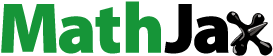
Abstract
Chlorofluorocarbons (CFCs) were introduced in the 1930s as the safe replacements for the toxic and flammable refrigerants being used at that time. Subsequently, hydrochlorofluorocarbons (HCFCs) were also developed. In addition to refrigerant applications, they were used as foam blowing agents, as solvents and as propellants for many aerosols. In the 1970s and 1980s, concern developed about their environmental impact, specifically on stratospheric ozone depletion. Industry began to consider acceptable replacements. In 1987, many of the governments of the world came together and drafted the Montreal Protocol, calling upon Industry to initially phase out production of the CFCs and later HCFCs. Within 4 months of the signing of the Montreal Protocol, the 15 global major producers joined together to form the Alternative Fluorocarbons Environmental Acceptability Study (AFEAS), which sponsored research into environmental effects and the Program for Alternative Fluorocarbons toxicity Testing, PAFT), which examined the toxicology of potential replacements for the CFCs and HCFCs. Nine replacements were identified by companies and, through this international cooperation; toxicology programs were designed, conducted, and evaluated without duplication of effort and testing; consequently these new products were introduced within less than 10 years. Indeed the Montreal Protocol has been recognized as the most appropriate international treaty to phase-down HFCs. In 2016 the Kigali Amendment to the Montreal Protocol set out a phase-down schedule for the consumption and production of HFCs. In order to reduce the consumption and emissions of high GWP HFCs. Recently lower GWP HFCs and very low GWP HFOs (hydrofluoroolefins and HCFOs (hydrochlorofluoroolefins) have been introduced into a range of applications. Summaries of the toxicology profiles of some of the original CFCs and HCFCs, the replacements and the new post-PAFT replacements are described. The chemicals in this review include CFC-11, CFC-12, CFC-113, CFC-114, HCFC 22, HCFC-123, HCFC-124, HCFC-141b, HCFC-142b, HCF-32, HFC-125, HFC-134a, HFC-143a, HFC-152a, HFC-245ea, HFC-245fa, HFO-1234yf, HFO-1234ze, and HCFO-1233zd.
Introduction
In 1974, Molina and Roland published a hypothesis that the UVB radiation from the sun could produce chlorine free radicals from chlorofluorocarbons (CFCs) reaching the stratosphere (Molina and Rowland Citation1974; Rowland Citation1990). These chlorine radicals could then react with ozone molecules in the stratosphere converting them to oxygen molecules. This reaction is described in reaction equation in below (Molina and Rowland Citation1974; NASA Citation1986) using one of the most widely used chlorofluorocarbons at the time (trichlorofluoromethane, CFC-11) as an example. In the stratosphere, ozone plays an important role in blocking most of the sun’s UVB radiation from reaching the surface of the earth and decreases in stratospheric ozone could affect crop growth and could lead to increases in non-melanoma skin cancer and cataracts in humans. During the 1980s measurements showed that there was a marked drop in column ozone levels (Farman et al. Citation1985). As a response to these observations, several nations drafted an agreement calling for the phase-out of the CFCs identified as major contributors to ozone depletion (UNEP/WMO Citation1989). This agreement is known as the Montreal Protocol.
The breakdown of CFC 11 in the presence of oxygen occurs as shown in the reaction equations below in .
Thus, each molecule of CFC 11 can release three atoms of chlorine (SORG Citation1987). These chlorine atoms are catalysts for the destruction of ozone (Hanst Citation1978; Ember Citation1996) as illustrated by the reaction sequence shown in (SORG Citation1987).
The chlorofluorocarbons were first introduced in the 1930s as safe alternatives to the refrigerants then currently in commerce, such as sulfur dioxide, ammonia, carbon tetrachloride, and chloroform. Ammonia is still a widely accepted commercial refrigerant where its use can be carefully controlled. As they were being developed, because of their low toxicity, stability and physical properties other important applications were also identified. In World War II, they were used to produce insecticide aerosols to protect troops in the tropics against malaria and other insect borne diseases. This application lead to their use as aerosol propellants in hair sprays, deodorants, vegetable sprays etc. During the next 40–50 years, the number of applications increased to include use as aerosol propellants, foam blowing, and precision cleaning as well as refrigeration and air conditioning.
These uses resulted in emission of the CFCs into the atmosphere. Because of their low chemical reactivity, they typically have long atmospheric residence times, and as a consequence, they are distributed globally (NASA Citation1986; UNEP/WMO Citation1989; Lashof and Ahuja Citation1990; AFEAS Citation1991; WMO Citation1991a, Citation1991b; AFEAS Citation1992; WMO Citation1999). The environmental properties of several of these products are listed in . Global warming is dependent on the atmospheric lifetime and molecular structure while ozone depletion is dependent on chlorine or bromine content and the amount of released material that gets into the stratosphere. The amount of material getting into the stratosphere will be dependent on the stability of the substance in the air and hence it’s atmospheric life time. However, this is different from atmospheric half-life in that it only considers the total amount that reached the stratosphere and not the residency time in the stratosphere.
Table 1. Boiling Point (BP); Ozone Depleting Potential (ODP); Atmospheric Lifetime in years (AL); Greenhouse Warming Potential (GWP) relative to CO2 (100 yr.) for various chlorofluorocarbons, hydrochlorofluorocarbons and hydrofluorocarbons.
Following the adoption of the Montreal Protocol, four of the world’s largest producers of CFCs, AlliedSignal, Atochem, DuPont and ICI, formed a consortium to develop preliminary programs for the environmental and toxicological evaluation of alternatives to the then current chlorofluorocarbons. The toxicologists from these four companies wrote summaries on the two primary substitutes for these chlorofluorocarbons, 1,1,-dichloro-2,2,2-trifluoroethane (HCFC-123), and 1,1,1,2-tetrafluoroethane (HFC-134a). These summaries were distributed to the other world’s major refrigerant producers. In November 1987, just months after the signing of the Montreal Protocol, the major global producers of fluorocarbons met in New York and formed an international consortium called the Program of the Development of Alternative Fluorocarbon Toxicity Testing (PAFT). The companies that joined the consortium included the four original companies, Allied-Signal Inc., DuPont de Nemours & Co., Elf Atochem SA, and ICI Chemicals and Polymers Ltd. and in addition, Akzo Chemicals International BV, Asahi Glass Co., Ausimont SpA, Central Glass Co., Daikin Industries Ltd., Hankock Shinwha Co., Hoechst AG, Inc., Pennwalt Chemical Co., RTZ Chemical (Rhone-Poulenc Chemicals Ltd.), Showa Denko K. K., and Solvay Fluor and Derivats GmbH. While HFCs (hydrofluorocarbons) are not ozone depleting substances it was recognized that the main HFCs used commercially had high global warming potentials (GWP).
Two types of replacements were considered, hydrofluorocarbons and hydrochlorofluorocarbons (Dekant Citation1996; Trochimowicz and Mullin Citation1973; Rusch Citation1990). Both contain hydrogen which makes them susceptible to attack by hydroxyl radicals in the atmosphere and thus they will have a shorter atmospheric lifetime compared to the chlorofluorocarbons. Hydrofluorocarbons do not contain chlorine or bromine and therefore are not ozone depleting substances. While hydrochlorofluorocarbons do contain chlorine and are ozone depleting substances, because of their shorter atmospheric lifetimes compared to the chlorofluorocarbons, a high percent will break down in the troposphere and will not reach the stratosphere. Therefore, their ozone depleting potential will be low compared to the chlorofluorocarbons.
The PAFT consortium was composed of three committees, the Management Committee, responsible for financing the program and communication of the results to the business areas in the member companies, the Toxicology Committee who were responsible for the development of the toxicology data that would allow for a determination of the safety of these products when used in their intended applications and a Product Standards Committee who were responsible for setting product purity specifications that could be achieved by all producers and would meet the safety standards set by the Toxicology Committee . After the creation of this PAFT consortium, other substances were considered for inclusion in the program. The Management Committee decided to set up product specific committees for each new suggestion. Where two products had similar profiles, they were combined into the same program.
Successful candidates had to have low toxicity as they were replacing the CFCs that were of low toxicity. Thus it was expected that they would have relatively high Occupational Exposure Levels. It was therefore important to limit levels of potentially toxic impurities so that their toxicity would not be a factor in the overall safety of the product. Also, the toxicology data would have to be applicable for all producers and, while different processes would lead to different impurities all had to be within acceptable limits. To achieve this, without members revealing what processes they might be using, all member companies were asked to compile a list of possible impurities and the maximum levels of these impurities that might be found in their finished product. These lists were then given to an independent accounting firm, which made separate compilations of all the impurities that might be present in each product along with the potential maximum level of each impurity. This activity was coordinated by the Product Standards Committee and the list was given to the Toxicology Committee. Each company had some toxicity data on some of these impurities, either test data or read across information. The Toxicology Committee reviewed the lists of potential impurities and the possible levels that might be present. Where the committee judged that the levels did not represent a hazard, they were accepted. Where the committee had a concern regarding a specific level, they would develop a recommendation that would represent a maximum safe level.
As a consequence of their many applications and wide spread use, there have been many reviews of these substances. These include the IPCS’s monograph on the chlorofluorocarbons (IPCS 1990), the IPCS’s review in the partially hydrogenated chlorofluorocarbons (IPCS 1992), the review of the alternate fluorocarbons by Dekant (Citation1996) and the review of fluorocarbons and their replacements (Rusch Citation2002). Finally, an ECETOC Task Force (European Centre for Ecotoxicology and Toxicology of Chemicals) has published a series of reviews in their Joint Assessment of Commodity Chemicals monographs. This series includes monographs on HCFC-21, HCFC-22, HFC-32, HCFC-123, HCFC-124, HFC-125, HCFC-133a, HFC-134a, HCFC-141b, HCFC-142b, HFC-143a, HFC-152a, and HFC-245fa (please see ECETOC references). From these reviews as well as data presented later in this manuscript, it can be seen that most of the chemicals used commercially are not very toxic. Some, in fact, do not show significant signs of toxicity even with exposures at and above 10,000 ppm (1%) (See ).
A generalized toxicology testing program was developed for the candidate substances. The studies in this program represented a general list of studies that would be considered for each substance. These studies were not intended to be run on all substances. Rather, they were to be considered for all substances and selections would be made from the list for each potential new product. Likewise, where other data needs emerged, other studies could be added to that program. The program was reviewed with the Management Committee along with the potential costs. As none of these materials were in commercial production and it was anticipated that toxicology tests would use high exposure levels, it was important to be selective with the choices of tests that were to be run on a given material. Also, data on many of these endpoints existed for some of these chemicals. Where that data was considered valid, it was used. Where there were concerns regarding the purity or the study design, those studies were repeated. The program was considered in 4 phases. In all cases where existing study guidelines were available they were used. First choice was the OECD guidelines (OECD).
The first phase included the short term, genotoxicity and screening studies designed to rapidly identify substances not suitable for further testing. They are listed below:
Acute inhalation toxicity (rat)
Cardiac sensitization (dog)
Ames assay
Chromosome aberration in human lymphocytes
In vivo mouse micronucleus assay
2-week repeat exposure inhalation toxicity screen (rat)
The second phase involved more complex, time consuming and expensive studies. It included:
4-week repeat exposure inhalation toxicity (rat)
Teratology (rat and rabbit)
Preliminary pharmacokinetics (blood levels, uptake and elimination)
Environmental toxicology: Typical studies included toxicity to algae, invertebrate aquatic species (Daphnia magna) and fish (e.g. zebra fish and trout).
The third phase included:
13-week repeat inhalation toxicity (rat)
Metabolism
The fourth and final phase included:
Chronic toxicity/carcinogenicity inhalation study (rat)
Generation inhalation reproduction study (rat)
As these materials were gases or volatile liquids, dermal irritation, dermal sensitization and eye irritation studies were generally not conducted as a part of this program. The Phase 1 studies are designed to provide a basic evaluation of acute toxicity and genotoxicity as well as a basis for repeat exposure studies.
The protocol for the cardiac sensitization study was initially developed in the 1970s by Dr. Charles Reinhardt (Reinhardt, Azar et al. Citation1971; Reinhardt, McLaughlin et al. Citation1971) following reports of deaths resulting from inhaling CFC vapors from aerosol propellants (Bass Citation1970; Linder et al. Citation1974; Russe et al. Citation1980). The commercial products are not highly toxic. Many do not show significant signs of toxicity even with exposures up to 5 or 10% v/v (50,000 or 100,000 ppm). The most typical response seen following over-exposure is CNS depression related to the anesthetic properties of many of these chemicals (Brock et al. Citation2003; ECETOC Citation2009). Inhalation of the vapors of some of the CFCs could produce chemically induced euphoria (Brock et al. Citation2003; ECETOC Citation2009). This was a pre-anesthetic effect associated with the central nervous system (CNS) depression. The products associated with this abuse included fry pan vegetable oil sprays, aerosol deodorants and hairsprays, and glass chiller sprays. The propellants most often involved were blends of CFCs 11 and 12, although non-chlorofluorocarbon substances such as toluene, benzene, and gasoline were also implicated (Bass Citation1970; Linder et al. Citation1974; Russe et al. Citation1980). Some individuals, typically teenagers, would spray substances containing CFC propellants, into a bag and then take several deep breaths of the vapor. Occasionally, one of the individuals would show a short period of excitement and then collapse and die. Autopsy generally was unremarkable. Death was attributed to cardiac arrhythmia resulting from sensitization of the heart to catechol amines (Reinhardt, Azar et al. Citation1971). In all, some 65 deaths were attributed to this CFC “sniffing.” Although rare, a few cases of death have also been reported for people using metered dose inhalants for asthma (Greenberg and Pines Citation1967). In these cases, it could not be determined whether death was due to the CFC propellant or a sympathomimetic agent. It did appear that the inhalant had been over-used. There were also a few reports of the accidental deaths attributed to over-exposure to CFCs in the workplace, most typically CFC 113. The pattern was similar to that reported in the abusive “sniffing” situations. An individual was working in a confined area where large quantities of CFC 113 were present. In such cases, the individual was over-exposed for a fairly short time, collapsed, and developed a rapid heartbeat, which led to cardiac arrhythmia and sometimes death (Stopps et al. 1967; Bass Citation1970).
Experimental protocols have been developed to determine the potency of fluorinated hydrocarbons as cardiac sensitizers. The various protocols that have been developed and the factors that should be taken into account when assessing cardiac sensitization potential have been documented and critically reviewed (ECETOC Citation2009). Several approaches using mice (Taylor and Harris Citation1970; Aviado and Belej 1974), monkeys (Belij et al. 1974), rabbits (White and Carlson Citation1981), and dogs (Reinhardt, McLaughlin et al. Citation1971) have been evaluated. The protocol that involves exposure of dogs to test a compound under conditions designed to simulate stress, while monitoring cardiac function, has provided good comparative information. As mentioned above, this protocol was developed in the late 1960s and early 1970s (Reinhardt, Azar et al. Citation1971; Mullin Citation1969; Mullin et al. Citation1972; Reinhardt et al. Citation1973) and has recently been reviewed (Wagner et al. Citation1996; Brock et al. Citation2003; ECETOC Citation2009). The protocol involves training a group of dogs to calmly accept the procedure for several days prior to the exposure. On the day of the exposure, the dog is placed in a sling and a snout-only exposure mask and ECG leads are attached. After 2 min, the dog is given an injection of epinephrine (adrenaline) of 4–12 μg/kg. The amount given to each dog had previously been individually determined to be just below that necessary to produce a spontaneous arrhythmia. The animal is observed for 5 min. If no arrhythmias are produced, the exposure is initiated. After 5 min of exposure, the dog is given a second injection of epinephrine and the exposure is continued for another 5 min with ECG monitoring for ventricular fibrillations or cardiac arrhythmias. The test is concluded at that point. The dogs are not anesthetized since the anesthesia would inhibit the development of the cardiac arrhythmia. The dogs can be used several times, each exposure being separated by at least 2 days and typically 1 week. The dogs are not sacrificed after the exposure but are returned to the colony. The study should be designed to allow for the determination of a no-observed-effect level (NOEL), a threshold, and the EC50 as median effect concentration. The two most important values are the NOEL and threshold. The level of epinephrine used represents approximately 10 times the level seen in people under stress; this makes the test highly sensitive. In fact, the NOEL determined in this test is well below the minimum exposure level required to induce cardiac effects in humans or animals resulting solely from a combination of stress and exposure (ECETOC Citation2009). This has been demonstrated using CFC 113 as an example. For CFC 113, with injections of epinephrine, the highest NOEL was 2500 ppm and the threshold was 5000 ppm (Reinhardt et al. Citation1973). When 12 dogs were exposed to 2000 ppm for 6 h and then given an injection of epinephrine, only one developed an arrhythmia. When dogs were exposed to concentrations of ≤12,000 ppm and frightened by electric shock or a loud noise, none developed arrhythmia. Likewise, when dogs were exercised on a treadmill and exposed to 20,000 ppm of CFC 113, none developed arrhythmia (Mullin Citation1969). No arrhythmias were seen in monkeys exposed to 50,000 ppm or in mice exposed to 100,000 ppm of CFC 113 without injections of epinephrine or other stressors (Aviado and Smith Citation1975). All of these studies demonstrate the sensitivity of the protocol design in determining the potential for exposure to a chemical to induce cardiac effects. A comparison of the dogs given an injection of epinephrine to those exercising on the treadmill indicates at least an eightfold difference in thresholds. Finally, with HCFC 142 b, in conjunction with epinephrine, arrhythmias were seen in all 12 dogs exposed to 100,000 ppm. Without epinephrine, exposures of 800,000 ppm of HCFC 142 b caused only a marked response in one of the dogs. When the dogs were exposed to 800,000 ppm of HCFC 142 b and startled with a noise, but not given epinephrine, only 42% gave a marked response (Reinhardt, Azar et al. Citation1971). Since cardiac sensitization to adrenalin appears to be the most sensitive response resulting from over exposure to these materials, this test is conducted on all new refrigerant molecules.
