Abstract
Human milk is a highly complex liquid food tailor-made to match an infant’s needs. Beyond documented positive effects of breastfeeding on infant and maternal health, there is increasing evidence that milk constituents also impact child neurodevelopment. Non-nutrient milk bioactives would contribute to the (long-term) development of child cognition and behavior, a process termed ‘Lactocrine Programming’. In this review we discuss the current state of the field on human milk composition and its links with child cognitive and behavioral development. To promote state-of-the-art methodologies and designs that facilitate data pooling and meta-analytic endeavors, we present detailed recommendations and best practices for future studies. Finally, we determine important scientific gaps that need to be filled to advance the field, and discuss innovative directions for future research. Unveiling the mechanisms underlying the links between human milk and child cognition and behavior will deepen our understanding of the broad functions of this complex liquid food, as well as provide necessary information for designing future interventions.
Human milk and child development
Human milk is a complex liquid food. There is increasing evidence that the constituents in human milk impact child cognition and behavior. Nonetheless, human milk remains one of the most under-studied biological system in life sciences. This manuscript is the fruit of a close collaboration between all the participants of a 4-day hybrid workshop on lactocrine programming held in The Netherlands (November 2020). This collaborative manuscript functions as a call and a source of inspiration for the scientific community to perform research on this extremely relevant, but unfortunately often-ignored topic.
The goal of this narrative review is to summarize and discuss the current state of the field on human milk composition in the light of its association with child behavioral and cognitive development. The sections covered include (i) introduction to human milk and child development, (ii) the different milk constituents and their associations with child cognition and behavior, and (iii) maternal and child factors affecting milk constituents, (iv) recommendations and best practices for researchers for future research studies section presents new studies that can adopt state-of-the-art methodologies and designs that will facilitate future meta-analytic endeavors to help fill knowledge gaps, and (v) finally, the most important scientific gaps that need to be filled in order to advance the field, along with innovative directions for future research, are presented in an open questions and innovative ideas section. Appendix A contains a glossary and Appendix B a list with abbreviations used in the text.
Human milk from a public health and clinical perspective
Breastfeeding, and the various nutrients and bioactive factors in human milk, are known for their undeniable long-term health benefits for both infant and mother (Fields et al. Citation2016; Hinde Citation2013; Victora et al. Citation2016). For infants, human milk protects against numerous severe infections including enterocolitis, diarrhea, and pneumonia, and has been shown to significantly reduce the rates of infant morbidity and mortality (Ip et al., Citation2007; Victora et al. Citation2016). In the long-term, the benefits of human milk include a reduced risk of developing later life metabolic disease, such as childhood obesity (Oddy Citation2012; Victora et al. Citation2016) and diabetes (Arshad, Karim, and Ara Hasan Citation2014; Desoye and Hauguel-de Mouzon Citation2007; Victora et al. Citation2016), For mothers, breastfeeding improves birth spacing, protects against breast cancer and also seems to protect against ovarian cancer and type 2 diabetes (Victora et al. Citation2016; Chowdhury et al. Citation2015). In turn, these positive effects on both mothers and infants lead to relevant societal economic impacts (e.g., Quesada, Méndez, and Martín-Gil Citation2020; Rollins et al. Citation2016; Stuebe et al. Citation2017).
These unique features of breastfeeding and human milk have led the World Health Organization (WHO) to endorse exclusive breastfeeding for the first six months of an infant’s life, followed by breastfeeding supplemented with complementary foods until the age of two years (World Health Organization Citation2003). Also in the most vulnerable infants, i.e., preterm, and low birth weight infants, human milk significantly reduces the chances of severe medical complications (Quigley, Embleton, and McGuire Citation2019; Furman et al., Citation2003; Villamor-Martínez et al. Citation2018; Manzoni et al. Citation2013; Nutrition et al. Citation2013), and appears to predict better health and neurodevelopment later in life (Singhal, Cole, and Lucas Citation2001; Schneider and Garcia-Rodenas Citation2017; Lechner and Vohr Citation2017). For example, a recent study in preterm infants revealed that exclusive human milk feeding in extremely low birth weight infants, while associated with increased extrauterine growth restriction, had a protective effect on neurodevelopmental outcomes at 2 years of age as assessed through the Bayley Scales of Infant Development (Rahman et al. Citation2020). Hence, especially for preterm and low birth weight infants, if a mother’s own milk is not available, donated human milk is currently considered the next best choice (Committee on Nutrition, Section on Breastfeeding, and Committee on Fetus and Newborn 2017; Nutrition et al. Citation2013).
Although the WHO has a global recommendation for exclusive breastfeeding, many countries have not been able to achieve this goal. In practice, breastfeeding is influenced by several maternal, environmental, and societal characteristics that can hinder or facilitate breastfeeding (Standish and Parker Citation2021). For example, in high-income countries, mothers with higher income and education are more likely to breastfeed, while in low-income countries, those with lower socioeconomic status are more likely to breastfeed (Victora et al. Citation2016). Other factors such as negative social perceptions of breastfeeding in public, lack of trained health care support, and early return to work pose challenges for breastfeeding initiation and duration (Mulready-Ward and Hackett Citation2014; Ahluwalia, Morrow, and Hsia Citation2005; Thulier and Mercer Citation2009). These factors are often complex and interact with one another, leading mothers to feed their infants in a variety of ways in the first six months, including direct milk at the breast, pumped milk through a bottle, donor human milk, infant formula, and solids, as well as varying combinations of these feeding types. This review includes data on human milk feeding in any form, acknowledging that there are varying ways this is supplied in practice. Note that the effects of human milk on infant neurological development may be different depending on the mode of feeding (direct or pumped) and dose (amount of human milk vs infant formulas and other foods), but that these distinctions are beyond the scope of the current review.
Human milk and child neurodevelopment
Next to documented positive effects on a range of physical health outcomes in both the infant and the mother, breastfeeding parameters (e.g., initiation and duration) have also been related to improved child neurodevelopment, cognition and behavior (Hou et al. Citation2021; Ikeda et al. Citation2014; Lucas Citation2005; Victora et al. Citation2016). The most active period of child brain and behavioral development occurs in the first 1000 days of life, the period beginning at conception and ending at the start of the third postnatal year (Moore Citation2016). For example, while at birth the brain of a child already has nearly all the neurons it will ever have, it still doubles in size during the first year of life. Synapses are overproduced and groups of neurons form pathways, which are refined through the elimination of cells and connections. This refining of neural pathways heavily depends on the child’s experience and input from the environment. Cells and connections that are activated are retained and strengthened, while those not used are eliminated. This refinement is thought to be one of the primary mechanisms of brain plasticity, allowing the brain to organize itself to adapt to the environment (Prado and Dewey Citation2014). Nutrition would provide the fuel that drives much of this early brain growth, development, and refinement (Prado and Dewey Citation2014).
Meta-analyses have associated breastfeeding initiation and duration with higher performance in intelligence tests in children and adolescents. Pooled estimates of 3 − 5 intelligence quotient (IQ) points favoring children who have been breastfed are reported in reviews of observational studies (Anderson, Johnstone, and Remley Citation1999; Drane and Logemann Citation2000; Jain et al. Citation2002; Victora et al. Citation2016). A large cluster-randomized trial in Belarus also provided evidence that breastfeeding has positive effects on child cognitive development (Kramer et al. Citation2008). Clinics were randomly assigned to a breastfeeding promotion intervention or standard healthcare. Mothers in the breastfeeding promotion group had higher rates of any and exclusive breastfeeding. At subsequent follow-up (N = 13,889; child’s age of 6.5 years), children in the breastfeeding promotion group had higher teacher ratings of reading and writing ability, and higher IQ scores. Promotion of breastfeeding thus not only prevents a range of physical health outcomes in infants and their mothers, but also seems to support children’s cognitive and behavioral development. To what extent associations persist into later life is less clear, but some recent studies also found cognitive benefits in later childhood (e.g., Kim and Choi Citation2020; Lopez et al. Citation2021).
It is important to note that the mixture of feeding methods (i.e., direct milk at the breast, pumped milk through a bottle, donor human milk, infant formula, solids, and combinations of these methods) has complicated the comparison of outcomes between breastfeeding and non-breastfeeding infants in this research area. Also, although studies controlled for multiple confounding variables, the associations found may still be confounded by unknown factors, and studies with experimental designs are scarce. Lastly, not all available studies have found positive associations between breastfeeding parameters and child cognitive performance (e.g., Jacobson et al. Citation1999; Wigg et al. Citation1998). As such, the available scientific evidence does not unequivocally demonstrate that human milk leads to better cognitive development in the offspring.
Human milk from an evolutionary perspective
Although not typically integrated within the public health and clinical framework, the evolutionary perspective is crucial for understanding the adaptive reaction norms that underlie how variance in milk synthesis in turn shapes and organizes infant developmental trajectories (Hinde et al. Citation2015; Fewtrell et al. Citation2020). The evolutionary success of human milk is largely dependent on its ability to alter its composition and volume to match the needs of a growing infant. Life History Theory, one of the existent evolutionary perspectives, organizes our understanding of how natural selection has (likely) shaped tradeoff allocations for maintenance, development, and reproduction across an organism’s life-course (Hill 1993; Hill and Kaplan Citation1999). For example, time and resources allocated toward growth and development early in life delay reproductive debut, but the establishment of key somatic resources can improve reproductive performance (Pittet, Johnson, and Hinde Citation2017).
Milk Bioactives and lactocrine programming
Environmental conditions will determine the resources available for a mother to maintain lactation and her infant. Environmental conditions may also influence the composition of a mother’s milk. As part of the lactation strategy, mothers may also transfer milk non-nutrient bioactives, also called milk-borne bioactive factors (MbFs), to the infant. These MbFs contribute to organizing infant microbial communities, behavior, cognition, and neurobiology, and as such play a role in determining the child’s trajectory of development, with long-term consequences in the adult (Blum and Baumrucker Citation2008; Brunner et al. Citation2015; Donovan and Odle Citation1994; Hamilton et al. Citation2011; Nicholas et al. Citation2019). This process was coined ‘Lactocrine Programming’ (Bartol, Wiley, and Bagnell Citation2008; Hinde Citation2013) and is depicted in . The effects of Lactocrine Programming have been documented in various mammalian species (Bartol et al. Citation2017; Hinde et al. Citation2015; H. Liu, Radlowski, et al. Citation2014; Neville et al. Citation2012; Nusser and Frawley Citation1997; Sharp et al. Citation2017). Growing evidence has suggested that milk bioactive components, such as opiates and hormones, influence feeding regulation and contribute to the organization of metabolism, growth, and energy balance (Savino and Liguori Citation2008; Neville, McFadden, and Forsyth Citation2002; Hahn-Holbrook et al. Citation2019). For example, ß-casomorphin, the natural opiate found in milk (Jarmołowska et al., Citation2007), is speculated to influence feeding behavior by acting as a sedative, possibly affecting infant appetite.
Figure 1. Lactocrine Programming is the process by which non-nutrient bioactives affect the developmental program of cells, tissues and organs in nursing offspring. The term ‘programming’ refers to the fact that while some effects on the offspring are short-term, others have lasting effects on form, function and/or health and well-being in adulthood. Mechanisms would include affecting the development of the infant’s brain as well as gastro-intestinal system and gut microbiota (indicated by the clockworks in the figure). While several maternal factors may influence this process, also the infant, e.g., through crying behavior, can affect the mother and lactation. Note that the maternal and infant factors presented in the figure may not be all factors involved in Lactocrine Programming.
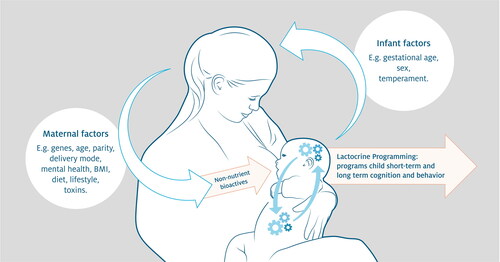
The presence and abundance of milk bioactives could be within the suite of maternal lactation tactics to shift infant phenotypes toward maternal optima as, importantly, such physiological signals embedded within milk would be difficult for offspring to reject (Allen-Blevins, Sela, and Hinde Citation2015). See Interplay of maternal-child factors section for an evolutionary perspective-oriented discussion on how the interplay between maternal and child factors further impacts lactation.
In sum, where public health and clinical perspectives provide accumulating evidence that breastfeeding parameters are linked to later child behavior and cognition, the evolutionary perspective offers an explanation for ‘why’ such mechanistic processes would operate. The combination of both perspectives leads to the hypothesis that human milk, through bioactive constituents, can influence and program child development (Hinde Citation2013). In the following section, we will discuss the current state of the field on milk constituents and their links with child cognitive and behavioral development.
Human milk constituents and their associations with child cognition and behavior
The following sub-sections discuss bioactive milk components, including hormones, immune factors, maternal cells, nutrients, microbes and pollutants that are relevant for offspring cognition and behavior. Background basics on human milk can be found in Box 1, details about specific milk components are summarized in , and presents a summary of milk constituents and their known associations with child cognition and behavior. At the end of this section interactions between milk constituents and links between milk energy output and child development are presented.
Figure 2. Summary of milk constituents and their known associations with child cognition and behavior. The color of the arrows represents the nature of the scientific evidence (i.e., animal, human or both). Microbes have no arrows because there is not yet evidence linking microbes to markers of neurodevelopment. CNS, central nervous system; HMOs, human milk oligosaccharides. Created with BioRender.com.
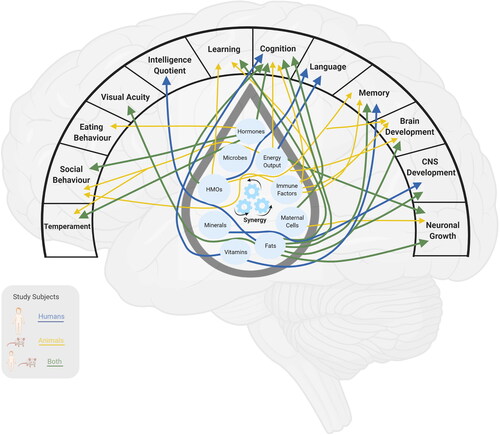
Table 1. Basic information on milk constituents.
Hormones
Glucocorticoids (GCs) are among the best-characterized hormones in milk with respect to offspring development. Animal and human studies have described apparent associations of milk GCs with diverse offspring outcomes, including temperament, social behavior, and cognition (reviewed in Hollanders et al. Citation2017). In an experimental study, milk cortisol altered rodent offspring neurobiology and behavior (Catalani et al. Citation2011). In associative studies in nonhuman primates, milk cortisol concentrations were differentially related to temperament based on offspring sex: male offspring’s nervous and confident temperament ratings were predicted by changes in milk cortisol concentrations across lactation, whereas female offspring’s nervous and confident temperament ratings were predicted by cortisol concentrations in early and peak lactation, respectively (Hinde et al. 2015). Additionally, in rhesus monkeys, higher neonatal milk cortisol concentrations predicted less impulsivity on a cognitive task at 6 months of age but had no associations to effect on global social behaviors (i.e., averages of social play, grooming, etc.) (Dettmer et al. Citation2018). However, female infant macaques ingesting mother’s milk with higher cortisol concentrations exhibited higher frequencies of play behaviors at 4–8 months of age (Dettmer et al. Citation2018).
Milk GCs have the potential to impact many facets of offspring development; however, results thus appear to be sex-specific and are mixed. For instance, in human studies, milk cortisol concentrations have been shown to be positively associated with infant negative emotions, and specifically with fear reactivity, especially in girls (Gray et al. 2013; Nolvi et al. Citation2018). However, separate studies did not observe an association with milk GCs and infant crying (Hechler et al. Citation2018) or with sleep (Toorop et al. Citation2020). Milk GCs are likely absorbed by the infant gut into systemic circulation (Hinde 2013), as shown in experimental studies in rodents (Angelucci et al. Citation1985; Yeh, Yeh, and Holt Citation1989) and observational studies in human infants (Benjamin Neelon et al. Citation2015; Cao et al. Citation2009). As GCs could impact infant brain development, this mechanism would argue for a link between milk GCs and infant behavior and cognitive development. Similarly, corticosterone levels in human milk might impact the programming of the neuroendocrine stress axis and thereby the individual’s stress sensitivity, which is a key determinant for the risk of developing psychopathologies later in life (Lucassen et al. Citation2015).
Other hormones present in milk may also affect offspring development. For example, one study posited that melatonin concentrations are associated with better infant sleep behavior and reduced colic (Engler et al. Citation2012). Additionally, early human milk metabolic hormone concentrations have been shown to be related to later infant growth and adiposity (reviewed in Mazzocchi et al. Citation2019). For example, leptin has been negatively associated with infant fat mass (Sims et al. Citation2020). However, results on other metabolic hormones including ghrelin, adiponectin, and insulin are mixed, as they have been associated with both higher and lower infant weight gain and fat mass gain (reviewed in Mazzocchi et al. Citation2019). Likewise, evidence related to early insulin-growth factor 1 and later adiposity is inconclusive (Galante et al. Citation2020; Kon et al. Citation2014), although a recent study suggested that higher bioactive concentrations of insulin-growth factor 1 are related to lower body mass index (BMI) beyond the first year of life (Galante et al. Citation2020). More research, particularly experimental studies, is needed to discern the effects of these types of hormones in milk on offspring development.
Despite several studies on milk hormones, few are experimental in nature, thus limiting interpretations of causality, and few have concentrated on the direct or indirect influence of these hormones on other aspects of infant behavior, although some research points to impacts of these hormones on appetite regulation and energy homeostasis. For example, in rodent models, availability of leptin is crucial for the development of hypothalamic circuits that control eating behavior and energy homeostasis in early postnatal life (Bouret, Draper, and Simerly Citation2004; Yam et al. Citation2017), and impact later risk for obesity (Skowronski et al. Citation2020). Although it is compelling to suggest that early postnatal leptin levels could have impact on later appetite regulation and eating behavior via the development of hypothalamic circuits (Oddy Citation2012), one must acknowledge that the patterns of brain development (Cardoso-Moreira et al. Citation2019) and systemic and milk leptin levels are different in humans as compared to nonhuman animal models (Schubring et al. Citation1998; Sims et al. Citation2020).
Immune factors
Human milk is a rich source of immune factors that help educate the infant’s innate immune system during a critically important period of development. Emerging research is showing a link between immune factors and neurodevelopment (Mudd et al. Citation2016; Taki et al. Citation2020). It is hypothesized that milk immunoglobulins may be linked to neurodevelopment through the gut-immune-brain axis (Azhari, Azizan, and Esposito Citation2019). Milk IgA contributes to the innate immunity of the infant gut and modulates how microbes interact with host cells (Ramanan et al. Citation2020). Cross-fostering studies in mice have shown that milk secretory IgA influences the composition and activity of commensal gut bacteria (Rogier et al. Citation2014), and emerging research links infant gut microbes to cognitive development and behavioral disorders such as autism spectrum disorder (Carlson et al. Citation2018; Laue et al. Citation2020). However, the underlying mechanisms and the specific bacterial taxa involved remain to be determined (Nitschke, Deonandan, and Konkle Citation2020).
Lactoferrin (Lf), also considered a component of the immune system, is one of the most abundant proteins in human milk. Supplementation of Lf was shown to promote some measures of neurodevelopment and cognition in piglets and rats compared to a standard formula with low Lf (Chen et al. Citation2015; Mudd et al. Citation2016; Shumake et al. Citation2014). Rats tended to show less risk-taking behavior and faster escape responses compared to non-supplemented controls (Shumake et al. Citation2014); however, there was no effect on spatial memory. Of interest, sex differences were observed, with stronger effects for males compared to females (Shumake et al. Citation2014). Piglets supplemented with bovine Lf showed enhanced cognitive functioning and learning as assessed by the eight-arm radial maze test (Chen et al. Citation2015). Further, a cocktail of prebiotics, lactoferrin and milk fat globule membrane administered to piglets resulted in decreased gray brain matter (indicating earlier synapse development and pruning), but no improvement in the spatial T-maze behavioral assessment, compared to controls (Mudd et al. Citation2016). A mechanistic study to explore the function of Lf showed that Lf appears to upregulate the brain-derived neurotrophic factor signaling pathway which is important in memory development, synaptic plasticity, and learning (Chen et al. Citation2015). The authors suggested that the sialic acid moieties of Lf are cleaved from the protein and absorbed to be used for hippocampal and prefrontal cortex development (Chen et al. Citation2015). These same sialic acid moieties and other nitrogen-containing glycans could also promote growth of probiotic gut bacteria and be metabolized to compounds related to neurodevelopment through the gut-brain axis (Cerdó et al. Citation2016; Vega-Bautista et al. Citation2019).
Recent literature has linked other milk immune factors, including specific cytokines and chemokines, to decreased social dominance behavior and improved hippocampal development and memory in rodents (B. Liu, Radlowski, et al. Citation2014; Taki et al. Citation2020). However, how this translates to human biology remains to be determined. More clinical and experimental research is needed to elucidate these complex pathways linking human milk immunoglobulins, lactoferrin, and other milk immune factors to cognitive development and behavior in offspring through the gut-immune-brain pathway.
Maternal cells
Immune cells
Immunologically active cells, including neutrophils, lymphocytes, macrophages, and epithelial cells, are normal constituents of human colostrum and milk. Milk leukocytes can translocate from the gastrointestinal tract to the blood and distant sites, including the lymph nodes, spleen, liver, and mucosa-associated lymphoid tissues (Cabinian et al. Citation2016). In this way, lactocrine-mediated maternal microchimerism may also affect postnatal developmental trajectories (Bartol et al. Citation2017).
Maternal immune cells have been found to play relevant roles for infant behavior and cognitive development (Bilbo and Schwarz Citation2012). Human milk contains a relatively high concentration of macrophages and a plethora of immune factors (cytokines, chemokines, and their receptors) with the potential to interact with microglia (the primary phagocytic cells of the central nervous system) and other brain immunocompetent cells, including astrocytes (Bilbo and Schwarz Citation2009; Salvador, de Lima, and Kipnis Citation2021). There are direct physical interactions between immune cells (dendritic cells) and the nervous system (vagus nerve) during lactation and, in fact, it has been suggested that dendritic cells may act as modulators of neuroinflammation in mood disorders (Leite Dantas et al. Citation2021).
Although there appears to be crosstalk between immune cells and factors present in human milk and in the central nervous system of the infant, the mechanisms and implications for brain development remain essentially unknown. In contrast with adulthood, microglia are activated in early life and are sensitive to immune signals (Bilbo Citation2010). These cells have been considered as potential markers for long-term changes within the brain and may have an early role in later life cognitive decline (Hoeijmakers et al. Citation2016, 2017). Microglia appear to play direct roles in cognition (Tremblay et al. Citation2011), as these cells harbor receptors for several neurotransmitters and neuromodulators (Pocock and Kettenmann Citation2007), many of which are present in human milk. Alterations in glial priming or cytokine production during this period may have lifelong consequences on behavior and cognitive functions (Williamson et al. Citation2011). The functionality of the blood-brain barrier (formed by endothelial cells, astrocytes, and microglia) is pivotal in the interactions between the immune components of human milk and the central nervous system and, as a consequence, in the pathophysiology of many neuropsychiatric disorders that may have a developmental origin (Yarlagadda, Alfson, and Clayton Citation2009). It must be reminded that permeability of the blood-brain barrier is higher during early life (Saunders, Knott, and Dziegielewska Citation2000). Further studies are required to elucidate mechanisms underlying these complex interactions, as well as their clinical significance.
Non-immune cells
Studies carried out during the last decade have shown that human milk cells are more heterogeneous than previously thought. For example, human milk contains mammary gland stem cells and other types of stem cells that can differentiate in vitro into a variety of cell types, including neurons (Hassiotou et al. Citation2012; Hosseini et al. Citation2014). The properties of human milk stem cells make them suitable candidates for microchimerism in the infant tissue (Ninkina et al. Citation2019). Indeed, some studies suggest that human milk stem cells may differentiate and become integrated into different infant tissues (Twigger et al. Citation2013; Hosseini et al. 2014), and it has been found that human milk stem cells reach the brain of mouse pups, settling there and differentiating into both neuronal and glial cell types (Aydın et al. Citation2018). Thus, these cells could have an association with child behavior and cognitive development, although this is currently only a hypothesis (Molès et al. Citation2017). The implications of breastfeeding–induced microchimerism remain poorly understood but this phenomenon might be involved in proliferation, development, or epigenetic regulation of tissues in the infant (Molès et al. Citation2018). Future studies are required to explore their properties and benefits for mothers and infants as well as their potential roles in therapy and regenerative medicine.
Nutrients
Fats
Human milk fats are the major energy source for infant growth. Human milk contains a considerable amount of long-chain polyunsaturated fatty acids (LC-PUFA), docosahexanoic acid (DHA) and arachidonic acid (Ara) and it has been suggested that this is one of the key advantages of breastfeeding over formula feeding with respect to brain development (Basak, Vilasagaram, and Duttaroy Citation2020; Steiner Citation2019). However, this has not yet been proven in humans and the data is somewhat conflicted, as two recent meta-analyses could not draw a conclusion regarding the importance of these nutrients for child cognitive development (Verfuerden et al. Citation2020; Lehner et al. Citation2021). This may not be surprising, as the studies were done in formula-fed infants and many of the important controlling/influencing variables were not, or could not, be collected. In a study by Colombo et al. (Citation2019), higher maternal blood DHA concentrations in pregnancy were related to higher IQs but the effect was confounded by social economic status, a factor that is associated with the choice and ability to breastfeed. Recent advances in high-resolution mass spectrometry methods have enabled a more detailed lipidomic profiling of human milk. Using this method, marked differences in the lipidomic profiles between exclusively breastfed and formulafed infants have been reported (Prentice et al. Citation2015). These methods have identified other lipid components that may contribute to the cognitive development of the infant. Perhaps the most studied is the human milk fat globule membrane, which in addition to containing important proteins, also contains several bioactive lipids that can potentially change brain structure and function (Brink and Lönnerdal Citation2020). The polar lipids (phospholipids) are major structural and functional components of the brain and are involved in brain function (Zheng et al. Citation2019). An exploratory observational study in children found preliminary evidence that sphingomyelin, one of the phospholipids, was associated with brain development and better verbal development (Schneider et al. Citation2019). Human milk fat has a distinctive distribution pattern of palmitic acid (C16:0) with approximately 85% distributed at the sn-1 and sn-2 position of triacylglycerol which in addition to improving absorption, may also influence brain development (Viriato et al. Citation2020). Finally, there are several sialylated oligosaccharides and glycoconjugates that are also present in human milk which have been demonstrated in animal models to influence brain structure and development (Lis-Kuberka and Orczyk-Pawiłowicz Citation2019).
Sphingolipids
Rapid neurodevelopment and growth of the brain during the first two years of life coincide with a peak rate in the accretion of brain complex polar lipids, including sphingolipids (Schnaar, Gerardy-Schahn, and Hildebrandt Citation2014). Sphingolipids are classified as sphingomyelins and glycosphingolipids. Sphingomyelins are particularly rich in the myelin sheath of the central nervous system and play an essential role in axonal maturation and myelin integrity (Bienias et al. Citation2016; Narayan and Thomas Citation2011). This suggests a critical role for these lipids in brain development and function, including neuronal growth, migration, maturation, and myelination (Olsen and Færgeman Citation2017; Zheng et al. Citation2019; B. Wang, Xu, et al. Citation2021).
The majority of brain sphingolipids originate from endogenous synthesis; however, dietary supplementation enhances sphingolipid composition (Ortega-Anaya and Jiménez-Flores Citation2019). Studies conducted in young animals and human infants demonstrated a critical role of sphingolipids and sphingomyelins for cognitive development. Young rats and piglets supplemented with complex milk lipids, rich in gangliosides, sphingomyelins, and phospholipids, displayed improved learning and memory (H. Liu, Radlowski, et al. Citation2014; Vickers et al. Citation2009). Complex milk lipids and milk fat globule membranes (MFGM) supplementation in human infants showed a similar effect. In RCTs, supplemented or breastfed infants achieved higher scores for hand and eye coordination, performance, and general IQ at 6 months (Gurnida et al. Citation2012), enhanced cognitive scores at 12 months of age (Timby et al. Citation2014), accelerated neurodevelopmental profile at 12 months and improved language development at 18 months (Li et al. Citation2019). The administration of sphingomyelin-fortified formula to very low birth weight infants improved latency of visual evoked potentials, sustained attention, intelligence, and behavior rating (Tanaka et al. Citation2013). These few studies demonstrate enhanced cognitive skills in breastfed, as well as complex milk lipid and MFGM supplemented infants at an early developmental stage. It must be acknowledged that both complex milk lipids and MFGM supplementation delivers a mixture of complex lipids. Thus, the enhanced neurodevelopment observed in supplemented pups and infants might not only be confined to the effect of sphingolipids. Therefore, further studies should focus on more precise definitions of exposures and explore if early cognitive advantages associated with sphingolipids prolong later in life.
Proteins and amino acids
Proteome and peptidome analyses show that human milk contains proteins and also free amino acids, and that these change in composition especially in the first few weeks following birth (Dingess et al. Citation2017; Zhang et al. Citation2016). Furthermore, the concentration of the major milk proteins in human milk decrease over the first 6 months postpartum (Zhang et al. Citation2021). Cohort studies in compromised pediatric populations, such as preterm infants, suggest a positive association between protein intake and IQ (van Goudoever et al. Citation2018; Rozé et al. Citation2021). However, the RCTs performed to date, especially in preterm formula-fed infants, do not provide an optimal range of protein intake for studying cognitive outcomes (Hortensius et al. Citation2021; Roelants et al. Citation2018; Ruys et al. Citation2019). High early amino acid intake in preterm infants was associated with higher survival rates in boys, but with a lower mental developmental index among a subgroup of girls who survived without disability (Uthaya et al. Citation2016; van den Akker et al. Citation2014). Finally, a systematic review on the administration of the amino acid glutamine in preterm infants concluded that no significant effect was observed in the three RCTs that examined neurocognitive development in children aged 18 to 24 months and beyond (Moe-Byrne, Brown, and McGuire Citation2016). In sum, the evidence on effects of protein and amino acid on cognition and behavior comes from RCTs in infants with compromised health and results are mixed. To our knowledge, there is a lack of (observational) studies on associations between protein and amino acid content of human milk and cognitive and behavioral development of fullterm infants.
