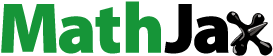
Abstract
Heat resistant chocolate (HRC) which can retain the desired texture and mouthfeel in tropical and subtropical climatic conditions has become a major research area in the chocolate industry. Liking of the chocolate products keeps on changing with the geographical conditions of the world due to the availability of ingredients from local resources and consumer’s taste preferences. The geographical changes also bring about the change in climatic conditions and as such no chocolates have been formulated to withstand the hot tropical or sub-tropical temperature conditions. Textural issues and various storage related problems faced due to meltability of chocolate in different countries has opened up a broad research field of sustainable HRC manufacturing. Over the years, there are broadly three different approaches (fat modification, sugar structure modification and innovative process approach) to develop the HRC and all these scientific approaches have given different scientific insights about improving the heat resistance characteristics and textural stability of chocolate. There is a lack or coordinated fundamental and applied research related to cocoa butter polymorphism, and thermal-textural issues during product development/storage. This review paper is an attempt to describe the different scientific approaches for developing HRC and how they affect the physical/sensory chocolate attributes.
Introduction
Heat Resistant Chocolate (HRC) is a chocolate that is resistant to heat, i.e. does not melt at the usual temperature of 33-34 °C and can retain its structure, shape and snap even at higher temperatures without losing its textural properties. Interestingly, the specific distribution in cocoa butter of native dietary TAGs is responsible for the characteristic melting point of chocolate (Karupaiah and Sundram Citation2007) which is 33.8 °C (DeMan Citation1999). HRC is considered to be special than the usual chocolate products present in the world, to be used specifically in the tropical and sub-tropical countries directly as well as for enrobing and coating the edible centers. It is defined as a chocolate that can resist adhering to wrappers at temperature exceeding 30 °C, maintain shape at temperature above 35 °C and not stick to hands at 40 °C (Schenk and Peschar Citation2004; Beckett Citation2008; Best et al. Citation2005). Many of these countries are the growing markets for the chocolate industry since a large population of the world lives in these countries. Optimally, an ideal HRC will be the one that has a balance of temperature in such a way that it does not lose its shape and structure at elevated temperatures but should have the desired meltability character in the mouth to stimulate the normal oral sensation of chocolate. Over the years, it has been seen that the usual trend in the early 2000s was more done by chocolate research and development wings of major companies. Industries such as Hershey’s, Mars, Mondelez etc. tried to find the technical solutions where the heat resistance problems of chocolate can be addressed. Furthermore, their research groups provided much more of an industry oriented approach for imparting the heat resistant to chocolate. But understanding the scientific principles behind the chocolate polymorphism behavior or meltability issues, were not studied in depth. This created a gap between the industrial and scientific research done in this field. The HRC research approaches were done in a specific piece meal approach and not in a collective/collaborative approach taking the fundamental scientific understanding to realistic technical solution. Thus in the later part of 2000s, it was seen that the scientific approach behind using a specific ingredient or a processing technique was addressed directly or indirectly by a number of research groups. This review paper is an attempt to understand the scientific rationale behind different scientific approaches for development of HRC.
Chocolate production and consumption
The global chocolate industry is worth more than $103 billion (Demarest, Citation2019). The global cocoa production for the year 2019–20 was about 4697 thousand tons (ICCO, Citation2020). Cocoa trees need high temperatures (usually between 21 °C and 23 °C), humid conditions, plenty of rainfall (1000-2500 mm rainfall per year), nitrogen-rich soil, and protection from wind to grow successfully (Afoakwa Citation2010; NOAA, 2016). This is the reason cocoa is produced mainly in hot and humid regions of Africa, Asia, Central and Southern America and Australia (). The top cocoa producing countries come from West Africa-the Ivory Coast (2180 thousand tons, 30% of the world’s cocoa) and Ghana (about 812 thousand tons) followed by Indonesia (about 784 thousand tonnes), Nigeria (350 thousand tonnes), Cameroon (280 thousand tonnes), and Brazil (260 thousand tonnes) () (FAOSTAT Citation2019; Cocoa Producing Countries, Citation2020). Netherlands is the world’s top cocoa importer followed by U.S.A. and Germany (ICCO, Citation2020). Switzerland also has the highest per capita rate of chocolate consumption in the world followed by Austria, Germany, Ireland etc. (ICCO). It can be seen that none of the major chocolate consuming countries are the major producers of cocoa beans and vice versa.
Figure 2. Statistical distribution of cocoa production around the globe and the share of the world’s top cocoa bean producing countries (Data source: ICCO 2017).
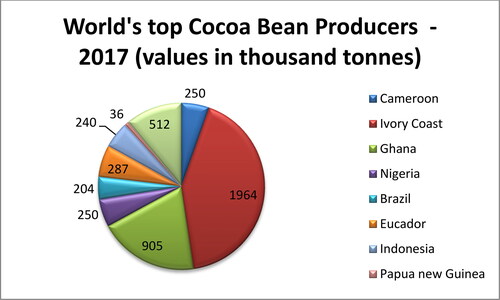
Although maximum per capita chocolate consumption happens in the northern hemisphere (North America and Europe) it is mostly grown in the tropical conditions in countries which are undergoing tremendous environmental and ecological stress in cocoa production due to climate change. The impact of climate change such as increasing temperature and decreasing rainfall leads to reduced soil fertility and increased water scarcity, both of which are detrimental to the crop production. Further, unsustainable agricultural practices expand and amplify the impacts of climate change by reducing forest covers (up to 85%) and degrading forest ecosystems with more disease infestations (FAO, 2020). There are several other social, economic and environmental factors like child labor, poverty, lack of transparency between the government and the industry, cocoa price volatility, deforestation, biodiversity loss etc. affecting the cocoa industry (French Development Agency and Barry Callebaut Study Citation2017).
Science of chocolate
The science behind chocolate mouthfeel is a complex mechanism of its melting characteristics that comes from the property of polymorphism of fat. This meltdown phenomena or desirable mouthfeel character of the chocolate in mouth is inter-connected with the sensory appreciation and flavor perception. The brittle snap of chocolate at room temperature and its quick melting properties in the mouth releasing its maximum flavor are due to the rather narrow melting range (30–36 °C) of Cocoa Butter (CB). CB also has a narrow plastic range, having high solid fat content of more than 70% at 10 °C and of 0% at 37 °C. This distinguishing character allows the chocolate incorporated with CB to possess exclusive sensorial properties in which the chocolate exists as solid state when held in hands but melts rapidly in mouth when consumed (Jahurul et al. Citation2013; Mohamed Citation2012). There are about 600 volatiles and partially odor active compounds present in cocoa and chocolate. This unique assembly of flavor volatiles which are mainly present in cocoa fat interact with each other to develop a pleasant and unique sensory experience (Hoskin and Dimick Citation1984; Carlin et al. Citation1986; Ziegleder and Biehl Citation1988; Ziegleder Citation1991; Nijssen et al. Citation1996; Schnermann and Schieberle Citation1997). The particle size distribution, the viscosity, and hardness of chocolate dispersion determine the perceived taste of the final chocolate product. The characteristic pleasant mouthfeel is produced by the melting behavior of cocoa butter (CB) because of its relatively simple composition being highly polymorphic.
Understanding the fat phase of chocolate
Processed chocolate is majorly composed of its natural fat, i.e. cocoa butter (CB). Modified fat phase of chocolate is a potential route for HRC development. Thus, it is important to understand CB and its chemical composition. CB mainly consists of triglycerides of three main fatty acids: oleic acid (O; unsaturated), stearic acid (St; saturated) and palmitic acid (P; saturated). When these fatty acids are linked through a glycerol backbone, they form triglycerides (TAG) such as POSt, StOSt, and POP, etc. Generally, SOS is used to majorly represent the symmetrical mono-unsaturated CB triglyceride composition (S: any saturated fatty acid, either palmitic or stearic).
CB has a high amount of mono- unsaturated fats (SOS crystals) which are solid at room temperature. It also has about 1–2% of all saturated solid fats (the SSS crystals) which melt at a much higher temperature than the usual SOS crystals due to the presence of unsaturated fat (O: Oleic) in the middle. It also contains liquid SOO crystal fats in 5–20% amount (due to two oleic acid molecules). These different combinations of fatty acids are responsible for the formulation of CB mix and its melting characteristics. The resultant fat is partially liquid at room temperature. Microscopic investigation of CB revealed that it is composed of a mass of tiny crystals in a matrix of liquid fat (due to SOO crystals). In the tropical climatic conditions, the fat is always in warm temperature zone; therefore number of solid crystals distributed through the liquid fat diminishes increasing the amount of liquid, leading to softening of fat and loss of textural hardness.
When CB is melted and then cooled, the three types of triglycerides behave differently. The SSS type crystallize first, making the chocolate thicker as there is less liquid fat present, but due to the higher percentage of SOS crystals present in CB, they finally determine the chocolate texture and resistance to fat bloom. SOO crystals only affect in the determining the melting profile of the chocolate over a range of temperature (Garti and Widlak Citation2012).
The development of HRC is strongly associated with the crystallization and polymorphic behavior of the fat phase of chocolate. Polymorphism is the ability of a molecule to crystallize in several different crystal packing configurations (Loisel et al. Citation1998). The main reason for this is that different fat molecules can fit together in a different number of ways. CB can crystallize in six different forms, in the following order: ϒ→α→β2'→β1'→β2→β1 (these are arranged from least to most stable forms, ). Traditionally they are designated from I to VI of which form V was considered to be the most stable form imparting glossy appearance, good snap and bloom resistance to the chocolate (Beckett Citation2008). The behavior of the β' form of SOS crystals is of particular importance in chocolate since it has a slightly lower melting temperature (33.8 °C) than that of the human mouth and is responsible for the physically driven sensory sensations and mouth-feel (DeMan Citation1999).
Figure 3. Temperature regimes and degree of stability of six polymorphic forms of cocoa butter (Adapted from: Beckett 2008).
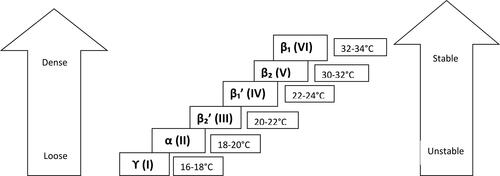
The formation of high melting forms (form V or β’ form) can be produced by controlling the temperature of solidification (tempering process). The crystalline structure of CB is also responsible for the transition from a semi-hard material to melted liquid product at the mouth temperature. For a perfect mouthfeel, it is necessary to temper chocolate to crystallize CB in polymorphic form V, more ordered in crystal packing structure than any other form, being packed in a triple chain system. In controlled crystallization, all three major groups of triglycerides crystallize from CB through the process of nucleation. The chocolate contains a small but significant amount of long-chain trisaturated triglycerides (SSS) which start to crystallize first from the molten mass, increasing the overall chocolate viscosity. Then the SOS triglycerides begin to crystallize, which are responsible for seeding the bulk of chocolate in polymorphic stable form V, affecting the overall chocolate melting profile. The SOO triglycerides remain liquid at room temperatures (Beckett Citation2000).
Sensory character and flavor perception of chocolate
Chocolate has a distinct set of sensory characteristics which are responsible for the choice and acceptability by the consumers. This sensory appreciation is generally influenced by flavor precursors when consumer tastes chocolate and evaluates the eating quality in terms of characteristics such as appearance, taste, mouthfeel, and after-taste. The chocolate flavor is a complex combination of olfactory, gustatory and trigeminal sensations perceived during consumption which comes from the volatile flavor compounds present in it. These compounds are perceived when released into the fat phase and are consequently vaporized and detected as they pass from the gustatory to olfactory space during mastication. To give a ‘melt in the mouth’ characteristic to chocolate, it melts at the body temperature of 37 °C during consumption, giving a smooth suspension of particulate solids (sugar, cocoa and nonfat solids) in fat. As the chocolate melts, the continuous fat phase goes though inversion to a continuous water phase and mixes with saliva, which acts as a solvent for the sugar particles and coats all particle surfaces to facilitate swallowing. When chocolate melts between the tongue and palate, it is subjected to high shear forces resulting in coalescence of fat droplets, wetting the mucosal surface with oil, increasing the surface area available for volatilization and allowing more intimate contact between the oil and taste receptors (Prinz and de Wijk Citation2004). The ease with which chocolate can be melted and manipulated in the mouth depends on the chocolate viscosity which in turn depends upon the careful combination of sugar and fat as well as moisture present in the chocolate. It has been reported that several aroma compounds undergo chemical reactions during mastication and contact with saliva in the mouth, enhancing the sensory quality of chocolate (Dimick and Hoskin Citation1994; Counet et al. Citation2002; Granvogl, Bugan, and Schieberle Citation2006; Ramli et al. Citation2006). Waxy mouthfeel comes when fat remains solid even after 37 °C. Also, post-hardening is one of the major step for the sensory characteristics to develop in chocolate, which not only improves the hardness but also increases the solid fat content at 35 °C in cocoa butter replacer (CBR) based chocolates (Talbot Citation2009).