The Phase 2 studies extend testing to the guideline 4-week inhalation toxicity study, often with a recovery group, developmental toxicity and environmental toxicity. Since these substances are not very soluble in water and have high vapor pressures, often the environmental tests were omitted. As would be expected for materials that are chemically un-reactive, of limited solubility in aqueous media, and have high vapor pressures, pharmacokinetic measurements in animals and humans show limited uptake and rapid clearance (Meridian Citation1991; Trane 1992; Loizou et al. Citation1994; Vinegar et al. Citation1994; Gunnare et al. Citation2003).
The Phase 3 studies include metabolism and a guideline 13-week inhalation toxicity study. It should be noted that, unless indicated by toxicity seen in prior studies, for all repeat exposure inhalation studies, the highest exposure level selected was 50,000 ppm (5%). This was taken as the upper limit as there was concern that exposures above this level could result in artifacts resulting from anoxia. Although supplemental oxygen was often added during the acute studies, it was not practical to do this during the 6-h repeat whole-body exposure studies.
The final phase of this program included a chronic toxicity/carcinogenicity study in rats and a two-generation inhalation toxicity study also in rats. These studies were not conducted on all prospective new refrigerants. For example, HFC-134a showed very low toxicity in several studies and was not active in a chronic inhalation toxicity study. Concurrent with its development as a propellant for metered dose asthma inhalers, it was decided that a one generation inhalation reproduction study would be adequate. Also since both HCFC-124 and HFC-134a were not active in a chronic inhalation toxicity/carcinogenicity study in rats and HFC-125 was less toxic than HCFC-124 and was not active in any of the genotoxicity studies, it was decided not to conduct a carcinogenicity study with HFC-125.
Eventually, the program grew to include eight chemicals. Some were developed principally for air conditioning and refrigeration such as difluoromethane (HFC-32), 1,1,1,2-tetrafluoroethane (HFC-134a), 1-chloro-1,2,2,2-tetrafluoroethane (HCFC-124), and pentafluoroethane (HFC-125). HFC-134a was also used as the propellant in metered dose asthma inhalers. Two were developed for low pressure air conditioning, foam blowing and as solvents: 1,1-dichloro-2,2,2-trifluoroethane (HFC-123), and 1-chloro-1,1-difluoroethane (HCFC-141b). Two were developed as solvents: 3,3-dichloro-1,1,1,2,2-pentafluoropentane (HCFC-225ca) and 1,3-dichloro-1,1,2,2,3-pentafluoropentane (HCFC-225cb). In addition to the chemicals evaluated under the PAFT program, a few chemicals such as 1-chloro-1,1-difluoroethane (HCFC-142b); 1,1-difluoroethane (HFC-152a) and 1,1,1-triluoroethane (HFC-143a) were independently evaluated. HCF-142b was primarily used as a refrigerant. HFC-152a is being used as an intermediate in the production of fluorinated polymers, as an aerosol propellant in some specialty applications and as a component in refrigeration blends (WEEL Citation1994a). HFC-143a is used as a component in refrigeration blends (ECETOC Citation2006).
The program was completed in 1997. Although the environmental properties of these products represented marked improvements over the chlorofluorocarbons they replaced, some of these products still had high greenhouse warming potentials and those with chlorine were ozone depleting substances, therefore they represent interim solutions. This led to the commercial use of lower GWP HFCs and to the evaluation of a new class of substances: hydrofluoroolefins and hydrochlorofluoroolefins. With the introduction of the double bond into the molecule, these molecules have very short atmospheric lifetimes and only a small percent if any will get into the stratosphere, resulting in very low greenhouse warming potentials and for the hydrochlorofluoroolefins, which contain chlorine, the very short atmospheric lifetimes prevents the substance from reaching the stratosphere. However, some members of this class of chemicals are highly toxic such as perfluoroisobutene (Smith et al. Citation1982) where the 10 min LC50 in rats is 17 ppm and the 2-h LC50 in mice is 1.6 ppm, making selection of acceptable products more difficult. At present, these new substances are being developed primarily as refrigerants, solvents and foam blowing agents. Individual producers are evaluating various chemicals to determine what will best suit their requirements. There are no consortium lead programs and published data is limited.
As the PAFT programs were nearing completion, several members of the Toxicology Committee formed a new committee, under the auspices of the European Fluorocarbon Technical Committee (EFCTC), which continued the review of these products and impurities. The committee reviewed 185 substances that could be products or impurities in products. The goal was to establish recommendations for exposure levels for these substances that could be used to also develop maximum impurity levels for impurities in the final products. Of these 185 chemicals, 29 had existing TLVs, 12 had existing WEELs and 24 had existing internal company exposure levels. For the remaining 120 chemicals, the toxicologists used existing data and read across extrapolations to develop recommendations for maximum exposure levels. Reviews of the toxicity of several substances were published by ECETOC (technical Report 103, 2008). Dr. Rene Millischer, (Millischer et al. Citation2007) developed a procedure for using this information to develop “Maximum Recommended Guidance Levels (MRGLs) for Organohalogenated Impurities Potentially Present in Commercial Fluorocarbons”. This procedure, described below, allows the toxicologist to use the recommended occupational exposure levels for the product and the impurities to determine if the impurity levels are satisfactory for the product (Millischer et al. Citation2007). Initially an occupational exposure level (OEL) must be determined for the product and for each impurity. The OEL is in parts per million Volume (ppmV). Next an uncertainty factor (UF) is determined for each impurity. If the volatility of the impurity and the toxicity of the impurity is similar to the product typically the uncertainty factor would be 10. If the impurity could concentrate (fractionation) during a release or could potentiate the toxicity of the product (i.e. interactive effects), an uncertainty factor of 30 would be appropriate. For a single impurity the calculation for the maximum level allowed for that impurity (where OEL = occupational exposure level and UF = uncertainty factor) is calculated as follows:
Eq 1
Eq 1
For example:
Where multiple impurities are present, first the composite impurity OEL (CIOEL = chemical impurity occupational exposure level) for the impurities is calculated, where X is the decimal proportion of each impurity:
Eq 2
Eq 2
Next the CIOEL in mg/m3 is converted to the CIOEL in ppm where CFn is the conversion factor relating ppm to mg/m3 (24.45/MW) and, 24.45 is the gas law constant:
Eq 3
Eq 3
Alternatively, calculate the weighted molecular weight (weighted MW) for the impurities
Eq 4
Eq 4
Then the conversion from CIOEL in mg/m3 to CIOEL in ppm is:
Eq 3(A)
Eq 3(A)
For example again using HFC-134a:
For CIOEL in ppmV
For the weighted MW:
For the composite Impurity MRGL, substitute the CIOEL and weighted MW into EquationEquation (1)Eq 1
Eq 1
This approach will work whenever there are OELs, Industry OELs or TEOELs (these may be developed by a toxicologist’s review of the toxicology data on the impurities). These calculations enable the producer to assure that the combinations of impurities will still result in a safe product.
Discussion
Results from the testing programs
Chlorofluorocarbons (CFCs) are the original fluorocarbons. The toxicity profiles and physical properties of the most widely used CFCs are presented here for comparison to the replacements.
Trichlorofluoromethane (CAS#75–69-4; Mol.Wt. 137 0.38; CCl3F)
Common synonyms include CFC 11, R-11, fluorotrichloromethane, F-11, and monofluorotrichloromethane. It has a vapor density of 5.86 g/L, its solubility in water is 0.11 g/L and its log Pow is 2.53 (IPCS 1990). It is nonflammable and has a light ethereal odor with poor warning properties.
Production and use
CFC 11 was used mainly for foam blowing, also as a refrigerant and solvent and, with CFC 12, as an aerosol propellant for food, cosmetics, and medical aerosols. Along with CFCs 12 and 113, it was one of the highest production volume chlorofluorocarbons with production levels of over several 100,000 tons/year (IPCS 1990). Traditionally, it was produced by catalytically replacing one of the chlorines on carbon tetrachloride with fluorine from hydrogen fluoride (IPCS 1990). However, under the terms of the Montreal Protocol, it, along with other CFCs, has been phased out (UNEP/WMO Citation1989; SORG Citation1993).
Air exposure assessment
CFC 11 has an atmospheric lifetime of 52 years (SPARC Citation2013). It does not undergo oxidative degradation or attack by atmospheric hydroxyl radicals in the troposphere (Lillian et al. Citation1975; Cox et al. Citation1976; Hanst Citation1978; SPARC Citation2013). It does not photo-dissociate in the troposphere and eventually diffuses into the stratosphere (Hanst Citation1978), where it undergoes photodegradation by the more energetic UV radiation.
Toxicity
Experimental studies have previously been reviewed in great detail (Aviado and Micozzi Citation1981).
Acute Toxicity: The approximate lethal oral dose in rats was reported as 3725 mg/kg (Slater Citation1965). When a 40% solution of CFC 11 in sesame oil was sprayed on the backs of rabbits for 12 exposures, no effect was noted (Scholz Citation1962). When applied to the skin of rats 1–2 times/day, 5 days/week for 5–6 weeks or once/day, 5 days/week for 1 month and to the eye of rabbits, only slight, reversible irritation was noted (Quevauviller et al. Citation1964; Quevauviller Citation1965). Tremor and dyspnea were seen when guinea pigs were exposed to 22,000–25,000 ppm for 2 h. When the exposure levels increased to 45,000–51,000 ppm, deep narcosis was observed (Nuckolls Citation1933). Deep narcosis was also observed at 100,000 ppm for 50 min (Scholz Citation1962). The 30 min LC50 was reported to be 250,000 ppm (Paulet and Chevrier Citation1969). The 15 min LC50 in the rat was reported to be 130,000 ppm (Clark and Tinston Citation1982). In the mouse, the 30 min LC50 was reported to be 100,000 ppm in the rat it was 150,000 ppm for 2 h and 90,000 ppm for 30 min (Scholz Citation1962; 74). The 4 h LC50 in the rabbit was 100,000 ppm (Lester and Greenberg Citation1950), whereas a 60 min exposure at 100,000 ppm was lethal to the cat (Scholz Citation1962).
Cardiac sensitization
The effect of CFC-11 on the heart has been studied extensively (Taylor et al. Citation1971; Taylor and Drew 1974; Aviado and Belej Citation1974; Taylor and Drew 1974; Aviado and Belej Citation1975; Aviado Citation1978; Zakhari and Aviado Citation1982; Lessard and Paulet Citation1986). Some of this work also involved CFC 12 and is described in the next section. As with many CFCs and related substances, CFC 11 can sensitize the heart to the action of adrenaline (Brock et al. Citation2003 and ECETOC Citation2008). This can lead to the development of a potentially fatal cardiac arrhythmia. In a study in which dogs were exposed to CFC 11 for 5 min and then given an injection of epinephrine (adrenaline) to simulate stress with concurrent exposure for 5 additional minutes, no significant effects were seen at exposure levels up to 1000 ppm. One of 12 may have responded at 1000 ppm. The threshold level for reproducible effects was 5000 ppm (Reinhardt, Azar et al. Citation1971; Trochimowicz et al. Citation1976). This effect was not seen when dogs, exercising on a treadmill, received concurrent exposure to levels up to 10,000 ppm (Mullin Citation1969).
Subchronic and chronic toxicity
Paulet et al. (Citation1975) showed only minor changes in cellular oxidation when rats and rabbits were exposed at 50,000 ppm to CFC 11 for 1 h. Scholz (Citation1962) reported that no adverse effects were seen when five rats were exposed to 25,000 ppm, 3.5 h/day, 5 days/week for 4 weeks or when two dogs were exposed to 125,000 ppm for the same time periods. Clayton (Citation1966) reported in one study, that exposure of four rats to 12,000 ppm 4 h/day for 10 days caused pathological changes in the liver. In contrast, he reported that no adverse effects were seen when 2 cats, 12 rats, 2 guinea pigs, and 1 rabbit were exposed to 4000 ppm 6 h/day, 5 days/week for 28 exposures. In a similar study, Jenkins et al. (Citation1970) reported that no adverse effects were seen when groups of 15 rats, 15 guinea pigs, 9 monkeys, and 2 dogs were exposed to either 10,250 ppm 8 h/day, 5 days/week for 6 weeks or 1000 ppm 24 h/day for 90 days. Finally, Leuschner et al. (Citation1983) reported that no adverse effects were seen when 6 dogs were exposed to 5000 ppm or when 40 rats were exposed to 10,000 ppm both 6 h/day for 90 days. The absence of effects in these studies suggests that the single report by Clayton (Citation1966) may be spurious. Maltoni et al. (Citation1988) exposed groups of 90 male and 90 female rats and groups of 60 male and 60 female mice to levels of ≤5000 ppm 4 h/day, 5 days/week for life and did not see evidence of toxicity.
Pharmacokinetics, metabolism, and mechanisms
In one study by Azar et al. (Citation1973), dogs were exposed to 1000 ppm of CFC 11 for 10 min. Blood levels at the end of the exposure were 6.5–10 μg/mL. Clark and Tinston (Citation1972) reported that with exposures of ≤10,000 ppm in dogs for 5 min periods, CFC 11 is more readily absorbed than either CFC 12 or 114, both also components of aerosol propellants. This conclusion was further supported by the work of Adir et al. (Citation1975) and Brugnone et al. (Citation1981) in dogs and rabbits, respectively, which again indicated that CFC 11 is more readily absorbed than CFC 12. These results are not surprising since CFC 11, containing the three atoms of chlorine, is more fat-soluble than CFC 12, which contains two chlorine atoms. Jack (Citation1971) reported that when mice were exposed to CFC 11 for 5 min, the CFC 11 was concentrated to the greatest degree in the adrenals followed by fat and then the heart.
Cox et al. (Citation1972) found no evidence of reductive dehalogenation of CFC 11 in microsomal preparations from rats or chickens. Wolf et al. (Citation1978) reported reductive conversion of CFC 11 to HCFC 21 by rat liver microsomes. CFC 11 was also shown to bind in vitro to liver microsomal protein and lipid (Cox et al. Citation1972, Citation1972a; Uehleke et al. Citation1977; Wolf et al. Citation1977, Citation1978) and to cytochrome P450 (Cox et al. Citation1972, Citation1972a; Wolf et al. Citation1977, Citation1978). Pulmonary uptake by rabbits and dogs was rapid. Peak circulating blood levels reached a maximum within 15 s whereas steady state was reached in 15 min (Paulet et al. Citation1975). McClure (Citation1972) found that for the dog, the initiation elimination half-life was 0.6 ± 0.25 min. Blake and Mergner (Citation1974) exposed dogs for 6–20 min to levels of ≤5000 ppm of radiolabeled CFC 11. They measured the amount in the exhaled air. Virtually all was recovered unchanged within 1 h. Traces of labeled CO2 were found in the expired air and may have represented impurities in the test sample. The authors concluded that <1% of the inhaled dose of CFC 11 was metabolized. The terminal half-life in dogs exposed to levels of ≤75,000 ppm was 80 min (Amin et al. Citation1979). This work suggests minimal metabolism and rapid elimination following inhalation of CFC 11.
Reproductive and developmental
The only study on developmental toxicity of CFC 11 involved exposure of the groups of rats and rabbits to a blend consisting of 10% CFC 11 and 90% of CFC 12. In this study, rats were exposed on gestation days 4–16 and rabbits on gestation days 5–20 for 2 h each day to a level of 200,000 ppm. Rats were sacrificed on day 20 and rabbits on day 30. There was no evidence of embryotoxicity, fetotoxicity, or teratogenicity in either species (Sherman Citation1974).
No information was found on reproductive toxicity of CFC 11. However, given the low level of toxicity seen in the repeat exposure toxicity studies and lack of effects seen in the developmental toxicity study, it is unlikely that exposure to levels at or below current permissible exposure levels (1000 ppm) would result in reproductive effects.
Carcinogenesis
When administered by gavage to groups of 50 male and 50 female mice at daily doses of 1962 or 3952 mg/kg, 5 days/week for 78 weeks followed by a 13 week observation period, there was no evidence of carcinogenicity (NCI Citation1978). Also, when given to rats at daily doses of 488 and 977 mg/kg for males and 538 and 1077 mg/kg for females, again for 5 days/week for 78 weeks, but followed by a 28–33 week observation period, there was no evidence of carcinogenicity (NCI Citation1978). Maltoni et al. (Citation1988) exposed groups of 90 male and 90 female Sprague–Dawley rats and groups of 60 male and 60 female Swiss mice by inhalation to levels of 1000 and 5000 ppm 4 h/day, 5 days/week for life. The exposures did not produce evidence of carcinogenicity.
Genetic and related cellular effects studies
CFC 11 was not active in a series of reverse mutation assays conducted with and without metabolic activation in Salmonella typhimurium strains TA98, TA100, TA1535, TA1537, TA1538, and TA100 (Cox et al.Citation1972; Uehleke et al. Citation1977; Wolf et al. Citation1977). It was also inactive in a forward mutation HGPRT assay conducted with and without metabolic activation in CHO (Chinese hamster ovary) cells (Krahn et al. Citation1982) and in a cell transformation assay with BHK 21 cells (Longstaff et al. Citation1984).
Human experience
In one breath-holding study, it was determined that following inhalation of a single breath of CFC 11, 23% was retained by the body for more than 30 min (Paulet et al. 1969). In a second study (Aviado and Micozzi Citation1981), volunteers were exposed to 1000 ppm for 8 h. Blood levels were 4.69 μg/mL. In a study by Angerer et al. (Citation1985), three volunteers were exposed to a CFC 11 concentration of 657 ppm. CFC 11 levels in alveolar air were 537 ppm and in blood were 2.8 μg/mL, indicating that pulmonary uptake was 18.9%. Following exposure for 150–210 min, elimination was biphasic with half-lives for venous blood of 11 min and 1 h and half-lives for alveolar air of 7 min and 1.8 h, respectively. Brugnone et al. (Citation1981) also reported 19% retention of CFC 11 by workers during occupational exposures.
In studies designed to mimic exposures to CFC propellants from medical atomizers, the initial blood half-life for CFC 11 was in the range of 6–60 s (Paterson et al. Citation1971).
