Abstract
The US Environmental Protection Agency Endocrine Disruptor Screening Program (EDSP) is a tiered screening approach to determine the potential for a chemical to interact with estrogen, androgen, or thyroid hormone systems and/or perturb steroidogenesis. Use of high-throughput screening (HTS) to predict hazard and exposure is shifting the EDSP approach to (1) prioritization of chemicals for further screening; and (2) targeted use of EDSP Tier 1 assays to inform specific data needs. In this work, toxicology data for three triazole fungicides (triadimefon, propiconazole, and myclobutanil) were evaluated, including HTS results, EDSP Tier 1 screening (and other scientifically relevant information), and EPA guideline mammalian toxicology study data. The endocrine-related bioactivity predictions from HTS and information that satisfied the EDSP Tier 1 requirements were qualitatively concordant. Current limitations in the available HTS battery for thyroid and steroidogenesis pathways were mitigated by inclusion of guideline toxicology studies in this analysis. Similar margins (3–5 orders of magnitude) were observed between HTS-predicted human bioactivity and exposure values and between in vivo mammalian bioactivity and EPA chronic human exposure estimates for these products’ registered uses. Combined HTS hazard and human exposure predictions suggest low priority for higher-tiered endocrine testing of these triazoles. Comparison with the mammalian toxicology database indicated that this HTS-based prioritization would have been protective for any potential in vivo effects that form the basis of current risk assessment for these chemicals. This example demonstrates an effective, human health protective roadmap for EDSP evaluation of pesticide active ingredients via prioritization using HTS and guideline toxicology information.
Introduction
The US Environmental Protection Agency (EPA) Endocrine Disruptor Screening Program (EDSP) addresses the statutory requirements of the 1996 amendments to the Safe Drinking Water Act and Food Quality Protection Act. In 2009, EDSP Tier 1 battery test orders were issued, and 50 pesticide active ingredients (PAIs) and two inert ingredients were then evaluated with the EDSP Tier 1 screening assay battery. The EDSP Tier 1 battery includes five in vitro assays to assess estrogen, androgen and steroidogenic function and six in vivo assays to assess estrogen, androgen, steroidogenic and thyroid function (USEPA Citation2014a). Conducting all 11 assays requires a minimum of 520 animals and $800,000 USD per chemical (Willett et al. Citation2011; USEPA Citation2013). Approximately 10,000 chemicals may be subject to EDSP evaluation (USEPA Citation2014c), indicating the necessity to develop practical methods to prioritize both PAIs that undergo extensive pre-registration testing and other chemicals (not chemicals with food uses) that constitute most of this list and often lack extensive pre-registration testing. Combined utilization of high-throughput screening (HTS) for bioactivity and exposure may provide the needed efficiency for prioritization and screening.
In the case of endocrine-related adverse outcome pathways (AOPs), HTS bioactivity data are available from EPA’s Toxicity ForeCaster (ToxCast™) and Toxicology in the twenty-first century (Tox21) to inform the likelihood that a chemical activates molecular initiating events, e.g., receptor-based interactions, or early key events including upregulation of markers of Phase II metabolism that occur upstream of potential adverse outcomes related to endocrine function. Available (November 2014 release) (USEPA Citation2014e) HTS assays in Tox21 and ToxCast Phase II specifically related to endocrine outcomes include estrogen receptor (ER), androgen receptor (AR) and thyroid hormone receptor (TR) transcriptional activation assays; estrogen and androgen cofactor recruitment and dimerization assays; ER and AR binding assays; aromatase inhibition assays; and an estrogen-dependent cell proliferation assay. HTS assays indicative of nuclear receptor-mediated hepatic catabolism of thyroid hormones, i.e., nuclear receptor activation assays (ToxCast Phases I and II) and the CellzDirect mRNA expression assays in hepatocytes (ToxCast Phase I only) (Rotroff et al. Citation2010), may provide some mechanistic information to explain in vivo thyroid effects in rodents (Murk et al. Citation2013; Paul et al. Citation2014; Sueyoshi et al. Citation2014; Schraplau et al. Citation2015). EPA’s ToxCast and Exposure Forecasting (ExpoCast) programs (Wambaugh et al. Citation2014), along with the interagency Tox21 agreement, have yielded a large set of data that may be useful as screening-level information for prioritization tasks. Data from in vitro HTS assays may demonstrate a lack of effect on an endpoint or an effect on an endpoint that should be studied in a model of greater biological complexity. Two objectives of the current work are to help define the use of these HTS assay data as first-tier screening information and to highlight the guideline toxicology testing – considered higher-tier information – that might support or refute HTS assay results during EDSP evaluation.
The combined use of physicochemical properties, HTS assay data, exposure predictions and computational tools to characterize the potential for chemicals to trigger molecular-initiating events of AOPs related to endocrine function represents a significant advance in toxicology and risk assessment practice (USEPA Citation2011, Citation2013; Browne et al. Citation2015). In an initial effort to prioritize chemicals for further endocrine activity screening under the EDSP, EPA suggested a tiered consideration of chemical use type, physicochemical properties (acid-dissociation constant, corrosivity and hydrolysis half-life), available quantitative structure relationships, and assay results for endocrine-related activity (USEPA Citation2012). A classification model developed for endocrine activity using ToxCast and Tox21 HTS assay data previously demonstrated that available HTS data correctly indicated estrogenic/anti-estrogenic and androgenic/anti-androgenic activity in the relevant corresponding Tier 1 assays (Rotroff et al. Citation2013). Further, results from a predictive ER activity model corresponded qualitatively to the results of estrogen-related Tier 1 assays, including the ER binding and transcriptional activity assays and the uterotrophic assay (Rotroff et al. Citation2014; USEPA Citation2014b; Browne et al. Citation2015). Thus, hazard screening information from the predictive ER activity model (Rotroff et al. Citation2014; USEPA Citation2014b; Judson et al. Citation2015; Browne et al. Citation2015) and the predictive AR model under development (USEPA Citation2014b) are useful in determining a lack of ER or AR pathway-based hazards, indicating no need for the corresponding Tier 1 assays. Conversely, these ER and AR activity models may demonstrate a potential in vitro estrogen or androgen activity for a chemical that could be further evaluated using estrogen- or androgen-sensitive in vitro and in vivo assays. The continuing development of models that integrate results from multiple assay technologies and data sources in a pathway-based approach will ultimately decrease the amount of animal-based EDSP Tier 1 screening required.
To assess whether the use of HTS-based prioritization would be human health protective when compared with current risk assessment practices, a case study of three data-rich triazole fungicides – myclobutanil, propiconazole and triadimefon – was developed. These triazole fungicides were selected because they have been studied in a variety of models, with data available not only from EDSP Tier 1 screening but also from other scientifically relevant information (OSRI), including guideline toxicology studies, published studies, ToxCast/Tox21 assays and ExpoCast. Further, although these triazoles share structural features and a common fungicidal mode-of-action (Trosken et al. Citation2006a), the apical toxicities, including those potentially related to the endocrine system, manifest differently in vivo by triazole. Previously, these three triazole fungicides had been selected by the EPA Office of Research and Development (Hester et al. Citation2006; Tully et al. Citation2006; Wolf et al. Citation2006) for extensive in vivo and in vitro testing to explore their varying effects related to hepatic-, thyroid-, reproductive- and endocrine-related endpoints. We explored whether HTS bioactivity and exposure predictions would have created an initial prioritization for further endocrine activity screening that would be as human health protective as the traditional testing and risk assessments performed as part of the registration of these triazoles. First, a comparison of the three “lines of evidence” – including HTS bioactivity results, the EDSP Tier 1 battery plus published OSRI, and guideline toxicology studies – was assessed. Next, the margin between HTS bioactivity and human exposure predictions was compared with the margin separating mammalian in vivo bioactivity and chronic human exposure estimates derived by EPA for the registered uses of these chemicals. A key goal was to illustrate whether utilization of HTS-based prioritization would have been as protective as the point-of-departure currently used in risk assessment. The resultant case study provides a methodology and support for the utilization of HTS information in prioritization applications, a course currently suggested by EPA (USEPA Citation2015b) and likely to be improved as more HTS methods are developed and refined for this purpose. Based on the evaluation of these three triazoles, four questions are considered:
Are HTS assay data currently sufficient for an endocrine prioritization task, and does the current HTS battery (ToxCast/Tox21) provide appropriate biological coverage for each of the sub-types of endocrine pathways (estrogen, androgen, thyroid and steroidogenesis)?
Are HTS information, guideline toxicology data and the EDSP Tier 1 assays sufficiently concordant to support that HTS prioritization would be possible? In other words, are there major biological gaps or false negatives in HTS that would have affected EDSP evaluation and risk assessment for these three PAIs? Although false positives in HTS may indicate a need for additional evaluation, higher-tier testing (other in vitro or in vitro assays) would eventually reveal the false positive, erring on the side of conservatism.
Is prioritization based on the high-throughput exposure and bioactivity predictions as protective of human health as traditional risk assessment approaches?
In the absence of the EDSP Tier 1 battery, could human health protective prioritization decisions be made regarding the need for any additional hazard and exposure information, using HTS prioritization alone or in combination with the available 40 Code of Federal Regulations (CFR) Part 158 guideline toxicology study information?
Methods
High-throughput screening hazard prediction
The in vitro HTS assay data used in this evaluation were generated by the ToxCast and Tox21 research programs and are publicly available (http://www.epa.gov/chemical-research/toxicity-forecaster-toxcasttm-data) (Kavlock et al. Citation2012; Tice et al. Citation2013). As of the November 2014 data release, more than 800 assay endpoints were available via ToxCast and Tox21, with more than 400 assay endpoints available for each of the three triazoles in the present evaluation. All three triazole fungicides were in the initial ToxCast Phase I chemical library, and results are available for ToxCast Phase II assays (USEPA Citation2014e) (invitrodb_v1, accessed November 2014, http://www.epa.gov/chemical-research/toxicity-forecaster-toxcasttm-data), as well as previously released ToxCast Phase I assay data (December 2009, http://www.epa.gov/chemical-research/toxicity-forecaster-toxcasttm-data). A subset of the ToxCast Phase I assay dataset from the CellzDirect assay technology was selected for the current exercise because of its potential utility in predicting chemical induction of hepatic catabolism and transport of thyroid hormones via nuclear receptor activation (Rotroff et al. Citation2010). This assay technology utilized primary human hepatocytes (N = 2 donors) exposed to test chemicals for 6, 24 or 48 h with an assay endpoint of increased mRNA expression of xenobiotic metabolizing and transport enzyme targets, and at the time of this analysis, was included in archived data with only AC50 values available. Dose–response information and any dose–response curve-fitting caution flags are now publicly available for all of the data used in this report.
Endocrine-related HTS assay endpoints were selected and assigned to endocrine target hypotheses: estrogenic (E), anti-estrogenic (anti-E), androgenic (A), anti-androgenic (anti-A), steroidogenesis (S), thyroid (T) and anti-thyroid (anti-T). Assignment of the E/anti-E or A/anti-A hypotheses to specific HTS assays was based on description of the computational models developed by EPA for predicting receptor-based endocrine activity (USEPA Citation2014b; Browne et al. Citation2015). For the present exercise, assay endpoints related to S/T/anti-T were also considered based on the expert judgment. The numbers of HTS assay endpoints from ToxCast and Tox21 considered as a line of evidence for potential endocrine bioactivity hypotheses are illustrated in and the full assay names and descriptions are listed in Supplemental Table 1.
Figure 1. Number of HTS assays available for the three triazoles within each endocrine activity hypothesis. The assay endpoints available by estrogen (E), anti-estrogen (AE), androgen (A), anti-androgen (AA), steroidogenesis (S), thyroid receptor (TR), anti-thyroid receptor (ATR) and hepatic catabolism related to thyroid hormone (Liver). Not all triazoles were tested in all assays, leading to minor discrepancies between triazoles for the number of assay endpoints available.
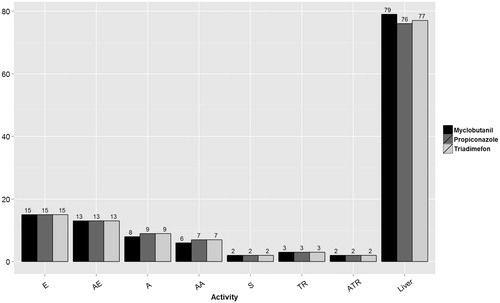
For additional comparison, scores from the ToxCast ER and AR agonist and antagonist area under the curve (AUC) models, developed by the EPA Office of Research and Development (USEPA Citation2014b; Browne et al. Citation2015), were compared with the HTS assay results. These ER and AR AUC models integrate multiple HTS assay results for ER and AR agonist and antagonist activity and use a systems biology approach to consider pathway activity along with potential sources of assay interference, in practice providing more context for interpreting HTS assay results, e.g., reducing the impact of false positives, for ER and AR activity. These model scores are also available in the EDSP21 Dashboard (actor.epa.gov/edsp21). AUC values ranged from 0.0 to 1.0 for the ER and AR pathways, with a greater value indicative of a greater response. The estrogen, anti-estrogen and androgen model scores must surpass a threshold of 0.1 to be considered positive, and the anti-androgen model score must surpass a threshold of 0.05 to be considered positive (USEPA Citation2014b).
In vitro to in vivo extrapolation of oral equivalent doses for HTS assay AC50 values
In vitro-to-in vivo extrapolation of oral equivalent doses for HTS assay concentrations that achieved 50% maximal activity (AC50) facilitates comparison of HTS assay data with oral doses administered in whole animal studies. A simplified derivation of oral equivalent doses was employed that applies only to first-order metabolism; it used the following assumption (Wetmore et al. Citation2012):
Steady-state concentrations (Css) in blood were predicted from measured intrinsic clearance and fraction unbound for each triazole and other standard physiological parameters (Wetmore et al. Citation2012). The upper 95th percentile of the potential distribution of Css values (Css95%) was estimated in SimCyp using a Monte Carlo approach to simulate population variability (Wetmore et al. Citation2012; Wetmore Citation2014). These high-throughput toxicokinetic predictions of the Css95% are publicly available (USEPA Citation2014b) (Appendix 3). The Css95% values used to calculate the oral equivalent doses for the AC50 values for myclobutanil, propiconazole and triadimefon are listed in . All the calculated oral equivalent doses for the endocrine-related HTS assay AC50 values in this case study, used in , are available in Supplemental File 2.
Figure 2. Triadimefon: Parallel comparison of predicted bioactivity and exposure and US EPA chronic exposure estimates and in vivo bioactivity. Annotated to show Bins 1–2. See text, , and Supplemental File 2 for additional details on AC50 by assay and bin. Dotted line = cytotoxicity caution flag (1.68 μM or 5.13 mkd). LPEAD, lowest potentially endocrine active dose (= 1800 ppm or 100 mkd for triadimefon).
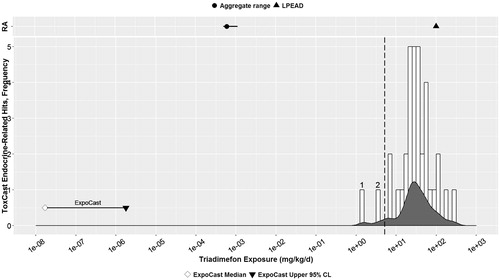
Figure 3. Propiconazole: Parallel comparison of predicted bioactivity and exposure and EPA chronic exposure estimates and in vivo bioactivity. Annotated to show Bins 1–8. See text, , and Supplemental File 2 for additional details on AC50 by assay and bin. Dotted line = cytotoxicity caution flag (4.6 μM or 4.15 mkd). LPEAD, lowest potentially endocrine active dose (= 2500 ppm or 200.4 mkd for propiconazole).
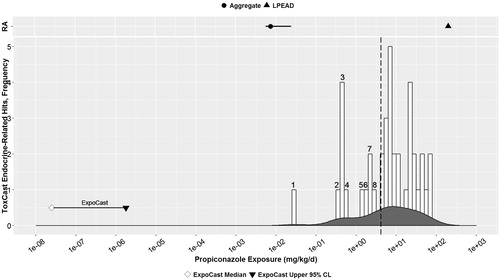
Figure 4. Myclobutanil: parallel comparison of predicted bioactivity and exposure and EPA chronic exposure estimates and in vivo bioactivity. Annotated to show Bins 1–4. See text, , and Supplemental File 2 for additional details on AC50 by assay and bin. Dotted line = cytotoxicity caution flag (4.69 μM or 5.13 mkd). LPEAD, lowest potentially endocrine active dose (= 200 ppm or 9.84–12.86 mkd for myclobutanil). For graphing purposes, the midpoint of the interval between 9.84 and 12.86, or 11.35 mkd, was used as the LPEAD.
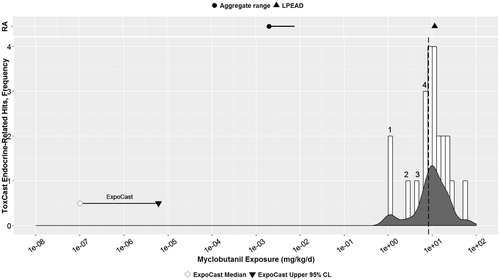
Table 1. Steady state systemic concentration values used to calculate oral equivalent doses.