Problems associated with chocolate: need for HRC
There are various quality issues associated with the melting characteristics of chocolate. Bloom is considered as one of the most pronounced defects responsible due to improper storage and transportation conditions. Sugar bloom occurs when water contacts chocolate and dissolves the sugar present in chocolate. Upon evaporation of water, this dissolved sugar re-crystallizes on the surface of chocolate as a thin film, which affects the overall appearance of the product. Sugar bloom also gives an unpleasant, rough and grainy mouth-feel to the product. Another category is the fat bloom, which comes into the picture due to the growth of the solid fat phase. The polymorphic form V of CB gets transformed to form VI on prolonged storage and in altering temperature conditions, this transformation is associated with the development of large crystals at the chocolate surface which scatters light and gives a whitish appearance to chocolate. Another issue associated with chocolate manufacture is seizure. It occurs when small amounts of water or polyol are incorporated into molten chocolate. The water dissolves some of the sugar molecules which then become sticky and bind to various particles in chocolate preventing flow and resulting in an unusable solid-like chocolate mass (Figoni Citation2007, Marangoni and Garti Citation2011). As several low and middle-income countries fall in tropical and subtropical climate conditions, the availability of proper storage conditions in the rural parts of these countries is scarce. These issues are therefore more common in chocolates in the tropical and subtropical countries since the temperature alterations are enhanced at a large scale due to tropical/subtropical climate as well as storage conditions across the supply chain. The application of CB from natural sources is limited by natural factors such as low CB yield as affected by climate and environmental change, causing issues with supply shortages in manufacturing (Biswas et al. Citation2017).
Apart from these above-mentioned issues faced due to improper processing or storage of chocolate, the product does not remain suitable for consumption in tropical countries as such because of the complex structural chemistry it has. The smearing of fat over the sugar surface so that particles can flow past one another, the setting of the proper polymorphic form of CB and the necessary removal of moisture from the emulsion are all the important factors responsible for giving the chocolate its proper taste and texture profile. In tropical and sub-tropical weathers, the temperature is as high as the melting temperature of CB. In that case, the proper emulsion structure formed in chocolate is not able to retain its shape since the fat gets oiled off or melted, thus not strong enough to hold onto the sugar matrix. The product can further develop the issues of contamination by the addition of water/moisture in the melted chocolate mass and loses its palatability. This phenomenon generally leads to dissatisfaction amongst the consumers and the common problem of chocolate in the supply chain.
Heat resistant chocolate has not been well studied scientifically apart from a review paper of Stortz and Marangoni (Citation2011). There are only two thesis works (Stortz et al. Citation2014 and Laughter Citation2013) and a short review paper by Ogunwolu and Jayeola (Citation2006) in the field of heat resistant chocolate. Stortz and Marangoni (Citation2011) studied the various methods developed till 2011 and segregated the process of manufacture of HRC in three main approaches: enhancement of the network microstructure of materials, the addition of polymer and increasing the melting point of the fat phase. Their review paper was an excellent attempt to document the scientific developments in theoretical as well as industrial research related to HRC till that time. But there was limited documentation of sensory studies of HRC in all of these research studies. Also, there have been newer developments in the field in the last decade. Thus, this review is done to bridge the gap between the theoretical and industrial research, to segregate and identify the scientific principles responsible for different approaches for development of HRC. This knowledge is important for the industry to manufacture the HRC product on a larger scale keeping consumer preferences in mind.
In this review paper, the preparation of HRC can be categorized into: (1) fat modification, (2) sugar modification and addition of polyol and (3) process modification (). The fat modification technique involves the addition of fat alternatives with CB in various proportions to increase the melting point of the whole azeotropic mixture. It also includes the formation of oleogels for gelation of existing oil with the help of single or combined gels/resins to form a structural framework of solid (saturated) oils in which the liquid oil gets entrapped and preventing it to ooze out. Sugar modifications involve the creation of sugar network by water incorporation (direct and indirect methods) and processing in such a way that some sugar surfaces remain uncoated by fat (Stortz and Marangoni Citation2011). The addition of polyol includes the use of polyol with a thermal structuring component (usually saccharides) to enhance the heat resistant structure of chocolate. Finally, process modification involves the application of high pressure on the modified particulate components to obtain a chocolate tablet and particle size modification of the composition of chocolate. The general fall-point of these techniques is in the final palatability and mouthfeel of the product. In creating HRC, the final delectability is still a matter of question of industrial and academic research since no product has been launched in the market. Also, the ingredient modifications would be limited by the various standards of chocolate identity in different countries.
Manufacturing of heat resistant chocolate (HRC)
There is limited academic and theoretical research in the field of HRC. The methods of manufacturing of HRC are broadly categorized into three approaches ():
Fat modification
When two or more fats are mixed, it is important that the final chocolate sets at a suitable rate and has the correct texture and melting properties in the mouth. Recent studies show that well-defined mixtures of triacylglycerols can be used to control crystal structures, polymorphism and melting temperatures (Silva et al. Citation2014). Melting behavior is important for flavor release, as fat acts as a transmission medium for chocolate flavor and it must melt before the flavor actually becomes available (ADM (Archer Daniels Midland) Cocoa Citation2009). The cocoa nib usually consists of about 55% of CB, which constitutes around 30% in the final chocolate. CB triglycerides generally have saturated fatty acids at sn1 and sn3 position and oleic acid in the sn2 position. Slip melting point of CB is 26.7–27.9 °C (Jin, Mu, et al. Citation2017). Some vegetable fats having similar triglyceride composition as CB are used in chocolate formulations mainly categorized as: cocoa butter equivalents (CBEs), cocoa butter substitutes (CBSs) and cocoa butter replacers (CBRs) (Depoortere Citation2011).
CBEs are miscible in any proportion with CB. Legally, such vegetable fats are permitted up to 5% in the EU for a product to be considered as chocolate under Cocoa and The Cocoa and Chocolate Products (England) Regulations (Citation2003). Vegetable oils such as palm oil can be used to extract POP or POSt fats by removal of easily melting (olein) and hardest melting (stearin) fractions by fractionation. Two types of fractionation are used to extract different triglyceride fractions: dry fractionation and solvent fractionation. In dry fractionation, fat is heated to a pre-defined temperature and then the liquid part is separated from a solid part by filtering or pressing. In solvent fractionation, fat is dissolved in acetone or hexane. The higher melting triglycerides are then allowed to crystallize and filtered out. Natural fats like shea butter, mango kernel oil, and sal oil are used for obtaining fractions rich in StOSt rich fat fractions. The addition of higher amount of POP fat in the blend causes a softer CBE formation. On the other hand, if high amount of StOSt rich fats are incorporated, the CBE will have higher melting point and can increase the hardness of CB, conferring more heat resistance with little or no waxiness in the mouth (). This type of fat is known as cocoa butter improver (CBI) which is observed to be generally used for the manufacture of HRC.
Figure 5. Ternary diagram showing the melting points for mixtures of the main cocoa butter TAG. (Adapted from Garti and Widlak Citation2012).
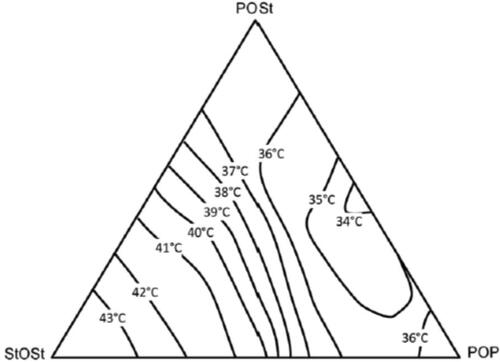
CBRs are the lauric fats that crystallize only in one crystal form (β'), in a very different way than CB but have a similar texture and mouthfeel as CB. Palm kernel oil and coconut oil contain about 50% lauric acid and are used as CBRs (Talbot Citation1999). It has been found out that lauric CBRs can be used only when very little CB is present in the chocolate composition and are thus used for coatings.
CBSs are non-lauric fats that can be used with CB in some amounts due to the limited compatibility (Babin Citation2005). This is because in these vegetable fats, although the fatty acid composition is the same, they are far more random than in CB e.g. oleic acid is generally present in 1 or 3 positions and elaidic acid (trans) is present in large amounts than CB. They set in β form and do not need tempering for proper crystallization.
To understand how fat modification affects melting characteristics of chocolate, Lonchampt and Hartel (Citation2004) studied the effect of amount and composition of fat present in chocolate on the crystal size, polymorphism and crystallization rate in chocolate products. Several crystals and range of sizes, shapes and polymorphic stability, as well as arrangement in network structures, indicated the role of fat modification on the mechanical and rheological properties of chocolate. Afoakwa, Paterson, and Fowler (Citation2008) demonstrated that chocolate with lower fat content required a longer time to melt than similar products with higher fat content. Fat fills voids between particles in molten chocolate and reduces resistance to flow, with a direct relationship between fat content and enthalpy of melting. The enthalpy is reduced in products of lower fat contents. It has been demonstrated that fat content has the greatest influence on melting characteristics in chocolates (Afoakwa, Paterson, and Fowler Citation2008). Also, it has been shown that the kinetics of crystallization of unseeded and seeded cocoa butter plays an important role in the selection of crystal to produce a better chocolate mouthfeel. Further, the crystallization temperature and time define the resulting crystal form of fat, the melting temperature and thus the mouth-feel (Marangoni and McGauley Citation2003) in chocolate. Chocolates exhibit certain textural attributes that are dependent on the structure, concentration and interaction among the fat crystals (Rios et al. Citation2014). To obtain perfect quality parameters for excellent chocolates, the pathway from liquid to solid phases needs to be controlled in detail to produce a desired HRC formula.
Fat modification involves two techniques: increasing the melting point of fat and entrapping of liquid fat in solid fat matrix (organogelation). Increasing the melting point of fat can be done by interesterification or by the addition of fat with a higher melting point (which may be modified further by seeding). Entrapping involves the use and formation of oleogels manufactured from different sources to create a solid matrix around the entrapped liquid fat.
Increasing the melting point of fat
This can be done either via interesterification of fat phase/addition of interesterified fats or by addition of fats for structural modifications.
Inter-esterification of the fat phase
This method was devised to obtain harder fats from natural vegetable fats to be used as CBIs. Inter-esterification is the process for obtaining slightly more saturated (solid) fats by the reaction between different triglycerides with a re-arrangement of the acyl groups within and between the triglycerides (). It alters the melting point of fat. In addition, it is also used to improve the plasticity of resulting solid by changing the (re)crystallization properties. This process can be directed to a certain extent, e.g. by segregation of the newly formed, high melting esters from reaction mixture through controlled crystallization. It is known that sn-StOSt and sn-POP have the characteristics of high solid fat content at low temperature (10–15 °C), enable shape retention and rapidly melt in the mouth when consumed (35–40 °C) (Jahurul, Zaidul, Nik Norulaini, Sahena, Kamaruzzaman, et al. Citation2014; Zhang, Song, et al. Citation2020).
Figure 6. Effect of chemical (or random) interesterification of a mixed TAG on a TAG composition (Adapted from Garti and Widlak Citation2012).