In studies described by Stewart et al. (Citation1978) in which several biological parameters were monitored, single exposures of volunteers to 250 ppm, 500 ppm, and 1000 ppm of CFC 11 for ≤8 h produced no adverse effects. When volunteers were exposed to 1000 ppm for 8 h/day, 5 days/week for 2–4 weeks, there was a statistically significant decrease in cognitive test performance. This was not seen in subjects exposed to the same regime of CFC 12. The authors, however, discounted these findings. Valic et al. (Citation1977) exposed 10 subjects to 2800–26,000 ppm for ≤60 s. They reported changes in ventilatory lung capacity (decrease) and bradycardia. Similar results were obtained with CFC 12, CFC 114, and mixtures of these three propellants. Similar respiratory results were reported by Skuric et al. (Citation1975) when 17 volunteers were exposed to 3300–9500 ppm of sprays of CFC 11. Also, Graff-Lonnevig (Citation1979) studied the effects of inhaling aerosols containing the β2-receptor antagonist fenoterol, or the propellant mixture alone, which contained CFCs 11, 12, and 114 in 18 children with a history of asthma. An untreated control group was included. The levels of exposure were not reported. The exposure to the propellant blend significantly reduced forced expiratory volume (FEV) for 2 h post-exposure compared to the air controls and for 8 h compared to the fenoterol treated group. However, the effect was minimal and overwhelmed by the dilating effect of fenoterol when both were given together.
Van Ketel (Citation1976) reported that patients with an allergic contact eczema from deodorant sprays also tested positive to CFCs 11 and 12.
Workers exposed to high levels of CFC 11, as a result of a spill, reported CNS depression. In one case, the worker lost consciousness; another worker developed tachycardia (Thomas Citation1965). In a study by Marier et al. (Citation1973), a group of housewives were exposed to products containing CFCs 11, 12, and 114 for 4 weeks. No significant adverse effects were seen.
Abusive exposure to both CFCs 11 and 12 has been associated with deaths. Probably the deaths were a result of cardiac arrhythmias induced by elevated levels of catecholamines resulting from the stress associated with the abusive use of the propellant or moderate hypercapnia in combination with the exposure to the propellant (Reinhardt, Azar et al. Citation1971; Bass Citation1970; Fogel Citation1976). Similar reports have described deaths among asthmatics. These deaths also appeared to be related to abusive use of inhalants (Greenberg and Pines Citation1967).
Standards, regulations, or guidelines of exposure
The OSHA permissible exposure limit (PEL) for CFC 11 is 1000 ppm as an 8 h time-weighted average (TWA). The American Conference of Governmental Industrial Hygienists (ACGIH; TLV) has developed a ceiling value of exposure of 1000 ppm. NIOSH recommends 1000 ppm as a ceiling value for exposure to CFC 11 (TLV Citation1991). The Dutch 8 h limit is 500 ppm and the 15 min limit is 1000 ppm (DEC Citation1987).
Studies on environmental impact
Because CFC 11 does not contain hydrogen, it does not undergo breakdown by hydroxyl radicals in the troposphere. This is true for all CFCs and contributes to their long atmospheric lifetimes. As with other CFCs, most CFC 11 released into the troposphere is transported to the stratosphere where it undergoes photolytic degradation, releasing two chlorine atoms while being converted to COClF as described above. It is these chlorine atoms that react with ozone catalytically converting it to oxygen. CFC 11 has been used as the reference compound when comparing ozone-depleting potentials and greenhouse warming potentials. It has been assigned a reference level ODP of 1 and GWP of 1. It has an estimated atmospheric lifetime of 60 years (AFEAS Citation1991). However, the current GWP standard is to compare the halogenated hydrocarbons to CO2 with a defined atmospheric lifetime of 100 years.
Dichlorodifluoromethane (CAS#75–71-8; mol wt 120.92; CCl2F2)
Common synonyms include CFC 12, R-12, Refrigerant 12, Halocarbon 12, Refrigerant R12, Fluorocarbon 12, FC 12. Its vapor density is 4.1, solubility in water is 0.28 g/L and its log Pow is 2.16 (IPCS 1990; TLV Citation1991). It is nonflammable and has a light ethereal odor with poor warning properties.
Production and use
CFC 12 is one of the chemicals that has been phased out of production and use under the terms of the Montreal Protocol. Along with CFCs 11 and 113, it was one of the most important CFCs with production levels up to 400,000 tons (SORG Citation1993). It was used in refrigeration and air conditioning, as a propellant for a variety of aerosols and as a foam-blowing agent (TLV Citation1991).
Air exposure assessment
CFC 12 has been released directly into the atmosphere as a consequence of its use as an aerosol propellant and indirectly through leaks in refrigeration systems and to a much lesser degree by diffusion from foam. In the air, it will eventually diffuse into the stratosphere. It is quite stable and does not undergo photodissociation in the troposphere. In the stratosphere, it can breakdown to give COF2 and two chlorine atoms. It has an average residence time in the atmosphere of 102 years (SPARC Citation2013).
Background Levels
In the water, most CFC 12 would be expected to volatize into the air due to its low water solubility and high vapor pressure. However, 0.28 ng/L has been measured in ocean samples, and a sample of water from Lake Ontario contained 572 ng/L (Kaiser et al. Citation1983).
Acute Toxicity
Dichlorodifluoromethane, was not a skin irritant to rats or rabbits and it did not cause eye irritation when sprayed as a 30/70 blend of CFC 12/11 (Quevauviller et al. Citation1964; Quevauviler 1965; Scholz 1965). Exposures of guinea pigs to levels of ≤900,000 ppm of CFC 12 for 30 min resulted in narcosis, but no deaths (Paulet Citation1969). The threshold for an effect on the CNS in the mouse during a 30 min exposure was 320,000 ppm (Paulet Citation1969). In rats, 30 min exposures of ≤800,000 ppm produced deep narcosis but no deaths (Lester and Greenberg Citation1950), although there was one report (Scholz Citation1962) that deaths occurred in rats but not guinea pigs exposed to 600,000 ppm CFC 12 for 2 h. Even a 6 h exposure at 800,000 ppm did not cause mortality (Lester and Greenberg Citation1950). In a 1930 report, exposure of dogs and monkeys for 7–8 h at 200,000 ppm resulted only in incoordination (Sayers Citation1930).
Cardiac Sensitization
As with many other halocarbons, inhalation of a high level of CFC 12, coupled with an intravenous epinephrine (adrenaline) challenge of 8 μg/kg body weight to stimulate stress, can induce a cardiac sensitization response in dogs. The threshold concentration for this effect was 75,000 ppm (Mullin et al. Citation1972). It was further reported that if the dose of adrenaline was reduced, the concentration of CFC 12 needed to induce an arrhythmia increased; thus, at 3 μg/kg of adrenaline, an exposure level of 100,000 ppm was required whereas at a dose of adrenaline of 2.5 μg/kg an exposure level of 200,000 ppm of CFC 12 was needed (Lessard et al. Citation1977). Clark and Tinston (Citation1972) reported that the EC50 in the dog was 77,000 ppm. In rabbits exposed to 200,000 ppm, a dose of 8 μg/kg of adrenaline was required to induce an arrhythmia.
Inhalation of CFC 12 has been reported to cause bradycardia in mice at 200,000 and 400,000 ppm (Aviado and Belej Citation1974); rats at ≤400,000 ppm (Doherty and Aviado Citation1975; Watanabe and Aviado Citation1975); and possibly in dogs (Flowers and Horan Citation1972). It did not affect the heart rate in monkeys at 50,000 or 100,000 ppm (Belej et al. Citation1974), in rats at ≤50,000 ppm (Friedman et al. Citation1973), or in cats at 250,000 ppm (Harriset Citation1971). It did cause tachycardia in monkeys at 50,000–100,000 ppm (Aviado and Belej Citation1975) and in un-anesthetized rats at 100,000–400,000 ppm (Watanabe and Aviado Citation1975). Hypoxia associated with high concentrations of CFC-12 (950,000 ppm, 95%) was required to produce a fatal arrhythmia in anesthetized dogs. This level resulted in arterial blood levels of CFC 12 of 1475 μg/mL (Flowers et al. Citation1975). In the absence of injected adrenaline or hypoxia, a concentration of 790,000 ppm of CFC 12 was required to cause an arrhythmia in dogs.
Subchronic and chronic toxicity
No adverse effects were seen when rats, cats, guinea pigs, and dogs were exposed to 100,000 ppm of CFC 12, 3.5 h/day, 5 days/week for 4 weeks (Scholz Citation1962). Prendergast et al. (Citation1967) reported fatty infiltration and necrosis of the liver in guinea pigs exposed to 810 ppm continuously for 8 h/day, 5 days/week for 90 days. No effects were seen in rats, rabbits, dogs, or monkeys. No effects were seen when dogs and rats were exposed to 5000–10,000 ppm, respectively, for 6 h/day for 90 days (Leuschner et al. Citation1983).
CFC 12 was given orally to rats at a daily dose level of 160–379 mg/kg and to dogs at doses of 84–95 mg/kg for approximately 12 weeks (Clayton Citation1967). No adverse effects were reported. No effects were seen when 40% solutions of CFC 12 in sesame oil were sprayed on the backs of rabbits 12 times (Scholz Citation1962). Also, groups of 50 male and 50 female rats were given average daily doses of 150 mg/kg or 15 mg/kg in corn oil for 6 weeks then 5 days/week for the balance of 2 years (Sherman Citation1974). No overt signs of toxicity or carcinogenicity were seen except for depression of body weight in the high dose females. Also in this study, groups of four male and four female dogs were given up to 80 mg/kg daily for 2 years. No signs of toxicity were seen. Groups of 90 male and 90 female rats and 60 male and 60 female mice were exposed to 1000 or 5000 ppm of CFC 12 for 4 h/day, 5 days/week (Maltoni et al. Citation1988). There was no evidence of toxicity or carcinogenicity.
Pharmacokinetics, metabolism, and mechanisms
When beagle dogs were exposed to 1000 ppm of CFC 12 for 10 min, 1.1 μg/mL was found in the arterial blood and 0.4 μg/mL in the venous blood (Azar et al. Citation1973). At higher concentrations, the arterial and venous levels were similar. Several studies have shown that CFC 11 is absorbed to a greater extent than CFC 12 in dogs and rabbits. The absorption data correlated well with the liquid/gas partition coefficients in whole blood, serum, and olive oil (Adir et al. Citation1975).
When 14C-labeled CFC 12 was given to rats per os (orally), about 2% was exhaled as 14CO2 and 0.5% was excreted in the urine (Eddy and Griffith Citation1971). CFC 12 and its metabolites were no longer present in the body 30 h after administration. Blake and Mergner (Citation1974) exposed beagle dogs for 6–20 min to 14C-labeled CFC 12 at 8000–12,000 ppm. Virtually all CFC 12 was eliminated within 1 h. In this study, less than 1% of the CFC 12 appeared to be metabolized. When CFC 12 was administered by intravenous infusion, elimination was triphasic. The half-lives for the three-compartment model were 1.47, 7.95, and 58.5 min (Niazi and Chiou Citation1975, Citation1977).
Reproductive and developmental
Sherman (Citation1974) conducted a three-generation oral gavage study in rats using daily doses of 15 or 150 mg/kg of CFC 12 dissolved in corn oil. He found no adverse effects. A developmental toxicity study was conducted by inhalation using a blend of CFC 11 (10%) and CFC 12 (90%). In this study, rats and rabbits were exposed to a level of 200,000 ppm of the blend on gestation days 4–16 (rats) or 5–20 (rabbits). There was no evidence for developmental, fetal, or teratogenic effects. Sherman (Citation1974) gave pregnant rats either 16.6 or 179 mg/kg of CFC 12 daily in corn oil on gestation days 6–15. Neither dose was embryotoxic or teratogenic.
Carcinogenesis
There was no evidence of carcinogenicity when groups of 50 male and 50 female rats were given oral doses of 15 or 150 mg/kg of CFC 12 daily for 2 years (Sherman Citation1974). As described above, there was no evidence of carcinogenicity when groups of 90 male and 90 female rats and of 60 male and 60 female mice were exposed by inhalation to levels of 1000 and 5000 ppm, 4 h/day, 5 days/week for 2 years (Maltoni et al. Citation1988).
Genetic and related cellular effects studies
CFC 12 was not mutagenic in a reverse mutation assay using S. typhimurium strain TA100 and TA1535 either with or without metabolic activation (Longstaff et al. Citation1984). It was inactive in the forward mutation HGPRT assay with CHO cells (Krahn et al. Citation1982) and in the cell transformation assay with BHK 21 cells ((Longstaff et al. Citation1984). It did not cause dominant lethal mutations in rats (Longstaff et al. Citation1984).
Human experience
Mergner et al. (Citation1975) confirmed that CFC 12 is poorly metabolized, less than 1%, by humans. Single exposures to 250 ppm, 500 and 1000 ppm for 1 min to 8 h caused no observable effects (Stewart et al. Citation1978). Kehoe (Citation1943) exposed one subject to 40,000 ppm for 80 min and to 110,000 ppm for 11 min. At 40,000 ppm, the volunteer experienced a tingling sensation, humming in his ears, and apprehension. EEG changes were noted, as well as slurred speech and decreased performance in psychomotor tests. These signs became more pronounced with increasing concentration. At 110,000 ppm, a significant degree of cardiac arrhythmia was seen, followed by CNS depression and amnesia. At 10,000 ppm for 150 min, a 7% decrease in psychomotor performance was seen (Azar et al. Citation1972). No effects were seen at 1000 ppm.
Valic et al. (Citation1977, Citation1982) exposed 10 volunteers to 300–3000 ppm for periods of 15–60 s. They reported significant acute reductions in ventilatory lung capacity and irregular heartbeat. In a subsequent study, 11 subjects were exposed to a variety of fluorocarbons, including CFC 12. The exposure lasted 130 min and included 92, 10,000, and 17,500 ppm. An acute reduction of ventilatory lung capacity and decrease in pulse was reported at the two highest levels. When one considers the length of the exposures and levels in the second study, the effects reported in the first clearly did not progress to more serious responses.
In a study of 89 refrigeration workers who were given 10 min exposures to a variety of halocarbon refrigerants, including CFC 12, exposures were measured, and some exceeded 750 ppm. Cardiac monitoring did not reveal any pattern of arrhythmias that would suggest a treatment-related problem (Edling and Olson Citation1988). Szmidt et al. (Citation1981) investigated death rates among 539 workers exposed occupationally to a variety of halocarbons, including CFC 12. There was no increase in total deaths, tumor deaths, or cardiovascular deaths.
In a study of six refrigeration workers who were exposed to levels of CFC 12 and HCFC 22 that occasionally reached 1300–10,000 ppm, no cardiac problems were noted. These workers were compared to a similar group of plumbers who had no exposure (Antti-Poika et al. Citation1990). Deaths from exposure to CFCs 11 and 12 have been associated with the abusive inhalation of aerosols (Fogel Citation1976). Bass (Citation1970) concluded that these deaths may have been caused by cardiac arrhythmia.
Accidental deaths have also been reported. In one case, a 4 year old boy discharged an aerosol can containing 50.5% CFC 11 and 43% CFC 12 while in the bathtub. He died 3 days later (Jefferson Citation1978).
Standards, regulations, or guidelines of exposure
The OSHA permissible exposure limit (PEL), the NIOSH REL and the TLV for CFC 12 is 1000 ppm as an 8 h TWA (TLV Citation1991). The Dutch occupational exposure limit for 8 h is also 1000 ppm; the 15 min exposure limit is 2000 ppm (DEC Citation1987).
1,1,2-Trichloro-1,2,2-Trifluoroethane (CAS# 76–13-1; Mol. Wt. 187.38; C2Cl3F3)
Common synonyms include CFC 113, R-113, trichlorotrifluoroethane, Fluorocarbon 113, Halocarbon 113, Refrigerant 113, CFC 113. It has a vapor density of 7.3 g/L and its solubility in water is 1.011 g/L and its log Pow is 3.30 (IPCS 1990; TLV Citation1991). It is nonflammable and has a light ethereal odor with poor warning properties.
Production and use
Along with CFCs 11 and 12, CFC 113 was produced in large quantities from the early 1940s until the present. Its primary use has been in precision cleaning of electronic parts. It has also been used as a solvent in other more general applications, and as a propellant for very specific applications. Production peaked at approximately 260,000 metric tons/year (SORG Citation1993). It is one of the ozone-depleting substances being phased out of production under the terms of the Montreal Protocol (SORG Citation1993).
Most production processes involved fluorination of carbon tetrachloride with hydrogen fluoride in the presence of an antimony pentafluoride catalyst.
Exposure assessment
CFC 113 is a stable chemical with low water solubility and high vapor pressure. It has an atmospheric lifetime of 90 years and is transported to the stratosphere, where it undergoes photolytic breakdown to release chlorine atoms. As a consequence, it is globally distributed and conveyed to the stratosphere, where it can undergo photolytic decomposition to release chlorine atoms (UNEP/WMO Citation1989).
Environmental levels of CFC 113 in the troposphere have been reported: in the Northern Hemisphere 145.9 ng/m3 (19 ppt) and in the Southern Hemisphere 138.1 ng/m3 (18 ppt) (Singh et al. Citation1979). Airborne levels tend to be higher in the Northern Hemisphere contrasted to the Southern Hemisphere with a ratio of 1.10:1.25 (Singh et al. Citation1983). Levels also tend to be higher in the populated areas. As CFC 113 is phased out of use, these levels should tend to decrease and become more uniform.
Acute toxicity
The oral LD50 for CFC 113 was reported to be 43,000 mg/kg (Clayton Citation1966). It may also be capable of inducing skin sensitization in the guinea pig (Horiguchi Citation1985). Several acute inhalation studies have been reported by Trochimowicz (Citation1984); all indicated that CFC 113 is of low inhalation toxicity. In the guinea pig, the 1 h LC50 was 120,000 ppm; in the mouse, the 2 h LC50 was 90,000 ppm; in the rabbit, the 2 h LC50 was 59,500 ppm; whereas in the rat, the 2 h LC50 was 52,500 ppm. Desoille and Truffert (Citation1968) also reported that the 2 h LC50 in the mouse was 90,000–95,000 ppm.
