Abstract
Fusarium and Neocosmospora are two fungal genera recently recognized in the list of fungal priority pathogens. They cause a wide range of diseases that affect humans, animals, and plants. In clinical laboratories, there is increasing concern about diagnosis due to limitations in sample collection and morphological identification. Despite the advances in molecular diagnosis, due to the cost, some countries cannot implement these methodologies. However, recent changes in taxonomy and intrinsic resistance to antifungals reveal the necessity of accurate species-level identification. In this review, we discuss the current phenotypic and molecular tools available for diagnosis in clinical laboratory settings and their advantages and disadvantages.
Keywords:
Introduction
Fusarium and Neocosmospora (formerly Fusarium solani species complex) are recognized as filamentous fungi that infect humans, animals, and plants. The World Health Organization (WHO) has recently recognized Fusarium in the list of fungal priority pathogens due to its fatality rates (30 days) ranging from 43 to 67%, moderate accessibility to diagnosis, and intrinsic resistance to most antifungal drugs (WHO Citation2022). A key aspect of fusariosis in a wide sense is the early detection of invasive infections, which is crucial for survival rates (van Diepeningen et al. Citation2015). In recent years, there has been increasing interest in developing point-of-care approaches for early diagnosis, including molecular and omics techniques (Perfect Citation2013; Kidd et al. Citation2019; Mendonça et al. Citation2022). However, there is rising concern about mold identification in clinical laboratories because traditional microscopy and culture diagnoses may require staff with relatively high degrees of specialized mycology training (Backx et al. Citation2014). In addition, morphological approaches often do not allow precise distinction at the species level, even when trained professionals perform evaluations. This occurs due to factors, such as high morphological homoplasy, cryptic speciation processes, and the great phenotypic plasticity observed in many fungi, including fusarioids (Chethana et al. Citation2021; Crous et al. Citation2021; Yilmaz et al. Citation2021; Steenwyk et al. Citation2023). Nevertheless, training and teaching of medical mycology face great challenges, for example, most of the medical schools dedicate a maximum of two weeks to teaching medical mycology in their curricula. Furthermore, postgraduate medical students and medical microbiologists are also provided with limited instruction in this field.
Recognition as a priority fungus requires new surveillance data that will be possible with an adequate diagnosis from the clinical laboratory. On the other hand, molecular testing is expensive in low- to middle-income nations, and sequencing is frequently needed for definitive identification (Hoenigl et al. Citation2021). This paper aims to review the phenotypic and molecular tools available for the laboratory diagnosis of Fusarium and Neocosmospora.
Taxonomy and clinical importance
Fusarium and Neocosmospora are two genera of fungi belonging to the family Nectriaceae and are part of a fungal group called the “Terminal Fusarium Clade” (TFC). To date, there has been little agreement on the taxonomy of this group (O’Donnell et al. Citation2020; Crous et al. Citation2021). This controversy is divided into two proposals: the TFC comprises the genus Fusarium sensu lato (which means the name Neocosmospora should not be used) (O’Donnell et al. Citation2020), and the TFC is composed of 10 recognized genera, including Fusarium and Neocosmospora (Crous et al. Citation2021). In this review, we will adhere to the taxonomy nomenclature proposed by Crous et al. (Citation2021) because it is based on phylogenetic relationships as well as phenotypic and ecological characteristics of species in this group. It was also recently accepted as an appropriate nomenclature by Crous et al. (Citation2021) and Kidd et al. (Citation2023).
These genera have clinical relevance, causing a wide range of diseases in humans, including superficial infections, such as keratitis and onychomycosis, as well as locally invasive and disseminated diseases (). Epidemiologically, there are more cases of keratitis caused by species of Neocosmospora compared to Fusarium (Al-Hatmi, Hagen, et al. Citation2016; Muraosa et al. Citation2017; Walther et al. Citation2017; Herkert et al. Citation2019; Huang et al. Citation2022), except for one report in Iran (Najafzadeh et al. Citation2020) and another in the Netherlands (Oliveira dos Santos et al. Citation2020). The main causal agents of this pathology are Neocosmospora falcifomis (formerly Fusarium falciforme) and Neocosmospora keratoplastica (formerly Fusarium keratoplasticum) (Al-Hatmi, Hagen, et al. Citation2016; Muraosa et al. Citation2017; Walther et al. Citation2017; Huang et al. Citation2022). Regarding disseminated diseases, there is a similar prevalence between the genera as keratitis (Muraosa et al. Citation2017; Herkert et al. Citation2019; Rosa et al. Citation2019). In contrast, for Onychomichosis, Fusarium species associated with the Fusarium fujikuroi species complex (FFSC) and Fusarium oxysporum species complex (FOSC) are more prevalent than Neocosmospora (Al-Hatmi, Hagen, et al. Citation2016; Muraosa et al. Citation2017; Najafzadeh et al. Citation2020). These data show the difference in epidemiology between these two genera. However, these data correspond to active surveillance in specific hospitals and countries. Therefore, the global epidemiology of these genera is still unknown due to the lack of available diagnostic tools and epidemiology surveillance in most countries.
Figure 1. Most common species of Fusarium and Neocosmospora isolated in human diseases. *Mycetoma has poor reports in this group. Data obtained from (Al-Hatmi, Hagen, et al. Citation2016; Guevara-Suarez et al. Citation2016; Muraosa et al. Citation2017; Walther et al. Citation2017; Rosa et al. Citation2019; Herkert et al. Citation2019; Najafzadeh et al. Citation2020; Oliveira dos Santos et al. Citation2020; Huang et al. Citation2022).
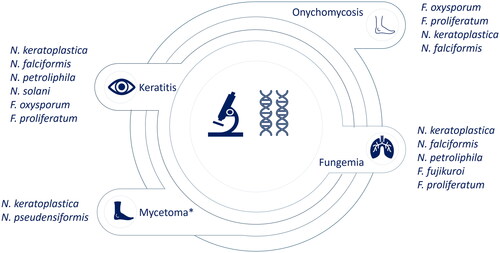
Furthermore, species-specific in vitro susceptibility patterns, particularly against voriconazole, have been described. For example, voriconazole patterns in Neocosmospora solani (formerly F. solani) and N. keratoplastica from disseminated diseases, onychomycosis and keratitis are generally greater, with MICs (minimum inhibitory concentrations) ranging between 4 and 16 µg/mL, than those of the FFSC, with MICs ranging between 0.5 and 8 µg/mL (Al-Hatmi, Meis, et al. Citation2016; Oliveira dos Santos et al. Citation2020). A similar pattern is shown for posaconazole; N. solani and N. keratoplastica have MICs >16 µg/mL, and FFSC has MICs ranging between 0.25 and >16 µg/mL (Al-Hatmi, Meis, et al. Citation2016; Oliveira dos Santos et al. Citation2020). Additionally, the amphotericin B MICs of N. keratoplastica have been reported to be higher (0.5–16 µg/mL) than those (0.5 and 4 µg/mL) of the closely related species Neocosmospora petroliphila (formerly Fusarium petroliphilum) (Herkert et al. Citation2019; Rosa et al. Citation2019; Oliveira dos Santos et al. Citation2020). Recent data report an MIC50 of >16 µg/ml for most species complexes in isavuconazole activity, showing no relevant difference between species (Broutin et al. Citation2020). Natamycin is the most effective antifungal in N. solani keratitis isolates (Rosa et al. Citation2019). However, a relationship between MIC and mortality has not been found (Nucci et al. Citation2021). On the other hand, in terms of human pathogenicity, N. solani has been reported to be more resistant to human phagocyte attack, while F. oxysporum is more sensitive to phagocytosis (Winn et al. Citation2003). These data demonstrate the need for species-level identification to generate more accurate data on the susceptibility of species to antifungals. This will be useful for making future therapeutic decisions based on previous knowledge of antifungal susceptibility.