Micronutrients and related compounds
Adequate maternal intakes of minerals and trace elements, including iron, calcium, copper, iodine, selenium, and zinc during pregnancy and lactation are critical for a healthy pregnancy and for fetal growth and infant development (González and Visentin Citation2016; Wu et al. Citation2004). Human milk provides minerals and trace elements for the developing infant (Allen and Hampel Citation2020) with implication for adequate brain development and cognition (Deoni et al. Citation2018; Pang et al. Citation2020). Minerals are known to have a range of effects on the processes of neurodevelopment. For example, calcium and iron are known to have effects of myelination, dopamine receptors, and neurotransmission (Deoni et al. Citation2013). Selenium is essential for the newborn (Dórea Citation2002) and has a range of functions depending on the form; for example, the recently discovered Selenoprotein P has important neurological functions (Arias-Borrego et al. Citation2019; Pitts et al. Citation2014). Iodine is necessary for the biosynthesis of thyroid hormones and the function of the central nervous system (Zimmermann Citation2011). Deficiency or excess of those minerals would affect the human milk mineral and trace elements profile with potential influence in the infant neurocognitive and behavioral development.
Choline, a nutrient with an amino acid–like metabolism, has been associated to recognition memory abilities (Cheatham and Sheppard Citation2015). Higher concentrations of long-chain PUFAs, choline, folic acid, sphingolipids and phosphatides, have been associated with higher myelin levels and cognitive scores (Deoni et al. Citation2018).
There is pre-clinical evidence that early life adversity leads to a deficit in micronutrient composition in the plasma and the brain, possibly contributing to later life cognitive impairment. Supplementation of micronutrients (including Vit B6, B12, folate and additional minerals and amino acids, including zinc, choline and methionine) to lactating dams during stress exposure, restored the micronutrient deficit observed in their early life adversity exposed pups, reaching control levels, as well as partly protected against the early life adversity–induced cognitive decline (Naninck et al. Citation2017).
Vitamins known to have specific effects on early brain development and subsequent function include vitamin B12, folate and choline (Georgieff et al. Citation2018). It has been reported that term milk contains lower concentrations of vitamins (group B) and higher concentrations of vitamins A, E, as well as of carotenes (photosynthetic pigments β-carotene, β-cryptoxanthin, lutein, zeaxanthin and lycopene) than those observed in preterm milk, with potential effects on development (Redeuil et al. Citation2021). Carotenes would exert pivotal effects on brain and ocular development (Giampietri et al. Citation2016) but, in general, there is as yet limited data linking vitamins and carotenes to optimal infant neurocognitive development.
Human milk oligosaccharides (HMOs)
After lactose and lipids, human milk oligosaccharides (HMOs) are the largest solid component of human milk (Bode Citation2012; Kunz et al. Citation2000). HMOs are a group of unconjugated glucans (complex carbohydrates/sugars) composed of glucose, galactose, N-acetylglucosamine, fucose, and sialic acid, with lactose at their reducing end. More than 150 different HMOs have been identified so far. HMO amount and composition vary between women, are remarkably constant throughout the day and over a week within the same woman but change over the longer course of lactation. How the intake of different HMOs affects immediate and long-term infant health and development, including cognition and behavior, is an active area of research (Docq et al. Citation2020). HMOs may directly interact with infant cells and tissues or/and act indirectly by shaping infant intestinal microbiota (Underwood et al. Citation2015; Totten et al. Citation2012). In many cases, the structure of the HMO determines the function of the HMO (Bode and Jantscher-Krenn Citation2012).
There have been several studies on the impact of HMOs on cognition in both animals and humans. Two rodent studies showed a positive impact of the HMO 2′-fucosyllactose (2′-FL) on memory and learning. In one study, newborn rat pups were gavaged with either 1 g 2′-FL/kg body weight or water during the suckling period (Oliveros et al. Citation2016). Rats were then evaluated at 4–6 weeks and 1 year of age using methods to assess cognition. Just after weaning, both groups performed similarly on the Novel Object Recognition test, but at 1 year, rats receiving 2′-FL as pups performed significantly better on this test as well as the Y maze test compared to controls (Oliveros et al. Citation2016). In a separate study, adult rodents were fed 350 mg of 2′-FL/kg body weight for either 5 weeks in rats or 12 weeks in mice (Vázquez et al. Citation2015). Both rats and mice fed 2′-FL performed better on cognitive challenges than the controls in each study. Moreover, there was evidence to show increased expression of brain-derived neurotrophic factor (BDNF) in the hippocampus and striatum, increased cytoplasmic phosphorylated calcium/calmodulin-dependent kinase II in the hippocampus, and post-synaptic density protein 95 in the hippocampus and frontal cortex (Vázquez et al. Citation2015). More recently, a mouse model was developed where one of the genes responsible for synthesis of 6’SL was knocked out (Hauser et al. Citation2021). Compared to control mice, wild-type mice fostered on dams unable to produce 6’SL during lactation exhibited alterations in cognition.
Several studies on piglets confirm the importance of supplementation of sialic acid and/or 2′-FL on cognition. In one study, 3-day old male piglets were provided milk containing up to 830 mg/L of sialic acid (Wang et al. Citation2007). Piglets receiving the sialic acid supplement performed better on learning and memory tests than those not receiving the supplement, and also showed higher mRNA expression of ST8SIA4, a sialyltransferase enzyme, in the frontal cortex and hippocampus, and GNE, an enzyme that regulates biosynthesis of N-acetylneuraminic acid, in the hippocampus and liver (Wang et al. Citation2007). Preterm piglets supplemented with a formula containing 433 mg/L sialyllactose performed better than preterm piglets, not fed sialyllactose, in a spatial T-maze task, and additionally had upregulated genes related to myelination and ganglioside biosynthesis in the hippocampus including glial fibrillary acidic protein (GFAP), myelin-associated glycoprotein (MAG), myelin basic protein (MBP), neuraminidase 1 (NEU1), sialin (SLC17A5), and b-1,3-galactosyltransferase 4 (B3GALT4) (Obelitz-Ryom et al. Citation2019). However, in term piglets, provision of 380 mg/L of sialyllactose from post-natal day (PND) 2 to 22 did not alter recognition memory at PND17 (Fleming et al. Citation2018). In a separate study, piglets provided a combination of 1 g/L 2′-FL + 0.5 g/L LNnT in addition to 12.4 g/L of bovine milk derived oligosaccharides (containing galactooligosaccharides as well as 3′-SL and 6′-SL) had larger volumes of the cortices and corpus callosum, and they exhibited increased recognition memory, and increased time investigating objects compared to control piglets (Fleming et al. Citation2020a). In a separate study by the same group, provision of oligofructose with 2′-FL also resulted in increased recognition memory after a 48 h delay (Fleming et al. Citation2020b). Mediation analysis on several of the piglet studies done by this group revealed an association between bacterial genera selected for by oligosaccharides and short-term as well as long-term memory through GABAergic and glutamatergic genes, as well as myelination transcription factors, brain volume and exploratory behavior (Fleming et al. Citation2021).
Whether HMO effects on cognition translate from animal models to humans is currently under investigation. Several recent human studies revealed correlations between HMOs and infant cognition. In one study on 50 mother-infant dyads of Hispanic origin living in the Los Angeles area, a higher level of 2′-FL in milk at 1 month was correlated with higher cognitive scores of infants on the Bayley-III test at 24 months (Berger et al. Citation2020). Similarly, in the PREOBE study, human milk 2’Fl concentrations were associated with infant composite motor scores at 6 months and 6’SL concentrations were associated with composite cognitive scores at 18 months (Oliveros et al. Citation2021). In a separate study of 659 Malawian mother-infant dyads, it was determined that there was a positive association between the relative abundance of fucosylated and sialylated HMOs in milk and language at 18 months (Jorgensen et al. Citation2020). Finally, a study on 99 mother-child dyads revealed that maternal blood type may have an important role to play in the relationship between HMOs and cognition (Cho et al. Citation2021). For women having blood type A and able to synthesize the HMO alpha-tetrasaccharide, significant associations between 3′-SL and the Mullen Scales of Early Learning (MSEL), particularly receptive and expressive language, was observed. Analysis of the entire dataset did not reveal any associations between HMOs and cognition (Cho et al. Citation2021). Ultimately, human intervention studies with structurally defined HMOs and long-term infant follow-up are required to fully define the potential benefits of HMOs on human cognitive and behavioral development.
Microorganisms
Several studies have revealed the existence of a site-specific microbiota and bacteriome in the pre-colostrum, colostrum, and mature milk of healthy women (Cabrera-Rubio et al. Citation2012; Fernández et al. Citation2020; Hunt et al. Citation2011; Jiménez et al. Citation2015; Jost et al. Citation2014; Ruiz et al. Citation2019; Ward et al. Citation2013) (). In addition to bacteria, human milk harbor viruses, phages, archaea, fungi, and protozoa (Boix-Amorós et al. Citation2019; Jiménez et al. 2015; Pannaraj et al. Citation2018), although our knowledge about the presence of these microbes in this biological fluid is very limited.
Table 2. Summary of the bacterial genera identified with different methodologies in human breast milk samples.
Human milk is an important source of microorganisms that may play a key role in shaping the infant oral and gut microbiome (Biagi et al. Citation2017; Dzidic et al. Citation2018; Le Doare et al. Citation2018; Martín et al. Citation2003; Martín et al. Citation2012; Milani et al. Citation2017; Solís et al. Citation2010). In fact, the infant fecal microbiome seems to be dominated by human milk bacteria for as long as infants are breastfed, independently of the introduction or not of other foods (Bäckhed et al. Citation2015). As a consequence, such early and persistent colonizers may play a role in priming the development and function of many infant systems (Ojo-Okunola, Nicol, and du Toit Citation2018), including the postnatal development of the infant brain through the establishment of the microbiota-gut-brain axis (de Weerth Citation2017; Ratsika et al. Citation2021). And indeed, infant gut microbiota community composition, including milk-oriented microbes, is associated with cognitive and behavioral development (Aatsinki et al. Citation2019; Carlson et al. Citation2018; Carlson et al. Citation2021; de Weerth, Fuentes, and de Vos Citation2013a, de Weerth et al. Citation2013b; Loughman et al. Citation2020).
There are many pathways to communicate between the gut microbiota and the brain, including (a) direct or metabolite-mediated interactions with the autonomic nervous system (ANS), the enteric nervous system (ENS) and the hypothalamic-pituitary-adrenal axis (HPA); (b), interactions with the innate and the adaptive immune system; (c) enteroendocrine signaling; and (d) participation in the biosynthesis and response to several key neurochemicals (e.g., tryptophan precursors and their metabolites, serotonin, γ-amino butyric acid [GABA], catecholamines, etc.), bile metabolites, peptidoglycan, branched chain amino acids, short-chain fatty acids and other compounds that are involved in host cognition, behavior and mood (Allen-Blevins, Sela, and Hinde Citation2015; Carlson et al. Citation2021; Cryan et al. Citation2019; Fox et al. Citation2021; Rhee, Pothoulakis, and Mayer Citation2009).
Adequate gut microbial colonization has been associated with enhanced neurodevelopment (Allen-Blevins, Sela, and Hinde Citation2015; Oliphant et al. Citation2021; Tamana et al. Citation2021). Some strains belonging to lactobacilli and bifidobacteria species, which are frequently detected in human milk, can exert an influence in neurogenesis, neurotransmission, expression of neuropeptides, neuroinflammation and behavior (Cryan et al. Citation2019; Janik et al. Citation2016; Perez-Burgos et al. Citation2013; Sherwin et al. Citation2016). More specifically, they seem to contribute to the establishment of brain neural circuits (Luck et al. Citation2020), in hypothalamic posterior pituitary activity (Erdman and Poutahidis Citation2014), and in the restoration of social behavior after its alteration (Buffington et al. Citation2016). It must be highlighted that the beneficial effects of some of these bacterial species on social behavior using a genetic mouse model of autism were not detected in vagotomized animals (Sgritta et al. Citation2019). In addition, DNA of some strict anaerobes (e.g., Faecalibacterium prausnitzii, Akkermansia muciniphila or Bacteroides spp.), has been detected in human milk and infant feces (Benítez-Páez et al. Citation2020; Collado et al. Citation2012; Jeurink et al. Citation2013; Jiménez et al. 2015; Ward et al. Citation2013). These organisms can potentially exert strong influences on infant neurodevelopment (Hao et al. Citation2019; Song et al. Citation2019; Tamana et al. Citation2021; Yaghoubfar et al. Citation2020; Yaghoubfar et al. Citation2021; J. Wang, Xu, et al. Citation2021).
Finally, human milk bacteria are one of the drivers of the differences existing between the gut microbiota of breastfed infants and that of formula-fed infants, mainly due to the presence of Bifidobacterium strains. Some Bifidobacterium strains are able to modulate the tryptophan metabolism (Tian et al. Citation2022), or to produce neurotransmitters, such as Gamma aminobutyric acid GABA (Duranti et al. Citation2020; Yunes et al. Citation2016), and Indole-3-lactic acid (a metabolite of tryptophan, Meng et al. Citation2020). Interestingly, formula diet alters the colon microbiota of piglets and appears to shift tryptophan metabolism from serotonin to tryptamine (Saraf et al. Citation2017). Altogether, studies performed so far suggest that milk bacteria play relevant roles for infant cognitive and behavioral development, but their actual relevance and impact are far from elucidated yet.
Pollutants
Human milk does not only contain naturally occurring constituents. Environmental pollution leads to the introduction of novel and often toxic substances to milk. In many cases, these milk components are poorly metabolized and hardly excreted. The accumulation of milk pollutants in an infant’s bones, kidneys, liver, and fat tissue is associated with long-term and negative consequences for health and development (Al-Saleh Citation2021; Rebelo and Caldas Citation2016). Persistent organic pollutants (POPs) and heavy metals are the most extensively studied air and food pollutants found in human milk sampled from different regions worldwide (reviewed in Pajewska-Szmyt, Sinkiewicz-Darol, and Gadzała-Kopciuch Citation2019). POPs and heavy metals present strong geno-, immuno-, and cytotoxic properties and have been shown to be associated with a range of adverse health outcomes, including neurological and behavioral disorders regardless of the period of exposure (Bauer et al. Citation2020; Nelson et al. Citation2019; Vrijheid et al. Citation2016). Concentrations of various POP groups in maternal milk have been estimated to be up to six times higher than in maternal serum (Thundiyil, Solomon, and Miller Citation2007). Compared to maternal serum, elevated levels in milk have also been demonstrated for some heavy metals (Sharma et al. Citation2019; Dórea Citation2021).
Although maternal exposure to both POPs and heavy metals during pregnancy has been shown to cause child neurodevelopmental delay (Benjamin et al. Citation2017; Castriotta et al. Citation2020; Nishijo et al. Citation2014; Yamazaki et al. Citation2018), epidemiological studies on postnatal exposure via breastfeeding are inconclusive, demonstrating from none to significant effects (Gascon et al. Citation2013; Pajewska-Szmyt, Sinkiewicz-Darol, and Gadzała-Kopciuch Citation2019). A recent study conducted in Taiwanese mother–breastfed infant pairs (Kao et al. Citation2019) demonstrated that higher milk concentrations of 4,4′-dichlorodiphenyltrichloroethane were related to lower infant cognitive and language performance, while higher milk trans-chlordane concentrations were related to lower social and emotional performance, between 8 and 12 months of age. Similar negative associations were found for concentrations of milk dioxins and cognitive and motor skills in 4-month-old Vietnamese infants (Tai et al. Citation2013).
Drinking water and soil are important sources of exposure for heavy metals (cadmium, chromium, mercury, manganese, and lead) in breastfed infants (Cardoso et al. Citation2014). Concentrations of metals in breast milk vary significantly between countries and studies (Dórea Citation2019). These concentrations reflect prenatal exposure when most neurodevelopmental effects are already programmed (Dórea Citation2021). Long half-life metals (lead, cadmium) accumulating in various maternal organs pose a risk for breastfed infants even before conception. In mothers with a high body accumulation of pollutants, breast milk is an important determinant of infant health risks (Ettinger et al. Citation2014). For example, the physiological mechanisms that are in place to supply calcium to the fetus and infant, lead to the mobilization of maternal bone lead, an important lead source in breast milk. Ronchetti et al. (Citation2006) hypothesized that maternal bone lead storage is the most important factor predicting its neurotoxicity associated with child IQ. However, in addition to milk concentrations, neurodevelopmental outcomes will also depend on current maternal diet, child nutritional status, and genetic makeup, and the nature of exposure to metals (acute vs. chronic). Although many studies have investigated the concentrations of heavy metals in breast milk, only a few specifically assessed the effect of the exposure to heavy metals from breast milk on neurodevelopmental outcomes. An epidemiological study in Brazil demonstrated that higher milk concentrations of lead and mercury correlated negatively with mental and psychomotor development, as well as the age of walking and talking, in infants and toddlers living in the vicinity of tin ore kilns and smelters (Marques et al. Citation2014). Increased concentrations of mercury in breast milk were also associated with lower parental evaluations of neurodevelopmental status (Al-Saleh et al. Citation2016). In the same population, increased multichemical exposure, including manganese and selenium in breastmilk, was related to a higher risk of lower parental evaluations of neurodevelopmental status (Al-Saleh et al. Citation2019). Contrarily, no consistent association between breast milk mercury levels and infant cognitive outcomes was found in Mediterranean countries (Greece, Croatia, Slovenia, Italy); however, higher milk mercury concentrations were related to suboptimal infant fine motor performance (Barbone et al. Citation2019).
Interactions among milk constituents
The majority of research on the composition of human milk evaluates the effects of individual milk components. This is a result of mostly siloed research areas, lack of statistical methods to integrate different types of data, and lack of advanced laboratory analysis techniques to measure multiple milk components concurrently. Nonetheless, interactions and synergistic relations between human milk components are now an area of much interest due to the rise of interdisciplinary and collaborative team science, the development of multi-omic techniques that can simultaneously examine multiple components from the same milk sample, and the emergence of machine learning based methods for integrating and analyzing diverse datasets. Human milk scientists are calling for new approaches and proposing advanced computational methods to study milk as a “system within a system” (Christian et al. Citation2021; Shenhav and Azad Citation2022) – that is, milk itself is a biological system comprising many components, which collectively serve as a fundamental component of the mother-milk-infant “triad” that directs early development (Bode et al. Citation2020).
Recent studies show correlations between different human milk components, including amino acids, sugars, lipids, proteins, hormones, HMOs, and milk microbiota (Gómez-Gallego et al. Citation2018; Linderborg et al. Citation2020; Moossavi et al. Citation2019a; Williams et al. Citation2017; Pace et al. Citation2021). Gómez-Gallego et al. (2018), showed that lactate, creatine, proline, lacto-N-fucopentaose 1, 2′-fucosyllactose and very low-density lipoprotein particles were positively correlated with gammaproteobacteria and negatively correlated with alphaproteobacteria, betaproteobacteria and bacilli. In addition, Moossavi et al. (Citation2019a), showed that certain HMOs are positively correlated with bifidobacteria in milk, and certain fatty acids are correlated with milk microbiota richness and diversity. Williams et al. (Citation2017), showed correlations between complex bacterial communities in milk and HMOs, maternal cells, and other nutrients. The same group further showed that variations in these milk components were collectively associated with differences in the microbial community structures of infant feces and the abundance of specific taxa (Pace et al. Citation2021). Finally, Linderborg et al. (Citation2020), showed that higher milk cortisol concentrations are related to a greater abundance of lauric and myristic fatty acids. These correlational studies suggest that individual milk components are inter-related and potentially influence one another. Next steps involve moving beyond correlational analyses to understand how different milk components may be modifying the biological effects of other components through synergistic interactions that can up or down regulate their individual functions and impact on neurodevelopment. This type of systems biology approach has been used successfully in other areas of research, such as multi-omic characterization of maternal serum in the context of preterm birth (Jehan et al. Citation2020).
Two observational studies have examined the synergistic effects of milk components on human brain and cognitive development (Cheatham and Sheppard Citation2015; Wang et al. Citation2003). Cheatham and Sheppard (Citation2015), showed that higher choline combined with higher lutein, and increased DHA combined with higher choline in milk samples were related to better recognition memory in infants at 6 months. Wang et al. (Citation2003) found significant correlations between brain sialic acid and brain LC-PUFA concentrations among breastfed infants, which may also indicate that these individual components work together to improve infant brain development. Overall, more research is needed to characterize the interactions and synergies between milk components and to evaluate milk “as a whole” – both in general, and in the context of infant neurodevelopment.
Milk energy output
Adequate energy intake is essential for brain development, especially during sensitive periods (Uberos et al. Citation2021, Bautista et al. Citation2019). Additionally, energy deprivation in preterm neonates is associated with poorer indicators of brain development, such as language development (Lithoxopoulou et al. Citation2019). Animal studies have shown considerable evidence that milk energy output is particularly important for offspring growth and development, including biobehavioral development (Hinde Citation2007, Citation2009). Moreover, higher milk energy may facilitate offspring engagement in energy-requiring behavior and social interactions and potentially program behavioral development. Hinde and Capitanio (Citation2010) presented the first evidence for the association between the natural variation in Available Milk Energy (AME) and infant behavior and temperament in rhesus macaques: heavier mothers with multiple previous pregnancies produced greater AME in the early postnatal period and their infants showed higher activity levels and greater confidence in a stressful setting (Hinde and Capitanio 2010). The availability of milk energy might reflect the maternal environment and constitute a signal that helps the infant to adapt appropriately. Likewise, Dettmer and colleagues (Dettmer et al. Citation2018) showed that rhesus macaques’ milk yield, which affects AME, was associated with social behavior in female offspring and cognition in both sexes. Female infants who received more milk during early postnatal life showed more mounting behavior. Higher early postnatal milk yield also accounted for better cognitive inhibitory control at later developmental stages (Dettmer et al. Citation2018).
Interestingly, infant formula may have higher energy content than human milk (Hester et al. Citation2012), and formula feeding has been associated with adverse developmental outcomes compared to human milk feeding (Pang et al. Citation2020), although multiple mechanisms and human milk components may underlie this association. A double-blinded, randomized controlled trial (RCT) showed that infants fed with reduced-energy MFGM supplemented formula had better cognitive scores at the age of one year compared to infants fed with standard formula (Timby et al. Citation2014), although the differences in neurocognitive performance were attenuated by the age of 6 years (Timby et al. Citation2021). This suggests that at least in WEIRD (Western, Educated, Industrialized, Rich, and Democratic) populations, reducing the energy content of formula in early life does not have significant impact on later neurocognitive outcomes, and subsequently, that other components of milk may be more relevant for neurocognitive development.
Factors affecting milk constituents
Maternal factors
There is considerable variation in milk composition between mothers. Numerous maternal factors, such as age, parity, smoking, delivery mode, and environmental pollution, have been suggested to contribute to this inter-individual variation in milk composition (Azad et al. Citation2018; Bachour et al. Citation2012; Bahreynian, Feizi, and Kelishadi Citation2020; Bernstein and Hinde Citation2016; Burianova et al. Citation2019; Dritsakou et al. Citation2017; Hahn et al. Citation2018; Fernández et al. 2020; Moossavi et al. Citation2019b; Pittet, Johnson, and Hinde Citation2017; Pundir et al. Citation2019; Samuel et al. Citation2020; Whitfield et al. Citation2020). For example, a recent study found an association between lipid and energy contents in breast milk and maternal pregestational nutritional status, gestational weight gain, smoking and hypertension (Amaral et al. Citation2021). However, maternal factors affecting milk composition, overall, show inconsistent findings or may have been understudied (Samuel et al. Citation2020). Also, the evidence comes mainly from high-income rather than low-middle income populations, while the variability of some human milk components could be influenced by geographical locations and sociodemographic factors (Samuel et al. Citation2020). Modifiable maternal factors may also interrelate with fixed factors such as maternal traits, characteristics, or genetics, and might be important targets for interventions to optimize the lactation process (see also ). Nevertheless, there is a large body of literature on maternal diet and mental health in relation to human milk composition and below we will describe the current state of research of those two factors.
Maternal diet
There is a general acceptance of the importance of maternal diet in determining breast milk composition. This mostly accounts for human milk’s fatty acids composition, such as PUFA’s, which have been studied extensively and appear to be influenced by maternal diet (Bravi et al. Citation2016; Jonsson et al. Citation2016; Keikha et al. Citation2017; Perrin et al. Citation2019). In addition to the intake of individual nutrients or foods that have been related to human milk’s fatty acid composition, Bravi and colleagues (Bravi et al. Citation2021) recently also found an association between maternal dietary patterns and the fatty acid composition in human milk. Traditionally, other milk macronutrients such as carbohydrates and protein were considered less sensitive to maternal dietary intake (Bravi et al. Citation2021; Dror and Allen Citation2018; Keikha et al. Citation2017), leading to the hypothesis that gross human milk composition is buffered for variations in maternal dietary intake (Mitoulas et al. Citation2002). However, this idea has been challenged by recent findings (Samuel et al. Citation2020). For example, Ward and colleagues (Ward et al. Citation2021) showed that high-fat and high-sugar meals result in acute changes (i.e., within 24 hours) in fat, lactose and protein levels in breast milk. Regarding human milk’s micronutrients, there is probable evidence for an influence of maternal diet, although this differs for specific constituents (for a review, see Samuel et al. Citation2020). For instance, adherence to a Mediterranean diet was found to influence the concentration of iodine and selenium in human milk (Sánchez et al. Citation2020; Valent et al. Citation2011). Contrarily, Butts and colleagues (Butts et al. Citation2018) found that maternal variations in calcium intake, were not reflected in their milk. This may be explained by physiological changes in calcium metabolism during lactation (Olausson et al. Citation2012). The levels of certain vitamins in human breast milk are associated with maternal dietary intake (Keikha et al. Citation2017). Specifically, the intake of fat-soluble vitamins such as vitamin B1, B2, and C is related to their concentrations in human milk (Keikha et al. Citation2021). There is some first evidence suggesting that certain bioactive factors, such as human milk microbiota, are sensitive to maternal diet (Moubareck Citation2021; Williams et al. Citation2017). For example, Kumar and colleagues (Kumar et al. Citation2016) reported on multiple associations between the intake of fatty acids and milk microbiota, for example monounsaturated fatty acids of milk were negatively associated with proteobacteria, but positively associated with the Lactobacillus genus.
There are more indications from both human and animal studies for potential programming effects of maternal diet on offspring’s behavioral and cognitive development, mediated by human milk composition. Specifically, maternal consumption of fatty acids would be of great importance for infant brain development, as previously described in the Fats section. De Melo and colleagues (de Melo et al. Citation2019) supplemented female rats with avocado oil and pulp resulting in improved memory in adolescent and adult offspring. Similarly, memory performance and cognitive functioning of offspring improved when the lactating dam’s diet was supplemented with cashew nuts (de Melo et al. Citation2017), fish oil (Rachetti et al. Citation2013), olive oil (Pase et al. Citation2015), and linseed oil (Fernandes et al. Citation2011). On the contrary, maternal intake of unhealthy fats such as trans fatty acids (Islam et al. Citation2019), resulted in adverse effects on offspring’s cognitive development. For example, Pase and colleagues (Pase et al. Citation2017) found that memory functioning was impaired in adult offspring of the rats that received a diet rich in trans fatty acids during lactation, compared to receiving a diet with an optimal ratio of n-6/n-3. There are a few longitudinal human studies investigating maternal fatty acid intake, specifically DHA supplementation during lactation, and offspring behavioral and/or cognitive development. Positive relationships have been found between maternal DHA supplementation, DHA levels in human milk and infant and child’s cognition and behavior, such as improved mental development (Jensen et al., Citation2005), sustained attention (Jensen et al., Citation2010), a sex-specific association on problem-solving and language development (Lauritzen et al. Citation2005), boy’s prosocial behavior (Cheatham et al. Citation2011) and mental test scores (Helland et al., Citation2003). However, an association of DHA supplementation and most of the (other) studied behavioral and cognitive outcomes is lacking or only present at a certain age. Since evidence is still limited on potential programming effects, caution is warranted in interpreting these results and more investigation is needed.
Finally, certain food flavors of the mother’s diet transfer from mother to child through the amniotic fluid and breast milk and shape the child’s later food preferences and acceptance (Cooke and Fildes Citation2011; Ventura Citation2017). Consistent with this, a recent systematic review shows moderate evidence that maternal diet during lactation can influence the flavors profile of human milk within hours or months of recurrent food intake (Spahn et al. Citation2019). Next to flavor, human milk odor has been shown to promote more (non-)nutritive sucking in a feeding context compared to infant formula odor or water (Loos, Reger, and Schaal Citation2019).
Maternal mental health
Another important factor influencing milk composition and volume is the mother’s mental health and psychological state. Several studies indicate that maternal psychological distress may influence the immune properties of human milk, but the results are conflicting. For example, postpartum-specific stress and negative states (e.g., anxiety and hostility) were inversely associated with the milk concentration of secretory IgA (Hart et al., Citation2004; Kawano and Emori Citation2015; Moirasgenti et al. Citation2019) and lactoferrin (Ziomkiewicz et al. Citation2021b). In contrast, a positive correlation was found between maternal distress and milk secretory IgA (Groër et al., Citation1994; Hart et al., 2004; O’Connor et al. Citation1998). Finally, a recent study reported no association between maternal psychosocial distress and 22 immunological factors in milk (Aparicio et al. Citation2020). With respect to positive mental health factors, maternal social support was recently found to be correlated with milk IgG (Ziomkiewicz et al. Citation2021a). Therefore, larger studies with long-term follow-up are suggested to further investigate the potential influence of maternal mental health factors on breast milk immunoglobulins. Findings also suggest a potential association between maternal psychosocial distress and milk microbiota, with the bacterial diversity in milk at 3 months post-delivery being lower in women experiencing high maternal psychosocial distress (Browne et al. Citation2019).