Cardiac sensitization
The potential for CFC 113 to induce cardiac sensitization has been studied in greater detail than most other CFCs. In the standard evaluation protocol, where dogs are exposed for 5 min and then given an injection of epinephrine (adrenaline) to simulate stress with concurrent exposure to CFC 113 for an additional 5 min, the no-observed-effect level was 2500 ppm and the threshold for inducing arrhythmias was 5000 ppm (Reinhardt et al. Citation1973). When the exposures were conducted over a 6 h time period at 2000 ppm, still only 1 of 12 dogs showed a response . Also, when other stimuli such as noise or electric shock were substituted for the injection of adrenaline, no effects were seen with exposure levels of ≤12,000 and ≤20,000 ppm, respectively (Mullin Citation1969). When no external stimulation was applied, no arrhythmias were seen in monkeys with exposures of ≤50,000 ppm or mice with exposures of ≤100,000 ppm.
Subchronic and chronic toxicity
Trochimowicz (Citation1988) described a 2 year inhalation study in which groups of 100 male and 100 female rats were exposed to levels of CFC 113 of ≤20,000 ppm 6 h/day, 5 days/week for 2 years. The only signs of toxicity were decreases in mean body weight and body weight gain in the females exposed to 10,000 and 20,000 ppm and the males exposed to 20,000 ppm. Desoille and Truffert (Citation1968) reported on a limited study with groups of six rats and an unspecified number of rabbits. These animals were exposed to a single level of 12,000 ppm or air. No significant signs of toxicity were reported.
Trochimowicz (Citation1984) described several subchronic studies with CFC 113. In one study, rats, mice, dogs, and monkeys were exposed to a level of 2000 ppm 24 h/day for 14 days. No significant signs of toxicity were noted. In other studies, rats and guinea pigs received twenty 3.5 h exposures at 12,500 ppm; and rats, dogs, and guinea pigs received twenty 6 h exposures to 5100 ppm; and rats received exposures up to 20,000 ppm, 6 h/day, 5 days/week for 90 days, all without adverse effects. Carter (Citation1970) reported that monkeys had enlarged thyroids and rats had increased kidney weights, but mice and dogs showed no effects when exposed to 2000 ppm 24 h/day for 14 days. Clayton (Citation1966) reported that exposure of rats to 2075–2850 ppm, 7 h/day for 30 exposures produced no adverse effects, although the rate of body weight gain was reduced and some liver discoloration was noted. Lastly, Leuschner et al. (Citation1983) reported that no adverse effects were seen when dogs were exposed to 5000 ppm or rats were exposed to 10,000 ppm of CFC 113 6 h/day for 90 days.
Although severe skin irritation was reported when 5 g/kg of CFC 113 was applied to the backs of rabbits daily for 5 days (Waritz Citation1971), only mild irritation was seen following one application of 11 g/kg (Clayton Citation1966). When a pad saturated in CFC 113 was applied to the backs of hairless mice for 5 min twice a day for ≤40 days, only mild irritation was seen (McKnight and McGraw Citation1983). This finding suggests that CFC 113 can penetrate the skin, although to only a small degree. Also, since chronic inhalation did not cause liver effects, the possibility that this may be an indirect response should not be discounted.
Pharmacokinetics, metabolism, and mechanisms
Radiolabeled CFC 113 was inhaled by a group of volunteers, 19.8% was retained for >30 min (Paulet and Chevrier Citation1969; Morgan et al. Citation1972). Trochimowicz et al. (Citation1974) found that after inhalation by dogs of 1000 ppm for 1 min, the venous blood levels were 1.9 μg/mL, whereas arterial levels were 2.7 μg/mL. Carter (Citation1970) reported that on inhalation of CFC 113 by rats, the majority of the material was found in the fat. It was further reported (Furuya Citation1979) that on inhalation, tissue distribution was highest in the fat, followed by brain, liver, kidney, heart, lung, muscle, and blood, in that order. Reinhardt, McLaughlin et al. (Citation1971) reported that when volunteers were exposed to either 500 or 1000 ppm of CFC 113 for 3 h in the morning and again for 3 h in the afternoon, 4 subjects from the 500 ppm exposure level still had detectable levels present the next day, whereas 14 people exposed to 1000 ppm had detectable levels on the next day.
Reproductive and developmental
There were no adverse reproductive effects in a study in which male and female rats were exposed to levels of 5000 or 12,500 ppm 6 h/day, 5 days/week for either 10 weeks (males) or 3 weeks (females) and then bred for 2 weeks. Exposures during the mating period were 6 h/day, 7 days/week for the females, and daily exposures were continued through gestation. Pups were followed for 4 weeks after parturition (Kelly Citation1978).
No effects were seen in a rat developmental toxicity study where groups of 24 pregnant animals were exposed to levels of ≤25,000 ppm, 6 h/day on gestation days 6–15. At the higher level, there was some evidence of slight maternal toxicity. Two studies with rabbits were described. In the first, groups of 12 rabbits were exposed to level of ≤20,000 ppm of CFC 113 2 h/day on gestation days 8–16. In the second, groups of eight rabbits were given doses of 1000 or 5000 mg/kg of CFC 113 by oral gavage. No effects were seen in the first rabbit study. In the second study, again no developmental effects were seen. However, both control and treated animals had low pregnancy rates and fetal deaths were seen in control and test groups (Trochimowicz et al. Citation1974).
Carcinogenesis
A 2 year inhalation toxicity/carcinogenicity study was conducted by Trochimowicz (Citation1988) in which groups of 100 male and 100 female rats were exposed to levels of up to of ≤20,000 ppm of CFC 113 6 h/day, 5 days/week for 2 years. Although five nasal tumors were seen, one at 20,000 ppm and four at 10,000 ppm, all five were different morphological types and were judged not to be exposure related. In addition, there was a small increase (5.8%) in pancreatic islet cell adenomas in the females exposed to 20,000 ppm; however, based on historical controls, this was within the normal control range.
In a second study, injection of 0.1 ml of 10% CFC 113 was not carcinogenic. But when given with a 5% solution of piperonyl butoxide, hepatomas were induced in male mice (Epstein et al. Citation1967). The significance of this experimental finding has never been determined.
Genetic and related cellular effects studies
An in vitro reverse mutation assay was conducted using S. typhimurium strains TA100 and TA1535 both with and without S9 metabolic activation. CFC 113 was not active in this assay (Longstaff et al. Citation1984). An in vivo dominant lethal assay was conducted using i.p. injections of 200 and 1000 mg/kg (Epstein et al. Citation1972). CFC 113 was not active in this assay.
Human experience
Stopps and McLaughlin (Citation1967) exposed volunteers to levels of 1500, 2500, 3500, and 4500 ppm for 2 h, 45 min. The subjects were asked to perform certain psychomotor tests. No effects were seen at 1500 ppm. Starting at 2500 ppm, there was a dose-related decrease in performance, as well as an increase in “heaviness” in the head, drowsiness, and loss of orientation—all indications of slight CNS depression. Reinhardt, McLaughlin et al. (Citation1971) exposed volunteers to levels of 500 or 1000 ppm for 3 h periods, morning and afternoon, for 5 days. No decreases in psychomotor ability were seen.
No cardiac effects were seen when groups of workers exposed to average levels of 442 ppm were compared to workers exposed to average levels of 64 ppm (Egeland et al. Citation1992). No adverse effects were noted in a population of workers exposed to average levels of 700 ppm with peaks to 4780 ppm (Imbus and Adkins Citation1972; NIOSH Citation1980). However, deaths of two workers have been reported when individuals have been overexposed to CFC 113, especially in “confined spaces” (May and Blotzer Citation1984). In one case, the exposure level was estimated to be 128,000 ppm; in the other, it was estimated to have been in the range of 6000–37,000 ppm for 15 min. The deaths appear to be the result of either asphyxiation or cardiac arrhythmia. In one case of an industrial exposure, CFC 113 was used as a cleanser for a washer tub filter. A large quantity was left in the tub, creating an atmosphere of 120,000–140,000 ppm in the room. Death was attributed to inhalation of this vapor. In another case, CFC 113 was used to clean the inside of a military tank. The material was not drained from the tank. When an employee entered the tank to drive it to a parking area, he was overcome by the vapors, estimated to be between 5000 and 20,000 ppm, and developed a fatal cardiac arrhythmia (Kaufman et al. Citation1994).
CFC 113 was used as the propellant in an aircraft window rain repellant “RainBoe.” There is one report of a leak in the system releasing the contents of the RainBoe canister into the cockpit. The plane ran off the runway because someone in the cockpit increased the power as they were landing. The pilots speech was slow and without emotion just prior to the crash. Fortunately, there were no deaths or serious injuries (Voge Citation1997).
Although there was one report of a reversible neuropathy in a laundry worker exposed to CFC 113 with tetrachloroethane (Raffi and Violante Citation1981), the absence of additional reports from other workers in other fields suggests that this may not have been due solely to CFC 113, if at all. Another nonfatal exposure occurred when an anesthetized patient was accidentally given 1 L of CFC 113 in his stomach. During the next 3 days, he experienced severe rectal irritation but otherwise recovered (Clayton Citation1966).
In a study of 16 workers exposed to levels below 1000 ppm, no cardiac effects were seen (Egeland et al. Citation1992). In a study with exposure levels of 260–1000 ppm for 4 h, Woollen et al. (Citation1990) demonstrated that the elimination of CFC 113 from humans is a three-compartment model with average half-lives of elimination of 0.22, 2.3, and 29 h. Because of the solubility of CFC 113 in blood, uptake was low. The ratio of retained CFC to that found in expired air suggests that some metabolism does occur. The rate is, however, so low that it could not be concluded with certainty that CFC 113 is metabolized by man (Auton and Woollen Citation1991).
Standards, regulations, or guidelines of exposure
The OSHA PEL for CFC 113 is 1000 ppm as an 8 h TWA. The NIOSH REL is also 1000 ppm with a STEL of 1250 ppm. Many governments have adopted similar values (TLV Citation1991). The Dutch occupational exposure limit for 8 h is 150 ppm; for 15 min it is 300 ppm (DEC Citation1987).
As noted previously, CFC 113 is one of the substances being phased out of production and use under the terms of the Montreal Protocol, since it is considered to be an ozone-depleting material. Production in the developed countries has been halted and will soon be halted in the developing countries (SORG Citation1993).
Studies on environmental impact
CFC 113 has an ozone-depleting potential of 0.9–0.8 (WMO Citation1999). Because CFC 113 does not contain hydrogen, it does not undergo breakdown by hydroxyl radicals in the troposphere. This is true for all CFCs and contributes to their long atmospheric lifetimes. Most CFC 113 released into the atmosphere is transported to the stratosphere, where it undergoes photolytic degradation, releasing chlorine atoms. It is these chlorine atoms that react with environmental ozone converting it to oxygen (AFEAS Citation1991).
1,2-Dichloro-1,1,2,2-Tetrafluoroethane (CAS#76–14-2; Mol.Wt. 170.92; C2Cl2F4)
Common synonyms include CFC 114, R-114, Refrigerant 114, Freon 114, and Halocarbon 114. Its vapor density is 5.9; solubility in water is 0.01% (0.10 g/L) and log Pow is 2.82. It is nonflammable and is a nearly odorless gas with poor warning properties. (IPCS 1990)
Production and use
CFC 114 has been used as an aerosol propellant, refrigerant, solvent, fire extinguisher, blowing agent, and dielectric fluid. It has been classified as an ozone-depleting substance and is being phased out of production and use (AFEAS Citation1991).
Background levels
CFC 114 is a chlorofluorocarbon with an atmospheric lifetime of189 years. As a consequence, it is found distributed globally in the air in both the troposphere and stratosphere (SPARC Citation2013).
Biomonitoring/biomarkers
It is unlikely that CFC 114 will show passive bioaccumulation as it shows rapid clearance from the body (Trochimowicz et al. Citation1974) under experimental conditions.
Acute toxicity
CFC 114 has a low acute toxicity. The 30 min LC50s for mice, rats, and rabbits were reported to be 700,000, 720,000, and 750,000 ppm, respectively. Oxygen was added to maintain oxygen level at 20% (Paulet and Desbrousses Citation1969). CNS depression, but not lethality, was observed when rats and guinea pigs were exposed for 2 h to levels of 300,000–400,000 ppm (Scholz Citation1962). Some CNS depression was also reported in guinea pigs during a 2 h exposure to 8,000 to 47,000 ppm (Paulet Citation1969). Yant et al. (Citation1932) reported that dogs survived single 8 h exposures at 200,000 ppm; however, a single 16 h exposure or three or four 8 h exposures caused death. Signs of CNS depression such as tremors, convulsions, and incoordination were seen.
Cardiac sensitization
As with many other CFCs, exposure to a high level of CFC 114 coupled with an intravenous injection of epinephrine (adrenaline) to stimulate stress can induce a cardiac sensitization response in dogs. The threshold concentration for this effect was 25,000 ppm, and marked responses were seen at 50,000 ppm (Reinhardt, Azar et al. Citation1971). Following a similar protocol, Clark and Tinston (Citation1972) reported the median effective concentration (EC50) causing cardiac arrhythmias to be 100,000 ppm. Trochimowicz et al. (Citation1974) reported that an arterial blood level of 13.8 μg/mL and a venous blood level of 7.2 μg/mL were associated with the development of arrhythmias.
Subchronic and chronic toxicity
Repeated application of a 40% solution of CFC 114 in sesame-seed oil to the back of a rabbit did not cause irritation (Scholz Citation1962). However, repeated spraying on skin and conjunctiva caused some irritation to these tissues. The eye did not show signs of injury (Quevauviller et al. Citation1964; Quevauviller Citation1965).
Yant et al. (Citation1932) reported that dogs survived twenty-one 8 h exposures at levels of 142,000–150,000 ppm of CFC 114 with only minor blood changes and limited signs of CNS depression. Also Paulet and Desbrousses (Citation1969) reported that an exposure of rats to a level of 200,000 ppm of CFC 114, 2–5 h/day, 5 days/week for 2 weeks resulted in decreased body weight gain and pulmonary and hematological effects. The NOEL in this study was 100,000 ppm. Likewise, exposure of dogs, cats, guinea pigs, and rats to 100,000 ppm, 3.5 h/day, 5 days/week for 4 weeks did not cause any adverse effects (Scholz Citation1962) Finally, exposure of rats and rabbits to a level of 10,000 ppm for 2 h/day, 5 days/week for 8–9 months did not cause any adverse effects (Desoille and Truffert 1968).
Pharmacokinetics, metabolism, and mechanisms
Animal studies demonstrated that uptake in the dog exposed to 25,000 ppm of CFC 114 is rapid, with blood level reading a maximum within approximately 5 min. Elimination appeared to be a two-step process, initially rapid followed by a more prolonged decline (Trochimowicz et al. Citation1974). Human studies likewise showed a rapid elimination of CFC 114 (Paulet and Chevrier Citation1969; Morgan et al. Citation1972).
Carcinogenesis
The only chronic study conducted with CFC 114 was in rats and rabbits for a period of 9 months. This would not be adequate for an assessment of carcinogenicity (Desoille Citation1973).
Genetic and related cellular effects studies
CFC 114 was not active in a reverse mutation assay in S. typhimurium strain 1535 when tested both with and without metabolic activation (IPCS 1990).
Human experience
Ten subjects were exposed to CFC 114 at levels of 2300 and 21,400 ppm for 15, 45, and 60 s. Acute reduction in ventilatory lung capacity, bradycardia, irregular heartbeat, and atrioventricular block were reported (Valic et al. Citation1977).
Standards, regulations, or guidelines of exposure
OSHA established an 8 h PEL TWA of 1000 ppm. The NIOSH REL is 1000 ppm (TLV Citation1991). The Dutch limits for CFC 114 are 1000 ppm as an 8 h TWA and 2000 as a 15 min TWA (DEC Citation1987). Germany MAK Commission limit is 1000 ppm 8 h TWA and 2000 ppm 60 min TWA (DFG Citation1989).
Studies on environmental impact
CFC 114 has an atmospheric lifetime of 200 years. As such, it is distributed on a global basis (SORG Citation1993). Its ozone-depleting potential is 0.85–1.
Hydrochlorofluorocarbons were occasionally used concurrently with the CFCs and were some of the initial replacements. They had applications as low pressure refrigerants, foam blowing agents and solvents. Because they contain chlorine they are still ozone depleting substances, however, the presence of hydrogen in the molecule makes them susceptible to attack by the hydroxylradicals in the air and thus they have much shorter atmospheric lifetimes and compared to CFCs, only small amounts are transported to the stratosphere.
Chlorodifluoromethane (CAS# 75–45-6; Mol. Wt. 86.47; CClF2H)
Common synonyms include HCFC 22, R-22, Chlorofluorocarbon 22, and difluorochloromethane. It has a vapor density of 3.87g/L; its solubility in water is 0.28 g/L and its log Pow is 1.08. It is nonflammable and has a light ethereal odor with poor warning properties (ECETOC Citation1991).
Production and use
HCFC 22 has been in production since the mid-1940s. It is used primarily as a chemical intermediate and as a refrigerant in residential, commercial, and mobile air-conditioning units. An azeotropic mixture of HCFC 22 and CFC 115 (48.8:51.2 wt%) has been used as a refrigerant in food display cases, icemakers, home freezers, and heat pumps (ECETOC Citation1991).
Acute Toxicity
In the dog, a 90 min exposure at 690,000 ppm caused lethality but a 90 min exposure at 400,000 ppm did not (Van Poznak and Artusio Citation1960). In the rat, a 2 min exposure at 600,000 ppm was not lethal. However, 2 h exposures to an atmosphere of 400,000 ppm (40%) HCFC 22 caused death in both rats and guinea pigs. Exposure to 300,000 ppm for 2 h was the minimum lethal concentration in the rat and did not cause death in the guinea pig. Exposure to 200,000 ppm did not cause death in rats (von Weigand Citation1971). The LC50 for a 4 h exposure in rats was 220,000 ppm (Litchfeild et al. 1963). Whereas 2 h exposures of mice to 360,000 was the minimum lethal concentration. Exposures at 315,000 ppm did not cause any mortality (Karpov Citation1963a). However, in another series of studies, Sakata et al. (Citation1981) reported that the 30 min LC50 in rabbits was 300,000 ppm and for mice the 30 min LC50 was 175,000 ppm. Aviado and Smith (Citation1975) also reported that a 5 min exposure to 200,000 ppm was not lethal in monkeys. Typically, signs of CNS depression such as tremors, convulsions, narcosis, and shallow respiration were reported.