Phenotypic tools
Specimen collection
For a successful laboratory diagnosis of fungi, medical personnel and nurses must collect and transport the specimens properly. Like other infectious disorders, the active infection site yields the best sample for identifying the causal agent. For example, Fusarium and Neocosmospora species are usually isolated from cultures of nail scrapes (onychomycosis), corneal scrapes (keratitis), skin biopsies (skin lesions), sinus aspirates (sinusitis), respiratory secretions (pneumonia), and blood cultures (fungemia, disseminated disease) (van Diepeningen et al. Citation2015).
Nevertheless, fungal keratitis sample collection remains a challenge due to the limited sample volume, bedside specimen inoculation, and limited ocular anatomy/pathophysiology training in clinical microbiology laboratories (Leal et al. Citation2021). These limitations suggest that the ophthalmologist perform a corneal scrape using a Kimura/platinum spatula and inoculate the sample directly on agar using a “C” pattern () (Sharma Citation2012; Leal et al. Citation2021).
Figure 2. Growth on “C” pattern of Neocosmospora solani on potato dextrose agar after 48 h incubation at 25 °C.
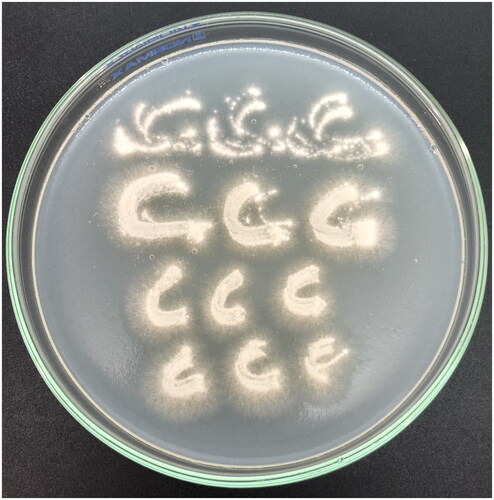
The utilization of in vivo confocal microscopy (IVCM) has been proposed as a valuable technique for visualizing filamentous fungi in the cornea, given the complexity associated with diagnosing fungal keratitis. However, it is crucial to acknowledge that despite variations in hyphal diameters between Aspergillus spp. (3–10 m) and Fusarium spp. (3–5
m), these discrepancies lack clinical significance and cannot reliably differentiate between the two genera (Tabatabaei et al. Citation2018, Citation2020). Furthermore, there are no observable variations in the branching angles. As a result, IVCM can be used to evaluate the effectiveness of treatment by conducting regular follow-ups and monitoring changes in hyphal density (Tabatabaei et al. Citation2020). This could serve as an indicator to predict the favorable response of fungal ulcers to treatment. However, while IVCM allows for swift diagnosis and initiation of treatment based on the identification of fungal structures, it must be complemented by fungal culture, which remains the gold standard in fungal keratitis.
Hence, our suggestion is for the ophthalmology services, in collaboration with the clinical microbiology services, to develop guidelines for the collection of cornea eye sampling via Kimura spatula. Additionally, as previously stated, it is the ophthalmologists who should be responsible for inoculating the corneal scrapings on agar plates.
Direct examination
Potassium hydroxide (KOH) is a direct procedure used to examine specimens. This alkaline solution degrades keratin, improving the visualization of fungal structures in the skin, nails, and tissues (Dasgupta and Sahu Citation2012). When observed directly in tissues using KOH 10–20%, septate and hyaline branched hyphae of Fusarium and Neocosmospora are frequently observed at 45-degree angle (Liu et al. Citation1998). Data from a meta-analysis indicate that the sensitivity of KOH in nails for the diagnosis of onychomycosis was 95% (95% CI = 94–97; I2 = 93.8%) (Velasquez-Agudelo and Cardona-Arias Citation2017). KOH performed from corneal scrapings is a straightforward procedure with sensitivity dependent on country epidemiology and laboratory expertise. Several studies show sensitivity ranges from 60 to 90% (Sharma et al. Citation2002; Chatterjee Citation2007; Rathi et al. Citation2017; Hoffman et al. Citation2022). The test performs better when the corneal ulcer is large (>2 mm), suggesting that the amount of sample available for processing may be the cause of the difference in sensitivity (Bharathi et al. Citation2006; Hoffman et al. Citation2022).
A fluorescence microscope clarifies cell debris and colors fungal structures bright blue–white or green when in contact with clinical samples (Weinberg et al. Citation2003). Sample staining is possible using stains, such as Calcofluor White (CFW), a bleaching agent that binds to cellulose and chitin in fungal cell walls. CFW is a more sensitive (92%) technique than KOH in diagnosing nondermatophyte onychomycosis (Weinberg et al. Citation2003). In addition, according to previous reports, CFW has a high sensitivity (97%) and is a well-established stain for identifying fungal components in corneal scrapings (Sharma et al. Citation2002; Zhang et al. Citation2010; Hoffman et al. Citation2022). The rate of accurate diagnosis of fungal keratitis is increased due to the combination staining method of KOH and CFW (Sharma et al. Citation2002; Bacorn et al. Citation2022). Nevertheless, based on our observations, some clinical laboratories that handle corneal samples do not employ these methods in processing corneal scrapings. As a result, we strongly advocate for their implementation and the training of laboratory staff in fungal keratitis. Direct examination of clinical samples does not allow recognition of the type of fungus, and, as a result, culture is needed for specific diagnosis.
Fungal culture
Fungal culture is crucial for diagnosing fungal infections. Therefore, it is necessary to obtain a fungus culture from skin biopsies and other affected tissues (such as sinuses, lungs, and blood) to diagnose invasive Fusarium and Neocosmospora infections. Culture also enables antifungal susceptibility testing (Backx et al. Citation2014; Rathi et al. Citation2017). Furthermore, blood cultures yield positive results in 40% of invasive cases, with fungal blood culture bottles detecting growth more faster than conventional aerobic bottles (Hoenigl et al. Citation2021).
Fusarium and Neocosmospora species can, in general, quickly recover in standard mycological medium without special growing conditions. Potato dextrose agar (PDA) is strongly recommended. In PDA, most Fusarium and Neocosmospora species grow in ∼5–7 days at 25–28 °C without potentially inhibiting cycloheximide (Nelson et al. Citation1994; Crous et al. Citation2021). Although fusarioid isolates thrive on most mycological media, the medium can significantly impact the colony’s morphology, color, and conidium development (Nelson et al. Citation1994; Crous et al. Citation2021). Moreover, based on our expertise, the growth and pigment production of a fusarioid isolate cultivated on various PDA agar brands can vary, thus posing challenges in the phenotypic identification. Regarding clinical practice, the unavailability of commercially accessible PDA agar in some countries like Colombia poses a challenge for clinical laboratories, hindering the morphological colony characterization.
The microscopic characteristic feature of Fusarium and Neocosmospora species is the production of banana-shaped macroconidia. However, macroconidia are not produced by all fusarioid species. Other important characteristics include (1) the shape and septation of microconidia and macroconidia; (2) the shape of phialides; (3) the number of openings on the phialides (monophialides or polyphialides); (4) the formation of conidia in heads or chains; and (5) the presence and arrangement of chlamydospores (Nelson et al. Citation1994; Crous et al. Citation2021). Despite these phenotypic characteristics, molecular analysis is necessary because morphology alone is unreliable for species identification. For example, species may differ from each other only by the number of septa in macroconidia or the characteristics of microconidia and chlamydospores. In certain cases, even individuals with a wealth of knowledge and experience may encounter difficulties when attempting to accurately differentiate between species, particularly in those two fusarioid genera.