Regarding milk hormones, a positive association was found between maternal psychosocial distress in the early postnatal period and milk cortisol concentrations (Aparicio et al. Citation2020). Another study found a relation between maternal hostility and milk cortisol concentrations (Hart et al., 2004). However, a recent study found no significant difference in breast milk glucocorticoid circadian rhythm between mothers seeking consultation for psychiatric complaints and a control group with no mental health issues (Romijn et al. Citation2021). Interestingly, an RCT demonstrated that relaxation therapy reduced maternal stress and hindmilk cortisol concentrations in the early postnatal period (2 weeks), but no effect on milk cortisol was found later on. Moreover, relaxation group infants were also reported to sleep longer, have higher milk intake, and demonstrate greater weight gain (Mohd Shukri et al. Citation2019). Other experimental studies using relaxation therapies, such as meditation and music therapy, showed beneficial effects of the intervention on milk volume or expression, energy and milk fat (Ak et al. Citation2015; Dabas et al. Citation2019; Feher et al., Citation1989; Keith, Weaver, and Vogel Citation2012; Kittithanesuan et al. Citation2017; Varişoğlu and Güngör Satilmiş Citation2020). Furthermore, milk energy density, fat, and medium-chain and long-chain saturated fatty acids were also found to be associated with long-term stress during the postpartum period (Ziomkiewicz et al. Citation2021b).
Child factors
Some fetal and child factors, such as biological sex and gestational age at birth, are associated with milk constituents that are related to offspring cognition and behavior, as demonstrated by human and nonhuman animal studies (reviewed in Galante et al. Citation2018). One of the best studied factors is offspring sex. Accumulating evidence suggests that the concentration of milk bioactives as well as the sensitivity and time windows for their effects vary between male and female offspring (Dettmer et al. Citation2018; Galante et al. 2018; Hinde et al. 2013, 2014; Hinde 2009; Petrullo, Hinde, and Lu Citation2019). At present, sex effects are difficult to test experimentally.
Milk constituents have been found to differ as a consequence of offspring sex in several mammal species. In rhesus monkeys, mothers produce different milk for males than for females: during peak lactation at 3–4 months of age, male infants receive milk higher in energy density whereas female infants receive higher milk yield (Hinde 2009); these differences relate to sex-specific differences in infant mass and growth (Hinde 2009; Hinde et al. 2015). Rhesus monkey female offspring also receive mothers’ milk with greater calcium concentrations (Hinde et al. 2013), which is likely related to earlier skeletal ossification in female primates (Cheverud Citation1981). However, mothers of male and female offspring exhibit no differences in the amount of milk cortisol in early (1 month) or peak (3–4 months) lactation (Hinde et al. 2015), or in phosphorous at peak lactation (Hinde et al. 2013). In dairy cows, Holsteins produce more milk for female than male offspring, and gestating a female (heifer) first results in greater milk yield for the second offspring (Hess et al., Citation2016; Hinde et al. 2014). One study also found higher fat content in the colostrum of cows that birthed heifers (Angulo et al. Citation2015), and another found reduced saturated fatty acid content in milk produced by mothers of heifers (Gillespie et al., Citation2017). Additionally, in marsupials (e.g., kangaroos and wallabies), mothers produced milk with higher protein content for male offspring (though there were no sex differences in energy content or milk volume; Robert and Braun Citation2012). In humans, differences relating infant sex to mother’s milk composition are much less clear and likely due to the paucity of studies on the topic, other differences in global regions studied, as well as sampling and analytical techniques used (reviewed in Galante et al. 2018). For example, one study of Filipino mothers that did not include foremilk samples found no relations of sex with milk composition, whereas other studies in Iraq, the United States, South Korea, Kenya, and Australia found associations of infant sex with calcium, phosphorous, insulin, and leptin concentrations and the macronutrient and energy content, depending on the study (see Galante et al. 2018).
Another factor influencing milk composition is gestational age at birth, a factor that has only been studied in humans thus far, and consequently has not been studied experimentally (although one can envision experimental studies in nonhuman animals). An early study found that, across the first 12 weeks postpartum, mothers of preterm infants had higher protein nitrogen and lower calcium and phosphorous concentrations than those of full-term infants (with no differences in non-protein nitrogen, energy, fat, sodium, magnesium, or zinc; Butte et al. Citation1984). More recent studies have confirmed differences in calcium and phosphate in preterm milk (Underwood Citation2013), and reported less zinc, copper, and selenium than observed in milk from term deliveries (Sabatier et al. Citation2019). Likewise, the milk of mothers who delivered prenatally had higher fat and energy content (Fischer Fumeaux et al., Citation2019), higher concentration of 3FL and lower concentration of other HMO’s (Austin et al. Citation2019). A review of studies comparing fatty acid composition in the milk of mothers of preterm compared to full-term infants found that DHA values were higher in preterm milk, with no differences in the concentration of saturated and monounsaturated fatty acids (Bokor, Koletzko, and Decsi Citation2007), while a more recent study suggested a lower concentration of medium- and short-chain fatty acids in the milk of mothers of preterm infants (Dai et al. Citation2020). Human milk microbiota also appears to be different according to gestational age at birth (Khodayar-Pardo et al. Citation2014), but gestational age was not associated with the microbiota composition at 3–4 months postpartum (Moossavi et al. Citation2019b). However, the microbial communities in the human milk microbiota of mothers delivering prematurely are highly individualized in the first two months postpartum, and are affected by maternal factors (Asbury et al. Citation2020).
Finally, certain hormones are present in higher concentrations in milk of mothers delivering preterm compared to term. The list includes neurotrophic factors (Collado et al. Citation2015) and glucocorticoids (Pundir et al. Citation2019), but not gonadotrophins, thyroid hormones (Vass et al. Citation2020) or leptin (Resto et al. Citation2001). Infant factors that may be expected to influence maternal milk composition but that have not been extensively studied to date include infant birth order, oral microbiota, behavior, and illness. For example, illness in the infant has been associated with changes in immune factors in the mother’s milk (Breakey et al. Citation2015; Bryan et al. Citation2007; Riskin et al. Citation2012).
Interplay of maternal-child factors
Tradeoff allocations
As stated previously (Human milk and child development section), evolutionary theory helps inform lactation and human milk composition in the light of natural selection. Life History Theory organizes our understanding of how natural selection has (likely) shaped lifespan investments and tradeoff allocations for maintenance, development, and reproduction across an organism’s life-course (Hill 1993; Hill and Kaplan 1999). Due to the high resource demands of female reproduction, investment strategies critical to reproductive success have been strongly shaped by natural selection (Jasienska, Citation2009). Lactation often exceeds the costs of gestation due to increased energy demands of larger, more active ex utero offspring and due to lower efficiency of energy transfer via milk compared to nutrient transport via the placenta (Jasienska, Citation2009; Tully and Ball Citation2013; Hinde and Milligan 2011). At any given time, tradeoff allocations can therefore be between maternal condition and reproduction, maternal survival and reproduction, the quantity and condition of offspring, and for young mothers, their own growth/development and reproduction (Clutton-Brock Citation1991; Stearns Citation1992). Additionally, a female’s reproductive success is determined by her production of surviving, reproductive offspring across a reproductive career; hence, there can be tradeoffs for the mother between allocation of resources toward current offspring and transitions to allocation for future reproduction (Tully and Ball Citation2013; Clutton-Brock Citation1991; Wells Citation2003). All these tradeoffs have the potential to impact milk composition.
Parent-offspring conflict
At the same time, within a life history framework, we must simultaneously consider the developmental priorities and tradeoffs of the offspring (Trivers Citation1974). Due to divergent relatedness between self and siblings, an individual offspring’s tradeoff priorities are expected to often diverge from the mother’s tradeoff priorities. This is the core tenet of parent-offspring conflict (Trivers Citation1974) and an essential evolutionary theory construct for investigating mother’s milk and infant development (Allen-Blevins, Sela, and Hinde Citation2015).
During lactation, maternal-offspring conflict may occur across all dimensions of the lactation strategy including the frequency of nursing bouts, the duration of lactation until completion of weaning, and the composition and yield of mother’s milk (Hinde and Milligan 2011). Communication and signaling between mother and infant are the interface in which mother and offspring negotiate nursing behavior and milk production and transfer (Allen-Blevins, Sela, and Hinde Citation2015). Infants can signal with behaviors such as high vocalization and/or frequent non-nutritive suckling, whereas the mother could respond through restricting nipple access, affecting the amount and composition of milk produced (Wells Citation2003; Fewtrell et al. 2020).
Maternal and offspring lactation tradeoffs are even starker for malnourished, ill, stressed, or adolescent mothers and their offspring with greater mortality risk (Pittet, Johnson, and Hinde Citation2017). Under such marginal conditions, transferring milk components to regulate infant feeding behavior may be more about coordination than conflictual, so that the infant might prioritize honest signals when truly hungry rather than wasting energy to demand for feed (Wells Citation2003; Allen-Blevins, Sela, and Hinde Citation2015).
RCTs can help uncover how clinical interventions may reduce the tension of parent-offspring conflict through mother-infant signaling. For example, mothers who received relaxation therapy during breastfeeding (reducing maternal stress, hence preventing waste of energy) showed altered milk composition and increased infant milk intake, which subsequently increased infant sleeping duration and promoted growth (Mohd Shukri et al. Citation2019). Although the overall evidence on the relation between milk components and infant behavioral outcomes are often equivocal and remain poorly understood, these new studies are demonstrating important opportunities to understanding underappreciated lactation adaptations with the potential for improving clinical support of lactation and mother-infant dyads (Fewtrell et al. 2020; Pittet, Johnson, and Hinde Citation2017; Neville et al. Citation2012).
Recommendations and best practices for future research studies
Study set-up
Following best practices in milk research requires careful study design that accounts for many variables, including an in-depth understanding of breastfeeding practices of the population studied for human studies, choosing appropriate animal models for specific research questions, and the logistics of milk collection, storage, and analysis. To ensure best practices are being followed, a large amount of work needs to be done prior to starting a human milk study. presents a comprehensive set of questions that researchers can use to guide their study design. The questions are divided into more general study design issues and more specific methodological issues. This guide is not necessarily exhaustive, but by using it, researchers can avoid inadvertently overlooking important criteria when setting up new studies. is a detailed graphical representation of the necessary steps to take and decisions to make when setting up a human milk study. Further resources that may be useful for setting up a human milk study are the articles by Miller et al. (Citation2013) on field and laboratory methods and Neville et al. (Citation2012) on defining and refining critical questions, and the books by McGuire and O’Connor (Citation2020) on sampling and measuring constituents, and by Akers (Citation2002) on lactation and the mammary gland.
Figure 3. Detailed visual representation of the steps that need to be considered and the decisions that need to be taken when setting up a human milk study, from study design to laboratory analyses and bioinformatics.
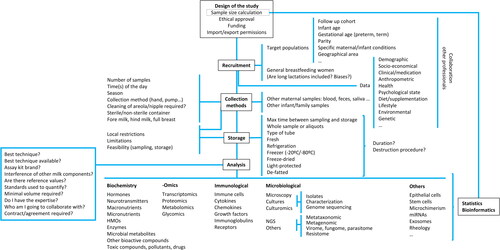
Table 3. Guide for setting up a research study with human milk.
The majority of milk research has been conducted in WEIRD populations, and extending this research beyond these populations is critical to our advancement of knowledge in this field. However, researchers may have to account for unique challenges in research design and limits to data collection when doing so. For example, when possible, it is ideal to collect and report information from study participants about gestational age and infant maturity at birth, but this information may be lacking or inaccurate in populations with limited access to prenatal care. Similarly, it is ideal to collect data about potential interactions with the milk components being investigated, such as medications and vitamins the participant is taking, but this information may be difficult to obtain in some populations, such as populations with high rates of illiteracy. Thus, gold standard collection, storage, and analysis protocols may need to be modified for some studies. Taking on these challenges means planning for the realities of fieldwork in these settings and acknowledging how adjustments made to accommodate logistic compromises may bias the sample integrity.
Assessment of child cognitive and behavioral development
A cognitive assessment for (young) children usually includes an assessment of a) comprehensive background information through interviews with the child (if old enough), parents and, in some cases also school teachers, and b) the administration of standardized tests by trained professionals. Example standardized tests that are often used include the Wechsler Preschool and Primary Scale of Intelligence (WPPSI; ages 2.5–7 years) and the Wechsler Intelligence Scales for Children (WISC; ages 6–17 years) (Wechsler Citation1967; Wechsler Citation2014). These tests are continuously updated, making it important to use the most current one. It is also possible to use tests for more specific cognitive functions, such as the STROOP task to measure the ability to inhibit cognitive interference (Stroop Citation1935) and the Rey–Osterrieth Complex Figure Task to measure visuospatial constructional ability and memory (Davies et al. Citation2011). Also, parental report to measure child executive functions, like the Behavior Rating Inventory of Executive Function (BRIEF), are often used, preferably in combination with cognitive tasks (Wallisch et al. Citation2018).
To test the development of even younger children and infants, the Bayley Scales of Infant and Toddler Development are often used (ages 1–42 months) (Bayley Citation1969). This measure consists of a series of developmental play tasks and derives a developmental quotient (DQ) rather than an intelligence quotient (IQ). A comparable instrument of widespread use is the Mullen Scales of Early Learning (MSEL; Mullen Citation1995) that measures cognitive and motor development in children ages 0–68 months. Also in this younger age group, parental report is often used to measure toddler executive functioning (e.g., the BRIEF for Preschoolers, the BRIEF-P; Wallisch et al. Citation2018; Sherman and Brooks Citation2010).
As many standardized tests are often tailored to Western populations and costly, they are not available nor adequate for non-Western children. Recently, an open-source WHO-supported instrument has been developed to increase accessability and promote universal use, the Infant and Young Child Development (IYCD) Indicators. This simple-to-use caregiver report tool is for children ages 0–3 years, contains 100 items, performs well for cognitive testing, and has been shown to be a reliable across countries (Gladstone et al., Citation2021; Lancaster et al. Citation2018).
The cognitive tests described above have the advantage of being often used. However, they render measures of cognition that may be too crude to accurately measure the specific cognitive processes that are (slightly) quantitatively impacted by early nourishment. Tests that make use of advanced methodologies that deliver fine-grained measurements are also showing to be promising instruments for predicting cognition. For example, the use of eye-tracking technology has delivered research results relating pupil size (only in adults to date; Tsukahara and Engle Citation2021) and infant habituation performance to cognitive abilities. Infant habituation performance (i.e., looking behavior) has considerable predictive power from early infancy to school age, adolescence, and even adulthood intelligence (Bornstein and Sigman Citation1986; Fagan et al., Citation2007; Kavšek Citation2004), and explains as much as 40% of the variance in cognitive performance (Fagan & McGrath, Citation1981).
Behavior assessments are different from cognitive assessments, as they have no right or wrong answers. Instead, behavior assessments investigate how children interact with the people and world around them. Many behavior assessments can identify behavior patterns as well as reasons for the behavior. Often parents, teachers and/or others are asked to observe the child in daily life and answer questions. Also, children can be asked to fill out a questionnaire about their own behavior, thoughts and emotions, but, pending on the length and difficulty of the questionnaire, this often proves difficult before the age of 10. Some commonly used behavior assesments include the Behavior Assessment System for Children (BASC; Reynolds and Kamphaus Citation2004), Achenbach Child Behavior Checklist (Achenbach Citation1991), and the Strengths and Difficulties Questionnaire (Goodman, Citation1997). As these behavior assessments are mostly developed for use in Western populations, the cross-cultural suitability should be checked before use. Next to reports and questionnaires, child behavior can also be observed. For example, standardized instruments designed to assess behavioral dimensions through a series of episodes that mimic everyday situations can be used. Examples are the Laboratory Temperament Assessment Battery (Lab-TAB; infancy to middle childhood; Gagne et al. Citation2011) and the Neonatal Behavioral Assessment Scale (Brazelton and Nugent Citation2011).
Minimum reporting requirements
presents a list of study characteristics that ideally should be included in all studies of human milk. These recommendations for researchers on minimal reporting issues for human milk studies and analyses are meant to ensure that the quality of reporting is high, the research is replicable, and that the data can eventually be re-used, e.g., integrated to that of other studies for meta-analytic purposes. It may not always be possible to follow gold standard practices in every research setting, but detailed high-quality reporting of processes is fundamental for understanding study results.
Table 4. Minimum reporting requirements for human milk studies.
Open questions and innovative ideas
Our understanding of human milk research has come a long way in the past 20 years; however, there is still much that we do not know. Also, despite having a growing number of studies with persuading results, we cannot yet state that there is compelling scientific evidence that unequivocally demonstrates that breast milk affects offspring behavior and builds better cognition. As discussed in the Assessment of child cognitive and behavioral development section, many of the tools used to date may be too coarse to detect subtle effects on developmental outcomes that are quantitatively impacted by early nourishment. Additionally, despite much having been established regarding analysis (McGuire and O’Connor Citation2020), methodological studies are still needed to refine our knowledge on the stability of milk components across lactation, days and feeds, optimal storage and thawing protocols, and methods to analyze the different components. Finally, it is important to keep in mind that a large amount of the current knowledge on human milk comes from WEIRD societies. In fact, while we do not know how this impacts human milk composition studies, there is evidence across other fields that samples derived from WEIRD societies might not be adequate for generalizations across all populations (Henrich et al. Citation2010). To develop a richer literature on the science of human milk, including other populations in this field of research is necessary. However, research designs will need to be adapted to suit different populations.
In we present a strategic plan to cultivate and advance the field of research on human milk. This plan will aid in filling relevant gaps in knowledge, hence providing the necessary solid basis, both theoretical as well as methodological, for internationally streamlined and cost-efficient future research. In time, results will contribute to designing prevention, intervention, and policy for infants and their families. In the following sections, several relevant and innovative sub-goals of this strategic plan are discussed in more detail.
Figure 4. Strategic plan to advance the field of research on human milk. Pursuing these goals and sub-goals will help fill important gaps in knowledge and, in the future, provide a solid theoretical and methodological basis for streamlined and cost-efficient research.
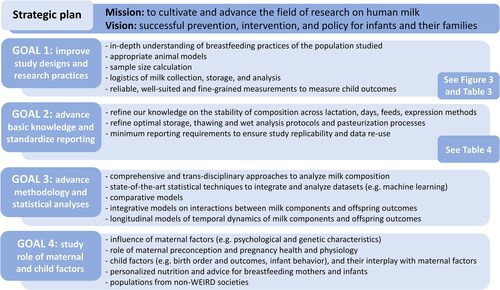
Pumping
An important question to investigate in this context is whether pumping milk impacts its composition. There are many reasons why mothers pump their milk, including breastfeeding issues, trying to increase milk supply, and because of returning to work. Previous studies have shown that women’s pumping and milk storage practices vary greatly (Felice et al. Citation2017a, Citation2017b; O’Sullivan, Geraghty, and Rasmussen Citation2017). Therefore, pumping can be hypothesized to alter milk composition. Indeed, an in-home, randomized, crossover trial of two collection methods indicated that milk collected with mothers’ own supplies yielded more Proteobacteria, including higher relative abundances of Acinetobacter and Stenotrophomonas, compared to milk collected with (sterile) hospital supplies (Reyes et al. Citation2021). Moreover, in-depth, semi-structured interviews to investigate maternal attitudes and perceptions toward pumping indicate that, although pumping fills important roles and enables continued breastfeeding success, many mothers reported it to be time-consuming, costly, and unpleasant, compared to the infant feeding at the breast (Felice et al. 2017a). Whether and how these negative attitudes and perceptions toward pumping affect milk composition remains a question for future research.
Donated milk and pasteurization
The process of pasteurization is also in need of more research. As mentioned before, in clinical settings, donated human milk is often used to replace the mother’s milk when it is unavailable. In order to provide a safe product, most centers require that donated milk is pasteurized before administration. However, while the most commonly used heat treatment, Holder Pasteurization (i.e., milk heated to 62.5 °C for 30 minutes), has a minimal effect on the macronutrient content, it can impact several functional aspects of human milk by deactivating enzymes and destroying immune cells and other factors (Ewaschuk et al. Citation2011; Peila et al. Citation2016). Promising alternatives to Holder Pasteurization are being tested in lab settings (see Pitino et al. Citation2019; Wesolowska et al. Citation2018; Wesolowska et al. Citation2019). Given the large impact of pasteurization on minor but likely important bioactive milk constituents, future efforts should focus on improving the pasteurization process or alternatively, the donor screening process, to enable better preservation of the biological functional aspects of donor human milk while ensuring safety.
Data modeling and study designs
A large portion of the work to date has been done on isolated or small groups of milk constituents. New methodologies in systems biology will assist in integrating multiple components in human milk with other biological, social and psychological inputs. We recommend taking a comprehensive and trans-disciplinary approach to analyze human milk composition (i.e., targeted and exploratory approaches to assess nutritive and non-nutritive components) and to apply statistical techniques, such as cluster analyses and machine learning, to integrate and analyze these datasets (Munblit et al. Citation2017; Shenhav and Azad Citation2022). Additionally, to be able to develop first comprehensive models unveiling causality and mechanisms, there is a dire need for deeply phenotyped longitudinal studies with a large number of repeated assessments. These studies will also help shed light on the critical windows for lactocrine programming of child cognitive and behavioral outcomes.
Comparative models
Comparative models can also shed light on causality and mechanisms underlying lactational programming. Specific constituents of milk, including proteins, carbohydrates, and lipids, are common to the primary mammalian lineages (Langer Citation2009; Oftedal Citation2012). Genomic studies indicate that there are several conserved milk proteins and other elements of the lactome in monotreme, marsupial and eutherian mammals (Lefèvre, Sharp, and Nicholas Citation2010). To further understand the complex interactions between milk components and their implications for offspring cognition and behavior, we recommend identifying and using carefully selected experimental animal models to manipulate milk components and test the impact on offspring behavior and neurodevelopment. In biology, the comparative method facilitates organization of information and recognition of unique and conserved patterns in nature (Martinez Citation2018). Milk composition varies significantly among mammalian species, and studies designed to compare milk composition are complicated by the fact that milk composition differentially changes throughout lactation (Langer Citation2009; Oftedal Citation2012; Park Citation2009; Park and Haenlein Citation2006; Sharp et al. Citation2017).
Sharp and colleagues (2014) observed that efforts to define the functions of milk bioactives, and to identify functional components of milk that affect lactocrine programming of organ development, can benefit from the use of comparative mammalian models that display extreme adaptations to lactation. In this regard, marsupials may be especially valuable. In contrast to the situation in eutherian mammals (including humans), the majority of development in marsupial young occurs postnatally and is supported exclusively by milk bioactives (Nicholas et al. Citation2019; Sharp et al. Citation2014; Sharp et al. Citation2017). Further, in contrast to eutherian milk, which remains relatively unchanged in composition throughout lactation (Langer Citation2009), milk composition in both marsupial and monotreme mammals changes systematically throughout lactation. This provides a natural model system for identification of unique and conserved roles of milk bioactives in lactocrine programming of organ development, including the central nervous system. In marsupial pouch young both the brain and spinal cord develop rapidly in offspring during the first 100 days of milk consumption (Harrison and Porter Citation1992; Saunders et al. Citation1989; Sharp et al. Citation2014). Insights into the role of lactocrine-active milk bioactives in regulation of central nervous system development will benefit markedly by studies designed to exploit comparative methodologies.
Maternal and child factors
The influence of maternal factors, especially psychological and genetic characteristics, on milk composition is still a field with limited research. Additionally, we know little on how maternal pregnancy health and physiology impact the mammary gland (e.g., placenta-to-mammary pathway) and milk composition. Moreover, investigations have shifted to an even earlier period, the pre-conception period, to better understand the role of this formative period on human health and disease (Keenan et al. Citation2018). Prior to conception, the health status of the mother, including aspects related to her diet and well-being, is an important determinant of pregnancy outcomes, maternal perinatal health, and child development. Questions on how maternal pre-conception factors are associated with breastfeeding and milk composition remain to be answered in future studies. Moreover, relatively little work has been done on human milk to understand how child factors (e.g., birth order, birth outcomes, infant temperament and behavior) contribute to variation in milk composition.
Formula and applied research
One example of an area of application is infant formula production and regulation. Infants might be fed formula for various reasons, including medical indications and adoption. Human milk research will lead to advancements in formula manufacturing, including the ability to optimize formula composition based on infant age, sex, or specific health needs. Outcomes for formula-fed infants can be better served with evidence-based formula design (Kent Citation2014). Another area for future applied research is that of personalized nutrition for breastfeeding mothers. As discussed before (Maternal diet section), what a mother eats will importantly impact her milk composition, and in turn, most probably her infant’s physical, cognitive, and behavioral development. Depending on maternal factors (e.g., mental and physical health and age), as well as on infant factors (e.g., age, sex, and physical health), personalized nutrition advice as well as personalized food supplements may be developed to optimize maternal well-being and lactation, and infant physical and mental development. Finally, an innovative area of application using the knowledge gleaned from how milk components stimulate healthy brain development in infants, is to develop future food supplements designed to prevent or treat cognitive decline in the elderly.
General conclusion
This narrative review presents a complex picture of the current state of knowledge on human milk, as well as its relevance in scientific studies. It constitutes a tantalizing window into the role of human milk and its components in the development of neurological processes and cognitive performance in infants. Several conclusions can be drawn. First, human milk is a tailored nutrition that varies over time, between persons, and with maternal and child factors. Second, there is highly compelling emerging evidence that breast milk is central in nourishing, protecting, and guiding neurological development in human infants. Third, potential effects and mechanisms of lactocrine programming on child cognition and behavior are starting to be uncovered.
The review also offers both practical and theoretical recommendations to advance this emerging area of research. Cultivating the body of research on human milk is important in the face of development of future interventions and policy for (breastfed) infants and their families. Research aimed at highlighting the cost of stressors and lack of support for human milk production and composition has the power to inspire social and political support for improvements in breastfeeding opportunities and enhancement of families’ social, physical, psychological, and environmental context.
Author contributions
CdW: Writing - Conceptualization, Review & Editing, Supervision, Project administration, Funding acquisition, AA: Writing - Original Draft, MA: Writing - Original Draft, Visualization, FB: Writing - Original Draft, LB: Writing - Original Draft, MCC: Writing - Original Draft, AMD: Writing - Original Draft, CJF: Writing - Original Draft, MG: Writing - Original Draft, KH: Writing - Original Draft, AK: Writing - Original Draft, HL: Writing - Original Draft, NHMS: Writing - Original Draft, SEM: Writing - Original Draft, SP: Writing - Original Draft, JMR: Writing - Original Draft, Visualization, CMS: Writing - Original Draft, ST: Writing - Original Draft, Visualization, JBvG: Writing - Original Draft, AZ: Writing - Original Draft, RB: Writing - Review & Editing, Visualization, Supervision, Funding acquisition.
Acknowledgements
We wish to warmly thank Irene van Kroonenburg, who played an essential role in the logistics of the ‘Lactational Programming: joining forces to optimize research on how maternal milk composition influences child cognition and behavior’ meeting and manuscript preparation.
Funding
All authors participated in the four-day hybrid meeting on ‘Lactational Programming: joining forces to optimize research on how maternal milk composition influences child cognition and behavior’ (Zeist, the Netherlands, 16-19 November 2020), which was financed by an Early Career Partnership 2020 Grant of the Royal Netherlands Academy of Arts and Sciences (awarded to Beijers). Funding sources for individual authors: Netherlands Organization for Scientific Research VICI grant (016.Vici.185.038-to de Weerth), The National Center for Advancing Translational Sciences Clinical Science and Translational Award (UL1TR001863-to Dettmer), NWO Food Cognition and Behaviour (057-14-003-to Korosi), Turku University Foundation, Maire Taponen Foundation and Finnish Psychiatry Foundation (-to Aatsinki), Polish National Science Centre OPUS grant (2015/17/B/NZ8/02436 -to Ziomkiewicz), Canadian Research Chair in Human Nutrition and Metabolism and funding from Natural Science and Engineering Council of Canada NSERC (RGPIN-2017-04746-to Field), The USDA National Institute of Food and Agriculture Hatch Project (1021411-to Slupsky), USDA NRI (2007-35203-18098 and 2013-67016-20523-to Bartol), Spanish Ministry of Science and Innovation grant (PID2019-105606RB-I00-to Rodríguez), UC San Diego Chair of Collaborative Human Milk Research, endowed by the Family Larsson-Rosenquist Foundation, Switzerland (-to Bode), Wellcome Trust (220225/Z/20/Z-to Moore), Tier 2 Canada Research Chair and Fellow in the CIFAR Humans and the Microbiome Program, Canadian Institutes of Health Research (CIHR), Research Manitoba, the Canada Foundation for Innovation, the Bill and Melinda Gates Foundation, the Manitoba Children’s Hospital Foundation, Prolacta Biosciences, Mitacs, CIFAR, and the Garfield G. Weston Foundation (-to Azad), CIHR Vanier Canada Graduate Scholarship (-to Turner), European Research Council (ERC) under the European Union’s Horizon 2020 research and innovation program (ERC starting grant, no. 639226-to Collado), Netherlands Organization for Scientific Research VENI grant (016.195.197-to Beijers). The funding sources had no further role in the writing of the manuscript, nor in the decision to submit the paper for publication.
References
- Aatsinki, A. K., L. Lahti, H. M. Uusitupa, E. Munukka, A. Keskitalo, S. Nolvi, S. O’Mahony, S. Pietilä, L. L. Elo, E. Eerola, et al. 2019. Gut microbiota composition is associated with temperament traits in infants. Brain, Behavior, and Immunity 80:849–58. doi: 10.1016/j.bbi.2019.05.035.
- Abbot, P, and J. A. Capra. 2017. What is a placental mammal anyway? eLife 6:e30994. doi: 10.7554/eLife.30994.
- Achenbach, T. M. 1991. Manual for the child behavior checklist/4-18. Burlington, VT: Department of Psychiatry, University of Vermont.
- Ahluwalia, I. B., B. Morrow, and J. Hsia. 2005. Why do women stop breastfeeding? Findings from the Pregnancy Risk Assessment and Monitoring System. Pediatrics 116 (6):1408–12. doi: 10.1542/peds.2005-0013.
- Ak, J., P. B. Lakshmanagowda, P. G C M, and J. Goturu. 2015. Impact of music therapy on breast milk secretion in mothers of premature newborns. Journal of Clinical and Diagnostic Research: JCDR 9 (4):CC04–CC6. doi: 10.7860/JCDR/2015/11642.5776.
- Akers, R. M. 2002. Lactation and the mammary gland. New York: John Wiley & Sons.
- Allen, L. H, and D. Hampel. 2020. Human milk as the first source of micronutrients. Nestle Nutrition Institute Workshop Series 93:67–76. doi: 10.1159/000503359.
- Allen-Blevins, C. R., D. A. Sela, and K. Hinde. 2015. Milk bioactives may manipulate microbes to mediate parent-offspring conflict. Evolution, Medicine, and Public Health 2015 (1):106–21. doi: 10.1093/emph/eov007.