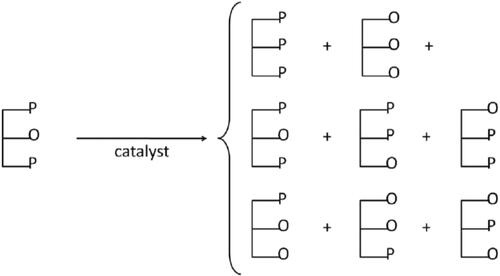
Unilever used a similar approach (Macrae and Bench Citation1983) of upgrading palm mid- fraction (PMF) as a CBE. PMF (unsaturated fatty acid: saturated fatty acid – 0.93)) has too little amount of stearic acid, the deficiency was fixed by enzyme-catalyzed acidolysis with stearic acid subjected only to the 1 and 3 positions of triglycerides ((Aini and Miskandar Citation2007; Gunstone Citation2004). Loders-Croklaan later created a product called Betapol closely mimicking the structure of human milk fat, created by enzymatic action of sn-1, 3 specific lipases such as Rhizomucor Meihei under anhydrous conditions (Kuo and Gardner Citation2002). The free stearic acid was esterified onto the 1 and 3 positions, where original fats were removed by suitable enzyme. The central oleic acid remained untouched, so that fat became very rich in StOSt fats. It was compatible with CB and thus could be used in chocolate manufacture (Gunstone Citation2004).
There have been further academic developments in the field of finding substituted interesterified fats (CBEs) with higher melting points that can be added to chocolate compositions to produce heat resistant properties. Oleic acid containing mango kernel oil, palm olein, refined olive pomace oil, sunflower oil, olive oil as well as CB itself has been esterified at the central position of the glycerol backbone to be used as CBEs. Also, oils such as illipe butter, with higher stearic acid content than CB (Bahari & Akoh, Citation2018; Illiyin et al. Citation2013) were also considered as CBEs. Adding partly interesterified CB also increases the melting point of the chocolate. A brief description of the study of interesterified fats to be used as CBEs is given in the following .
Table 1. Use of interesterified fats as coco butter equivalents (CBE).
Although interesterification is a novel approach to create fats that can be used to impart heat resistance properties if added to chocolate, but the process of interesterification is prohibited by the European Union and is thought to exert a negative effect on human health. However, the ability of the methods to use immobilized enzymes offered a way to manage with the cost- effectiveness (Fennema, Damodaran, and Parkin Citation2008; Stortz and Marangoni Citation2011).
Addition of fat for structural modification
For the creation of higher melting fats, the incorporation of fats with a higher melting point in the regular fat mixture is another strategic approach. Traditionally, as explained above, CB equivalents (CBE), CB replacers (CBR) and CB substitutes (CBS) are added as CB alternatives (CBA) to improve melting characteristics and ease of chocolate formation. The melting behavior of fat is critical to its behavior in chocolate formulations. This is best determined by the solid fat content at a particular temperature. Eutectic compositions are formed when multiple fats are constituted in the same product forming a relationship between them. This is also used to determine the compatibility between various fats to check their overall meltability and compatibility to be used with CB in chocolate compositions. Blending of symmetrical monounsaturated TAG rich fats partially with other vegetable fats in cocoa butter or chocolate products increases their desirable physiochemical and thermal properties (Aydemir Citation2019; Bootello et al. Citation2012, Maheshwari and Reddy Citation2005; Reddy and Prabhakar Citation1994; Norazlina, Jahurul, Hasmadi, Sharifudin, Patricia, Mansoor, et al. Citation2020). By adding high melting SOS-rich fats into CB- based fat, the melting profiles can be increased several degrees higher than that of natural CB without leaving waxiness (Beckett Citation2000; Salas et al. Citation2011).
Till 2011, the incorporation of higher melting (natural) fats included the combination of mahua and kokum fat, the use of only kokum fat are given in detail by Stortz and Marangoni (Citation2011). The further studies are summarized in and their scientific principles are given below:
Table 2. Development in “Fat structure modification” method to produce HRC after 2011.
Kernel fat addition: The use of plant (kernel) fats to act as Cocoa Butter Extenders (CBEs) was further done using mango kernel, palm fractions, shea butter and Bambangan fats. The mango almond fat solid content profile is very similar to that of CB (with its fractions having melting thermogram ranging from 38 °C to 42.2 °C (Solís-Fuentes and Durán-de-Bazúa Citation2004; Sonwai et al. Citation2014; Jin, Mu, et al. Citation2017). Also, initial studies showed that the blends of mango seed fat and palm oil mid fractions in different ratios have similar fatty acid profile as CB (Sonwai, Kaphueakngam, and Flood Citation2014; Jin et al. Citation2016) and some blends (with palm stearin, SMP: 51.2 °C) had a slip melting point as high as 43.7 °C that can be used in chocolate formulations (Jahurul, Zaidul, Nik Norulaini, Sahena, Kamaruzzaman, et al. Citation2014). It was seen that high StOSt and POSt content in mango kernel fats and its fractions created a highly packed matrix entrapping liquid fat (Dewettinck et al. 2015; Jin et al. Citation2016). With palm olein fraction present, transformation from stable β2 form to more stable β1 form in the presence of high StOSt levels was responsible for better thermal properties (Beckett Citation2000; Toro-Vazquez et al. Citation2004). Heat resistant compound chocolate melting up-to 40 °C was made with fully hydrogenated palm olein and crystal modifiers strengthening the chocolate network (Rosales, Klinkesorn, and Suwonsichon Citation2017a, 2017b). But the exothermic peaks of palm mid fractions (PMF) were quite different from CB, indicating that too high percentages of PMF in HRC can have undesirable effects on the thermal properties (Sonwai et al. Citation2014; Jin, Akoh, et al. Citation2018). From the nutritional point of view, used of mango fat is beneficial due to high un-saponifiable matter present, often associated with several health benefits.
Another kernel fat that has a potential to be used as CBE for increasing the heat resistance of chocolate is Bambangan (Mangifera pajang) kernel fat (BKF), a tropical fruit found in parts of Indonesia, Malaysia and Brunei. BKF is composed of 11.6% POS, 28.7% SOS and 11.2% SOO triacylglycerides and shows polymorphism like CB, with the β form having the highest melting point and stability relative to other forms with closest molecule arrangement (Solís-Fuentes et al. Citation2005; Norazlina, Jahurul, Hasmadi, Sharifudin, Patricia, Mansoor, et al. Citation2020). This high similarity in TAGs as well as fatty acids made BKF very suitable for manufacturing of confectionary products, especially chocolate products (Hassim and Dian Citation2017; Jahurul et al. Citation2019; Jahurul et al. Citation2018, Jin, Akoh, et al. Citation2018; Jin, Mu, et al. Citation2017; Reddy and Jeyarani Citation2001; Norazlina, Jahurul, Hasmadi, Sharifudin, Patricia, Mansoor, et al. Citation2020). It was found that the stearin fraction of BKF has a slip melting point between 34.3 and 36.3 °C with higher solid fat content (66.06–76.31%) and better crystallinity than olein fraction and CB (Jahurul et al. Citation2018). Further, in two stage acetone fractionation, the stearin fractions obtained showed higher peaks for DSC melting thermograms (up-to 42.45 °C) with higher StOSt content (as high as 64.70%) indicating the presence of high-melting symmetrical TAGs that increase the hardness of fat and can lead to desirable thermal properties if further studied and used in chocolate (HRC) formulation.
Crystalline seed addition: This is another method to create a stable fat crystal and further control the crystal growth mechanics of the overall fat composition. A crystalline seed is a seed comprising of crystals capable of seeding chocolate in predominantly form V. The stable nuclei in the seeding agent serves as crystal nuclei by controlling the chocolate mix to a temperature lower than the melting point of the fat in the chocolate mix, thus accelerating formation of stable crystal and crystal growth in the chocolate mix (Oonishi and Hachiya Citation2016). The seeding material is either prepared directly from the XOX containing fats such as shea fat, sal fat, mango kernel oil etc. or by mixing an XOX containing fat with other oils/fats (Oonishi and Hachiya Citation2016).
Chire-Fajardo, Ureña-Peralta, and Hartel (Citation2020) investigated the improvement in heat resistance of chocolate using Peruvian cocoa bean varieties CCN 51 (Colección Castro Naranjal) and ICS 6 (Imperial College Selection, District of Uchiza, Peru). Earlier studies had shown that the fatty acid profiles of cocoa beans vary with the geographical conditions (Torres-Moreno et al. Citation2015). 60%, 70% and 80% CB along with the combination of CCN 51 and ICS 6 (in ratios of 1:9, 5:5, 9:1) was used to formulate chocolate samples. It was seen that the resultant blend had heat resistance between 25 and 35 °C and became waxier at 35–40 °C (due to high solid fat content). This butter had improved nutritional profile in terms of PUFA content (about 4.36%). Thus, it was suggested that this modified blended cocoa butter could be used in formulation of chocolate in tropical countries.
Organogelation/oleogelation
Organogel (or oleogel) is a novel technological approach for structuring of edible oils in the field of fat modification and has potential application for creation of HRC. An organogel can be defined as an organic liquid (edible oil) entrapped within a thermo-reversible, anhydrous and structured visco-elastic material by a three-dimensional gel network. This simply means the transformation of liquid oil into a 'gel-like' structure with visco-elastic properties. This gel network is formed by the self-assembly of a relatively low concentration of low molecular weight compounds (organogelator molecules), which are capable of gelling organic solvents (Marangoni and Garti Citation2011). It can also be formulated in a way to improve the overall nutritional profile of the food product.
The majority of fat products available in the market are structured by a colloidal network of crystalline triacylglyceride (TAG) particles. It is considered that saturated fatty acids become dissolved in the unsaturated TAG phase and this leads to the formation of solid nuclei of TAG particles which further grow into crystalline nano-particles or spherulitic particles. Organization of these spherulitic particles into arbitrary flocs establishes non-covalent interactions between fat crystal networks, which provide physical functionality to the fat material (Kaushik et al. Citation2017). A novel concept for oil structuring using a lesser amount of trans fat or saturated fats is based on the use of structurants which have the potential to replace the natural network of TAG structure in native fat being efficient as a lipid and matching physical properties to be used in various food applications. Through the molecular self-assembly of particle or fibril crystallization, they can lead to the formation of a 3-D gel network that mimics this TAG structure. Generally, low-molecular-weight organogelators are used in the food industry which can be categorized as lyotropic phases and crystalline dispersions. Examples of low molecular weight organogelators are triacylglyceride (TAG), diacylglyceride (DAG), monoacylglyceride (MAG), fatty acids, fatty alcohols, waxes, wax esters, and sorbitan monostearate (SMS). Another category, polymeric organogelators e.g. ethylcellulose, shows promising ability in the food sector being inexpensive and food-grade compared with the former options (Kaushik et al. Citation2017). Structuring edible oils with an organogel network may lower some of the risk factors for cardio vascular diseases (Hughes et al. Citation2009). Edible oleogels have been used to formulate baked goods, heat resistant chocolate, dairy and meat products (Patel, Nicholson, and Marangoni Citation2020). It has been demonstrated that oleogels provide higher melting points to chocolate or chocolate derived products (Blake, Co, and Marangoni Citation2014) as well as are effective in overcoming the fat bloom problems in chocolate and filled chocolate products (Demirkesen and Mert Citation2020). Recent studies so far conducted with oleogel in chocolate are summarized in the given below. Earlier studies showed that ethyl cellulose (EC) forms anhydrous polymer organogels of edible oils at a concentration greater than 3%. The melting point of EC based oleogel is greater than 100 °C (Davidovich-Pinhas, Barbut and Marangoni Citation2015). This oleogel formation was believed to hold on the liquid fat present in the chocolate at higher temperatures (Pieve et al. Citation2010; Davidovich-Pinhas, Barbut and Marangoni Citation2015). When combined with lecithin and ethylcellulose, the sucrose suspension in chocolate showed overwhelming effect on the fluidization and dispersibility of particles, improving the preparation of chocolate with reduced-fat content (Do et al. Citation2010).
Table 3. Development of organogelation technique for HRC.
