Abstract
Brain aging is one of the unavoidable aspects of geriatric life. As one ages, changes such as the shrinking of certain parts (particularly the frontal cortex, which is vital to learning and other complex mental activities) of the brain may occur. Consequently, communications between neurons are less effective, and blood flow to the brain could also decrease. Efforts made at the biological level for repair become inadequate, leading to the accumulation of β-amyloid peptide in the brain faster than its probable degradation mechanism, resulting in cognitive malfunction. Subsequent clinical usage of drugs in battling related brain-aging ailments has been associated with several undesirable side effects. However, recent research has investigated the potential use of natural compounds from food in combating such occurrences. This review provides information about the use of Piper guineense (black pepper) as a possible agent in managing brain aging because of its implications for practical brain function. P. guineense contains an alkaloid (piperine) reported to be an antioxidant, anti-depressant, and central nervous system stimulant. This alkaloid and other related compounds are neuroprotective agents that reduce lipid oxidation and inhibit tangles in the brain tissues.
Introduction
Aging is a progressive and unavoidable biological process, which results in several physiological changes in the body. Aging may be due to programmed factors, where it is normal for cells to grow, develop, age, and subsequently die, or damage-related factors where there is accumulated internal or external damage to cells. Accumulated oxidative stress, increased nucleic acid instability, reduced metabolic efficiency, and hormonal imbalances are some of the molecular mechanisms equally implicated in the aging process (Choi et al. Citation2021).
The brain also goes through an aging process due to pathophysiological changes throughout an individual’s lifespan. Various external factors, infections, and inflammation of the human system lead to cellular, subcellular, and tissue abnormalities, resulting in adaptation of the neurons and neurodegeneration that culminates in the complex phenomenon of brain aging (Nikhra Citation2017). Reports have shown that the brain does not age regularly or uniformly. Some areas are more sensitive to aging than others, especially the prefrontal areas (Wahl et al. Citation2019). It has been reported that at least about 10% decrease in brain weight occurs in men and women as they age between 25 and 75 years (Wang et al. Citation2013), due to overall reduction in brain volume caused mainly by gray matter shrinkage, especially in the hippocampus and prefrontal cortex (Wahl et al. Citation2019).
A study has shown that as the brain ages, abnormal protein assembly, and abnormal lysosomes occur more frequently, indicating the presence of amyloid plaques, and neuronal loss (Wyss-Coray Citation2016). Some other subcellular structures with cross-linked proteins, lipids, and carbohydrates accumulate either in extracellular space or inside glial cells or neuronal cells apart from the classic protein deposits in the aging brain (Safaiyan et al. Citation2016). The effect of these abnormalities in the pathophysiology of neurodegenerative diseases is not apparent. A study has shown that the accumulation of proteins due to their over-expression resulted in the progression of Alzheimer’s disease in genetically manipulated transgenic mice (Wyss-Coray Citation2016). Granules formed in response to stress have also been implicated in amyotrophic lateral sclerosis (ALS) and frontotemporal dementia (Ash et al. Citation2014).
These aging processes have been discovered to accompany other cognitive and behavioral changes (Laks and Engelhardt Citation2010). Aging-related cognitive decline varies in different individuals. Depending on lifestyles (exercise, diet, alcohol intake, etc.) (Peters Citation2006), environmental factors (Mattson and Arumugam Citation2018), education, and other intellectually stimulating activities that could affect the brain cells growth and regeneration (Nikhra Citation2017). With the increasing proportion of the aged population (people over 60 years) projected to double by 2050 (World Health Organization Citation2018), there is a need to study interventions that can delay the onset of brain aging-related diseases. This will ensure the quality of life of the aged and reduce the burden associated with these diseases (Mattson and Arumugam Citation2018; Wahl et al. Citation2019).
Piper guineense (black pepper) is a known species of the Piperaceae family grown in Africa and other parts of the world, majorly for its culinary purpose. The fruit (pepper) is one of the most used spices around the globe. It possesses a pungent aromatic smell making it suitable as a flavoring and seasoning agent in food. Apart from its use in culinary preparations, it has found application for medicinal, cosmetics, and insecticidal purposes (Anyanwu and Nwosu Citation2014; Ogbunugafor, Ugochukwu, and Kyrian-Ogbonna Citation2017). P. guineense has high nutritional qualities and is rich in vitamins and minerals (Ezenobi, Amaku, and Agbidi Citation2016; Imo et al. Citation2018). Studies have shown that the plant possesses antioxidant, anticonvulsant, anti-inflammatory, and neuropharmacological activities (Oyemitan et al. Citation2015; Salehi et al. Citation2019). Reports have shown that bioactive compounds in P. guineense, such as piperine, phenolic acids, and antioxidants, can alleviate oxidative stress and improve memory deficit (Mirmosayyeb et al. Citation2017; Oyemitan et al. Citation2015 Butt et al. 2013).
According to Aluko (Citation2021), synthetic drugs are also available for the treatment of brain aging-related diseases. However, studies have shown that the clinical usage of these drugs causes several undesirable side effects such as nausea, cramps, decreased appetite, and bradycardia. Since food-derived compounds may be better metabolized and tolerated by the human system (Aluko Citation2021), there is a need to investigate the potential use of food-related options, such as P. guineense, for brain-aging management.
Mechanisms involved in brain aging and associated diseases
The brain ages biologically via cellular and molecular mechanisms. These include inflammation, dysregulated energy metabolism, mitochondrial dysfunction, dysregulated neuronal Ca2+ handling, and so on (Mattson and Arumugam Citation2018). When the body tries removing pathogens, foreign bodies, and damaged cells that result from acute cellular stress or aging, it experiences some low level of chronic inflammation. These inflammatory responses cause oxidative damage that brings about pathological changes (such as a decrease in some neuronal populations, dendritic spines, presynaptic markers, and cortical volume), resulting in cognitive impairment, and memory loss (Chinta et al. Citation2015).
However, biological aging might not progress as fast as chronological aging due to environmental factors such as diet, exercise, emotional trauma, chemical exposure, and so on. Traumatic brain injury (TBI) or emotional trauma experienced earlier in life could cause late-life cognitive impairment (Dams-O’Connor et al. Citation2016). A report has it that sport-related TBI has been associated with a higher risk of several neurodegenerative diseases such as Alzheimer’s disease (AD), multiple sclerosis, and Parkinson’s disease (Esopenko and Levine Citation2015). The accumulation of environmental pollutants and chemicals in the body system has also been found to cause cognitive decline (Grossman Citation2014) linked to symptoms such as anxiety, tension, hostility, and depression. For instance, occupational exposure to lead (Pb) induces oxidative stress, mitochondrial damage, neural apoptosis, and hippocampal changes (Fenga et al. Citation2016). While exposure to cadmium (Cd) hinder the release of acetylcholine (Ach), thereby inhibiting synaptic neurotransmission in such individual (Wang and Du Citation2013).
A schematic diagram in theoretically describes the biological aging and the hallmarks of the aging brain. In simple terms, a healthy person is at higher risk of cognitive impairment and brain diseases with increasing age and might eventually reach a threshold where symptoms will appear. Cognitive decline and neurodegenerative diseases associated with brain aging include mild cognitive impairment, anxiety, depression, Alzheimer’s, and Parkinson’s disease, with dementia being the endpoint to most age-related neuropathologies. The subsequent subsections discussed these brain-aging diseases.
Figure 1. Paths of brain aging (Adapted from Mattson and Arumugam Citation2018; UCDavis).