- Anderson, J. W., B. M. Johnstone, and D. T. Remley. 1999. Breast-feeding and cognitive development: A meta-analysis. The American Journal of Clinical Nutrition 70 (4):525–35. doi: 10.1093/ajcn/70.4.525.
- Al-Saleh, I. 2021. Health risk assessment of trace metals through breast milk consumption in Saudi Arabia. Biological Trace Element Research 199 (12):4535–45. doi: 10.1007/s12011-021-02607-3.
- Al-Saleh, I., S. Al-Mohawes, R. Al-Rouqi, and R. Elkhatib. 2019. Selenium status in lactating mothers-infants and its potential protective role against the neurotoxicity of methylmercury, lead, manganese, and DDT. Environmental Research 176:108562. doi: 10.1016/j.envres.2019.108562.
- Al-Saleh, I., M. Nester, M. Abduljabbar, R. Al-Rouqi, C. Eltabache, T. Al-Rajudi, and R. Elkhatib. 2016. Mercury (Hg) exposure and its effects on Saudi breastfed infant’s neurodevelopment. International Journal of Hygiene and Environmental Health 219 (1):129–41. doi: 10.1016/j.ijheh.2015.10.002.
- Amaral, Y., L. Silva, F. Soares, D. Marano, S. Nehab, A. Abranches, A. C. Costa, and M. E. Moreira. 2021. What are the maternal factors that potentially intervenes in the nutritional composition of human milk? Nutrients 13 (5):1587. doi: 10.3390/nu13051587.
- Angelucci, L., F. R. Patacchioli, S. Scaccianoce, A. Di Sciullo, A. Cardillo, and S. Maccari. 1985. A model for later-life effects of perinatal drug exposure: Maternal hormone mediation. Neurobehavioral Toxicology and Teratology 7 (5):511–7.
- Angulo, J., L. M. Gómez, L. Mahecha, E. Mejía, J. Henao, and C. Mesa. 2015. Calf’s sex, parity and the hour of harvest after calving affect colostrum quality of dairy cows grazing under high tropical conditions. Tropical Animal Health and Production 47 (4):699–705. doi: 10.1007/s11250-015-0781-z.
- Aparicio, M., P. D. Browne, C. Hechler, R. Beijers, J. M. Rodríguez, C. de Weerth, and L. Fernández. 2020. Human milk cortisol and immune factors over the first three postnatal months: Relations to maternal psychosocial distress. PloS One 15 (5):e0233554. doi: 10.1371/journal.pone.0233554.
- Arias-Borrego, A., B. Callejón-Leblic, M. Calatayud, J. L. Gómez-Ariza, M. C. Collado, and T. García-Barrera. 2019. Insights into cancer and neurodegenerative diseases through selenoproteins and the connection with gut microbiota - Current analytical methodologies. Expert Review of Proteomics 16 (10):805–14. doi: 10.1080/14789450.2019.1664292.
- Arshad, R., N. Karim, and J. Ara Hasan. 2014. Effects of insulin on placental, fetal and maternal outcomes in gestational diabetes mellitus. Pakistan Journal of Medical Sciences 30 (2):240–4. doi: 10.12669/pjms.302.4396.
- Asbury, M. R., J. Butcher, J. K. Copeland, S. Unger, N. Bando, E. M. Comelli, V. Forte, A. Kiss, L. LeMay-Nedjelski, P. M. Sherman, et al. 2020. Mothers of preterm infants have individualized breast milk microbiota that changes temporally based on maternal characteristics. Cell Host & Microbe 28 (5):669–82.e4. doi: 10.1016/j.chom.2020.08.001.
- Austin, S., C. A. De Castro, N. Sprenger, A. Binia, M. Affolter, C. L. Garcia-Rodenas, L. Beauport, J. F. Tolsa, and C. J. Fischer Fumeaux. 2019. Human milk oligosaccharides in the milk of mothers delivering term versus preterm infants. Nutrients 11 (6):1282. doi: 10.3390/nu11061282.
- Aydın, M. Ş., E. N. Yiğit, E. Vatandaşlar, E. Erdoğan, and G. Öztürk. 2018. Transfer and integration of breast milk stem cells to the brain of suckling pups. Scientific Reports 8 (1):14289. doi: 10.1038/s41598-018-32715-5.
- Azad, M. B., B. Robertson, F. Atakora, A. B. Becker, P. Subbarao, T. J. Moraes, P. J. Mandhane, S. E. Turvey, D. L. Lefebvre, M. R. Sears, et al. 2018. Human milk oligosaccharide concentrations are associated with multiple fixed and modifiable maternal characteristics, environmental factors, and feeding practices. The Journal of Nutrition 148 (11):1733–42. doi: 10.1093/jn/nxy175.
- Azhari, A., F. Azizan, and G. Esposito. 2019. A systematic review of gut-immune-brain mechanisms in Autism Spectrum Disorder. Developmental Psychobiology 61 (5):752–71. doi: 10.1002/dev.21803.
- Bachour, P., R. Yafawi, F. Jaber, E. Choueiri, and Z. Abdel-Razzak. 2012. Effects of smoking, mother’s age, body mass index, and parity number on lipid, protein, and secretory immunoglobulin A concentrations of human milk. Breastfeeding Medicine 7 (3):179–88. doi: 10.1089/bfm.2011.0038.
- Bäckhed, F., J. Roswall, Y. Peng, Q. Feng, H. Jia, P. Kovatcheva-Datchary, Y. Li, Y. Xia, H. Xie, H. Zhong, et al. 2015. Dynamics and stabilization of the human gut microbiome during the first year of life. Cell Host & Microbe 17 (5):690–703. doi: 10.1016/j.chom.2015.04.004.
- Bahreynian, M., A. Feizi, and R. Kelishadi. 2020. Is fatty acid composition of breast milk different in various populations? A systematic review and meta-analysis. International Journal of Food Sciences and Nutrition 71 (8):909–20. doi: 10.1080/09637486.2020.1746958.
- Ballard, O, and A. L. Morrow. 2013. Human milk composition: Nutrients and bioactive factors. Pediatric Clinics of North America 60 (1):49–74. doi: 10.1016/j.pcl.2012.10.002.
- Barbone, F., V. Rosolen, M. Mariuz, M. Parpinel, A. Casetta, F. Sammartano, L. Ronfani, L. Vecchi Brumatti, M. Bin, L. Castriotta, et al. 2019. Prenatal mercury exposure and child neurodevelopment outcomes at 18 months: Results from the Mediterranean PHIME cohort. International Journal of Hygiene and Environmental Health 222 (1):9–21. doi: 10.1016/j.ijheh.2018.07.011.
- Bartol, F. F., A. A. Wiley, and C. A. Bagnell. 2008. Epigenetic programming of porcine endometrial function and the lactocrine hypothesis. Reproduction in Domestic Animals 43 (Suppl 2): 273–9. doi: 10.1111/j.1439-0531.2008.01174.x.
- Bartol, F. F., A. A. Wiley, A. F. George, D. J. Miller, and C. A. Bagnell. 2017. Physiology and endocrinology symposium: Postnatal reproductive development and the lactocrine hypothesis. Journal of Animal Science 95 (5):2200–10. doi: 10.2527/jas.2016.1144.
- Basak, S., S. Vilasagaram, and A. K. Duttaroy. 2020. Maternal dietary deficiency of n-3 fatty acids affects metabolic and epigenetic phenotypes of the developing fetus. Prostaglandins, Leukotrienes, and Essential Fatty Acids 158:102109. doi: 10.1016/j.plefa.2020.102109.
- Bauer, J. A., V. Fruh, C. G. Howe, R. F. White, and B. C. Henn. 2020. Associations of metals and neurodevelopment: A review of recent evidence on susceptibility factors. Current Epidemiology Reports 7 (4):237–62. doi: 10.1007/s40471-020-00249-y.
- Bautista, C. J., R. J. Bautista, S. Montaño, L. A. Reyes-Castro, O. N. Rodriguez-Peña, C. A. Ibáñez, P. W. Nathanielsz, and E. Zambrano. 2019. Effects of maternal protein restriction during pregnancy and lactation on milk composition and offspring development. The British Journal of Nutrition 122 (2):141–51. doi: 10.1017/S0007114519001120.
- Bayley, N. 1969. Manual for the Bayley scales of infant development. New York: Psychological Corporation.
- Benítez-Páez, A., M. Olivares, H. Szajewska, M. Pieścik-Lech, I. Polanco, G. Castillejo, M. Nuñez, C. Ribes-Koninckx, I. R. Korponay-Szabó, S. Koletzko, et al. 2020. Breast-milk microbiota linked to celiac disease development in children: A pilot study from the PreventCD cohort. Frontiers in Microbiology 11:1335. doi: 10.3389/fmicb.2020.01335.
- Benjamin, S., E. Masai, N. Kamimura, K. Takahashi, R. Anderson, and P. A. Faisal. 2017. Phthalates impact human health: Epidemiological evidences and plausible mechanism of action. Journal of Hazardous Materials 340:360–83. doi: 10.1016/j.jhazmat.2017.06.036.
- Benjamin Neelon, S. E., M. Stroo, M. Mayhew, J. Maselko, and C. Hoyo. 2015. Correlation between maternal and infant cortisol varies by breastfeeding status. Infant Behavior & Development 40:252–8. doi: 10.1016/j.infbeh.2015.06.005.
- Berger, P. K., J. F. Plows, R. B. Jones, T. L. Alderete, C. Yonemitsu, M. Poulsen, J. H. Ryoo, B. S. Peterson, L. Bode, and M. I. Goran. 2020. Human milk oligosaccharide 2’-fucosyllactose links feedings at 1 month to cognitive development at 24 months in infants of normal and overweight mothers. PloS One 15 (2):e0228323. doi: 10.1371/journal.pone.0228323.
- Bernstein, R. M, and K. Hinde. 2016. Bioactive factors in milk across lactation: Maternal effects and influence on infant growth in rhesus macaques (Macaca mulatta). American Journal of Primatology 78 (8):838–50. doi: 10.1002/ajp.22544.
- Biagi, E., S. Quercia, A. Aceti, I. Beghetti, S. Rampelli, S. Turroni, G. Faldella, M. Candela, P. Brigidi, and L. Corvaglia. 2017. The bacterial ecosystem of mother’s milk and infant’s mouth and gut. Frontiers in Microbiology 8:1214. doi: 10.3389/fmicb.2017.01214.
- Bielicki, J., R. Huch, and U. von Mandach. 2004. Time-course of leptin levels in term and preterm human milk. European Journal of Endocrinology 151 (2):271–6. doi: 10.1530/eje.0.1510271.
- Bienias, K., A. Fiedorowicz, A. Sadowska, S. Prokopiuk, and H. Car. 2016. Regulation of sphingomyelin metabolism. Pharmacological Reports: PR 68 (3):570–81. doi: 10.1016/j.pharep.2015.12.008.
- Bilbo, S. D. 2010. Early-life infection is a vulnerability factor for aging-related glial alterations and cognitive decline. Neurobiology of Learning and Memory 94 (1):57–64. doi: 10.1016/j.nlm.2010.04.001.
- Bilbo, S. D, and J. M. Schwarz. 2009. Early-life programming of later-life brain and behavior: A critical role for the immune system. Frontiers in Behavioral Neuroscience 3:14. doi: 10.3389/neuro.08.014.2009.
- Bilbo, S. D, and J. M. Schwarz. 2012. The immune system and developmental programming of brain and behavior. Frontiers in Neuroendocrinology 33 (3):267–86. doi: 10.1016/j.yfrne.2012.08.006.
- Bishara, R., M. S. Dunn, S. E. Merko, and P. Darling. 2009. Volume of foremilk, hindmilk, and total milk produced by mothers of very preterm infants born at less than 28 weeks of gestation. Journal of Human Lactation 25 (3):272–9. doi: 10.1177/0890334409334606.
- Blum, J. W, and C. R. Baumrucker. 2008. Insulin-like growth factors (IGFs), IGF binding proteins, and other endocrine factors in milk: Role in the newborn. Advances in Experimental Medicine and Biology 606:397–422. doi: 10.1007/978-0-387-74087-4_16.
- Bode, L. 2012. Human milk oligosaccharides: Every baby needs a sugar mama. Glycobiology 22 (9):1147–62. doi: 10.1093/glycob/cws074.
- Bode, L, and E. Jantscher-Krenn. 2012. Structure-function relationships of human milk oligosaccharides. Advances in Nutrition 3 (3):383S–91S. doi: 10.3945/an.111.001404.
- Bode, L., A. S. Raman, S. H. Murch, N. C. Rollins, and J. I. Gordon. 2020. Understanding the mother-breastmilk-infant triad. Science (New York, N.Y.) 367 (6482):1070–2. doi: 10.1126/science.aaw6147.
- Boix-Amorós, A., F. Puente-Sánchez, E. du Toit, K. M. Linderborg, Y. Zhang, B. Yang, S. Salminen, E. Isolauri, J. Tamames, A. Mira, et al. 2019. Mycobiome profiles in breast milk from healthy women depend on mode of delivery, geographic location, and interaction with bacteria. Applied and Environmental Microbiology 85 (9):e02994–18. doi: 10.1128/AEM.02994-18.
- Bokor, S., B. Koletzko, and T. Decsi. 2007. Systematic review of fatty acid composition of human milk from mothers of preterm compared to full-term infants. Annals of Nutrition & Metabolism 51 (6):550–6. doi: 10.1159/000114209.
- Bornstein, M. H, and M. D. Sigman. 1986. Continuity in mental development from infancy. Child Development 57 (2):251–74. doi: 10.1111/j.1467-8624.1986.tb00025.x.
- Bouret, S. G., S. J. Draper, and R. B. Simerly. 2004. Trophic action of leptin on hypothalamic neurons that regulate feeding. Science (New York, N.Y.) 304 (5667):108–10. doi: 10.1126/science.1095004.
- Bourlieu, C, and M. C. Michalski. 2015. Structure-function relationship of the milk fat globule. Current Opinion in Clinical Nutrition and Metabolic Care 18 (2):118–27. doi: 10.1097/MCO.0000000000000138.
- Bravi, F., F. Wiens, A. Decarli, A. Dal Pont, C. Agostoni, and M. Ferraroni. 2016. Impact of maternal nutrition on breast-milk composition: A systematic review. The American Journal of Clinical Nutrition 104 (3):646–62. doi: 10.3945/ajcn.115.120881.
- Bravi, F., M. Di Maso, S. Eussen, C. Agostoni, G. Salvatori, C. Profeti, P. Tonetto, P. A. Quitadamo, I. Kazmierska, E. Vacca, et al. 2021. Dietary patterns of breastfeeding mothers and human milk composition: Data from the Italian MEDIDIET study. Nutrients 3 (5):1722. doi: 10.3390/nu13051722.
- Brazelton, T. B, and J. K. Nugent. 2011. The neonatal behavioral assessment scale. Cambridge: Mac Keith Press.
- Breakey, A. A., K. Hinde, C. R. Valeggia, A. Sinofsky, and P. T. Ellison. 2015. Illness in breastfeeding infants relates to concentration of lactoferrin and secretory Immunoglobulin A in mother’s milk. Evolution, Medicine, and Public Health 2015 (1):21–31. doi: 10.1093/emph/eov002.
- Brink, L. R, and B. Lönnerdal. 2020. Milk fat globule membrane: The role of its various components in infant health and development. The Journal of Nutritional Biochemistry 85:108465. doi: 10.1016/j.jnutbio.2020.108465.
- Browne, P. D., M. Aparicio, C. Alba, C. Hechler, R. Beijers, J. M. Rodríguez, L. Fernández, and C. de Weerth. 2019. Human milk microbiome and maternal postnatal psychosocial distress. Frontiers in Microbiology 10:2333. doi: 10.3389/fmicb.2019.02333.
- Brunner, S., D. Schmid, K. Zang, D. Much, B. Knoeferl, J. Kratzsch, U. Amann-Gassner, B. L. Bader, and H. Hauner. 2015. Breast milk leptin and adiponectin in relation to infant body composition up to 2 years. Pediatric Obesity 10 (1):67–73. doi: 10.1111/j.2047-6310.2014.222.x.
- Bryan, D. L., P. H. Hart, K. D. Forsyth, and R. A. Gibson. 2007. Immunomodulatory constituents of human milk change in response to infant bronchiolitis. Pediatric Allergy and Immunology 18 (6):495–502. doi: 10.1111/j.1399-3038.2007.00565.x.
- Buffington, S. A., G. V. Di Prisco, T. A. Auchtung, N. J. Ajami, J. F. Petrosino, and M. Costa-Mattioli. 2016. Microbial reconstitution reverses maternal diet-induced social and synaptic deficits in offspring. Cell 165 (7):1762–75. doi: 10.1016/j.cell.2016.06.001.
- Burianova, I., J. Bronsky, M. Pavlikova, J. Janota, and J. Maly. 2019. Maternal body mass index, parity and smoking are associated with human milk macronutrient content after preterm delivery. Early Human Development 137:104832. doi: 10.1016/j.earlhumdev.2019.104832.
- Butte, N. F., C. Garza, C. A. Johnson, E. O. Smith, and B. L. Nichols. 1984. Longitudinal changes in milk composition of mothers delivering preterm and term infants. Early Human Development 9 (2):153–62. doi: 10.1016/0378-3782(84)90096-3.
- Butts, C. A., D. I. Hedderley, T. D. Herath, G. Paturi, S. Glyn-Jones, F. Wiens, B. Stahl, and P. Gopal. 2018. Human milk composition and dietary intakes of breastfeeding women of different ethnicity from the Manawatu-Wanganui region of New Zealand. Nutrients 10 (9):1231. doi: 10.3390/nu10091231.
- Cabinian, A., D. Sinsimer, M. Tang, O. Zumba, H. Mehta, A. Toma, D. Sant’Angelo, Y. Laouar, and A. Laouar. 2016. Transfer of maternal immune cells by breastfeeding: Maternal cytotoxic T lymphocytes present in breast milk localize in the peyer’s patches of the nursed infant. Plos One 11 (6):e0156762. doi: 10.1371/journal.pone.0156762.
- Cabrera-Rubio, R., M. C. Collado, K. Laitinen, S. Salminen, E. Isolauri, and A. Mira. 2012. The human milk microbiome changes over lactation and is shaped by maternal weight and mode of delivery. The American Journal of Clinical Nutrition 96 (3):544–51. doi: 10.3945/ajcn.112.037382.
- Cao, Y., S. D. Rao, T. M. Phillips, D. M. Umbach, J. C. Bernbaum, J. I. Archer, and W. J. Rogan. 2009. Are breast-fed infants more resilient? Feeding method and cortisol in infants. The Journal of Pediatrics 154 (3):452–4. doi: 10.1016/j.jpeds.2008.09.025.
- Cardoso, O. O., F. C. Julião, R. I. Alves, A. R. Baena, I. G. Díez, M. N. Suzuki, B. S. Celere, M. Nadal, J. L. Domingo, and S. I. Segura-Muñoz. 2014. Concentration profiles of metals in breast milk, drinking water, and soil: Relationship between matrices. Biological Trace Element Research 160 (1):116–22. doi: 10.1007/s12011-014-0030-8.
- Cardoso-Moreira, M., J. Halbert, D. Valloton, B. Velten, C. Chen, Y. Shao, A. Liechti, K. Ascenção, C. Rummel, S. Ovchinnikova, et al. 2019. Gene expression across mammalian organ development. Nature 571 (7766):505–9. doi: 10.1038/s41586-019-1338-5.
- Carlson, A. L., K. Xia, M. A. Azcarate-Peril, B. D. Goldman, M. Ahn, M. A. Styner, A. L. Thompson, X. Geng, J. H. Gilmore, and R. C. Knickmeyer. 2018. Infant gut microbiome associated with cognitive development. Biological Psychiatry 83 (2):148–59. doi: 10.1016/j.biopsych.2017.06.021.
- Carlson, A. L., K. Xia, M. A. Azcarate-Peril, S. P. Rosin, J. P. Fine, W. Mu, J. B. Zopp, M. C. Kimmel, M. A. Styner, A. L. Thompson, et al. 2021. Infant gut microbiome composition is associated with non-social fear behavior in a pilot study. Nature Communications 12 (1):3294. doi: 10.1038/s41467-021-23281-y.
- Castriotta, L., V. Rosolen, A. Biggeri, L. Ronfani, D. Catelan, M. Mariuz, M. Bin, L. V. Brumatti, L. Horvat, and F. Barbone. 2020. The role of mercury, selenium and the Se-Hg antagonism on cognitive neurodevelopment: A 40-month follow-up of the Italian mother-child PHIME cohort. International Journal of Hygiene and Environmental Health 230 (113604):113604. doi: 10.1016/j.ijheh.2020.113604.
- Catalani, A., G. S. Alemà, C. Cinque, A. R. Zuena, and P. Casolini. 2011. Maternal corticosterone effects on hypothalamus-pituitary-adrenal axis regulation and behavior of the offspring in rodents. Neuroscience and Biobehavioral Reviews 35 (7):1502–17. doi: 10.1016/j.neubiorev.2010.10.017.
- Cerdó, T., L. García-Valdés, S. Altmäe, A. Ruíz, A. Suárez, and C. Campoy. 2016. Role of microbiota function during early life on child’s neurodevelopment. Trends in Food Science & Technology 57:273–88. doi: 10.1016/j.tifs.2016.08.007.
- Cheatham, C. L., A. S. Nerhammer, M. Asserhøj, K. F. Michaelsen, and L. Lauritzen. 2011. Fish oil supplementation during lactation: Effects on cognition and behavior at 7 years of age. Lipids 46 (7):637–45. doi: 10.1007/s11745-011-3557-x.
- Cheatham, C. L, and K. W. Sheppard. 2015. Synergistic effects of human milk nutrients in the support of infant recognition memory: An observational study. Nutrients 7 (11):9079–95. doi: 10.3390/nu7115452.
- Chen, Y., Z. Zheng, X. Zhu, Y. Shi, D. Tian, F. Zhao, N. Liu, P. S. Hüppi, F. A. Troy, 2nd, and B. Wang. 2015. Lactoferrin promotes early neurodevelopment and cognition in postnatal piglets by upregulating the BDNF signaling pathway and polysialylation. Molecular Neurobiology 52 (1):256–69. doi: 10.1007/s12035-014-8856-9.
- Cheverud, J. M. 1981. Epiphyseal union and dental eruption Macaca mulatta. American Journal of Physical Anthropology 56 (2):157–67. doi: 10.1002/ajpa.1330560207.
- Cho, S., Z. Zhu, T. Li, K. Baluyot, B. R. Howell, H. C. Hazlett, J. T. Elison, J. Hauser, N. Sprenger, D. Wu, et al. 2021. Human milk 3’-Sialyllactose is positively associated with language development during infancy. The American Journal of Clinical Nutrition 114 (2):588–97. doi: 10.1093/ajcn/nqab103.
- Chowdhury, R., B. Sinha, M. J. Sankar, S. Taneja, N. Bhandari, N. Rollins, R. Bahl, and J. Martines. 2015. Breastfeeding and maternal health outcomes: A systematic review and meta-analysis. Acta Paediatrica (Oslo, Norway: 1992) 104 (467):96–113. doi: 10.1111/apa.13102.
- Christian, P., E. R. Smith, S. E. Lee, A. J. Vargas, A. A. Bremer, and D. J. Raiten. 2021. The need to study human milk as a biological system. The American Journal of Clinical Nutrition 113 (5):1063–72. doi: 10.1093/ajcn/nqab075.
- Clutton-Brock, T. H. 1991. The evolution of parental care. Vol. 64. Princeton, NJ: Princeton University Press.
- Collado, M. C., K. Laitinen, S. Salminen, and E. Isolauri. 2012. Maternal weight and excessive weight gain during pregnancy modify the immunomodulatory potential of breast milk. Pediatric Research 72 (1):77–85. doi: 10.1038/pr.2012.42.
- Collado, M. C., M. Santaella, L. Mira-Pascual, E. Martínez-Arias, P. Khodayar-Pardo, G. Ros, and C. Martínez-Costa. 2015. Longitudinal study of cytokine expression, lipid profile and neuronal growth factors in human breast milk from term and preterm deliveries. Nutrients 7 (10):8577–91. doi: 10.3390/nu7105415.
- Colombo, J., D. J. Shaddy, K. Gustafson, B. J. Gajewski, J. M. Thodosoff, E. Kerling, and S. E. Carlson. 2019. The Kansas University DHA Outcomes Study (KUDOS) clinical trial: Long-term behavioral follow-up of the effects of prenatal DHA supplementation. The American Journal of Clinical Nutrition 109 (5):1380–92. doi: 10.1093/ajcn/nqz018.
- Committee on Nutrition, Section on Breastfeeding, and Committee on Fetus and Newborn. 2017. Donor human milk for the high-risk infant: Preparation, safety, and usage options in the United States. Pediatrics 139 (1):e20163440. doi: 10.1542/peds.2016-3440.
- Cooke, L, and A. Fildes. 2011. The impact of flavour exposure in utero and during milk feeding on food acceptance at weaning and beyond. Appetite 57 (3):808–11. doi: 10.1016/j.appet.2011.05.317.
- Cregan, M. D., Y. Fan, A. Appelbee, M. L. Brown, B. Klopcic, J. Koppen, L. R. Mitoulas, K. M. Piper, M. A. Choolani, Y. S. Chong, et al. 2007. Identification of nestin-positive putative mammary stem cells in human breastmilk. Cell and Tissue Research 329 (1):129–36. doi: 10.1007/s00441-007-0390-x.
- Cryan, J., F. O’Riordan, K. J. Cowan, C. Sandhu, K. V. Bastiaanssen, T. Boehme, M. Codagnone, M. G. Cussotto, S. Fulling, C. Golubeva, et al. 2019. The microbiota-gut-brain axis. Physiological Reviews 99 (4):1877–2013. doi: 10.1152/physrev.00018.2018.
- Dabas, S., P. Joshi, R. Agarwal, R. K. Yadav, and G. Kachhawa. 2019. Impact of audio assisted relaxation technique on stress, anxiety and milk output among postpartum mothers of hospitalized neonates: A randomized controlled trial. Journal of Neonatal Nursing 25 (4):200–4. doi: 10.1016/j.jnn.2019.03.004.
- Dai, X., T. Yuan, X. Zhang, Q. Zhou, H. Bi, R. Yu, W. Wei, and X. Wang. 2020. Short-chain fatty acid (SCFA) and medium-chain fatty acid (MCFA) concentrations in human milk consumed by infants born at different gestational ages and the variations in concentration during lactation stages. Food & Function 11 (2):1869–80. doi: 10.1039/C9FO02595B.
- Davies, S. R., A. R. J. Field, T. Andersen, and C. Pestell. 2011. The ecological validity of the Rey-Osterrieth complex figure: Predicting everyday problems in children with neuropsychological disorders. Journal of Clinical and Experimental Neuropsychology 33 (7):820–31. doi: 10.1080/13803395.2011.574608.
- de Melo, M., D. E. Pereira, M. M. Sousa, D. Medeiros, L. Lemos, M. S. Madruga, N. M. Santos, M. de Oliveira, C. C. de Menezes, and J. Soares. 2017. Maternal intake of cashew nuts accelerates reflex maturation and facilitates memory in the offspring. International Journal of Developmental Neuroscience 61 (1):58–67. doi: 10.1016/j.ijdevneu.2017.06.006.
- de Melo, M., D. E. Pereira, R. L. Moura, E. B. da Silva, F. de Melo, C. Dias, M. Silva, M. de Oliveira, V. B. Viera, M. Pintado, et al. 2019. Maternal supplementation with avocado (Persea Americana Mill.) pulp and oil alters reflex maturation, physical development, and offspring memory in rats. Frontiers in Neuroscience 13:9. doi: 10.3389/fnins.2019.00009.
- de Weerth, C. 2017. Do bacteria shape our development? Crosstalk between intestinal microbiota and HPA axis. Neuroscience and Biobehavioral Reviews 83:458–71. doi: 10.1016/j.neubiorev.2017.09.016.
- de Weerth, C., S. Fuentes, and W. M. de Vos. 2013a. Crying in infants: On the possible role of intestinal microbiota in the development of colic. Gut Microbes 4 (5):416–21. doi: 10.4161/gmic.26041.
- de Weerth, C., S. Fuentes, P. Puylaert, and W. M. de Vos. 2013b. Intestinal microbiota of infants with colic: Development and specific signatures. Pediatrics 131 (2):e550–e558. doi: 10.1542/peds.2012-1449.
- Demmelmair, H, and B. Koletzko. 2018. Lipids in human milk. Best Practice & Research. Clinical Endocrinology & Metabolism 32 (1):57–68. doi: 10.1016/j.beem.2017.11.002.
- Deoni, S., D. Dean, S. Joelson, J. O’Regan, and N. Schneider. 2018. Early nutrition influences developmental myelination and cognition in infants and young children. NeuroImage 178:649–59. doi: 10.1016/j.neuroimage.2017.12.056.
- Deoni, S., D. Dean, I. Piryatinsky, J. O’Muircheartaigh, N. Waskiewicz, K. Lehman, M. Han, and H. Dirks. 2013. Breastfeeding and early white matter development: A cross-sectional study. NeuroImage 82:77–86. doi: 10.1016/j.neuroimage.2013.05.090.
- Desoye, G, and S. Hauguel-de Mouzon. 2007. The human placenta in gestational diabetes mellitus. The insulin and cytokine network. Diabetes Care 30 (Suppl 2):S120–S126. doi: 10.2337/dc07-s203.
- Dettmer, A. M., A. M. Murphy, D. Guitarra, E. Slonecker, S. J. Suomi, K. L. Rosenberg, M. A. Novak, J. S. Meyer, and K. Hinde. 2018. Cortisol in neonatal mother’s milk predicts later infant social and cognitive functioning in rhesus monkeys. Child Development 89 (2):525–38. doi: 10.1111/cdev.12783.
- Díaz, J. R., M. Alejandro, G. Romero, F. Moya, and C. Peris. 2013. Variation in milk cortisol during lactation in Murciano-Granadina goats. Journal of Dairy Science 96 (2):897–905. doi: 10.3168/jds.2012-5614.
- Dingess, K. A., M. de Waard, S. Boeren, J. Vervoort, T. T. Lambers, J. B. van Goudoever, and K. Hettinga. 2017. Human milk peptides differentiate between the preterm and term infant and across varying lactational stages. Food & Function 8 (10):3769–82. doi: 10.1039/C7FO00539C.