Fat substitution method
When EC is used for organogelation, it gels the oil by itself and produces a stable, translucent and stiff gel. A surfactant is generally added to the oleogel to reduce its brittle and grainy texture as well as decreasing high gelation temperature. EC plasticizes the gel to a lower gelation temperature of the formed gel (between 60 and 80 °C). The mixture of EC in oil remains readily miscible with other ingredients of the chocolate composition during mixing. The results suggested that the effect of EC on the heat resistance of chocolate may be due to a complex interaction between EC, the fat phase and one or more solid phases in chocolate but no scientific evidence was provided (Pieve et al. Citation2010).
Solvent substitution method
Stortz and Marangoni (Citation2011) hypothesized that by adding ethyl cellulose (EC) solubilized in ethanol (EtOH) to chocolate and evaporating the EtOH, an organogel could be formed in situ with the fat phase of the chocolate. The results of this method suggested that heat resistance was caused by small amounts of water added to chocolate via absorption to the EtOH (Stortz and Marangoni Citation2013). The water caused the surface of the sugar particles in the chocolate to dissolve and then stick to one another creating a sugar network that resisted deformation and trapped liquid fat at elevated temperatures (Stortz and Marangoni Citation2011). This was supported by the observation that dark chocolate had much lower heat resistance than white and milk chocolate due to the difference in sugar content. It was also advocated that EtOH itself may play a role in the improvement of heat resistance since some of the phospholipids in soy lecithin are soluble in EtOH (Teberikler, Koseoglu, and Akgerman Citation2001). It was also believed that some of the minor polar components which may be present in the palm kernel oil such as sterols or triterpene alcohols (Goh, Choo, and Ong Citation1985; Itoh, Tamura, and Matsumoto 1973) may have been displaced from the center of the chocolate upon evaporation of the EtOH due to their solubility in this solvent (Liu Citation2011); with their removal affecting the crystallization of the fat leading to a higher melting point. When efficacy of other solvents for HRC manufacture was checked, it was observed that the samples made with EC in ethyl acetate had significantly reduced heat resistance at 40 °C compared to those made with EtOH. This indicated that EtOH was responsible for greater heat resistance maybe because of the lecithin phospholipids which are soluble in EtOH but are not soluble in ethyl acetate (Janak Citation1987).
Stortz and Marangoni (Citation2011) suggested a promising approach to entrap the molten fat with a secondary crystalline network of high melting point (50–90 °C) emulsifier. Sorbitan monostearatae (SMS) was capable of gelling vegetable oils and fatty acid esters at a concentration as low as 1% (w/w) (Murdan, Gregoriadis, and Florence Citation1999). In the studies conducted by Peyronel and Marangoni (Citation2014), X-Ray diffraction (XRD) results showed two kinds of crystals morphologies in the formulated chocolate: the sub-cell packing of the fatty acid chains in SMS was similar to the ‘α’ phase in crystalline TAG but the fat blend also showed polymorphic stability in the β' form after prolonged storage (77 days; 22 °C). It was suggested that these two kinds of crystals were held together by strong forces forming some solid solution which imparted better structural integrity at higher temperatures. SMS took less time to nucleate and crystallizing before the fat blend, showing that growth mechanism was affected by SMS addition. But there was still an uncertainty of the fat blend and SMS crystal growth relationship. Also, the minimal amount of SMS used (<40%) for imparting heat resistance exceeds the limit under standards of food applications.
When Patel et al. (Citation2014) studied the oil binding characteristics of shellac oleogels, they indicated that due to this extensive network of shellac crystallites in liquid chocolate the oleogels served as a continuous phase to create emulsifier-free, structured water in oil emulsion. The formation of these emulsions in the absence of an emulsifier was attributed to the high level (30% w/w) of surface-active wax alcohols that contribute to the surface activity of shellac. This study needs to be further researched for its use in the formation of HRC.
Fayaz, Goli, and Kadivar (Citation2017) used beeswax and propolis wax as structuring agents because they contain long-chain fatty acid esters that can crystallize forming a three-dimensional network entrapping liquid oil (Doan et al. Citation2015; Fayaz, Goli, and Kadivar Citation2017); and monoglycerides can self-assemble into inverse bi-layer nanostructures organized at micro-level into lamellar platelets that interact (hydrogen bonding) immobilizing liquid oil (Pieve et al. Citation2010). Crystallization of beeswax and propolis wax in liquid oils resulted in particulate gels where the network based on van-der Waals interactions of crystals and crystalline aggregates immobilize the liquid oil into a three-dimensional structure (Doan et al. Citation2015). The better results obtained for monoglycerides indicated that in monoglyceride containing samples, strong reciprocal interactions were formed between monoglyceride and palm oil crystals, leading to a close 3-D network with strong gel properties. The presence of sugar further increased the number of solids in the system and interactions between the OH groups of monoglyceride crystals and free OH groups of sugar crystals led to a stronger network in monoglyceride spread (Doan et al. Citation2016). Since there is a low level of chemical compatibility between wax and palm oil, the structural framework could not be as strong (Hwang et al. Citation2012). Also, the reason for propolis wax forming a weaker gel in comparison to beeswax was attributed to the presence of different phenolic compounds in it disturbing the formation of the crystalline network (Fayaz, Goli, and Kadivar Citation2017). Prolonged storage showed some increase in the hardness of the wax samples attributed to the reorganization of wax crystals in the post-production isothermal crystallization. The decrease of firmness in monoglyceride samples over time was attributed to the changes in the monoglyceride structure during storage proved by XRD analysis showing the phenomenon of polymorphic transformation from α-form to β polymorph (Lopez-Martínez et al. Citation2015; Sintang et al. Citation2017). Therefore phase separation between solid crystals and liquid oil took place which resulted in the collapse of the gel-network during aging, decreasing the hardness (Chen and Terentjev Citation2009). The major concern in studies conducted by Francis and Chidambaram (Citation2019) was that due to increased water content in the hydrogel formulation, the stability of the polymorphic forms during storage were not studied.
The formation of oleogels by solvent addition or resin/gels used from different sources has significantly proved to show the gel formation characteristics entrapping the liquid oil in a solid gel matrix. Some of these studies just indicated the ways in which oil migration can be retarded in chocolate and needs to be further researched extensively for HRC formulation. Also, in all these studies, it has not been shown how the sensory attributes of chocolate mouthfeel and taste are affected since bounded oil, even if liquid, cannot give the 'melt in the mouth' texture of the chocolate. Gel can only prevent it from oozing out from the matrix at higher temperatures. Further, the legal limits on the use of resins and solvents can prevent their use considering the limits and the actual definition of chocolate in many countries. But this approach of research is still in nascent stage and requires further studies before coming to any further conclusions.
Sugar microstructure modification
Sugar microstructure modification involves the development of a secondary sugar network by the addition of water either via direct or indirect incorporation methods or by the use of sugar hydrate additive or via addition of ethyl cellulose. The development of HRC by water incorporation is described in detail by Stortz and Marangoni (Citation2011). The addition of sugar hydrate additive used dextrose monohydrate to bind water at lower temperatures in the chocolate to create a heat resistant structure between sugar and water. The addition of ethyl cellulose was done by the solvent substitution method and its potential advantages in improving the heat resistance of chocolate were extensively researched and studied.
An interesting study, not directly relating to the formation of HRC but just to determine the role of fat, water and sugar in its formation was performed by Rousseau et al. (Citation2015) when they tried to find an effective extraction method to remove cocoa butter fat phase from chocolate while leaving the dispersed particulate network intact. They were able to remove about 88% of CB after 120 h. It was observed that absence of water resulted in dispersed particulates to fell apart; therefore addition of water (as emulsifier stabilized water-in-oil emulsion) was necessary. Increasing the moisture content consolidated loose agglomerates through adhesive forces (surface tension) associated with interstitial liquid bridges between the grains which are responsible for capillary bonding between grains (Stortz and Marangoni Citation2011; Hoffmann et al. Citation2014). It was also attributed to the dissolution and recrystallization of sugar crystals into a solid network (bridges) by water addition. Also, emulsifier polyglycerol polyricinoleate (PGPR) was necessary for defatted network stabilization. Characterization with scanning electron microscopy and confocal microscopy demonstrated that the backbone structure consisting of dispersed particulates could be made into a self-supporting network. This study can further open up newer ways to study the role of dispersed particulates on the rheological and textural properties of heat resistant chocolate.
Direct water incorporation
The updated summary of this approach for creating microstructure suitable for heat resistance chocolate is presented in the given below.
Table 4. Development of direct water incorporation technique in HRC.
Indirect water incorporation
The indirect incorporation of water is done by two ways: addition of a humectant and indirect incorporation of water in encapsulated form. Incorporating a humectant creates a sugar or sugar-water matrix, which can entrap the liquid fat under higher melting temperatures. This approach is decribed in detail in the paper by Stortz and Marangoni (Citation2011). The further development in this framework is given in detail below.
Killian and Coupland (Citation2012) used a cross flow membrane emulsification system to make 30% water-in-soybean oil emulsions. These emulsions were incorporated into chocolate made from dispersions of sugar crystals in oil. Liquid bridging (via capillary forces) occurred between sugar molecules, creating a heat resistant structure. The degree of sample spread at 50 °C was less for samples containing water and was affected by the mode of water addition.
To produce an emulsion Lillah et al. (Citation2017) used gum arabic and golden sirup along with water and polyglycerol polyricinoleate (PGPR) in the chocolate blend. The principle behind the study was to improve the heat stability of chocolate by strengthening the sugar microstructure with water and delaying the factors responsible for its crystallization. The addition of PGPR increased the continuous phase portion and linked leftover water present in chocolate leading to make it unavailable for hydration and swelling of solid units (Rector Citation2000). Gum arabic was used to inhibit sugar crystallization and to emulsify fat to keep it uniformly dispersed all through the product (Kenyon Citation1995). The resultant bars (80 ml/l emulsion added) became soft in the temperature range of 36–40 °C, but still maintained their shapes. Although heat stability, firmness and brittleness increased with the additional amount of emulsion in the samples, there was no proper quantification of ingredients to be added for the formulation of HRC using this technique. Also, the addition of gum arabic is not permitted as an ingredient for the preparation of chocolate in most of the countries and thus cannot be used as such hereof. In a similar approach when Aliakbari, Asefi, and Yeganezad (Citation2018) used 10% gelatin and corn starch to produce milk chocolate, the highest melting temperature attained was 38.5 °C. However, there was no significant difference observed in the organoleptic properties of the formulated samples.
Kriel et al. (Citation2017) used EC along with a sweetener (particle size: 4–20 μm) or non-aqueous solvent with less than 4% water (w/w), for indirect water incorporation in the chocolate composition. The hardness of the product improved by keeping it incubated for 5–20 days at 25–40 °C suggesting that reducing the water content of non-aqueous solvent is beneficial for creating HRC.
Stortz and Marangoni (Citation2011) provided an extensive review on the incorporation of polyol as humectants as well as incorporation of encapsulated water. The addition of polyol is reviewed here in detail & its development is being tabulated in the table given below (). Scientific principles behind the incorporation of polyol to improve the heat resistant properties in chocolate were based on different approaches in the earlier studies. It was believed that when liquid polyols were added to chocolate, they chemically interact with the fat in the chocolate, leading to an increase in the viscosity of the mix ascending with an increase in time and/or temperature as well as increased amount of polyol added. It was also suggested that since polyols were required to be incorporated in the liquid state for all the above studies, water may have been incorporated (due to polyols absorbing water during storage), which may be responsible for the heat resistant properties. Further, it was suggested that the sugar network could be formed in chocolate via hygroscopic action of polyols during the heat resistance development period. In HRC produced by Kincs (Citation1992), the chocolate formula also had milk solids suggesting that perhaps hydration of proteins played a role in the development of heat resistance in chocolate (Kempf Citation1958). Also, the presence of lactose sugar in milk solids can transit from its glass state, becoming sticky enough to glue the molecules together forming an inter-particle bridging, crystallizing into a welded mass (Laughter Citation2013). It was also addressed that the molecule of water present in dextrose monohydrate might interact with bulk sweeteners in combination with glycerol to form/enhance the heat resistant structure of the confection (Glazier et al. Citation2014). It was believed that glycerol may interact or assist with the dispersion of fat in the confection (Potter Citation2014).