High-throughput human exposure prediction (ExpoCast)
The ExpoCast project is a rapidly evolving program at EPA intended to predict human exposure to chemicals with limited or no exposure-related data (Wambaugh et al. Citation2013, Citation2014). The second-generation analysis employs Bayesian methods to infer exposure ranges that align with National Health and Nutrition Examination Survey (NHANES) data, using chemical descriptors (chemical use type and production volume) and demographics to calibrate the regression coefficients for the predictive model. Note that the purpose of the current ExpoCast model is to provide an approximate range of human exposures for use in prioritization efforts. The current ExpoCast model uses five indicators of exposure, including four use categories (industrial and consumer use, pesticide inert, pesticide active and industrial with no consumer use) and production volume (expressed in logarithmic scale), as inputs for a model to predict exposure. These five factors accounted for approximately 50% of the variability in exposures measured in NHANES across demographic groups. Demographic groups included in this analysis mirrored those in NHANES: children ages 6–11 and 12–19 years; adults ages 20–65 and greater than 65 years; body mass index greater than 30; females; males; and reproductive-age females (16–49 years). Demographic group parameters did not appear to be significant for predicting different levels of exposure in this version of the model (Wambaugh et al. Citation2014).
The predicted human oral exposure range for each of the three triazoles, from the median to the 95th percentile, for the total population was selected from the ExpoCast project second-generation analysis for the comparisons in this manuscript (Wambaugh et al. Citation2014) (Supplemental Table 1); the total population median and total population upper 95% predicted exposures for myclobutanil, propiconazole and triadimefon are listed in . The total population exposure range was selected for comparison because the ExpoCast predictions ranged two orders of magnitude, and perhaps because of the large uncertainty around the possible range of exposure, the total population-predicted exposure range was roughly equivalent to the ranges predicted for all the various subpopulations in the second-generation ExpoCast analysis.
Table 2. Predicted human oral exposure range for three triazoles.
The ExpoCast project has not yet extended to prediction of ecological exposures for pertinent species or concentrations in environmentally relevant matrices beyond a nascent research stage, though this is the subject of ongoing research (USEPA, Citation2014c). Further, prediction of relevant ecological exposures is beyond the scope of this manuscript, which is focused on human health applications. Thus, the analysis presented in this paper using HTS bioactivity and exposure predictions is not yet possible for environmental prioritization applications, and, therefore, cannot be evaluated for its utility for ecological risk assessment at this time.
Exposure estimates from EPA human risk assessment documents
To facilitate comparison, a range of the exposure estimates for each PAI is presented in , using the largely unrefined chronic aggregate exposure values from EPA risk assessment documents. The estimated chronic aggregate (dietary and drinking water) exposure range for all subpopulations and the total US general population, the model used and the reference for these values are provided in . It should be underscored that these values include both dietary and drinking water exposures and are unrefined estimates of exposure based on modeling data. The standard EPA approach is to conduct initial, conservative first-tier exposure assessments and subsequently refine the model inputs only if the level of concern is exceeded using conservative inputs. These exposure assessment estimates are considered conservative and protective of human health and are not necessarily accurate measurements of exposure. Possible refinements to the estimates derived from dietary exposure models include the percentage of crop treated, i.e., the proportion of the marketplace treated with a particular PAI; the use of US Department of Agriculture Pesticide Data Program residue monitoring data in place of tolerance values; and the use of specific (measured) food processing factors in place of default processing factors. Unrefined estimates of drinking water concentration are one to five orders of magnitude higher, with the majority between three and five orders of magnitude higher, than actual measured values, because a series of conservative assumptions are applied. These assumptions include the following: every acre of the watershed is treated at the maximum single application rate, with the maximum number of applications; all fields in the catchment are assumed to be treated at the same time; and runoff instantaneously moves to the drinking water reservoir (Jones Citation2005; Winchell & Snyder Citation2014).
Table 3. Chronic aggregate exposure estimates from EPA risk assessments.
The EDSP Tier 1 battery and published OSRI
The methods and availability of EDSP Tier 1 battery studies (Series 890) and key publications that, in addition to guideline studies, constituted the OSRI used to support EDSP Tier 1 test waivers for myclobutanil, propiconazole and triadimefon are described in . The EDSP Tier 1 battery consists of 11 different assays that assess the potential for a test compound to perturb the estrogen, androgen or thyroid systems, or interfere with steroidogenesis. Analysis of the Tier 1 screening battery results for each of these endocrine pathways considers the strength of responses, consistency across studies, plus all other supporting data (e.g., OSRI) that can be used to indicate whether or not a particular endocrine mechanism may be perturbed. The EDSP Tier 1 battery is considered to be screening and, therefore, may not demonstrate adversity or a complete dose-response. Studies in non-mammalian species, including a fish short-term reproduction study (FSTRA) and an amphibian metamorphosis assay (AMA), are included in the EDSP Tier 1 battery (methods described in ). Results of these non-mammalian assays are described only briefly in this manuscript (Tables 9b, 10b, and 11b in Supplemental File 4), as these results are relevant to comparisons with ecological exposures that are not considered in this manuscript. For data rich compounds, such as PAIs, however, data are often available in more human-relevant species from 40 CFR Part 158 guideline toxicology studies (both in terms of taxonomy and in terms of exposure) in relation to reproduction and thyroid homeostasis. Therefore, the scope of this manuscript does not extend into detailed descriptions of all ecotoxicology data for these data-rich compounds, and instead focuses on two key objectives: (1) examination of the concordance across the three lines of evidence related to human/mammalian health effects; and (2) facilitation of a revised EDSP framework for data-rich chemicals via consideration of both human exposure estimates and potential endocrine activity. Qualitative review of the studies used to satisfy the EDSP Tier 1 data requirements is provided in ; any potential effects on the endocrine system are reported by pathway (estrogen, anti-estrogen, androgen, anti-androgen, steroidogenesis or anti-thyroid), and any other findings in the study (e.g., decreases in body weight [BW], changes in liver weight, or histopathology) are all reported in a separate column.
Table 4. EDSP Tier 1 assays plus published OSRI for the three triazoles.
Guideline toxicology studies relevant for demonstrating potential endocrine activity
PAIs are evaluated in a system of required guideline toxicity tests in mammals for registration for use. Together, these guideline studies constitute an important line of evidence for predicting endocrine bioactivity (). The multigenerational study in rodents provides the most endocrine-relevant information, including observation of reproductive performance, pregnancy and sexual maturation across two generations. Developmental toxicity studies in rodents and rabbits provide an assessment of reproductive and endocrine parameters in dams and offspring following gestational exposure. The developmental neurotoxicity study provides assessment of hypothalamic-pituitary-thyroid axis function following perinatal (gestational and postnatal) exposure. Several other types of studies, including sub-chronic, chronic and carcinogenicity studies, yield relevant information on endocrine tissues following repeat dosing. The presence of an apical effect in a guideline study may suggest more than one endocrine or non-endocrine hypothesis as to its origin. Often a lack of effects on endpoints related to endocrine function is more conclusive than the presence of an effect, especially when that effect may be caused by one or more modes-of-action (MOAs). Qualitative review of these studies is presented in ; again, any effects considered potentially endocrine-relevant are listed by pathway. Any additional effects (e.g., decreases in BW, changes in liver weight, or histopathology) are also listed in in a separate column. Key endpoints in 40 CFR Part 158 guideline toxicology studies related to endocrine system function, along with the limitations to their interpretation, are described in .
Table 5. Guideline toxicity studies.
Results
HTS information: line of evidence 1
present the HTS results for the three triazoles, organized by endocrine hypothesis, similar to the system of endocrine hypotheses suggested by Borgert et al. (Citation2014), including steroidogenesis (S), estrogenic (E), anti-estrogenic (anti-E), androgenic (A), anti-androgenic (anti-A), thyroid receptor agonist (TR) and thyroid receptor antagonist activity (anti-TR), as well as hepatic catabolism and thyroid hormone clearance (liver). Some of the HTS assays are repeated if they can be assigned to more than one hypothesis, e.g., an ER binding assay can inform both anti-estrogenic and estrogenic hypotheses. The number of positives out of the total number of assays for each hypothesis and the AC50 (μM) for each positive assay endpoint ID are indicated for the relevant endocrine hypothesis. The estimated “cytotoxicity limit” provided in the EPA EDSP21 Dashboard (actor.epa.gov/edsp21, Bioactivity tab) for each chemical is also indicated to assist in interpretation of these data; this cytotoxicity limit is simply a useful prediction, but not necessarily a strict “cut-off” for reporting these assay data. AC50 values at concentrations that exceed the cytotoxicity caution limit may represent assay positives of little to no biological relevance, and may have resulted from assay interference rather than selective in vitro activity. In addition, the available ToxCast AUC ER and AR model scores are reported for four hypotheses: E, anti-E, A and anti-A (USEPA Citation2014b; Browne et al. Citation2015). Pathway models for steroidogenesis and thyroid disruption are not yet available.
Table 6. Triadimefon: Endocrine-related HTS bioactivity data.
Table 7. Propiconazole: Endocrine-related HTS bioactivity data.
Table 8. Myclobutanil: endocrine-related HTS bioactivity data.
Table 9. Triadimefon: mammalian EDSP Tier 1 assay results plus published OSRI.
Table 10. Propiconazole: mammalian EDSP Tier 1 assay results plus published OSRI.
Table 11. Myclobutanil: mammalian EDSP Tier 1 assay results plus published OSRI.
Table 12. Triadimefon: Part 158 Guideline Toxicology Studies.
Table 13. Propiconazole: Part 158 Guideline Toxicology Studies.
Table 14. Myclobutanil: Part 158 Guideline Toxicology Studies.
The HTS data for triadimefon, propiconazole and myclobutanil can be compared and contrasted as a group based on the results shown in , respectively. In general, the HTS data suggest that these three chemicals activate markers of hepatic metabolism, possibly via interaction with nuclear receptors, including the constitutive androstane receptor (CAR) and pregnane-X receptor (PXR), that are consistent with previously published studies (Tully et al. Citation2006; Goetz & Dix 2009). HTS data also suggest that all three triazoles inhibit aromatase in vitro, but they have differing potency in these in vitro assays. Each of the three triazoles shows little to no potential to interact with ER and AR pathways directly. A detailed review of HTS information (from November 2014 release) for these three triazoles is described in Supplemental File 5.
EDSP Tier 1 assays plus published OSRI: line of evidence 2
An overview of the EDSP Tier 1 assays plus published OSRI for each chemical is provided in . A detailed, narrative review of the EDSP Tier 1 assays plus published OSRI for each chemical is provided in Supplemental File 5.
Effects on endocrine-sensitive reproductive measures were also assessed in the FSTRA (890.1350; see Supplemental File 4). The AMA (890.1100; Supplemental File 4) was used to screen for potential effects on thyroid signaling. This manuscript focuses on mammalian models, but further interpretation of the results of all three triazoles in the FSTRA and AMA is publicly available (USEPA Citation2015a), and a brief overview is provided in Supplemental File 4.
Triadimefon
Triadimefon does not appear to interact directly with the ER, AR, or TR pathways () (Willoughby Citation2012a–c). The EDSP Tier 1 assays for triadimefon suggest high-dose effects on steroidogenesis that include inhibition of aromatase (50% inhibitory concentration [IC50] range of 1.7–32 μM) and decreased testosterone levels (at 10–100 μM) in the H295R cell steroidogenesis assays () (Goetz et al. Citation2009). Although this direction of change is inconsistent with increased serum testosterone observed in rats in vivo in a male pubertal study design (Goetz et al. Citation2007), the combined results for triadimefon suggest some potential for disruption of steroidogenesis rather than a direct impact on AR signaling, as the Hershberger assay was negative (Davis Citation2011). Changes in estradiol production in the H295R assay were not dose-responsive (Goetz et al. Citation2009), and effects on relative ovary weight in a pubertal study design failed to correspond to changes in histopathology or serum estradiol, although vaginal opening (VO) was delayed and estrous cyclicity was transiently disrupted (Rockett et al. Citation2006). Neurotoxicity, liver toxicity, BW decreases and other signs of systemic toxicity likely represent the primary toxicities of triadimefon, and the inconsistent direction of change in testosterone between in vitro and in vivo models used to satisfy the data requirements of EDSP Tier 1 screening, along with any minor effects on estrogen-sensitive markers, may be a result of the interaction of these toxicities at high doses in vivo.
The AMA (Table 9b in Supplemental File 4), used to determine potential thyroid effects, was waived based on both OSRI and guideline toxicology studies that already demonstrated high-dose effects on thyroid histopathology and serum thyroid hormone concentrations in rodents. These high-dose effects are considered sequelae to primary effects on the liver (USEPA Citation2015a), as rat thyroid perturbation occurred at doses associated with upregulated hepatic catabolism and thyroid hormone clearance based on co-incidence of increased liver weight and hepatic centrilobular hypertrophy. Taken together, these potentially endocrine-related effects on the thyroid appear to require a dose that exceeds the threshold for induction of effects in the liver and other markers of systemic toxicity.
Propiconazole
The EDSP Tier 1 assays and published OSRI used to evaluate propiconazole identified effects at high in vitro concentrations on steroidogenesis that did not manifest consistent effects in in vivo studies in mammalian species in which the full steroidogenesis pathways and control mechanisms were present (). For example, a series of in vitro studies showed inhibition of aromatase by propiconazole with IC50 values of 0.968–8.25 μM, and steroidogenesis assays in H295R cells showed decreased testosterone and estradiol concentrations at ≥1 μM, but there were no consistent patterns of change that would reflect altered steroidogenesis in the variety of in vivo EDSP Tier 1 and OSRI studies (). Similarly, a series of in vitro assays suggests anti-androgenic potential of propiconazole at high concentrations, likely confounded by cytotoxicity, but corroborative anti-androgenic effects in the in vivo assays were not observed. Overall, liver and systemic toxicity appear to be the primary toxicities for propiconazole that are evident from mammalian in vitro and in vivo EDSP Tier 1 screening assays and OSRI.
Myclobutanil
The studies that satisfied the EDSP Tier 1 data requirements for myclobutanil suggest in vitro aromatase inhibition (an IC50 range of 0.1–47 μM) and decreased estradiol (≥1 μM) and testosterone production (1—100 μM) in in vitro steroidogenesis assays, with no evidence of any direct interaction with ER, AR or TR pathways (). Given the lack of evidence of anti-estrogenicity in EDSP Tier 1 in vivo study data, the relevance of decreased estradiol production in vitro is not supported by higher tier data. More specifically, the uterotrophic assay (Marty & Brooks, Citation2011) and the female pubertal assay (Marty et al. Citation2011) were negative. Myclobutanil was negative in the Hershberger assay (Marty et al. Citation2011), suggesting a lack of direct effect on the AR pathway. In the male pubertal assay, a significant decrease in testosterone levels correlated with a decrease in multiple androgen-dependent tissue weights and a slight delay in preputial separation (PPS) (Marty et al. Citation2011), but at doses that increased liver weights and hypertrophy. Alteration of testosterone homeostasis in mammals may also be influenced via activation of CAR, PXR and subsequent enzyme induction in the liver, as demonstrated by all three of these triazole fungicides (Goetz & Dix, 2009; Goetz et al. Citation2009). Therefore, although myclobutanil altered steroidogenesis in vitro (likely via aromatase inhibition), the effects on androgen-sensitive endpoints in vivo may result from more than one MOA, possibly including altered liver-mediated steroid hormone metabolism.
Thyroid-related changes in vivo were observed at high-dose levels in the male pubertal assay (Marty et al. Citation2011) and a published study (Wolf et al. Citation2006) ( and Supplemental File 5) in the presence of liver toxicity. These effects on serum thyroid hormones and thyroid histology likely occurred via a well-recognized indirect mechanism involving liver enzyme induction and subsequently increased clearance of thyroid hormones (Barter & Klaassen Citation1992; Hood et al. Citation1999; Vansell & Klaassen Citation2002).
Overall, myclobutanil altered in vitro steroidogenesis via aromatase inhibition, but in vivo changes in androgen-sensitive endpoints occurred in the presence of systemic toxicity, including increased liver weight and hepatocellular hypertrophy suggestive of enhanced enzyme induction in the liver and altered steroid hormone homeostasis.
Guideline toxicology studies: line of evidence 3
Guideline toxicology study results for triadimefon, propiconazole, and myclobutanil are presented in . Detailed narrative reviews of this information are provided for each chemical in Supplemental File 5.
Triadimefon
Available 40 CFR Part 158 guideline toxicology study information suggests high-dose effects of triadimefon on male mating behavior in rats in multi-generation studies, possibly resultant to altered steroidogenesis pathways and/or systemic toxicity (including a likely contribution of neurotoxicity via inhibition of dopamine reuptake), along with effects on hepatic metabolism in multiple species that may have increased thyroid hormone clearance and may be associated with a slightly increased incidence of hyperplasia and follicular cell adenomas in the thyroid of rats following chronic exposure (). Neither effects on male mating behavior nor potential effects on the thyroid in rats appeared to be mediated directly; there were no effects observed on sperm function or histopathology in endocrine-relevant tissues in a multi-generation study (Eiben Citation1984), and triadimefon effects on the thyroid were concomitant with liver effects and required high-dose (1800 ppm), chronic exposure in rats (Bomhard & Schilde Citation1991). Thus, a dietary exposure of 1800 ppm or doses of approximately 100 mkd triadimefon (dependent on size and age of the animal) appeared to correspond to a dose that may produce systemic toxicity, as well as some changes in rat mating behavior (and resultant fecundity) and perturbations in liver-mediated thyroid hormone homeostasis in rats.
Propiconazole
Guideline mammalian toxicology (40 CFR Part 158) studies in various mammalian species with propiconazole showed no consistent evidence of effects on estrogen, androgen, steroidogenesis or thyroid systems (). Sub-chronic and chronic toxicity studies in rat, mice and dogs did not show any effects on weights of endocrine-sensitive organs or histological changes, including testes, ovaries and thyroid. In mice and rats, effects in the liver were the most consistent target-organ toxicity, with an increase in hepatocellular adenomas and carcinomas in male mice at 2500 ppm. Any effects reported on offspring viability and BW (Borders & Salamon Citation1985) in the two-generation rat study appear to be evidence of offspring toxicity rather than a primary endocrine-mediated effect. Thus, dietary exposure of 2500 ppm or doses of approximately 200 mkd propiconazole (dependent on size and age of the animal) appear to correspond to systemic and liver toxicity, with any effects on offspring survival in the multi-generation reproduction study secondary to these primary effects.