- Docq, S., M. Spoelder, W. Wang, and J. R. Homberg. 2020. The protective and long-lasting effects of human milk oligosaccharides on cognition in mammals. Nutrients 12 (11):3572. doi: 10.3390/nu12113572.
- Donovan, S. M, and J. Odle. 1994. Growth factors in milk as mediators of infant development. Annual Review of Nutrition 14:147–67. doi: 10.1146/annurev.nu.14.070194.001051.
- Dórea, J. G. 2002. Selenium and breast-feeding. The British Journal of Nutrition 88 (5):443–61. doi: 10.1079/BJN2002692.
- Dórea, J. G. 2019. Multiple low-level exposures: Hg interactions with co-occurring neurotoxic substances in early life. Biochimica et Biophysica Acta. General Subjects 1863 (12):129243. doi: 10.1016/j.bbagen.2018.10.015.
- Dórea, J. G. 2021. Neurodevelopment and exposure to neurotoxic metal(loid)s in environments polluted by mining, metal scrapping and smelters, and e-waste recycling in low and middle-income countries. Environmental Research 197:111124. doi: 10.1016/j.envres.2021.111124.
- Drane, D. L, and J. A. Logemann. 2000. A critical evaluation of the evidence on the association between type of infant feeding and cognitive development. Paediatric and Perinatal Epidemiology 14 (4):349–56. doi: 10.1046/j.1365-3016.2000.00301.x.
- Dritsakou, K., G. Liosis, G. Valsami, E. Polychronopoulos, and M. Skouroliakou. 2017. The impact of maternal- and neonatal-associated factors on human milk’s macronutrients and energy. The Journal of Maternal-Fetal & Neonatal Medicine: the official journal of the European Association of Perinatal Medicine, the Federation of Asia and Oceania Perinatal Societies, the International Society of Perinatal Obstetricians 30 (11):1302–8. doi: 10.1080/14767058.2016.1212329.
- Dror, D. K, and L. H. Allen. 2018. Overview of nutrients in human milk. Advances in Nutrition (Bethesda, Md.) 9 (suppl_1):278S–94S. doi: 10.1093/advances/nmy022.
- Duranti, S., L. Ruiz, G. A. Lugli, H. Tames, C. Milani, L. Mancabelli, W. Mancino, G. Longhi, L. Carnevali, A. Sgoifo, et al. 2020. Bifidobacterium adolescentis as a key member of the human gut microbiota in the production of GABA. Scientific Reports 10 (1):14112. doi: 10.1038/s41598-020-70986-z.
- Dzidic, M., M. C. Collado, T. Abrahamsson, A. Artacho, M. Stensson, M. C. Jenmalm, and A. Mira. 2018. Oral microbiome development during childhood: an ecological succession influenced by postnatal factors and associated with tooth decay. The ISME Journal 12 (9):2292–306. doi: 10.1038/s41396-018-0204-z.
- Engelking, L. R. 2015. Chapter 59 – Sphingolipids. In Textbook of Veterinary Physiological Chemistry, ed. L. R. Engelking, 378–83. London: Academic Press.
- Engler, A. C., A. Hadash, N. Shehadeh, and G. Pillar. 2012. Breastfeeding may improve nocturnal sleep and reduce infantile colic: potential role of breast milk melatonin. European Journal of Pediatrics 171 (4):729–32. doi: 10.1007/s00431-011-1659-3.
- Erdman, S. E, and T. Poutahidis. 2014. Probiotic ‘glow of health’: it’s more than skin deep. Beneficial Microbes 5 (2):109–19. doi: 10.3920/BM2013.0042.
- Estienne, M. J., A. F. Harper, C. R. Barb, and M. J. Azain. 2000. Concentrations of leptin in serum and milk collected from lactating sows differing in body condition. Domestic Animal Endocrinology 19 (4):275–80. https://doi.org/10.1016/s0739-7240. doi: 10.1016/S0739-7240(00)00082-5.
- Ettinger, A. S., A. Roy, C. J. Amarasiriwardena, D. Smith, N. Lupoli, A. Mercado-García, H. Lamadrid-Figueroa, M. M. Tellez-Rojo, H. Hu, and M. Hernández-Avila. 2014. Maternal blood, plasma, and breast milk lead: lactational transfer and contribution to infant exposure. Environmental Health Perspectives 122 (1):87–92. doi: 10.1289/ehp.1307187.
- Ewaschuk, J. B., S. Unger, D. L. O’Connor, D. Stone, S. Harvey, M. T. Clandinin, and C. J. Field. 2011. Effect of pasteurization on selected immune components of donated human breast milk. Journal of Perinatology 31 (9):593–8. doi: 10.1038/jp.2010.209.
- Fagan, J. F., C. R. Holland, and K. Wheeler. 2007. The prediction, from infancy, of adult IQ and achievement. Intelligence 35 (3):225–31. doi: 10.1016/j.intell.2006.07.007.
- Fagan, J. F, and S. K. McGrath. 1981. Infant recognition memory and later intelligence. Intelligence 5 (2):121–30. doi: 10.1016/0160-2896(81)90002-7.
- Fan, Y., Y. S. Chong, M. A. Choolani, M. D. Cregan, and J. K. Chan. 2010. Unravelling the mystery of stem/progenitor cells in human breast milk. PloS One 5 (12):e14421. doi: 10.1371/journal.pone.0014421.
- Feher, S. D., L. R. Berger, J. D. Johnson, and J. B. Wilde. 1989. Increasing breast milk production for premature infants with a relaxation/imagery audiotape. Pediatrics 83 (1):57–60.
- Felice, J. P., S. R. Geraghty, C. W. Quaglieri, R. Yamada, A. J. Wong, and K. M. Rasmussen. 2017a. Breastfeeding” without baby: a longitudinal, qualitative investigation of how mothers perceive, feel about, and practice human milk expression. Maternal & Child Nutrition 13 (3):e12426. doi: 10.1111/mcn.12426.
- Felice, J. P., S. R. Geraghty, C. W. Quaglieri, R. Yamada, A. J. Wong, and K. M. Rasmussen. 2017b. Breastfeeding” but not at the breast: Mothers’ descriptions of providing pumped human milk to their infants via other containers and caregivers. Maternal & Child Nutrition 13 (3):e12425. doi: 10.1111/mcn.12425.
- Fernandes, F. S., A. S. de Souza, d. do Carmo, and G. T. Boaventura. 2011. Maternal intake of flaxseed-based diet (Linum usitatissimum) on hippocampus fatty acid profile: implications for growth, locomotor activity and spatial memory. Nutrition (Burbank, Los Angeles County, Calif.) 27 (10):1040–7. doi: 10.1016/j.nut.2010.11.001.
- Fernández, L., P. S. Pannaraj, S. Rautava, and J. M. Rodríguez. 2020. The Microbiota of the Human Mammary Ecosystem. Frontiers in Cellular and Infection Microbiology 10:586667. doi: 10.3389/fcimb.2020.586667.
- Fewtrell, M. S., N. H. Mohd Shukri, and J. Wells. 2020. Optimising’ breastfeeding: What can we learn from evolutionary, comparative and anthropological aspects of lactation? BMC Medicine 18 (1):4–10. doi: 10.1186/s12916-019-1473-8.
- Fields, D. A., B. George, M. Williams, K. Whitaker, D. B. Allison, A. Teague, and E. W. Demerath. 2017. Associations between human breast milk hormones and adipocytokines and infant growth and body composition in the first 6 months of life. Pediatric Obesity 12:78–85. doi: 10.1111/ijpo.12182.
- Fields, D. A., C. R. Schneider, and G. Pavela. 2016. A narrative review of the associations between six bioactive components in breast milk and infant adiposity. Obesity (Silver Spring, Md.) 24 (6):1213–21. doi: 10.1002/oby.21519.
- Fischer Fumeaux, C. J., C. L. Garcia-Rodenas, C. A. De Castro, M. C. Courtet-Compondu, S. K. Thakkar, L. Beauport, J. F. Tolsa, and M. Affolter. 2019. Longitudinal analysis of macronutrient composition in preterm and term human milk: a prospective cohort study. Nutrients 11 (7):1525. doi: 10.3390/nu11071525.
- Fleming, S. A., M. Chichlowski, B. M. Berg, S. M. Donovan, and R. N. Dilger. 2018. Dietary sialyllactose does not influence measures of recognition memory or diurnal activity in the young pig. Nutrients 10 (4):395. doi: 10.3390/nu10040395.
- Fleming, S. A., J. Hauser, J. Yan, S. M. Donovan, M. Wang, and R. N. Dilger. 2021. A mediation analysis to identify links between gut bacteria and memory in context of human milk oligosaccharides. Microorganisms 9 (4):846. doi: 10.3390/microorganisms9040846.
- Fleming, S. A., A. T. Mudd, J. Hauser, J. Yan, S. Metairon, P. Steiner, S. M. Donovan, and R. N. Dilger. 2020a. Human and bovine milk oligosaccharides elicit improved recognition memory concurrent with alterations in regional brain volumes and hippocampal mRNA expression. Frontiers in Neuroscience 14:770. doi: 10.3389/fnins.2020.00770.
- Fleming, S. A., A. T. Mudd, J. Hauser, J. Yan, S. Metairon, P. Steiner, S. M. Donovan, and R. N. Dilger. 2020b. Dietary oligofructose alone or in combination with 2’-fucosyllactose differentially improves recognition memory and hippocampal mRNA expression. Nutrients 12 (7):2131. doi: 10.3390/nu12072131.
- Fox, M., S. M. Lee, K. S. Wiley, V. Lagishetty, C. A. Sandman, J. P. Jacobs, and L. M. Glynn. 2021. Development of the infant gut microbiome predicts temperament across the first year of life. Development and Psychopathology :1–12. doi: 10.1017/S0954579421000456.
- Furman, L., G. Taylor, N. Minich, and M. Hack. 2003. The effect of maternal milk on neonatal morbidity of very low-birth-weight infants. Archives of Pediatrics & Adolescent Medicine 157 (1):66–71. doi: 10.1001/archpedi.157.1.66.
- Gagne, J. R., C. A. Van Hulle, N. Aksan, M. J. Essex, and H. H. Goldsmith. 2011. Deriving childhood temperament measures from emotion-eliciting behavioral episodes: scale construction and initial validation. Psychological Assessment 23 (2):337–53. doi: 10.1037/a0021746.
- Galante, L., A. M. Milan, C. M. Reynolds, D. Cameron-Smith, M. H. Vickers, and S. Pundir. 2018. Sex-specific human milk composition: the role of infant sex in determining early life nutrition. Nutrients 10 (9):1194. doi: 10.3390/nu10091194.
- Galante, L., S. Pundir, H. Lagström, S. Rautava, C. M. Reynolds, A. M. Milan, D. Cameron-Smith, and M. H. Vickers. 2020. Growth factor concentrations in human milk are associated with infant weight and BMI from birth to 5 years. Frontiers in Nutrition 7:110. doi: 10.3389/fnut.2020.00110.
- Gascon, M., M. A. Verner, M. Guxens, J. O. Grimalt, J. Forns, J. Ibarluzea, N. Lertxundi, F. Ballester, S. Llop, S. Haddad, et al. 2013. Evaluating the neurotoxic effects of lactational exposure to persistent organic pollutants (POPs) in Spanish children. Neurotoxicology 34:9–15. https://doi.org/10.1542/peds.2011-3289. doi: 10.1016/j.neuro.2012.10.006.
- Georgieff, M. K., S. E. Ramel, and S. E. Cusick. 2018. Nutritional influences on brain development. Acta Paediatrica (Oslo, Norway : 1992) 107 (8):1310–21. doi: 10.1111/apa.14287.
- Giampietri, M., F. Lorenzoni, F. Moscuzza, A. Boldrini, and P. Ghirri. 2016. Lutein and neurodevelopment in preterm infants. Frontiers in Neuroscience 10:411. doi: 10.3389/fnins.2016.00411.
- Gidrewicz, D. A, and T. R. Fenton. 2014. A systematic review and meta-analysis of the nutrient content of preterm and term breast milk. BMC Pediatrics 14:216. doi: 10.1186/1471-2431-14-216.
- Gillespie, A. V., J. L. Ehrlich, and D. H. Grove-White. 2017. Effect of calf gender on milk yield and fatty acid content in Holstein dairy cows. PloS One 12 (1):e0169503. doi: 10.1371/journal.pone.0169503.
- Giuffrida, F., C. Cruz-Hernandez, E. Bertschy, P. Fontannaz, I. Masserey Elmelegy, I. Tavazzi, C. Marmet, B. Sanchez-Bridge, S. K. Thakkar, C. A. De Castro, et al. 2016. Temporal Changes of Human Breast Milk Lipids of Chinese Mothers. Nutrients 8 (11):715. doi: 10.3390/nu8110715.
- Gladstone, M., G. Lancaster, G. McCray, V. Cavallera, C. Alves, L. Maliwichi, M. A. Rasheed, T. Dua, M. Janus, and P. Kariger. 2021. Validation of the infant and young child development (IYCD) indicators in three countries: Brazil, Malawi and Pakistan. International Journal of Environmental Research and Public Health 18 (11):6117. doi: 10.3390/ijerph18116117.
- Gómez-Gallego, C., J. M. Morales, D. Monleón, E. du Toit, H. Kumar, K. M. Linderborg, Y. Zhang, B. Yang, E. Isolauri, S. Salminen, et al. 2018. Human breast milk NMR metabolomic profile across specific geographical locations and its association with the milk microbiota. Nutrients 10 (10):1355. doi: 10.3390/nu10101355.
- González, H. F, and S. Visentin. 2016. Micronutrients and neurodevelopment: an update. Micronutrientes y neurodesarrollo: actualización. Archivos Argentinos de Pediatria 114 (6):570–5. doi: 10.5546/aap.2016.eng.570.
- Goodman, R. 1997. The strengths and difficulties questionnaire: a research note. Journal of Child Psychology and Psychiatry, and Allied Disciplines 38 (5):581–6. doi: 10.1111/j.1469-7610.1997.tb01545.x.
- Grey, K. R., E. P. Davis, C. A. Sandman, and L. M. Glynn. 2013. Human milk cortisol is associated with infant temperament. Psychoneuroendocrinology 38 (7):1178–85. doi: 10.1016/j.psyneuen.2012.11.002.
- Groër, M. W., S. Humenick, and P. D. Hill. 1994. Characterizations and psychoneuroimmunologic implications of secretory immunoglobulin A and cortisol in preterm and term breast milk. The Journal of Perinatal & Neonatal Nursing 7 (4):42–51. doi: 10.1097/00005237-199403000-00005.
- Gurnida, D. A., A. M. Rowan, P. Idjradinata, D. Muchtadi, and N. Sekarwana. 2012. Association of complex lipids containing gangliosides with cognitive development of 6-month-old infants. Early Human Development 88 (8):595–601. doi: 10.1016/j.earlhumdev.2012.01.003.
- Hahn, W. H., T. Jeong, S. Park, S. Song, and N. M. Kang. 2018. Content fat and calorie of human milk is affected by interactions between maternal age and body mass index. The Journal of Maternal-Fetal & Neonatal Medicine 31 (10):1385–8. doi: 10.1080/14767058.2017.1315660.
- Hahn-Holbrook, J., D. Saxbe, C. Bixby, C. Steele, and L. Glynn. 2019. Human milk as “chrononutrition”: implications for child health and development. Pediatric Research 85 (7):936–42. doi: 10.1038/s41390-019-0368-x.
- Hamilton, M. J., A. D. Davidson, R. M. Sibly, and J. H. Brown. 2011. Universal scaling of production rates across mammalian lineages. Proceedings. Biological Sciences 278 (1705):560–6. doi: 10.1098/rspb.2010.1056.
- Hampel, D., S. Shahab-Ferdows, M. M. Islam, J. M. Peerson, and L. H. Allen. 2017. Vitamin concentrations in human milk vary with time within feed, circadian rhythm, and single-dose supplementation. The Journal of Nutrition 147 (4):603–11. doi: 10.3945/jn.116.242941.
- Hao, Z., W. Wang, R. Guo, and H. Liu. 2019. Faecalibacterium prausnitzii (ATCC 27766) has preventive and therapeutic effects on chronic unpredictable mild stress-induced depression-like and anxiety-like behavior in rats. Psychoneuroendocrinology 104:132–42. doi: 10.1016/j.psyneuen.2019.02.025.
- Harrison, P. H, and M. Porter. 1992. Development of the brachial spinal cord in the marsupial Macropus eugenii (tammar wallaby). Brain Research. Developmental Brain Research 70 (1):139–44. doi: 10.1016/0165-3806(92)90112-A.
- Hart, S., L. M. Boylan, B. Border, S. R. Carroll, D. McGunegle, and R. M. Lampe. 2004. Breast milk levels of cortisol and secretory immunoglobulin A (SIgA) differ with maternal mood and infant neuro-behavioral functioning. Infant Behavior and Development 27 (1):101–6. doi: 10.1016/j.infbeh.2003.06.002.
- Hassiotou, F., A. Beltran, E. Chetwynd, A. M. Stuebe, A. J. Twigger, P. Metzger, N. Trengove, C. T. Lai, L. Filgueira, P. Blancafort, et al. 2012. Breastmilk is a novel source of stem cells with multilineage differentiation potential. Stem Cells (Dayton, Ohio) 30 (10):2164–74. doi: 10.1002/stem.1188.
- Hassiotou, F., A. R. Hepworth, P. Metzger, C. Tat Lai, N. Trengove, P. E. Hartmann, and L. Filgueira. 2013. Maternal and infant infections stimulate a rapid leukocyte response in breastmilk. Clinical & Translational Immunology 2 (4):e3. doi: 10.1038/cti.2013.1.
- Hauser, J., E. Pisa, A. Arias Vásquez, F. Tomasi, A. Traversa, V. Chiodi, F. P. Martin, N. Sprenger, O. Lukjancenko, A. Zollinger, et al. 2021. Sialylated human milk oligosaccharides program cognitive development through a non-genomic transmission mode. Molecular Psychiatry 26 (7):2854–71. Advance online publication. doi: 10.1038/s41380-021-01054-9.
- Hechler, C., R. Beijers, J. M. Riksen-Walraven, and C. de Weerth. 2018. Are cortisol concentrations in human breast milk associated with infant crying? Developmental Psychobiology 60 (6):639–50. doi: 10.1002/dev.21761.
- Helland, I. B., L. Smith, K. Saarem, O. D. Saugstad, and C. A. Drevon. 2003. Maternal supplementation with very-long-chain n-3 fatty acids during pregnancy and lactation augments children’s IQ at 4 years of age. Pediatrics 111 (1):e39–44. doi: 10.1542/peds.111.1.e39.
- Henrich, J., S. J. Heine, and A. Norenzayan. 2010. The weirdest people in the world? The Behavioral and Brain Sciences 33 (2-3):61–135. doi: 10.1017/S0140525X0999152X.
- Hess, M. K., A. S. Hess, and D. J. Garrick. 2016. The effect of calf gender on milk production in seasonal calving cows and its impact on genetic evaluations. PloS One 11 (3):e0151236. doi: 10.1371/journal.pone.0151236.
- Hester, S. N., D. S. Hustead, A. D. Mackey, A. Singhal, and B. J. Marriage. 2012. Is the macronutrient intake of formula-fed infants greater than breast-fed infants in early infancy? Journal of Nutrition and Metabolism 2012:891201. doi: 10.1155/2012/891201.
- Hill, K. 2005. Life history theory and evolutionary anthropology. Evolutionary Anthropology: Issues, News, and Reviews 2 (3):78–88. doi: 10.1002/evan.1360020303.
- Hill, K, and H. Kaplan. 1999. Life history traits in humans: theory and empiricial studies. Annual Review of Anthropology 28:397–430. doi: 10.1146/annurev.anthro.28.1.397.
- Hinde, K. 2007. First-time macaque mothers bias milk composition in favor of sons. Current Biology : CB 17 (22):R958–9. doi: 10.1016/j.cub.2007.09.029.
- Hinde, K. 2009. Richer milk for sons but more milk for daughters: sex-biased investment during lactation varies with maternal life history in rhesus macaques. American Journal of Human Biology : The Official Journal of the Human Biology Council 21 (4):512–9. doi: 10.1002/ajhb.20917.
- Hinde, K. 2013. Lactational programming of infant behavioral phenotype. In Building babies: Primate development in proximate and ultimate perspective, ed. K. B. H. Clancy, K. Hinde, & J. N. Rutherford, 187–207. New York: Springer. doi: 10.1007/978-1-4614-4060-4_9.
- Hinde, K, and J. P. Capitanio. 2010. Lactational programming? Mother’s milk energy predicts infant behavior and temperament in rhesus macaques (Macaca mulatta). American Journal of Primatology 72 (6):522–9. doi: 10.1002/ajp.20806.
- Hinde, K., A. J. Carpenter, J. S. Clay, and B. J. Bradford. 2014. Holsteins favor heifers, not bulls: Biased milk production programmed during pregnancy as a function of fetal sex. PloS One 9 (2):e86169. doi: 10.1371/journal.pone.0086169.
- Hinde, K., A. B. Foster, L. M. Landis, D. Rendina, O. T. Oftedal, and M. L. Power. 2013. Daughter dearest: Sex-biased calcium in mother’s milk among rhesus macaques. American Journal of Physical Anthropology 151 (1):144–50. doi: 10.1002/ajpa.22229.
- Hinde, K, and L. A. Milligan. 2011. Primate milk: proximate mechanisms and ultimate perspectives. Evolutionary Anthropology 20 (1):9–23. doi: 10.1002/evan.20289.
- Hinde, K., A. L. Skibiel, A. B. Foster, L. Del Rosso, S. P. Mendoza, and J. P. Capitanio. 2015. Cortisol in mother’s milk across lactation reflects maternal life history and predicts infant temperament. Behavioral Ecology : official Journal of the International Society for Behavioral Ecology 26 (1):269–81. doi: 10.1093/beheco/aru186.
- Hoeijmakers, L., Y. Heinen, A. M. van Dam, P. J. Lucassen, and A. Korosi. 2016. Microglial priming and Alzheimer’s disease: a possible role for (early) immune challenges and epigenetics? Frontiers in Human Neuroscience 10:398. doi: 10.3389/fnhum.2016.00398.
- Hoeijmakers, L., S. R. Ruigrok, A. Amelianchik, D. Ivan, A. M. van Dam, P. J. Lucassen, and A. Korosi. 2017. Early-life stress lastingly alters the neuroinflammatory response to amyloid pathology in an Alzheimer’s disease mouse model. Brain, Behavior, and Immunity 63:160–75. doi: 10.1016/j.bbi.2016.12.023.
- Hoffmann, M. R. H. F., Shoctor, and C. J. Field. 2020. Chapter 13: minor lipids in human milk: cholesterol, gangliosides, and phospholipids. In Human milk: Sampling, measurement, and content of energy-yielding nutrients and other macromolecules, ed. M. McGuire & D. L. O’Connor, 357–85. Academic Press.
- Hollanders, J. J., A. C. Heijboer, B. van der Voorn, J. Rotteveel, and M. Finken. 2017. Nutritional programming by glucocorticoids in breast milk: targets, mechanisms and possible implications. Best Practice & Research. Clinical Endocrinology & Metabolism 31 (4):397–408. doi: 10.1016/j.beem.2017.10.001.
- Hollanders, J. J., S. Kouwenhoven, B. van der Voorn, J. B. van Goudoever, J. Rotteveel, and M. Finken. 2019. The association between breastmilk glucocorticoid concentrations and macronutrient contents throughout the day. Nutrients 11 (2):259. doi: 10.3390/nu11020259.
- Hortensius, L. M., E. Janson, P. E. van Beek, F. Groenendaal, N. Claessens, H. Swanenburg de Veye, M. Eijsermans, C. Koopman-Esseboom, J. Dudink, R. M. van Elburg, et al. 2021. Nutritional intake, white matter integrity, and neurodevelopment in extremely preterm born infants. Nutrients 13 (10):3409. doi: 10.3390/nu13103409.
- Hosseini, S. M., T. Talaei-Khozani, M. Sani, and B. Owrangi. 2014. Differentiation of human breast-milk stem cells to neural stem cells and neurons. Neurology Research International 2014:807896. doi: 10.1155/2014/807896.
- Hou, L., X. Li, P. Yan, Y. Li, Y. Wu, Q. Yang, X. Shi, L. Ge, and K. Yang. 2021. Impact of the duration of breastfeeding on the intelligence of children: a systematic review with network meta-analysis. Breastfeeding Medicine 16 (9):687–96. Advance online publication. doi: 10.1089/bfm.2020.0364.
- Hunt, K. M., J. A. Foster, L. J. Forney, U. M. Schütte, D. L. Beck, Z. Abdo, L. K. Fox, J. E. Williams, M. K. McGuire, and M. A. McGuire. 2011. Characterization of the diversity and temporal stability of bacterial communities in human milk. PloS One 6 (6):e21313. doi: 10.1371/journal.pone.0021313.
- Hurley, W. L, and P. K. Theil. 2011. Perspectives on immunoglobulins in colostrum and milk. Nutrients 3 (4):442–74. doi: 10.3390/nu3040442.
- Ikeda, N., H. Shoji, Y. Murano, M. Mori, N. Matsunaga, H. Suganuma, M. Ikeno, K. Hisata, S. Hirayama, T. Ueno, et al. 2014. Effects of breastfeeding on the risk factors for metabolic syndrome in preterm infants. Journal of Developmental Origins of Health and Disease 5 (6):459–64. doi: 10.1017/S2040174414000397.
- Ilcol, Y. O, and B. Hizli. 2007. Active and total ghrelin concentrations increase in breast milk during lactation. Acta Paediatrica 96 (11):1632–9. doi: 10.1111/j.1651-2227.2007.00493.x.
- Illnerová, H., M. Buresová, and J. Presl. 1993. Melatonin rhythm in human milk. The Journal of Clinical Endocrinology and Metabolism 77 (3):838–41. doi: 10.1210/jcem.77.3.8370707.
- Ip, S., M. Chung, G. Raman, P. Chew, N. Magula, D. DeVine, T. Trikalinos, and J. Lau. 2007. Breastfeeding and maternal and infant health outcomes in developed countries. Evidence Report/Technology Assessment (153):1–186.
- Islam, M. A., M. N. Amin, S. A. Siddiqui, M. P. Hossain, F. Sultana, and M. R. Kabir. 2019. Trans fatty acids and lipid profile: A serious risk factor to cardiovascular disease, cancer and diabetes. Diabetes & Metabolic Syndrome 13 (2):1643–7. doi: 10.1016/j.dsx.2019.03.033.
- Italianer, M. F., E. Naninck, J. A. Roelants, G. van der Horst, I. Reiss, J. Goudoever, K. Joosten, I. Chaves, and M. J. Vermeulen. 2020. Circadian variation in human milk composition, a systematic review. Nutrients 12 (8):2328. doi: 10.3390/nu12082328.
- Jackson, K. M, and A. M. Nazar. 2006. Breastfeeding, the immune response, and long-term health. The Journal of the American Osteopathic Association 106 (4):203–7.
- Jacobson, S. W., L. M. Chiodo, and J. L. Jacobson. 1999. Breastfeeding effects on intelligence quotient in 4- and 11-year-old children. Pediatrics 103 (5):e71. doi: 10.1542/peds.103.5.e71.
- Jain, A., J. Concato, and J. M. Leventhal. 2002. How good is the evidence linking breastfeeding and intelligence? Pediatrics 109 (6):1044–53. doi: 10.1542/peds.109.6.1044.
- Janik, R., L. Thomason, A. M. Stanisz, P. Forsythe, J. Bienenstock, and G. J. Stanisz. 2016. Magnetic resonance spectroscopy reveals oral Lactobacillus promotion of increases in brain GABA, N-acetyl aspartate and glutamate. NeuroImage 125:988–95. doi: 10.1016/j.neuroimage.2015.11.018.
- Jarmołowska, B., K. Sidor, M. Iwan, K. Bielikowicz, M. Kaczmarski, E. Kostyra, and H. Kostyra. 2007. Changes of beta-casomorphin content in human milk during lactation. Peptides 28 (10):1982–6. doi: 10.1016/j.peptides.2007.08.002.
- Jasienska, G. 2009. Reproduction and lifespan: trade-offs, overall energy budgets, intergenerational costs, and costs neglected by research. American Journal of Human Biology : The Official Journal of the Human Biology Council 21 (4):524–32. doi: 10.1002/ajhb.20931.
- Jehan, F., S. Sazawal, A. H. Baqui, M. I. Nisar, U. Dhingra, R. Khanam, M. Ilyas, A. Dutta, D. K. Mitra, U. Mehmood, Alliance for Maternal and Newborn Health Improvement, the Global Alliance to Prevent Prematurity and Stillbirth, and the Prematurity Research Center at Stanford University, et al. 2020. Multiomics characterization of preterm birth in low- and middle-income countries. JAMA Network Open 3 (12):e2029655. doi: 10.1001/jamanetworkopen.2020.29655.
- Jensen, C. L., R. G. Voigt, A. M. Llorente, S. U. Peters, T. C. Prager, Y. L. Zou, J. C. Rozelle, M. R. Turcich, J. K. Fraley, R. E. Anderson, et al. 2010. Effects of early maternal docosahexaenoic acid intake on neuropsychological status and visual acuity at five years of age of breast-fed term infants. The Journal of Pediatrics 157 (6):900–5. doi: 10.1016/j.jpeds.2010.06.006.
- Jensen, C. L., R. G. Voigt, T. C. Prager, Y. L. Zou, J. K. Fraley, J. C. Rozelle, M. R. Turcich, A. M. Llorente, R. E. Anderson, and W. C. Heird. 2005. Effects of maternal docosahexaenoic acid intake on visual function and neurodevelopment in breastfed term infants. The American Journal of Clinical Nutrition 82 (1):125–32. doi: 10.1093/ajcn.82.1.125.
- Jeurink, P. V., J. van Bergenhenegouwen, E. Jiménez, L. M. Knippels, L. Fernández, J. Garssen, J. Knol, J. M. Rodríguez, and R. Martín. 2013. Human milk: a source of more life than we imagine. Beneficial Microbes 4 (1):17–30. doi: 10.3920/BM2012.0040.