In , we summarize the main phenotypic features of the most common clinical species of Fusarium and Neocosmospora. Some of the aforementioned microscopic features are illustrated in .
Figure 3. Neocosmospora and Fusarium clinical species. (A–C) N. solani: conidiophores, macro- and microconidia, and chlamydospores, respectively. (D–F) N. keratinoplastica: macro, microconidia, and chlamydospores, respectively. (G,H) N. falciformis: macroconidia and chlamydospores, respectively. (I) F. oxysporum: monophialides producing microconidia. (J) F. fujikuroi: Mono-, polyphialides, and microconidia. (K,L) F. verticillioides: monophialides producing conidia in chains. Scale bars: (A–L) = 10 µm.
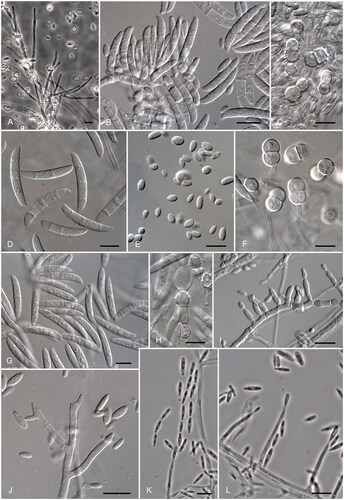
Table 1. Phenotypical characteristics of some clinically relevant species of Fusarium and Neocosmospora.
To facilitate morphological identification, a Web tool (https://www.fusahelp.com) has recently been developed, which is available on any device (desktop computer, tablet, mobile phone) (Infantino et al. Citation2023). This tool helps the user to identify Fusarium and Neocosmospora based on their morphological characteristics. However, this tool has some limitations. Firstly, it has a limited species coverage, encompassing only 83 species, primarily focused on plant pathogens rather than clinically relevant ones. Secondly, it lacks taxonomic updates. It includes species complexes designated by sensu lato (in the broad sense) that have recently been reclassified into multiple distinct species. For example, the tool includes Fusarium oxysporum sensu lato without acknowledging the newly described species within this complex. Thirdly, the tool incorporates Neocosmospora under Fusarium solani sensu lato, making it unsuitable for Neocosmospora species-level identification. Despite these disadvantages, this tool could be considered a useful instrument in the training of clinical laboratory personnel.
Histopathology
By displaying tissue invasion, angioinvasion, and inflammatory response, histopathology has the advantage of providing diagnostic significance of positive cultures to distinguish contamination, colonization, or true infection. Tissue biopsy with culture and histopathology provides definitive evidence of invasive disease (Guarner and Brandt Citation2011; Challa and Sistla Citation2022). Biopsies can be stained with hematoxylin and eosin (HE), Periodic Acid-Shiff (PAS), and Gomori methenamine silver (GMS) (Challa and Sistla Citation2022). Fusarium and Neocosmospora present acute-angle, hyaline and septate hyphae; Fusarium and Neocosmospora hyphae resemble Aspergillus hyphae in appearance. They differ in that fusarioid hyphae are randomly branched with regular contours or varicosities. The presence of constrictions at the site of septa, hyphal varicosities, and terminal or intercalated vesicles is a helpful, although not pathognomonic, indicator of the identity of fusarioid species (Guarner and Brandt Citation2011; Challa and Sistla Citation2022).
Antigen test
Currently, no commercial system is available to detect specific antigens secreted by Fusarium or Neocosmospora species during infection. Using tissue samples from patients with IFI (invasive fungal infections) caused by Fusarium, the diagnostic value of polyclonal fluorescent antibody reagents was evaluated. However, significant cross-staining was observed with sections containing Aspergillus and Scedosporium boydii (Kaufman et al. Citation1997).
Due to its presence in the blood of patients with IFI and its ability to activate factor G of the coagulation cascade, (1,3)-b-D-glucan (BDG) can be detected and quantified (Theel and Doern Citation2013). For IFI detection, the BDG test in Fusarium differs significantly. For instance, it can be found in patients with pneumonia and fungemia, but because of its cross-reaction with Aspergillus, its presence is not a marker for fusariosis (Odabasi et al. Citation2004). However, most patients with invasive fusariosis have BDG positivity (sensitivity of 85% and specificity of 69%), often before the first clinical symptom and diagnosis of the disease. Once treatment has begun, dropping BDG readings indicates a positive response (Nucci et al. Citation2019). Unfortunately, false positive results can also occur in patients with renal failure using immunoglobulin products (Nucci et al. Citation2019).
Similarly, Aspergillus and Fusarium/Neocosmospora have cross-reactivity in galactomannan (GM) assays; a positive result cannot assist medical professionals in making a precise diagnosis (Tortorano et al. Citation2012). A recent systematic review and meta-analysis revealed that the serum GM test exhibits a sensitivity of 76% and a specificity of 92% in individuals diagnosed with invasive pulmonary aspergillosis (Bukkems et al. Citation2023). However, the interpretation of this diagnostic tool becomes challenging when applied to patients with IFI. For example, individuals with hematologic conditions who have invasive fusariosis can produce positive GM test results despite the absence of Aspergillus spp. (Tortorano et al. Citation2012). Furthermore, the GM test can yield positive results even before the diagnosis of invasive fusariosis by positive culture or histopathology (Nucci and Anaissie Citation2023).
Therefore, the presence of septate acute branched hyphae in tissue, a fungal culture, and a positive BDG or GM test should be carefully interpreted based on the clinical context and the prevalence of fusariosis in the health center (Tortorano et al. Citation2012; Nucci and Anaissie Citation2023). This is because even when probable invasive aspergillosis is diagnosed, the patient can have fusariosis, as recently demonstrated by using Universal Digital High Resolution Melting Analysis (U-dHRM). By utilizing machine learning, U-dHRM employs digital technology to detect pathogens at the single genome level. Interestingly, U-dHRM has shown a higher frequency of detecting Fusarium/Neocosmospora in positive GM samples that did not exhibit the growth of Aspergillus spp. in culture (Goshia et al. Citation2024).
In this section, we have outlined the advantages and disadvantages of the most utilized phenotypic tools for identifying fusarioid genera in clinical settings. While phenotypic diagnosis may require the application of various techniques, such as direct examination, culture, histopathology, and antigen tests, we advocate for the comprehensive use of all available tools to facilitate prompt diagnosis and timely treatment. Clinical laboratories are recommended to conduct cultures to allow antifungal susceptibility testing, followed by molecular tests for species identification. The availability of antifungal susceptibility and species data is crucial for effective fusarioid surveillance.
Molecular tools
PCR
Polymerase chain reaction (PCR) is a molecular tool that helps to correctly identify different microorganisms based on DNA markers (Khot and Fredrick Citation2009; Lass-Flörl et al. Citation2013; Liu et al. Citation2019). This technique has been used primarily for diagnosing IFI and not superficial infections. One of its advantages is that it reduces the time to diagnosis (Liu et al. Citation2019). The second advantage is that this technique does not require a purified culture because some variants of this technique could be made in clinical specimens, specifically from primary sterile body sites (Rahn et al. Citation2016; Springer et al. Citation2019; Scharf et al. Citation2021; Dellière et al. Citation2022). Third, it allows for more accurate identification than phenotypic tools by selecting an appropriate DNA marker (Chethana et al. Citation2021; Crous et al. Citation2021; Steenwyk et al. Citation2023). On the other hand, one disadvantage is that a set of specific primers is available only for a limited list of causative agents. Another disadvantage is the relatively high cost of the technique (Liu et al. Citation2019).