Myclobutanil
A review of the 40 CFR Part 158 guideline toxicology data indicates that myclobutanil can affect male reproductive endpoints in rats at high-dose levels (). In studies with a high dose that did not exceed the maximum tolerated dose (MTD), male rats exhibited decreased prostate or testis weights and alterations in testicular histopathology and spermatogenesis. Although aromatase inhibitors have been shown to transiently alter spermatogenesis in male rats (Nunez et al. Citation1996; Gerardin & Pereira Citation2002; Pouliot et al. Citation2013), myclobutanil did not demonstrate the expected pattern of additional effects typically seen with potent aromatase inhibitors (Moudgal et al. Citation1996). In particular, ovarian histology and estrous cycles are thought to be very sensitive to aromatase inhibitors but were not affected by myclobutanil. Further, aromatase inhibitors have been associated with multiple developmental effects in rats, including decreased survival, fetal hematomata, and skeletal anomalies (Tamada et al. Citation2004; Tiboni et al. Citation2008, Citation2009), but these effects were not observed in the rat (or rabbit) developmental studies. Thus, myclobutanil did not display a consistent pattern of effects typical for aromatase inhibition, suggesting that in vivo this triazole may have more than one MOA for toxicity, including altered liver-mediated steroid hormone metabolism at high-dose levels. Thyroid effects (increased thyroid weight and/or histopathology) in the rat sub-chronic study appear to have been secondary to increased liver weights and hepatocellular hypertrophy; any observed thyroid effects appeared to be secondary to increased hepatic catabolism of T4 at high doses of myclobutanil. Overall, a dietary exposure of 200 ppm or doses of approximately 9.8–12.8 mkd myclobutanil appeared to correspond to a dose that may affect male reproductive endpoints via aromatase inhibition and/or liver toxicity, resulting in altered liver hormone homeostasis in rats.
Bioactivity concordance across three lines of evidence: HTS results, EDSP Tier 1 results and 40 CFR Part 158 guideline studies plus other OSRI
The purpose of this concordance assessment is to identify, on a qualitative basis, whether HTS results and 40 CFR Part 158 guideline studies, considered together in a weight-of-evidence approach, would have provided the needed information for EDSP screening. To evaluate this question, the degree of concordance across the three difference lines of evidence for each triazole fungicide is examined for each of the different endocrine pathways ().
Table 15. Triadimefon: Bioactivity concordance in mammalian toxicology assays across three lines of evidence.
Table 16. Propiconazole: Bioactivity concordance in mammalian toxicology assays across three lines of evidence.
Table 17. Myclobutanil: Bioactivity concordance across three lines of evidence.
Triadimefon
The HTS data, EDSP Tier 1 assays and published OSRI and 40 CFR Part 158 guideline toxicology tests were generally concordant for in vitro and in vivo endocrine activity hypotheses, when data were available from one or more lines of evidence for comparison (). The negative ToxCast ER and AR AUC model scores for triadimefon demonstrate bioactivity concordance with the in vitro screens and Hershberger results of the EDSP Tier 1 evaluation and the published OSRI studies that were similar to male and female pubertal studies (Rockett et al. Citation2006; Goetz et al. Citation2007). Triadimefon was classified as “non-interacting” in the guideline AR and ER binding assays (Willoughby Citation2012a, Citationb). Overall, it appears clear that any potential endocrine activity is associated with a high in vivo dose in rats of 1800 ppm. For triadimefon, the HTS results do not suggest estrogenic/anti-estrogenic or androgenic/anti-androgenic potential via a receptor interaction. Rather, these HTS data forecast a potential inhibition of aromatase activity using two separate assay platforms – cell-free and cell-based aromatase inhibition assays – but at concentrations that may exceed a cytotoxicity threshold. These data suggest a potential interference with steroidogenesis with low potency and at concentrations that might cause non-specific assay responses. Concordant with the Goetz et al. (Citation2009) steroidogenesis assay results, triadimefon decreased testosterone production levels in a testis organ culture from the same study, with simultaneous increases in progesterone production, suggestive of CYP17A1 inhibition (Goetz et al. Citation2009). In contrast, high-dose (1800 ppm) triadimefon exposure for 30 d increased serum testosterone levels in vivo in male rats (Goetz et al. Citation2009) and also increased serum testosterone in a perinatal exposure study in male rats (Goetz et al. Citation2007), but it did not change estradiol levels in these studies or in a study similar to a female pubertal assay (Rockett et al. Citation2006) (). For triadimefon, although it inhibited aromatase in vitro, the direction of effects on testosterone production was not consistent between in vitro and in vivo assays, possibly because any in vivo effects were obscured by systemic toxicity.
Effects on reproductive endpoints with triadimefon may have been mediated at least in part by systemic toxicity and the known effects of triadimefon on neurobehavior at these doses. Triadimefon exposure (1800 ppm) increased relative ovary weight and delayed VO in a published study similar to a female pubertal assay (Rockett et al. Citation2006) and delayed PPS, increased anogenital distance (AGD), increased testis weight and increased serum testosterone in the absence of any histopathological changes in the testes or effects on sperm morphology and motility in a published study similar to male pubertal assay (Goetz et al. Citation2007) (). Effects observed at 1800 ppm in the rat were coincident with systemic toxicity markers, including decreased BW and litter survival (Rockett et al. Citation2006; Goetz et al. Citation2007). Lower reproductive success for F1 male rats was also observed with triadimefon exposure at 1800 ppm in a study similar to a male pubertal (Goetz et al. Citation2007) and in two 2-generation reproduction studies (Loser & Lorke Citation1979; Eiben Citation1984). Goetz et al. (Citation2007) observed that mating 1800 ppm-treated males with control females still resulted in depressed fertility rates. These effects, however, were demonstrated to be isolated to male reproductive behavior, as when untreated male F1 rats were cross-mated with 1800 ppm-treated F1 females, the insemination and fertilization rates were not different from control (Eiben Citation1984).
Additional published reports (Crofton Citation1996; Walker & Mailman Citation1996) have demonstrated that triadimefon inhibits dopamine transporter-mediated uptake, which may contribute to decreased pup BW and lactation index (survival) via diminished milk production and altered maternal behavior (Richardson et al. Citation1984; Freeman et al. Citation2000; Ben-Jonathan & Hnasko Citation2001; Price & Bridges Citation2014), as well as contributing to altered male mating behavior and subsequent reduced fecundity in rat multi-generation studies. Thus, it appears that the HTS assay data, EDSP Tier 1 data, and guideline toxicology data were concordant, i.e., there was evidence for potential endocrine activity resultant to effects on steroidogenesis, which in vivo occurred at doses that also resulted in systemic toxicity and possibly neurotoxicity.
Concordance is also apparent for potential bioactivity related to thyroid signaling. All three lines of evidence suggest a potential activation of hepatic nuclear receptors, including CAR and/or PXR, which could result in increased metabolism and clearance of thyroid hormones in vivo (Goetz & Dix 2009). HTS assays also indicated that triadimefon might upregulate UGT1A1 and SULT2A1 expression in primary hepatocytes, enzymes known to catabolize T4 (thyroxine) and T3 (triiodothyronine) (Butt & Stapleton Citation2013; Tong et al. Citation2007; Richardson et al. Citation2014). Chronic triadimefon administration in rats increased liver weight, induced microsomal enzymes and caused centrilobular hypertrophy consistent with upregulated Phase I (and II) metabolism (Bomhard & Schilde Citation1991) (). This chronic, high-dose (1800 ppm) exposure to rats also resulted in a slight increase in the incidence of thyroid follicular cell adenomas and cystic hyperplasia (Bomhard & Schilde Citation1991), consistent with decreased thyroid hormones and increased thyroid stimulating hormone (TSH) signaling. Long-term exposures are absent from the EDSP Tier 1 battery, but a study similar to a male pubertal assay did not produce thyroid histological alterations despite decreased blood T4 levels at 1800 ppm (Goetz et al. Citation2007). Exposure of male rats to 1800 ppm triadimefon decreased T4 and T3 concentrations after 4 and 30 d, decreased TSH at 4 d of exposure, and increased follicular cell hypertrophy, colloid depletion and cell proliferation in the thyroid at 30 d of exposure; however, no thyroid effects were observed at 90 d of exposure (Wolf et al. Citation2006). In this same study, 1800 ppm triadimefon exposure induced hepatic UDP-glucuronosyltransferase (UDPGT) activity (with 1-naphthol as substrate) and increased hepatocellular hypertrophy. In a study similar to a male pubertal assay, relative liver weights increased, BW decreased and pup survival was reduced at this same dose (1800 ppm) (Goetz et al. Citation2007). In aggregate, the data suggest a primary effect on hepatic catabolism that may correlate with decreased serum thyroid hormones in rodents in vivo. Under chronic, high-dose exposure conditions, this may lead to increased TSH that can culminate in aberrant thyroid histopathology in rodents, a tumor pathway that is not considered human relevant (McClain Citation1995; IARC Citation1999; McClain & Rice Citation1999; Dellarco et al. Citation2006; Rouquie et al. Citation2014).
Propiconazole
Comparison of the three lines of evidence for propiconazole suggests in vitro inhibition of aromatase that is largely without endocrine-related apical effects in mammalian in vivo studies (). Thus, the HTS assay data for aromatase inhibition is concordant with in vitro assay data used to satisfy the EDSP Tier 1 data requirements and serves as a conservative screen, because related effects were not observed in vivo. For propiconazole, the AUC ER and AR model scores and HTS assay results were also concordant with the in vitro screens that were part of the EDSP Tier 1 testing and published OSRI. Propiconazole did not interact with the ER, and ER transactivation assays (HTS screens and EDSP Tier 1 results) demonstrated inconsistent results at high concentrations that were likely cytotoxic. The AUC AR model score for androgenic effects was 0, and this correlated with the lack of AR activation in the OSRI that satisfied the EDSP Tier 1 data requirements. An AUC score of 0.11 for anti-androgenic effects with propiconazole (indicating a weak positive) was concordant with OSRI study results, including four published in vitro studies () that displayed evidence of antagonism of the AR at relatively high concentrations (3–96 μM). In contrast, in vivo studies that were part of the EDSP Tier 1 testing plus OSRI and the guideline 40 CFR Part 158 in vivo studies showed no evidence of anti-androgenic activity with propiconazole.
Propiconazole was negative in the HTS screens related to thyroid receptor activation (0 of 3 assays). The overall pattern in HTS assays for anti-thyroid activity was generally negative, with some induction of hepatic CYP enzymes and a positive response in one of two thyroid antagonist assays (but at concentrations >50 μM). No in vitro assays for direct thyroid receptor effects were available for the EDSP Tier 1 battery, but ample evidence from in vivo EDSP Tier 1 assays and published OSRI indicated little to no evidence of thyroid activation nor of anti-thyroid effects. The one study with slight evidence of an anti-thyroid perturbation in rats (Wolf et al. Citation2006) was negative for morphological endpoints, i.e., there were no effects on thyroid histology or follicular cell proliferation. The liver UDPGT activity increase observed by Wolf et al. (Citation2006) with propiconazole used 1-naphthol (a phenolic compound) as substrate, and 1-naphthol is a primary substrate for UGT1A6 and a minor substrate for UGT1A1. In contrast, the ToxCast assays conducted in human primary hepatocytes, with mRNA levels of human UGT1A1 as the assay endpoint, were negative for propiconazole. Both UGT1A1 and UGT1A6 have been suggested to play a role in the conjugation of T4 (Vansell & Klaassen Citation2002), but some inherent differences in the inducibility, substrate specificity and relative contribution of glucuronidation versus sulfation to the catabolism of T4 between rats and humans has been shown (Richardson et al. Citation2014). Overall, a lack of induction of human UGT1A1 by propiconazole may be considered concordant with its comparatively limited effects on rat thyroid structure and function in the majority of in vivo studies. Propiconazole induced mRNA expression of SULT2A1 at one time point in the HTS screens; this sulfotransferase plays a possible role in sulfation and clearance of T3 (Butt & Stapleton Citation2013). In accordance with the weak evidence for altered thyroid catabolism in vitro, no alterations in thyroid histology were observed in 40 CFR Part 158 guideline studies of sub-chronic or chronic propiconazole exposure.
Propiconazole inhibited aromatase in vitro in two HTS assays, as well as in the EDSP Tier 1 aromatase inhibition assays that were satisfied by published OSRI. The HTS data demonstrated two out of two assays positive for aromatase inhibition, with AC50 values of 2 and 24 μM; published OSRI reported aromatase inhibition at similar concentrations (AC50 values from 1 to 8.25 μM), as well as altered steroidogenesis in two studies at 1–30 μM. The estimated cytotoxicity limit in ToxCast for propiconazole (4.6 μM) indicated that these findings might have occurred in the presence of cytotoxicity. Currently available HTS assays in the ToxCast/Tox21 dataset used in this analysis (USEPA Citation2014e) did not include any in vitro or in vivo tests for steroidogenesis, but the results from 40 CFR Part 158 guideline studies and EDSP Tier 1 screens plus OSRI were concordant in that a weak potential to alter steroidogenesis in vitro did not translate into a consistent or definitive pattern of effects in vivo. Across a wide spectrum of studies, the pattern in mammalian species was negative for effects that would be suggestive of a mechanism related to altered steroidogenesis with propiconazole. The HTS assay data for aromatase inhibition are qualitatively consistent with the EDSP Tier 1 in vitro assay data for steroidogenesis. Further, the HTS assay data are consistent with apparent effects in vivo on the liver and metabolism. In summary, comparison of the HTS in vitro data for aromatase inhibition and AR antagonism with in vivo data from the EDSP Tier 1 battery and from guideline toxicology studies highlights that HTS assay data are useful for conservative screening; i.e., although propiconazole inhibits aromatase and demonstrates weak AR antagonism in vitro, effects on steroidogenesis or androgen systems were not observed in vivo.
Myclobutanil
The HTS assay data and EDSP Tier 1 battery together suggest that myclobutanil may inhibit aromatase to mediate effects on the endocrine system, independent of direct ER and/or AR interactions, but at concentrations or in vivo doses that tend to coincide with systemic toxicity (). The ToxCast AUC ER and AR model scores for myclobutanil were negative, indicating that myclobutanil did not act as a direct agonist or antagonist with the ER or AR. These results were consistent with the EDSP Tier 1 in vivo mechanistic assays, as myclobutanil was negative in both the uterotrophic assay for estrogenicity and the Hershberger assay for anti-androgenicity/androgenicity.
The HTS results for myclobutanil indicated aromatase inhibition as a potential MOA for endocrine effects following myclobutanil exposure, with positive responses in both available aromatase inhibition assays (AC50 values = 0.672 μM and 5.16 μM), although one of these AC50 values exceeded the estimated cytotoxicity limit (4.69 μM). These data suggest an interaction with aromatase, which converts androgens to estrogens, at concentrations that may approach cytotoxicity. If aromatase was inhibited or steroidogenesis was otherwise perturbed in vivo, this MOA would not be detected by uterotrophic or Hershberger assays, and the female pubertal assay also may be relatively insensitive to aromatase inhibitors (Marty et al. Citation1999; USEPA Citation2007a). Accordingly, these EDSP Tier 1 studies were negative for myclobutanil. Although the results of the two in vivo male pubertal studies were inconsistent, i.e., decreased or increased testosterone, depending on the route of administration and different effects on androgen-sensitive organ weights and/or histopathology, the effects that were seen may suggest changes related to altered steroidogenesis. The relatively high-dose levels required to achieve these effects in vivo, however, produced concurrent systemic toxicity. Similar results were seen with the mammalian guideline toxicology database, wherein ovarian, testicular and reproductive effects were seen at ≥39, ≥ 10 and ≥80 mkd, respectively, generally in the presence of BW and/or liver weight changes, as well as histopathological changes.
With respect to thyroid homeostasis, HTS data did not indicate a direct interaction with thyroid hormone receptors, but it did suggest possible induction of hepatic enzymes that could correspond to enhanced thyroid hormone metabolism and clearance ( and Supplemental File 5). These HTS findings are in agreement with the minor thyroid histopathological changes and markers of hepatic enzyme induction observed in the male pubertal assay in which rats were dosed via oral gavage. These thyroid histopathological changes were observed in the EDSP Tier 1 studies only when myclobutanil was administered by bolus gavage at a high-dose level (400 mkd); signs of hepatic enzyme induction also were seen at this dose level, e.g., increased liver weight and hypertrophy. The amphibian metamorphosis assay was negative, supporting the conclusion that myclobutanil does not interact directly with thyroid hormone receptors (Supplemental Table 11b). The female pubertal assay was also negative for thyroid effects. Published OSRI did not indicate direct interaction with the hypothalamic–pituitary–thyroid axis (Goetz et al. Citation2006; Rockett et al. Citation2006; Tully et al. Citation2006). The lack of thyroid-related findings is also consistent with the guideline toxicology data, where no effects on thyroid parameters were observed unless the MTD was exceeded, i.e., in a sub-chronic rat study with dietary administration at 3000–10,000 ppm. Thus, the indirect thyroid effects observed in vivo in the male pubertal assay resulted only from exposures that exceeded the MTD, with increased T4 metabolism and clearance likely occurring together with systemic toxicity.
Thus, it appears that the HTS assay data, EDSP Tier 1 data, and guideline toxicology data for myclobutanil were concordant, i.e., there was evidence for aromatase inhibition and effects on hepatic metabolism that may correspond to increased enzymatic activity and perturbations of steroid hormone homeostasis. As such, the HTS data appear useful as a bioactivity screen, but toxicokinetics and exposure predictions are necessary to provide enough context to interpret the likelihood of observing hazard in vivo.