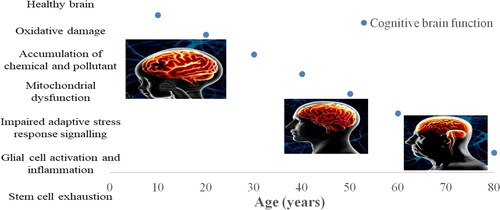
Alzheimer’s disease and brain aging
The fifth leading cause of death due to brain aging among the geriatric population is Alzheimer’s disease. It accounts for about 80% of dementia cases in persons over 65 years (Alzheimer’s Association Citation2020). Alzheimer’s disease is a neurodegenerative disorder clinically characterized by progressive loss of memory, cognitive function, and adverse behavioral changes (Adewusi and Steenkamp Citation2011). It has become a significant public health concern since about 25 million people have been estimated to be suffering from the disease worldwide, and over 5 million new cases are reported annually (Tobore Citation2019).
Several cellular and molecular abnormalities have been associated with the pathophysiology of AD (). A disruption in neuronal connection causing massive loss of neurons throughout the brain is a common feature of Alzheimer’s disease. The β-amyloid peptide is a product of the segmental cleavage of amyloid-β protein precursor (AβPP), its accumulation in the brain forms plaques that further cause neuropathological lesions. Another abnormality observed is the hyperphosphorylation of tau protein which is a vital component of neurofibrillary tangles (Hritcu et al. Citation2015). However, one majorly reported feature responsible for memory deficits in Alzheimer’s disease is synaptic dysfunction, and this coincides with the onset of memory loss in a mouse model (Rudy et al. Citation2015). A dysregulation of excitatory glutamatergic neurotransmitters by soluble amyloid-β peptides causes synaptic alterations and tau phosphorylation (Rudy et al. Citation2015).
Figure 2. Hypothesis for the pathophysiology of Alzheimer’s disease (Adapted from Kumar Thakur et al. Citation2018).
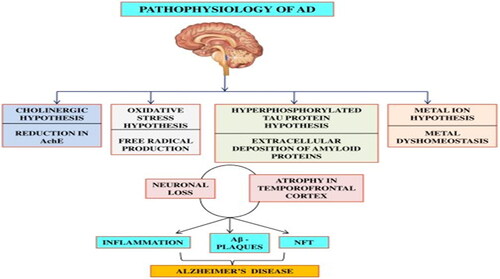
Ca2+ is a ubiquitous intracellular messenger that helps to regulate several physiological functions. Dysregulation in Ca2+ homeostasis has been implicated in many molecular alterations observed in Alzheimer’s disease, such as synaptic loss, mitochondrial dysfunction, oxidative stress, inflammation, aggregation of β-amyloid peptide, and neuronal death (Magi et al. Citation2016). Several reports have stated that oxidative stress is critical in β-amyloid induced neurotoxicity and pathogenesis of Alzheimer’s disease (Butterfield and Boyd-Kimball Citation2018; Cai, Zhao, and Ratka Citation2011; Cheignon et al. Citation2018; Montiel et al. Citation2006; Zhao and Zhao Citation2013). This is because free radicals lead to increased levels of protein oxidation, lipid peroxidation, and oxidative damage to the mitochondria due to the ability of the β-amyloid peptide to act as pro-oxidant. Thus clearly leading to rapid downward spiraling into Alzheimer’s disease (Martorell et al. Citation2013). People with Alzheimer’s disease exhibit emotional instabilities like phobia, anxiety, and psychiatric symptoms in conjunction with cognitive decline. This emotional behavior can be attributed to the amygdala, a part of the temporal lobe affected by β-amyloid at the early stage of Alzheimer’s disease (Hritcu et al. Citation2015).
Parkinson’s disease and brain aging
Parkinson’s disease is the second most common cause of motor disability in geriatrics after stroke, affecting about 6.3 million worldwide (Doria et al. Citation2016). The pathophysiology of the disease is shown in . It is clinically characterized by movement disorders such as resting tremor, rigidity, and bradykinesia, with postural instability occurring later (Doria et al. Citation2016; Kouli, Torsney, and Kuan Citation2018). This disorder in movement has been linked to the loss of dopaminergic neurons that secrete dopamine, a neuromodulator vital in controlling the ease and balance of movement (Chang and Chen Citation2020) and helping in processing cortical information carried by the glutamatergic synapses (Benazzouz et al. Citation2014). Evidence has shown that a cascade of events brought about by oxidative damage and mitochondrial dysfunction are factors that lead to the degeneration of these dopaminergic neurons (Dias, Junn, and Mouradian Citation2013). The increased level of oxidative stress and loss of dopaminergic neurons in Parkinson’s disease has also been associated with high metals (Fe and Ca) in the substantia nigra (Chang and Chen Citation2020). These metals though necessary for the synthesis and action of dopamine, have been found to react with superoxide ion (O2−) and hydrogen peroxide radicals (H2O2) to generate the hydroxyl radical (OH−), which is toxic to the neurons when their concentration is more than required (Chang and Chen Citation2020).
Figure 3. Schematic diagram showing the different factors in play in the pathophysiology of Parkinson’s disease (Adapted from Farooqui and Farooqui Citation2011).
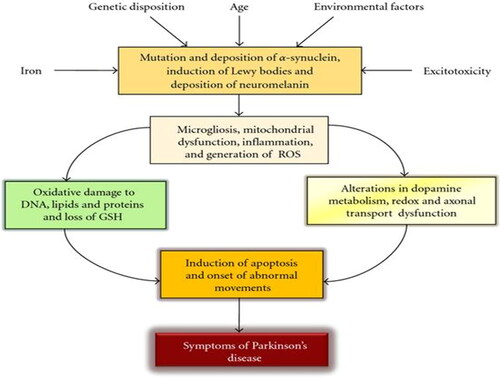
In another report, apart from the loss of dopaminergic neurons in the substantia nigra pars compacta, the loss or degeneration of serotonergic, noradrenergic, cholinergic, and glutamatergic neurons is a feature in the pathology of Parkinson’s disease (Ztaou and Amalric Citation2019). Other non-motor features of Parkinson’s disease include disturbed sleep, hyposmia, dysautonomia, psychiatric, and behavioral troubles. These often precede the motor features by decades and are quite challenging to diagnose. It has also been shown that Parkinson’s disease may start from the peripheral autonomous nervous system or the olfactory bulbs before spreading to the central nervous system and then affecting the substantia nigra (Postuma et al. Citation2012).
Anxiety, depression, and brain aging
Anxiety and depression are the most significant cause of disability of all neuropsychiatric disorders among the geriatric population. They are life-threatening with symptoms such as low mood and anhedonia (Sibille Citation2013). Anxiety/depression contributes to cognitive decline, especially in AD patients (Wuwongse, Chang, and Law Citation2010). Anxiety is rifer in persons with dementia than in those without dementia. It is associated with poor quality of life, behavioral problems, limitations in activities of daily living, sleep disorders, and ineffective neuropsychological performance, even after controlling for depression (Kwak, Yang, and Koo Citation2017). Chronic stress, inflammation, and metabolic syndrome also contribute to or cause depression (Sibille Citation2013). Some changes in gene expression with age could equally promote neurological and neuropsychiatric disorders, depression inclusive. For instance, suppressing genes coding for some neuropeptides during aging could lead to the downregulation of such neuropeptides, thus increasing susceptibility to neurodegenerative and neuropsychiatric disorders (Rakofsky, Ressler, and Dunlop Citation2012).