- Jiménez, E., J. de Andrés, M. Manrique, P. Pareja-Tobes, R. Tobes, J. F. Martínez-Blanch, F. M. Codoñer, D. Ramón, L. Fernández, and J. M. Rodríguez. 2015. Metagenomic analysis of milk of healthy and mastitis-suffering women. Journal of Human Lactation : official Journal of International Lactation Consultant Association 31 (3):406–15. doi: 10.1177/0890334415585078.
- Jonsson, K., M. Barman, S. Moberg, A. Sjöberg, H. K. Brekke, B. Hesselmar, S. Johansen, A. E. Wold, and A. S. Sandberg. 2016. Fat intake and breast milk fatty acid composition in farming and nonfarming women and allergy development in the offspring. Pediatric Research 79 (1-1):114–23. doi: 10.1038/pr.2015.187.
- Jorgensen, J. M., R. Young, P. Ashorn, U. Ashorn, D. Chaima, J. Davis, E. Goonatilleke, C. Kumwenda, C. B. Lebrilla, K. Maleta, et al. 2021. Associations of human milk oligosaccharides and bioactive proteins with infant growth and development among Malawian mother-infant dyads. The American Journal of Clinical Nutrition 113 (1):209–20. doi: 10.1093/ajcn/nqaa272.
- Jost, T., C. Lacroix, C. P. Braegger, F. Rochat, and C. Chassard. 2014. Vertical mother-neonate transfer of maternal gut bacteria via breastfeeding. Environmental Microbiology 16 (9):2891–904. doi: 10.1111/1462-2920.12238.
- Kaingade, P. M., I. Somasundaram, A. B. Nikam, S. A. Sarang, and J. S. Patel. 2016. Assessment of growth factors secreted by human breastmilk mesenchymal stem cells. Breastfeeding Medicine : The Official Journal of the Academy of Breastfeeding Medicine 11 (1):26–31. doi: 10.1089/bfm.2015.0124.
- Kakulas, F., D. T. Geddes, and P. E. Hartmann. 2016. Breastmilk is unlikely to be a source of mesenchymal stem cells. Breastfeeding Medicine 11 (3):150–1. doi: 10.1089/bfm.2016.0021.
- Kao, C. C., D. E. Que, S. J. Bongo, L. L. Tayo, Y. H. Lin, C. W. Lin, S. L. Lin, Y. Y. Gou, W. L. Hsu, C. G. Shy, et al. 2019. Residue levels of organochlorine pesticides in breast milk and its associations with cord blood thyroid hormones and the offspring’s neurodevelopment. International Journal of Environmental Research and Public Health 16 (8):1438. doi: 10.3390/ijerph16081438.
- Kavšek, M. 2004. Predicting later IQ from infant visual habitation and dishabituation: A meta-analysis. Journal of Applied Developmental Psychology 25 (3):369–93. doi: 10.1016/j.appdev.2004.04.006.
- Kawano, A, and Y. Emori. 2015. The relationship between maternal postpartum psychological state and breast milk secretory immunoglobulin A level. Journal of the American Psychiatric Nurses Association 21 (1):23–30. doi: 10.1177/1078390314566882.
- Keenan, K., A. E. Hipwell, Q. A. Class, and K. Mbayiwa. 2018. Extending the developmental origins of disease model: Impact of preconception stress exposure on offspring neurodevelopment. Developmental Psychobiology 60 (7):753–64. doi: 10.1002/dev.21773.
- Keikha, M., M. Bahreynian, M. Saleki, and R. Kelishadi. 2017. Macro- and micronutrients of human milk composition: Are they related to maternal diet? A comprehensive systematic review. Breastfeeding Medicine 12 (9):517–27. doi: 10.1089/bfm.2017.0048.
- Keikha, M., R. Shayan-Moghadam, M. Bahreynian, and R. Kelishadi. 2021. Nutritional supplements and mother’s milk composition: A systematic review of interventional studies. International Breastfeeding Journal 16 (1):1. doi: 10.1186/s13006-020-00354-0.
- Keith, D. R., B. S. Weaver, and R. L. Vogel. 2012. The effect of music-based listening interventions on the volume, fat content, and caloric content of breast milk-produced by mothers of premature and critically ill infants. Advances in Neonatal Care 12 (2):112–9. doi: 10.1097/ANC.0b013e31824d9842.
- Kent, J. C., L. R. Mitoulas, M. D. Cregan, D. T. Ramsay, D. A. Doherty, and P. E. Hartmann. 2006. Volume and frequency of breastfeedings and fat content of breast milk throughout the day. Pediatrics 117 (3):e387–e395. doi: 10.1542/peds.2005-1417.
- Kent, G. 2014. Regulating fatty acids in infant formula: Critical assessment of U.S. policies and practices. International Breastfeeding Journal 9 (1):2–10. doi: 10.1186/1746-4358-9-2.
- Khodayar-Pardo, P., L. Mira-Pascual, M. C. Collado, and C. Martínez-Costa. 2014. Impact of lactation stage, gestational age and mode of delivery on breast milk microbiota. Journal of Perinatology 34 (8):599–605. doi: 10.1038/jp.2014.47.
- Kim, K. M, and J. W. Choi. 2020. Associations between breastfeeding and cognitive function in children from early childhood to school age: A prospective birth cohort study. International Breastfeeding Journal 15 (1):83. doi: 10.1186/s13006-020-00326-4.
- Kittithanesuan, Y., S. Chiarakul, J. Kaewkungwal, and Y. Poovorawan. 2017. Effect of music on immediately postpartum lactation by term mothers after giving birth: A randomized controlled trial. Journal of Medical Association of Thailand 100 (8):834–42.
- Koletzko, B., M. Rodriguez-Palmero, H. Demmelmair, N. Fidler, R. Jensen, and T. Sauerwald. 2001. Physiological aspects of human milk lipids. Early Human Development 65 (Suppl):S3–S18. doi: 10.1016/S0378-3782(01)00204-3.
- Kominiarek, M. A., and P. Rajan. 2016. Nutrition recommendations in pregnancy and lactation. The Medical Clinics of North America 100 (6):1199–215. doi: 10.1016/j.mcna.2016.06.004.
- Kon, I. Y., N. M. Shilina, M. V. Gmoshinskaya, and T. A. Ivanushkina. 2014. The study of breast milk IGF-1, leptin, ghrelin and adiponectin levels as possible reasons of high weight gain in breast-fed infants. Annals of Nutrition and Metabolism 65 (4):317–23. doi: 10.1159/000367998.
- Kordon, E. C, and G. H. Smith. 1998. An entire functional mammary gland may comprise the progeny from a single cell. Development 125 (10):1921–30. doi: 10.1242/dev.125.10.1921.
- Krachler, M., F. S. Li, E. Rossipal, and K. J. Irgolic. 1998. Changes in the concentrations of trace elements in human milk during lactation. Journal of Trace Elements in Medicine and Biology: Organ of the Society for Minerals and Trace Elements (GMS) 12 (3):159–76. doi: 10.1016/S0946-672X(98)80005-9.
- Kramer, M. S., F. Aboud, E. Mironova, I. Vanilovich, R. W. Platt, L. Matush, S. Igumnov, E. Fombonne, N. Bogdanovich, T. Ducruet, et al. 2008. Breastfeeding and child cognitive development: New evidence from a large randomized trial. Archives of General Psychiatry 65 (5):578–84. doi: 10.1001/archpsyc.65.5.578.
- Kumar, H., E. du Toit, A. Kulkarni, J. Aakko, K. M. Linderborg, Y. Zhang, M. P. Nicol, E. Isolauri, B. Yang, M. C. Collado, et al. 2016. Distinct patterns in human milk microbiota and fatty acid profiles across specific geographic locations. Frontiers in Microbiology 7:1619. doi: 10.3389/fmicb.2016.01619.
- Kunz, C., S. Rudloff, W. Baier, N. Klein, and S. Strobel. 2000. Oligosaccharides in human milk: Structural, functional, and metabolic aspects. Annual Review of Nutrition 20:699–722. doi: 10.1146/annurev.nutr.20.1.699.
- Lancaster, G. A., G. McCray, P. Kariger, T. Dua, A. Titman, J. Chandna, D. McCoy, A. Abubakar, J. D. Hamadani, G. Fink, et al. 2018. Creation of the WHO Indicators of Infant and Young Child Development (IYCD): Metadata synthesis across 10 countries. BMJ Global Health 3 (5):e000747. doi: 10.1136/bmjgh-2018-000747.
- Langer, P. 2009. Differences in the composition of colostrum and milk in eutherians reflect differences in immunoglobulin transfer. Journal of Mammalogy 90 (2):332–9. doi: 10.1644/08-MAMM-A-071.1.
- Laue, H. E., S. A. Korrick, E. R. Baker, M. R. Karagas, and J. C. Madan. 2020. Prospective associations of the infant gut microbiome and microbial function with social behaviors related to autism at age 3 years. Scientific Reports 10 (1):15515. doi: 10.1038/s41598-020-72386-9.
- Lauritzen, L., M. H. Jørgensen, S. F. Olsen, E. M. Straarup, and K. F. Michaelsen. 2005. Maternal fish oil supplementation in lactation: Effect on developmental outcome in breast-fed infants. Reproduction Nutrition Development 45 (5):535–47. doi: 10.1051/rnd:2005044.
- Lechner, B. E, and B. R. Vohr. 2017. Neurodevelopmental outcomes of preterm infants fed human milk: A systematic review. Clinics in Perinatology 44 (1):69–83. doi: 10.1016/j.clp.2016.11.004.
- Le Doare, K., B. Holder, A. Bassett, and P. S. Pannaraj. 2018. Mother’s milk: A purposeful contribution to the development of the infant microbiota and immunity. Frontiers in Immunology 9:361. doi: 10.3389/fimmu.2018.00361.
- Lee, H., E. Padhi, Y. Hasegawa, J. Larke, M. Parenti, A. Wang, O. Hernell, B. Lönnerdal, and C. Slupsky. 2018. Compositional dynamics of the milk fat globule and its role in infant development. Frontiers in Pediatrics 6:313. doi: 10.3389/fped.2018.00313.
- Lefèvre, C. M., J. A. Sharp, and K. R. Nicholas. 2010. Evolution of lactation: Ancient origin and extreme adaptations of the lactation system. Annual Review of Genomics and Human Genetics 11:219–38. doi: 10.1146/annurev-genom-082509-141806.
- Legrand, D. 2012. Lactoferrin, a key molecule in immune and inflammatory processes. Biochemistry and Cell Biology = Biochimie et Biologie Cellulaire 90 (3):252–68. doi: 10.1139/o11-056.
- Lehner, A., K. Staub, L. Aldakak, P. Eppenberger, F. Rühli, R. D. Martin, and N. Bender. 2021. Impact of omega-3 fatty acid DHA and EPA supplementation in pregnant or breast-feeding women on cognitive performance of children: Systematic review and meta-analysis. Nutrition Reviews 79 (5):585–98. doi: 10.1093/nutrit/nuaa060.
- Leite Dantas, R., J. Freff, O. Ambrée, E. C. Beins, A. J. Forstner, U. Dannlowski, B. T. Baune, S. Scheu, and J. Alferink. 2021. Dendritic cells: Neglected modulators of peripheral immune responses and neuroinflammation in mood disorders? Cells 10 (4):941. doi: 10.3390/cells10040941.
- Li, C., N. W. Solomons, M. E. Scott, and K. G. Koski. 2016. Minerals and trace elements in human breast milk are associated with guatemalan infant anthropometric outcomes within the first 6 months. The Journal of Nutrition 146 (10):2067–74. doi: 10.3945/jn.116.232223.
- Li, F., S. S. Wu, C. L. Berseth, C. L. Harris, J. D. Richards, J. L. Wampler, W. Zhuang, G. Cleghorn, C. D. Rudolph, B. Liu, et al. 2019. Improved neurodevelopmental outcomes associated with bovine milk fat globule membrane and lactoferrin in infant formula: A randomized, controlled trial. The Journal of Pediatrics 215:24–31. doi: 10.1016/j.jpeds.2019.08.030.
- Linderborg, K. M., M. Kortesniemi, A. K. Aatsinki, L. Karlsson, H. Karlsson, B. Yang, and H. M. Uusitupa. 2020. Interactions between cortisol and lipids in human milk. International Breastfeeding Journal 15 (1):66. doi: 10.1186/s13006-020-00307-7.
- Lis-Kuberka, J, and M. Orczyk-Pawiłowicz. 2019. Sialylated oligosaccharides and glycoconjugates of human milk. The impact on infant and newborn protection, development and well-being. Nutrients 11 (2):306. doi: 10.3390/nu11020306.
- Lithoxopoulou, M., D. Rallis, H. Christou, E. Goutsiou, A. Varaklioti, P. Karagianni, C. Tsakalidis, P. Domeyer, G. Kuriakeli, and V. Soubasi. 2019. Early caloric deprivation in preterm infants affects Bayley-III scales performance at 18–24 months of corrected age. Research in Developmental Disabilities 91:103429. doi: 10.1016/j.ridd.2019.103429.
- Liu, H., E. C. Radlowski, M. S. Conrad, Y. Li, R. N. Dilger, and R. W. Johnson. 2014. Early supplementation of phospholipids and gangliosides affects brain and cognitive development in neonatal piglets. The Journal of Nutrition 144 (12):1903–9. doi: 10.3945/jn.114.199828.
- Liu, B., B. Zupan, E. Laird, S. Klein, G. Gleason, M. Bozinoski, J. Gal Toth, and M. Toth. 2014. Maternal hematopoietic TNF, via milk chemokines, programs hippocampal development and memory. Nature Neuroscience 17 (1):97–105. doi: 10.1038/nn.3596.
- Lönnerdal, B., P. Erdmann, S. K. Thakkar, J. Sauser, and F. Destaillats. 2017. Longitudinal evolution of true protein, amino acids and bioactive proteins in breast milk: A developmental perspective. The Journal of Nutritional Biochemistry 41:1–11. doi: 10.1016/j.jnutbio.2016.06.001.
- Loos, H. M., D. Reger, and B. Schaal. 2019. The odour of human milk: Its chemical variability and detection by newborns. Physiology & Behavior 199:88–99. doi: 10.1016/j.physbeh.2018.11.008.
- Lopez, D. A., J. J. Foxe, Y. Mao, W. K. Thompson, H. J. Martin, and E. G. Freedman. 2021. Breastfeeding duration is associated with domain-specific improvements in cognitive performance in 9-10-year-old children. Frontiers in Public Health 9:657422. doi: 10.3389/fpubh.2021.657422.
- Loughman, A., T. Quinn, M. L. Nation, A. Reichelt, R. J. Moore, T. Van, V. Sung, and M. Tang. 2020. Infant microbiota in colic: Predictive associations with problem crying and subsequent child behavior. Journal of Developmental Origins of Health and Disease 12 (2):260–270. doi: 10.1017/S2040174420000227.
- Lucas, A. 2005. Long-term programming effects of early nutrition -Implications for the preterm infant. Journal of Perinatology 25 (S2):S2–S6. doi: 10.1038/sj.jp.7211308.
- Lucassen, P. J., C. A. Oomen, E. F. Naninck, C. P. Fitzsimons, A. M. van Dam, B. Czeh, and A. Korosi. 2015. Regulation of adult neurogenesis and plasticity by (early) stress, glucocorticoids, and inflammation. Cold Spring Harbor Perspectives in Biology 7 (9):a021303. doi: 10.1101/cshperspect.a021303.
- Luck, B., M. A. Engevik, B. P. Ganesh, E. P. Lackey, T. Lin, M. Balderas, A. Major, J. Runge, R. A. Luna, R. V. Sillitoe, et al. 2020. Bifidobacteria shape host neural circuits during postnatal development by promoting synapse formation and microglial function. Scientific Reports 10 (1):7737. doi: 10.1038/s41598-020-64173-3.
- Mandel, D., R. Lubetzky, S. Dollberg, S. Barak, and F. B. Mimouni. 2005. Fat and energy contents of expressed human breast milk in prolonged lactation. Pediatrics 116 (3):e432–e435. doi: 10.1542/peds.2005-0313.
- Malven, P. V., H. H. Head, R. J. Collier, and F. C. Buonomo. 1987. Periparturient changes in secretion and mammary uptake of insulin and in concentrations of insulin and insulin-like growth factors in milk of dairy cows. Journal of Dairy Science 70 (11):2254–65. doi: 10.3168/jds.S0022-0302(87)80285-0.
- Manzoni, P., A. Dall’Agnola, D. Tomé, D. A. Kaufman, E. Tavella, M. Pieretto, A. Messina, D. De Luca, M. Bellaiche, A. Mosca, et al. 2018. Role of lactoferrin in neonates and infants: An update. American Journal of Perinatology 35 (6):561–5. doi: 10.1055/s-0038-1639359.
- Manzoni, P., I. Stolfi, R. Pedicino, F. Vagnarelli, F. Mosca, L. Pugni, L. Bollani, M. Pozzi, K. Gomez, C. Tzialla, et al. 2013. Human milk feeding prevents retinopathy of prematurity (ROP) in preterm VLBW neonates. Early Human Development 89:S64–S68. doi: 10.1016/S0378-3782(13)70019-7.
- Martinez, P. 2018. The comparative method in biology and the essentialist trap. Frontiers in Ecology and Evolution 6:130. doi: 10.3389/fevo.2018.00130.
- Martín, R., S. Langa, C. Reviriego, E. Jimínez, M. L. Marín, J. Xaus, L. Fernández, and J. M. Rodríguez. 2003. Human milk is a source of lactic acid bacteria for the infant gut. The Journal of Pediatrics 143 (6):754–8. doi: 10.1016/j.jpeds.2003.09.028.
- Martín, V., Maldonado-Barragán, A. Moles, L. Rodriguez-Baños, M. Campo, R. D. Fernández, L. Rodríguez, J. M, and Jiménez, E. 2012. Sharing of bacterial strains between breast milk and infant feces. Journal of Human Lactation 28 (1):36–44. doi: 10.1177/0890334411424729.
- Marques, R. C., J. V. Bernardi, J. G. Dórea, R. M. Maria de Fatima, and O. Malm. 2014. Perinatal multiple exposure to neurotoxic (lead, methylmercury, ethylmercury, and aluminum) substances and neurodevelopment at six and 24 months of age. Environmental Pollution 187:130–5. https://doi.org/10.1002/ajhb.20410. doi: 10.1016/j.envpol.2014.01.004.
- Mazzocchi, A., M. L. Giannì, D. Morniroli, L. Leone, P. Roggero, C. Agostoni, V. De Cosmi, and F. Mosca. 2019. Hormones in breast milk and effect on infants’ growth: A systematic review. Nutrients 11 (8):1845. doi: 10.3390/nu11081845.
- McGuire, M, and D. L. O’Connor. 2020. Human milk: Sampling and measurement of energy-yielding nutrients and other macromolecules. Cambridge, MA: Academic Press.
- Meng, D., E. Sommella, E. Salviati, P. Campiglia, K. Ganguli, K. Djebali, W. Zhu, and W. A. Walker. 2020. Indole-3-lactic acid, a metabolite of tryptophan, secreted by Bifidobacterium longum subspecies infantis is anti-inflammatory in the immature intestine. Pediatric Research 88 (2):209–17. doi: 10.1038/s41390-019-0740-x.
- Milani, C., S. Duranti, F. Bottacini, E. Casey, F. Turroni, J. Mahony, C. Belzer, S. Delgado Palacio, S. Arboleya Montes, L. Mancabelli, et al. 2017. The first microbial colonizers of the human gut: Composition, activities, and health implications of the infant gut microbiota. Microbiology and Molecular Biology Reviews 81 (4):e00036–17. doi: 10.1128/MMBR.00036-17.
- Miller, E. M., M. O. Aiello, M. Fujita, K. Hinde, L. Milligan, and E. A. Quinn. 2013. Field and laboratory methods in human milk research. American Journal of Human Biology 25 (1):1–11. doi: 10.1002/ajhb.22334.
- Mills, L., L. Coulter, E. Savage, and N. Modi. 2019. Macronutrient content of donor milk from a regional human milk bank: Variation with donor mother-infant characteristics. British Journal of Nutrition 122 (10):1155–67. doi: 10.1017/S0007114519002228.
- Mitoulas, L. R., J. C. Kent, D. B. Cox, R. A. Owens, J. L. Sherriff, and P. E. Hartmann. 2002. Variation in fat, lactose and protein in human milk over 24 h and throughout the first year of lactation. The British Journal of Nutrition 88 (1):29–37. doi: 10.1079/BJNBJN2002579.
- Moe-Byrne, T., J. V. Brown, and W. McGuire. 2016. Glutamine supplementation to prevent morbidity and mortality in preterm infants. The Cochrane Database of Systematic Reviews 4 (4):CD001457. doi: 10.1002/14651858.CD001457.pub6.
- Mohd Shukri, N. H., J. Wells, S. Eaton, F. Mukhtar, A. Petelin, Z. Jenko-Pražnikar, and M. Fewtrell. 2019. Randomized controlled trial investigating the effects of a breastfeeding relaxation intervention on maternal psychological state, breast milk outcomes, and infant behavior and growth. The American Journal of Clinical Nutrition 110 (1):121–30. doi: 10.1093/ajcn/nqz033.
- Moirasgenti, M., K. Doulougeri, E. Panagopoulou, and T. Theodoridis. 2019. Psychological stress reduces the immunological benefits of breast milk. Stress and Health 35 (5):681–5. doi: 10.1002/smi.2903.
- Molès, J. P., E. Tuaillon, C. Kankasa, A. S. Bedin, N. Nagot, A. Marchant, J. M. McDermid, and P. Van de Perre. 2017. Breastfeeding-related maternal microchimerism. Nature Reviews. Immunology 17 (11):729. doi: 10.1038/nri.2017.115.
- Molès, J. P., E. Tuaillon, C. Kankasa, A. S. Bedin, N. Nagot, A. Marchant, J. M. McDermid, and P. Van de Perre. 2018. Breastmilk cell trafficking induces microchimerism-mediated immune system maturation in the infant. Pediatric Allergy and Immunology 29 (2):133–43. doi: 10.1111/pai.12841.
- Montagne, P., M. L. Cuillière, C. Molé, M. C. Béné, and G. Faure. 2001. Changes in lactoferrin and lysozyme levels in human milk during the first twelve weeks of lactation. Advances in Experimental Medicine and Biology 501:241–7. doi: 10.1007/978-1-4615-1371-1_30.
- Moore, S. E. 2016. Early life nutritional programming of health and disease in The Gambia. Journal of Developmental Origins of Health and Disease 7 (2):123–31. doi: 10.1017/S2040174415007199.
- Moossavi, S., F. Atakora, K. Miliku, S. Sepehri, B. Robertson, Q. L. Duan, A. B. Becker, P. J. Mandhane, S. E. Turvey, T. J. Moraes, et al. 2019a. Integrated analysis of human milk microbiota with oligosaccharides and fatty acids in the CHILD cohort. Frontiers in Nutrition 6:1–16. doi: 10.3389/fnut.2019.00058.
- Moossavi, S., S. Sepehri, B. Robertson, L. Bode, S. Goruk, C. J. Field, L. M. Lix, R. J. de Souza, A. B. Becker, P. J. Mandhane, et al. 2019b. Composition and variation of the human milk microbiota are influenced by maternal and early-life factors. Cell Host & Microbe 25 (2):324–35. doi: 10.1016/j.chom.2019.01.011.
- Moran-Lev, H., F. B. Mimouni, A. Ovental, L. Mangel, D. Mandel, and R. Lubetzky. 2015. Circadian macronutrients variations over the first 7 weeks of human milk feeding of preterm infants. Breastfeeding Medicine: The Official Journal of the Academy of Breastfeeding Medicine 10 (7):366–70. doi: 10.1089/bfm.2015.0053.
- Moubareck, C. A. 2021. Human milk microbiota and oligosaccharides: A glimpse into benefits, diversity, and correlations. Nutrients 13 (4):1123. doi: 10.3390/nu13041123.
- Mudd, A. T., L. S. Alexander, K. Berding, R. V. Waworuntu, B. M. Berg, S. M. Donovan, and R. N. Dilger. 2016. Dietary prebiotics, milk fat globule membrane, and lactoferrin affects structural neurodevelopment in the young piglet. Frontiers in Pediatrics 4:1–10. doi: 10.3389/fped.2016.00004.
- Mullen, E. M. 1995. Mullen scales of early learning. AGS ed. Circle Pines, MN: American Guidance Service Inc.
- Mulready-Ward, C, and M. Hackett. 2014. Perception and attitudes: Breastfeeding in public in New York City. Journal of Human Lactation 30 (2):195–200. doi: 10.1177/0890334414524988.
- Munblit, D., D. Peroni, A. Boix-Amorós, P. Hsu, B. Land, M. Gay, A. Kolotilina, C. Skevaki, R. Boyle, M. Collado, et al. 2017. Human milk and allergic diseases: An unsolved puzzle. Nutrients 9 (8):894. doi: 10.3390/nu9080894.
- Munblit, D., S. Sheth, P. Abrol, M. Treneva, D. G. Peroni, L. Y. Chow, A. L. Boner, A. Pampura, J. O. Warner, and R. J. Boyle. 2016. Exposures influencing total IgA level in colostrum. Journal of Developmental Origins of Health and Disease 7 (1):61–7. doi: 10.1017/S2040174415001476.
- Naninck, E., E. Oosterink, K. Yam, L. Vries, H. Schierbeek, J. Goudoever, R. Verkaik‐Schakel, J. Plantinga, T. Plosch, P. Lucassen, et al. 2017. Early micronutrient supplementation protects against early stress-induced cognitive impairments. The FASEB Journal 31 (2):505–18. doi: 10.1096/fj.201600834R.
- Narayan, S, and E. A. Thomas. 2011. Sphingolipid abnormalities in psychiatric disorders: A missing link in pathology? Frontiers in Bioscience 16 (1):1797–810. doi: 10.2741/3822.
- Nelson, W. Y. X. Wang, G. Sakwari, and Y. B. Ding. 2019. Review of the effects of perinatal exposure to endocrine-disrupting chemicals in animals and humans. In Reviews of environmental contamination and toxicology, ed. P. de Voogt. Cham: Springer. doi: 10.1007/398_2019_30.
- Neville, M. C. 2001. Anatomy and physiology of lactation. Pediatric Clinics of North America 48 (1):13–34. doi: 10.1016/s0031-3955(05)70283-2.
- Neville, M. C., S. M. Anderson, J. L. McManaman, T. M. Badger, M. Bunik, N. Contractor, T. Crume, D. Dabelea, S. M. Donovan, N. Forman, et al. 2012. Lactation and neonatal nutrition: Defining and refining the critical questions. Journal of Mammary Gland Biology and Neoplasia 17 (2):167–88. doi: 10.1007/s10911-012-9261-5.
- Neville, M. C., T. B. McFadden, and I. Forsyth. 2002. Hormonal regulation of mammary differentiation and milk secretion. Journal of Mammary Gland Biology and Neoplasia 7 (1):49–66. doi: 10.1023/a:1015770423167.
- Nicholas, K. R., V. Modepalli, A. P. Watt, L. A. Hinds, A. Kumar, C. Lefevre, and J. A. Sharp. 2019. Guiding development of the neonate: Lessons from mammalia. Nestle Nutrition Institute Workshop Series 90:203–15. doi: 10.1159/000490319.
- Ninkina, N., M. S. Kukharsky, M. V. Hewitt, E. A. Lysikova, L. N. Skuratovska, A. V. Deykin, and V. L. Buchman. 2019. Stem cells in human breast milk. Human Cell 32 (3):223–30. doi: 10.1007/s13577-019-00251-7.
- Nitschke, A., R. Deonandan, and A. T. Konkle. 2020. The link between autism spectrum disorder and gut microbiota: A scoping review. Autism 24 (6):1328–44. doi: 10.1177/1362361320913364.
- Nishijo, M., T. T. Pham, A. T. N. Nguyen, N. N. Tran, H. Nakagawa, L. V. Hoang, A. H. Tran, Y. Morikawa, M. D. Ho, T. Kido, et al. 2014. 2, 3, 7, 8-Tetrachlorodibenzo-p-dioxin in breast milk increases autistic traits of 3-year-old children in Vietnam. Molecular Psychiatry 19 (11):1220–6. doi: 10.1038/mp.2014.18.
- Nolvi, S., H. M. Uusitupa, D. J. Bridgett, H. Pesonen, A. K. Aatsinki, E. L. Kataja, R. Korja, H. Karlsson, and L. Karlsson. 2018. Human milk cortisol concentration predicts experimentally induced infant fear reactivity: Moderation by infant sex. Developmental Science 21 (4):e12625. doi: 10.1111/desc.12625.
- Nommsen, L. A., C. A. Lovelady, M. J. Heinig, B. Lönnerdal, and K. G. Dewey. 1991. Determinants of energy, protein, lipid, and lactose concentrations in human milk during the first 12 mo of lactation: The DARLING Study. The American Journal of Clinical Nutrition 53 (2):457–65. doi: 10.1093/ajcn/53.2.457.
- Nusser, K. D, and S. Frawley. 1997. Depriving neonatal rats of milk from early lactation has long-term consequences on mammotrope development. Endocrine 7 (3):319–23. doi: 10.1007/BF02801325.
- Nutrition, E. C. O., S. Arslanoglu, W. Corpeleijn, G. Moro, C. Braegger, C. Campoy, V. Colomb, T. Decsi, M. Domellöf, M. Fewtrell, et al. 2013. Donor human milk for preterm infants: Current evidence and research directions. Journal of Pediatric Gastroenterology and Nutrition 57 (4):535–42. doi: 10.1097/MPG.0b013e3182a3af0a.
- Nyberg, L., A. Farooqi, L. Bläckberg, R. D. Duan, A. Nilsson, and O. Hernell. 1998. Digestion of ceramide by human milk bile salt-stimulated lipase. Journal of Pediatric Gastroenterology and Nutrition 27 (5):560–7. doi: 10.1097/00005176-199811000-00013.
- Obelitz-Ryom, K., S. B. Bering, S. H. Overgaard, S. F. Eskildsen, S. Ringgaard, J. L. Olesen, K. Skovgaard, S. Pankratova, B. Wang, A. Brunse, et al. 2019. Bovine milk oligosaccharides with sialyllactose improves cognition in preterm pigs. Nutrients 11 (6):1335. doi: 10.3390/nu11061335.
- O’Connor, M. E., W. Schmidt, C. Carroll-Pankhurst, and K. N. Olness. 1998. Relaxation training and breast milk secretory IgA. Archives of Pediatrics & Adolescent Medicine 152 (11):1065–70. doi: 10.1001/archpedi.152.11.1065.