Some of the DNA markers used for fungal identification are the internal transcribed spacer (ITS) regions, including the 5.8S gene, both the large (LSU; 28S) and small (SSU, 18S) subunits of the nuclear ribosomal RNA genes, as well as protein-encoding genes, such as cytochrome oxidase subunit 1 (COX1, COI), the largest subunit of RNA polymerase II (RPB1), the second largest subunit of RNA polymerase II (RPB2), β-tubulin (TUB2), translational elongation factor 1-α (TEF1-α), γ-actin and calmodulin (CaM) (Tekpinar and Kalmer Citation2019). Sadly, no gene marker globally helps to identify all fungi at the species level. In clinical diagnosis, some markers used are LSU, SSU, and ITS (Khot and Fredrick Citation2009; Lass-Flörl et al. Citation2013). Unfortunately, none of the ribosomal markers have been proven to identify Fusarium and Neocosmospora at the species level. The reported markers that have a great species identification are TEF1-α and RPB2 (Crous et al. Citation2021). However, in our experience, TEF1-α have some difficulties in identifying some Neocosmospora species and RPB2 has a low PCR successful rate making it unlikely to be used in routine laboratory diagnosis. Single- or multi-locus identification could be performed on the FUSARIOID-ID database (www.fusarium.org/), which is a curated database of fusarioids.
Some studies have designed specific PCR assays to detect Fusarium and Neocosmospora from clinical specimens. Unfortunately, since these assays only detect one fungus, they probably cannot be used as a routine clinical diagnosis tool. In 2019, Springer et al. designed a probe-based real-time PCR using the LSU marker and a locked nucleic acid (LNA) probe (Springer et al. Citation2019). They proved that their technique could detect Fusarium and Neocosmospora DNA from clinical specimens, specifically bronchoalveolar lavage fluid (BALF). However, they recommend validating positive samples by sequencing (Springer et al. Citation2019). Dellière et al. (Citation2022) designed another real-time PCR assay of the LSU marker that shows high sensitivity (93% for blood and 100% for tissue) and specificity (100% for the two types of samples) in detecting Fusarium and Neocosmospora from blood and tissue samples (Dellière et al. Citation2022).
Other assessments designed a set of real-time PCR assays to detect 14 genera of fungi, including Fusarium and Neocosmospora (Rahn et al. Citation2016; Scharf et al. Citation2021). They demonstrate the technique in clinical specimens, such as respiratory washes, respiratory swabs, whole blood anticoagulation with EDTA, and blood serum with significant detection (Rahn et al. Citation2016; Scharf et al. Citation2021). The major advantage of these tests (Rahn et al. Citation2016; Springer et al. Citation2019; Scharf et al. Citation2021; Dellière et al. Citation2022) is the fast detection of the fungal pathogen, which allows rapid empirical treatment selection. However, since the studies were designed with the previous fungal nomenclature, these assays cannot differentiate between Fusarium and Neocosmospora (Rahn et al. Citation2016; Springer et al. Citation2019; Scharf et al. Citation2021; Dellière et al. Citation2022). Additionally, these assays cannot identify the pathogen at the species level (Rahn et al. Citation2016; Springer et al. Citation2019; Scharf et al. Citation2021; Dellière et al. Citation2022), so other techniques should be nested to gain this knowledge.
Previous studies have reported the efficiency of PCR techniques in diagnosing IFI, including the diagnosis of Fusarium and Neocosmospora. These results suggest that this technique could be used in a routine clinical diagnosis of IFI caused by these pathogens. One of the main problems is that current protocols are only developed to diagnose at the genus level and do not differentiate between Fusarium and Neocosmospora species (Rahn et al. Citation2016; Springer et al. Citation2019; Scharf et al. Citation2021; Dellière et al. Citation2022). As mentioned, species-level diagnosis is relevant for empirical treatment choices and epidemiological characterization. The future development of PCR assays should focus on the species-level diagnosis of these pathogens and other relevant fungal pathogens.
Next generation sequencing (NGS)
The availability of NGS technologies is an excellent tool for the laboratory, including fungal diagnosis. There are three possible uses of NGS in the clinical laboratory: whole genome sequencing (WGS), targeted NGS (tNGS), and metagenomic NGS (mNGS) (Mitchell and Simner Citation2019). The main advantages of tNGS and mNGS are that they do not require a fungal culture, which could improve and reduce the time for identification. However, the cost could be a disadvantage in lower- and middle-income countries. Another disadvantage of mNGS is the poor data availability for less common pathogens, and it requires highly trained staff to perform the analysis (Mitchell and Simner Citation2019).
There have been reports of Fusarium and Neocosmospora being successfully identified by mNGS or tNGS. For example, there is a case report in Japan that identified Neocosmospora as the pathogen causing fungal keratitis using multiple techniques, including mNGS (Shigeyasu et al. Citation2018). However, based on the information available, we consider that the species was not successfully identified (identified as N. solani) (Shigeyasu et al. Citation2018). On the other hand, one study identified fungal pathogens in fungal stain-positive formalin-fixed, paraffin-embedded tissues using tNGS (Larkin et al. Citation2020). This study performed tNGS with ITS as a marker and identified four cases of Neocosmospora infection (Larkin et al. Citation2020), but as we previously described, ITS is not a good gene marker for the identification of Neocosmospora at the species level (Crous et al. Citation2021). A study that performed mNGS on BALF samples from patients with lower respiratory tract infections (LRTIs) identified one probable infection of F. oxysporum and one probable infection of F. verticillioides that were not obtained successfully by traditional culture (Chen et al. Citation2020). Another study identified F. oxysporum on aqueous humor in a patient with infectious uveitis using Nanopore mNGS but did not obtain a positive culture (Lee et al. Citation2023). These results show the relevance of mNGS when traditional microbiology fails.
Even when mNGS has been increasingly used, we consider that there is a disadvantage in the diagnosis of Neocosmospora, as shown in a previous example (Shigeyasu et al. Citation2018). This approach requires an available genome to compare and identify the pathogen (Shigeyasu et al. Citation2018; Chen et al. Citation2020). However, until 23 February 2024, only the genomes of the clinically relevant species Neocosmospora metavorans, N. solani, N. keratoplastica, and N. falciformis were available in the NCBI assembly database (https://www.ncbi.nlm.nih.gov/assembly). Yet, some of these genomes are labeled with outdated species names, posing a challenge for non-expert laboratory personnel to accurately identify and interpret them. Therefore, there is no genome of N. petroliphila that is relevant in fungemia and keratitis or N. pseudensiformis that is relevant in mycetoma (). There is no available genome of other Neocosmospora pathogens that are less frequent, such as Neocosmospora gamsii, Neocosmospora catenata, Neocosmospora suttoniana, and Neocosmospora tonkinensis (Sandoval-Denis and Crous Citation2018). The absence of these data could make it difficult to identify Neocosmospora species and could lead to the misinterpretation of results. Therefore, we propose that sequencing the genomes of clinically relevant Neocosmospora species could improve the species-level identification of this genus in the clinical laboratory that has already performed this technique.
MALDI-TOF MS
Matrix-assisted laser desorption ionization-time of flight mass spectrometry (MALDI-TOF MS) is a technique that helps identify microorganisms through a mass spectrum of the most abundant proteins and other biomarkers (Hou et al. Citation2019; Patel Citation2019; Gómez-Velásquez et al. Citation2021). The main advantage of this technique is the rapid and reproducible identification of microorganisms (Gómez-Velásquez et al. Citation2021). One of the main limitations of this method is the lack of or limited representations of some microorganisms in the databases used for identification. These databases could be commercial or developed by users (in-house), and the database selection could alter the results obtained (Hou et al. Citation2019; Patel Citation2019; Gómez-Velásquez et al. Citation2021). Another disadvantage is the equipment and commercial database cost, which could limit access to this technique in clinical laboratories, particularly in low- and middle-income countries (Gómez-Velásquez et al. Citation2021).