Table 5. Development of Polyol Addition Technique in heat resistant chocolate (HRC).
Use of sugar hydrate additive
Wang et al. (Citation2016) devised a method to create HRC by carefully controlling the water releasing step using pre-sized sugar hydrate (dextrose monohydrate, <60 microns). The sugar hydrate was pre-sized to enhance its ability to form heat resistance by lowering the temperature at which dehydration occurs. It was believed that smaller particles have loose bonds between sugar and water molecules which made the latter readily available to develop heat stability. Thus water was bound at lower temperature, imparting the same creamy texture as regular chocolate. But when it was released at high temperatures, it formed a stable sugar-water matrix and thus heat resistant properties. When the resultant chocolate was stored at 18.3 °C and 23.4 °C for four weeks, the product developed heat stability as well as firmer texture which further increased with increasing the amount of sugar hydrate added.
Addition of ethyl cellulose (EC)
Development in the field of application of EC in the creation of HRC (post 2011) both in the categories of fat and sugar modification as well as organogelation has been slow. There are different hypothesis to determine the possible scientific reason for the development of HRC by the addition of EC so the further studies were addressed to study various interactions in the sugar network formation due to addition of EC. However, in EC contained chocolate coating, the heat resistance of products increased while the sensorial quality diminished (Gravelle et al. Citation2012).
Ibrahim et al. (Citation1988) showed that when EC dissolved in EtOH was used to coat sucrose, dissolution occurred only at holes in the coating where aqueous phase was able to penetrate. Also, interactions were observed between EC and dialkyl phthalates, propylene glycol dicaprylate and nifedipine which had structural similarity to phosphatidylcholine (PC), a lecithin phospholipid, indicating that EC contains slightly polarized hydroxyl groups that can form hydrogen bonds (H-bonds) with similar groups at ester linkages of PC (Stortz et al. Citation2014).
When Stortz et al. (Citation2014, Citation2015) studied various interactions occurring between EC and sucrose with lecithin, H-bonds were observed between ring oxygen of EC and hydroxyl hydrogen of uncoated sucrose. Formation of H-bonds between hydroxylated R-groups of EC and ether oxygen of sn1 and sn2 tails of PC (esp. 1-palmitoyl-2-oleoyl-sn-glycerol-3-phosphocholine (POPC)) were also observed. Further, H-bond formation between sucrose hydroxyl groups and POPC ether groups was also confirmed. It was suggested that EC acted as glue between sucrose particles enhancing interactions and improving heat stability and mechanical strength of the jammed network. The resultant chocolate samples, prepared via solvent substitution, were about 3 times harder than the samples prepared via heat method. This was attributed to a combination of better dissolution of EC in EtOH in the oil phase and slight solubility of sucrose in EtOH. Hardness of samples was further increased by addition of surfactants (glycerol monostearate). It was suggested that the surfactant could plasticize EC allowing the polymer to be more extended in oil causing better interaction with surface of sucrose. It was also attributed to the formation of secondary network within the sample which resisted deformation up-to 40 °C as confirmed by SEM. Thus, the idea that organogelation was responsible for heat resistance by EC addition was rejected due to very little mechanical strength shown by samples with no sucrose. The results of this research (Stortz et al. (Citation2014)) provided significant scientific reasons behind the attainment of heat resistant property in chocolate by addition of EC.
Stortz et al. (Citation2014, 15) further studied the role of lecithin and solvent addition in EC- stabilized HRC. It was seen that the presence of lecithin at the surface of sucrose reduced heat resistance by impeding interactions between EC and sucrose. Further, EtOH was able to remove the EtOH soluble lecithin phospholipids from the surface of sucrose leading to harder samples. This, along with slight solubility of sucrose in EtOH improved the heat resistance of chocolate. It was also determined that EC is likely to interact with lactose in skimmed milk powder and with large amount of naturally present starch in cocoa powder (Sivasankar Citation2002) leading to greater heat resistance. Also, as the concentration of EtOH increased, the samples became stronger, less fragile, and easier to handle due to greater proportion of sucrose being dissolved in EtOH as its concentration increased.
Modification of processing techniques
Heat resistant properties of the chocolate improved as the structure was modified via processing techniques applied for chocolate production. Cost effectiveness is an added advantage because generally no additional ingredients are required for the production of HRC. The methods mainly address to improve the micro-crystal network of chocolate to improve its heat resistance. Two processing methods are studied so far in developing HRC.
Application of pressure
Application of pressure was found to significantly increase density and reduce diffusion of TAG molecules, suggesting that pressure play an important role in processing and crystallization of TAGs (Ferstl et al., Citation2011). The application of pressure to induce heat resistance in chocolate was first studied by Fountain and Penner (Citation2015) who proposed that the compression of the powdered composition of chocolate at high pressure fuses the particles to form a matrix providing heat resistant to the product with similar taste profile. Powdered chocolate composition (particle size: 10-30 µm) with no emulsifier was compressed at a pressure between 7000 kPa and 130,000 kPa to obtain the chocolate tablet. When the pressure of about 40,000–70,000 kPa was applied, the resulting tablet was structurally strong but still soft enough for a consumer to bite into. It was suggested that the protein-fat interaction between milk solids and CB prevented the fat from melting within the protein matrix. Samples kept in the ovens at 40 °C were still found stable even after 6 months of storage maintaining the usual snap.
Nielsen et al. (Citation2016) also used compression for constituent fat modification as a step to develop HRC. Hydrocolloids such as functional proteins, gums, gels and other macromolecular entities such as glucans, starches, clays, etc. were also used in some compositions (<12% w/w). The resultant chocolate samples maintained their shape, snap and form after being kept at 50 °C for 4 weeks. It was suggested that the heat resistant property of this chocolate was induced due to the fat in the composition being dispersed in the continuous powder, and thus the structure of chocolate composition may be less vulnerable to fat phase changes.
Changing the particle size of the composition
It was suggested that by utilizing certain ingredients in un-milled or enhanced size, the moisture inherently present in such components remain available to the confection for the formation of heat resistant structure therein. When nano-sized sucrose (particle size: 50–1000 nm; >1% w/w) was used in making chocolate, it resisted deformation up-to 35 °C. The samples with 9% (w/w) nano-sized sucrose particles showed almost negligible change in structure above the temperature of 35 °C (Hess et al. Citation2010).
Wang et al. (Citation2014) took similar approach where they milled a portion of dry ingredients (in particular the sweetener) to a particle size in the range of 5–50 microns (preferably between 20 and 30 microns) and included it later to the composition (5–72% w/w). The reduction of particle size increased the surface area of the non- fat ingredients so that more free fats are trapped to the newly formed surface. The product maintained its shape above the melting point of the fat present in the chocolate and had a shelf life of at least 6 months at 32.2 °C or above. It also exhibited smooth, non- grainy texture, with a mouthfeel and taste compatible with regular chocolate.
Wentzel et al. (Citation2015) produced a heat resistant confection wherein at least a portion of ingredients (sweetener or cocoa or milk solids) had an average particle size greater than 50 microns (50–2000 microns) along with a polyol. Milk powders with higher vacuole volumes occupy larger spaces of continuous fat phase, increasing the viscosity and yield value of chocolate (Keogh, Murray, and O’Kennedy Citation2003). This higher viscosity can lead to more temperature robust chocolate by entrapping cocoa butter (Laughter Citation2013). Chocolates made with desired premix and aged (3–20 days), showed heat resistance at 38 °C or more. A combination of glycerin and dextrose monohydrate was considered as ideal for producing HRC. Though not bound by a scientific theory, it was believed that the polyol may interact with an un-milled granular component and/or the un-milled granular component may assist with the dispersion of polyol, within the fat-based confection. But the product had un-milled dextrose which was detected in the mouth feel imparting a gritty texture to the product.
Conclusion
There are different scientific approaches are being used for the development of heat resistant chocolates. The researchers have been able to establish different rational scientific principles that can be used in creating heat resistant properties in chocolate. It can be seen that this field of research is mainly industry driven and there is need of academic collaboration for understanding fundamental principles to develop HRC in a creative way for the manufacture of simple, inexpensive and palatable chocolate at an industrial scale. It is of utmost importance to understand the science behind these principles in defining the quality characteristics, such as melting and sensory properties, in both chocolates and compound chocolates. There has been little progress in the field of incorporation of ingredients that are least altered from conventional 'chocolate'. Most of the vegetable fats that are used in the studies have not found their direct application to the chocolate industry and will not be able to come in the compliance of ‘chocolate’ in many countries. Research done in the field of addition of water (directly or indirectly) and improving the heat resistance via processing techniques is old and it further needs to be looked at or addressed in a better way now that we know the scientific principles that act behind the addition of some ingredients. There has been limited sensory mapping in terms of chocolate product quality as well as imparting heat resistance characteristics. There is a need to identify and find the right ingredients for the production of HRC that are in accordance with the regulatory compliance and principles of ‘chocolate’ description in various countries. Oleogelation, is a promising field that combines the use of various scientific principles backed up by proper scientific evidence based knowledge. This field of research can thus impart some answers to the questions that we are looking forward to address when the production has to be done on an industrial scale but needs to be studied further. There is need of understanding the chemistry and physics of polymorphic cocoa butter forms and how the right chcolate microstructure creation can be done by using generally regarded as safe (GRAS) ingredients and innovative approaches to have desired macroscale attributes like textural and thermal characteristics.
Disclosure statement
No potential conflict of interest was reported by the authors.
Correction statement
This article was originally published with errors, which have now been corrected in the online version. Please see Correction (http://dx.doi.org/10.1080/10408398.2021.1949103)
References
- ADM (Archer Daniels Midland) Cocoa. 2009. De Zaan cocoa manual. Switzerland: ADM Cocoa International. Accessed January 10, 2021. https://www.yumpu.com/document/view/44355148/dezaan-cocoa-manual-adm.
- Afoakwa, E. O. 2010. Chocolate science and technology. Oxford, UK: Wiley-Blackwell.
- Afoakwa, E. O., A. Paterson, and M. Fowler. 2008. Effects of particle size distribution and composition on rheological properties of dark chocolate. European Food Research and Technology 226 (6):1259–68. doi: 10.1007/s00217-007-0652-6.
- Afoakwa, E. O., A. Paterson, M. Fowler, and J. Vieira. 2008. Effects of tempering and fat crystallization behaviour on microstructure, mechanical properties and appearance in dark chocolate systems. Journal of Food Engineering 89 (2):128–36. doi: 10.1016/j.jfoodeng.2008.04.021.
- Aini, I. N., and M. S. Miskandar. 2007. Utilization of palm oil and palm products in shortenings and margarines. European Journal of Lipid Science and Technology 109 (4):422–32. doi: https://doi.org/10.1002/ejlt.2006.00232. doi: 10.1002/ejlt.200600232.
- Aliakbari, F., N. Asefi, and S. Yeganezad. 2018. Effect of gelatin and cornstarch on Hheat resistance and sensory properties of milk chocolate. Iranian Journal of Nutrition Sciences and Food Technology 13 (2):99–106.
- Aydemir, O. 2019. Utilization of different oils and fats in cocoa hazelnut cream production. Journal of Food Processing and Preservation 43 (5):e13930. doi: 10.1111/jfpp.13930.
- Babin, H. 2005. Colloidal properties of sugar particle dispersions in food oils with relevance to chocolate processing. PhD Thesis., University of Leeds, Woodhouse, UK.
- Bahari, A., and C. Akoh. 2018. Synthesis of cocoa butter equivalent by enzymatic interesterification of illipe butter and palm mid-fraction. Journal of the American Oil Chemists' Society 95 (5):547–55. doi: 10.1002/aocs.12083.
- Bahari, A., and C. C. Akoh. 2018. Texture, rheology and fat bloom study of chocolates made from cocoa butter equivalent synthesized from illipe butter and palm mid-fraction. Lwt 97:349–54. doi: 10.1016/j.lwt.2018.07.013.