- Oddy, W. H. 2012. Infant feeding and obesity risk in the child. Breastfeeding Review: Professional Publication of the Nursing Mothers’ Association of Australia 20 (2):7–12.
- Oftedal, O. T. 2012. The evolution of milk secretion and its ancient origins. Animal 6 (3):355–68. doi: 10.1017/S1751731111001935.
- Ojo-Okunola, A., M. Nicol, and E. du Toit. 2018. Human breast milk bacteriome in health and disease. Nutrients 10 (11):1643. doi: 10.3390/nu10111643.
- Olausson, H., G. R. Goldberg, M. A. Laskey, I. Schoenmakers, L. M. Jarjou, and A. Prentice. 2012. Calcium economy in human pregnancy and lactation. Nutrition Research Reviews 25 (1):40–67. doi: 10.1017/S0954422411000187.
- Oliphant, K., M. Ali, M. D’Souza, P. D. Hughes, D. Sulakhe, A. Z. Wang, B. Xie, R. Yeasin, M. E. Msall, B. Andrews, et al. 2021. Bacteroidota and lachnospiraceae integration into the gut microbiome at key time points in early life are linked to infant neurodevelopment. Gut Microbes 13 (1):1997560. doi: 10.1080/19490976.2021.1997560.
- Oliveros, E., M. J. Martin, F. J. Torres-Espinola, M. T. Segura-Moreno, M. Ramirez, A. Santos-Fandila, R. Buck, R. Rueda, M. Escudero, A. Catena, et al. 2021. Human milk levels of 2´-fucosyllactose and 6´-sialyllactose are positively associated with infant neurodevelopment and are not impacted by maternal BMI or diabetic status. Journal of Nutrition & Food Sciences 4:024.
- Oliveros, E., M. Ramirez, E. Vazquez, A. Barranco, A. Gruart, J. M. Delgado-Garcia, R. Buck, R. Rueda, and M. J. Martin. 2016. Oral supplementation of 2’-fucosyllactose during lactation improves memory and learning in rats. The Journal of Nutritional Biochemistry 31:20–7. doi: 10.1016/j.jnutbio.2015.12.014.
- Olsen, A, and N. J. Faergeman. 2017. Sphingolipids: Membrane microdomains in brain development, function and neurological diseases. Open Biology 7 (5):170069. doi: 10.1098/rsob.170069.
- Ortega-Anaya, J, and R. Jiménez-Flores. 2019. Symposium review: The relevance of bovine milk phospholipids in human nutrition-Evidence of the effect on infant gut and brain development. Journal of Dairy Science 102 (3):2738–48. doi: 10.3168/jds.2018-15342.
- O’Sullivan, E. J., S. R. Geraghty, and K. M. Rasmussen. 2017. Human milk expression as a sole or ancillary strategy for infant feeding: A qualitative study. Maternal & Child Nutrition 13 (3):e12332. doi: 10.1111/mcn.12332.
- Pace, R. M., J. E. Williams, B. Robertson, K. A. Lackey, C. L. Meehan, W. J. Price, J. A. Foster, D. W. Sellen, E. W. Kamau-Mbuthia, E. W. Kamundia, et al. 2021. Variation in human milk composition is related to differences in milk and infant fecal microbial communities. Microorganisms 9 (6):1153. doi: 10.3390/microorganisms9061153.
- Pajewska-Szmyt, M., E. Sinkiewicz-Darol, and R. Gadzała-Kopciuch. 2019. The impact of environmental pollution on the quality of mother’s milk. Environmental Science and Pollution Research 26 (8):7405–27. doi: 10.1007/s11356-019-04141-1.
- Pang, W. W., P. T. Tan, S. Cai, D. Fok, M. C. Chua, S. B. Lim, L. P. Shek, S. Y. Chan, K. H. Tan, F. Yap, et al. 2020. Nutrients or nursing? Understanding how breast milk feeding affects child cognition. European Journal of Nutrition 59 (2):609–19. doi: 10.1007/s00394-019-01929-2.
- Pannaraj, P. S., M. Ly, C. Cerini, M. Saavedra, G. M. Aldrovandi, A. A. Saboory, K. M. Johnson, and D. T. Pride. 2018. Shared and distinct features of human milk and infant stool viromes. Frontiers in Microbiology 9:1162. doi: 10.3389/fmicb.2018.01162.
- Park, Y. W. 2009. Bioactive components in milk and dairy products. Hoboken, NJ: Wiley-Blackwell.
- Park, Y. W, and G. F. W. Haenlein. 2006. Handbook of milk of non-bovine mammals. Hoboken, NJ: Blackwell Publishing.
- Pase, C. S., K. Roversi, K. Roversi, L. T. Vey, V. T. Dias, J. C. Veit, L. H. Maurer, T. Duarte, T. Emanuelli, M. Duarte, et al. 2017. Maternal trans fat intake during pregnancy or lactation impairs memory and alters BDNF and TrkB levels in the hippocampus of adult offspring exposed to chronic mild stress. Physiology & Behavior 169:114–23. doi: 10.1016/j.physbeh.2016.11.009.
- Pase, C. S., A. M. Teixeira, K. Roversi, V. T. Dias, F. Calabrese, R. Molteni, S. Franchi, A. E. Panerai, M. A. Riva, and M. E. Burger. 2015. Olive oil-enriched diet reduces brain oxidative damages and ameliorates neurotrophic factor gene expression in different life stages of rats. The Journal of Nutritional Biochemistry 26 (11):1200–7. doi: 10.1016/j.jnutbio.2015.05.013.
- Patki, S., S. Kadam, V. Chandra, and R. Bhonde. 2010. Human breast milk is a rich source of multipotent mesenchymal stem cells. Human Cell 23 (2):35–40. doi: 10.1111/j.1749-0774.2010.00083.x.
- Paulaviciene, I. J., A. Liubsys, A. Molyte, A. Eidukaite, and V. Usonis. 2020. Circadian changes in the composition of human milk macronutrients depending on pregnancy duration: A cross-sectional study. International Breastfeeding Journal 15 (1):49. doi: 10.1186/s13006-020-00291-y.
- Peila, C., G. E. Moro, E. Bertino, L. Cavallarin, M. Giribaldi, F. Giuliani, F. Cresi, and A. Coscia. 2016. The effect of holder pasteurization on nutrients and biologically-active components in donor human milk: A review. Nutrients 8 (8):477. doi: 10.3390/nu8080477.
- Perez-Burgos, A., B. Wang, Y. K. Mao, B. Mistry, K. A. McVey Neufeld, J. Bienenstock, and W. Kunze. 2013. Psychoactive bacteria Lactobacillus rhamnosus (JB-1) elicits rapid frequency facilitation in vagal afferents. American Journal of Physiology. Gastrointestinal and Liver Physiology 304 (2):G211–G220. doi: 10.1152/ajpgi.00128.2012.
- Perrin, M. T., R. Pawlak, L. L. Dean, A. Christis, and L. Friend. 2019. A cross-sectional study of fatty acids and brain-derived neurotrophic factor (BDNF) in human milk from lactating women following vegan, vegetarian, and omnivore diets. European Journal of Nutrition 58 (6):2401–10. doi: 10.1007/s00394-018-1793-z.
- Pitino, M. A., S. Unger, A. Doyen, Y. Pouliot, S. Aufreiter, D. Stone, A. Kiss, and D. L. O’Connor. 2019. High hydrostatic pressure processing better preserves the nutrient and bioactive compound composition of human donor milk. The Journal of Nutrition 149 (3):497–504. doi: 10.1093/jn/nxy302.
- Petrullo, L., K. Hinde, and A. Lu. 2019. Steroid hormone concentrations in milk predict sex-specific offspring growth in a nonhuman primate. American Journal of Human Biology 31 (6):e23315. doi: 10.1002/ajhb.23315.
- Pittet, F., C. Johnson, and K. Hinde. 2017. Age at reproductive debut: Developmental predictors and consequences for lactation, infant mass, and subsequent reproduction in rhesus macaques (Macaca mulatta). American Journal of Physical Anthropology 164 (3):457–76. doi: 10.1002/ajpa.23286.
- Pitts, M. W., C. N. Byrns, A. N. Ogawa-Wong, P. Kremer, and M. J. Berry. 2014. Selenoproteins in nervous system development and function. Biological Trace Element Research 161 (3):231–45. doi: 10.1007/s12011-014-0060-2.
- Pocock, J. M, and H. Kettenmann. 2007. Neurotransmitter receptors on microglia. Trends in Neurosciences 30 (10):527–35. doi: 10.1016/j.tins.2007.07.007.
- Prado, E. L, and K. G. Dewey. 2014. Nutrition and brain development in early life. Nutrition Reviews 72 (4):267–84. doi: 10.1111/nure.12102.
- Prentice, P., A. Koulman, L. Matthews, C. L. Acerini, K. K. Ong, and D. B. Dunger. 2015. Lipidomic analyses, breast- and formula-feeding, and growth in infants. The Journal of Pediatrics 166 (2):276–81.e6. doi: 10.1016/j.jpeds.2014.10.021.
- Pundir, S., J. Mäkelä, A. Nuora, N. Junttila, C. R. Wall, K. Linderborg, D. Cameron-Smith, and H. Lagström. 2019. Maternal influences on the glucocorticoid concentrations of human milk: The STEPS study. Clinical Nutrition (Edinburgh, Scotland) 38 (4):1913–20. doi: 10.1016/j.clnu.2018.06.980.
- Pundir, S., C. R. Wall, C. J. Mitchell, E. B. Thorstensen, C. T. Lai, D. T. Geddes, and D. Cameron-Smith. 2017. Variation of human milk glucocorticoids over 24 hour period. Journal of Mammary Gland Biology and Neoplasia 22 (1):85–92. doi: 10.1007/s10911-017-9375-x.
- Quesada, J. A., I. Méndez, and R. Martín-Gil. 2020. The economic benefits of increasing breastfeeding rates in Spain. International Breastfeeding Journal 15 (1):34. doi: 10.1186/s13006-020-00277-w.
- Quigley, M., N. D. Embleton, and W. McGuire. 2019. Formula versus donor breast milk for feeding preterm or low birth weight infants. The Cochrane Database of Systematic Reviews 7 (7):CD002971. doi: 10.1002/14651858.CD002971.pub5.
- Rachetti, A. L., R. M. Arida, C. L. Patti, K. A. Zanin, L. Fernades-Santos, R. Frussa-Filho, S. Gomes da Silva, F. A. Scorza, and R. M. Cysneiros. 2013. Fish oil supplementation and physical exercise program: Distinct effects on different memory tasks. Behavioural Brain Research 237:283–9. doi: 10.1016/j.bbr.2012.09.048.
- Rahman, A., J. S. Kase, Y. L. Murray, and B. Parvez. 2020. Neurodevelopmental outcome of extremely low birth weight infants fed an exclusive human milk diet is not affected by growth velocity. Breastfeeding Medicine 15 (6):362–9. doi: 10.1089/bfm.2019.0214.
- Ramanan, D., E. Sefik, S. Galván-Peña, M. Wu, L. Yang, Z. Yang, A. Kostic, T. V. Golovkina, D. L. Kasper, D. Mathis, et al. 2020. An immunologic mode of multigenerational transmission governs a gut treg setpoint. Cell 181 (6):1276–90.e13. doi: 10.1016/j.cell.2020.04.030.
- Ratsika, A., M. Codagnone, S. O’Mahony, C. Stanton, and J. Cryan. 2021. Priming for life: Early life nutrition and the microbiota-gut-brain axis. Nutrients 13 (2):423. doi: 10.3390/nu13020423.
- Rebelo, F. M, and E. D. Caldas. 2016. Arsenic, lead, mercury and cadmium: Toxicity, levels in breast milk and the risks for breastfed infants. Environmental Research 151:671–88. doi: 10.1016/j.envres.2016.08.027.
- Redeuil, K., A. Lévêques, J. M. Oberson, S. Bénet, E. Tissot, K. Longet, A. de Castro, C. Romagny, L. Beauport, C. J. Fischer Fumeaux, et al. 2021. Vitamins and carotenoids in human milk delivering preterm and term infants: Implications for preterm nutrient requirements and human milk fortification strategies. Clinical Nutrition (Edinburgh, Scotland) 40 (1):222–8. doi: 10.1016/j.clnu.2020.05.012.
- Reilly, J. J., S. Ashworth, and J. C. Wells. 2005. Metabolisable energy consumption in the exclusively breast-fed infant aged 3-6 months from the developed world: A systematic review. The British Journal of Nutrition 94 (1):56–63. doi: 10.1079/bjn20051464.
- Resto, M., D. O’Connor, K. Leef, V. Funanage, M. Spear, and R. Locke. 2001. Leptin levels in preterm human breast milk and infant formula. Pediatrics 108 (1):E15–e15. doi: 10.1542/peds.108.1.e15.
- Reyes, S. M., D. L. Allen, J. E. Williams, M. A. McGuire, M. K. McGuire, A. G. Hay, and K. M. Rasmussen. 2021. Pumping supplies alter the microbiome of pumped human milk: An in-home, randomized, crossover trial. The American Journal of Clinical Nutrition 114 (6):1960–70. Advance online publication. doi: 10.1093/ajcn/nqab273.
- Reynolds, C. R, and R. W. Kamphaus. 2004. Behavior assessment system for children. Circle Pines, MN: American Guidance Service.
- Rhee, S. H., C. Pothoulakis, and E. A. Mayer. 2009. Principles and clinical implications of the brain-gut-enteric microbiota axis. Nature Reviews. Gastroenterology & Hepatology 6 (5):306–14. doi: 10.1038/nrgastro.2009.35.
- Riskin, A., M. Almog, R. Peri, K. Halasz, I. Srugo, and A. Kessel. 2012. Changes in immunomodulatory constituents of human milk in response to active infection in the nursing infant. Pediatric Research 71 (2):220–5. https://doi.org/10.1038/pr.2011. doi: 10.1038/pr.2011.34.
- Robert, K. A, and S. Braun. 2012. Milk composition during lactation suggests a mechanism for male biased allocation of maternal resources in the tammar wallaby (Macropus eugenii). PloS One 7 (11):e51099. doi: 10.1371/journal.pone.0051099.
- Roelants, J. A., H. Vlaardingerbroek, C. van den Akker, R. de Jonge, J. B. van Goudoever, and M. J. Vermeulen. 2018. Two-year follow-up of a randomized controlled nutrition intervention trial in very low-birth-weight infants. Journal of Parenteral and Enteral Nutrition 42 (1):122–31. doi: 10.1177/0148607116678196.
- Rogier, E. W., A. L. Frantz, M. E. Bruno, L. Wedlund, D. A. Cohen, A. J. Stromberg, and C. S. Kaetzel. 2014. Lessons from mother: Long-term impact of antibodies in breast milk on the gut microbiota and intestinal immune system of breastfed offspring. Gut Microbes 5 (5):663–8. doi: 10.4161/19490976.2014.969984.
- Rollins, N. C., N. Bhandari, N. Hajeebhoy, S. Horton, C. K. Lutter, J. C. Martines, E. G. Piwoz, L. M. Richter, and C. G. Victora. 2016. Why invest, and what it will take to improve breastfeeding practices? Lancet (London, England) 387 (10017):491–504. doi: 10.1016/S0140-6736(15)01044-2.
- Romijn, M., L. van Tilburg, J. J. Hollanders, B. van der Voorn, P. de Goede, K. M. Dolman, A. C. Heijboer, B. Broekman, J. Rotteveel, and M. Finken. 2021. The association between maternal stress and glucocorticoid rhythmicity in human milk. Nutrients 13 (5):1608. doi: 10.3390/nu13051608.
- Ronchetti, R., P. van den Hazel, G. Schoeters, W. Hanke, Z. Rennezova, M. Barreto, and M. P. Villa. 2006. Lead neurotoxicity in children: Is prenatal exposure more important than postnatal exposure? Acta Paediatrica (Oslo, Norway: 1992) Supplement 95 (453):45–9. doi: 10.1080/08035320600886224.
- Rothbart, M. 2007. Temperament, development, and personality. Current Directions in Psychological Science 16 (4):207–12. [Database] doi: 10.1111/j.1467-8721.2007.00505.x.
- Rozé, J. C., B. Morel, A. Lapillonne, S. Marret, I. Guellec, D. Darmaun, N. Bednarek, T. Moyon, L. Marchand-Martin, V. Benhammou, et al. 2021. Association between early amino acid intake and full-scale IQ at age 5 years among infants born at less than 30 weeks’ gestation. JAMA Network Open 4 (11):e2135452. doi: 10.1001/jamanetworkopen.2021.35452.
- Ruiz, L., Bacigalupe, R. García-Carral, C. Boix-Amoros, A. Argüello, H. Silva, C. B. d. Los Angeles Checa, M. Mira, A, and Rodríguez, J. M. 2019. Microbiota of human precolostrum and its potential role as a source of bacteria to the infant mouth. Scientific Reports 9 (1):8435. doi: 10.1038/s41598-019-42514-1.
- Ruiz, L., I. Espinosa-Martos, C. García-Carral, S. Manzano, M. K. McGuire, C. L. Meehan, M. A. McGuire, J. E. Williams, J. Foster, D. W. Sellen, et al. 2017. What’s normal? Immune profiling of human milk from healthy women living in different geographical and socioeconomic settings. Frontiers in Immunology 8:696. doi: 10.3389/fimmu.2017.00696.
- Ruys, C. A., T. Bröring, P. van Schie, M. van de Lagemaat, J. Rotteveel, M. Finken, K. J. Oostrom, and H. N. Lafeber. 2019. Neurodevelopment of children born very preterm and/or with a very low birth weight: 8-year follow-up of a nutritional RCT. Clinical Nutrition ESPEN 30:190–8. doi: 10.1016/j.clnesp.2018.12.083.
- Saarela, T., J. Kokkonen, and M. Koivisto. 2005. Macronutrient and energy contents of human milk fractions during the first six months of lactation. Acta Paediatrica (Oslo, Norway: 1992) 94 (9):1176–81. doi: 10.1111/j.1651-2227.2005.tb02070.x.
- Sabatier, M., C. L. Garcia-Rodenas, C. A. Castro, P. Kastenmayer, M. Vigo, S. Dubascoux, D. Andrey, M. Nicolas, J. R. Payot, V. Bordier, et al. 2019. Longitudinal changes of mineral concentrations in preterm and term human milk from lactating Swiss women. Nutrients 11 (8):1855. doi: 10.3390/nu11081855.
- Salvador, A. F., K. A. de Lima, and J. Kipnis. 2021. Neuromodulation by the immune system: A focus on cytokines. Nature Reviews. Immunology 21 (8):526–41. doi: 10.1038/s41577-021-00508-z.
- Samuel, T. M., Q. Zhou, F. Giuffrida, D. Munblit, V. Verhasselt, and S. K. Thakkar. 2020. Nutritional and non-nutritional composition of human milk is modulated by maternal, infant, and methodological factors. Frontiers in Nutrition 7:576133. doi: 10.3389/fnut.2020.576133.
- Sánchez, C., C. Fente, R. Barreiro, O. López-Racamonde, A. Cepeda, and P. Regal. 2020. Association between breast milk mineral content and maternal adherence to healthy dietary patterns in Spain: A transversal study. Foods 9 (5):659. doi: 10.3390/foods9050659.
- Saraf, M. K., B. Piccolo, A. Bowlin, K. Mercer, T. LeRoith, S. Chintapalli, K. Shankar, T. Badger, and L. Yeruva. L 2017. Formula diet driven microbiota shifts tryptophan metabolism from serotonin to tryptamine in neonatal porcine colon. Microbiome 5 (1):77. doi: 10.1186/s40168-017-0297-z.
- Saunders, N. R., E. Adam, M. Reader, and K. Møllgård. 1989. Monodelphis domestica (grey short-tailed opossum): An accessible model for studies of early neocortical development. Anatomy and Embryology 180 (3):227–36. doi: 10.1007/BF00315881.
- Saunders, N. R., G. W. Knott, and K. M. Dziegielewska. 2000. Barriers in the immature brain. Cellular and Molecular Neurobiology 20 (1):29–40. doi: 10.1023/a:1006991809927.
- Savino, F, and S. A. Liguori. 2008. Update on breast milk hormones: Leptin, ghrelin and adiponectin. Clinical Nutrition (Edinburgh, Scotland) 27 (1):42–7. doi: 10.1016/j.clnu.2007.06.006.
- Schnaar, R. L., R. Gerardy-Schahn, and H. Hildebrandt. 2014. Sialic acids in the brain: Gangliosides and polysialic acid in nervous system development, stability, disease, and regeneration. Physiological Reviews 94 (2):461–518. doi: 10.1152/physrev.00033.2013.
- Schneider, N, and C. L. Garcia-Rodenas. 2017. Early nutritional interventions for brain and cognitive development in preterm infants: A review of the literature. Nutrients 9 (3):187. doi: 10.3390/nu9030187.
- Schneider, N., J. Hauser, M. Oliveira, E. Cazaubon, S. C. Mottaz, B. V. O’Neill, P. Steiner, and S. Deoni. 2019. Sphingomyelin in brain and cognitive development: Preliminary data. eNeuro 6 (4):ENEURO.0421-18.2019. doi: 10.1523/ENEURO.0421-18.2019.
- Schubring, C., P. Englaro, T. Siebler, W. F. Blum, T. Demirakca, J. Kratzsch, and W. Kiess. 1998. Longitudinal analysis of maternal serum leptin levels during pregnancy, at birth and up to six weeks after birth: Relation to body mass index, skinfolds, sex steroids and umbilical cord blood leptin levels. Hormone Research 50 (5):276–83. doi: 10.1159/000023290.
- Schwab, C., E. Voney, A. Ramirez Garcia, M. Vischer, and C. Lacroix. 2019. Characterization of the cultivable microbiota in fresh and stored mature human breast milk. Frontiers in Microbiology 10:2666. doi: 10.3389/fmicb.2019.02666.
- Schwalm, J. W, and H. A. Tucker. 1978. Glucocorticoids in mammary secretions and blood serum during reproduction and lactation and distributions of glucocorticoids, progesterone, and estrogens in fractions of milk. Journal of Dairy Science 61 (5):550–60. doi: 10.3168/jds.s0022-0302(78)94409-0.
- Sgritta, M., S. W. Dooling, S. A. Buffington, E. N. Momin, M. B. Francis, R. A. Britton, and M. Costa-Mattioli. 2019. Mechanisms underlying microbial-mediated changes in social behavior in mouse models of autism spectrum disorder. Neuron 101 (2):246–59.e6. doi: 10.1016/j.neuron.2018.11.018.
- Sharma, B. M., O. Sáňka, J. Kalina, and M. Scheringer. 2019. An overview of worldwide and regional time trends in total mercury levels in human blood and breast milk from 1966 to 2015 and their associations with health effects. Environment International 125:300–19. doi: 10.1016/j.envint.2018.12.016.
- Sharp, J. A., V. Modepalli, A. K. Enjapoori, S. Bisana, H. E. Abud, C. Lefevre, and K. R. Nicholas. 2014. Bioactive functions of milk proteins: A comparative genomics approach. Journal of Mammary Gland Biology and Neoplasia 19 (3–4):289–302. doi: 10.1007/s10911-015-9331-6.
- Sharp, J. A., S. Wanyonyi, V. Modepalli, A. Watt, S. Kuruppath, L. A. Hinds, A. Kumar, H. E. Abud, C. Lefevre, and K. R. Nicholas. 2017. The tammar wallaby: A marsupial model to examine the timed delivery and role of bioactives in milk. General and Comparative Endocrinology 244:164–77. doi: 10.1016/j.ygcen.2016.08.007.
- Shenhav, L, and M. B. Azad. 2022. Using community ecology theory and computational microbiome methods to study human milk as a biological system. mSystems 7 (1):e0113221. doi: 10.1128/msystems.01132-21.
- Sherman, E. M. S, and B. L. Brooks. 2010. Behavior rating inventory of executive function – Preschool version (BRIEF-P): Test review and clinical guidelines for use. Child Neuropsychology 16 (5):503–19. doi: 10.1080/09297041003679344.
- Sherwin, E., K. V. Sandhu, T. G. Dinan, and J. F. Cryan. 2016. May the force be with you: The light and dark sides of the microbiota-gut-brain axis in neuropsychiatry. CNS Drugs 30 (11):1019–41. doi: 10.1007/s40263-016-0370-3.
- Shumake, J., D. W. Barrett, M. A. Lane, and A. J. Wittke. 2014. Behavioral effects of bovine lactoferrin administration during postnatal development of rats. BioMetals 27 (5):1039–55. doi: 10.1007/s10534-014-9735-6.
- Silvestre, D., C. Martìnez-Costa, M. J. Lagarda, J. Brines, R. Farré, and G. Clemente. 2001. Copper, iron, and zinc contents in human milk during the first three months of lactation: A longitudinal study. Biological Trace Element Research 80 (1):1–11. doi: 10.1385/BTER:80:1:01.
- Sims, C. R., M. E. Lipsmeyer, D. E. Turner, and A. Andres. 2020. Human milk composition differs by maternal BMI in the first 9 months postpartum. The American Journal of Clinical Nutrition 112 (3):548–57. doi: 10.1093/ajcn/nqaa098.
- Singh, S. P., S. Häussler, J. J. Gross, F. J. Schwarz, R. M. Bruckmaier, and H. Sauerwein. 2014. Short communication: Circulating and milk adiponectin change differently during energy deficiency at different stages of lactation in dairy cows. Journal of Dairy Science 97 (3):1535–42. doi: 10.3168/jds.2013-7598.
- Singhal, A., T. J. Cole, and A. Lucas. 2001. Early nutrition in preterm infants and later blood pressure: Two cohorts after randomised trials. Lancet (London, England) 357 (9254):413–9. doi: 10.1016/S0140-6736(00)04004-6.
- Skowronski, A. A., C. A. LeDuc, K. S. Foo, Y. Goffer, L. C. Burnett, D. Egli, and R. L. Leibel. 2020. Physiological consequences of transient hyperleptinemia during discrete developmental periods on body weight in mice. Science Translational Medicine 12 (524):eaax6629. doi: 10.1126/scitranslmed.aax6629.
- Solís, G., C. G. de Los Reyes-Gavilan, N. Fernández, A. Margolles, and M. Gueimonde. 2010. Establishment and development of lactic acid bacteria and bifidobacteria microbiota in breast-milk and the infant gut. Anaerobe 16 (3):307–10. doi: 10.1016/j.anaerobe.2010.02.004.
- Song, J., W. Ma, X. Gu, L. Zhao, J. Jiang, Y. Xu, L. Zhang, M. Zhou, and L. Yang. 2019. Metabolomic signatures and microbial community profiling of depressive rat model induced by adrenocorticotrophic hormone. Journal of Translational Medicine 17 (1):224. doi: 10.1186/s12967-019-1970-8.
- Spahn, J. M., E. H. Callahan, M. K. Spill, Y. P. Wong, S. E. Benjamin-Neelon, L. Birch, M. M. Black, J. T. Cook, M. S. Faith, J. A. Mennella, et al. 2019. Influence of maternal diet on flavor transfer to amniotic fluid and breast milk and children’s responses: A systematic review. The American Journal of Clinical Nutrition 109 (Suppl 1):1003S–26S. doi: 10.1093/ajcn/nqy240.
- Standish, K. R., and M. G. Parker. 2021. Social determinants of breastfeeding in the United States. Clinical Therapeutics S0149-2918 (21)00464-1. doi: 10.1016/j.clinthera.2021.11.010.
- Stearns, S. C. 1992. The evolution of life histories. Oxford: Oxford University Press.
- Steiner, P. 2019. Brain fuel utilization in the developing brain. Annals of Nutrition and Metabolism 75 (1):8–18. doi: 10.1159/000508054.
- Stroop, J. R. 1935. Studies of interference in serial verbal reactions. Journal of Experimental Psychology 18 (6):643–62. hdl:11858/00-001M-0000-002C-5ADB-7. Retrieved 2008-10-08. doi: 10.1037/h0054651.
- Stuebe, A. M., B. J. Jegier, E. B. Schwarz, B. D. Green, A. G. Reinhold, T. T. Colaizy, D. L. Bogen, A. J. Schaefer, J. T. Jegier, N. S. Green, et al. 2017. An online calculator to estimate the impact of changes in breastfeeding rates on population health and costs. Breastfeeding Medicine 12 (10):645–58. doi: 10.1089/bfm.2017.0083.
- Tai, P. T., M. Nishijo, N. T. N. Anh, S. Maruzeni, H. Nakagawa, H. V. Luong, T. H. Anh, R. Honda, T. Kido, and H. Nishijo. 2013. Dioxin exposure in breast milk and infant neurodevelopment in Vietnam. Occupational and Environmental Medicine 70 (9):656–62. https://doi.org/10.1371/journal.pone.0040273. doi: 10.1136/oemed-2012-101021.
- Taki, F., K. Lopez, B. Zupan, P. Bergin, M. D. Docampo, M. Alves-Bezerra, J. G. Toth, Q. Chen, K. V. Argyropoulos, L. Barboza, et al. 2020. Maternal programming of social dominance via milk cytokines. Maternal Programming of Social Dominance via Milk Cytokines. iScience 23 (8):101357. doi: 10.1016/j.isci.2020.101.357.
- Tamana, S. K., H. M. Tun, T. Konya, R. S. Chari, C. J. Field, D. S. Guttman, A. B. Becker, T. J. Moraes, S. E. Turvey, P. Subbarao, et al. 2021. Bacteroides-dominant gut microbiome of late infancy is associated with enhanced neurodevelopment. Gut Microbes 13 (1):1–17. doi: 10.1080/19490976.2021.1930875.
- Tanaka, K., M. Hosozawa, N. Kudo, N. Yoshikawa, K. Hisata, H. Shoji, K. Shinohara, and T. Shimizu. 2013. The pilot study: Sphingomyelin-fortified milk has a positive association with the neurobehavioural development of very low birth weight infants during infancy, randomized control trial. Brain and Development 35 (1):45–52. doi: 10.1016/j.braindev.2012.03.004.
- Thulier, D, and J. Mercer. 2009. Variables associated with breastfeeding duration. Journal of Obstetric, Gynecologic, and Neonatal Nursing: JOGNN 38 (3):259–68. doi: 10.1111/j.1552-6909.2009.01021.x.