Bioactivity and exposure: use in prioritization
Science-based prioritization of chemicals for any further endocrine testing under the EDSP should depend on characterization of the margin separating predicted exposure and bioactivity. As an illustration of this concept, the use of high-throughput prioritization methods for the three data-rich triazoles in terms of further characterization of endocrine activity is demonstrated in this manuscript. The key sources of information for this analysis include HTS hazard information from ToxCast, high-throughput human exposure predictions from ExpoCast (Wambaugh et al. Citation2014) and the publicly available high-throughput toxicokinetic information, i.e., the 95th percentile of the predicted systemic steady-state concentration in humans following oral uptake of a daily 1 mkd administration (USEPA Citation2014b) (see Methods section and ) that was needed to convert in vitro AC50 values to human oral equivalent doses for comparison with exposure predictions.
In addition to determining the fit-for-purpose utility of this high-throughput prioritization, a parallel comparison of prioritizations based on high-throughput and more traditional risk assessment approaches is also illustrated. This comparison is critical because it clarifies whether or not HTS-based prioritization would have been conservative and human health protective to enable its use in place of running the EDSP Tier 1 screening battery. Traditional risk assessment approaches included a comparison of projected human exposures based on unrefined models and the oral dose associated with any endocrine-related changes in extensive testing using whole animal models, i.e., in in vivo mammalian guideline toxicology studies, as investigated in this work. Acceptance of high-throughput prioritization, particularly for PAIs or chemicals with extensive available data, necessitates that the prioritization system be adaptable to new information sources and similarly protective compared to traditional risk assessment approaches. present a comparison of high-throughput prioritization versus more traditional risk assessment approaches for endocrine prioritization for myclobutanil, propiconazole and triadimefon. As a tool to facilitate comparisons in these figures, the upper risk assessment panel (RA panel) compares the most recent range of human exposure estimates from EPA risk assessments to a lowest potentially endocrine active dose (LPEAD). The LPEAD is the lowest dose level in in vivo mammalian toxicology studies at which there are apical effects potentially related to endocrine systems. Establishing this dose is a conservative exercise for prioritization purposes, and it does not necessarily establish that those effects defining the LPEAD were in fact mediated by a primary endocrine mechanism. Seemingly endocrine-related effects may have resulted from mechanisms secondary to systemic toxicity, e.g., secondary to BW decreases or liver-mediated increases in hormone metabolism and clearance. In such instances, systemic toxicity could precede or occur concomitantly with endocrine-related effects at the LPEAD. Also included in is a dotted line indicator of the cytotoxicity caution flag; as indicated previously, this estimated “cytotoxicity limit” is not necessarily a strict “cut-off” for reporting HTS assay data. AC50 values at concentrations that exceed the cytotoxicity caution limit, however, may represent assay positives of little to no biological relevance and may have resulted from assay interference rather than selective in vitro activity. At this time, more research is needed to understand how to predict systemic or overt toxicity from in vitro data, and the cytotoxicity limit as defined within ToxCast may not necessarily be an indicator of the in vivo responses that would occur at equivalent exposure levels.
Triadimefon
RA panel, or values from traditional risk assessment approaches, demonstrates a five orders-of-magnitude separation between the US general population chronic aggregate exposure, a conservative measure of combined dietary and water exposure (USEPA Citation2009) (see Methods section), and the LPEAD that elicited systemic toxicity and potential endocrine effects in rats in multiple guideline toxicology studies. Specifically, effects observed at the triadimefon LPEAD (1800 ppm or approximately 100 mkd for the rat depending on lifestage) included increased incidence of thyroid follicular adenomas in the chronic rat study (Bomhard & Schilde Citation1991); decreased male mating behavior in the F1 generation of the multigenerational study (Loser & Lorke Citation1979); effects on body and liver weights and liver histopathology in multiple studies; increased serum testosterone, increased AGD and decreased male mating behavior in a study similar to a male pubertal assay (Goetz et al. Citation2007); and decreased serum T4 and other thyroid cellular changes in a non-guideline study (Wolf et al. Citation2006) ().
The lower prediction panel of illustrates that greater than five orders of magnitude separate the range of human oral exposures predicted by ExpoCast (Wambaugh et al. Citation2014) from the distribution of positive responses in ToxCast related to in vitro endocrine activity. The distribution of positive assay responses, especially at the lower end of the exposure range, corresponds largely to assay endpoints associated with upregulated hepatic metabolism (see ), which when considered in aggregate, are consistent with high-dose effects of triadimefon that may produce rodent liver histopathological changes and a slightly increased incidence of thyroid tumors following chronic exposure (see ). The assays corresponding to each white bar, or “bin,” are listed in Supplemental File 2. The current reference dose used in EPA risk assessments for triadimefon, 0.034 mg/kg-bw/d, is based on the no-observed-adverse-effect level (NOAEL) in the oral sub-chronic neurotoxicity study (3.4 mkd) with hyperactivity observed at higher doses; hyperactivity has also been observed in rats 30 min to 2 h following exposure to 50–60 mkd triadimefon (Crofton Citation1996). Thus, the risk assessment that was focused on the traditional endpoints and lowest dose effects is protective for any potential effects on the endocrine system, as these effects occur at much higher doses, if at all.
The white bars indicate the frequency or number of positive assay AC50 values at oral equivalent dose ranges or “bins”. Detailed review of the bins occurring at oral equivalent doses lower than the cytotoxicity caution flag (1.68 μM or 5.13 mkd) corresponded to hepatic catabolism assays, namely NVS_ADME_rCYP2B1 (1.54 mkd) and NVS_ADME_hCYP2C19 (3.62 mkd) (see and Supplemental File 2). Indeed, 28 of the 36 endocrine-related ToxCast AC50 values indicate effects on liver metabolism, generally at concentrations that exceed the cytotoxicity limit for triadimefon, which may indicate potential implications for systemic thyroid hormone clearance (refer to for endocrine-related positive HTS assay endpoints).
The estimated margin between the 95% confidence limit on the ExpoCast exposure prediction distribution and the lower end of the distribution of endocrine-related assay results from ToxCast is very similar to the safety margin between the EPA aggregate chronic exposure estimates and an effect level (LPEAD) from in vivo studies of endocrine relevance. The positive assay endpoints of endocrine relevance for triadimefon are largely related to hepatic catabolism with potential significance for increased systemic thyroid hormone clearance. This is concordant with the rat thyroid histological changes observed in vivo (Bomhard & Schilde Citation1991; Rockett et al. Citation2006), plus decreased serum T3 and T4 in the rat (Rockett et al. Citation2006; Wolf et al. Citation2006; Goetz et al. Citation2007) and increased hepatic glucuronyltransferase activity in the rat (Wolf et al. Citation2006) with 1800 ppm dietary exposure (100 mkd in rats). These in vivo data suggest that high-dose, prolonged exposures in rats result in liver toxicity with concomitant potential for increased thyroid hormone clearance that may correspond to thyroid histological changes in vivo, but at doses that may also result in systemic toxicity and that exceed exposure thresholds for neurotoxicity with triadimefon. Thus, using neurotoxicity for the point of departure protects for all other effects, including the potential perturbation of thyroid and steroid synthesis pathways.
Triadimefon appears to be of low priority for further endocrine activity screening, based on the large margins of separation between HTS predictions of exposure and effects (five orders of magnitude) and similar margins in a more traditional approach to estimating possible exposure and hazard (five orders of magnitude).
Propiconazole
For propiconazole, a closer examination of the HTS assays that are binned together in the graph () at the lower dose levels (0.03–0.514 mkd) indicates that the positive results for propiconazole at lower estimated doses (Bins 1–4) were all assay endpoints related to liver metabolism or transporter enzymes ( and Supplemental File 2). Because no evidence of thyroid effects was observed in the in vivo 40 CFR Part 158 guideline studies with propiconazole (), these in vitro activities related to thyroid hormone catabolism are considered adaptive changes in the liver that have no effect on the endocrine-relevant risk evaluation for propiconazole. At slightly higher concentrations (Bins 5–8 in Supplemental File 2; equivalent to 1.486–4.999 mkd), the majority of the assays are again related to thyroid hormone catabolism, with the exception of one assay related to estrogenic effects and one indicating CYP19A1 (aromatase) inhibition related to steroidogenesis. As discussed earlier, the other HTS assay for aromatase inhibition was positive at 23.56 μM (21.4 mkd), which is well above the estimated cytotoxicity limit. All the other bins (5.3–64.3 mkd) are above the estimated cytotoxicity limit (4.6 μM = 4.15 mkd) for propiconazole and are thus of lower relevance, considering the potential for confounding as a result of cytotoxicity in the HTS assays (dotted line in ).
Approximately four orders of magnitude separate the range of predicted human oral exposures in ExpoCast (Wambaugh et al. Citation2014) from the lowest estimated effect level (Bin 1; 0.03 mkd) of any ToxCast result for propiconazole. Greater than five orders of magnitude separate the ExpoCast estimated exposures from the lowest endocrine-relevant response from ToxCast (Bin 5; 1.486 mkd).
The LPEAD, represented by a triangle in the top panel of , represents the dose from the two-generation reproduction study in rats with propiconazole (2500 ppm =200 mkd) that is pertinent to endocrine activity evaluation. Effects at this dose included parental and offspring effects such as decreased BW and offspring survival; there were no effects on reproduction (USEPA Citation2014d). The separation between the LPEAD and the US general population chronic aggregate exposure (dietary and water combined; 0.00743 mkd, see Methods section) (USEPA Citation2014d) represents a margin of greater than four orders of magnitude.
In summary, the estimated margin between ExpoCast human exposure estimates and endocrine-related HTS assay results is very similar to the margin separating EPA aggregate chronic exposure estimates and an effect level (LPEAD) from in vivo mammalian studies of possible endocrine relevance. Further, the types of effects seen at lower estimated doses in the ToxCast assays (hepatic enzyme induction) are of low relevance to endocrine pathways given the lack of thyroid effects in the in vivo profile of propiconazole. These in vitro HTS results may point to the potential for adaptive hepatic effects, which were seen at lower doses, e.g., 50 mkd, in in vivo rodent studies with propiconazole. Propiconazole appears to be of low priority for further endocrine activity screening based on the large margins of separation between HTS predictions of exposure and effects (five orders of magnitude) and similar margins in a more traditional approach to estimating possible exposure and hazard (four orders of magnitude).
Myclobutanil
The main panel of shows the range of predicted human oral exposures for myclobutanil from ExpoCast [median, upper 95% confidence limit from Wambaugh et al. Citation2014] versus the frequency distribution of possible endocrine-related bioactivity hits in ToxCast, represented as oral equivalent doses (mkd). Seven positive ToxCast assay responses occur in Bins 1–4 at oral doses equivalent to ∼1–10 mkd, which are below the cytotoxicity caution flag (4.69 μM), represented as a dotted line. These positive responses correspond to assay endpoints related to aromatase inhibition (Bin 1; AC50 = 1.18 mkd) and hepatic enzyme induction (Bins 1–4), consistent with induction of hepatic metabolism observed with myclobutanil in vivo.
RA panel shows the separation between the chronic aggregate exposure estimate from the US general population (0.005013 mkd) (see Methods section) (USEPA 2007b, Citation2008) and the LPEAD (9.84–12.86 mkd). The LPEAD, represented by a triangle in the top panel of , represents the lowest dose of myclobutanil from the chronic rat study that is relevant for endocrine evaluation, based on male reproductive endpoints via aromatase inhibition and/or liver toxicity resulting in altered liver hormone homeostasis in rats.
The estimated margins betweenthe HTS prioritization approach and the traditional risk assessment approach were similar. There were five orders of magnitude between the oral doses corresponding to the ExpoCast 95% confidence limit on predicted human exposure and the lowest oral equivalent doses for endocrine-related HTS bioactivity. In comparison, the separation between the LPEAD and the US general population chronic aggregate exposure was greater than three orders of magnitude.
Both these margins demonstrate conservative separation between predicted exposure and potential bioactivity. Overall, myclobutanil appears to be of low priority for further endocrine activity screening, based on these large margins between HTS-based predictions of exposure and effects, as well as a more traditional approach to estimating exposure and hazard.
Comparison to the integrated bioactivity and exposure ranking approach
An integrated bioactivity and exposure ranking (IBER) approach was developed by EPA (USEPA Citation2014b; Browne et al. Citation2015) as a means of prioritizing the universe of approximately 10,000 chemicals that could be potentially subject to EDSP evaluation. Bioactivity predictions for the IBER approach to prioritization are derived from pathway-based models for ER and AR that utilize multiple receptor-based assay endpoints using assay data from ToxCast and Tox21. AUC model scores and IBER scores for the three triazoles are shown in .
Table 18. AUC model and IBER values for the three triazoles.
The current ER-based bioactivity model, or the ToxCast ER AUC model, incorporates information from 18 ER pathway-based assays (16 agonist and/or receptor binding assays, and two antagonist assays) and employs thresholds for positive response scores, with the ER agonist and antagonist AUC model score threshold ≥0.1 for a positive. The AR-based bioactivity model, or the ToxCast AR AUC model, similarly incorporates information from nine AR pathway assays (seven agonist and/or receptor binding assays, plus two antagonist assays) and employs thresholds for positive response scores, with the AR agonist threshold score ≥0.1 for a positive, and the AR antagonist threshold score ≥0.05 for a positive. All three of these triazoles were negative for ER agonist, ER antagonist, and AR agonist pathway activity using the AUC model approach. Propiconazole was a weak positive for AR antagonist activity using the AUC model approach.
The IBER score is the ratio between minimum positive activity, i.e., the oral equivalent exposure for threshold activity in the pathway model, and the maximum (95th percentile) predicted human exposure using high-throughput methods, i.e., ExpoCast (Wambaugh et al. Citation2014). To derive this score, the chemical concentration predicted to result in the minimum (threshold) model activity is converted to an oral equivalent using high-throughput toxicokinetic modeling (USEPA Citation2014b). This value is then divided by the 95th percentile of maximum predicted human exposure. This ratio is the IBER score. A score of 106 is a default for a negative score, arising from a lack of AUC model activity, i.e., cannot divide zero by a predicted exposure.
These three triazoles demonstrated negative ER IBER scores because they did not have any associated ER pathway activity in the model. Similarly, myclobutanil and triadimefon demonstrated negative AR IBER scores. Propiconazole demonstrated a weak positive AR antagonist AUC score (0.111), but the predicted 95th percentile exposure (1.77 E-6 mkd; ) is so low that the AR IBER value reflects a wide margin of separation (643056), and thus a lack of priority. The IBER score for propiconazole is the sixth IBER score from the bottom of the list for positive AR IBER scores (USEPA Citation2014b). Strong positive IBER scores for AR were demonstrated for testosterone propionate (0.000101) and selective AR modulators, such as 17β-estradiol (110) and linuron (4357), with scores reflecting a combination of potency and potential for human exposure. Thus, the IBER system suggests that these three triazoles would be of little to no priority for further ER or AR pathway-related screening or testing under the EDSP.
Discussion
This case study examined the concordance among three lines of evidence for endocrine activity for a small set of well-studied triazole fungicides: (1) HTS results, (2) EDSP Tier 1 assay results (plus published OSRI), and (3) 40 CFR Part 158 guideline mammalian toxicology studies. In addition, human exposure estimates from a high-throughput modeling approach (ExpoCast) were compared with oral equivalent doses for positive findings in ToxCast/Tox21 HTS assays to assess the relative priority of these compounds for further endocrine screening and testing. After compiling this information for triadimefon, propiconazole and myclobutanil, the four critical questions listed below are addressed:
Are HTS assay data currently sufficient for an endocrine prioritization task, and does the current HTS battery (ToxCast/Tox21) provide appropriate biological coverage for each of the sub-types of endocrine pathways (estrogen, androgen, thyroid and steroidogenesis)?
Are HTS information, guideline toxicology data, and the EDSP Tier 1 assays sufficiently concordant in this example such that HTS prioritization would be possible? In other words, are there major biological gaps or false negatives in HTS that would have affected EDSP evaluation and risk assessment for these three PAIs?
Is prioritization based on high-throughput exposure and bioactivity predictions as protective of human health as traditional risk assessment approaches?
In the absence of the EDSP Tier 1 battery, could human health-protective prioritization decisions be made regarding the need for any additional hazard and exposure information using HTS prioritization alone or in combination with the available 40 CFR Part 158 guideline toxicology study information?
Regarding biological coverage in HTS assays for each of the sub-types of endocrine pathways (estrogen, androgen, thyroid and steroidogenesis)
The HTS assays currently available via ToxCast and Tox21 provide bioactivity pathway coverage for ER- and AR-mediated activity, effects on steroidogenesis via inhibition of aromatase and potential effects on thyroid hormone homeostasis via thyroid hormone receptor assays and markers of nuclear receptor-mediated hepatic catabolism. The question of suitable ToxCast assay coverage to predict in vivo outcomes in the EDSP Tier 1 battery was previously investigated using ToxCast Phase I assay information and led to the conclusion that, at that time, enough assay data for predictive modeling purposes was likely only available for ER and AR pathways (Rotroff et al. Citation2013). In agreement with this earlier assessment, the current case studies with three data-rich triazole fungicides indicated that sufficient HTS assays are available to cover estrogen, anti-estrogen, androgen and anti-androgen effects, based on their ability to predict the potential (or lack of potential) for estrogen and androgen pathway effects in the 40 CFR Part 158 guideline studies and EDSP Tier 1 testing of these molecules.