- Beckett, S. T. 2000. The science of chocolate. London, England: Royal Society of Chemistry.
- Beckett, S. T. 2008. The science of chocolate. London, England: Royal Society of Chemistry.
- French Development Agency & Barry Callebaut Study: Forever Chocolate Progress Report. 2017-2018. Accessed January 10, 2021. www.barry-callebaut.com/sites/default/files/2019-01/barry-callebaut-forever-chocolate-progress-report-2017-18.pdf
- Best, E. T., D. Oakenfull, M. Maladen-Percy, R. T. Boehm, and L. A. Kibler. 2005. Torpicalizing agent, and methods for making and using the same. Google Patents.
- Biswas, N., Y. L. Cheow, C. P. Tan, S. Kanagaratnam, and L. F. Siow. 2017. Cocoa butter substitute (CBS) produced from palm mid-fraction/palm kernel oil/palm stearin for confectionary fillings. Journal of the American Oil Chemists’ Society 94:234–45. doi: 10.1007/s11746-016-2940-4.
- Blake, A. I., E. D. Co, and A. G. Marangoni. 2014. Structure and physical properties of plant wax crystal networks and their relationship to oil binding capacity. Journal of the American Oil Chemists' Society 91 (6):885–903. doi: 10.1007/s11746-014-2435-0.
- Bootello, M. A., R. W. Hartel, R. Garcés, E. Martinez-Force, and J. J. Salas. 2012. Evaluation of high oleic-high stearic sunflower hard stearins for cocoa butter equivalent formulation. Food Chemistry 134 (3):1409–17. doi: 10.1016/j.foodchem.2012.03.040.
- Bruse, F., J. R. P. Wallecan, and C. S. Arruda. 2008. Chocolate composition. Google Patents.
- Carlin, J. T., K. N. Lee, O. A.-L. Hsieh, L. S. Hwang, C. T. Ho, and S. S. Chang. 1986. Comparison of acidic and basic volatile compounds of cocoa butters from roasted and unroasted cocoa beans. Journal of the American Oil Chemists' Society 63 (8):1031–6. doi: 10.1007/BF02673793.
- Chen, C. H., and E. M. Terentjev. 2009. Aging and metastability of monoglycerides in hydrophobic solutions. Langmuir: The ACS Journal of Surfaces and Colloids 25 (12):6717–24. doi: 10.1021/la9002065.
- Chire-Fajardo, G. C., M. O. Ureña-Peralta, and R. W. Hartel. 2020. Fatty acid profile and solid fat content of Peruvian cocoa for optimal production of trade chocolate. Revista chilena de nutrición 47 (1):50–6. doi: 10.4067/S0717-75182020000100050.
- Climate Change. 2020. Food and Agriculture Organization (FAO) of the United Nations. Accessed January 10, 2021. https://fao.org/climate-change/news/detail/en/c/1314699/.
- The Cocoa and Chocolate Products (England) Regulations. 2003. Statutory Instrument 2003 No. 1659. Regulations for Cocoa and Chocolate Products. Accessed January 10, 2021. www.extwprlegs1.fao.org/docs/html/uk39181.htm.
- Cocoa Producing Countries. 2020. World Population Review. Accessed January 10, 2021. https://www.worldpopulationreview.com/country-rankings/cocoa-producing-countries.
- Counet, C.,. D. Callemien, C. Ouwerx, and S. Collin. 2002. Use of gas chromatography-olfactometry to identify key odorant compounds in dark chocolate. Comparison of samples before and after conching. Journal of Agricultural and Food Chemistry 50 (8):2385–91. doi: 10.1021/jf0114177.
- Davidovich-Pinhas, M., S. Barbut, and A. Marangoni. 2015. The gelation of oil using ethyl cellulose. Carbohydrate Polymers 117 (6):869–78. doi: https://doi.org/10.1016/j.carbpol.2014.10.035. doi: 10.1016/j.carbpol.2014.10.035.
- DeMan, J. M. 1999. Principles of food chemistry. Gaithersburg, Maryland, USA: Aspen Publishers Inc.
- Demarest, A. A. 2019. 8 unbelievable facts about the $103 billion chocolate industry. Insider. www.insider.com/chocolate-industry-facts-statistics-consumption-2019-11.
- Demirkesen, I., and B. Mert. 2020. Recent developments of oleogel utilizations in bakery products. Critical Reviews in Food Science and Nutrition 60 (14):2460–79. doi: 10.1080/10408398.2019.1649243.
- Depoortere, L. 2011. The use and applicability of cocoa butter equivalents (CBEs) in chocolate products. Faculteit Bio-ingenieurswetenschappen, Universiteit Gent.
- Dimick, P. S., and J. C. Hoskin. 1994. Chemistry of flavour development in chocolate. In Industrial chocolate manufacture and use, 137–52. Boston, Massachusetts, USA: Springer. doi: 10.1007/978-1-4615-2111-2_8.
- Do, T. A. L., J. R. Mitchell, B. Wolf, and J. Vieira. 2010. Use of ethylcellulose polymers as stabilizer in fat-based food suspensions examined on the example of model reduced-fat chocolate. Reactive and Functional Polymers 70 (10):856–62. doi: 10.1016/j.reactfunctpolym.2010.07.012.
- Doan, C. D., D. V. de Walle, K. Dewettinck, and A. R. Patel. 2015. Evaluating the oil‑gelling properties of natural waxes in rice bran oil: Rheological, thermal, and microstructural study. Journal of the American Oil Chemists' Society 92 (6):801–11. doi: 10.1007/s11746-015-2645-0.
- Doan, C. D., A. R. Patel, I. Tavernier, N. D. Clercq, K. V. Raemdonck, D. V. de Walle, C. Delbaere, and K. Dewettinck. 2016. The feasibility of wax-based oleogel as a potential co-structurant with palm oil in low-saturated fat confectionery fillings. European Journal of Lipid Science and Technology 118 (12):1903–14. doi: 10.1002/ejlt.201500172.
- Fayaz, G., S. A. H. Goli, and M. Kadivar. 2017. A novel propolis wax‑based organogel: Effect of oil type on its formation, crystal structure and thermal properties. Journal of the American Oil Chemists' Society 94 (1):47–55. doi: 10.1007/s11746-016-2915-5.
- Fayaz, G., S. A. H. Goli, M. Kadivar, F. Valoppi, L. Barba, C. Balducci, L. Conte, S. Calligaris, and M. C. Nicoli. 2017. Pomegranate seed oil organogels structured by propolis wax, beeswax, and their mixture. European Journal of Lipid Science and Technology 119 (10):1700032. doi: 10.1002/ejlt.201700032.
- Fayaz, G., S. A. H. Goli, M. Kadivar, F. Valoppi, L. Barba, S. Calligaris, and M. C. Nicoli. 2017. Potential application of pomegranate seed oil oleogels based on monoglycerides, beeswax and propolis wax as partial substitutes of palm oil in functional chocolate spread. Lwt 86:523–9. doi: 10.1016/j.lwt.2017.08.036.
- Fennema, O. R., S. Damodaran, and K. L. Parkin. 2008. Fennema’s food chemistry. Boca Raton, Florida, USA: CRC Press.
- Ferstl, P., C. Eder, W. Ruß, and A. Wierschem. 2011. Pressure induced crystallization of triacylglycerides. High Pressure Research 31 (2):339–49. doi: 10.1080/08957959.2011.582870.
- Figoni, P. 2007. How baking works: Exploring the fundamentals of baking science. Hoboken, New Jersey, USA: John Wiley & Sons, Inc.
- Finkel, G. 1987. Chocolate compositions of increased viscosity and method of preparing such compositions. Google Patents.
- Finkel, G. 1989. Chocolate enrobed wafer products and method for preparing the same. Google Patents.
- Finkel, G. 1990. Chocolate compositions of increased viscosity and method for preparing such compositions. Google Patents.
- FAOSTAT. 2019. Food and Agriculture Organization of the United Nations. Accessed January 10, 2021. https://fao.org/faostat/en/#data/QC.
- Fountain, G. O., and A. L. Penner. 2015. Temperature tolerant chocolate. Google Patents.
- Francis, F. P., and R. Chidambaram. 2019. Hybrid hydrogel dispersed low fat and heat resistant chocolate. Journal of Food Engineering 256:9–17. doi: 10.1016/j.jfoodeng.2019.03.012.
- Friedman, J. 1921. Process of making soluble chocolate. Google Patents.
- Garti, N., and N. R. Widlak. 2012. Cocoa Butter and Related Compounds. Urbana, IL: AOCS Press.
- Giddey, C., and G. Dove. 1991. Aqueous foaming composition and method for homogeneously incorporating water into lipophilic materials. Google Patents.
- Glazier, B. D., K. Wild, J. Wentzel, M. Myers, M. Hess, S. Lease, and D. Hausman. 2014. Heat resistant chocolate. Google Patents.
- Goh, S. H., Y. M. Choo, and S. H. Ong. 1985. Minor constituents of palm oil. Journal of the American Oil Chemists' Society 62 (2):237–40. doi: 10.1007/BF02541384.
- Gunstone, F. D. 2004. The chemistry of oils and fats: Sources, composition, properties and uses. Oxford, UK: Blackwell.
- Granvogl, M., S. Bugan, and P. Schieberle. 2006. Formation of amines and aldehydes from parent amino acids during thermal processing of cocoa and model systems: New insights into pathways of the strecker reaction. Journal of Agricultural and Food Chemistry 54 (5):1730–9. doi: 10.1021/jf0525939.
- Gravelle, A. J., S. Barbut, and A. G. Marangoni. 2012. Ethylcellulose oleogels: Manufacturing considerations and effects of oil oxidation. Food Research International 48 (2):578–83. doi: 10.1016/j.foodres.2012.05.020.
- Hassim, N. A. M., and L. N. H. M. Dian. 2017. Usage of palm oil, palm kernel oil and their fractions as confectionary fats. Journal of Oil Palm Research 29 (3):301–10. doi: 10.21894/jopr.2017.2903.01.
- Hess, S. J., E. J. Windhab, C. S. Curschellas, and W. Hanselamnn. 2010. Temperature resistant chocolate composition and method. Google Patents.
- Hoffmann, S., E. Koos, and N. Willenbacher. 2014. Using capillary bridges to tune stability and flow behavior of food suspensions. Food Hydrocolloids. 40:44–52. doi: 10.1016/j.foodhyd.2014.01.027.
- Hoskin, J. C., and P. S. Dimick. 1984. Role of sulphur compounds in the development of chocolate flavour- a review. Process Biochemistry 19 (4):150–6.
- Hughes, N. E., A. G. Marangoni, A. J. Wright, M. A. Rogers, and J. W. E. Rush. 2009. Potential applications of edible oil organogels. Trends in Food Science & Technology 20 (10):470–80. doi: 10.1016/j.tifs.2009.06.002.
- Hwang, H. S., S. Kim, M. Singh, J. K. Winkler-Moser, and S. X. Liu. 2012. Organogel formation of soybean oil with waxes. Journal of the American Oil Chemists' Society 89 (4):639–47. doi: 10.1007/s11746-011-1953-2.
- Ibrahim, H., E. Sallam, M. Takieddin, and M. A. Shamat. 1988. Dissolution characteristics of interactive powder mixtures. Part one: Effect of solubility and particle size of excipients. Drug Development and Industrial Pharmacy 14 (9):1249–76. doi: 10.3109/03639048809151932.
- Illiyin, M. R., J. M. N. Marikkar, M. Shuhaimi, B. Mahiran, and M. S. Miskandar. 2013. A comparison of the thermo-physical behavior of Engkabang (Shorea marcophylla) seed fat-canola oil blends and lard. Journal of the American Oil Chemists' Society 90 (10):1485–93. doi: 10.1007/s11746-013-2307-z.
- Jahurul, M. A. H., I. S. M. Zaidul, N. A. Nik Norulaini, F. Sahena, B. Y. Kamaruzzaman, K. Ghafoor, and A. K. M. Omar. 2014. Cocoa butter replacers from blends of mango seed fat extracted by supercritical carbon dioxide and palm stearin. Food Research International 65 (C):401–6. doi: 10.1016/j.foodres.2014.06.039.