Reports have also shown that depression increases the risk of morbidities such as stroke, cognitive impairment, cerebrovascular and cardiovascular diseases, and death (Buigues et al. Citation2015; Penninx Citation2017; Taylor, Aizenstein, and Alexopoulos Citation2013). Several psychosocial (unemployment, divorce/widowhood, and social isolation) and medical problems have been signified as stressors causing inflammatory responses, suppressed neurogenesis, or increased oxidative stress, which may lead to depression, thus triggering low mood anhedonia (Alexopoulos Citation2019).
Neurodegenerative diseases are usually not curable but can be managed or their progression delayed. Most drugs, such as levodopa, ropinirole (dopaminergic), used in the treatment of Parkinson’s disease and other motor disorders (Lee Citation2019); donepezil, galantamine (cholinesterase inhibitors), used in the treatment of cognitive disorders (Hogan et al. Citation2008); and antipsychotic drugs (chlorpromazine, haloperidol, aripiprazole, and benzodiazepines), used for the management of behavioral and psychological disorders (Tifratene et al. Citation2017) are symptomatic (Chen and Pan Citation2015). These drugs have been associated with several undesirable side effects, including decreased heart rate, decreased appetite, and loss of weight (Aluko Citation2021). Hence, the direction has been shifted toward the use of some foods with acclaimed anti-neurodegenerative properties to prevent and manage these geriatric brain aging conditions. One such acclaimed food/plant is P. guineense (African black pepper) (Salehi et al. Citation2019).
Piper guineense and its role in managing brain aging
Piper guineense (), also known as African black pepper, uziza in South-Eastern Nigeria, iyere in South-Western Nigeria, and Ashanti pepper in Ghana, is a plant from the family Piperaceae and genus Piper. Some of the phytochemical constituents in P. guineense that are majorly responsible for anti-brain aging effects are the massive amount of polyphenols, flavonoids, alkaloid “piperine,” and related compounds () (Isikhuemen, Ogbomwan, and Efenudu Citation2020; Luca et al. Citation2021; Sulaimon et al. Citation2020). The phytochemical compounds present in P. guineense and other Piper spp. and the amount of extracted piperine content with their analytical extraction methods are summarized in .
Figure 4. (a). P. guineense fresh leaves, ripened bunches of fruits and dried fruits (black pepper) (Imo et al. Citation2018; Oyemitan, Kolawole, and Oyedeji Citation2014). (b) Structure of piperine and other naturally occurring phytochemicals in P. guineense.
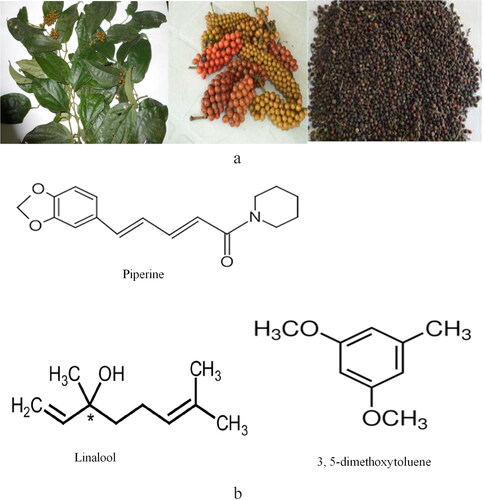
Table 1. Phytochemical compounds and extracted piperine content of Piper spp.
Piper guineense role in managing Alzheimer’s disease
Research has proven that the methanolic extract of Piper suggestively improves spatial working memory. It attenuates the effect of oxidative stress caused by amyloid-β-induced neurotoxicity by inhibiting lipid peroxidation, protein, and DNA oxidation in the brain. It also modulates the activity of antioxidant enzymes superoxide dismutase and catalase in the rats’ hippocampus (Hritcu et al., Citation2014). The essential oil of P. guineense inhibited acetylcholine esterase (AChE) activity, thereby improving cholinergic functions in the scopolamine-induced rat model of Alzheimer’s disease. It inhibits lipid peroxidation and protein oxidation, thus preserving membrane integrity. It also reverses the effect of oxidative stress brought on by the administration of scopolamine by increasing the levels of endogenous antioxidant enzymes (superoxide dismutase, glutathione peroxidase, and catalase) in the brain, which is usually depleted in AD patients (Rajashri et al. Citation2020).
The antineurodegenerative activity of Piper guineense and most Piper spp. has mostly been linked to the alkaloid piperine. Piperine exhibited high antioxidant properties by reducing oxidative stress in the experimentally induced AD model (Mirmosayyeb et al. Citation2017). Its antioxidant and anticholinergic effect reversed neuroinflammation and restored abnormal neurotransmission sporadic Alzheimer’s disease mice (Wang et al. Citation2019). The therapeutic use of piperine restores monoamine neurotransmission, thus alleviate cognitive impairment and slow the progression of Alzheimer’s disease (Wang et al. Citation2019). Piperine can attenuate the increase in lipid peroxidation and AChE activity (Mirmosayyeb et al. Citation2017) and has been shown to reduce brain levels of AChE, which is connected to improvements in memory functions (Aluko Citation2021). It also exerts an anti-inflammatory effect in attenuating cerebral ischemic damage by modulating nuclear factor-kappa β (NF-κβ) (Vaibhav et al. Citation2012). Piperine enhanced cognitive function by inhibiting cytotoxicity and the activity of AChE in the hippocampus of the AF64A-induced AD mouse model. Piperine increased neuron density in the hippocampus due to its neurotrophic activity, thus improving spatial memory and cognitive function (Chonpathompikunlert et al., Citation2010).
Piperine can be effective either singly or in combination with other vallinoids or orthodox drugs. The combinative use of piperine with curcumin could inhibit AChE and prevent amyloid-β aggregation more effectively than when used singly (Abdul Manap et al., Citation2019). Piperine, either used singly or combined with donepezil (choline esterase inhibitor), attenuates behavioral impairment, severe oxidative stress, and hippocampal neurotransmission deficit caused by intracerebroventricular (ICV) infusion of streptozocin (STZ) in the rat model of Alzheimer’s disease (Nazifi et al. Citation2021). It also reduced the levels of malondialdehyde and nitrites while increasing ferric activity, reducing antioxidant power in the hippocampal tissues. The administration of piperine was found to be more effective on the efficacy of synaptic plasticity than the medication donepezil (Nazifi et al. Citation2021).
Piper guineense role in managing depression and anxiety
Depression is one debilitating neuropsychiatric condition with increased mortality and morbidity that also affects the aged. Some clinical interventions for this condition are anti-depressants such as imipramine, citalopram most of which come with numerous side effects. However, several reports have shown that P. guineense and its alkaloid piperine is a potential anti-depressant. The study of Tankam and Ito (Citation2013) demonstrated that inhalation of the essential oil of P. guineense produced an anti-aggressive and anti-depressant effect in mice due to the presence of linalool and 3,5-dimethoxytoluene in the oil. This suggests that the oil exerts its anti-depressant effect via post-synaptic inhibition of γ-aminobutyric acid (GABA) through allosteric modification GABAA receptors (Tankam and Ito Citation2013). Linalool is the essential oil of P. guineense that produces an anti-depressant effect similar to the anti-depressant drug fluoxetine (dos Santos et al. Citation2018). It interferes with anhedonia behavior in rats by significantly reducing the time taken to begin self-cleansing after the splash test, as reluctance in care of oneself or lack of enjoyment in pleasurable activities is one of the symptoms of depression. The anti-depressant effect of linalool from P. guineense occurs via its interaction with the serotonergic route through post-synaptic serotonin 1A (5-HT1A) receptors and the adrenergic system through α2 receptors (dos Santos et al. Citation2018).