There are some known gaps in the current ToxCast/Tox21 assay battery related to steroidogenesis and thyroid pathways (Rotroff et al. Citation2013; Paul et al. Citation2014) that limit current efforts to develop a predictive system for these endocrine activities, but predictive models for these pathways are the subject of ongoing research (USEPA Citation2014b). Despite known limitations inherent to current screening assay technologies in the HTS set, combining HTS information with 40 CFR Part 158 guideline toxicity testing and published OSRI comprises a viable strategy for determination of potential endocrine activity and the presence of possible data gaps that could be addressed by any further endocrine activity evaluation for these PAIs. Importantly, for data-rich chemicals, thyroid and steroidogenesis information can be inferred from guideline and published non-guideline toxicology studies as a second step in the prioritization process. In line with reduction and refinement of animal resources, this type of approach would also focus further screening and testing on clear areas of unknown hazard, and this reflects the approach taken by EPA in recently released weight-of-evidence documents for evaluating the Tier 1 data for the EDSP List 1 chemicals (USEPA Citation2015a).
At the time this manuscript was written, the HTS battery of ToxCast and Tox21 included two assays to predict aromatase inhibition as indicators for potential impact on steroidogenesis, indicating a potential screening gap for other enzymatic or transport functions necessary for timely steroid production (Stoker et al. Citation2000; Rotroff et al. Citation2013). HTS using the H295R steroidogenesis model has been conducted, and the data were publicly released in November 2015; however, these results are not part of the current manuscript. Further, a systems biology model for steroidogenesis similar to the relatively new models for integrating multiple assay predictors for the ER and AR pathways is not yet available, but this is anticipated as part of ongoing work by EPA and the Tox21 initiative (USEPA Citation2014b). At this time, it is clear that in the case of these PAIs with large toxicology databases, a weight-of-evidence approach that combines HTS information on aromatase inhibition and in vivo data from guideline toxicology studies would have provided sufficient data for prioritization for EDSP. Once a more complete systems biology model is available for steroidogenesis that covers a wider range of steroidogenic enzymes, and the predictive accuracy of this model can be compared to results from aromatase and steroidogenesis assays, in addition to in vivo pubertal and multi-generation study results, a further assessment of the sufficiency of HTS methods for predicting perturbed steroidogenesis, without considering other data sources, will become possible.
Another key limitation of current HTS is the absence of screening assays for all the known molecular-initiating events of thyroid perturbation. Thyroid hormone homeostasis is regulated at multiple nodes, including synthesis in the thyroid gland as modulated by the sodium-iodide symporter and thyroperoxidase; release and transport of thyroid hormones systemically; peripheral conversion of T4 to T3 by deiodinases; increased systemic clearance related to upregulation of nuclear receptor-regulated pathways of thyroid hormone metabolism and transport; systemic transport via binding to serum proteins; and, potentially, interactions with thyroid hormone nuclear receptors in target cells (Murk et al. Citation2013). As of this analysis, ToxCast/Tox21 coverage for direct thyroid receptor interaction is available, and some data are available for nuclear receptor-mediated and thyroid hormone-related hepatic catabolism. In November 2015, additional assays that detect possible in vitro inhibitors of thyroperoxidase were added to the ToxCast assay suite (Paul Friedman et al. Citation2016). HTS data are not available in the ToxCast/Tox21 assay results for many other bioregulatory nodes related to thyroid function (Paul et al. Citation2014), although the relative importance of these additional nodes and the proportion of thyroid-perturbing agents that act via these nodes is not fully known at this time. Additional HTS assays for steroidogenesis and thyroid perturbation would support development of systems biology models to predict endocrine system interactions via many different MOAs, outside of aromatase inhibition and effects on hepatic catabolism of thyroid hormones.
Similar to our conclusion for steroidogenesis pathway coverage, a weight-of-evidence approach that combines HTS information on hepatic catabolism endpoints related to thyroid hormone homeostasis and in vivo data from guideline toxicology studies would have provided sufficient data for prioritization for EDSP for these three triazoles. Once a more complete systems biology model is available for thyroid hormone regulation, including thyroidal and extrathyroidal endpoints (Murk et al. Citation2013), and the predictive accuracy of this model can be compared with results from in vivo study results, further assessment of the sufficiency of HTS methods for predicting perturbed thyroid hormone homeostasis, without inclusion of other data sources, will move forward.
Regarding concordance of HTS information, guideline toxicology data and the EDSP Tier 1 battery plus published OSRI for each triazole
A key result of this effort is the finding that HTS provides an informative line of evidence for endocrine prioritization that is concordant with the EDSP Tier 1 assays plus published OSRI and the 40 CFR Part 158 guideline toxicology study set for these three triazole fungicides. The combined ToxCast and Tox21 HTS assay battery provides multiple, orthogonal endocrine bioactivity predictions related to the ER and AR pathways; this multi-assay coverage has enabled the development of systems biology models to integrate these data for increased reliability based on greater accounting for sources of assay interference and degree of concordance across technologies (Rotroff et al. Citation2014; USEPA Citation2014b; Browne et al. Citation2015). The resultant models, referred to as the ToxCast ER and AR AUC models, enable greater utilization of these receptor pathway data because false positives in various assay technologies are less likely to affect the overall model result. The ToxCast ER AUC model has been suggested as a replacement for screens in the EDSP Tier 1 battery, including the ER binding and transactivation assays and the uterotrophic assay, based on the high predictivity of this model for uterotrophic assay study results (USEPA Citation2014b, Citation2015b). The results of the ER and AR AUC models for estrogenic, anti-estrogenic, androgenic and anti-androgenic effects were concordant with the outcomes of the EDSP Tier 1 assays for the three triazole fungicides evaluated in this manuscript. All three triazoles are considered negative for ER pathway activity in HTS.
Triadimefon and myclobutanil were negative for any effects on AR binding in the assays and in the AR AUC models. Propiconazole demonstrated a very high Ki binding constant for AR (indicating a low affinity) and also demonstrated a marginally positive anti-androgenic ToxCast AR AUC model score (0.111) based on AR antagonism and binding, but at concentrations that are likely irrelevant to in vivo models. This anti-AR score was concordant with OSRI study results, in which four published in vitro studies () provided evidence for antagonism of the AR but only at relatively high concentrations (3–96 μM). This weak in vitro signal, however, did not translate into any evidence of anti-androgenic effects with propiconazole based on in vivo studies. Propiconazole was negative in EDSP Tier 1 assays and/or OSRI that satisfied requirements for the Hershberger assay (Kjaerstad et al. Citation2007; Taxvig et al. Citation2008) and the male pubertal assay (Tully et al. Citation2006; Goetz et al. Citation2007), and no evidence of adverse anti-androgenic effects exists in the 40 CFR Part 158 guideline toxicology database.
In summary, the HTS results and EDSP Tier 1 battery did not suggest primary effects on ER or AR pathway signaling for any of the three triazoles. Despite a small number of weak positive results in HTS anti-estrogen assays and in the anti-androgen assays, all three triazoles were negative in the Hershberger assay, and they failed to demonstrate consistent effects on estrogenic signaling in either a female pubertal assay design (Rockett et al. Citation2006) or in the uterotrophic assay (though the uterotrophic study was waived for triadimefon). These comparisons indicate that the ER and AR AUC models would have been reasonable substitutes for the ER- and AR-related assays in the EDSP Tier 1 screening battery for these triazoles.
All three triazoles inhibited aromatase (CYP19A1) in both assays available in the HTS battery. This is consistent with prior knowledge that triazoles have the potential to inhibit certain isoforms of CYP enzymes, based on the common fungicidal MOA for triazoles via inhibition of Cyp51 in fungi (Trosken et al. Citation2006b). These HTS results correctly correspond to the in vitro aromatase assay results in the EDSP Tier 1 battery: all three triazoles inhibited aromatase in the OCSPP 890.1200 guideline test and/or in various publications deemed OSRI. Though the HTS predictions and EDSP Tier 1 aromatase and steroidogenesis assays were in agreement, the apical outcomes observed in the in vivo portion of the EDSP Tier 1 battery varied by triazole. Thus, in vitro inhibition of aromatase may provide important screening information, but it does not necessarily translate into endocrine-mediated effects in more complex study designs, such as the male and female pubertal assays or two-generation reproduction studies. As shown in , the AC50 values in both HTS aromatase inhibition assays were lower for myclobutanil than for triadimefon or propiconazole. The EDSP Tier 1 plus published OSRI results for aromatase inhibition were concordant with this pattern: myclobutanil had an IC50 = 0.1 μM in a guideline aromatase assay, compared with values of 1–32 μM for propiconazole and triadimefon (). All three triazoles inhibited steroidogenesis in H295R cells (Goetz et al. Citation2009), but further delineation of relative potency was not possible from this published assay. The direction of change in testosterone or estradiol levels varied by compound at lower concentrations (1–3 μM), but these triazoles tended to decrease concentrations of both estradiol (propiconazole and myclobutanil) and testosterone (all three triazoles) at concentrations >3 μM (Goetz et al. Citation2009).
Table 19. AC50 values in HTS aromatase inhibition assays for the three triazoles
For triadimefon, although it inhibited aromatase in vitro, the direction of effects on testosterone production was not consistent between in vitro and in vivo assays, possibly because any in vivo effects were obscured by systemic toxicity. As discussed previously in Triadimefon section, both increases and decreases in testosterone were reported in vivo, and concurrent effects were seen in the presence of systemic toxicity, including decreased BW and litter survival (Rockett et al. Citation2006; Goetz et al. Citation2007). Effects of triadimefon on reproductive success for F1 male rats at 1800 ppm in a study similar to a male pubertal assay (Goetz et al. Citation2007) and in two-generation reproduction studies (Loser & Lorke Citation1979; Eiben Citation1984) resulted in decreased male reproductive behavior and may have reflected a non-endocrine inhibition of dopamine reuptake with triadimefon (Crofton Citation1996). This assessment is consistent with the weak potential for triadimefon to inhibit steroidogenesis and the lack of any effects on testis or ovary micropathology or sperm parameters. The simultaneous demonstration of systemic toxicity in vivo at doses that may have caused neurotoxicity underscores the idea that aromatase inhibition may not have occurred in rat studies in vivo, or may have occurred, but only at doses above those that produced other non-endocrine toxicities.
Propiconazole inhibited aromatase in vitro, but it failed to elicit anti-estrogenic or androgenic responses that would be consistent with aromatase inhibition in vivo in rodent models of the EDSP Tier 1 assays or in the 40 CFR Part 158 guideline toxicology studies. Propiconazole did not produce any consistent, treatment-related changes in serum testosterone or estradiol levels in any mammalian species in the in vivo EDSP Tier 1 assays (). Propiconazole, as described in this case study, had no effects on reproduction in rats at dose levels up to 2500 ppm (200 mkd). A slightly lower number of F2A pups delivered, pups delivered viable, and pups surviving to postnatal day (PND) 4 did not match precisely the effects in F2B litters, in which numbers of pups delivered were similar to controls, but survival was slightly lower at PND7–21 (Borders & Salamon Citation1985). This minor effect in the F2 litters with propiconazole is considered a marker of offspring systemic toxicity, as indicated by large BW deficits at this high dose in dams and in pups.
Myclobutanil inhibited aromatase in vitro, and some of the effects observed in vivo might have been a result of aromatase inhibition. These effects, however, were observed only at doses that coincided with liver or systemic toxicity. Dietary myclobutanil exposure (2000 ppm or approximately 153.5 mkd) resulted in androgenic effects – including increased AGD in male pups, increased testes weight, increased testosterone levels and decreased male reproductive success (without any effect on sperm parameters or histology of androgen-sensitive organs) – in a published male pubertal study cited as OSRI (Goetz et al. Citation2007). These effects were contrary to the anti-androgenic effects observed in the guideline male pubertal assay that employed gavage exposures (Marty et al. Citation2011). At a high-dose level of 400 mkd in the guideline male pubertal assay (Marty et al. Citation2011), anti-androgenic effects including decreased testosterone, slightly delayed PPS and decreased androgen-sensitive organ weights (in the absence of histopathology changes) were observed (). It is noteworthy that the dose levels at which these effects were observed also accompanied by hepatic changes, including increased liver weights and hypertrophy in both the studies.
Myclobutanil increased AGD in female pups, delayed VO and increased ovary weights (with no effects on serum estradiol or estrous cyclicity) in a published female pubertal assay cited as OSRI (Rockett et al. Citation2006). In contrast, a guideline in vivo EDSP Tier 1 pubertal assay in female rats (Marty et al. Citation2011) with myclobutanil demonstrated a lack of effects on estradiol levels, VO or weight and histology of estrogen-sensitive organs (). The 40 CFR Part 158 guideline chronic rat studies with myclobutanil at dose levels ≥200 ppm demonstrated increased incidences of testicular atrophy, decreased testes weight, aspermatogenesis and hypospermia in the epididymides (). In a rat reproduction study, myclobutanil exposures of 1000 ppm increased testis and prostrate atrophy, decreased spermatozoa in the testis and epididymides (P2 generation only), decreased reproductive parameters and increased numbers of stillborn pups following mating. Other sensitive endpoints typically altered by aromatase inhibitors were not affected, e.g., estrous cyclicity and ovarian histopathology (refer to Myclobutanil section), and moreover, the contribution of liver effects to altered steroid metabolism cannot be excluded, as liver changes were seen at similar or lower dose levels. Thus, these results, while concordant with in vitro data, may be confounded by other MOAs, e.g., liver metabolism, contributing to the in vivo effects observed.
Regarding thyroid effects, all three triazoles were negative for direct effects on the thyroid hormone receptor in the HTS results. Concordant with the HTS results, the amphibian metamorphosis assay was negative for thyroid-mediated effects with propiconazole and myclobutanil. The AMA assay was waived for triadimefon, but the wider database for triadimefon suggested a weak potential for effects on rodent thyroid at 1800 ppm in the chronic rat study, a dose that also increased liver weights and decreased BW (Bomhard & Schilde Citation1991). Further data with triadimefon indicated that the rodent thyroid effects most likely were secondary to increased clearance of thyroid hormones, via induction of liver metabolism enzymes. In addition, the effects on the thyroid via this indirect mechanism were more consistently observed in the database for triadimefon than for propiconazole or myclobutanil.
All three triazoles likely affected nuclear receptor activation (discussed further below) based on increased expression of some specific Phase I enzymes, e.g., CYP2B, CYP3A and Phase II conjugation enzymes (e.g., UGT1A1 and/or SULT2A1) that could modulate thyroid hormone catabolism (Rotroff et al. Citation2010; Paul et al. Citation2013). Differences between the three triazoles, however, were identified in the potential for thyroid changes from in vivo rat studies that were part of the 40 CFR Part 158 guideline studies and the in vivo studies that were part of the EDSP Tier 1 plus published OSRI data. Triadimefon produced histologic changes in the rat thyroid in a chronic rat study, including cystic hyperplasia and a very slight increase in follicular cell adenomas at 1800 ppm (Bomhard & Schilde Citation1991). Effects with triadimefon in a sub-chronic rat study were limited to increased thyroid weight in females only at 2000 ppm (Mohr Citation1976), and a published study similar to a female pubertal assay demonstrated mild follicular hypertrophy in females at 1800 ppm (Rockett et al. Citation2006), but no effects were seen in males at 1800 ppm in a published study similar to the male pubertal assay (Goetz et al. Citation2007). A 90-d mechanistic study demonstrated thyroid histopathological changes, including follicular cell hypertrophy, colloid depletion and cell proliferation at 30 d, along with decreased T4 and T3 at 4 and 30 d, but not at 90 d (Wolf et al. Citation2006). These authors suggested that the mechanism for the observed thyroid effects was via increased T4 clearance resulting from induction of UGT1A1 and similar isoforms, but this induced catabolism was likely not of sufficient magnitude to result in a measurable increase in TSH levels at the time points that were examined. Myclobutanil and propiconazole exposures were not associated with any changes in thyroid weight or histology in rat sub-chronic and chronic studies; they were also not associated with any increased incidence in thyroid tumors. Myclobutanil exposure increased relative thyroid weights and increased the number of small follicles in the sub-chronic rat study at 3000–10,000 ppm, doses that exceed the MTD for myclobutanil. The study by Wolf et al. (Citation2006) demonstrated decreased T4 and/or T3 at 4 and 30 d of propiconazole or myclobutanil exposure at the same doses that increased liver weights, but with no increases in TSH and no histologic changes in the thyroid at any measured time-point (4, 30 or 90 d). Thus, all three triazoles may upregulate hepatic catabolism of T4 and T3 in the rat, but with varying potential to affect downstream key events related to the thyroid gland at the in vivo doses tested.
The HTS assay results for enzyme activities that are known to be related to thyroid hormone conjugation and clearance are summarized in for the three triazoles. UGT1A1 is one enzyme responsible for conjugation and subsequent biliary excretion of T4, and SULT2A1 is one enzyme responsible for conjugation and clearance of T3 (Butt & Stapleton Citation2013). Triadimefon induced both UGT1A1 and SULT2A1, whereas propiconazole (SULT2A1) and myclobutanil (UGT1A1) each induced only one of these enzymes. The AC50 values for each of these activities were similar across the three triazoles. From this limited HTS dataset, it is uncertain whether greater induction of these conjugating enzymes for T4 and T3 by triadimefon may correlate with its slightly greater tendency to ultimately produce histological changes in the thyroid of rats compared to propiconazole and myclobutanil. Future development of systems biology models of thyroid hormone metabolism may increase understanding of how the potency and efficacy of chemical effects in in vitro model systems may relate to thyroid homeostasis in vivo; currently such a model is unavailable.
Table 20. AC50 values in HTS assays for UGT1A1 and SULT2A1 induction.