The relevance of MALDI-TOF MS for identifying fungi has recently increased in clinical laboratories worldwide. In a multicenter study in the United States, the precision of Vitek 3.0 systems for the identification of filamentous fungi was between 85 and 100%, with an overall accuracy of 91%, and the precision of the identification of Fusarium and Neocosmospora was 96.8 and 84.6%, respectively (Rychert et al. Citation2018). A mass spectrometry library comparison of three laboratories in Canada showed an accuracy of 13.6–72% for filamentous fungi species-level identification, depending on the library chosen. Fusarium and Neocosmospora isolate identification accuracies vary between 0–80 and 0–100%, respectively (Stein et al. Citation2018). Using the MicroIDSys Elite database, a multilaboratory study in South Korea determined a species-level accuracy between 48.8 and 98.2%, an overall accuracy of 86.5%, and an accuracy of Fusarium and Neocosmospora isolate identification of 85.7 and 100%, respectively (Lee et al. Citation2021). In Colombia, a comparison study between a commercial and a validated in-house database shows an identification accuracy of 58 and 88.7%, respectively (Gómez-Velásquez et al. Citation2020). This study only includes one reference strain of F. oxysporum and does not include any Neocosmospora species (Gómez-Velásquez et al. Citation2020). It is noteworthy that some of these studies only have a few species of Fusarium and Neocosmospora, with fewer than ten isolates per species (Stein et al. Citation2018; Lee et al. Citation2021). These studies show the relevance of database selection in identifying fungi with this technique.
Few previous studies have analyzed this technique’s potential in identifying clinical isolates of Fusarium and Neocosmospora at the species level. In 2015, Al-Hatmi and colleagues validated an in-house database to identify clinical and environmental F. fujikuroi species complex isolates. The precision of this database for this complex was 93.3%, despite being unable to recognize clinical isolates of Fusarium ananatum and F. verticillioides (Al-Hatmi et al. Citation2015). In China, a study defined the accuracy of the Bruker Filamentous Fungi Library 1.0 in the identification of Fusarium and Neocosmospora clinical isolates. The identification accuracy for Fusarium was 86.25%, and that for Neocosmospora was 98.61% (Song et al. Citation2021). In general, these studies demonstrate the potential of MALDI-TOF MS in species-level identification of Fusarium and Neocosmospora. The primary limitations for this technique to be used as a routine clinical diagnosis are the cost of the equipment (Gómez-Velásquez et al. Citation2021), the lack of some species spectra in libraries/databases, and the need for a purified culture. The second limitation could be the easiest to overcome by creating an open and curated multicenter database of MALDI-TOF MS spectra.
Diagnosis difficulties in clinical practice
In the clinical setting, the diagnosis of fusariosis is based on clinical manifestations and laboratory tests. However, the diagnosis of these pathologies presents some challenges, such as (1) high mortality in a disseminated clinical presentation associated with immunocompromised patients, especially HSCT (hematopoietic stem cell transplantation) recipients and patients with severe and prolonged neutropenia (Muhammed et al. Citation2011); (2) most clinical laboratories are not trained in medical mycology or do not have the molecular techniques to determine the species; and (3) susceptibility tests are not routinely performed, and there are still no defined breakpoints. Therefore, the diagnosis is based on a fungal culture (when it is possible to isolate the fungus), histopathological tests, or clinical suspicion (Guarner and Brandt Citation2011).
To improve the laboratory diagnosis and epidemiological characterization of these fungi, we advocate for collaboration between routine diagnostic laboratories and mycological research laboratories.
Based on the available tools and the difficulties encountered in the laboratory diagnosis of these fungi, we propose the following workflow when the physician suspects an infection by Fusarium or Neocosmospora:
Clinical specimen collection should preferably be done before starting antifungal treatment based on the infectious site and laboratory conditions.
When available in the laboratory, a real-time/probe-based PCR should be performed to provide a rapid initial response to the physician.
Direct examination of the clinical specimen using the available laboratory stains.
Perform fungal culture on agar at 25 °C. Preferably, use PDA agar and Sabouraud-Dextrose agar for sterile samples, and Rose Bengal Chloramphenicol Agar for non-sterile samples.
After observing colony growth, examine the microscopic characteristics of the fungus 5–7 days post-detection. Early examination may not reveal the characteristic conidia of Fusarium and Neocosmospora, potentially leading to misdiagnosis.
Purify the fungus, preferably on PDA or Oatmeal agar to induce conidia production. Perform two independent isolations: one for microscopic validation and the other for DNA extraction.
Microscopically verify conidia and chlamydospore production at days 7, 15, and 21.
Perform DNA extraction on day 5.
If available, reserve one isolation for MADI-TOF analysis.
Conduct antifungal susceptibility test based on CLSI or EUCAST recommendations.
Perform PCR of selected gene marker. Preferably, use TEF1-α over ITS, LSU, or SSU if available in the laboratory.
Sequence PCR product, process sequence and compare against curated databases. We recommend using FUSARIOID-ID (www.fusarium.org/) and Mycobank (www.mycobank.org/), cautioning against relying solely on NCBI database for gene marker identification in clinical diagnosis.
The following steps are suggested when it’s possible:
Send isolates to specialized mycology laboratories with comprehensive information.
Consider genome sequencing of isolates.
Note: results of steps 1a, 2, 4, 5a, 5c, 6, and 8 should be promptly communicated to the physician to facilitate rapid treatment selection and change.
Conclusions
Recognition of Fusarium and Neocosmospora as priority fungi requires new surveillance data, which will only be possible with increased awareness of the effects of these fungi on human, animal, and environmental health. This is greatly facilitated by the training of health personnel in the diagnosis of fungal infections and by the development of new rapid diagnostic tests, such as LFA (lateral flow assays), currently available for other filamentous fungi, such as Aspergillus (Jenks et al. Citation2020). The recent outbreak (May 2023) of fungal meningitis associated with procedures performed under epidural anesthesia in Mexico, where five cerebrospinal fluid (CSF) samples tested positive for N. solani using real-time polymerase chain reaction (RT–PCR) (WHO Citation2023a), reveals the necessity of a rapid diagnostic test. This need coincides with the 2030 global research agenda for antimicrobial resistance in human health, where fungal diagnosis based on point-of-care diagnostic tests (including antigen and multiplex panel-based tests) is a priority for WHO fungal priority pathogens (WHO Citation2023b).
Additional information
Funding
References
- Al-Hatmi AM, Hagen F, Menken SB, Meis JF, Hoog GSD. 2016. Global molecular epidemiology and genetic diversity of Fusarium, a significant emerging group of human opportunists from 1958 to 2015. Emerg Microbes Infect. 5(12):e124. doi: 10.1038/emi.2016.126.
- Al-Hatmi AM, Normand AC, Diepeningen AD, Van Hendrickx M, Hoog GS, De, Piarroux R. 2015. Rapid identification of clinical members of Fusarium fujikuroi complex using MALDI-TOF MS. Future Microbiol. 10(12):1939–1952. doi: 10.2217/fmb.15.108.
- Al-Hatmi AMS, Meis JF, Hoog GS 2016. Fusarium: molecular diversity and intrinsic drug resistance. PLOS Pathog. 12(4):e1005464. doi: 10.1371/journal.ppat.1005464.
- Backx M, White PL, Barnes RA. 2014. New fungal diagnostics. Br J Hosp Med. 75(5):271–276. doi: 10.12968/hmed.2014.75.5.271.
- Bacorn C, Luu KY, Li JY. 2022. Fungal keratitis: diagnostic characteristics of the potassium hydroxide preparation with calcofluor white in northern California. Cornea. 41(3):347–352. doi: 10.1097/ICO.0000000000002907.