- Jahurul, M. A. H., I. S. M. Zaidul, N. N. A. Norulaini, F. Sahena, J. M. Jaffri, and A. K. M. Omar. 2014. Supercritical carbon dioxide (SC-CO2) extraction and studies of mango seed kernel for cocoa butter analogy fats. Cyta - Journal of Food 12 (1):97–103. doi: 10.1080/19476337.2013.801038.
- Jahurul, M. H. A., I. S. M. Zaidul, N. A. N. Norulaini, F. Sahena, M. Z. Abedin, K. Ghafoor, and A. K. Mohd Omar. 2014. Characterization of crystallization and melting profiles of blends of mango seed fat and palm oil mid-fraction as cocoa butter replacers using differential scanning calorimetry and pulse nuclear magnetic resonance. Food Research International 55:103–9. doi: 10.1016/j.foodres.2013.10.050.
- Jahurul, M. A. H., I. S. M. Zaidul, N. A. Nik Norulaini, F. Sahena, M. Z. Abedin, A. Mohamed, and A. K. Mohd Omar. 2014. Hard cocoa butter replacers from mango seed fat and palm stearin. Food Chemistry 154:323–9. doi: 10.1016/j.foodchem.2013.11.098.
- Jahurul, M. A. H., I. S. M. Zaidul, N. A. N. Norulaini, F. Sahena, S. Jinap, J. Azmi, K. M. Sharif, and A. K. Mohd Omar. 2013. Cocoa butter fats and possibilities of substitution on foods products concerning cocoa varieties, alternative sources, extraction methods, composition, and characteristics. Journal of Food Engineering 117 (4):467–76. doi: 10.1016/j.jfoodeng.2012.09.024.
- Jahurul, M. A. H., L. L. Ping, M. S. Sharifudin, M. Hasmadi, A. H. Mansoor, J. S. Lee, B. W. Noorakmar, H. M. S. Amir, S. Jinap, A. K. Mohd Amir, et al. 2019. Thermal properties, triglycerides and crystal morphology of Bambangan (Mangifera pajang) kernel fat and palm stearin blends as cocoa butter alternatives. Lwt 107:64–71. doi: 10.1016/j.lwt.2019.02.053.
- Jahurul, M. A. H., Y. Soon, M. S. Sharifudin, M. Hasmadi, A. H. Mansoor, I. S. M. Zaidul, J. S. Lee, M. E. Ali, K. Ghafoor, W. Zzaman, et al. 2018. Bambangan (Mangifera pajang) kernel fat: A potential new source of cocoa butter alternative. International Journal of Food Science & Technology 53 (7):1689–97. doi: 10.1111/ijfs.13753.
- Janak, J. 1987. Thin-layer chromatography with flame ionization detection, 86. Norwell, MA: Kluwer Academic Press.
- Jeffery, M. S. 1979. Chocolate material and method of manufacturing same. Google Patents.
- Jeyarani, T., and S. Y. Reddy. 1999. Heat-resistant cocoa butter extenders from mahua (Madhuca latifolia) and kokum (Garcinia indica) fats. Journal of the American Oil Chemists' Society 76 (12):1431–6. doi: 10.1007/s11746-999-0180-6.
- Jin, J., C. C. Akoh, Q. Jin, and X. Wang. 2018. Preparation of mango kernel fat stearin-based had chocolate fats via physical blending and enzymatic interesterification. Lwt 97:308–16. doi: 10.1016/j.lwt.2018.07.018.
- Jin, J., L. Jie, L. Zheng, M. Cheng, D. Xie, Q. Jin, and X. Wang. 2018. Characteristics of palm mid-fractions produced from different fractionation paths and their potential usages. International Journal of Food Properties 21 (1):58–69. doi: 10.1080/10942912.2018.1437632.
- Jin, J., H. Mu, Y. Wang, W. Pembe, Y. Liu, J. Huang, Q. Jin, and X. Wang. 2017. Production of high-melting symmetrical monounsaturated triacylglycerol-rich fats from mango kernel fat by acetone fractionation. Journal of the American Oil Chemists' Society 94 (2):201–13. doi: 10.1007/s11746-016-2929-z.
- Jin, J., L. Zheng, W. M. Pembe, J. Zhang, D. Xie, X. Wang, J. Huang, Q. Jin, and X. Wang. 2017. Production of sn-1,3-distearoyl-2-oleoyl-glycerol-rich fats from mango kernel fat by selective fractionation using 2-methylpentane based isohexane. Food Chemistry 234:46–54. doi: 10.1016/j.foodchem.2017.04.165.
- Jin, J., P. Warda, C. Qi, C. Sun, L. Jie, D. Xie, J. Huang, Q. Jin, and X. Wang. 2016. Mango kernel fat based chocolate fat with heat resistant triglycerols: Production via blending using mango kernel fat mid-fraction and palm mid-fractions produced in different fractionation paths. RSC Advances 6 (110):108981–8. doi: 10.1039/C6RA19438A.
- Jin, J., Q. Jin, X. Wang, and C. C. Akoh. 2019. Improving heat and fat bloom stabilities of “dark chocolates” by addition of mango kernel fat-based chocolate fats. Journal of Food Engineering 246:33–41. doi: 10.1016/j.jfoodeng.2018.10.027.
- Karupaiah, T., and K. Sundram. 2007. Effects of stereospecific positioning of fatty acids in triacylglycerol structures in native and randomized fats: A review of their nutritional implications. Nutrition & Metabolism 4:16. doi: 10.1186/1743-7075-4-16.
- Kaushik, I., Jain, A. R. B. Grewal, S. Siddiqui, and R. Gahlot, Rekha, Anju. 2017. Organogelation: It’s food applications. MOJ Food Processing & Technology 4 (2):66–72. doi: 10.15406/mojfpt.2017.04.00089.
- Kempf, N. W. 1958. Chocolate product and process. Google Patents.
- Kenyon, M. M. 1995. Modified starch, maltodextrin, and corn syrup solids as wall materials for food encapsulation. American Chemical Society Symposium Series 590. doi: 10.1021/bk-1995-0590.ch004.
- Keogh, M. K., C. A. Murray, and B. T. O’Kennedy. 2003. Effects of ultrafiltration of whole milk on some properties of spray-dired milk powders. International Dairy Journal 13 (12):995–1002. doi: 10.1016/S0958-6946(03)00123-7.
- Killian, L. A., and J. N. Coupland. 2012. Manufacture and application of water-in-oil emulsions to induce the aggregation of sucrose crystals in oil: A model for melt resistant chocolate. Food Biophysics 7 (2):124–31. doi: 10.1007/s11483-012-9249-0.
- Kincs, F. R. 1992. Structured fat emulsion in confectionary coating. Google Patents.
- Kriel, T., A. Holt, A. Cattaruzza, A. Marangoni, and T. Stortz. 2017. Confectionary production. Google Patents.
- Kuo, T. M., and H. W. Gardner. 2002. Lipid biotechnology. New Your, USA: Taylor & Francis, CRC Press.
- Lataner, H. 1949. Edible product and method of making the same. Google Patents.
- Laughter, J. A. 2013. Understanding heat resistant structure formation in white chocolate. M.Sc. Thesis., The Pennsylvania State University, Pennsylvania, USA.
- Lillah, A., Asghar, I. Pasha, G. Murtuza, and M. Ali. 2017. Improving heat stability along with quality of compound dark chocolate by adding optimized cocoa butter substitute (hydrogenated palm kernel stearin) emulsion. LWT- Food Science and Technology 80:531–6. doi: 10.1016/j.lwt.2017.02.042.
- Liu, W. J. H. 2011. Traditional herbal medicine research method: Identification, analysis, bioassay, and pharmaceutical and clinical studies. Hoboken, NJe: John Wiley & Sons, Inc.
- Loisel, C., G. Keller, G. Lecq, C. Bourgaux, and M. Ollivon. 1998. Phase transitions and polymorphism of cocoa butter. Journal of the American Oil Chemists' Society 75 (4):425–39. doi: 10.1007/s11746-998-0245-y.
- Lonchampt, P., and R. W. Hartel. 2004. Fat bloom in chocolate and compound coatings. European Journal of Lipid Science and Technology 106 (4):241–74. doi: 10.1002/ejlt.200400938.
- Lopez-Martínez, A., M. A. Charó-Alonso, A. G. Marangoni, and J. F. Toro-Vazquez. 2015. Monoglyceride organogels developed in vegetable oil with and without ethylcellulose. Food Research International 72:37–46. doi: 10.1016/j.foodres.2015.03.019.
- Macrae, R. A., and W. A. Bench. (Unilever). 1983. Edible fat process. Google Patents.
- Maheshwari, B., and S. Y. Reddy. 2005. Application of kokum (Garcinia indica) fat as cocoa butter improver in chocolate. Journal of the Science of Food and Agriculture 85 (1):135–40. doi: 10.1002/jsfa.1967.
- Mandralis, Z. I., D. P. Weitzenecker. 2001. Heat resistant chocolate-based confectionary products. Google Patents.
- Marangoni, A. G. 2010. Chocolate compositions containing ethylcellulose. Google Patents
- Marangoni, A. G. 2015. Polymer gelation of oils. Google Patents.
- Marangoni, A. G., and N. Garti. 2011. An overview of the past, present, and future of organogels. In Edible oleogels: Structure and health implications, 1–17. Urbana, IL: AOCS Press.
- Marangoni, A. G., and S. E. McGauley. 2003. Relationship between Crystallization Behavior and Structure in Cocoa Butter. Crystal Growth & Design 3 (1):95–108. doi: 10.1021/cg025580l.
- Mohamed, I. O. 2012. Lipase-catalyzed synthesis of cocoa butter equivalent from palm olein and saturated fatty acid distillate from palm oil physical refinery. Applied Biochemistry and Biotechnology 168 (6):1405–15. doi: 10.1007/s12010-012-9866-6.
- Murdan, S., G. Gregoriadis, and A. T. Florence. 1999. Novel sorbitan monostearate organogels. Journal of Pharmaceutical Sciences 88 (6):608–14. doi: 10.1021/js980342r.
- Nielsen, J. M., S. B. Lund, E. Lynenskjold, and M. D. Laursen. 2016. Process for the preparation of a heat-resistant chocolate preparation. Google Patents.
- Nijssen, L. M., C. A. Visscher, H. Maarse, L. C. Willemsens, and M. H. Boelens. 1996. Volatile compounds in food. Qualitative and quantitative data. Zeist, The Netherlands: TNO Nutrition and Food Research Institute.
- Norazlina, M. R., M. H. A. Jahurul, M. Hasmadi, M. S. Sharifudin, M. Patricia, A. H. Mansoor, and J. S. Lee. 2020. Characteristics of Bambangan kernel fat fractions produced by solvent fractionation and their potential industrial applications. Journal of Food Processing and Preservation 44 (6): e14446. doi: 10.1111/jfpp.14446.
- Norazlina, M. R., M. H. A. Jahurul, M. Hasmadi, M. S. Sharifudin, M. Patricia, J. S. Lee, H. M. S. Amir, A. W. Noorakmar, and I. Riman. 2020. Effects of fractionation technique on triacylglycerols, melting and crystallization and the polymorphic behavior of bambangan kernel fat as cocoa butter improver. LWT- Food Science and Technology 129: 109558. doi: 10.1016/j.lwt.2020.109558.
- Noznick, P. P., C. W. Tatter, and C. F. Obenauf. 1963. Process for preparing a compressed chocolate chip product and the resulting product. Google Patents.
- Ogunwolu, S. O., and C. O. Jayeola. 2006. Development of non-conventional thermo-resistant chocolate for the tropics. British Food Journal 108 (6):451–5. doi: 10.1108/00070700610668423.
- Oonishi, K., and I. Hachiya. 2016. Method for manufacturing water-containing heat-resistant chocolate, water-containing heat-resistant chocolate, method for suppressing increase in viscosity of water-containing chocolate mix, and method for forming saccharide skeleton in water-containing heat-resistant chocolate. Google Patents.