The in vitro HTS bioactivity predictions suggest that myclobutanil, propiconazole and triadimefon target the liver, likely via interaction with nuclear receptors (CAR, PXR and/or possibly others) that act as transcriptional activators of Phase I and II metabolism enzymes, as well as hepatic transport proteins. Although the majority of the nuclear receptor reporter assays and CYP or Phase II enzyme induction assays in ToxCast are for human forms, the 40 CFR Part 158 guideline toxicity studies and EDSP Tier 1 testing protocols are primarily based on rat or mouse results. Depending on the assay endpoint, some species differences can occur in the activation profile for a particular chemical structure. For example, published studies have demonstrated that propiconazole and a closely related triazole (etaconazole) are potent activators of mouse and rat CAR in a CAR3 reporter assay, but only weak activators of human CAR (Omiecinski et al. Citation2011; Currie et al. Citation2014). In accordance with the HTS results, it is possible that specific nuclear receptors, e.g., CAR and/or PXR, may also be operative in the liver responses to each of these three triazole fungicides, and this response may be divergent by species (Omiecinski et al. Citation2011; Paul et al. Citation2013). However, the pattern of induction of Phase I and Phase II metabolism enzymes and liver transporters for the three triazoles, based primarily on human isoforms tested in ToxCast Phase I, is concordant with the established sub-chronic and chronic studies in rodents, where the liver is a key target organ. The EDSP Tier 1 assays produced concordant results, including increased microsomal enzyme induction, liver weights and incidence of centrilobular hypertrophy with the three triazoles. While these effects in the liver may not be directly related to the endocrine systems being evaluated in EDSP Tier 1 testing, they are important in terms of (1) a possible role of liver enzyme activities in steroidogenesis (either via induction or inhibition); (2) a possible role of liver enzyme induction in catabolism of thyroid hormones; and (3) the possible role that liver toxicity may play in contributing to systemic toxicity and/or establishing the MTD for these three triazoles.
In conclusion, the HTS data were useful as a conservative screen to delineate the endocrine pathways that were not affected by these triazoles, i.e., the ER, AR and TR pathways, as well as steroidogenesis and thyroid hormone metabolism pathways that may be affected. In vitro positives related to steroidogenesis and thyroid hormone metabolism did not always correspond to effects in vivo in higher-tier studies, but these “false positives” can be tolerated in screening because further screening and testing, along with the potential for exposure, can be used to put these bioactivity predictions in the appropriate context.
Regarding prioritization for endocrine testing based on high-throughput human exposure and bioactivity predictions
In addition to examination of the agreement between these three separate lines of evidence for endocrine activity screening, we present in this manuscript a visualization tool () to facilitate endocrine prioritization decisions based on high-throughput human exposure and bioactivity predictions. The goal of this visualization is to simultaneously compare high-throughput exposure and bioactivity predictions and estimates of exposure and hazard using more traditional risk assessment approaches, and then to determine whether the margin of separation would have been similar using either of the two approaches and/or conservative enough to be used in prioritization. EPA has suggested using such an approach, i.e., integrated bioactivity and exposure ranking (IBER), when limited data are available to characterize the potential for a chemical to demonstrate endocrine activity (USEPA Citation2014b). Thus, provide key examples of how such an approach might have worked had it been available to the EDSP during the evaluation of List 1 chemicals. The case studies presented herein suggest that prioritization using high-throughput predictions would have been human health protective for these PAIs.
In the upper panel of , the distance separating the LPEAD (based on mammalian in vivo study outcomes) and EPA chronic aggregate exposure estimates is illustrated. This degree of separation is compared to the outputs available from high-throughput endocrine screening (ToxCast/Tox21) and high-throughput human exposure estimates (ExpoCast) for that molecule. For triadimefon, the separation of the high-throughput values (greater than five orders of magnitude) was similar to the separation of EPA exposure estimates and the LPEAD values (greater than four orders of magnitude). For propiconazole, the separation of the high-throughput values was virtually the same as EPA exposure estimates and the LPEAD value (each greater than four orders of magnitude). For myclobutanil, the separation via the high-throughput values (greater than five orders of magnitude) was slightly greater than the separation between EPA exposure estimates and the LPEAD (greater than three orders of magnitude), but both demonstrated a high degree of conservatism for prioritization and risk assessment, respectively.
Consideration of these safety margins for potential endocrine activity of the three example triazoles that were evaluated indicates two important conclusions. First, the margins of separation attained using HTS bioactivity and human exposure methods provide similar margins to those using traditional in vivo toxicity studies and EPA human exposure estimates. Second, based on the large margins of separation between predicted bioactivity and exposure, triadimefon, propiconazole and myclobutanil should be of low priority for additional endocrine testing.
In reviewing the margins separating the LPEAD and the chronic aggregate exposure estimate, it should be underscored that traditional EPA human health risk assessment processes would have been protective of any endocrine activity observed in in vivo mammalian studies in the EDSP Tier 1 battery or on endocrine-related endpoints in 40 CFR Part 158 guideline toxicology studies. Triadimefon provides a clear example of a chemical for which any potentially endocrine-relevant effects clearly do not affect the human health risk assessment because other effects occur at much lower doses. The human health risk assessment for triadimefon is based on increased motor activity in rats that received a sub-chronic exposure, with a lowest-observed-adverse-effect level (LOAEL) of 54.6 mkd and a NOAEL of 3.4 mkd (USEPA Citation2009). The NOAEL for hyperactivity is approximately 3% of the LPEAD presented in . Triadimefon is thought to block reuptake of dopamine, leading to hyperactivity in rodents (Crofton Citation1996). A chronic reference dose is derived by dividing this NOAEL by 10 for rat to human interspecies extrapolation and another factor of 10 for intraspecies variability. The Food Quality Protection Act safety factor has been set to “1” because no increased prenatal or postnatal susceptibility was identified in a developmental neurotoxicity study with rats. Thus, the chronic reference dose used in a relatively unrefined risk assessment process is 0.034 mkd (USEPA Citation2009). These dose levels can be compared to the dose necessary to elicit effects on male mating behavior or mildly increased incidence of thyroid tumors in rats with triadimefon (1800 ppm or approximately 100 mkd in rats), which are used to set the LPEAD in . In the guideline multi-generation studies for triadimefon, 1800 ppm dietary exposures (approximately 100 mkd) in rats produced a number of findings, including liver toxicity and BW effects, as well as effects on male mating behavior, some minor changes in reproductive tissue weights, and minor effects on serum testosterone concentrations. In chronic 2-year exposures in rats, the same dose, 1800 ppm, elicited a slightly higher incidence of follicular adenomas in the rat thyroid concomitantly with histopathological findings in the liver and BW decrements. The NOAEL for these effects in both studies was 300 ppm, which is equivalent to approximately 15 mkd in the rat. Assuming similar uncertainty factors are appropriate for this NOAEL based on possible endocrine-related effects (100×), the chronic reference dose would be approximately 0.15 mkd, which is approximately four-fold higher than the chronic reference dose based on hyperactivity in rodents. Thus, from a risk perspective, neither the effects on male mating behavior (and resultant fecundity) in the multi-generation study nor the rodent thyroid tumors in the rat chronic study would affect the human health risk assessment of triadimefon. For propiconazole, EPA defines the chronic reference dose as 0.1 mkd based on non-neoplastic liver effects seen in a 24-month mouse study with a NOAEL of 10 mkd (USEPA Citation2014d); this NOAEL is less than 5% of the LPEAD in . For myclobutanil, EPA defines the chronic reference dose as 0.025 mkd, based on testicular atrophy in the chronic rat study with a NOAEL of 2.49 mkd (USEPA Citation2008), approximately 25% of the LPEAD. It is important to note that the exposure assessments used to compare to the LPEAD are largely unrefined and conservative (Methods section), indicating that the margins between the LPEAD and EPA exposure range estimates are likely even greater than the values shown herein; thus, the margins suggested by the approach illustrated in the top panels of are likely underestimated. This illustrates the protective nature of the current risk assessment process for PAIs, regardless of the target toxicity.
In this case study, published values from EPA risk assessments, HTS data from the ToxCast/Tox21 programs and ExpoCast exposure estimates were used for comparisons. The Css from a high-throughput toxicokinetic prediction approach was also published by EPA in Scientific Advisory Panel materials from July 2014 (USEPA Citation2014c). It is understood by stakeholders that the high-throughput toxicokinetic Css values, ExpoCast exposure ranges and even AC50 values from ToxCast are approximations helpful to prioritization, but they are not refined measures of human exposure, hazard or pharmacokinetic/pharmacodynamic parameters for chemicals. For screening, these values are useful in determining relative priority for further endocrine testing. As such, the Css values could be refined via further measurement of the biological variables for each PAI, e.g., fraction unbound in plasma, intrinsic metabolic clearance and glomerular filtration rate, which are involved in estimation of the Css (Wetmore et al. Citation2012; USEPA Citation2014c). Similarly, human exposure estimates could be further refined based on current risk assessment approaches to better capture use scenarios and environmental fate, as the estimated values used in this assessment were largely unrefined (see Methods section). These opportunities for refinements to inputs for science-based prioritization provide a mechanism for chemical registrants to supply additional information as needed to correct relative prioritization, noting that these refinements may only be needed when a relative priority ranking indicates a priority that is likely under- or over-estimated.
Regarding whether, in the absence of the EDSP Tier 1 assays, human health-protective prioritization decisions could be made regarding the need for any additional hazard and exposure information
The three triazole fungicides that were used as the source of data for evaluation in the present paper have a rich dataset developed from assessment in HTS assays (ToxCast/Tox21), 40 CFR Part 158 guideline toxicology studies and EDSP Tier 1 assays along with published OSRI that expands the available knowledgebase of toxicity and mechanistic testing for these PAIs. In fact, PAIs represent one of the most studied groups of chemicals in global commerce. Based on this rich dataset, these compounds provide a worthy case study to evaluate the strength and predictive power of high-throughput endocrine screens in combination with high-throughput human exposure predictions to correctly assess the priority of a chemical for EDSP testing.
As discussed earlier, good concordance between HTS assays and the EDSP Tier 1 assay results was observed regarding the absence of ER agonism, ER antagonism, AR agonism and AR antagonism for all three triazoles. Propiconazole had a weak AR antagonism AUC value (0.111) that was matched by consistent effects in the in vitro EDSP screens related to this endpoint, but only at high concentrations; the lack of evidence for any AR antagonist effects in the full in vivo database with propiconazole confirmed that this weak signal was not translated into effects within the whole organism. Based on the lack of effects in these pathways from the HTS results and the 40 CFR Part 158 guideline toxicology studies, the three triazoles would be of low to no priority for any further testing of direct effects on estrogen or androgen signaling. This lack of direct effects on ER and AR pathway activity was confirmed in the EDSP Tier 1 assays and published OSRI.
Some perturbations of thyroid catabolism signals were observed for the three triazoles in the HTS assays, with triadimefon possibly having a slightly greater signal (based on both UGT1A1 and SULT2A1 induction) than propiconazole or myclobutanil. The HTS data demonstrated a potential upregulation of hepatic catabolism that could affect thyroid hormone homeostasis, as reflected in the mild histologic changes in the thyroid of rats treated with high doses (1800 ppm) of triadimefon over a chronic period (Bomhard & Schilde Citation1991) and decreased T4 and/or T3 in rats with the same dose (1800 ppm) over shorter exposure periods (Rockett et al. Citation2006; Wolf et al. Citation2006; Goetz et al. Citation2007). Based on the HTS assay data that suggested upregulated markers of Phase I and Phase II metabolism for triadimefon as well as myclobutanil and propiconazole, however, prediction of the presence or degree of in vivo changes in thyroid hormone homeostasis or thyroid histology is currently limited without consideration of in vivo study information, e.g., from 40 CFR Part 158 guideline toxicology studies, male/female pubertal assays in the EDSP Tier 1 battery, or alternative mechanistic studies. The HTS dataset indicated that effects on regulation of thyroid hormone catabolism were of weak potency and likely occurred at concentrations equivalent to or in excess of the estimated cytotoxicity limit, in accordance with the in vivo database, which suggested for all three triazoles that adaptive metabolic changes (at lower dose levels) and adverse histopathology changes (at higher dose levels) occurred in the livers of rats and mice.
Finally, for steroidogenesis, HTS assays suggested aromatase inhibition for all three triazoles, concordant with aromatase inhibition and steroidogenesis perturbation signals observed in the in vitro aromatase inhibition and steroidogenesis assays in the EDSP Tier 1 battery and OSRI. The in vivo effects of the three triazoles, however, varied greatly, as already discussed. Propiconazole did not produce clear effects in mammalian species that related to this pathway, including a two-generation reproduction study in rats and pubertal male and female studies (Rockett et al. Citation2006; Goetz et al. Citation2007) where no effects on reproductive success were observed. The effects for triadimefon on F1 male mating behavior are likely secondary to systemic toxicity and/or the neuroactivity of triadimefon following increased exposure in the F1 generation. For myclobutanil, because of the presence of histologic findings in the testis and prostate, along with decreased sperm counts, these effects may result from concomitant aromatase inhibition and systemic toxicity.
In , we present a visual tool for contextualizing HTS indicators of endocrine-related bioactivity with HTS human exposure predictions. Despite the differences between the three triazoles in the eventual in vivo outcomes of EDSP Tier 1 testing and the 40 CFR Part 158 guideline toxicity studies, these comparisons in show a consistent pattern across all three compounds, namely, that at least three orders of magnitude on a log scale separate the high-throughput predicted exposures and the lowest oral equivalent dose for endocrine-related HTS assay positive results. This wide margin of separation was very similar to the differences between EPA chronic aggregate exposure estimates based on unrefined models, which are generally conservative, and the LPEAD, building confidence that the use of HTS-based prioritization is sufficiently human health protective for prioritization-level decision making related to endocrine activity. Clearly, for compounds with wide margins separating endocrine-related HTS bioactivity assay results and high-throughput human exposure predictions, similar to those demonstrated by the dataset for myclobutanil, propiconazole and triadimefon, there would be little to no priority for further EDSP Tier 1 screening.
An approach for the evaluation of PAIs for endocrine potential is presented in . This roadmap enables an organized, science-based assessment of the potential for endocrine effects in combination with the potential for human exposure. High-throughput bioactivity and exposure information should be considered first; in the future, given further development of integrated, predictive bioactivity models, this step may be comprehensive. For PAIs undergoing EDSP evaluation currently, evaluation of the available high-throughput data for bioactivity and exposure should be followed by examination of corresponding 40 CFR Part 158 guideline toxicology studies, published OSRI and other exposure information to reach a decision point based on the combined assessment of all existing data. Three possible outcomes of the initial decision are (1) more hazard data are needed; (2) more exposure data are needed; or (3) sufficient data are available to both describe the overall prioritization and perform a risk assessment. For most PAIs, a rich bioactivity database is likely to be available, and thus a very limited need for additional hazard data, i.e., EDSP Tier 1 testing or additional exposure information, would be anticipated. Somewhat similar methods for systematic evaluation of endocrine potential, including available bioactivity and exposure information, have been proposed previously to make science-based decisions and reduce animal and resource usage (Willett et al. Citation2011).
Figure 5. Proposed roadmap for EDSP evaluation of pesticide active ingredients (PAIs). For PAIs, the available high-throughput (HT) hazard and exposure information would be considered first, followed by evaluation of the available guideline toxicology study information before making any priority decisions. Priority decisions could include decisions to collect additional hazard or exposure information, or to make a risk assessment (RA) decision based on adequate available data or suitable margins of separation between predicted exposure and bioactivity.
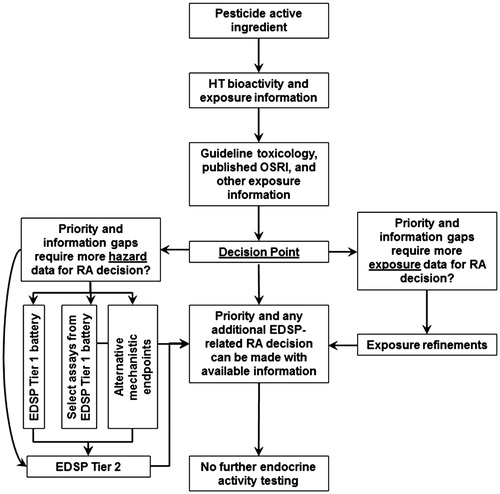
Additionally, the Organization for Economic Co-operation and Development (OECD) has previously developed a conceptual framework (OECD Citation2012) for interpreting information related to potential endocrine activity from different study types and sources. HTS data, including data from the ToxCast and Tox21 research programs, were not specifically addressed in this OECD framework. Based on the present case study, we assert that HTS assay data, like that available from the ToxCast and Tox21 initiatives, can be considered in a systematic evaluation as conducted herein as prioritization-level information, in concert with human exposure predictions, to make human health-protective prioritization decisions. Furthermore, the HTS bioactivity data and 40 CFR Part 158 guideline toxicology data together comprise a data resource that should be completely reviewed when available to generate the best science-based prioritization decision, thereby optimizing resources used for any additional endocrine activity testing.
Conclusions
For three data-rich triazole fungicides (triadimefon, propiconazole and myclobutanil), the HTS assay dataset was concordant with two other lines of evidence (40 CFR Part 158 guideline toxicology studies and the combined EDSP Tier 1 screens plus published OSRI) in the types of endocrine activity that it predicted. The combined lines of evidence indicated that these three triazoles do not directly act on estrogen, androgen or thyroid systems. In vitro evidence of altered steroidogenesis via inhibition of aromatase was observed for all three triazoles, but the degree to which these in vitro properties were expressed in mammals in vivo differed greatly. Comparison of the endocrine-related HTS bioactivity information with high-throughput human exposure predictions demonstrated the following conclusions:
Comparison of HTS for endocrine-related bioactivity with high-throughput exposure estimates was useful for prioritization of these data-rich compounds.