Piperine from the methanolic extract of Piper spp. has been reported to have an inhibitory effect on monoamine oxidase activity and increase the levels of monoamine transporter, a combination of these effects produce anti-depressant activity in a mouse model of behavioral despair (Hritcu et al. Citation2015). Likewise, piperine can act as an anti-depressant via up-regulation of hippocampal progenitor cell proliferation and mediation of brain-derived neurotrophic factor (BDNF) signaling in mice exposed to chronic stress (Hritcu et al. Citation2015; Mao et al. Citation2014a). Piperine inhibits inducible nitric oxide synthase (iNOS) mRNA expression in immobilized stress-induced depressed mice. This is because clinical examinations have shown that depressed patients usually show elevated plasma nitrate levels with increased expression of nitric oxide synthase in their hippocampus (Harshita et al. Citation2015). Khom et al. (Citation2013) report demonstrated piperine anti-depressant effect in rodents by modulating ϒ-aminobutyric acid (GABA) type A receptors.
Furthermore, piperine possesses antioxidant activity to restore the specific activity of antioxidant enzymes such as superoxide dismutase, catalase, and glutathione peroxidase in the amygdala homogenates of Aβ (1-42) treated rats (Hritcu et al. Citation2015). This antioxidant effect is vital as the report has shown that increased oxidative stress in the hypothalamus and amygdala can alter fear-related circuits in the brain, affecting behavioral regulation and anxiety development (Hritcu et al. Citation2015). Piperine regulates the serotonergic system and inhibits oxidative stress and hypothalamic-pituitary-adrenal (HPA)-axis hyperactivity (Mao et al. Citation2014b). The report also suggests that the administration of piperine reversed the effect of corticosterone-induced depression by increasing the expression of BDNF protein and mRNA levels in the hippocampus of mice. It also protects against neurotoxicity induced by corticosterone in rat pheochromocytoma (PC12) cells via inhibition of oxidative stress and upregulation of BDNF mRNA levels (Mao et al. Citation2014b).
Piperine acts in combination with other compounds, especially polyphenols, as an anti-depressant with improved efficacy. The study of Huang et al. (Citation2013) revealed that piperine used in combination with trans-resveratrol (a stilbene found in wine or grapes) produced a far more favorable anti-depressant effect than when either compound is used singly. The synergism of these two compounds brought about an increase in monoamine neurotransmitters, especially 5-hydroxytryptamine (5-HT) and norepinephrine, via the inhibition of monoamine oxidase-A activity. Piperine enhances the bioavailability of trans-resveratrol and aids its absorption while exerting its anti-depressant effect (Huang et al. Citation2013). Likewise, piperine administered in combination with ferulic acid was more effective as an anti-depressant since the two compounds work synergistically by increasing monoamine neurotransmitters 5-hydroxytryptamine, norepinephrine, and dopamine in the brain regions relevant to emotional disorders (hypothalamus, hippocampus, and frontal cortex) (Li et al. Citation2015). Piperine acts as a bio-enhancer of ferulic acid and decreases its metabolism by inhibiting hepatic and intestinal glucuronidation. The combination of piperine and ferulic acid exerts an anti-depressant effect via potentiation of the serotonergic and noradrenergic systems. This combination also modulates the dopaminergic function as it influences the synthesis or metabolism of dopamine. It also inhibits the activity of monoamine oxidase-A in the hypothalamus (Li et al. Citation2015).
Piper guineense role in managing Parkinson’s disease
Parkinson’s disease (PD) is another neurodegenerative disease clinically characterized by tremor at rest, rigidity, bradykinesia, postural instability, which sometimes occur due to autonomic factors (Correia et al. Citation2015). Neuroinflammation and oxidative stress are some of the underlying causes of damage to dopaminergic neurons in the brain (Kouti et al. Citation2013), with an increase in pro-inflammatory cytokines tumor necrosis factor-alpha (TNF-α), interleukin 1β (IL-1β), and interleukin 6 (IL-6) occurring in the substantia nigra and putamen during the progression of Parkinson’s disease (Correia et al. Citation2015). Piperine, however, attenuates degeneration of dopaminergic neurons in substantia nigra pars compacta and depletion of dopamine and its metabolite in nigrostriatal progression (Correia et al. Citation2015). It reverses almost entirely apomorphine-induced rotational behavior in 6-hydroxydopamine (6-OHDA) lesion-treated rats while decreasing locomotor activity (Correia et al. Citation2015).
Piperine protects the striatum from lipid peroxidation by increasing the levels of glutathione. It also exerts an anti-inflammatory effect by inhibiting the release of cytochrome C from the mitochondria and caspase 3 and 9. And inhibits the synthesis of inflammatory markers tumor necrosis factor-alpha (TNF-α) and interleukin 1β (IL-1β) in the 6-OHDA rat model of Parkinson’s disease (Correia et al. Citation2015). The study of Shrivastava et al. (Citation2013) revealed that piperine inhibits apoptosis and inflammation in 6-OHDA induced rat model of Parkinson’s disease. It acts by reducing the production of thiobarbituric acid reactive substances (TBARS), inhibits the mediator of apoptosis poly (ADP-ribose) polymerase (PARP), cytochrome C, caspase-3, and caspase-9, and increase glutathione levels (Shrivastava et al. Citation2013). Furthermore, piperine exerts a neuroprotective effect in 1 methyl-4-phenyl-1,2,3,6-tetrahydropyridine (MPTP) induced rat model of Parkinson’s disease via its antioxidant, anti-inflammatory, and anti-apoptotic activities, by alleviating fall latency (Yang et al. Citation2015). It also protects against oxidative damage to neuronal lipids, proteins, and nucleic acids by increasing the antioxidant enzymes superoxide dismutase and reducing lipid peroxidation (Yang et al. Citation2015).
Piperine replenishes the enzyme tyrosine hydroxylase involved in the dopamine synthesis in the substantia nigra and striatum, which was depleted in rotenone-induced Parkinson’s disease rats, thereby mitigating against the loss of dopaminergic neurons and decrease in dopamine (Liu et al. Citation2016). It also helps activate autophagy by inhibiting the AKT-mTORC1 signaling pathway, therefore protecting SK-N-SH (neuroblastoma) cells and primary neurons against mitochondrial damage as impairment in autophagy has been impaired reported in patients with Parkinson disease (Liu et al. Citation2016). In another report, the combinative use of piperine with quercetin alleviates behavioral deficits in the rotenone-iron induced rat model of Parkinson’s disease. This combination restores the levels of neurotransmitters (norepinephrine, dopamine, and serotonin (5-HT)), reduces oxidative damage (malondialdehyde and nitrite levels) while increasing the level of glutathione (Sharma, Raj, and Singh Citation2020).
West African black pepper (Piper guineense) and its chemical compounds perform a huge role in brain health, including those summarized in . Piperine promotes the absorption of therapeutically essential nutrients, and drugs (Haq et al. Citation2021) in the prevention and management of brain-aging diseases amount geriatrics.
Table 2. Neuroprotective potential of some components of Piper guineense.