- Bharathi MJ, Ramakrishnan R, Meenakshi R, Mittal S, Shivakumar C, Srinivasan M. 2006. Microbiological diagnosis of infective keratitis: comparative evaluation of direct microscopy and culture results. Br J Ophthalmol. 90(10):1271–1276. doi: 10.1136/bjo.2006.096230.
- Broutin A, Bigot J, Senghor Y, Moreno-Sabater A, Guitard J, Hennequin C. 2020. In vitro susceptibility of Fusarium to isavuconazole. Antimicrob Agents Chemother. 64(2):1–5. doi: 10.1128/AAC.01621-19.
- Bukkems LMP, Dommelen L, van Regis M, Heuvel E, van den, Nieuwenhuizen L. 2023. The use of galactomannan antigen assays for the diagnosis of invasive pulmonary aspergillosis in the hematological patient: a systematic review and meta-analysis. J Fungi. 9(6):674. doi: 10.3390/jof9060674.
- Challa S, Sistla R. 2022. Histopathology diagnosis of filamentous fungi. Curr Fungal Infect Rep. 16(1):17–32. doi: 10.1007/s12281-021-00428-4.
- Chatterjee S. 2007. Evaluation of impression smears in the diagnosis of fungal keratitis. Indian J Ophthalmol. 55(5):403–404. doi: 10.4103/0301-4738.33843.
- Chen H, Yin Y, Gao H, Guo Y, Dong Z, Wang X, Zhang Y, Yang S, Peng Q, Liu Y, et al. 2020. Clinical utility of in-house metagenomic next-generation sequencing for the diagnosis of lower respiratory tract infections and analysis of the host immune response. Clin Infect Dis. 71(Suppl 4):S416–S426. doi: 10.1093/cid/ciaa1516.
- Chethana KWT, Manawasinghe IS, Hurdeal VG, Bhunjun CS, Appadoo MA, Gentekaki E, Raspé O, Promputtha I, Hyde KD. 2021. What are fungal species and how to delineate them? Fungal Divers. 109(1):1–25. doi: 10.1007/s13225-021-00483-9.
- Crous PW, Lombard L, Sandoval-Denis M, Seifert KA, Schroers H-J, Chaverri P, Gené J, Guarro J, Hirooka Y, Bensch K, et al. 2021. Fusarium: more than a node or a foot-shaped basal cell. Stud Mycol. 98:100116. doi: 10.1016/j.simyco.2021.100116.
- Dasgupta T, Sahu J. 2012. Origins of the KOH technique. Clin Dermatol. 30(2):238–242. doi: 10.1016/j.clindermatol.2011.11.020.
- Dellière S, Guitard J, Sabou M, Angebault C, Moniot M, Cornu M, Hamane S, Bougnoux M-E, Imbert S, Pasquier G, et al. 2022. Detection of circulating DNA for the diagnosis of invasive fusariosis: retrospective analysis of 15 proven cases. Med Mycol. 60(9):myac049. doi: 10.1093/mmy/myac049.
- Gómez-Velásquez JC, Loaiza-Díaz N, Hernández GN, Lima N, Mesa-Arango AC. 2020. Development and validation of an in-house library for filamentous fungi identification by MALDI-TOF MS in a clinical laboratory in Medellin (Colombia). Microorganisms. 8(9):1362. doi: 10.3390/microorganisms8091362.
- Gómez-Velásquez JC, Mojica-Figueroa IL, Santos C, Lima N, Mesa-Arango AC. 2021. MALDI-TOF MS: foundations and a practical approach to the clinically relevant filamentous fungi identification. Curr Fungal Infect Rep. 15(4):162–170. doi: 10.1007/s12281-021-00423-9.
- Goshia T, Aralar A, Wiederhold N, Jenks JD, Mehta SR, Karmakar A, E S M, Sharma A, Sun H, Kebadireng R, et al. 2024. Universal digital high resolution melt for the detection of pulmonary mold infections. J Clin Microbiol. 62(6):e0147623. doi: 10.1128/jcm.01476-23.
- Guarner J, Brandt ME. 2011. Histopathologic diagnosis of fungal infections in the 21st century. Clin Microbiol Rev. 24(2):247–280. doi: 10.1128/CMR.00053-10.
- Guevara-Suarez M, Cano-Lira JF, Cepero de García MC, Sopo L, De Bedout C, Cano LE, García AM, Motta A, Amézquita A, Cárdenas M, et al. 2016. Genotyping of Fusarium isolates from onychomycoses in Colombia: detection of two new species within the Fusarium solani species complex and in vitro antifungal susceptibility testing. Mycopathologia. 181:165–174.
- Herkert PF, Al-Hatmi AMS, Oliveira Salvador GLD, Muro MD, Pinheiro RL, Nucci M, Queiroz-Telles F, Hoog GSD, Meis JF. 2019. Molecular characterization and antifungal susceptibility of clinical Fusarium species from Brazil. Front Microbiol. 10:737. doi: 10.3389/fmicb.2019.00737.
- Hoenigl M, Salmanton-García J, Walsh TJ, Nucci M, Neoh CF, Jenks JD, Lackner M, Sprute R, Al-Hatmi AMS, Bassetti M, et al. 2021. Global guideline for the diagnosis and management of rare mould infections: an initiative of the European Confederation of Medical Mycology in Cooperation with the International Society for Human and Animal Mycology and the American Society for Microbiology. Lancet Infect Dis. 21:e246–e257.
- Hoffman JJ, Yadav R, Sanyam S, Das Chaudhary P, Roshan A, Singh SK, Arunga S, Hu VH, Macleod D, Leck A, et al. 2022. Diagnosis of fungal keratitis in low-income countries: evaluation of smear microscopy, culture, and in vivo confocal microscopy in Nepal. J Fungi. 8(9):955. doi: 10.3390/jof8090955.
- Hou TY, Chiang-Ni C, Teng SH. 2019. Current status of MALDI-TOF mass spectrometry in clinical microbiology. J Food Drug Anal. 27(2):404–414. doi: 10.1016/j.jfda.2019.01.001.
- Huang TE, Ou JH, Hung N, Yeh LK, Ma DHK, Tan HY, Chen HC, Hung KH, Fan YC, Sun PL, et al. 2022. Fusarium keratitis in Taiwan: molecular identification, antifungal susceptibilities, and clinical features. J Fungi. 8(5):476. doi: 10.3390/jof8050476.
- Infantino A, Grottoli A, Bergamaschi V, Oufensou S, Burgess LW, Balmas V. 2023. FusaHelp: a web site program for the morphological identification of Fusarium species. J Plant Pathol. 105(2):429–436. doi: 10.1007/s42161-023-01349-6.
- Jenks JD, Miceli MH, Prattes J, Mercier T, Hoenigl M. 2020. The Aspergillus lateral flow assay for the diagnosis of invasive aspergillosis: an update. Curr Fungal Infect Rep. 14(4):378–383. doi: 10.1007/s12281-020-00409-z.
- Kaufman L, Standard PG, Jalbert M, Kraft DE. 1997. Immunohistologic identification of Aspergillus spp. and other hyaline fungi by using polyclonal fluorescent antibodies. J Clin Microbiol. 35(9):2206–2209. doi: 10.1128/jcm.35.9.2206-2209.1997.
- Khot PD, Fredrick DN. 2009. PCR-based diagnosis of human fungal infections. Expert Rev Anti Infect Ther. 7(10):1201–1221. doi: 10.1586/eri.09.104.
- Kidd SE, Abdolrasouli A, Hagen F. 2023. Fungal nomenclature: managing change is the name of the game. Open Forum Infect Dis. 10(1):ofac559. doi: 10.1093/ofid/ofac559.