The margins between high-throughput exposure predictions and the endocrine-related HTS bioactivity (converted to oral mkd dose levels) were similar to the margins between unrefined EPA exposure estimates and apical outcomes of in vivo studies related to potential endocrine effects (LPEAD). These margins (three to five orders of magnitude) were human health protective and suitable for EDSP screening prioritization decisions.
Based on the wide margins present between estimates of predicted bioactivity and human exposure, compounds with profiles like those of triadimefon, propiconazole and myclobutanil would have been of low priority for further endocrine screening and testing, had this methodology been available prior to EDSP Tier 1 screening.
Using an EDSP prioritization roadmap for PAIs that is outlined within this manuscript, whereby a prioritization decision would have been made via sequential consideration of HTS for bioactivity and exposure and the 40 CFR Part 158 guideline toxicology studies and other available information (), it is unlikely that the EDSP Tier 1 assay results would have been necessary to make a prioritization and risk assessment decision for these three triazoles.
Abbreviations | ||
abs | = | absolute |
admin | = | administration |
AMA | = | amphibian metamorphosis assay |
A | = | androgenic pathway |
BW | = | body weight |
BWG | = | body weight gain |
d | = | day |
↓ | = | decreased |
dep | = | dependent |
dev | = | development or developmental |
E | = | estrogenic pathway |
♀ | = | female |
GD | = | gestational day |
histopath | = | histopathology |
↑ | = | increased |
inhib | = | inhibition |
♂ | = | male |
mkd | = | mg per kg BW per day |
PND | = | postnatal day |
rel | = | relative |
repro | = | reproductive or reproduction |
S | = | steroidogenesis |
T | = | thyroid/thyroid pathway |
VTG | = | vitellogenin |
wk | = | weeks |
wt | = | weight |
Supplemental_Files_Friedman_et_al..zip
Download Zip (848 KB)Acknowledgements
Marco Corvaro, Kerry Hastings, Daland Juberg and James R. Wheeler (Dow AgroSciences); Curt Lunchick, Russell Jones, Tilghman Hall and Lisa Ortego (Bayer CropScience); and Arpad Szarka and Paul Hinderliter (Syngenta Crop Protection, LLC), are gratefully acknowledged for critical review and/or contributions to the content of this manuscript. The authors gratefully acknowledge the extensive and insightful comments of three reviewers selected by the Editor and anonymous to the Authors. The review comments were very helpful in guiding revision of the manuscript.
Declaration of interest
The contents of this manuscript are solely the responsibility of the authors and do not necessarily reflect the views or policies of their employers. All authors are employed by registrants of triazole fungicides. In the current manuscript, interpretations of EDSP Tier 1 studies, OSRI (including published results), and 40 CFR Part 158 guideline studies are based on the data as presented, and as interpreted by the current authors after viewing the wider database for each test substance. Discussion or interpretation of results from individual publications by the authors of those publications is identified, when needed.
References
- Andersen DM, O’Hara, GP, Brown WR. 1993. RH-3866: dietary oncogenicity study in female mice. final report: Report No. 89R-261. Unpublished study prepared by Rohm and Haas Co. MRID 42809102.
- Barter RA, Klaassen CD. 1992. UDP-glucuronosyltransferase inducers reduce thyroid hormone levels in rats by an extrathyroidal mechanism. Toxicol Appl Pharmacol. 113:36–42.
- Bauer ER, Bitsch N, Brunn H, Sauerwein H, Meyer HH. 2002. Development of an immuno-immobilized androgen receptor assay (IRA) and its application for the characterization of the receptor binding affinity of different pesticides. Chemosphere. 46:1107–1115.
- Ben-Jonathan N, Hnasko R. 2001. Dopamine as a prolactin (PRL) inhibitor. Endocr Rev. 22:724–763.
- Bomhard E, Schilde B. 1991. MEB 6447: Chronic toxicity and cancerogenicity studies on Wistar rats with administration in diet over a period of 105 weeks: lab project number: 20774: 101922. Unpublished study prepared by Bayer AG, Dept of Toxicology. MRID 42153901:1029 p.
- Borders C, Salamon C. 1985. Two-generation reproduction study in albino rats with CGA-64250 technical. Unpublished study prepared by Toxigenics, Inc. MRID 00151514.
- Borgert CJ, Stuchal LD, Mihaich EM, Becker RA, Bentley KS, Brausch JM, Coady K, Geter DR, Gordon E, Guiney PD, et al. 2014. Relevance weighting of tier 1 endocrine screening endpoints by rank order. Birth Defects Res Part B: Dev Reprod Toxicol. 101:90–113.
- Browne P, Judson RS, Casey W, Kleinstreuer N, Thomas RS. 2015. Screening chemicals for estrogen receptor bioactivity using a computational model. Environ Sci Technol.
- Butt CM, Stapleton HM. 2013. Inhibition of thyroid hormone sulfotransferase activity by brominated flame retardants and halogenated phenolics. Chem Res Toxicol. 26:1692–1702.
- Coder P. 2012. Propiconazole – uterotrophic assay in ovariectomized rats (OECD guideline 440; US EPA OPPTS 890.1600): Unpublished study prepared by WIL Research Laboratories, LLC, Ashland, OH USA. MRID 48619003.
- Costlow R, Kane W. 1984a. Teratology study with RH-53,866 in rats: Final Report: Report No. 83R-024. Unpublished study prepared by Rohm and Haas Co. 123 p. MRID 00141672.
- Costlow R, Kane W. 1984b. Teratology study with RH-53, 866 in rabbits: Report No. 83R-217. Unpublished study prepared by Rohm and Haas Co. 139 p. MRID 00164971.
- Costlow R, Harris J. 1985. RH-53,866: two generation reproduction study in rats: Report No. 84R-117. Unpublished study prepared by Rohm and Haas Co. 225 p. MRID 00149581.
- Coady KK, Sosinski LK. 2011. Myclobutanil: evaluation of myclobutanil in the human recombinant aromatase assay. Unpublished study prepared by Toxicology & Environmental Research and Consulting, The Dow Chemical Company, Midland, MI. Laboratory Project Study ID No.: 111015, November 28, 2011. MRID 48618103.
- Crofton KM. 1996. A structure–activity relationship for the neurotoxicity of triazole fungicides. Toxicol Lett. 84:155–159.
- Clemens G, Jasty V, Troup C, Hartnagel R. 1990. A toxicity study in the rabbit with MEB 6447 (Triadimefon): Report No. 100035. Upublished study prepared by Miles Laboratories, Inc. MRID 41616005: 179 p.
- Currie RA, Peffer RC, Goetz AK, Omiecinski CJ, Goodman JI. 2014. Phenobarbital and propiconazole toxicogenomic profiles in mice show major similarities consistent with the key role that constitutive androstane receptor (CAR) activation plays in their mode of action. Toxicology 321:80–88.
- Davis J. 2011. The Hershberger bioassay (OPPTS 890.1400); triadimefon. Unpublished study prepared by ILS, Integrated Laboratory Systems, Inc. MRID 48619604:107.
- Dellarco VL, McGregor D, Berry SC, Cohen SM, Boobis AR. 2006. Thiazopyr and thyroid disruption: case study within the context of the 2006 IPCS Human Relevance Framework for analysis of a cancer mode of action. Crit Rev Toxicol. 36:793–801.
- Eiben R. 1984. MED 6447 (Triadimefon): Two-Generation Study with Rats: Supplementary Study: Report No. 12712. Unpublished study prepared by Bayer AG, Institute of Toxicology. MRID 155075:168p.
- Fritz H. 1984a. Expanded report on CGA-64250 technical segment II teratology study in rats. Project No. 790011, 21 September 1984. Unpublished study prepared by Ciba-Geigy. Syngenta File No. CGA64250/2150. MRID 00058604.
- Fritz H. 1984b. Expanded report on CGA64250 technical segment II reproductive study in rabbits. Project No. 790009. Unpublished study prepared by Ciba-Geigy. Syngenta File No. CGA64250/2151. MRID 00058605.
- Freeman ME, Kanyicska B, Lerant A, Nagy G. 2000. Prolactin: structure, function, and regulation of secretion. Physiol Rev. 80:1523–1631.
- Gerardin DC, Pereira OC. 2002. Reproductive changes in male rats treated perinatally with an aromatase inhibitor. Pharmacol Biochem Behav. 71:301–305.
- Giknis M. 1987. CGA 64250 technical: teratology (segment II) study in rats. Project No.86004. Syngenta File No. CGA64250/1586. Unpublished study prepared by Ciba-Geigy. MRID 40425001.
- Goetz AK, Bao W, Ren H, Schmid JE, Tully DB, Wood C, Rockett JC, Narotsky MG, Sun G, Lambert GR, et al. 2006. Gene expression profiling in the liver of CD-1 mice to characterize the hepatotoxicity of triazole fungicides. Toxicol Appl Pharmacol. 215:274–284.
- Goetz AK, Dix DJ. 2009a. Mode of action for reproductive and hepatic toxicity inferred from a genomic study of triazole antifungals. Toxicol Sci. 110:449–462.
- Goetz AK, Dix DJ. 2009b. Toxicogenomic effects common to triazole antifungals and conserved between rats and humans. Toxicol Appl Pharmacol. 238:80–89.
- Goetz AK, Ren H, Schmid JE, Blystone CR, Thillainadarajah I, Best DS, Nichols HP, Strader LF, Wolf DC, Narotsky MG, et al. 2007. Disruption of testosterone homeostasis as a mode of action for the reproductive toxicity of triazole fungicides in the male rat. Toxicol Sci. 95:227–239.
- Goetz AK, Rockett JC, Ren H, Thillainadarajah I, Dix DJ. 2009. Inhibition of rat and human steroidogenesis by triazole antifungals. Syst Biol Reprod Med. 55:214–226.
- Goldman P, Harris J. 1986. RH-3866: Dietary chronic and oncogenicity study in mice: Final Report: Report Number 84R-023. Unpublished study prepared by Rohm and Haas Co. 3326 p. MRID 00164990.
- Goldman P, Harris J, Lampe K. 1986a. RH-3866: a three-month dietary toxicity study in mice: Report No. 83R-136. Unpublished study prepared by Rohm & Haas Co. 522 p. MRID 00165245.
- Goldman P, Harris J, Frantz J. 1986b. RH-3866: one year dietary study in Beagle dogs: Protocol No. 84P-203: Report No. 84R-078. Unpublished study prepared by Rohm & Haas Co. 775 p. MRID 00165248.
- Hester SD, Wolf DC, Nesnow S, Thai SF. 2006. Transcriptional profiles in liver from rats treated with tumorigenic and non-tumorigenic triazole conazole fungicides: propiconazole, triadimefon, and myclobutanil. Toxicol Pathol. 34:879–894.
- Hoffman K, Groning P. 1978. Meb 6447 long-term toxicity study on dogs (two-year feeding study): Report No. 7882; Report No. 66656. Unpublished study received Apr 28, 1980 under 3125-318; prepared by Bayer, AG, submitted by Mobay Chemical Corp, Kansas City: Mo; CDL: 099413-D. MRID 32539.
- Hunter B, Slater N, Heywood R. 1982a. CGA 64 250: potential tumorigenic and toxic effects in prolonged dietary administration to rats: CBG 193/8284 (Test No. 789023). Final rept. Unpublished study prepared by Huntingdon Research Centre, England. MRID 00129918.
- Hunter B, Scholey D, Haywood R. 1982b. CGA 64 250: long-term feeding study in mice. CBGI195/81827. Final Report. Unpublished study prepared by Huntingdon Research Centre, England. MRID 00129570.
- Hood A, Hashmi R, Klaassen CD. 1999. Effects of microsomal enzyme inducers on thyroid-follicular cell proliferation, hyperplasia, and hypertrophy. Toxicol Appl Pharmacol. 160:163–170.
- Hurst MR, Sheehan DA. 2003. The potential for oestrogenic effects of pesticides in headwater streams in the UK. Sci Total Environ 301:87–96.
- IARC. 1999. Species Differences in Thyroid, Kidney and Urinary Bladder Carcinogenesis, IARC Scientific Publication No. 147. International Agency for Research on Cancer, Lyon, France.
- Johnson W, Thompson S. 1985. One-year subchronic oral toxicity study in Beagle dogs with CGA-64250 technical (Final Report). FDRL Study No. 7737. Unpublished study prepared by Research Laboratories, Inc. 570 p. Syngenta File No. CGA64250/1544. MRID 00151515.
- Jones RL. 2005. Comparison of regulatory estimates of drinking water concentrations with monitoring data. J Agric Food Chem. 53:8835–8839.
- Judson RS, Magpantay FM, Chickarmane V, Haskell C, Tania N, Taylor J, Xia M, Huang R, Rotroff DM, Filer DL, et al. 2015. Integrated model of chemical perturbations of a biological pathway using 18 in vitro high throughput screening assays for the estrogen receptor. Toxicol Sci. 148:137–54.
- Kavlock R, Chandler K, Houck K, Hunter S, Judson R, Kleinstreuer N, Knudsen T, Martin M, Padilla S, Reif D, et al. 2012. Update on EPA's ToxCast program: providing high throughput decision support tools for chemical risk management. Chem Res Toxicol. 25:1287–1302.
- Kjaerstad MB, Andersen HR, Taxvig C, Hass U, Axelstad M, Metzdorff S, Vinggaard AM. 2007. Effects of azole fungicides on the function of sex and thyroid hormones. Pesticides Research No. 111.
- Kojima H, Katsura E, Takeuchi S, Niiyama K, Kobayashi K. 2004. Screening for estrogen and androgen receptor activities in 200 pesticides by in vitro reporter gene assays using Chinese hamster ovary cells. Environ Health Perspect. 112:524–531.
- Laville NP, Brion F, Hinfray N, Casellas C, Porcher J-M, Ait-Aissa S. 2006. Modulation of aromatase activity and mRNA by various selected pesticides in the human choriocarcinoma JEG-3 cell line. Toxicology. 228:98–108.
- LeBaron MJ, Schisler MR, Visconti NR. 2011a. Evaluation of myclobutanil in an in vitro estrogen receptor binding assay. Unpublished study prepared by Toxicology & Environmental Research and Consulting, The Dow Chemical Company, Midland, MI. Laboratory Study No.: 111118, December 14, 2011. MRID 48618104.
- LeBaron MJ, Schisler MR, Visconti NR. 2011b. Evaluation of myclobutanil in an in vitro androgen receptor binding assay. Unpublished study prepared by Toxicology & Environmental Research and Consulting, The Dow Chemical Company, Midland, MI. Laboratory Study No.: 111113, December 5, 2011. MRID 48618102.
- LeBaron MJ, Kan HL. 2011c. Evaluation of myclobutanil in an in vitro estrogen receptor transcriptional activation assay in human cell line hERα-HeLa-9903. Unpublished study prepared by Toxicology & Environmental Research and Consulting, The Dow Chemical Company, Midland, MI. Laboratory Report No.: 101193, December 12, 2011. MRID 48618105.
- LeBaron MJ, Kan HL, Perala AW. 2011d. Evaluation of myclobutanil in the in vitro steroidogenesis assay. Unpublished study prepared by Toxicology & Environmental Research and Consulting, The Dow Chemical Company, Midland, MI. Laboratory Study No.: 101192, December 5, 2011. MRID 48618110.
- Loser E, Lorke D. 1979. Meb 6447 Multigeneration Reproduction Study on Rats: Report No. 8297; Report No. 67752. Unpublished study received Apr 28, 1980 under 3125-318; prepared by Bayer, AG, submitted by Mobay Chemical Corp, Kansas City: Mo; CDL: 099413-F. MRID 32541.
- Mallow S. et al. 1987. CGA 64250: a modified teratology (segment II) study in albino rats. Project No.86189. Syngenta File No. CGA64250/1587. Unpublished study prepared by Ciba-Geigy. MRID 40425002.
- Marty MS, Andrus AK, Stebbins KE. 2011. Myclobutanil: pubertal development and thyroid function in intact juvenile/peripubertal male Crl:CD(SD) rats. Midland (MI): Unpublished study prepared by Toxicology & Environmental Research Consulting of The Dow Chemical Company. MRID 48618109.
- Marty MS, Brooks KJ. 2011. Myclobutanil: uterotrophic assay in the immature female Crl:CD(SD) rat. Midland (MI): Unpublished study prepared by Toxicology & Environmental Research Consulting of The Dow Chemical Company. MRID 48618111.
- Marty MS, Crissman JW, Carney EW. 1999. Evaluation of the EDSTAC female pubertal assay in CD rats using 17beta-estradiol, steroid biosynthesis inhibitors, and a thyroid inhibitor. Toxicol Sci. 52:269–277.
- Marty MS, Marshall VA, Jeong YC, Sura R. 2011. Myclobutanil: Hershberger Assay in Castrated Male Crl:CD(SD) Rats. Midland (MI): Unpublished study prepared by Toxicology & Environmental Research Consulting of The Dow Chemical Company. MRID 48618107.
- Marty MS, Zablotny CL, Stebbins KE. 2011. Myclobutanil: pubertal development and thyroid function in intact juvenile/peripubertal female Crl:CD(SD) rats. Midland (MI): Unpublished study prepared by Toxicology & Environmental Research Consulting of The Dow Chemical Company. MRID 48618108.
- McClain RM. 1995. Mechanistic considerations for the relevance of animal data on thyroid neoplasia to human risk assessment. Mutat Res. 333:131–142.
- McClain RM, Rice JM. 1999. A mechanistic relationship between thyroid follicular cell tumours and hepatocellular neoplasms in rodents. Lyon: IARC Scientific Publications. p. 61–68.
- McLaughlin JE, DiDonato LJ 1984. RH-3866: a three month dietary study in dogs. Unpublished study report prepared by Rohm and Hass Company, a wholly-owned subsidiary of The Dow Chemical Company, Midland, Michigan.