Conclusion and future recommendation
The effect of brain aging in the geriatric population cannot be over-emphasized with the increasing population of geriatrics due to better health care facilities and social services, which have increased life expectancy worldwide. Several clinical drugs have improved mental health among this population but have shown undesirable side effects. In exploring natural alternatives to improve the quality of life of older people, P. guineense is a very excellent plant material for this purpose. Piperine, phenolics, and essential oil of P. guineense are neuroprotective agents, which the geriatric system may better tolerate in different forms. However, there is a need for further research to illuminate the extent to which consuming piper spp. can prevent or manage brain-aging disease among this populace.
Acknowledgements
Postdoctoral Research Fellowship granted to Dr. B.M. Fasogbon from the University of Johannesburg (UJ) Global Excellence and Stature (GES) Grant is acknowledged.
Disclosure statement
No potential competing interest.
Additional information
Funding
References
- Abdul Manap, A. S. A., A. C. W. Tan, W. H. Leong, A. Y. Y. Chia, S. Vijayabalan, A. Arya, E. H. Wong, F. Rizwan, U. Bindal, S. Koshy, et al. 2019. Synergistic effects of curcumin and piperine as potent acetylcholine and amyloidogenic inhibitors with significant neuroprotective activity in sh-sy5y cells via computational molecular modeling and in vitro assay. Frontiers in Aging Neuroscience 11 (206):1–17. doi: 10.3389/fnagi.2019.00206.
- Adewusi, E. A., and V. Steenkamp. 2011. In vitro screening for acetylcholinesterase inhibition and antioxidant activity of medicinal plants from southern Africa. Asian Pacific Journal of Tropical Medicine 4 (10):829–35. doi: 10.1016/S1995-7645(11)60203-4.
- Alexopoulos, G. S. 2019. Mechanisms and treatment of late-life depression. Translational Psychiatry 9 (1):188. doi: 10.1038/s41398-019-0514-6.
- Aluko, R. E. 2021. Food-derived acetylcholinesterase inhibitors as potential agents against Alzheimer’s Disease. EFood. doi: 10.2991/efood.k.210318.001.
- Alzheimer’s Association. 2020. What is Alzheimer’s disease? Symptoms and causes | alz.org. Alzheimer’s Association. https://www.alz.org/alzheimers-dementia/what-is-alzheimers
- Amadioha, A. C., and K. P. Chidi. 2019. Phytochemical Composition of aqueous and ethanolic leaf extracts of Piper guineense, Cassia alata, Tagetes erecta and Ocimum graticimum. Journal of Pharmaceutical Research International 26 (3):1–8. doi: 10.9734/jpri/2019/v26i330136.
- Anyanwu, C. U., and G. C. Nwosu. 2014. Assessment of the antimicrobial activity of aqueous and ethanolic extracts of Piper guineense leaves. Journal of Medicinal Plants Research 8 (10):436–40. doi: 10.5897/JMPR12.976.
- Ash, P. E., T. E. Vanderweyde, K. L. Youmans, D. J. Apicco, and B. Wolozin. 2014. Pathological stress granules in Alzheimer’s disease. Brain Research 1584:52–8. doi: 10.1016/j.brainres.2014.05.052.
- Benazzouz, A., O. Mamad, P. Abedi, R. Bouali-Benazzouz, and J. Chetrit. 2014. Involvement of dopamine loss in extrastriatal basal ganglia nuclei in the pathophysiology of Parkinson’s disease. Frontiers in Aging Neuroscience 6:87. doi: 10.3389/fnagi.2014.00087.
- Buigues, C., C. Padilla-Sánchez, J. Fernández Garrido, R. Navarro-Martínez, V. Ruiz-Ros, and O. Cauli. 2015. The relationship between depression and frailty syndrome: A systematic review. Aging and Mental Health 19 (9):762–72. doi: 10.1080/13607863.2014.967174.
- Butt, M. S., I. Pasha, M. T. Sultan, M. A. Randhawa, F. Saeed, and W. Ahmed. 2013. Black pepper and health claims: A comprehensive treatise. Critical Reviews in Food Science and Nutrition 53 (9):875–86. doi: 10.1080/10408398.2011.571799.
- Butterfield, D. A., and D. Boyd-Kimball. 2018. Oxidative stress, amyloid-β peptide, and altered key molecular pathways in the pathogenesis and progression of Alzheimer’s disease. Journal of Alzheimer’s Disease: JAD 62 (3):1345–67. doi: 10.3233/JAD-170543.
- Cahyono, B., Hasanah, E. F. Judiono, Suzery, and M. Widayat. 2019. Analysis of piperine content in cabe jawa extracts (Piper retrofractum Vahl) using UV spectrophotometry and HPLC. IOP Conference Series: Materials Science and Engineering 509 (012025):1–5. doi: 10.1088/1757-899X/509/1/012025.
- Cai, Z., B. Zhao, and A. Ratka. 2011. Oxidative stress and β-amyloid protein in Alzheimer’s disease. Neuromolecular Medicine 13 (4):223–50. doi: 10.1007/s12017-011-8155-9.
- Chang, K. H., and C. M. Chen. 2020. The role of oxidative stress in Parkinson’s disease. In Antioxidants 9 (7). doi: 10.3390/antiox9070597.
- Cheignon, C., M. Tomas, D. Bonnefont-Rousselot, P. Faller, C. Hureau, and F. Collin. 2018. Oxidative stress and the amyloid beta peptide in Alzheimer’s disease. Redox Biology 14:450–64. doi: 10.1016/j.redox.2017.10.014.
- Chen, X., and W. Pan. 2015. The treatment strategies for neurodegenerative diseases by integrative medicine. Integrative Medicine International 1 (4):223–5. doi: 10.1159/000381546.
- Chinta, S. J., G. Woods, A. Rane, M. Demaria, J. Campisi, and J. K. Andersen. 2015. Cellular senescence and the aging brain. Experimental Gerontology 68:3–7. doi: 10.1016/j.exger.2014.09.018.
- Choi, S.-H., R. Lee, S. M. Nam, D.-G. Kim, I.-H. Cho, H.-C. Kim, Y. Cho, H. Rhim, and S.-Y. Nah. 2021. Ginseng gintonin, aging societies, and geriatric brain diseases. Integrative Medicine Research 10 (1):100450. doi: 10.1016/j.imr.2020.100450.
- Chonpathompikunlert, P., J. Wattanathorn, and S. Muchimapura. 2010. Piperine, the main alkaloid of Thai black pepper, protects against neurodegeneration and cognitive impairment in animal model of cognitive deficit like condition of Alzheimer’s disease. Food and Chemical Toxicology: An International Journal Published for the British Industrial Biological Research Association 48 (3):798–302. doi: 10.1016/j.fct.2009.12.009.
- Correia, A., A. Cruz, A. Aquino, J. Diniz, K. Santana, P. Cidade, J. Peixoto, D. Lucetti, M. Nobre, G. Cruz, et al. 2015. Neuroprotective effects of piperine, an alkaloid from the Piper genus, on the Parkinson’s disease model in rats. Journal of Neurology and Therapeutics 1 (1):1–8. doi: 10.14312/2397-1304.2015-1.
- Dams-O’Connor, K., G. Guetta, A. E. Hahn-Ketter, and A. Fedor. 2016. Traumatic brain injury as a risk factor for Alzheimer’s disease: Current knowledge and future directions. NNeurodegenerative disease management 6 (5):417–29. doi: 10.2217/nmt-2016-0017.