- Kidd SE, Chen SCA, Meyer W, Halliday CL. 2019. A new age in molecular diagnostics for invasive fungal disease: are we ready? Front Microbiol. 10:2903. doi: 10.3389/fmicb.2019.02903.
- Larkin PMK, Lawson KL, Contreras DA, Le CQ, Trejo M, Realegeno S, Hilt EE, Chandrasekaran S, Garner OB, Fishbein GA, et al. 2020. Amplicon-based next-generation sequencing for detection of fungi in formalin-fixed, paraffin-embedded tissues: correlation with histopathology and clinical applications. J Mol Diagn. 22(10):1287–1293. doi: 10.1016/j.jmoldx.2020.06.017.
- Lass-Flörl C, Mutschlechner W, Aigner M, Grif K, Marth C, Girschikofsky M, Grander W, Greil R, Russ G, Cerkl P, et al. 2013. Utility of PCR in diagnosis of invasive fungal infections: real-life data from a multicenter study. J Clin Microbiol. 51(3):863–868. doi: 10.1128/JCM.02965-12.
- Leal SM, Rodino KG, Fowler WC, Gilligan PH. 2021. Practical guidance for clinical microbiology laboratories: diagnosis of ocular infections. Clin Microbiol Rev. 34(3):e0007019. doi: 10.1128/CMR.00070-19.
- Lee H, Oh J, Sung GH, Koo J, Lee MH, Lee HJ, Cho S, Il Choi JS, Park YJ, Shin JH, et al. 2021. Multilaboratory evaluation of the MALDI-TOF mass spectrometry system, MicroIDSys Elite, for the identification of medically important filamentous fungi. Mycopathologia. 186(1):15–26. doi: 10.1007/s11046-020-00507-z.
- Lee J, Jeong H, Kang HG, Park J, Choi EY, Lee CS, Byeon SH, Kim M. 2023. Rapid pathogen detection in infectious uveitis using nanopore metagenomic next-generation sequencing: a preliminary study. Ocul Immunol Inflamm. 32(4):463–469. doi: 10.1080/09273948.2023.2173243.
- Leslie JF, Summerell BA. 2006. The Fusarium laboratory manual. Iowa, USA: Blackwell Publishing.
- Liu HY, Hopping GC, Vaidyanathan U, Ronquillo YC, Hoopes PC, Moshirfar M. 2019. Polymerase chain reaction and its application in the diagnosis of infectious keratitis. Medical hypothesis. Med Hypothesis Discov Innov Ophthalmol. 8(3):152–155.
- Liu K, Howell DN, Perfect JR, Schell WA. 1998. Morphologic criteria for the preliminary identification of Fusarium, Paecilomyces, and Acremonium species by histopathology. Am J Clin Pathol. 109(1):45–54. doi: 10.1093/ajcp/109.1.45.
- Mendonça A, Santos H, Franco-Duarte R, Sampaio P. 2022. Fungal infections diagnosis – past, present and future. Res Microbiol. 173(3):103915. doi: 10.1016/j.resmic.2021.103915.
- Mitchell SL, Simner PJ. 2019. Next-generation sequencing in clinical microbiology: are we there yet? Clin Lab Med. 39(3):405–418. doi: 10.1016/j.cll.2019.05.003.
- Muhammed M, Coleman JJ, Carneiro HA, Mylonakis E. 2011. The challenge of managing fusariosis. Virulence. 2(2):91–96. doi: 10.4161/viru.2.2.15015.
- Muraosa Y, Oguchi M, Yahiro M, Watanabe A, Yaguchi T, Kamei K. 2017. Epidemiological study of Fusarium species causing invasive and superficial fusariosis in Japan. Med Mycol J. 58(1):E5–E13. doi: 10.3314/mmj.16-00024.
- Najafzadeh MJ, Dolatabadi S, de Hoog S, Esfahani MK, Haghani I, Aghili SR, Ghazvini RD, Rezaei-Matehkolaei A, Abastabar M, Al-Hatmi AMS. 2020. Phylogenetic analysis of clinically relevant Fusarium species in Iran. Mycopathologia. 185(3):515–525. doi: 10.1007/s11046-020-00460-x.
- Nalim FA, Samuels GJ, Wijesundera RL, Geiser DM. 2011. New species from the Fusarium solani species complex derived from perithecia and soil in the Old World tropics. Mycologia. 103(6):1302–1330. doi: 10.3852/10-307.
- Nelson PE, Dignani MC, Anaissie EJ. 1994. Taxonomy, biology, and clinical aspects of Fusarium species. Clin Microbiol Rev. 7(4):479–504. doi: 10.1128/CMR.7.4.479-504.1994.
- Nucci M, Anaissie E. 2023. Invasive fusariosis. Clin Microbiol Rev. 36(4):e0015922. doi: 10.1128/cmr.00159-22.
- Nucci M, Barreiros G, Reis H, Paixão M, Akiti T, Nouér SA. 2019. Performance of 1,3-beta-D-glucan in the diagnosis and monitoring of invasive fusariosis. Mycoses. 62(7):570–575. doi: 10.1111/myc.12918.
- Nucci M, Jenks J, Thompson GR, Hoenigl M, Dos Santos MC, Forghieri F, Rico JC, Bonuomo V, López-Soria L, Lass-Flörl C, et al. 2021. Do high MICs predict the outcome in invasive fusariosis? J Antimicrob Chemother. 76(4):1063–1069. doi: 10.1093/jac/dkaa516.
- O’Donnell K, Al-Hatmi AMS, Aoki T, Brankovics B, Cano-Lira JF, Coleman JJ, de Hoog GS, Di Pietro A, Frandsen RJN, Geiser DM, et al. 2020. No to Neocosmospora: phylogenomic and practical reasons for continued inclusion of the Fusarium solani species complex in the genus Fusarium. mSphere. 5(5):1–7. doi: 10.1128/mSphere.00810-20.
- Odabasi Z, Mattiuzzi G, Estey E, Kantarjian H, Saeki F, Ridge RJ, Ketchum PA, Finkelman MA, Rex JH, Ostrosky-Zeichner L. 2004. β-D-glucan as a diagnostic adjunct for invasive fungal infections: validation, cutoff development, and performance in patients with acute myelogenous leukemia and myelodysplastic syndrome. Clin Infect Dis. 39(2):199–205. doi: 10.1086/421944.
- Oliveira dos Santos C, Kolwijck E, Rooij J, van Stoutenbeek R, Visser N, Cheng YY, Santana NTY, Verweij PE, Eggink CA. 2020. Epidemiology and clinical management of Fusarium keratitis in the Netherlands, 2005–2016. Front Cell Infect Microbiol. 10:133. doi: 10.3389/fcimb.2020.00133.
- Patel R. 2019. A moldy application of MALDI: MALDI-ToF mass spectrometry for fungal identification. J Fungi. 5(1):4. doi: 10.3390/jof5010004.
- Perfect JR. 2013. Fungal diagnosis: how do we do it and can we do better? Curr Med Res Opin. 29 Suppl 4(sup4):3–11. doi: 10.1185/03007995.2012.761134.
- Rahn S, Schuck A, Kondakci M, Haas R, Neuhausen N, Pfeffer K, Henrich B. 2016. A novel comprehensive set of fungal Real time PCR assays (fuPCR) for the detection of fungi in immunocompromised haematological patients—a pilot study. Int J Med Microbiol. 306(8):611–623. doi: 10.1016/j.ijmm.2016.10.003.
- Rathi VM, Thakur M, Sharma S, Khanna R, Garg P. 2017. KOH mount as an aid in the management of infectious keratitis at secondary eye care centre. Br J Ophthalmol. 101(11):1447–1450. doi: 10.1136/bjophthalmol-2017-310241.