- Mohr U. 1976. Meb 6447: Subchronic Toxicity Study on Rats (Twelve-week Feeding Experiment): Report No. R 840a; Report No. 44300. Unpublished study received Aug 15, 1977 under 3125-318; prepared by Medizinische Hochschule Hannover, Abteilung fur Experimentalle Pathologie, West Germany, submitted by Mobay Chemical Corp, Kansas City, Mo; CDL: 2311312-C.
- Moudgal NR, Shetty G, Selvaraj N, Bhatnagar AS. 1996. Use of a specific aromatase inhibitor for determining whether there is a role for oestrogen in follicle/oocyte maturation, ovulation and preimplantation embryo development. J Reprod Fertil Suppl. 50:69–81.
- Murk AJ, Rijntjes E, Blaauboer BJ, Clewell R, Crofton KM, Dingemans MM, Furlow JD, Kavlock R, Kohrle J, Opitz R, et al. 2013. Mechanism-based testing strategy using in vitro approaches for identification of thyroid hormone disrupting chemicals. Toxicol In Vitro: Int J Published Assoc BIBRA. 27:1320–1346.
- Nagumo K, Teraki Y, Chiba T. 1981. Teratogenicity test of MEB 6447 in pregnant rats: submitter 80257. Unpublished study received Jan 5, 1982 under 1F2474; prepared by St Marianna Univ, First Dept of Anatomy, Laboratory of Embryology, submitted by Mobay Chemical Corp, Kansas City, Mo; CDL:070570-A. MRID 89023.
- Nunez SB, Blye RP, Thomas PM, Reel JR, Barnes KM, Malley JD, Cutler GB Jr. 1996. Recovery of reproductive function in rats treated with the aromatase inhibitor fadrozole. Reprod Toxicol. 10:373–377.
- OECD. 2012. Guidance Document on Standardised Test Guidelines for Evaluating Chemicals for Endocrine Disruption, Environment Directorate, Joint Meeting of the Chemicals Committee and the Working Party on Chemicals, Pesticides and Biotechnology, 524.
- O’Hara G, DiDonato L. 1984. RH-3866: three month dietary toxicity study in rats: Final Report: Report No. 83R-068. Unpublished study prepared by Rohm and Haas Co. 702 p. MRID 00145048.
- Omiecinski CJ, Coslo DM, Chen T, Laurenzana EM, Peffer RC. 2011. Multi-species analyses of direct activators of the constitutive androstane receptor. Toxicol Sci. 123:550–562.
- Ohno K, Araki N, Yanase T, Nawata H, Iida M. 2004. A novel nonradioactive method for measuring aromatase activity using a human ovarian granulosa-like tumor cell line and an estrone ELISA. Toxicological Sci. 82:443–450.
- Paul Friedman K, Watt ED, Hornung MW, Hedge JM, Judson RS, Crofton KM, Houck KA, Simmons SO. 2016. Tiered high-throughput screening approach to identify thyroperoxidase inhibitors within the ToxCast Phase I and II chemical libraries. Toxicol Sci. 151:160–80.
- Paul KB, Hedge JM, Rotroff DM, Hornung MW, Crofton KM, Simmons SO. 2014. Development of a thyroperoxidase inhibition assay for high-throughput screening. Chem Res Toxicol. 27:387–399.
- Paul KB, Thompson JT, Simmons SO, Vanden Heuvel JP, Crofton KM. 2013. Evidence for triclosan-induced activation of human and rodent xenobiotic nuclear receptors. Toxicol In Vitro: Int J Publ Assoc BIBRA. 27:2049–2060.
- Pouliot L, Schneider M, De Cristofaro M, Samadfam R, Smith SY, Beckman DA. 2013. Assessment of a nonsteroidal aromatase inhibitor, letrozole, in juvenile rats. Birth Defects Res Part B: Dev Reprod Toxicol. 98:374–390.
- Price AK, Bridges RS. 2014. The effects of bromocriptine treatment during early pregnancy on postpartum maternal behaviors in rats. Dev Psychobiol. 56:1431–1437.
- Raab D, Youreneff M, Giknis M, et al. 1987. Propiconazole: a teratology study in New Zealand rabbits: final report amendment No. 1: Laboratory Project ID 86043. Unpublished study prepared by Ciba-Geigy. Syngenta File No. CGA64250/1589. MRID 40425004.
- Richardson BP, Turkalji I, Fluckiger E. 1984. Bromocriptine. In: Laurence DR, McLean AEM, Weatherall M, editors. Safety testing of new drugs. London, UK: Academic Press. p. 19–63.
- Richardson VM, Ferguson SS, Sey YM, Devito MJ. 2014. In vitro metabolism of thyroxine by rat and human hepatocytes. Xenobiotica: Fate Foreign Compds. Biol. Syst. 44:391–403.
- Rockett JC, Narotsky MG, Thompson KE, Thillainadarajah I, Blystone CR, Goetz AK, Ren H, Best DS, Murrell RN, Nichols HP, et al. 2006. Effect of conazole fungicides on reproductive development in the female rat. Reprod Toxicol. 22:647–658.
- Rotroff DM, Beam AL, Dix DJ, Farmer A, Freeman KM, Houck KA, Judson RS, Le Cluyse EL, Martin MT, Reif DM, Ferguson SS. 2010. Xenobiotic-metabolizing enzyme and transporter gene expression in primary cultures of human hepatocytes modulated by ToxCast chemicals. J Toxicol Environ Health Part B: Crit Rev. 13:329–346.
- Rotroff DM, Dix DJ, Houck KA, Knudsen TB, Martin MT, McLaurin KW, Reif DM, Crofton KM, Singh AV, Xia M, et al. 2013. Using in vitro high throughput screening assays to identify potential endocrine-disrupting chemicals. Environ Health Perspect. 121:7–14.
- Rotroff DM, Martin MT, Dix DJ, Filer DL, Houck KA, Knudsen TB, Sipes NS, Reif DM, Xia M, Huang R, Judson RS. 2014. Predictive endocrine testing in the 21st century using in vitro assays of estrogen receptor signaling responses. Environ Sci Technol. 48:8706–8716.
- Rouquie D, Tinwell H, Blanck O, Schorsch F, Geter D, Wason S, Bars R. 2014. Thyroid tumor formation in the male mouse induced by fluopyram is mediated by activation of hepatic CAR/PXR nuclear receptors. Regul Toxicol Pharmacol. 70:673–680.
- Sanderson, IT, Boerma J, Lansbergen GWA, van den Berg M. 2002. Induction and Inhibition of aromatase (CYP 19) activity by various classes of pesticides in H295R human adrenocortical carcinoma cells. Toxicol Appl Pharmacol. 182, 44–54.
- Sachsse K, Suter P, Luetkemeier H. 1979a. CGA-64250 Techn. Three months toxicity study on rats: Project No. 790014. Final rept. Unpublished study prepared by Ciba Geigy Ltd., Switzerland, Syngenta File No. 64250/1538. MRID 00058606.
- Sachsse K, Bathe R, Luetkemeier H. 1979b. CGA-64250 3-month toxicity study on dogs: Report No. 78/5751. CDL: 244271-R. Final rept. Unpublished study prepared by Ciba Geigy Ltd., Switzerland. Syngenta File No. CGA64250/1539. MRID 00058607.
- Schraplau A, Schewe B, Neuschafer-Rube F, Ringel S, Neuber C, Kleuser B, Puschel GP. 2015. Enhanced thyroid hormone breakdown in hepatocytes by mutual induction of the constitutive androstane receptor (CAR, NR1I3) and arylhydrocarbon receptor by benzo[a]pyrene and phenobarbital. Toxicology 328:21–28.
- Sheets LP, Gilmore R, Hoss H. 2008. A developmental neurotoxicity study with technical grade triadimefon in wistar rats. Project Number 07/D72/IL, 201766. Unpublished study prepared by Bayer CropScience. p. 1179.
- Shellenberger T, Billups L. 1986. Chronic toxicity and oncogenicity study with RH 3866 in rats: final report: Project No. 8342. Unpublished study prepared by Rohm & Haas Co. Project No. 85RC-61 prepared by Tegeris Laboratories, Inc. 3536 p. MRID 00165247.
- Shimizu Y. 1987. A thirteen week feeding subchronic study of RH-3866 in rats. Report No. NRILS 86-2024;R & H Report No 87RC-038. Unpublished study from NRI Life Science, Kanagawa, Japan and prepared by Rohm & Haas Co. 09 July 1987.
- Stoker TE, Parks LG, Gray LE, Cooper RL. 2000. Endocrine-disrupting chemicals: prepubertal exposures and effects on sexual maturation and thyroid function in the male rat. A focus on the EDSTAC recommendations. Endocrine Disrupter Screening and Testing Advisory Committee. Crit Rev Toxicol. 30:197–252.
- Sueyoshi T, Li L, Wang H, Moore R, Kodavanti PR, Lehmler HJ, Negishi M, Birnbaum LS. 2014. Flame retardant BDE-47 effectively activates nuclear receptor CAR in human primary hepatocytes. Toxicol Sci. 137:292–302.
- Tamada H, Shimizu Y, Inaba T, Kawate N, Sawada T. 2004. The effects of the aromatase inhibitor fadrozole hydrochloride on fetuses and uteri in late pregnant rats. J Endocrinol. 180:337–345.
- Taxvig C, Vinggaard AM, Hass U, Axelstad M, Metzdorff S, Nellemann C. 2008. Endocrine-disrupting properties in vivo of widely used azole fungicides. Int J Androl. 31:170–177.
- Tiboni GM, Marotta F, Castigliego AP, Rossi C. 2009. Impact of estrogen replacement on letrozole-induced embryopathic effects. Hum Reprod. 24:2688–2692.
- Tiboni GM, Marotta F, Rossi C, Giampietro F. 2008. Effects of the aromatase inhibitor letrozole on in utero development in rats. Hum Reprod. 23:1719–1723.
- Tice RR, Austin CP, Kavlock RJ, Bucher JR. 2013. Improving the human hazard characterization of chemicals: a Tox21 update. Environ Health Perspect. 121:756–765.
- Tong Z, Li H, Goljer I, McConnell O, Chandrasekaran A. 2007. In vitro glucuronidation of thyroxine and triiodothyronine by liver microsomes and recombinant human UDP-glucuronosyltransferases. Drug Metab Dispos: Biol Fate Chem. 35:2203–2210.
- Trosken ER, Scholz K, Lutz RW, Volkel W, Zarn JA, Lutz WK. 2004. Comparative assessment of the inhibition of recombinant human CYP19 (aromatase) by azoles used in agriculture and as drugs for humans. Endocr Res. 30:387–394.
- Trosken ER, Adamska M, Arand M, Zarn JA, Patten C, Volkel W, Lutz WK. 2006a. Comparison of lanosterol-14 alpha-demethylase (CYP51) of human and Candida albicans for inhibition by different antifungal azoles. Toxicology 228:24–32.
- Trosken ER, Fischer K, Volkel W, Lutz WK. 2006b. Inhibition of human CYP19 by azoles used as antifungal agents and aromatase inhibitors, using a new LC-MS/MS method for the analysis of estradiol product formation. Toxicology 219:33–40.
- Tully DB, Bao W, Goetz AK, Blystone CR, Ren H, Schmid JE, Strader LF, Wood CR, Best DS, Narotsky MG, et al. 2006. Gene expression profiling in liver and testis of rats to characterize the toxicity of triazole fungicides. Toxicol Appl Pharmacol. 215:260–273.
- Unger T, Van Goethem D, Shellenberger T. 1982. A teratological evaluation of bayleton in mated female rats: MRI Project No. 7272-B. Unpublished study prepared by Midwest Research Institute.MRID 149336:52 p.
- USEPA. 2007a. Integrated Summary Report on Aromatase.
- USEPA. 2007b. Myclobutanil: Acute and Chronic Food and Drinking Water Dietary Exposure and Risk Assessments for Section 3 Use on Snap Bean, Mint, Papaya, Gooseberry, Currant, Caneberry, Bell and Non-Bell Pepper, Head and Leaf Lettuce, and Artichoke PC Code 128857 DP 341690.
- USEPA. 2008. Mycobutanil: Amended Human Health Risk Assessment for Proposed Use on Section 3 Requests for Use on Snap Bean, Mint, Papaya, Gooseberry, Currant, Caneberry, Bell and Non-Bell Pepper, Head and Leaf Lettuce, and Artichoke PC Code 128857 DP Barcode: 341689.
- USEPA. 2009. Triadimefon: Human Health Risk Assessment to Support Reinstatement of Use on Residential Turf Decision No. 391164.38.
- USEPA. 2011. US EPA EDSP21 Work Plan. Available from: https://wwwepagov/endocrine-disruption/endocrine-disruptor-screening-program-21st-century-edsp21-workplan-summary.
- USEPA. 2012. Prioritization of the Endocrine Disruptor Screening Program Universe of Chemicals for an Estrogen Receptor Adverse Outcome Pathway Using Computational Toxicology Tools. Available from: https://wwwregulationsgov/#!documentDetail;D=EPA-HQ-OPP-2012-0818-0017.
- USEPA. 2013. Information Collection Request for the Endocrine Disruptor Screening Program, EPA ICR #2488.01, Attachment F: Calculations for Paperwork Burden and Costs for Data Generation Activities.
- USEPA. 2014a. Endocrine Disruptor Screening Program Comprehensive Management Plan.
- USEPA. 2014b. Integrated Bioactivity and Exposure Ranking: A Computational Approach for the Prioritization and Screening of Chemicals in the Endocrine Disruptor Screening Program. Available from: http://www.regulations.gov/#!documentDetail;D = EPA-HQ-OPP-2014-0614-0003.
- USEPA. 2014c. New High-throughput Methods to Estimate Chemical Exposure.
- USEPA. 2014d. Propiconazole Human Health Risk Assessment for the Section 3 Registration on Rapeseed Crop Subgroup 20A.
- USEPA. 2014e. ToxCast Data Invitrodb_v1.
- USEPA. 2015a. Endocrine Disruptor Screening Program Tier 1 Assessments. Available from: http://www2epagov/ingredients-used-pesticide-products/endocrine-disruptor-screening-program-tier-1-assessments.
- USEPA. 2015b. Use of High-Throughput Assays and Computational Tools; Endocrine Disruptor Screening Program; Notice of Availability and Opportunity for Comment. Available from: http://federalregister.gov/a/2015-15182, Federal Register.
- Vansell NR, Klaassen CD. 2002. Increase in rat liver UDP-glucuronosyltransferase mRNA by microsomal enzyme inducers that enhance thyroid hormone glucuronidation. Drug Metab Dispos: Biol Fate Chem. 30:240–246.
- Vinggaard AM, Hnida C, Breinholt V, Larsen JC. 2000. Screening of selected pesticides for inhibition of CYP19 aromatase activity in vitro. Toxicol in vitro. 14:227–234.
- Walker QD, Mailman RB. 1996. Triadimefon and triadimenol: effects on monoamine uptake and release. Toxicol Appl Pharmacol. 139:227–233.
- Wambaugh JF, Setzer RW, Reif DM, Gangwal S, Mitchell-Blackwood J, Arnot JA, Joliet O, Frame A, Rabinowitz J, Knudsen TB, et al. 2013. High-throughput models for exposure-based chemical prioritization in the ExpoCast project. Environ Sci Technol. 47:8479–8488.
- Wambaugh JF, Wang A, Dionisio KL, Frame A, Egeghy P, Judson R, Setzer RW. 2014. High throughput heuristics for prioritizing human exposure to environmental chemicals. Environ Sci Technol. 48:12760–12767.
- Wetmore BA. 2014. Quantitative in vitro-to-in vivo extrapolation in a high-throughput environment. Toxicology. 332:94–101.
- Wetmore BA, Wambaugh JF, Ferguson SS, Sochaski MA, Rotroff DM, Freeman K, Clewell HJ III, Dix DJ, Andersen ME, Houck KA, et al. 2012. Integration of dosimetry, exposure, and high-throughput screening data in chemical toxicity assessment. Toxicol Sci. 125:157–174.
- Wolfe GW. 1993. RH-3866 technical: 104-week dietary oncogenicity toxicity study in rats. Report No. 89RC-260Unpublished study prepared by Rohm & Haas Co. 522 p. MRID 42809101.
- Willett CE, Bishop PL, Sullivan KM. 2011. Application of an integrated testing strategy to the U.S. EPA endocrine disruptor screening program. Toxicol Sci. 123:15–25.
- Willoughby JA. 2012a. Triadimefon: androgen receptor binding (rat prostate cytosol)-amended report 1. Kalamazoo (MI): Unpublished study prepared by CeeTox, Inc. MRID 48619602:81.
- Willoughby JA. 2012b. Triadimefon: estrogen receptor binding (rat uterine cytosol)-amended report 1. Kalamazoo (MI): Unpublished study prepared by CeeTox, Inc. MRID 48619602:81.
- Willoughby JA. 2012c. Triadimefon: estrogen receptor transcriptional activation (human cell line HeLa-9903)-amended report 1. Kalamazoo (MI): Unpublished study prepared by CeeTox, Inc. MRID 48619605:63.
- Winchell MF, Snyder NJ. 2014. Comparison of simulated pesticide concentrations in surface drinking water with monitoring data: explanations for observed differences and proposals for a new regulatory modeling approach. J Agric Food Chem. 62:348–359.
- Wolf DC, Allen JW, George MH, Hester SD, Sun G, Moore T, Thai SF, Delker D, Winkfield E, Leavitt S, et al. 2006. Toxicity profiles in rats treated with tumorigenic and nontumorigenic triazole conazole fungicides: propiconazole, triadimefon, and myclobutanil. Toxicol Pathol. 34:895–902.