- Dias, V., E. Junn, and M. M. Mouradian. 2013. The role of oxidative stress in Parkinson’s disease. Journal of Parkinson’s Disease 3 (4):461–91. doi: 10.3233/JPD-130230.
- Doria, M., L. Maugest, T. Moreau, G. Lizard, and A. Vejux. 2016. Contribution of cholesterol and oxysterols to the pathophysiology of Parkinson’s disease. Free Radical Biology & Medicine 101:393–400. doi: 10.1016/j.freeradbiomed.2016.10.008.
- dos Santos, É. R. Q., C. S. F. Maia, E. A. Fontes Junior, A. S. Melo, B. G. Pinheiro, and J. G. S. Maia. 2018. Linalool-rich essential oils from the Amazon display antidepressant-type effect in rodents. Journal of Ethnopharmacology 212:43–9. doi: 10.1016/j.jep.2017.10.013.
- Esopenko, C., and B. Levine. 2015. Aging, neurodegenerative disease, and traumatic brain injury: The role of neuroimaging. Journal of Neurotrauma 32 (4):209–20. doi: 10.1089/neu.2014.3506.
- Ezenobi, U. V., F. J. Amaku, and C. Agbidi. 2016. Phytochemical, proximate, minerals and vitamin composition of Monodora myristica Piper Guineese seeds. Research Journal of Science and Technology 8 (4):209. doi: 10.5958/2349-2988.2016.00031.0.
- Farooqui, T., and A. A. Farooqui. 2011. Lipid-mediated oxidative stress and inflammation in the pathogenesis of Parkinson’s disease. Parkinson’s Disease 2011:247467. doi: 10.4061/2011/247467.
- Fenga, C., S. Gangemi, A. Alibrandi, C. Costa, and E. Micali. 2016. Relationship between lead exposure and mild cognitive impairment. Journal of Preventive Medicine and Hygiene 57 (4):E205–E210. doi: 10.15167/2421-4248/jpmh2016.57.4.514.
- Grossman, E. 2014. Time after time: Environmental influences on the aging brain. Environmental Health Perspectives 122 (9):A238–A243. doi: 10.1289/ehp.122-A238.
- Haq, I. U., M. Imran, M. Nadeem, T. Tufail, T. A. Gondal, and M. S. Mubarak. 2021. Piperine: A review of its biological effects. Phytotherapy Research: PTR 35 (2):680–700. doi: 10.1002/ptr.6855.
- Harshita, J., J. Prateek, A. Bharti, and A. Dheeraj. 2015. Ameliorating effect of piperine on NO-cGMP pathway in stress induced depression. Science, Technology and Arts Research Journal 4 (1):109. doi: 10.4314/star.v4i1.18.
- Hogan, D. B., P. Bailey, S. Black, A. Carswell, H. Chertkow, B. Clarke, C. Cohen, J. D. Fisk, D. Forbes, M. Man-Son-Hing, et al. 2008. Diagnosis and treatment of dementia: 5. Nonpharmacologic and pharmacologic therapy for mild to moderate dementia. CMAJ 179 (10):1019–1026. doi: 10.1503/cmaj.081103.
- Hritcu, L., J. A. Noumedem, O. Cioanca, M. Hancianu, V. Kuete, and M. Mihasan. 2014. Methanolic extract of Piper nigrum fruits improves memory impairment by decreasing brain oxidative stress in amyloid beta(1-42) rat model of Alzheimer’s disease . Cellular and Molecular Neurobiology 34 (3):437–49. doi: 10.1007/s10571-014-0028-y.
- Hritcu, L., J. A. Noumedem, O. Cioanca, M. Hancianu, P. Postu, and M. Mihasan. 2015. Anxiolytic and antidepressant profile of the methanolic extract of Piper nigrum fruits in beta-amyloid (1-42) rat model of Alzheimer’s disease . Behavioral and Brain Functions: BBF 11 (1):13. doi: 10.1186/s12993-015-0059-7.
- Hu, Y., H. B. Liao, P. Liu, D. H. Guo, and Y. Y. Wang. 2009. [Antidepressant effects of piperine and its neuroprotective mechanism in rats] . Zhong xi yi Jie he Xue Bao = Journal of Chinese Integrative Medicine 7 (7):667–70. doi: 10.3736/jcim20090712.
- Huang, W., Z. Chen, Q. Wang, M. Lin, S. Wu, Q. Yan, F. Wu, X. Yu, X. Xie, G. Li, et al. 2013. Piperine potentiates the antidepressant-like effect of trans-resveratrol: Involvement of monoaminergic system. Metabolic Brain Disease 28 (4):585–95. doi: 10.1007/s11011-013-9426-y.
- Imo, C., O. E. Yakubu, N. G. Imo, I. S. Udegbun, S. V. Tatah, and O. J. Onukwug. 2018. Proximate, mineral and phytochemical composition of Piper guineense seeds and leaves. Journal of Biological Sciences 18 (7):329–37. doi: 10.3923/jbs.2018.329.337.
- Isikhuemen, E. M., B. O. Ogbomwan, and I. U. Efenudu. 2020. Evaluation of phytochemical and mineral constituents of Piper guineense Schum. and Thonn. and Piper umbellatum Linn: Implications for ethnomedicine. European Journal of Medicinal Plants 31 (1):84–97. doi: 10.9734/ejmp/2020/v31i130209.
- Khom, S., B. Strommer, A. Schöffmann, J. Hintersteiner, I. Baburin, T. Erker, T. Schwarz, C. Schwarzer, J. Zaugg, M. Hamburger, et al. 2013. GABAA receptor modulation by piperine and a non-TRPV1 activating derivative. Biochemical Pharmacology 85 (12):1827–36. doi: 10.1016/j.bcp.2013.04.017.
- Kouli, A., K. M. Torsney, and W.-L. Kuan. 2018. Parkinson’s disease: Etiology, neuropathology, and pathogenesis. Parkinson’s Disease: Pathogenesis and Clinical Aspects. Brisbane (AU): Codon Publications. doi: 10.15586/codonpublications.parkinsonsdisease.2018.ch1.
- Kouti, L., M. Noroozian, S. Akhondzadeh, M. Abdollahi, M. R. Javadi, M. A. Faramarzi, S. Mousavi, and P. Ghaeli. 2013. Nitric oxide and peroxynitrite serum levels in Parkinson’s disease: Correlastion of oxidative stress and the severity of the disease. European Review for Medical and Pharmacological Sciences 17 (7):964–70.
- Kumar Thakur, A., P. Kamboj, K. Goswami, and K. Ahuja. 2018. Pathophysiology and management of Alzheimer’s disease: An overview. Journal of Analytical & Pharmaceutical Research 7 (2):226–35. doi: 10.15406/japlr.2018.07.00230.
- Kwak, Y. T., Y. Yang, and M.-S. Koo. 2017. Anxiety in dementia. Dementia and Neurocognitive Disorders 16 (2):33–9. doi: 10.12779/dnd.2017.16.2.33.
- Laks, J., and E. Engelhardt. 2010. Peculiarities of geriatric psychiatry: A focus on aging and depression. CNS Neuroscience and Therapeutics 16 (6):374–79. doi: 10.1111/j.1755-5949.2010.00196.x.
- Lee, J. G., Y. Chae, Y. Shin, and Y. J. Kim. 2020. Chemical composition and antioxidant capacity of black pepper pericarp. Applied Biological Chemistry 63 (1):1–9. doi: 10.1186/s13765-020-00521-1.