- Oonishi, K., Y. Toyoma, and N. Murayama. 2017. Heat-resistant chocolate and method of manufacturing the same. Google Patents.
- Patel, A. R., P. S. Rajarethinem, A. Grędowska, O. Turhan, A. Lesaffer, W. H. D. Vos, D. V. de Walle, and K. Dewettinck. 2014. Edible applications of shellac oleogels: Spreads, chocolate paste and cakes. Food Function 5 (4):645–52. doi: 10.1039/C4FO00034J.
- Patel, A. R., R. A. Nicholson, and A. G. Marangoni. 2020. Applications of fat mimetics for the replacement of saturated and hydrogenated fat in food products. Current Opinion in Food Science 33:61–8. doi: 10.1016/j.cofs.2019.12.008.
- Peyronel, F., and A. G. Marangoni. 2014. In search of confectionary fat blends stable to heat: Hydrogenated palm kernel oil stearin with sorbitan monostearate. Food Research International 55:93–102. doi: 10.1016/j.foodres.2013.10.036.
- Pieve, S. D., S. Calligaris, E. Co, M. C. Nicoli, and A. G. Marangoni. 2010. Shear nanostructuring of monoglyceride organogels. Food Biophysics 5 (3):211–7. doi: 10.1007/s11483-010-9162-3.
- Potter, S. J. 2014. Heat resistant coatings, confections comprising the coatings and methods of making these. Google Patents.
- Prinz, J. F., and R. A. de Wijk. 2004. The role of oral processing inflavour perception. In Flavor perception, ed. A.J. Taylor and D.D. Roberts, 39–56. Oxford, UK: Blackwell Publishing. doi: 10.1002/9780470995716.ch2.
- Quarterly Bulletin of Cocoa Statistics. 2020. Volume XLVI, Issue No., 4 - Cocoa Year 2019-2020. International Cocoa Organization (ICCO). Accessed January 10, 2021. https://www.icco.org/statistics/.
- Ramli, N., O. Hassan, M. Said, W. Samsudin, and N. A. Idris. 2006. Influence of roasting condition on volatile flavor of roasted Malaysian cocoa beans. Journal of Food Processing and Preservation 30 (3):280–98. doi: 10.1111/j.1745-4549.2006.00065.x.
- Rector, D. 2000. Chocolate – Controlling the flow: Benefits of polyglycerol polyricinoleic acid. The Manufacturing Confectioner 80 (5):63–70.
- Reddy, S. Y., and T. Jeyarani. 2001. Trans-free bakery shortenings from mango kernel and mahua fats by fractionation and blending. Journal of the American Oil Chemists' Society 78 (6):635–40. doi: 10.1007/s11746-001-0318-8.
- Reddy, S. Y., and J. V. Prabhakar. 1994. Cocoa butter extenders from Kokum (Garcinia indica) and Phulwara (Madhuca butyracea) butter. Journal of the American Oil Chemists' Society 71 (2):217–9. doi: 10.1007/BF02541559.
- Rios, R. V., M. D. F. Pessanha, PFd Almeida, C. L. Viana, and SCdS. Lannes. 2014. Application of fats in some food products. Food Science and Technology (Campinas) 34 (1):3–15. doi: 10.1590/S0101-20612014000100001.
- Rosales, C. K., U. Klinkesorn, and S. Suwonsichon. 2017a. Effect of crystal promoters on viscosity and melting characteristics of compound chocolate. International Journal of Food Properties 20 (1):119–32. doi: 10.1080/10942912.2016.1147458.
- Rosales, C. K., S. Suwonsichon, and U. Klinkesorn. 2017b. Ability of crystal promoters to delay fat bloom development in heat-resistant compound chocolate with or without the presence of crystal inhibitor. International Journal of Food Science & Technology 52 (11):2343–51. doi: 10.1111/ijfs.13517.
- Rousseau, D., A. Coutouly, P. Hendricks, S. Hodge, and N. L. Green. 2015. Development of an extraction protocol for the removal of the fat phase within chocolate. Lwt - Food Science and Technology 64 (1):61–6. doi: 10.1016/j.lwt.2015.05.019.
- Salas, J. J., M. A. Bootello, E. Martínez-Force, and R. Garcés. 2011. Production of stearate-rich butters by solvent fractionation of high stearic-high oleic sunflower oil. Food Chemistry 124 (2):450–8. doi: 10.1016/j.foodchem.2010.06.053.
- Schenk, H., and R. Peschar. 2004. Understanding the structure of chocolate. Radiation Physics and Chemistry 71 (3-4):829–35. doi: 10.1016/j.radphyschem.2004.04.105.
- Schnermann, P., and P. Schieberle. 1997. Evaluation of Key Odorants in Milk Chocolate and Cocoa Mass by Aroma Extract Dilution Analyses. Journal of Agricultural and Food Chemistry 45 (3):867–72. doi: 10.1021/jf960670h.
- Silva, R. C., F. A. S. D. M. Soares, J. M. Maruyama, N. R. Dagostinho, Y. A. Silva, G. A. Calligaris, A. P. B. Ribeiro, L. P. Cardoso, and L. A. Gioielli. 2014. Effect of diacylglycerol addition on crystallization properties of pure triglycerols. Food Research International 55:436–44. doi: 10.1016/j.foodres.2013.11.037.
- Sintang, M. D. B., T. Rimaux, D. V. de Walle, K. Dewettinck, and A. R. Patel. 2017. Oil structuring properties of monoglycerides and phytosterols mixtures. European Journal of Lipid Science and Technology 119 (3):1500517–4. doi: 10.1002/ejlt.201500517.
- Sivasankar, B. 2002. Food processing and preservation. New Delhi, India: PHI Learning Pvt Ltd.
- Soekopitojo, S.,. P. Hariyadi, T. R. Muchtadi, and N. Andarwulan. 2009. Enzymatic interesterification of palm oil mid-fraction blends for the production of cocoa butter equivalents. Asian Journal of Food and Agro-Industry 2 (4):807–16.
- Solís-Fuentes, J. A., and M. C. Durán-de-Bazúa. 2004. Mango seed uses: Thermal behaviour of mango seed almond fat and its mixtures with cocoa butter. Bioresource Technology 92 (1):71–8. doi: 10.1016/j.biortech.2003.07.003.
- Solís-Fuentes, J. A., M. del Rosario, M. Hernandez, M. del Carmen, and C. Durán-de-Bazúa. 2005. Determination of the predominant polymorphic form of mango (Mangifera indica) almond fat by differential scanning calorimetry and X-ray diffraction. European Journal of Lipid Science and Technology 107 (6):395–401. doi: 10.1002/ejlt.200401072.
- Sonwai, S.,. P. Kaphueakngam, and A. Flood. 2014. Blending of mango kernel fat and palm oil mid-fraction to obtain cocoa butter equivalent. Journal of Food Science and Technology 51 (10):2357–69. doi: 10.1007/s13197-012-0808-7.
- Stortz, T. A., and A. G. Marangoni. 2011. Heat resistant chocolate. Trends in Food Science & Technology 22 (5):201–14. doi: 10.1016/j.tifs.2011.02.001.
- Stortz, T. A., and A. G. Marangoni. 2013. Ethylcellulose solvent substitution method of preparing heat resistant chocolate. Food Research International 51 (2):797–803. doi: 10.1016/j.foodres.2013.01.059.
- Stortz, T. A., D. C. D. Moura, T. Laredo, and A. G. Marangoni. 2014. Molecular interactions of ethylcellulose with sucrose particles. RSC Advances 4 (98):55048–61. doi: 10.1039/C4RA12010H.
- Stortz, T. A., T. Laredo, and A. G. Marangoni. 2015. The role of lecithin and solvent addition in ethylcellulose-stabilized heat resistant chocolate. Food Biophysics 10 (3):253–63. doi: 10.1007/s11483-014-9379-7.
- Takemori, T., T. Tsurumi, M. Takagi, and M. Ito. 1993. Heat-resistant chocolate and production method thereof. Google Patents.
- Talbot, G. 1999. Chocolate temper. In Industrial chocolate manufacture and use, 218–30. Oxford, UK: Blackwell Science.
- Talbot, G. 2009. Compound Coatings. In Science and technology of enrobed and filled chocolate, confectionary and bakery products, 80–100. Cambridge, UK: Woodhead Publishing Ltd.
- Teberikler, L., S. Koseoglu, and A. Akgerman. 2001. Selective extraction of phosphatidylcholine from lecithin by supercritical carbon dioxide/ethanol mixture. Journal of the American Oil Chemists' Society 78 (2):115–20. doi: 10.1007/s11746-001-0230-2.
- Toro-Vazquez, J. F., D. Péréz-Martínez, E. Dibildox-Alvarado, M. Charó-Alonso, and J. Reyes-Hernández. 2004. Rheometry and polymorphism of cocoa butter during crystallization under static and stirring conditions. Journal of the American Oil Chemists' Society 81 (2):195–202. doi: 10.1007/s11746-004-0881-z.
- Torres-Moreno, M., E. Torrescasana, J. Salas-Salvadó, and C. Blanch. 2015. Nutritional composition and fatty acids profile in cocoa beans and chocolates with different geographical origin and processing conditions. Food Chemistry 166 (1):125–32. doi: 10.1016/j.foodchem.2014.05.141.
- Tran, P. D., D. V. de Walle, M. Hinneh, C. Delbaere, N. D. Clercq, D. N. Tran, and K. Dewettinck. 2015. Controlling the stability of chocolates through the incorporation of soft and hard StOSt-rich fats. European Journal of Lipid Science and Technology 117 (11):1700–13. doi: 10.1002/ejlt.201400584.
- Wang, X., B. Baker, D. Worthing, M. J. P. Gonzalez, and G. Mongia. 2014. Method of making a heat stable chocolate confectionary product. Google Patents.
- Wang, X., M. J. P. Gonzalez, B. D. Brown, S. M. Benjamin, C. Hoffman, D. Worthing, and D. Teets. 2016. Heat stable chocolate confectionary product and method of making same. Google Patents.
- Wendt, A., K. Abraham, C. Wernecke, J. Pfeiffer, and E. Flöter. 2017. Application of β- Sitosterol + γ- Oryzanol- Structured Organogel as Migration Barrier in Filled Chocolate Products. Journal of the American Oil Chemists' Society 94 (9):1131–40. doi: 10.1007/s11746-017-3024-9.
- Wentzel, J., D., Hausman, B. D. Glazier, and G. C. Tweedie. 2014. Heat resistant chocolate. Google patents.
- Wentzel, J., B. D. Glazier, M. V. Damme, and I. Bernarda. 2015. Heat resistant confections. Google Patents.
- Yamoneka, J., P. Malumba, G. Lognay, F. Béra, C. Blecker, and S. Danthine. 2018. Enzymatic inter-esterification of binary blends containing Irvingia gabonensis seed fat to produce cocoa butter substitute. European Journal of Lipid Science and Technology 120 (4):1700423. doi: 10.1002/ejlt.201700423.
- Zhang, Z., J. Song, W. J. Lee, X. Xie, and Y. Wang. 2020. Characterization of enzymatically interesterified palm oil-based fats and its potential application as cocoa butter substitute. Food Chemistry 318:126518. doi: 10.1016/j.foodchem.2020.126518.
- Zhang, Z.,. W. J. Lee, and Y. Wang. 2020. Evaluation of enzymatic interesterification in structured triglycerols preparation: A concise review and prospect. Critical Reviews in Food Science and Nutrition. Advance online publication. doi: 10.1080/10408398.2020.1793725..
- Ziegleder, G. 1991. Composition of flavor extracts of raw and roasted cocoas. Zeitschrift f⏧r Lebensmittel-Untersuchung und -Forschung 192 (6):521–5. doi: 10.1007/BF01202506.
- Ziegleder, G., and B. Biehl. 1988. Analysis of cocoa flavor precursors. In Modern methods of plant analysis, new series, Volume 8. Analysis of Nonalcoholic Beverages, 321–93. Berlin/West, Germany: Springer.