Although HCFC 22 has been reported as causing skin irritation, these effects were probably caused by freezing of tissues rather than toxicity of the substance (Quevauviller et al. Citation1964; Atochem Citation1986). It is also considered slightly irritating to the eyes when sprayed as a gas onto the eyes for 5–30 s (Atochem Citation1986). It did not cause dermal sensitization in the guinea pig in a minimization test (Atochem Citation1986).
Cardiac sensitization
As with many other hydrohalocarbons, inhalation of a high level of HCFC 22, coupled with an intravenous injection of epinephrine (adrenaline) to simulate stress, can induce a cardiac sensitization response in dogs. The threshold concentration for this effect was 50,000 ppm, and the NOEL was 25,000 ppm (Reinhardt, Azar et al. Citation1971) The EC50 in dogs was reported to be 140,000 ppm (Clark and Tinston Citation1982).
In another experiment, Pantaleoni and Luzi (Citation1975) exposed rats to concentrations of ≤600,000 ppm without injections of adrenaline. Although some changes were seen in cardiac function, no arrhythmias were produced. A similar observation was reported by Belej et al. (Citation1974) in studies where monkeys were exposed to levels of ≤200,000 ppm.
Subchronic and chronic toxicity
Few clinical signs and no histopathological effects were seen when rats were exposed to 10,000 ppm 6 h/day for 63 days (Karpov Citation1963b). Neither were any adverse effects seen when rats, guinea pigs, dogs, and cats were exposed to 50,000 ppm 3.5 h/day, 5 days/week for 4 weeks (von Weigand Citation1971) nor when rats were exposed to 50,000 ppm 5 h/day for 8 weeks (Lee and Suzuki Citation1981). Leuschner et al. (Citation1983) reported that no effects were seen when dogs exposed to 5000 ppm or rats to 10,000 ppm 6 h/day, 5 days/week for 13 weeks.
Groups of rats, mice, and rabbits were exposed to 14,000 ppm of HCFC 22 while 80 rats and mice were also exposed to 2000 ppm 6 h/day, 6 days/week over a 10 month period. At 14,000 ppm, depressed body weight gain was reported for the mice and other effects were reported for various species on hematology, liver, lungs, and nervous tissue. No effects were reported at 2000 ppm (Karpov Citation1963a). None of the effects seen in rats or mice have been subsequently reported in studies conducted at higher levels for longer periods of time.
Tinston et al. (Citation1981a) conducted a study in which 80 male and 80 female mice were exposed to levels of ≤50,000 ppm of HCFC 22 5 h/day, 5 days/week for 83 weeks (females) or 94 weeks (males). Other than hyperactivity seen in the males at 50,000 ppm, no adverse effects were reported at any level.
In a similar study (Tinston et al. Citation1981b), rats were exposed to levels of ≤50,000 ppm for either 118 weeks (females) or 131 weeks (males). The only signs of toxicity seen were a transient decrease in body weight gain for males at 50,000 ppm and increased liver, kidney, adrenal, and pituitary weights in females at the same exposure level. The clear NOEL was 10,000 ppm.
Pharmacokinetics, metabolism, and mechanisms
Carney (1977) determined blood levels in cannulated, anesthetized rats following exposure to 10,000 or 50,000 ppm of HCFC 22 to be 31 and 155 mg/L, respectively. He also reported that following exposure, the clearance was rapid, with a half-life of approximately 3 min. In a subsequent study with rabbits (Sakata et al. Citation1981), blood levels of 148 mg/L of HCFC 22 were measured following exposure at 50,000 ppm, and at an exposure level of 200,000 ppm the blood level was 583 mg/L. It took approximately 5 min to reach a steady state, and the clearance half-life was 1 min. Woollen (Citation1988) using pregnant rats exposed to 50,000 ppm, reported that blood levels reached 118 mg/L after 30 min and remained constant throughout the remainder of a 6 h exposure. Sakata et al. (Citation1981) measured tissue distribution in rabbits following inhalation of 50,000 ppm of HCFC 22. Except for fat, he found fairly uniform distribution regardless of length of exposure (7–92 min). The levels of HCFC 22 in fat rose slowly in relation to exposure time. In studies with 14C-labeled material (Salmon et al. Citation1979), only 0.1% of the HCFC 22 was metabolized to CO2 following exposure to 500 ppm, and at 10,000 ppm only 0.06% was metabolized to CO2 (Salmon et al. Citation1985). Subsequently, Peter et al. (Citation1986) reported that HCFC 22 was not metabolized to any significant degree after either i.p. or inhalation exposure.
Reproductive and developmental
A male reproduction study was conducted in which one group of 16 males was exposed to air and a second to 50,000 ppm HCFC 22 5 h/day for 8 weeks (Lee and Suzuki Citation1981). Following the last exposure, each male was housed with a virgin female for 7 days. This regime was followed for 10 weeks. Overall, there was no effect on fertility of the male rats and no evidence for a dominant lethal effect.
A series of teratology studies were conducted with rats (Palmer Citation1978a, Citation1978b). Initially, in three separate studies, groups of 20–40 Sprague–Dawley rats were exposed to levels of 100–50,000 ppm of HCFC 22, 6 h/day from either gestation days 4–13 or gestation days 6–15. The only abnormality reported was a low incidence (typically a single fetus) in one or two litters with anophthalmia or microphthalmia. There was no evidence of maternal or overt fetal toxicity. As this is a rare finding, a study was developed to assess this in greater detail. This study involved exposure of multiple groups of pregnant rats to exposure levels of 0, 100, 1000, or 50,000 ppm 6 h/day on gestation days 6–15, yielding a very large number of litters. Again a very low level of anophthalmia (15.678/1000 litters) and microphthalmia (10.44/1000 litters) was reported in the high level exposure groups. When compared with controls (1.65 or 3.29/1000 litters, respectively), only the increased incidence of anophthalmia was statistically significant. As this finding has been reported in more than one study with rats, it would appear that it may be related to exposure to HCFC 22; however, the low incidence at the high exposure levels suggests that it is a very weak response.
A teratology study was also conducted in the rabbit with exposure levels of ≤50,000 ppm and exposures on days 8–18 of pregnancy. Maternal body weight was lower at 50,000, but not 1000 ppm. There were no treatment related effects on the pups (Palmer Citation1978b), including a lack of eye defects.
Carcinogenesis
In one study, an oral dose of 300 mg of HCFC 22/kg of body weight was given to 36 male and 36 female rats 5 days/week for 52 weeks. The rats were held for 125 weeks and compared to controls. There was no evidence of any treatment related increase in tumors (Longstaff et al. Citation1984). Maltoni et al. (Citation1982, Citation1988) conducted inhalation studies with 60 male and 60 female rats exposed to levels of 1000 and 5000 ppm of HCFC 22. The exposures were 4 h/day, 5 days/week for 104 weeks (rats) or 78 weeks (mice). There was no evidence for a carcinogenic effect.
In another inhalation study (Litchfield and Longstaff Citation1984), rats were exposed to 0, 1000, 10,000, or 50,000 ppm 5 h/day, 5 days/week for 118 weeks (females) or 131 weeks (males). At 50,000 ppm there was an increase in fibrosarcomas (18/80 compared with 5/80) in the male rats, but not females. No increases were seen at the lower levels. In a concurrent study, 80 male and 80 female mice were exposed to the same levels of HCFC 22 for 83 weeks (males) or 94 weeks (females). There were no increases in tumors in any treatment group (Tinston et al. Citation1981a). Given that only the male rats in one study showed the increase in fibrosarcomas, and that this is not a common response to chemicals of this class, the significance of these finding is uncertain.
Genetic and related cellular effects studies
In Vitro
The mutagenic potential of HCFC 22 was evaluated in several reverse mutation assays S. typhimurium strains TA90, TA100, TA1535, and TA1538 both with and without activation (Butterworth Citation1976; Krahn Citation1977; Longstaff and McGregor Citation1978; Bartsch et al. Citation1980; Russell et al. Citation1980; Litchfield and Longstaff et al. Citation1984). Although occasionally a positive response was seen with strains TA1535 and TA100, overall HCFC 22 did not appear to be active. When it was evaluated in cultures with Schizosaccharomyces pombe and S. cerevisiae, again with and without metabolic activation, it was not active. It was also not active in the Chinese hamster HGPRT locus assay with CHO cells or V-79 cells. It also did not induce unscheduled DNA synthesis in human EVE cells. (McCooey Citation1980) A study of chromosome aberrations was conducted using bone marrow samples from rats exposed to levels of ≤1000 ppm of HCFC 22 for 6 h/day for 5 days. Although there was an increase in the number of the cells with chromosome abnormalities at the lowest exposure level (10 ppm). There was an inverse dose response leading to the conclusion that HCFC 22 was inactive in this assay (ECETOC Citation1991).
In Vivo
HCFC 22 given at a dose of 816 mg/kg in corn oil was not active in a mouse micronucleus assay. It also did not induce dominant lethal effects in mice when given by inhalation at levels of ≤50,000 ppm (Van Poznak and Artusio Citation1960).
Other: Neurological, pulmonary, skin sensitization
HCFC 22 caused a slight elevation in pulmonary resistance in cannulated monkeys at an exposure level of 400,000 ppm but not at 200,000 ppm (Aviado, and Belej Citation1975).
Human experience
Simulated use studies have been conducted to assess the potential human exposure to HCFC 22 when it is used as an aerosol propellant. It was determined that exposures were generally lower than the recommended occupational exposure limit of 1000 ppm (Hartop and Adams Citation1989).
The uptake and elimination were studied in two groups of three male volunteers who were exposed to 100 or 5000 ppm for 4 h. During exposure, blood levels reached 0.25 and 1.36 μg/mL, respectively. The blood/air partition coefficient was measured to be 0.77, which compared well with calculated value of 0.79. Metabolism, as estimated from urinary fluoride levels, was minimal. Elevated levels of HCFC 22 were found in two individuals who had died from inhalation of HCFC 22 during an accident on a fishing vessel (Morita et al. Citation1977).
No cardiac abnormalities were found in a study of six refrigeration repairmen who occasionally were exposed to levels of ≤1300–10,000 ppm of either CFC 12 or HCFC 22 (Antti-Poika et al. Citation1990).
Woollen et al. (Citation1992) reported on elimination in volunteers exposed for 4 h to levels of 92 or 520 ppm. In the post-exposure periods, the elimination was triphasic with half-lives of 0.005, 0.2, and 2.6 h. It did not appear to be metabolized to a significant extent.
Standards, regulations, or guidelines of exposure
The Dutch Expert Committee for Occupational Standards (DEC Citation1987) established a health-based exposure limit of 1000 ppm as an 8 h TWA for HCFC 22. The German MAK Commission has established a MAK value of 500 ppm also as an 8 h TWA (Henschler Citation1987). The NIOSH REL is 1000 ppm (3500 mg/m3; STEL 1250 ppm, 4375 mg/m3) (TLV Citation1991f).
Studies on environmental impact
The atmospheric lifetime is 13 years The global warming potential is estimated to be approximately 10% of that calculated for CFC 11. It has a global warming potential of 1760 relative to a reference value of 1.0 for carbon dioxide at a 100 year integrated time horizon (Myhre et al. 2013; SPARC Citation2013) and an ozone-depleting potential of 0.05 relative to a reference value of 1.0 for CFC 11 (WMO Citation1999). It has been found to be globally distributed in the atmosphere with measured levels of up to 65 parts per trillion (v/v) (Kahlil and Rasmussen 1981).
1,1-Dichloro-2,2,2-Trifluoroethane (CAS# 306–83-2; Mol Wt 152.93, C2HCl2F3)
Common synonyms include HCFC 123, R-123, FC 123, and dichlorotrifluoroethane, It has a vapor density of 5.3; its solubility in water is 3.9 g/L; and its log Pow is 2.0–2.8. It is nonflammable and has a light ethereal odor with poor warning properties (ECETOC Citation1996, Citation2005).
Production and use
HCFC 123 is an interim replacement for the CFCs and is scheduled for a production phase-out during the 2020s (SORG Citation1993). Its main application is as a substitute for CFC 11 in centrifugal chillers used for air conditioning of large buildings. It is also used in smaller air-conditioning units, and may have applications in foam blowing, firefighting and as a chemical intermediate.
Acute toxicity
In dermal studies, 2000 mg/kg was applied to the back of both rats and rabbits. Mild irritation was seen, but no clinical signs of toxicity . Likewise, instillation of 0.1 ml of HCFC 123 into the conjunctival sac of the eye resulted in only mild to moderate, reversible irritation. The potential for HCFC 123 to cause dermal sensitization was evaluated in guinea pigs using 10% and 50% solutions of HCFC 123 in propylene glycol. HCFC 123 did not produce evidence of skin sensitization (Brock Citation1988a; Trochimowicz Citation1989). The approximate lethal dose following oral dosing was 9000 mg/kg body weight (ECETOC Citation2005).
The 4 h median lethal (LC50) exposure concentration in Chinese hamsters was reported as 28,400 ppm (Darr Citation1981). Surviving animals were anesthesized during exposures at ≥20,000 ppm, but recovered within approximately 15 min on cessation of the exposure. All animals died at 31,000 ppm, while none died at 26,000 ppm. In an earlier study (Hall Citation1975), the 4 h LC50 in Sprague–Dawley rats was reported to be 32,000 ppm. Similar signs of CNS depression were reported, starting at 20,600 ppm. The surviving rats recovered within 30 min on cessation of the exposure. A 30 min LC50 in mice was reported to be 74,000 ppm. The 30 min approximate median exposure level for anesthesia was 23,700 ppm (Raventos and Lemon Citation1965). A series of 4 h exposures were conducted with guinea pigs at levels of 1000–30,000 ppm. All exposure groups showed signs of hepatotoxicity such as centrilobular vacuola (fatty) change, multifocal random degeneration, and necrosis (Marit et al. Citation1994). Exposure of rats to levels of 1000–10,000 ppm for 15 min. resulted in reversible CNS depression at 5000 and 10,000 ppm. Recovery was complete within 15–20 min. The highest NOEL for CNS depression was 2500 ppm (Trochimowicz Citation1989).
Cardiac sensitization
As with other halocarbons, inhalation of high concentrations of HCFC 123, followed by an intravenous adrenaline (epinephrine) challenge to simulate stress, can induce a cardiac sensitization response in dogs. In one study (Foll Citation1976), it was reported that exposures to 10,000 ppm were sufficient to produce cardiac sensitization. However, no experimental details were provided. In a second study, with exposure levels of 10,000, 20,000, and 40,000 ppm, no effects were seen at 10,000 ppm, four of six responded at 20,000 ppm, and all three dogs responded at 40,000 ppm. In this study, the EC50 was 19,000 ppm and the NOEL was 10,000 ppm (Trochimowicz and Mullin Citation1973).
Subchronic and chronic toxicity
A 2 year inhalation toxicity/oncogenicity study was conducted using groups of 80 male and 80 female rats. Exposures were to levels of 0, 300, 1000, and 5000 ppm of HCFC 123, 6 h/day, 5 days/week. Serum triglyceride, cholesterol, and glucose concentrations were significantly decreased in both sexes. There was an exposure related pattern of decreased body weight gain, increased liver and kidney weights, and mild peroxisome induction. There were also increases in some benign tumors discussed in the section on carcinogenicity. Survival at 2 years improved with increasing exposure level, and survival in the high exposure females (59%) was significantly better than controls (23%). Histopathological examination revealed acinar cell hyperplasia of the pancreas at 1000 and 5000 ppm, basophilic cellular changes in the liver in all HCFC 123 exposure groups, Leydig cell hyperplasia in the testes in all male groups, and diffuse retinal atrophy at all exposure levels (Malley et al. Citation1995).
Five subchronic studies have been conducted with HCFC 123. Groups of rats were exposed to levels of ≤10,000 ppm of HCFC 123 6 h/day, 5 days/week for 2 weeks or levels of ≤20,000 ppm 6 h/day, 5 days/week for up to 13 weeks. Other than slight CNS depression, no effects were seen in the 2 week study (Kelly and Trochimowicz Citation1976). The other four studies were reported by Rusch et al. (Citation1994). In the 4 week study, although there were no histopathological changes, increases in liver weight and elevations in serum transaminases were reported along with some decreases in body weight gain and changes in other clinical parameters. Three 90 day inhalation toxicity studies were also conducted. All involved exposure 6 h/day, 5 days/week for 13 weeks. The first involved exposures of both rats (10 per level) and dogs (4 per level) to vapors of HCFC 123 at levels of ≤10,000 ppm. Some CNS depression was seen in both species at 10,000 ppm. Only minimal signs of toxicity were seen in the rats. In the dogs, there was clear histopathological evidence for liver damage; also, elevations were seen in alkaline phosphatase, ALT, and AST. No compound- related effects were seen at 1000 ppm, the lower exposure level. In the second study, groups of 35 male and 25 female rats were exposed to levels of ≤5000 ppm. Only minimal signs of toxicity were noted at 5000 ppm. In the third study, a modern GLP (good laboratory practice)-compliant study, it was reported that exposure to 5000 ppm causes mild CNS depression; depression of serum glucose, triglyceride, and cholesterol levels; and mild peroxisome induction. No adverse histopathological effects were seen. A level of 1000 ppm represented a NOEL of all three 13 week studies (Rusch et al. Citation1994).
Warheit (Citation1993) studied the inhalation toxicity of HCFC 123 in the guinea pig and the rat. The initial high exposure level was targeted for 20,000 ppm. Because of severe body weight losses in the 15 HCFC 123–exposed guinea pigs, exposure was reduced to 5000 ppm. Three guinea pigs died, presumably from the initial exposures. Alterations were seen in serum chemistry parameters, and clearly the guinea pig was more sensitive than the rat.
Pharmacokinetics, metabolism, and mechanisms
The partition coefficients of HCFC 123 in various tissues have been determined by a number of investigators (Dekant Citation1984; Loizou et al. Citation1994; Vinegar et al. Citation1994) utilizing the head space analysis method of Gargas et al. (Citation1989). The results of these studies indicate that HCFC 123 was less lipophilic than halothane (2-bromo-2-chloro-1,1,1-trifluoroethane, HCFC 123B1), the bromine analog. Because of its lipophilic characteristics, however, absorption would be expected to occur readily. The partition coefficients for HCFC 123 are shown in . Male and female rats were exposed for 6 h to HCFC 123 at ≤5000 ppm. A PBPK (physiologically based pharmacokinetic) model was used to describe the uptake, distribution, and metabolism (Dekant Citation1984; Loizou et al. Citation1994). The kinetic constants obtained from this modeling are shown in . Subsequent work with other fluorocarbons has shown similar results. The poorly perfused fatty tissue more readily adsorbs these materials.