- Lee, J. J. 2019. Pharmacological treatment in Parkinson’s disease. Journal of the Korean Neurological Association 37 (4):335–44. doi: 10.17340/jkna.2019.4.1.
- Li, G., L. Ruan, R. Chen, R. Wang, X. Xie, M. Zhang, L. Chen, Q. Yan, M. Reed, J. Chen, et al. 2015. Synergistic antidepressant-like effect of ferulic acid in combination with piperine: Involvement of monoaminergic system. Metabolic Brain Disease 30 (6):1505–14. doi: 10.1007/s11011-015-9704-y.
- Li, S., C. Wang, M. Wang, W. Li, K. Matsumoto, and Y. Tang. 2007. Antidepressant like effects of piperine in chronic mild stress treated mice and its possible mechanisms . Life Sciences 80 (15):1373–81. doi: 10.1016/j.lfs.2006.12.027.
- Liu, J., M. Chen, X. Wang, Y. Wang, C. Duan, G. Gao, L. Lu, X. Wu, X. Wang, and H. Yang. 2016. Piperine induces autophagy by enhancing protein phosphotase 2A activity in a rotenone-induced Parkinson’s disease model. Oncotarget 7 (38):60823–43. doi: 10.18632/oncotarget.11661.
- Luca, S. V., M. Minceva, J. Gertsch, and K. Skalicka-Woźniak. 2021. LC-HRMS/MS-based phytochemical profiling of Piper spices: Global association of piperamides with endocannabinoid system modulation. Food Research International (Ottawa, Ont.) 141:110123. doi: 10.1016/j.foodres.2021.110123.
- Magi, S., P. Castaldo, M. L. MacRi, M. Maiolino, A. Matteucci, G. Bastioli, S. Gratteri, S. Amoroso, and V. Lariccia. 2016. Intracellular calcium dysregulation: Implications for Alzheimer’s disease. BioMed Research International 2016:6701324. doi: 10.1155/2016/6701324.
- Mao, Q. Q., Z. Huang, X. M. Zhong, Y. F. Xian, and S. P. Ip. 2014a. Brain-derived neurotrophic factor signalling mediates the antidepressant-like effect of piperine in chronically stressed mice. Behavioural Brain Research 261:140–5. doi: 10.1016/j.bbr.2013.12.020.
- Mao, Q. Q., Z. Huang, X. M. Zhong, Y. F. Xian, and S. P. Ip. 2014b. Piperine reverses the effects of corticosterone on behavior and hippocampal BDNF expression in mice. Neurochemistry International 74:36–41. doi: 10.1016/j.neuint.2014.04.017.
- Martorell, P., E. Bataller, S. Llopis, N. Gonzalez, B. Álvarez, F. Montón, P. Ortiz, D. Ramón, and S. Genovés. 2013. A cocoa peptide protects Caenorhabditis elegans from oxidative stress and β-amyloid peptide toxicity. PLoS ONE 8 (5):e63283. doi: 10.1371/journal.pone.0063283.
- Mattson, M. P., and T. V. Arumugam. 2018. Hallmarks of brain aging: Adaptive and pathological modification by metabolic states. Cell Metabolism 27 (6):1176–99. doi: 10.1016/j.cmet.2018.05.011.
- Mirmosayyeb, O., A. Tanhaei, H. R. Sohrabi, R. N. Martins, M. Tanhaei, M. A. Najafi, A. Safaei, and R. Meamar. 2017. Possible role of common spices as a preventive and therapeutic agent for Alzheimer’s disease. International Journal of Preventive Medicine 8:5. doi: 10.4103/2008-7802.199640.
- Montiel, T., R. Quiroz-Baez, L. Massieu, and C. Arias. 2006. Role of oxidative stress on beta-amyloid neurotoxicity elicited during impairment of energy metabolism in the hippocampus: Protection by antioxidants . Experimental Neurology 200 (2):496–508. doi: 10.1016/j.expneurol.2006.02.126.
- Nazifi, M., S. Oryan, D. E. Esfahani, and M. Ashrafpoor. 2021. The functional effects of piperine and piperine plus donepezil on hippocampal synaptic plasticity impairment in rat model of Alzheimer’s disease. Life Sciences 265:118802. doi: 10.1016/j.lfs.2020.118802.
- Nikhra, V. 2017. The aging brain: Recent research and concepts. Gerontology & Geriatrics Studies 1 (3):1–11. doi: 10.31031/GGS.2017.01.000511.
- Ogbunugafor, H. A., C. G. Ugochukwu, and A. E. Kyrian-Ogbonna. 2017. The role of spices in nutrition and health: A review of three popular spices used in Southern Nigeria. Food Quality and Safety 1 (3):171–85. doi: 10.1093/fqsafe/fyx020.
- Oyemitan, I. A., F. Kolawole, and A. O. Oyedeji. 2014. Acute toxicity, antinociceptive and anti-inflammatory activity of the essential oil of fresh fruits of Piper guineense Schum Thonn (Piperaceae) in rodents. Journal of Medicinal Plants Research 8 (40):1191–7. doi: 10.5897/JMPR2014.5639.
- Oyemitan, I. A., O. A. Olayera, A. Alabi, L. A. Abass, C. A. Elusiyan, A. O. Oyedeji, and M. A. Akanmu. 2015. Psychoneuropharmacological activities and chemical composition of essential oil of fresh fruits of Piper guineense (Piperaceae) in mice. Journal of Ethnopharmacology 166:240–9. doi: 10.1016/j.jep.2015.03.004.
- Penninx, B. W. J. H. 2017. Depression and cardiovascular disease: Epidemiological evidence on their linking mechanisms. Neuroscience and Biobehavioral Reviews (74 part B):277–86. doi: 10.1016/j.neubiorev.2016.07.003.
- Peters, R. 2006. Ageing and the brain. Postgraduate Medical Journal 82 (964):84–8. doi: 10.1136/pgmj.2005.036665.
- Postuma, R. B., D. Aarsland, P. Barone, D. J. Burn, C. H. Hawkes, W. Oertel, and T. Ziemssen. 2012. Identifying prodromal Parkinson’s disease: Pre-motor disorders in Parkinson’s disease . Movement Disorders: Official Journal of the Movement Disorder Society 27 (5):617–26. doi: 10.1002/mds.24996.
- Rahman Khan, Z., F. Moni, S. Sharmin, M. A. Al-Mansur, A. Gafur, O. Rahman, and F. Afroz. 2017. Isolation of bulk amount of piperine as active pharmaceutical ingredient (API) from black pepper and white pepper (Piper nigrumand L.). Pharmacology & Pharmacy 08 (07):253–62. doi: 10.4236/pp.2017.87018.
- Rajashri, K., S. Mudhol, M. Serva Peddha, and B. B. Borse. 2020. Neuroprotective effect of spice oleoresins on memory and cognitive impairment associated with scopolamine-induced Alzheimer’s disease in rats. ACS Omega. 5 (48):30898–905. doi: 10.1021/acsomega.0c03689.
- Raman, G., and V. G. Gaikar. 2002. Extraction of Piperine from Piper nigrum (Black Pepper) by Hydrotropic Solubilization. Industrial & Engineering Chemistry Research. 41 (12):2966–76. doi: 10.1021/ie0107845.
- Rakofsky, J. J., K. J. Ressler, and B. W. Dunlop. 2012. BDNF function as a potential mediator of bipolar disorder and post-traumatic stress disorder comorbidity. Molecular Psychiatry 17 (1):22–35. doi: 10.1038/mp.2011.121.