- Thundiyil, J. G., G. M. Solomon, and M. D. Miller. 2007. Transgenerational exposures: Persistent chemical pollutants in the environment and breast milk. Pediatric Clinics of North America 54 (1):81–101. doi: 10.1016/j.pcl.2006.11.006.
- Tian, P., Y. Chen, H. Zhu, L. Wang, X. Qian, R. Zou, J. Zhao, H. Zhang, L. Qian, Q. Wang, et al. 2022. Bifidobacterium breve CCFM1025 attenuates major depression disorder via regulating gut microbiome and tryptophan metabolism: A randomized clinical trial. Brain, Behavior, and Immunity 100:233–41. doi: 10.1016/j.bbi.2021.11.023.
- Timby, N., M. Adamsson, E. Domellöf, T. Grip, O. Hernell, B. Lönnerdal, and M. Domellöf. 2021. Neurodevelopment and growth until 6.5 years of infants who consumed a low-energy, low-protein formula supplemented with bovine milk fat globule membranes: A randomized controlled trial. The American Journal of Clinical Nutrition 113 (3):586–92. doi: 10.1093/ajcn/nqaa354.
- Timby, N., E. Domellöf, O. Hernell, B. Lönnerdal, and M. Domellöf. 2014. Neurodevelopment, nutrition, and growth until 12 mo of age in infants fed a low-energy, low-protein formula supplemented with bovine milk fat globule membranes: A randomized controlled trial. The American Journal of Clinical Nutrition 99 (4):860–8. doi: 10.3945/ajcn.113.064295.
- Toorop, A. A., B. van der Voorn, J. J. Hollanders, L. R. Dijkstra, K. M. Dolman, A. C. Heijboer, J. Rotteveel, A. Honig, and M. Finken. 2020. Diurnal rhythmicity in breast-milk glucocorticoids, and infant behavior and sleep at age 3 months. Endocrine 68 (3):660–8. doi: 10.1007/s12020-020-02273-w.
- Totten, S. M., A. M. Zivkovic, S. Wu, U. Ngyuen, S. L. Freeman, L. R. Ruhaak, M. K. Darboe, J. B. German, A. M. Prentice, and C. B. Lebrilla. 2012. Comprehensive profiles of human milk oligosaccharides yield highly sensitive and specific markers for determining secretor status in lactating mothers. Journal of Proteome Research 11 (12):6124–33. doi: 10.1021/pr300769g.
- Tremblay, M. È., B. Stevens, A. Sierra, H. Wake, A. Bessis, and A. Nimmerjahn. 2011. The role of microglia in the healthy brain. Journal of Neuroscience 31 (45):16064–9. doi: 10.1523/JNEUROSCI.4158-11.2011.
- Trend, S., E. de Jong, M. L. Lloyd, C. H. Kok, P. Richmond, D. A. Doherty, K. Simmer, F. Kakulas, T. Strunk, and A. Currie. 2015. Leukocyte populations in human preterm and term breast milk identified by multicolour flow cytometry. PloS One 10 (8):e0135580. doi: 10.1371/journal.pone.0135580.
- Trivers, R. L. 1974. Parent-offspring conflict. American Zoologist 14 (1):249–64. doi: 10.1093/icb/14.1.249.
- Tsukahara, J. S, and R. W. Engle. 2021. Is baseline pupil size related to cognitive ability? Yes (under proper lighting conditions). Cognition 211:104643. doi: 10.1016/j.cognition.2021.104643.
- Tully, K. P, and H. L. Ball. 2013. Trade-offs underlying maternal breastfeeding decisions: A conceptual model. Maternal & Child Nutrition 9 (1):90–8. doi: 10.1111/j.1740-8709.2011.00378.x.
- Twigger, A. J., S. Hodgetts, L. Filgueira, P. E. Hartmann, and F. Hassiotou. 2013. From breast milk to brains: The potential of stem cells in human milk. Journal of Human Lactation 29 (2):136–9. doi: 10.1177/0890334413475528.
- Uberos, J., S. Jimenez-Montilla, I. Machado-Casas, C. Laynez-Rubio, E. Fernández-Marin, and A. Campos-Martínez. 2021. The association between restricted intra-uterine growth and inadequate postnatal nutrition in very-low-birth-weight infants and their neurodevelopmental outcomes: A 50-month follow-up study. The British Journal of Nutrition 127 (4):580–588. doi: 10.1017/S000711452100132X.
- Underwood, M. A. 2013. Human milk for the premature infant. Pediatric Clinics of North America 60 (1):189–207. https://doi.org/10.1016/j.pcl.2012.09. doi: 10.1016/j.pcl.2012.09.008.
- Underwood, M. A., J. B. German, C. B. Lebrilla, and D. A. Mills. 2015. Bifidobacterium longum subspecies infantis: Champion colonizer of the infant gut. Pediatric Research 77 (1–2):229–35. doi: 10.1038/pr.2014.156.
- Uthaya, S., X. Liu, D. Babalis, C. J. Doré, J. Warwick, J. Bell, L. Thomas, D. Ashby, G. Durighel, A. Ederies, et al. 2016. Nutritional evaluation and optimisation in neonates: A randomized, double-blind controlled trial of amino acid regimen and intravenous lipid composition in preterm parenteral nutrition. The American Journal of Clinical Nutrition 103 (6):1443–52. doi: 10.3945/ajcn.115.125138.
- Valent, F., M. Horvat, D. Mazej, V. Stibilj, and F. Barbone. 2011. Maternal diet and selenium concentration in human milk from an Italian population. Journal of Epidemiology 21 (4):285–92. doi: 10.2188/jea.je20100183.
- van den Akker, C. H., F. W. te Braake, N. Weisglas-Kuperus, and J. B. van Goudoever. 2014. Observational outcome results following a randomized controlled trial of early amino acid administration in preterm infants. Journal of Pediatric Gastroenterology & Nutrition 59 (6):714–9. doi: 10.1097/MPG.0000000000000549.
- van der Voorn, B., M. de Waard, J. B. van Goudoever, J. Rotteveel, A. C. Heijboer, and M. J. Finken. 2016. Breast-milk cortisol and cortisone concentrations follow the diurnal rhythm of maternal hypothalamus-pituitary-adrenal axis activity. The Journal of Nutrition 146 (11):2174–9. doi: 10.3945/jn.116.236349.
- van Goudoever, J., V. Carnielli, D. Darmaun, M. Sainz de Pipaon, C. Braegger, J. Bronsky, W. Cai, C. Campoy, V. Carnielli, D. Darmaun, et al. 2018. ESPGHAN/ESPEN/ESPR/CSPEN guidelines on pediatric parenteral nutrition: Amino acids. Clinical Nutrition (Edinburgh, Scotland) 37 (6):2315–23. doi: 10.1016/j.clnu.2018.06.945.
- Varişoğlu, Y, and I. Güngör Satilmiş. 2020. The effects of listening to music on breast milk production by mothers of premature newborns in the neonatal intensive care unit: A randomized controlled study. Breastfeeding Medicine 15 (7):465–70. doi: 10.1089/bfm.2020.0027.
- Vass, R. A., R. D. Roghair, E. F. Bell, T. T. Colaizy, K. J. Johnson, M. L. Schmelzel, J. R. Walker, and T. Ertl. 2020. Pituitary glycoprotein hormones in human milk before and after pasteurization or refrigeration. Nutrients 12 (3):687. doi: 10.3390/nu12030687.
- Vázquez, E., A. Barranco, M. Ramírez, A. Gruart, J. M. Delgado-García, E. Martínez-Lara, S. Blanco, M. J. Martín, E. Castanys, R. Buck, et al. 2015. Effects of a human milk oligosaccharide, 2’-fucosyllactose, on hippocampal long-term potentiation and learning capabilities in rodents. The Journal of Nutritional Biochemistry 26 (5):455–65. doi: 10.1016/j.jnutbio.2014.11.016.
- Vega-Bautista, A., M. de la Garza, J. C. Carrero, R. Campos-Rodríguez, M. Godínez-Victoria, and M. E. Drago-Serrano. 2019. The impact of lactoferrin on the growth of intestinal inhabitant bacteria. International Journal of Molecular Sciences 20 (19):4707. doi: 10.3390/ijms20194707.
- Ventura, A. K. 2017. Does breastfeeding shape food preferences? Links to obesity. Annals of Nutrition & Metabolism 70 (Suppl 3):8–15. doi: 10.1159/000478757.
- Verfuerden, M. L., S. Dib, J. Jerrim, M. Fewtrell, and R. E. Gilbert. 2020. Effect of long-chain polyunsaturated fatty acids in infant formula on long-term cognitive function in childhood: A systematic review and meta-analysis of randomised controlled trials. PloS One 15 (11):e0241800. doi: 10.1371/journal.pone.0241800.
- Vickers, M. H., J. Guan, M. Gustavsson, C. U. Krägeloh, B. H. Breier, M. Davison, B. Fong, C. Norris, P. McJarrow, and S. C. Hodgkinson. 2009. Supplementation with a mixture of complex lipids derived from milk to growing rats results in improvements in parameters related to growth and cognition. Nutrition Research (New York, N.Y.) 29 (6):426–35. doi: 10.1016/j.nutres.2009.06.001.
- Victora, C. G., R. Bahl, A. J. Barros, G. V. França, S. Horton, J. Krasevec, S. Murch, M. J. Sankar, N. Walker, and N. C. Rollins. 2016. Breastfeeding in the 21st century: Epidemiology, mechanisms, and lifelong effect. Lancet (London, England) 387 (10017):475–90. doi: 10.1016/S0140-6736(15)01024-7.
- Villamor-Martínez, E., M. Pierro, G. Cavallaro, F. Mosca, B. W. Kramer, and E. Villamor. 2018. Donor human milk protects against bronchopulmonary dysplasia: A systematic review and meta-analysis. Nutrients 10 (2):238. doi: 10.3390/nu10020238.
- Viriato, R., M. S. Queirós, G. A. Macedo, A. Ribeiro, and M. L. Gigante. 2020. Design of new lipids from bovine milk fat for baby nutrition. Critical Reviews in Food Science and Nutrition 62 (1):145–59. doi: 10.1080/10408398.2020.1813073.
- Vrijheid, M., M. Casas, M. Gascon, D. Valvi, and M. Nieuwenhuijsen. 2016. Environmental pollutants and child health: A review of recent concerns. International Journal of Hygiene and Environmental Health 219 (4–5):331–42. doi: 10.1016/j.ijheh.2016.05.001.
- Wallisch, A., L. M. Little, E. Dean, and W. Dunn. 2018. Executive function measures for children: A scoping review of ecological validity. OTJR: Occupation, Participation and Health 38 (1):6–14. doi: 10.1177/1539449217727118.
- Wang, B., P. McVeagh, P. Petocz, and J. Brand-Miller. 2003. Brain ganglioside and glycoprotein sialic acid in breastfed compared with formula-fed infants. The American Journal of Clinical Nutrition 78 (5):1024–9. doi: 10.1093/ajcn/78.5.1024.
- Wang, B., B. Yu, M. Karim, H. Hu, Y. Sun, P. McGreevy, P. Petocz, S. Held, and J. Brand-Miller. 2007. Dietary sialic acid supplementation improves learning and memory in piglets. The American Journal of Clinical Nutrition 85 (2):561–9. doi: 10.1093/ajcn/85.2.561.
- Wang, J., W. Xu, R. Wang, R. Cheng, Z. Tang, and M. Zhang. 2021. The outer membrane protein Amuc_1100 of Akkermansia muciniphila promotes intestinal 5-HT biosynthesis and extracellular availability through TLR2 signalling. Food & Function 12 (8):3597–610. doi: 10.1039/d1fo00115a.
- Wang, X., Y. Wang, J. Xu, and C. Xue. 2021. Sphingolipids in food and their critical roles in human health. Critical Reviews in Food Science and Nutrition 61 (3):462–91. doi: 10.1080/10408398.2020.1736510.
- Ward, E., N. Yang, B. S. Muhlhausler, G. E. Leghi, M. J. Netting, M. J. Elmes, and S. C. Langley-Evans. 2021. Acute changes to breast milk composition following consumption of high-fat and high-sugar meals. Maternal & Child Nutrition 17 (3):e13168. doi: 10.1111/mcn.13168.
- Ward, T. L., S. Hosid, I. Ioshikhes, and I. Altosaar. 2013. Human milk metagenome: A functional capacity analysis. BMC Microbiology 13:116. doi: 10.1186/1471-2180-13-116.
- Wechsler, D. 2014. Study citation. WISC-V: Technical and interpretive manual. Bloomington, MN: Pearson.
- Wechsler, D. 1967. WPPSI: Wechsler preschool and primary scale of intelligence. New York, N.Y.: Psychological Corporation.
- Wei, M., Z. Deng, B. Liu, W. Ye, Y. Fan, R. Liu, and J. Li. 2020. Investigation of amino acids and minerals in Chinese breast milk. Journal of the Science of Food and Agriculture 100 (10):3920–31. doi: 10.1002/jsfa.10434.
- Wells, J. C. 2003. Parent-offspring conflict theory, signaling of need, and weight gain in early life. The Quarterly Review of Biology 78 (2):169–202. doi: 10.1086/374952.
- Wesolowska, A., E. Sinkiewicz-Darol, O. Barbarska, U. Bernatowicz-Lojko, M. K. Borszewska-Kornacka, and J. B. van Goudoever. 2019. Innovative techniques of processing human milk to preserve key components. Nutrients 11 (5):1169. doi: 10.3390/nu11051169.
- Wesolowska, A., E. Sinkiewicz-Darol, O. Barbarska, K. Strom, M. Rutkowska, K. Karzel, E. Rosiak, G. Oledzka, M. Orczyk-Pawiłowicz, S. Rzoska, et al. 2018. New achievements in high-pressure processing to preserve human milk bioactivity. Frontiers in Pediatrics 6:323. doi: 10.3389/fped.2018.00323.
- Whitfield, K. C., S. Shahab-Ferdows, H. Kroeun, P. Sophonneary, T. J. Green, L. H. Allen, and D. Hampel. 2020. Macro- and micronutrients in milk from healthy Cambodian mothers: Status and interrelations. The Journal of Nutrition 150 (6):1461–9. doi: 10.1093/jn/nxaa070.
- Wigg, N. R., S. Tong, A. J. McMichael, P. A. Baghurst, G. Vimpani, and R. Roberts. 1998. Does breastfeeding at six months predict cognitive development? Australian and New Zealand Journal of Public Health 22 (2):232–6. doi: 10.1111/j.1467-842x.1998.tb01179.x.
- Williams, J. E., W. J. Price, B. Shafii, K. M. Yahvah, L. Bode, M. A. McGuire, and M. K. McGuire. 2017. Relationships among microbial communities, maternal cells, oligosaccharides, and macronutrients in human milk. Journal of Human Lactation 33 (3):540–51. doi: 10.1177/0890334417709433.
- Williamson, L. L., P. W. Sholar, R. S. Mistry, S. H. Smith, and S. D. Bilbo. 2011. Microglia and memory: Modulation by early-life infection. Journal of Neuroscience 31 (43):15511–21. doi: 10.1523/JNEUROSCI.3688-11.2011.
- Witkowska-Zimny, M, and E. Kaminska-El-Hassan. 2017. Cells of human breast milk. Cellular & Molecular Biology Letters 22:11. doi: 10.1186/s11658-017-0042-4.
- World Health Organization. 2003. Global strategy for infant and young child feeding. Geneva: World Health Organization.
- Wu, G., F. W. Bazer, T. A. Cudd, C. J. Meininger, and T. E. Spencer. 2004. Maternal nutrition and fetal development. The Journal of Nutrition 134 (9):2169–72. doi: 10.1093/jn/134.9.2169.
- Yaghoubfar, R., A. Behrouzi, F. Ashrafian, A. Shahryari, H. R. Moradi, S. Choopani, S. Hadifar, F. Vaziri, S. A. Nojoumi, A. Fateh, et al. 2020. Modulation of serotonin signaling/metabolism by Akkermansia muciniphila and its extracellular vesicles through the gut-brain axis in mice. Scientific Reports 10 (1):22119. doi: 10.1038/s41598-020-79171-8.
- Yaghoubfar, R., A. Behrouzi, A. Fateh, S. A. Nojoumi, F. Vaziri, S. Khatami, and S. D. Siadat. 2021. Effects of Akkermansia muciniphila and Faecalibacterium prausnitzii on serotonin transporter expression in intestinal epithelial cells. Journal of Diabetes and Metabolic Disorders 20 (1):1–5. doi: 10.1007/s40200-020-00539-8.
- Yam, K. Y., S. R. Ruigrok, I. Ziko, S. N. De Luca, P. J. Lucassen, S. J. Spencer, and A. Korosi. 2017. Ghrelin and hypothalamic NPY/AgRP expression in mice are affected by chronic early-life stress exposure in a sex-specific manner. Psychoneuroendocrinology 86:73–7. doi: 10.1016/j.psyneuen.2017.09.006.
- Yamazaki, K., A. Araki, S. Nakajima, C. Miyashita, T. Ikeno, S. Itoh, M. Minatoya, S. Kobayashi, F. Mizutani, Y. Chisaki, et al. 2018. Association between prenatal exposure to organochlorine pesticides and the mental and psychomotor development of infants at ages 6 and 18 months. The Hokkaido study on environment and children’s health. NeuroToxicology 69:201–8. https://doi.org/10.3390/ijerph15010120. doi: 10.1016/j.neuro.2017.11.011.
- Yarlagadda, A., E. Alfson, and A. H. Clayton. 2009. The blood brain barrier and the role of cytokines in neuropsychiatry. Psychiatry (Edgmont (PA : Township) 6 (11):18–22.
- Yeh, K. Y., M. Yeh, and P. R. Holt. 1989. Induction of intestinal differentiation by systemic and not by luminal corticosterone in adrenalectomized rat pups. Endocrinology 124 (4):1898–904. doi: 10.1210/endo-124-4-1898.
- Yunes, R. A., E. U. Poluektova, M. S. Dyachkova, K. M. Klimina, A. S. Kovtun, O. V. Averina, V. S. Orlova, and V. N. Danilenko. 2016. GABA production and structure of gadB/gadC genes in Lactobacillus and Bifidobacterium strains from human microbiota. Anaerobe 42:197–204. doi: 10.1016/j.anaerobe.2016.10.011.
- Zhang, L., M. de Waard, H. Verheijen, S. Boeren, J. A. Hageman, T. van Hooijdonk, J. Vervoort, J. B. van Goudoever, and K. Hettinga. 2016. Changes over lactation in breast milk serum proteins involved in the maturation of immune and digestive system of the infant. Journal of Proteomics 147:40–7. doi: 10.1016/j.jprot.2016.02.005.
- Zhang, J., A. Zhao, S. Lai, Q. Yuan, X. Jia, P. Wang, and Y. Zhang. 2021. Longitudinal changes in the concentration of major human milk proteins in the first six months of lactation and their effects on infant growth. Nutrients 13 (5):1476. doi: 10.3390/nu13051476.
- Zheng, L., M. Fleith, F. Giuffrida, B. V. O’Neill, and N. Schneider. 2019. Dietary polar lipids and cognitive development: A narrative review. Advances in Nutrition (Bethesda, MD) 10 (6):1163–76. doi: 10.1093/advances/nmz051.
- Zheng, J., S. Wittouck, E. Salvetti, C. Franz, H. Harris, P. Mattarelli, P. W. O’Toole, B. Pot, P. Vandamme, J. Walter, et al. 2020. A taxonomic note on the genus Lactobacillus: Description of 23 novel genera, emended description of the genus Lactobacillus Beijerinck 1901, and union of Lactobacillaceae and Leuconostocaceae. International Journal of Systematic and Evolutionary Microbiology 70 (4):2782–858. doi: 10.1099/ijsem.0.004107.
- Zimmermann, M. B. 2011. The role of iodine in human growth and development. Seminars in Cell & Developmental Biology 22 (6):645–52. doi: 10.1016/j.semcdb.2011.07.009.
- Ziomkiewicz, A., A. Apanasewicz, D. P. Danel, M. Babiszewska, M. Piosek, and M. Orczyk-Pawiłowicz. 2021a. Maternal distress and social support are linked to human milk immune properties. Nutrients 13 (6):1857. doi: 10.3390/nu13061857.
- Ziomkiewicz, A., M. Babiszewska, A. Apanasewicz, M. Piosek, P. Wychowaniec, A. Cierniak, O. Barbarska, M. Szołtysik, D. Danel, and S. Wichary. 2021b. Psychosocial stress and cortisol stress reactivity predict breast milk composition. Scientific Reports 11 (1):11576. doi: 10.1038/s41598-021-90980-3.
Appendix A.
Glossary.
16S Sequencing amplification of specific bacteria gene, 16S rRNA, by use of sequencing methodologies (Sanger, next generation sequencing) that allow the adequate bacterial phylogeny and taxonomy assignment. The 16S rRNA gene is commonly used for phylogenetic studiesas it is highly conserved between different bacterial species.
Available milk energy (AME) determined by the milk’s energy density (the kilocalories derived from protein, carbohydrate, and fat concentrations) and the amount of milk that mothers produce.
Colostrum is the first form of milk produced by the mammary glands immediately following delivery of the newborn. Colostrum has especially high amounts of bioactive compounds compared to mature milk. Specifically, colostrum contains antibodies to protect the newborn against disease and infection, and immune and growth factors, and other bioactives that help activate the newborn’s immune system, jumpstart the gut function, and seed a healthy gut microbiome in the first few days of life.
Culturomic approach is a high-throughput approach based on the isolation and grow of different microorganisms with different culture media and conditions.
Donor milk is defined as human milk expressed by a mother that is processed by a donor milk bank for use by a recipient that is not the mother’s own infant.
Eutherian mammals ‘Placental mammals’ (including humans) - represent the largest of three branches or clades of mammals. The others include Metatheria (Marsupials) and Prototheria (Monotremata). All eutherian mammals have a chorioallantoic placenta. In eutherian mammals, maternal energy invested in developing young prenatally (through the course of gestation via the placenta) and postnatally (via lactation and milk) is roughly equal. By contrast, marsupial gestation is brief and lactation is extended (Abbot and Capra Citation2017).
Fear reactivity Depicts propensity to express distress in response to sudden changes in stimulation or novel social or nonsocial stimuli.
Fore milk Milk produced toward the beginning of feeding.
Heavy metals Metals with relatively high densities, atomic weights, or atomic numbers. Within this large group, chromium, arsenic, cadmium, mercury, and lead have the greatest potential to pose a health threat on account of their extensive use and widespread distribution in the environment.
Hind milk Milk produced toward the end of feeding.
Human milk oligosaccharides (HMOs) a group of complex carbohydrates (sugars) that after lactose and lipids represent the third most abundant components of human milk and are thought to impact infant health and development through microbiome-dependent and -independent mechanisms.
Lactational Programming Aspects of the biobehavioral exchange between mother and baby, including nursing patterns and the communication of both nutrient and signaling molecules in milk, through which offspring development is orchestrated.
Lactocrine A mechanism through which milk-borne bioactive factors are delivered from mother to offspring by consequence of nursing.
Lactocrine Programming Effects of lactocrine-active factors (e.g., milk-borne bioactive factors) on the developmental program of cells, tissues and organs in nursing offspring that have lasting effects on form, function and/or health and well-being in adulthood.
Lactome The entire set of genes that contribute to production of milk.
Life-history theory Life-history theory describes the basic principle for a living organism in maximizing its fitness and reproduction throughout life in diverse environmental conditions, by making decision for energy or resources allocation used for one over the another life functions (growth, reproduction, storage and maintenance (including repair)).
Marsupial mammals Marsupialia – are a distinct class of mammals endemic to Australasia and the Americas. Included in this group are kangaroos, wallabies, koalas and opossums. Relative to eutherian mammals, marsupial mammals display short gestational periods and comparatively longer lactational periods during which a great deal of offspring development occurs supported by lactation and milk (Abbot and Capra Citation2017).
Mastitis is inflammation of the breast or udder, usually associated with breastfeeding. Symptoms typically include fever, local pain and redness. Onset is typically fairly rapid and usually occurs within the first few months of delivery.
Mature milk Milk produced around the later stages of lactation (around 3–4th week of postpartum), once lactation has become fully established.
Metagenomics is the analysis of the total DNA recovered from a milk sample by use of next generation sequencing
Microchimerism The presence of somatic cells within one individual that originate from another, genetically distinct individual. Maternal microchimerism can occur through lactocrine transmission of maternal somatic cells to nursing offspring.
Milk bioactives (milk-borne bioactive factors) Non-nutrient components in human milk that comprised immune components, hormones, naturally occurring opiates, enzymes and many other active molecules.
Moiety A functional subgroup of a molecule.
Monotreme mammals Monotremata –’egg-laying mammals’– include the echidna and duck-billed platypus (Abbot and Capra Citation2017).
Multi-lineage potential The property of (embryonic and/or adult) stem cells to develop into different types of cells depending upon micro-environmental conditions as defined in vitro or in vivo.
Negative emotionality One of the three main dimensions of temperament, which describes individual differences in predisposition to experience negative emotions (such as sadness, frustration and fearfulness), and includes the threshold, intensity, and duration of emotions (Rothbart Citation2007).
Next generation sequencing is the technology that allow a massively parallel sequencing of DNA and RNA from different samples.
Parent-offspring conflict The conflict arising between parents and offspring regarding investment of resources (including time and effort) given divergent investment optima for maximizing fitness (Trivers Citation1974).
Pasteurization is a process in which milk is usually treated with mild heat to eliminate pathogens and extend shelf life.
Persistent organic pollutants (POPs) Chemical compounds resistant to environmental degradation through chemical, biological, and photolytic processes, and frequently used as pesticides, solvents, pharmaceuticals, and industrial chemicals.
Polymerase Chain Reaction (PCR) is a technique used to "amplify" specific segments of DNA such us 16S rRNA regions or whole gene.
Prebiotic Non-digestible compounds in food (usually consisting of sugars, fibers, and carbohydrates) that induce the growth or activity of microorganisms such as bacteria and fungi.
Probiotic Live microorganisms that, when consumed in adequate amounts, confer a health benefit on the host organism.
Transitional milk produced after colostrum, starting about 2–5 days post-delivery, and present mature milk is produced.
WEIRD populations Populations that come from Western, Educated, Industrialized, Rich, and Democratic societies.
Appendix B.
Abbreviations in the text
Ara (arachidonic acid)
AME (available milk energy)
BMI (body mass index)
DHA (docosahexaenoic acid)
DNA (Deoxyribonucleic acid)
GCs (glucocorticoids)
HMOs (human milk oligosaccharides)
HPA axis (hypothalamic-pituitary-adrenal axis)
Ig (immunoglobulin)
IQ (intelligence quotient)
LC-PUFA (long-chain polyunsaturated fatty acids)
Lf (lactoferrin)
MbFs (milk-borne bioactive factors)
MFGM (milk fat globule membranes)
RCT (randomized controlled trial)
PCR (polymerase chain reaction)
POPs (persistent organic pollutants)
Box 1. Human milk composition: the basics
Human milk composition: the basics
Given the importance of human milk for infants and its long-term health benefits, significant research has been performed to understand the complexities of human milk and the factors modulating its composition (e.g., stage of lactation). Depending on the stage of lactation, human milk is most often differentiated into three categories: colostrum, transitional milk, and mature milk. Colostrum is the first fluid produced by mammary glands immediately after birth, and it provides high concentrations of essential nutrients (i.e., proteins, lipids, carbohydrates and micronutrients). Colostrum also contains many other components including immunologic factors, growth factors, and signaling peptides. Colostrum is known to carry different commensal bacteria (including species from the Lactobacillus and Bifidobacterium genuses), that have been recognized for helping to establish and shape the infant’s immune system and to develop a healthy microbiome (Fernández et al. 2020; Neville Citation2001). As breastfeeding continues, the immunoglobulins and protein/nitrogen concentrations decrease, changing colostrum into transitional milk, present from day 7 to 14. At around 2 weeks postpartum human milk changes from transitional to mature milk (Ballard and Morrow Citation2013; Ruiz et al. Citation2019).
In contrast to colostrum and transitional milk, mature milk exhibits less compositional variability. However, it is thought that more subtle, but possibly biologically relevant, changes occur over the remainder of lactation (Ballard and Morrow Citation2013). Mature milk composition varies over the course of the day. For example, the concentration of fats and hormones (e.g., cortisol, melatonin) changes significantly during the 24-hour period (see ). Also, the composition of mature milk composition varies within a feed. As such, mature milk is categorized into fore milk - produced toward the beginning of a feeding-- and hind milk -- produced toward the end of a feeding, and containing possibly 2–3 times higher fat than fore milk (Bishara et al. Citation2009). Note that maternal and child factors, as well as the interplay between both, play an important role in dictating the composition of milk (see Maternal factors, Child factors, and Interplay of maternal-child factors sub-sections), and that variations over the course of lactation, the course of the day, and/or across feeds have not yet been studied for all milk components.
A way of characterizing overall human milk composition is by the Available Milk Energy, or AME, that refers to the energy output of human milk. On average, human milk contains 0.62 kcal/ g (2.6 kJ/g) energy (Reilly, Ashworth, and Wells Citation2005). The main source of energy in human milk is fat (see ). Mature milk contains more energy than colostrum (Gidrewicz and Fenton Citation2014). It was believed at one point that milk energy content was relatively stable during the first year of lactation (Nommsen et al. Citation1991; Saarela, Kokkonen, and Koivisto Citation2005). However, there is preliminary evidence that mothers who breastfeed for over one year produce milk with higher fat and energy content (Mandel et al. Citation2005). Due to the changes in fat content discussed above, day and evening samples are more energy dense than night or morning samples (Moran-Lev et al. Citation2015; Paulaviciene et al. Citation2020). Moreover, energy content changes during the feed as hind milk contains more energy than fore milk (Saarela, Kokkonen, and Koivisto Citation2005).
Human milk energy content is affected by pregnancy duration (Mills et al. Citation2019), and by child characteristics as well as maternal factors (see Factors affecting milk constituents section). In comparison to a non-pregnant woman, a breastfeeding woman requires approximately 500 more kcal/day. The total volume of milk produced by a mother is nearly 780 mL/day (range 450–1200 mL/day), and the energy content of the milk ranges between 60 and 70 kcal/100 mL. Therefore, a breastfeeding mother who uses her nutrient stores will lose 0.5–1.0 kg/month after the first postpartum month (Kominiarek and Rajan Citation2016).