Table 2. Partition Coefficients of HCFC-123 in Rats.
The uptake of HCFC 123 was best described by a single saturable component model, although the model did not accurately predict the uptake in female rats at HCFC 123 concentrations of >2000 ppm. Indeed, a transition from first order to zero uptake appears to occur at about 2000 ppm, where first-order kinetics describe the uptake at lower concentrations. This concentration-limiting process may be due to a perfusion-limiting process in tissues, such as liver, or by suppression of metabolism (Vingar et al. 1994).
In another study by Dekant (Citation1984), male Sprague–Dawley rats and male Hartley guinea pigs were exposed by inhalation to 14C-labeled dichlorotrifluoroethane for 6 h at a concentration of 2000 ppm. This investigator measured the disappearance of HCFC 123 from the inhalation chamber and found that approximately 50–60% of the radiolabeled material was lost from the chamber of exposed rats, whereas about 95% of the applied dose was lost from the chamber of exposed guinea pigs. Only 20–30% of the applied radiolabel was recovered in these studies, and in the absence of a mass balance the loss of the remaining radiolabel is unknown but assumed to be absorbed.
In separate studies, Dodd et al. (Citation1993) and Vinegar et al. (Citation1994) exposed rats to HCFC 123 concentrations of 100–10,000 ppm for 2–6 h. They reported that the uptake of HCFC 123 was biphasic with a rapid initial uptake over 30–45 min followed by a slower absorption phase. Both groups of investigators also indicated that uptake was saturable at concentrations of >2000 ppm, a finding consistent with Dekant (Citation1984). As the concentration approached 2000 ppm there appeared to be a suppression of the production of the primary metabolite, trifluoroacetic acid (TFA). Concurrent with the uptake of HCFC 123, TFA blood concentrations also rose during exposure (Vingar et al., 1994). Following a 4 h exposure to 2000 ppm, the HCFC 123 and TFA blood concentrations were 15 and 93 mg/L, respectively. However, by the end of the 4 h exposure to a level of 2000 ppm, the HCFC 123 and TFA blood concentrations were about 94 and 38 mg/L, respectively. These data suggest that at 2000 ppm suppression of the oxidative metabolic pathway occurs, apparently because of substrate inhibition and not killing of the metabolic enzyme. Indeed, <24 h later, the TFA blood concentrations rebounded and approached 100 mg/L at the exposure concentration of 2000 ppm.
Urban et al. (Citation1994) examined the metabolism of HCFC 123 in rat and human liver microsomes. In both, the primary metabolite was trifluoroacetic acid, with small amounts of chlorodifluoroacetic acid, inorganic fluoride, and an unidentified minor metabolite. In human cells, the microsomal oxidation was about 10 times higher than in rat cells. It appeared that the oxidative metabolism utilized cytochrome P450 2E1. HCFC 123 is primarily eliminated unchanged in the expired air (90%). The major metabolite, TFA, is eliminated in the urine. The blood elimination half-life for the HCFC 123 was estimated to be 2–4 h (Dodd et al. Citation1993).
Under anaerobic conditions, Godin et al. (Citation1993) reported that rat liver microsomes metabolize HCFC 123 to HCFC 133a (1,2-dichloro-1,2,2-trifluoroethane). When oxygen levels were above 5%, no HCFC 133a was detected. Ferrara et al. (Ferrara Citation1995, Citation1997) provided further evidence for anaerobic reduction metabolism of HCFC 123 to reactive intermediates that can react with glutathione. Huwyler et al. (Citation1992, 1992a) found that metabolism by rat kidney was far slower than by rat liver. Harris et al. (Citation1992) reported that the reactive metabolite of HCFC 123 was capable of binding with liver microsomes. This finding is similar to that reported for halothane. It has been speculated that this could be the mechanism for halothane-induced hepatitis (Pohl et al. Citation1988). Huwyler and Gut (Citation1992) and Huwyler et al. (Citation1992a) reported that HCFC 123 metabolites can also bind to kidney and heart tissue, although to a much lesser extent (5% and 0.5%, respectively).
Reproductive and developmental
An inhalation teratology study was conducted in which groups of 30 pregnant rats were exposed to air or 5000 ppm of HCFC 123 6 h/day on gestation days 6–15 (Rusch et al. Citation1994). In a second study, groups of 25 pregnant rats were exposed to air or 10,000 ppm of HCFC 123 also for 6 h/day on gestation days 6–15 (Kelly Citation1978). There was no evidence of embryotoxicity, fetotoxicity, or teratogenicity in either study. In a third study (Malinverno et al. Citation1996), groups of 24 pregnant rabbits were exposed to ≤5000 ppm of HCFC 123 6 h/day during gestation days 6–8. Again, there was no evidence of embryotoxicity, fetotoxicity or teratogenicity.
A one-generation inhalation reproduction study was conducted using groups of 12 rats. Exposures in this study ranged from 0 to 5000 ppm 6 h/day, 7 days/week for a total of 17 weeks starting 4 weeks prior to mating. Litter size, survival, and pregnancy rate were all comparable. Pup body weights and body weight gain in treatment groups were retarded in a dose-related manner. Following this study, a two-generation study was conducted. In this study, groups of 32 male and 32 female rats in the F0 generation, and their offspring that remained on test, were exposed to levels of 0, 30, 100, 300, and 1000 ppm of HCFC 123 6 h/day, 7 days/week (Malinverno et al. Citation1996). Increased liver weights and centrilobular hepatocyte enlargement and vacuolation were observed in the F0 and F1 animals exposed to ≥100 ppm. At 30 ppm, only a slight liver weight increase was reported. Although birth weights tended to be comparable across all groups, pups in the higher exposure level group had reduced body weight gains during nursing at ≥100 ppm. After weaning, body weights were comparable.
A follow-up study was designed to determine if the decreased body weight gain was related to exposure to HCFC 123 during gestation, an effect on nutrient levels in the milk or exposure of the mothers to HCFC 123 during nursing (Buschmann Citation1996). When pregnant animals were exposed to HCFC 123 during gestation, but not while nursing, pup body weight gain was comparable to air-exposed controls. When the mothers were exposed to HCFC 123 during nursing, pup body weight gain was retarded. Analysis of the milk for fat, protein, and glucose showed no exposure-related effects. However, trifluoroacetic acid, the primary metabolite of HCFC 123, was found in both the milk and the pups’ urine. The reduction in body weight gain seen in the two-generation reproduction study described above was judged to be related to direct exposure to HCFC 123 and/or trifluoroacetic acid and not to reproductive effect.
To evaluate the relevance of the rat developmental effect to primates, groups of 4 lactating Rhesus monkeys and their infants were exposed for 6 h/day, 7 days/w for 3 weeks to air or 1000 ppm of HCFC 123. In addition to normal evaluations including liver biopsy, the effect of exposure on milk quality (fat and protein content) was evaluated and the levels of HCFC 123 and TFA were determined. While levels of HCFC 123 and TFA were found in the milk and TFA was found in the infants, there was no effect on body weight of the infants (Cappon et al. Citation2002).
Carcinogenesis
An inhalation toxicity/oncogenicity study was conducted with groups of 80 male and 80 female rats. This study was described earlier in this section. In addition to the effects on serum chemistry parameters and the retina, increases in benign tumors of the pancreas, testes, and liver were reported (Malley et al. Citation1995). As noted above, the survival in the HCFC 123–exposed animals was significantly (p = 0.05) better than in the air-exposed controls. These tumors tended to appear near the end of the study and generally were not considered to be the cause of death.
Genetic and related cellular effects studies
In Vitro
HCFC 123 was not active in several reverse mutation assays with S. typhimurium strains TA98, TA100, TA1535, TA1537, and TA1538 run both with and without metabolic activation (Brusick Citation1976; Longstaff et al. Citation1984; Callander Citation1989). It was also inactive in a cell transformation assay with BHK 21 cells (Longstaff et al. Citation1984). In a chromosome aberration study with human lymphocytes, HCFC 123 was positive in the presence of S9 metabolic activation and inactive without metabolic activation (Dance Citation1991; Edwards Citation1991; MacKay Citation1992).
In Vivo
HCFC 123 was negative when tested for chromosome aberration in rat lymphocytes at 5000 ppm and unscheduled DNS synthesis at 20,000 ppm with rat hepatocytes (Marshall Citation1992; Kennelly Citation1993). Additionally, it was negative in a mouse micronucleus assay with exposure levels of approximately 18,000 ppm (Muller and Hofmann Citation1988).
Other: Neurological, pulmonary, skin sensitization
Neurotoxicity
Groups of 10 male and 10 female rats were exposed to ≤5000 ppm 6 h/day, 5 days/week for 13 weeks. Animals were periodically evaluated, and at the end of the study, the brain and several nerve tissues were evaluated. The only abnormality seen was a decrease in arousal time at the 13 week interval in male rats. This may have been a spurious or residual effect from the anesthetic effects of HCFC 123. There was no evidence of neurotoxicity in this study (Coombs Citation1984).
Impact on reproductive hormones
Sandow (1996) studied serum levels of several steroids as well as testicular levels of luteinizing hormone (LH) and testosterone, under both normal and stimulated conditions, in male rats exposed to ≤1000 ppm of HCFC 123, 6 h/day, 7 days/week for 22 weeks. Compared to controls, no effects were seen on serum steroid levels or on basal levels of LH or testosterone. With stimulation, the levels of LH in both the 300 and 1000 ppm exposure level groups were lower than controls. Moreover, a slight reduction in testosterone was noted in all HCFC 123 exposure level groups. Likewise, Warheit (Citation1993) measured serum hormone levels in rats and guinea pigs exposed to 18,200 ppm or approximately 9000 ppm, respectively. No significant differences were seen in the rats. In the guinea pigs, there may have been a slight reduction in testosterone levels compared to control levels. Although guinea pigs did not show evidence of peroxisome induction, the hepatotoxicity was more severe than seen in the rat.
Peroxisome proliferation
In the same study, Warheit (Citation1993) reported that exposure to HCFC 123 or its metabolite trifluoroacetic acid resulted in peroxisome proliferation in the rats but not in the guinea pigs. A similar response was seen in the rats in the chronic exposure study (1993).
Human experience
HCFC 123 has been used effectively and safely in applications where exposures have been controlled to <10 ppm, such as in centrifugal chillers (Meridian Citation1991; The Train Co., 1992). In other situations, when exposures have been at much higher levels, reversible hepatotoxicity including a marked increase in serum amino acid transaminases (serum glutamic oxaloacetic transaminase and serum glutamic pyruvic acid transaminase) have been reported (Hoet et al. Citation1997; Takebayashi et al. Citation1998; Boucher et al. Citation2003). In all cases, removal from the exposure resulted in return of serum enzymatic activity to normal levels.
In response to concerns that occupational exposure to hydrofluorocarbons and hydrochlorofluorocarbons, including HCFC 123, could result in autoimmune responses to cytochrome P450 2E1 (CYP2E1), a group of 44 men occupationally exposed to halogenated hydrocarbons and a group of 35 men not exposed to these materials were screened for antibodies against CYP2E1. No increase in autoantibodies against CYP2E1 was detected in the exposed workers compared to the unexposed controls (Gunnare et al. Citation2007).
Standards, regulations, or guidelines of exposure
The American Industrial Hygiene Association’s Workplace Environmental Exposure Level (WEEL) Committee recommends 50 ppm as an 8 h TWA PEL (WEEL Citation1998). The AIHA’s ERP committee has developed emergency guideline levels for exposures lasting up to 1 h (ERPG Citation2017).
1-Chloro-1,2,2,2-Tetrafluoroethane (CAS# 2837–89-0; Mol Wt 136.5; C2HClF4)
Some common synonyms include HCFC 124, R-124, 2-chloro-1,1,1,2-tetrafluoroethane, and hydrochlorofluorocarbon 124. It has a vapor density of 4.7; its solubility in water is 1.45 g/L; and its log Pow is 1.9–2.0. It is nonflammable and has a slight ethereal odor with poor warning properties (ECETOC Citation1994a).
Production and use
HCFC 124 has recently been developed as a replacement for fully halogenated chlorofluorocarbons. Its primary applications are as a refrigerant and foam-blowing agent.
Acute toxicity
Groups of male rats were exposed for 4 h, nose-only, to atmospheres containing HCFC 124 at ≤300,000 ppm. At 162,000 and 230,000 ppm, rats showed signs of CNS depression. The rats showed rapid recovery at the end of these exposure periods. At 300,000 ppm all rats died during exposure. The 4 h LC50 was therefore, between 230,000 and 300,000 ppm (Kelly Citation1990). In an earlier study (Wada Citation1977), all mice exposed for 10 min to 400,000 ppm survived, but all mice exposed to 150,000 ppm showed signs of anesthesia. The NOEL was 100,000 ppm. The authors also reported a 10 min approximate lethal concentration of 440,000 ppm. Likewise, Coate (Citation1976) reported that single 6 h exposures of rats to ≤360,000 ppm was not lethal but rapidly depressed motor activity and induced anesthesia. The rats in this exposure group also had slower post-exposure body weight gain compared to controls.
Finally, when dogs were exposed for 10 min at levels of 400,000–700,000 ppm, light surgical anesthesia was rapidly induced. Complete recovery occurred within 7–10 min post-exposure (Van Poznak and Artusio Citation1960).
Cardiac sensitization
The potential for HCFC 124 to sensitize the heart to epinephrine (adrenaline) was investigated with beagle dogs (Mullin Citation1976). Inhalation of levels of 26,000 ppm and higher for 5 min followed by injection of adrenaline and concurrent inhalation of HCFC 124 for an additional 5 min induced cardiac sensitization. The NOEL was 10,000 ppm.
Subchronic and Chronic Toxicity
Trochimowicz et al. (Citation1977) exposed rats to a level of 100,000 ppm of HCFC 124 6 h/day, 5 days/week for 2 weeks. No adverse effects were seen. Malley et al. (Citation1996, 1998) exposed rats to levels of 0, 500, 2000, 10,000, and 50,000 ppm 6 h/day, 5 days/week for 4 weeks. There were no significant signs of toxicity, although some signs of CNS depression were seen during the exposures. These effects were rapidly reversible at the end of each exposure. In two separate studies, Malley et al. exposed male and female rats and mice to HCFC 124 at levels of 0, 5000, 15,000, and 50,000 ppm. The only significant effects seen with the rats consisted of reversible CNS depression during the exposure and, in a few males, post-exposure; and an increase in urinary fluoride levels accompanied by mild diuresis. The mice also showed reversible CNS depression and increased urinary fluoride levels. Following these studies, a study was conducted in which rats were again exposed to HCFC 124 at levels of 0, 5000, 15,000, and 50,000 ppm for 6 h/day, 5 days/week for 2 years. Similar effects were reported. In none of these studies were there consistent effects on body weight, hematology, serum chemistry parameters, or histopathology.
Pharmacokinetics, metabolism, and mechanisms
When rats were exposed to 100,000 ppm HCFC 124 for 2 h, both trifluoroacetic acid and inorganic fluoride were found in the urine, indicating oxidative metabolism (Olson et al. Citation1991). As noted above, increases in urinary fluoride levels were seen in both chronic and sub-chronic exposure studies (Malley et al. Citation1996). Harris et al. (Citation1992) further reported that when rats were exposed for 6 h to 100,000 ppm, trifluoroacylated proteins were found in the liver and trifluoroacetic acid was found in the urine. The rate of oxidative metabolism is approximately one-fifth that of halothane (1-bromo-1-chloro-2,2,2-trifluoroethane) or 1,1,-dichloro-2,2,2-trifluoroethane.
Gas-uptake pharmacokinetics and metabolism of 2-chloro-1,1,1,2-tetrafluoroethane (HCFC 124) were investigated in rats, mice, and hamsters. Species differences in the rate of uptake of HCFC 124 and urinary excretion of trifluoroacetic acid were observed in rats and mice. The uptake of HCFC 124 in the rats and mice was described by both saturable and first-order components, whereas in the hamster only first-order uptake was observed. The production and excretion of trifluoroacetic acid, the major urinary metabolite of HCFC 124, was also simulated in rats and mice, but not in hamsters. The blood:air partition coefficient of HCFC 124 in the hamster (0.76) was lower than in the rat (1.52) or mouse (1.15) (Loizou and Anders Citation1995).
Reproductive and developmental
In two separate studies, groups of pregnant rats or rabbits were exposed to HCFC 124 at levels of 0, 5000, 15,000, or 50,000 ppm. For the rats, the exposures were 6 h/day on gestation days 6–15; for the rabbits, the exposures were 6h/day on gestation days 6–18. The dams in the rat study showed slightly decreased body weight gain at 50,000 ppm. The does in the rabbit study showed decreased in-chamber activity and decreased food consumption at 50,000 ppm. No effects were seen in the offspring from either study (Malley et al. Citation1996).
There are no data available on the reproductive effects associated with exposure to HCFC 124. However, the absence of any effects on reproductive organs in the sub-chronic and chronic study, and the lack of developmental effects in the studies described above, indicate that it is unlikely that exposure to HCFC 124 would cause reproductive effects.
Carcinogenesis
No evidence for carcinogenicity was seen in the study described above, where rats were exposed to levels up to 50,000 ppm 6 h/day, 5 days/week for 2 years (Malley et al. Citation1998).
Genetic and related cellular effects studies
In Vitro
The in vitro mutagenicity of HCFC 124 was evaluated in a reverse mutation assay using S. typhimurium strains TA1535, TA1537, TA1538, TA98, and TA100 and Escherichia coli WP2P. No activity was seen (Barsky Citation1976; Brusick Citation1976; Longstaff et al. Citation1984; Malley et al. Citation1998). It was also inactive when tested with S. cerevisiae (Brusick Citation1976), Chinese hamster ovary cells (Edwards Citation1991), and human lymphocytes (Malley et al. Citation1998). All studies were conducted both with and without metabolic activation.