- Rudy, C. C., H. C. Hunsberger, D. S. Weitzner, and M. N. Reed. 2015. The role of the tripartite glutamatergic synapse in the pathophysiology of Alzheimer’s disease. Aging and Disease 6 (2):131–48. doi: 10.14336/AD.2014.0423.
- Safaiyan, S., N. Kannaiyan, N. Snaidero, S. Brioschi, K. Biber, S. Yona, A. L. Edinger, S. Jung, M. J. Rossner, and M. Simons. 2016. Age-related myelin degradation burdens the clearance function of microglia during aging. Nature Neuroscience 19 (8):995–8. doi: 10.1038/nn.4325.
- Salehi, B., Z. A. Zakaria, R. Gyawali, S. A. Ibrahim, J. Rajkovic, Z. K. Shinwari, T. Khan, J. Sharifi-Rad, A. Ozleyen, E. Turkdonmez, et al. 2019. Piper species: A comprehensive review on their phytochemistry, biological activities and applications. Molecules 24 (7):1364. doi: 10.3390/molecules24071364.
- Sharma, S.,. K. Raj, and S. Singh. 2020. Neuroprotective effect of quercetin in combination with piperine against rotenone- and iron supplement-induced Parkinson’s disease in experimental rats. Neurotoxicity Research 37 (1):198–209. doi: 10.1007/s12640-019-00120-z.
- Shrivastava, P., K. Vaibhav, R. Tabassum, A. Khan, T. Ishrat, M. M. Khan, A. Ahmad, F. Islam, M. M. Safhi, and F. Islam. 2013. Anti-apoptotic and anti-inflammatory effect of piperine on 6-OHDA induced Parkinson’s rat model. Journal of Nutritional Biochemistry 24 (4):680–87. doi: 10.1016/j.jnutbio.2012.03.018.
- Sibille, E. 2013. Molecular aging of the brain, neuroplasticity, and vulnerability to depression and other brain-related disorders. Dialogues in Clinical Neuroscience 15 (1). doi: 10.31887/dcns.2013.15.1/esibille.
- Sulaimon, L. A., E. O. Anise, E. M. Obuotor, T. A. Samuel, A. I. Moshood, M. Olajide, and T. Fatoke. 2020. In vitro antidiabetic potentials, antioxidant activities and phytochemical profile of african black pepper (Piper guineense). Clinical Phytoscience 6 (1):1–13. doi: 10.1186/s40816-020-00236-2.
- Tankam, J. M., and M. Ito. 2013. Inhalation of the essential oil of Piper guineense from Cameroon shows sedative and anxiolytic-like effects in mice. Biological & Pharmaceutical Bulletin 36 (10):1608–14. doi: 10.1248/bpb.b13-00491.
- Taylor, W. D., H. J. Aizenstein, and G. S. Alexopoulos. 2013. The vascular depression hypothesis: Mechanisms linking vascular disease with depression. Molecular Psychiatry 18 (9):963–74. doi: 10.1038/mp.2013.20.
- Tifratene, K., V. Manera, R. Fabre, A. Gros, S. Thummler, C. Pradier, P. Robert, and R. David. 2017. Antipsychotic prescribing for Alzheimer’s disease and related disorders in specialized settings from 2010 to 2014 in France: A repeated cross-sectional study. Alzheimer’s Research and Therapy 9 (1):1–10. doi: 10.1186/s13195-017-0256-8.
- Tobore, T. O. 2019. On the etiopathogenesis and pathophysiology of Alzheimer’s disease: A Comprehensive theoretical review. Journal of Alzheimer’s Disease: JAD 68 (2):417–37. doi: 10.3233/JAD-181052.
- Tu, Y., Y. Zhong, H. Du, W. Luo, Y. Wen, Q. Li, C. Zhu, and Y. Li. 2016. Anticholinesterases and antioxidant alkamides from Piper nigrum fruits. Natural Product Research 30 (17):1945–9. doi: 10.1080/14786419.2015.1089243.
- Vaibhav, K., P. Shrivastava, H. Javed, A. Khan, M. E. Ahmed, R. Tabassum, M. M. Khan, G. Khuwaja, F. Islam, M. Saeed Siddiqui, et al. 2012. Piperine suppresses cerebral ischemia-reperfusion-induced inflammation through the repression of COX-2, NOS-2, and NF-κB in middle cerebral artery occlusion rat model. Molecular and Cellular Biochemistry 367 (1-2):73–84. doi: 10.1007/s11010-012-1321-z.
- Wahl, D., S. M. Solon-Biet, V. C. Cogger, L. Fontana, S. J. Simpson, D. G. Le Couteur, and R. V. Ribeiro. 2019. Aging, lifestyle and dementia. Neurobiology of Disease 130:104481. doi: 10.1016/j.nbd.2019.104481.
- Wang, B., and Y. Du. 2013. Cadmium and its neurotoxic effects. Oxidative Medicine and Cellular longevity 2013:898034. doi: 10.1155/2013/898034.
- Wang, C., Z. Cai, W. Wang, M. Wei, D. Kou, T. Li, Z. Yang, H. Guo, W. Le, and S. Li. 2019. Piperine attenuates cognitive impairment in an experimental mouse model of sporadic Alzheimer’s disease. Journal of Nutritional Biochemistry 70:147–155. doi: 10.1016/j.jnutbio.2019.05.009.
- Wang, H. L. S., R. Yu, Y. T. Wu, W. Y. Lee, M. F. Lin, C. Y. Chen, and E. Y. Shen. 2013. The Changes of cerebral morphology related to aging in Taiwanese population. PLoS ONE 8 (1):e55241. doi: 10.1371/journal.pone.0055241.
- World Health Organization. 2018. WHO | Ageing and health. Website: WHO; Media centre.
- Wuwongse, S., R. C. C. Chang, and A. C. K. Law. 2010. The putative neurodegenerative links between depression and Alzheimer’s disease. Progress in Neurobiology 91 (4):362–75. doi: 10.1016/j.pneurobio.2010.04.005.
- Wyss-Coray, T. 2016. Ageing, neurodegeneration and brain rejuvenation. Nature 539 (7628):180–6. doi: 10.1038/nature20411.
- Yang, W., Y. H. Chen, H. Liu, and H. D. Qu. 2015. Neuroprotective effects of piperine on the 1-methyl-4-phenyl-1,2,3,6-tetrahydropyridine-induced Parkinson’s disease mouse model. International Journal of Molecular Medicine 36 (5):1369–76. doi: 10.3892/ijmm.2015.2356.
- Yusuf, M., M. Khan, R. A. Khan, and B. Ahmed. 2013. Preparation, characterization, in vivo and biochemical evaluation of brain targeted Piperine solid lipid nanoparticles in an experimentally induced Alzheimer’s disease model. Journal of Drug Targeting 21 (3):300–11. doi: 10.3109/1061186X.2012.747529.
- Zhao, Y., and B. Zhao. 2013. Oxidative stress and the pathogenesis of Alzheimer’s disease. Oxidative Medicine and Cellular Longevity 2013:316523. doi: 10.1155/2013/316523.
- Ztaou, S., and M. Amalric. 2019. Contribution of cholinergic interneurons to striatal pathophysiology in Parkinson’s disease. Neurochemistry International 126:1–10. doi: 10.1016/j.neuint.2019.02.019. https://give.ucdavis.edu/MNAL/324080.