- Rosa PD, Ramirez-Castrillon M, Borges R, Aquino V, Meneghello Fuentefria A, Zubaran Goldani L. 2019. Epidemiological aspects and characterization of the resistance profile of Fusarium spp. in patients with invasive fusariosis. J Med Microbiol. 68(10):1489–1496. doi: 10.1099/jmm.0.001059.
- Rychert J, Slechta ES, Barker AP, Miranda E, Babady NE, Tang YW, Gibas C, Wiederhold N, Sutton DA, Hanson KE. 2018. Multicenter evaluation of the Vitek MS v3.0 system for the identification of filamentous fungi. J Clin Microbiol. 56(2):1–11. doi: 10.1128/JCM.01353-17.
- Sandoval-Denis M, Crous PW. 2018. Removing chaos from confusion: assigning names to common human and animal pathogens in Neocosmospora. Persoonia. 41(1):109–129. doi: 10.3767/persoonia.2018.41.06.
- Scharf S, Bartels A, Kondakci M, Haas R, Pfeffer K, Henrich B. 2021. FuPCR as diagnostic method for the detection of rare fungal pathogens, such as Trichosporon, Cryptococcus and Fusarium. Med Mycol. 59(11):1101–1113. doi: 10.1093/mmy/myab045.
- Schroers H, Samuels GJ, Zhang N, Short DPG, Juba J, Geiser DM, Samuels GJ, Zhang N, Short DPG. 2016. Epitypification of Fusisporium (Fusarium) solani and its assignment to a common phylogenetic species in the Fusarium solani species complex. Mycologia. 108(4):806–819. doi: 10.3852/15-255.
- Sharma S, Kunimoto DY, Gopinathan U, Athmanathan S, Garg P, Rao GN. 2002. Evaluation of corneal scraping smear examination methods in the diagnosis of bacterial and fungal keratitis: a survey of eight years of laboratory experience. Cornea. 21(7):643–647. doi: 10.1097/00003226-200210000-00002.
- Sharma S. 2012. Diagnosis of infectious diseases of the eye. Eye. 26(2):177–184. doi: 10.1038/eye.2011.275.
- Shigeyasu C, Yamada M, Aoki K, Ishii Y, Tateda K, Yaguchi T, Okajima Y, Hori Y. 2018. Metagenomic analysis for detecting Fusarium solani in a case of fungal keratitis. J Infect Chemother. 24(8):664–668. doi: 10.1016/j.jiac.2017.12.019.
- Song Y, Liu X, Yang Z, Meng X, Xue R, Yu J, Al-Hatmi AMS, Hoog GS, de, Li R. 2021. Molecular and MALDI-ToF MS differentiation and antifungal susceptibility of prevalent clinical Fusarium species in China. Mycoses. 64(10):1261–1271. doi: 10.1111/myc.13345.
- Springer J, Walther G, Rickerts V, Hamprecht A, Willinger B, Teschner D, Einsele H, Kurzai O, Loeffler J. 2019. Detection of Fusarium species in clinical specimens by probe-based real-time PCR. J Fungi. 5(4):105. doi: 10.3390/jof5040105.
- Steenwyk JL, Rokas A, Goldman GH. 2023. Know the enemy and know yourself: addressing cryptic fungal pathogens of humans and beyond. PLOS Pathog. 19(10):e1011704. doi: 10.1371/journal.ppat.1011704.
- Stein M, Tran V, Nichol KA, Lagacé-Wiens P, Pieroni P, Adam HJ, Turenne C, Walkty AJ, Normand AC, Hendrickx M, et al. 2018. Evaluation of three MALDI-TOF mass spectrometry libraries for the identification of filamentous fungi in three clinical microbiology laboratories in Manitoba, Canada. Mycoses. 61(10):743–753. doi: 10.1111/myc.12800.
- Tabatabaei SA, Soleimani M, Tabatabaei SM, Beheshtnejad AH, Valipour N, Mahmoudi S. 2020. The use of in vivo confocal microscopy to track treatment success in fungal keratitis and to differentiate between Fusarium and Aspergillus keratitis. Int Ophthalmol. 40(2):483–491. doi: 10.1007/s10792-019-01209-2.
- Tabatabaei SA, Tabatabaei M, Soleimani M, Tafti ZF. 2018. Fungal keratitis caused by rare organisms. J Curr Ophthalmol. 30(1):91–96. doi: 10.1016/j.joco.2017.08.004.
- Tekpinar AD, Kalmer A. 2019. Utility of various molecular markers in fungal identification and phylogeny. Nova_Hedwigia. 109(1–2):187–224. doi: 10.1127/nova_hedwigia/2019/0528.
- Theel ES, Doern CD. 2013. β-D-Glucan testing is important for diagnosis of invasive fungal infections. J Clin Microbiol. 51(11):3478–3483. doi: 10.1128/JCM.01737-13.
- Tortorano AM, Esposto MC, Prigitano A, Grancini A, Ossi C, Cavanna C, Cascio GL. 2012. Cross-reactivity of Fusarium spp. in the Aspergillus galactomannan enzyme-linked immunosorbent assay. J Clin Microbiol. 50(3):1051–1053. doi: 10.1128/JCM.05946-11.
- van Diepeningen AD, Brankovics B, Iltes J, van der Lee TAJ, Waalwijk C. 2015. Diagnosis of Fusarium infections: approaches to Identification by the Clinical Mycology Laboratory. Curr Fungal Infect Rep. 9(3):135–143. doi: 10.1007/s12281-015-0225-2.
- Velasquez-Agudelo V, Cardona-Arias JA. 2017. Meta-analysis of the utility of culture, biopsy, and direct KOH examination for the diagnosis of onychomycosis. BMC Infect Dis. 17(1):166. doi: 10.1186/s12879-017-2258-3.
- Walther G, Stasch S, Kaerger K, Hamprecht A, Roth M, Cornely OA, Geerling G, Mackenzie CR, Kurzai O, Lilienfeld-Toal M, et al. 2017. Fusarium keratitis in Germany. J Clin Microbiol. 55(10):2983–2995. doi: 10.1128/JCM.00649-17.
- Weinberg JM, Koestenblatt EK, Tutrone WD, Tishler HR, Najarian L. 2003. Comparison of diagnostic methods in the evaluation of onychomycosis. J Am Acad Dermatol. 49(2):193–197. doi: 10.1067/s0190-9622(03)01480-4.
- Winn RM, Gil-Lamaignere C, Maloukou A, Roilides E, Maglaveras N, Gaustad P, Abrahamsen TG, Denning DW, Meis JFGM, Verweij PE, et al. 2003. Interactions of human phagocytes with moulds Fusarium spp. and Verticillium nigrescens possessing different pathogenicity. Med Mycol. 41(6):503–509. doi: 10.1080/1369378030001615394.
- World Health Organization. 2022. WHO fungal priority pathogens list to guide research, development and public health action.
- World Health Organization. 2023a. Disease outbreak news; outbreak of suspected fungal meningitis associated with surgical procedures performed under spinal anaesthesia – the United States of America and Mexico.
- World Health Organization. 2023b. Global research agenda for antimicrobial resistance in human health: policy brief: background: the global research agenda for antimicrobial resistance in human health prioritizes forty research topics for evidence generation to inform policy by 2030.
- Yilmaz N, Sandoval-Denis M, Lombard L, Visagie CM, Wingfield BD, Crous PW. 2021. Redefining species limits in the Fusarium fujikuroi species complex. Persoonia. 46:129–162. doi: 10.3767/persoonia.2021.46.05.
- Zhang W, Yang H, Jiang L, Han L, Wang L. 2010. Use of potassium hydroxide, giemsa and calcofluor white staining techniques in the microscopic evaluation of corneal scrapings for diagnosis of fungal keratitis. J Int Med Res. 38(6):1961–1967. doi: 10.1177/147323001003800609.