In Vivo
The mutagenic potential of HCFC 124 was also evaluated in one in vivo system, the mouse micronucleus assay. Mice were exposed to 99,000 ppm 6 h/day for 2 days. HCFC 124 did not induce micronuclei and was therefore inactive in this assay (Rickard Citation1990).
Neurological effects
It has been reported that HCFC 124 has good anesthetic properties when administered at levels of 40–70% (Van Poznak and Artusio Citation1960).
Human experience
Standards, Regulations, or Guidelines of Exposure
The American Industrial Hygiene Association’s WEEL Committee recommends a permissible exposure level of 1000 ppm as an 8 h TWA for HCFC 124 (WEEL Citation2005). The AIHA’s ERP committee has developed emergency guideline levels for exposures lasting up to 1 h (ERPG, 2008).
1,1-Dichloro-1-Fluoroethane (CAS Number: 1717–00-6; Mol Wt 116.95; C2H3Cl2F)
Common synonyms include HCFC 141 b, R-141b, dichlorofluoroethane. It is a liquid at room temperature and has a vapor density of 4.83 g/L; its solubility in water is 4 g/L and a log Pow of 2.3. HCFC 141 b is nonflammable and has a light ethereal odor with poor warning properties (ECETOC Citation1994b).
Production and use
HCFC 141 b has been developed as a substitute for the fully halogenated chlorofluorocarbons, mainly for use as a blowing agent for polyurethane and polyisocyanate insulating foams and as a solvent in electronic and other precision cleaning applications. It is viewed as an interim compound, and production is being phased out since 2003 (ECETOC Citation1994b).
Exposure assessment
There is no natural source for HCFC 141 b. On the basis of its physical properties, when released into the environment, it is expected to partition almost exclusively into the air. Aqueous discharges would be expected to have short half-lives in the order of days to a few weeks (ECETOC Citation1994b).
Acute toxicity
No mortality occurred when rats were given acute oral doses of 5000 mg/kg (Sarver Citation1988) or dermal doses of 2000 mg/kg (Gardner Citation1988; Janssen and Pot Citation1988) or when rabbits were given dermal doses of 2000 mg/kg (Brock Citation1988b).
Acute inhalation studies in mice resulted in a 30 min LC50 > 64,000 ppm and in rats it was 100,000 ppm (Davies et al. Citation2009). The 4 h LC50 in rats was 62,000 ppm (Hardy Citation1989). It was also reported that 60% of a group of mice exposed to 80,000 ppm of HCFC 141 b for 30 min died (Vlachos Citation1989).
Cardiac sensitization
As with other halogenated hydrocarbons, inhalation by dogs of HCFC 141 b, coupled with an intravenous injection of epinephrine (adrenaline) to stimulate stress, can induce a sensitization response. The threshold concentration for this effect with HCFC 141 b was 5000 ppm, and the NOEL was 2500 ppm in one study (Mullin Citation1977). In a later study, using both dogs and monkeys, cardiac sensitization was induced with the epinephrine challenge at 5000–10,000 ppm in monkeys, whereas with dogs the NOEL was 9000 ppm and the threshold was 13,000 ppm (Brock et al. Citation1995).
Subchronic and chronic toxicity
No adverse effects were reported when rats were exposed to 10,000 ppm of HCFC 141 b 6 h/day, 5 days/week for 2 weeks. Likewise, when inhalation studies were conducted using rats with exposure levels of ≤20,000 ppm 6h/day, 5 days/week for 4 or 13 weeks, the primary effects were slight body weight gain, and food consumption depressions and elevations of serum cholesterol, triglyceride, and glucose at 20,000 ppm. The NOEL was 8000 ppm in these studies (Hino et al. Citation1992; Brock et al. Citation1995).
A chronic study was also conducted with rats. In this study, initially the high exposure level was 15,000 ppm, which after 4 months was increased to 20,000 ppm as no adverse signs had been seen at 15,000 ppm. With the exception of an increase in testicular masses, which will be discussed in the carcinogenicity section, the only observations of toxicity were a slight decrease in body weight gain and food consumption (Millischer et al. Citation1995).
Pharmacokinetics, metabolism, and mechanisms
Van Dyke (Citation1974) reported a low level of dechlorination when rat hepatic microsomes were incubated with HCFC 141 b. There was no increase in urinary fluoride levels in the 2 year inhalation study where exposure levels were as high as 20,000 ppm supporting the observation that the rate of metabolism is slow.
Anders has shown that pyridine-induced rat hepatomicrosomes can convert HCFC 141 b into 2,2-dichloro-2-fluoroethanol presumably through cytochrome P450 2E1. The in vitro rate constants were Km = 0.39 and Vmax = 2.08 nmol/mg protein per hour. The rate of metabolism could not be quantified when studies were conducted using microsomes from nonpyridine-treated rats or rats treated with diallyl sulfide, a selective inhibitor of cytochrome P450 2E1 (Loizou and Anders Citation1993). They also exposed male rats to concentrations of ≤14,800 ppm for 6 h in a closed-loop inhalation chamber system. There was an initial rapid uptake period of 80 min followed by a slower uptake phase. The initial uptake was attributed to uptake and equilibrium for well-perfused tissues, but the latter phase appeared to be related to either saturable metabolism or deposition in poorly perfused tissues. The kinetic constants were Km = 59.9 μmol/L and Vmax = 1.75 μmol/h. 2,2-Dichloro-2-fluoroethanol was found in the urine. Similar results were obtained when exposure levels of 11,400 ppm and 40,000 ppm were used (Harris and Anders Citation1991a, Citation1991b).
Reproductive and developmental effects
A two-generation reproduction study was conducted with HCFC 141 b (Rusch et al. Citation1995). In this study, groups of 32 male and 32 female rats were exposed to levels up to 20,000 ppm 6 h/day, 7 days/week. The F0 generation (initial parents) were exposed for 10 weeks starting at 7 weeks of age. At that point, they were paired for the first of two matings. Exposures were continued until day 4 postpartum of the second litter (F1b). In this group, 28 male and 28 female pups from the F1a litters were exposed from day 28 for 16 weeks and then paired. Exposures were continued through weaning of the F2 generation. The only reproductive effect associated with the exposures was a decrease in the number of litters in the F0 parents exposed to 20,000 ppm. This was seen at both matings, but not seen in mating of the F1 parents exposed to 20,000 ppm. No reproductive effects were seen at the lower levels of 2000 and 8000. Litter size may also have been reduced at 20,000 ppm but not 8000 or 2000 ppm. At birth, pup weights in treated groups were comparable to controls. In the 20,000 ppm exposure group they tended to show a reduced rate of body weight gain. This may have contributed to a delay in male sexual maturation (50.8 days compared to 48.7 days) in this group. Otherwise, no effects were seen.
Developmental toxicity studies were conducted in both rats and rabbits (Rusch et al. Citation1995). Rats were exposed to levels of ≤20,000 ppm, 6 h/day on gestation days 6–15; the rabbits were exposed to levels of ≤12, 600 ppm 6 h/day on gestation days 7–19. The pregnant rats, especially in the high exposure group, showed transient signs of mild toxicity. At 20,000 ppm there was also an increase in early and late embryonic deaths and, as seen in the reproduction study, decreased pup weights. Delayed ossification was also seen at 20,000 ppm. There was no evidence for maternal or embryotoxic effects at 8000 ppm, and there was no evidence for teratogenicity at any level.
With the rabbits, there was some evidence for maternal toxicity at 4200 and 12,600 ppm but not 1400 ppm. There was no evidence of any treatment-related effects on the pups (Rusch et al. Citation1995).
Carcinogenesis
A 2 year inhalation study was conducted during which four groups of 80 male and 80 female Sprague-Dawley rats were exposed to levels of 0, 1500, 5000, and 15,000–20,000 ppm, 6 h/day, 5 days/week (Millischer et al. Citation1995). The high exposure level was increased from 15,000 to 20,000 ppm after 4 months since no signs of toxicity were seen at 15,000 ppm. At the end of 2 years, an increased incidence of benign Leydig cell tumors and Leydig cell hyperplasia was seen in the male rats 5000 and 20,000 ppm, but not at 1500 ppm. These tumors are common in the aging rat and did not follow an exposure related pattern. They are not considered to be a risk factor for humans (ECETOC Citation1994b). No other treatment related effects were seen.
Genetic and related cellular effects studies
The mutagenic potential of HCFC 141 b has been evaluated in a series of reverse mutation assays with S. typhimurium strains TA98, TA1535, TA1537, and TA1538 and E. coli WP2, WP67, and CMB71. It was inactive in one study and only weakly active in one strain (TS1535) in another study (Trochmowicz et al. 1978; Millischer et al. Citation1995). It was also inactive in the HGPRT Chinese hamster V79 cell (Bootman and Hodson-Walker. Citation1988) human lymphocyte chromosome aberration, and mouse micronucleus studies (Millischer et al. Citation1995). It did show positive results in the Chinese hamster ovary (CHO) cell chromosome aberration study (Bootman and Hodson-Walker Citation1988). Exposure of rats to levels up to 6000 ppm for 13 weeks resulted in a significant increase in micronuclei frequencies in the males exposed to 6000 ppm, but not the females (Brock et al. Citation1995; Maeng et al. Citation2004). Overall, it is not considered to be genotoxic agent (Bootman and Hodson-Walker Citation1988; Hodson-Walker Citation1990).
Dermal effects
HCFC 141 b was not a sensitizer when tested for dermal sensitization in the guinea pig (Brock et al. Citation1995).
Neurotoxicity
The potential for HCFC 141 b to cause neurotoxic effects was evaluated in an inhalation study in which rats were exposed to levels of ≤15,000 ppm, 6 h/day, 5 days/week for 16 week. No evidence of neurotoxicity was seen (Brock et al. Citation1995).
Human experience
A clinical study was conducted in which volunteers were exposed to ≤1000 ppm for ≤4 h. Evaluations were conducted for clinical signs, nasal irritation, cardiac effects, and effects on blood pressure and pulse. No effects were seen. Considering the metabolites found in blood and urine samples, it appeared that humans also metabolize HCFC 141 b to dichlorofluoroethanol. The rate could not be determined (Tong et al. Citation1998).
It has been reported that over-exposure to HCFC 141 b in humans resulted in reversible parenchymal lung damage that was characterized by ground-glass opacities in lung X-ray, elevated BAL, neutrophil counts, and restrictive ventilatory impairment. However, exposure levels were not provided. (Lee et al. Citation2009). Without estimates of the exposure levels, the significance of these observations can not be determined.
Standards, regulations, or guidelines of exposure
The American Industrial Hygiene Association’s WEEL Committee recommends 500 ppm as an 8 h TWA for HCFC 141 b (WEEL 2008).
The National Advisory Committee for Acute Exposure Guidance Levels has developed the following guidance levels for single, rare incidents, for exposures lasting up to 8 h (AEGL Citation2002).
1-Chloro-1,1-Difluorodifluroethane (CAS # 75–680-3; Mol Wt 100.5; C2H3ClF2)
Common synonyms include HCFC 142 b, R-142b, chlorodifluoroethane. It has a vapor density of 3.5; a water solubility of 1.9 g/L; and a log Pow of 1.75. Its flammability limits are 6–18% and it has a light ethereal odor with poor warning properties (IPCS 1992; ERPG 1999).
Production and use
HCFC 142 b is produced by liquid-phase hydrofluorination of methylchloroform or vinylidene chloride. It is used primarily as a chemical intermediate in the production of fluoropolymers and is a substitute for chlorofluorocarbons as a refrigerant and blowing agent (ERPG 1999).
Exposure assessment
Environmental levels and exposure of the general public are negligible since HCFC 142 b is produced in an enclosed system and used predominantly as a chemical intermediate.
Air
Its low water solubility (1.9 g/L) and high vapor pressure (42 psia at 20 °C) would suggest that it would partition primarily in air, not water (IPCS 1992).
Acute toxicity
HCFC 142 b is a gas at room temperature. No information is available on acute oral or dermal toxicity, nor on its potential to cause allergic dermal sensitization.
Mice exposed to HCFC 142 (isomer not specified) at concentrations above 440,000 ppm died after a few minutes and showed severe vascular disorders in the lungs and brain (plethora and perivascular edema); cells of the brain cortex were swollen. Concentrations between 320,000 and 440,000 ppm were fatal to mice exposed for 2 h (Nikitenko and Tolgskaya Citation1965). Davies et al. (Citation2009) found the 30 min LC50 for HCFC 142 b in mice to be 300,000 ppm.
Rats were exposed to HCFC 142 b concentrations of 150,000–800,000 ppm for 30 min. Although slight intoxication was apparent on exposure to 150,000 ppm, the postural reflex did not disappear until the concentration reached 200,000 ppm. Unconsciousness and death occurred at 300,000–500,000 ppm. Effusion of fluid from the respiratory tract occurred on exposure to concentrations of 300,000 ppm (Lester and Greenberg Citation1950).
Mecler and Knapinsky (Citation1978) exposed Sprague–Dawley CD rats to 200,000 and 400,000 ppm of HCFC 142 b for 6 h. Rapid and labored breathing, lethargy, and discharge from eyes and noses were observed only during exposure at the highest concentration. During exposure to 400,000 ppm, there was a 20% mortality after 5 or 5.5 h. Necropsy of the surviving rats after 14 days observation revealed dark red mottling of the lungs and kidneys, especially in animals at the highest exposure levels.
It was also reported in an early study (Carpenter et al. Citation1949) that mortality was seen with exposures in the range of 88,000–180,000 ppm.
Cardiac Sensitization
The ability of HCFC 142 b to sensitize the heart to adrenaline was studied in beagle dogs. No responses were noted in six animals exposed to a HCFC 142 b concentration of 25,000 ppm for 5 min and then challenged with adrenaline (epinephrine) to simulate stress. Of 12 animals exposed to 50,000 ppm, 5 dogs showed marked responses, and all 12 dogs exposed to 100,000 ppm showed marked responses (Reinhardt, Azar et al. Citation1971).
The effect of endogenous adrenaline on the cardiac sensitization potential was also studied in dogs. Groups of 12 beagle dogs were exposed to 800,000 ppm of HCFC 142 b (80% w/w + 20% oxygen) for 30 s. An amplified sound-effect tape recording was played immediately after the exposure to stimulate the release of endogenous adrenaline. In combination with exposure to 800,000 ppm HCFC 142 b, the noise stimulus resulted in 5 out of 12 animals showing marked responses compared with 1 out of 12 with the compound alone and none with the noise alone (Reinhardt, Azar et al. Citation1971).
These two studies allow us to compare the increase in sensitivity seen with the injection of adrenaline. When injections of adrenaline were employed in conjunction with exposure to HCFC 142 b, a response was seen in 5 out of 12 dogs at 50,000 ppm. In the absence of adrenaline, albeit with a shorter exposure time, a concentration of 800,000 ppm with noise was required to produce a similar response, representing a 16-fold difference. When only the compound exposure was used, without exogenous adrenaline or noise, even exposure to 800,000 ppm resulted in only one cardiac arrhythmia.
Cardiovascular and respiratory function
Groups of three rhesus monkeys were anesthetized by i.v. injection of sodium pentobarbital and exposed for periods of 5 min to concentrations of 50,000 and 100,000 ppm (Belej et al. Citation1974). HCFC 142 b did not produce arrhythmia or tachycardia; the most characteristic effect was depression of myocardial contractility and a fall in aortic blood pressure. In a second study, three anesthetized rhesus monkeys were again exposed to 50,000 ppm and four to 100,000 ppm HCFC 142 b for 5 min. There was no effect on pulmonary resistance or compliance, but respiratory stimulation was noted (Aviado and Smith Citation1975).
Subchronic and chronic toxicity
A few studies have indicated that repeat exposure of rats to HCFC 142 b at levels of 20,000 ppm 6 h/day, 5 days/week for 2 weeks or exposure of male beagle dogs and male and female rats to levels of 10,000 and 1000 ppm 6 h/day, 5 days/week for 13 weeks did not reveal any signs of toxicity (Kelly Citation1978; Moore and Trochimowicz Citation1976). In one poorly described early study (Nikitenko et al. 1965), exposure of rats and guinea pigs to a level of 109,000 ppm 2 h/day, 6 days/week for 4 weeks resulted in histopathological changes in the lungs and slight effects on body weight and some hematological changes. In a second study (Lester and Greenberg Citation1950), exposure of rats 16 h/day, for 9 days at 100,000 ppm resulted in the death of all animals. Exposures to 10,000 ppm 16 h/day for 2 months caused only minor irritation of the lungs. While no irritation was seen in the chronic study described below with exposures to ≤20,000 ppm. This study involved exposure periods of 6 h.
Pharmacokinetics, metabolism, and mechanisms
It was noted in the subchronic study in which dogs and rats were exposed to 10,000 ppm of HCFC 142 b, for 13 weeks, that there was no increase in urinary fluoride levels (ERPG 1999). This suggests that there was little or no metabolism. Van Dyke (Citation1974) reports that HCFC 142 b can undergo dechlorination in a reaction catalyzed by cytochrome P450.
Reproductive and Developmental Effects
There was no evidence for developmental, embryotoxic, or teratogenic effects when groups of 25 pregnant rats were exposed to levels of 0 (control), 1000, or 10,000 ppm 6 h/day, on days 3–15 of pregnancy (Kelly Citation1978). Likewise, there was no adverse effect when groups of 20 pregnant rats were exposed to 0 (control) to 2000 or 10,000 ppm 6 h/day on gestation days 6–15 (Damske et al. Citation1978). It did not induce adverse effects on fertility of male mice exposed to levels up to 20,000 ppm in a dominant lethal study (Seckar et al. Citation1986).
There is no information on studies of reproductive effects. However, the absence of any effects on reproductive organs in the subchronic study and the lack of developmental effects in the studies described above and the lack of effects on fertility in the dominant lethal study indicate that it is unlikely that exposure to HCFC 142 b would cause reproductive effects.
Carcinogenesis
In a combined chronic toxicity/carcinogenicity study, four groups of 130 male and 110 female Sprague–Dawley CD rats were exposed to HCFC 142 b concentrations of 2000, 10,000, and 20,000 ppm for 6 h/day, 5 days/week for 104 weeks. No treatment-related effects were found on mortality or body weight on hematological, clinical chemical, urine analysis, or ophthalmological examination; on histopathological examination of organ tissues; or on the incidence of the neoplasia (Seckar et al. Citation1986).
