabetes mellitus. Systematic Reviews 9 (1):7. doi: 10.1186/s13643-019-1265-4.
- Phillips, K. M., D. M. Ruggio, and M. Ashraf-Khorassani. 2005. Phytosterol composition of nuts and seeds commonly consumed in the United States. Journal of Agricultural and Food Chemistry 53 (24): 9436–9445.
- Quilliot, D., F. Boman, C. Creton, X. Pelletier, J. Floquet, and G. Debry. 2001. Phytosterols have an unfavourable effect on bacterial activity and no evident protective effect on colon carcinogenesis. European Journal of Cancer Prevention 10 (3):237–43.
- Revathidevi, S., and A. K. Munirajan. 2019. Akt in cancer: Mediator and more. Seminars in Cancer Biology 59:80–91. doi: 10.1016/j.semcancer.2019.06.002.
- Rohatgi, A. 2019. WebPlotDigitizer (v4.2). https://automeris.io/WebPlotDigitizer
- Segala, G., M. David, P. de Medina, M. C. Poirot, N. Serhan, F. Vergez, A. Mougel, E. Saland, K. Carayon, J. Leignadier, et al. 2017. Dendrogenin A drives LXR to trigger lethal autophagy in cancers. Nature Communications 8 (1):1903. doi: 10.1038/s41467-017-01948-9.
- Sharmila, R., and G. Sindhu. 2017a. Evaluate the antigenotoxicity and anticancer role of β-sitosterol by determining oxidative DNA damage and the expression of phosphorylated mitogen-activated protein kinases’, C-fos, C-jun, and endothelial growth factor receptor. Pharmacognosy Magazine 13 (49):95–101.
- Sharmila, R., and G. Sindhu. 2017b. Modulation of angiogenesis, proliferative response and apoptosis by β-sitosterol in rat model of renal carcinogenesis. Indian Journal of Clinical Biochemistry 32 (2):142–52. doi: 10.1007/s12291-016-0583-8.
- Shin, E. J.,H.-K. Choi,M. J. Sung,J. H. Park,M.-Y. Chung,S. Chung, andJ.-T. Hwang. 2018. Anti-tumour effects of beta-sitosterol are mediated by AMPK/PTEN/HSP90 axis in AGS human gastric adenocarcinoma cells and xenograft mouse models. Biochemical Pharmacology 152:60–70. doi:10.1016/j.bcp.2018.03.010.
- Sofi M. S., M. K. Sateesh, M. Bashir, M. A. Ganie, and S. Nabi. 2018. Chemopreventive and anti-breast cancer activity of compounds isolated from leaves of Abrus precatorius L. 3 Biotech. 8(8):371. doi: 10.1007/s13205-018-1395-8.
- Sundstrom, T., L. Prestegarden, F. Azuaje, S. N. Aasen, G. V. Rosland, J. K. Varughese, M. Bahador, S. Bernatz, Y. Braun, P. N. Harter, et al. 2019. Inhibition of mitochondrial respiration prevents BRAF-mutant melanoma brain metastasis. Acta Neuropathologica Communications 7 (1):55. doi: 10.1186/s40478-019-0712-8.
- The Nordic Cochrane Centre, T. C. C. 2014. Review Manager (RevMan) [Computer program].
- Thorne, J. L., G. Cioccoloni, L. Wallis, A. Websdale, C. Soteriou, and M. Zulyniak. 2020. Phytosterols and phytostanols (PSS) and the hallmarks of cancer: A systematic review of evidence from pre-clinical models. PROSPERO: International prospective register of systematic reviews. PROSPERO.
- Vesterinen, H. M., E. S. Sena, K. J. Egan, T. C. Hirst, L. Churolov, G. L. Currie, A. Antonic, D. W. Howells, and M. R. Macleod. 2014. Meta-analysis of data from animal studies: A practical guide. Journal of Neuroscience Methods 221:92–102. doi: 10.1016/j.jneumeth.2013.09.010.
- Wang, X.,M. Li,M. Hu,P. Wei, andW. Zhu. 2017. BAMBI overexpression together with β-sitosterol ameliorates NSCLC via inhibiting autophagy and inactivating TGF-β/Smad2/3 pathway. Oncology Reports 37 (5):3046–54. doi:10.3892/or.2017.5508.
- WCRF/AICR. 2018. Diet, nutrition, physical activity and cancer: A global perspective. Continuous update project expert report 2018. London: World Cancer Research Fund/American Institute for Cancer Research.
- Yaacob, N. S., H. M. Yankuzo, S. Devaraj, J. K. M. Wong, and C. S. Lai. 2015. Anti-tumor action, clinical biochemistry profile and phytochemical constituents of a pharmacologically active fraction of S. crispus in NMU-induced rat mammary tumour model. PLoS One 10 (5):e0126426. doi: 10.1371/journal.pone.0126426.
- Yang, R., L. Xue, L. Zhang, X. Wang, X. Qi, J. Jiang, L. Yu, X. Wang, W. Zhang, Q. Zhang, et al. 2019. Phytosterol contents of edible oils and their contributions to estimated phytosterol intake in the Chinese diet. Foods 8 (8):334. doi: 10.3390/foods8080334.
- Zhao, C.,T. She,L. Wang,Y. Su,L. Qu,Y. Gao,S. Xu,S. Cai, andC. Shou. 2015. Daucosterol inhibits cancer cell proliferation by inducing autophagy through reactive oxygen species-dependent manner. Life Sciences 137:37–43. doi:10.1016/j.lfs.2015.07.019.