Genetic and related cellular effects studies
In reverse mutation assays with S. typhimurium strains TA1535, TA1527, TA1538, TA98, and TA100 using exposure levels of ≤50% v/v in air and exposure periods of ≤48 h HCFC 142 b was weakly positive (Barsky and Butterworth Citation1976; Jagannath Citation1977; Koops and Krahn Citation1977; Longstaff et al. Citation1984).
In a cell transformation assay, Seckar et al. (Citation1986) reported a positive response with baby hamster kidney fibroblasts (BHK 21) with metabolic activation but not without metabolic activation.
HCFC 142 b was not active in a rat bone marrow cytogenetics assay and dominant lethal assay, both using exposures of ≤20,000 ppm for 6 h/day, 5 days/week for 15 weeks (Seckar et al. Citation1986).
Human experience
There is no information on exposureto HCFC 142b.
Standards, regulations, or guidelines of exposure
Occupational
The American Industrial Hygiene Association’s WEEL Committee recommends 1000 ppm (4100 mg/m3) as an 8 h TWA (WEEL Citation1994) for HCFC-142b. The Dutch occupational exposure limit for 8 h is 2000 ppm; the occupational exposure limit for 15 min is 4000 ppm (DEC Citation1987).
Emergency exposure guidance level
The American Industrial Hygiene Association’s Emergency Response Planning Committee (ERPG) recommends the following guidance levels for rare, accidental exposures to the general public, not exceeding 1 h (ERPG 2010):
Hydrofluorocarbons
Since hydrofluorocarbons do not contain chlorine or bromine, they have zero ozone depletion. They contain hydrogen making them susceptible to attack by the hydroxyl radicals in the troposphere and therefore having relatively short atmospheric lifetimes. However because they do not contain chlorine they are very poor solvents and are primarily used as refrigerants.
Difluoromethane (CAS# 75–10-5; mol wt 52.024; CH2F2)
Common synonyms include HFC 32, R-32, HFA 32. Its vapor density is 1.8; solubility in water is 4.4 g/L and log Pow is 0.21 (ECETOC Citation1995a). Its lower flammable limit is 13.6% and upper limit is 32.2%. It is odorless or has a light ethereal odor with poor warning properties.
Production and use
HFC 32 has been developed as a replacement for fully halogenated chlorofluorocarbons. Its main application is as a low temperature refrigerant, especially in blends with other hydrofluorocarbons. Its solubility in water is 4.4 g/L and its log Pow is 0.21, indicating a very low potential for bioaccumulation (ECETOC Citation1995a).
Acute toxicity
No mortality was observed in male or female rats exposed to concentrations of ≤520,000 ppm (52%). A reduced response to sound was noted during exposures at ≥82,000 ppm. The animals recovered rapidly after the exposures (ECETOC Citation1995b).
Cardiac sensitization
The potential of HFC 32 to sensitize the heart to epinephrine (adrenaline) was investigated by Hardy and Kieran (Citation1992) on a group of eight beagle dogs. Exposures to HFC 32 commenced at 150,000 ppm, and further exposures were carried to a maximum of 350,000 ppm. Adrenaline was administered before exposure to provide a baseline response and after 5 min during exposure to HFC 32 to simulate stress. No cardiac sensitization effects were seen with any animal at any concentration of HFC 32. It was concluded that HFC 32 did not cause a cardiac sensitization response in the dog at concentrations of ≤350,000 ppm. In this study, clinical signs indicative of a prenarcotic response (e.g., head and limb tremors, unsteady gait) were seen in all eight animals at 350,000 ppm HFC 32. Five of the animals were affected at a concentration of 300,000 ppm, and one was affected at 250,000 ppm.
Subchronic and chronic toxicity
Trochimowicz et al. (Citation1977), describe a study in which male rats were exposed 6 h/day, 5 days/week for 2 weeks to HFC 32 at a concentration of 200,000 ppm. There were no changes in clinical signs, hematology, or urine and blood biochemistry, and no histopathological changes were detected in any of the organs examined.
In a 4 week inhalation study Wistar-derived rats were exposed to concentrations of 0 (control), 2000, 10,000, or 50,000 ppm HFC 32, for 6 h/day, 5 days/week (Ellis, Naylor et al. Citation1996). There were no deaths or treatment related effects on clinical signs, body weights, food consumption, organ weights, or pathology. Clinical chemistry and hematological investigations revealed no treatment related effects. Hepatic cyanide insensitive palmitoyl CoA oxidation activity was similar in treated and control groups, indicating the absence of increased peroxisomal β-oxidation. The no observed effect level is 50,000 ppm the highest level tested.
In an inhalation study, Wistar-derived rats were exposed to concentrations of 0 (control), 5000, 15,000, or 50,000 ppm HFC 32, for 6 h, 5 days/week over a 90 day period (Ellis, Naylor et al. Citation1996). There were no mortalities and no treatment-related effects on clinical signs, food consumption, or growth during or after exposure to HFC 32. There were no effects on hematological parameters or clinical chemistry determinations, or on urinary measurements made during the study. There were no effects on group mean organ weights or in the incidence of gross or microscopic findings in treated animals. The NOEL in this study was 50,000 ppm, the highest level tested.
Pharmacokinetics, metabolism, and mechanisms
Gas uptake, tissue partition coefficients, and kinetic parameters of dihalomethanes were studied by Gargas et al. (Citation1986). The sample of HFC 32 used was a 30: 70 mixture with chlorofluoromethane (HCFC 31), which limits the usefulness of the data. However, in studies with exposure levels of ≤73 ppm and exposure periods of ≤6 h in duration, HFC 32 was not metabolized to any significant degree.
HFC 32 uptake in the rat was measured using 14C-labeled material (Ellis, Trebilcock et al. Citation1996) with exposure levels of 10,000, 25000, and 50,000 ppm for ≤8 h. Blood levels increased in a linear manner with respect to exposure level. The amount of HFC 32 eliminated as carbon dioxide increased in proportion to exposure concentration, reaching a maximum rate of 667 μmol/kg of body weight per hour by 4–5 h at 50,000 ppm. It was concluded that the body burden of HFC 32 and its metabolism are dependent on the partitioning between alveolar airspace and blood.
Ellis, Trebilcock et al. (Citation1996) studied the metabolism of HFC 32 in male rats and mice. The total retained dose after a 6 h exposure at 100,000 ppm was 1%. Of this, approximately one-half was exhaled as HFC 32 within 1 h. The remainder was either exhaled as carbon dioxide rats or mice (0.23 or 0.27%, respectively) or eliminated in the feces (0.03 or 0.07%, respectively). They noted that there was no significant carboxyhemoglobin formation, indicating that metabolism via carbon monoxide is not a significant pathway.
Reproductive and developmental effects
The potential teratogenic activity of HFC 32 was investigated using the modified Chernoff–Kavlock assay (Ellis, Trebilcock et al. Citation1996). Groups of 10 pregnant female Wistar-derived rats were exposed to 0, 10,000, or 50,000 ppm HFC 32 for 6 h/day on gestation days 7–16. The animals were allowed to litter and the number, weight, and viability of their offspring were assessed between days 1 and 5 postpartum. HFC 32 at either concentration had no effect on the females during gestation or on litter size at birth or pup survival to day 5. A slight reduction in pup weight gain at 50,000 ppm was recorded. However, according to the criteria for assessing the result of this assay, HFC 32 was neither teratogenic nor fetotoxic at the concentrations tested.
Pregnant rats (Wistar-derived) were exposed to concentrations of 0 (control), 5000, 15,000, and 50,000 ppm (Ellis, Trebilcock et al. Citation1996) for 6 h/day of difluoromethane. Exposures were on gestation days 7–16 to groups of 24 animals. There was no effect on maternal body weight gain. However, a slight reduction in food consumption associated with exposure to 50,000 ppm HFC 32 suggested a minimal effect on the dams. Overall there was no adverse effect on number, growth, and survival of fetuses in utero. There was no increase from control values in the incidence of major defects in any treated group. An increase in the proportion of fetuses with minor external or visceral defects achieved statistical significance in the 50,000 ppm group. These findings were of low incidence and were typical of the general minor variants or defects compared to controls, which is considered at most to indicate minimal fetotoxicity at 50,000 ppm in the presence of minimal maternal toxicity.
Groups of 24 pregnant New Zealand white rabbits were exposed to HFC 32 at concentrations of 0 (control), 5000, 15,000, and 50,000 ppm for 6 h/day on gestation days 6–18 (Ellis, Trebilcock et al. Citation1996). During days 8–10 of pregnancy, there was a slight but statistically significant reduction of maternal body weight gain. Subsequent body weight gain was similar to that of controls. There was no evidence of fetotoxicity. Numbers of malformations and skeletal and visceral anomalies were not statistically increased above control values in any exposed group.
These findings were confirmed by Ema et al. (Citation2010). They saw slight maternal and developmental toxicity in rats, but not rabbits, with exposures of 50,000 ppm.
In conclusion, HFC 32 was not teratogenic in the rat or rabbit at any exposure level. There was evidence of minimal maternal toxicity in both species, and minimal fetotoxicity in the rat at 50,000 ppm.
There are no data available on the reproductive effects associated with exposure to HFC 32. However, the absence of any effects on reproductive organs in the subchronic study and the lack of developmental effects in the studies described above indicate that it is unlikely that exposure to HFC 32 would cause reproductive effects.
Genetic and related cellular effects studies
In Vitro
Ellis, Trebilcock et al. (Citation1996) evaluated HFC 32 in a reverse-mutation assay using S. typhimurium strains TA1535, TA1537, TA98, and TA100 as well as E. coli strains WP2P and WP2P uvrA. The system employed a special chamber designed for gas-phase exposures and used test atmosphere levels of ≤100% HFC 32 both with and without S9 metabolic activation. HFC 32 was not active in these assays.
The clastogenic potential was evaluated using both Chinese hamster lung cells (Asakura Citation1993) and human lymphocytes (Ellis, Trebilcock et al. Citation1996) with gas-phase exposures of ≤800,000 ppm or 100,000 ppm, respectively. Both studies were conducted in the presence and absence of S9 metabolic activation. HFC 32 exhibited no clastogenic activity in either study.
In Vivo
The genotoxic potential of HFC 32 was investigated in the mouse bone marrow micronucleus test (Ellis, Naylor et al. Citation1996). Male and female mice were exposed to 132,000 ppm of HFC 32 for 6 h. No statistically significant increase in mononucleated polychromatic erythrocytes was seen.
Human experience
General Information
There are currently no reports on human exposures to this chemical.
Standards, Regulations, or Guidelines of Exposure
The American Industrial Hygiene Association’s WEEL Committee recommends 1000 ppm as an 8 h TWA exposure limit for HFC 32 (WEEL Citation2012).
Studies on environmental impact
Atmospheric Lifetime
The atmospheric degradation of HFC 32 will occur mainly in the troposphere, initiated by attack by naturally occurring hydroxyl radicals; reaction with other species, such as oxygen or chlorine atoms, is negligible even in the stratosphere, as is direct photolysis (Schmoltner et al. Citation1993).
Pentafluoroethane (CAS# 354–33-6; mol wt 120.02; C2HF5)
Common synonyms include HFC 125, R-125, HFA 125, 1,1,1,2,2-pentafluoroethane.
It has a solubility in water of 0.97 g/L and a logPow of 1.48. It is nonflammable and has a light ethereal odor with poor warning properties (ECETOC 1994).
Production and use
HFC 125 is used mainly as a high-pressure refrigerant. It has also found use as a total flooding fire-extinguishing agent.
Air
The atmospheric degradation of HFC 125 will take place mainly in the troposphere by reaction with naturally occurring hydroxyl radicals. HFC 125 degrades in the atmosphere to CO2 and HF (ECETOC Citation1994c).
Background levels
The low octanol/water partition coefficient indicates that HFC 125 is unlikely to bioaccumulate. Its physical properties (gas and low water solubility) suggest a low risk to the aquatic environment.
Acute toxicity
The acute inhalation toxicity of pentafluoroethane is very low. The 4 h LC50 in the rat is >800,000 ppm. During the exposure the only clinical signs were ataxia, decrease of locomotor activity, and dyspnea, all of which were rapidly reversible (Kawano et al. Citation1995).
Cardiac sensitization
As with many other fluorocarbons, inhalation of high concentrations of HFC 125, followed by an intravenous epinephrine challenge to simulate stress, can induce a cardiac sensitization response in dogs. The threshold concentration for this effect was 100,000 ppm, and the no-observed-effect concentration (NOEC) was 75,000 ppm (Kawano et al. Citation1995).
Subchronic and chronic toxicity
Exposure of rats by inhalation to ≤50,000 ppm for 5 days a week, for 4 and 13 consecutive weeks, did not induce any toxic effect; the NOEC was therefore >50,000 ppm (Kawano et al. Citation1995).
There are no data on carcinogenicity; however, similar compounds such as HCFC 124 and HFC 134a do not represent carcinogenic hazards.
Pharmacokinetics, metabolism, and mechanisms
In limited studies in vivo in the rat, the metabolism of HFC 125 was negligible. The only observed urinary metabolite was trifluoroacetic acid (Kawano et al. Citation1995).
Reproductive and developmental
Developmental toxicity studies by the inhalation route were carried out in both rats and rabbits. No evidence of embryotoxicity or teratogenicity was seen even at exposure levels as high as 50,000 ppm (Kawano et al. Citation1995). Reproductive toxicity has not been evaluated. Given the very low toxicity of HFC 125, the absence of any effects on reproductive organs in the subchronic study, and the lack of developmental effects, it is unlikely to be a reproductive toxin.
Carcinogenesis
HFC was not evaluated for carcinogenic potential. It did not show any signs of toxicity in a 90 day inhalation toxicity study. It was not active in a series of genotoxicity studies. Also, HCFC-124, 1-chloro-1,2,2,2-tetrafluoroethane, a chemical that is very similar to HFC-125, but more reactive, was not carcinogenic in a rat, 2 yr, inhalation carcinogenicity study.
Genetic and related cellular effects studies
In Vitro
HFC 125 was not active as a reverse mutation assay with S. typhimurium tester strains TA1535, TA1537, TA98, and TA100 and E. coli strains WP2P and WP2P uvrA both with and without metabolic activation. Concentrations of ≤100% in the air above the culture dishes were used (Kawano et al. Citation1995).
The clastogenic potential of HFC 125 was evaluated using both Chinese hamster lung (CHL) cells and human lymphocytes. In these studies, evaluations were conducted both with and without S9 metabolic activation. Exposure levels in the air above the cell cultures were ≤60% and ≤70% for the CHL and human lymphocyte studies, respectively. HFC 125 was not active in any of these assays (Kawano et al. Citation1995).
In Vivo
The genotoxic potential of HFC 125 was investigated in vivo in the mouse bone marrow micronucleus test. Groups of male and female CD1 mice were exposed for 6 h to concentrations of ≤60% of HFC 125 in air. No clastogenic activity was seen (Kawano et al. Citation1995).
Human experience
General Information
There are currently no reports on adverse effects on humans resulting from exposures to this chemical.
Standards, Regulations, or Guidelines of Exposure
The American Industrial Hygiene Association’s WEEL Committee recommends 1000 ppm as an 8 h TWA for workplace exposures (WEEL Citation2009).
1,1,1,2-Tetrafluoroethane (CAS# 811–97-2; mol wt 102.03; C2H2F4)
Common synonyms include HFC 134a, HFA 134a, R-134a, Fluorocarbon 134a It has a vapor density of 3.52, solubility in water of 1 g/L and a log Pow of 1.06 (ECETOC Citation1995b). It is nonflammable and is practically odorless with very poor warning properties.
Production and use
HFC 134a is being used in residential, commercial, and automotive air-conditioning systems; in foam blowing; in fire suppression; and as a propellant in metered dose inhalants (ECETOC Citation1995b).
Exposure assessment
There are no natural sources of HFC 134a. On the basis of its physical properties, it may be expected, when released, to partition almost exclusively into the atmosphere. Since it is a gas at room temperature with a low Henry’s law constant of 1.0 g/L⋅bar at 25 °C, any HFC 134a that might be discharged into aqueous waste streams would have a half-life of days or at most a few weeks. Since its atmospheric lifetime is 14 years (SPARC Citation2013), in the atmosphere it will be distributed uniformly on a global basis (Franklin Citation1993).
Acute toxicity
In rats, the 4 h LC50 has been reported as being >500,000 ppm, whereas the 30 min LC50 was approximately 750,000 ppm (WEEL 1997a). The 4 h approximate lethal concentration (ALC) was reported to be 567,000 ppm (Kennedy Citation1979) also with rats. Exposures for 3–5 h at levels of 700,000–800,000 ppm were not lethal to dogs (Shulman and Sadove Citation1967). Applications of liquid HFC 134a to the skin or eye produced only slight signs of irritation. There were no signs of inducing skin sensitization when applied to the guinea pig in the maximization test (Mercier Citation1989, Citation1990a, Citation1990b).
A study was conducted in which male Wistar rats were exposed to various concentrations of HFC 134a or CFC 12 for up to 30 min while performing in either a rotarod/motorized running wheel apparatus or in an operant chamber. The relative neurobehavioral toxicity was assessed by comparing both gross motor system incapacitation and more subtle changes in ability to perform an operant discrimination task. It was shown that exposure to HFC 134a or CFC 12 at concentrations from 40,000 to 470,000 ppm, for up to 30 min, induced neurobehavioral deficits in every subject, ranging from reduced operant efficiency to apparent anesthesia. HFC 134a inhalation was shown to induce deficits more rapidly, and at lower concentrations when compared to CFC 12 exposure (Ritchie et al. Citation2001).
Cardiac sensitization
The potential for HFC 134a to sensitize the heart to epinephrine (adrenaline) was investigated in beagle dogs. In one study (Mullin Citation1979), inhalation of levels of ≥75,000 ppm of HFC-134a for 5 min followed by injection of epinephrine to simulate stress and concurrent inhalation of HFC 134a induced a cardiac sensitization response at an exposure level of 75,000 ppm. No response was seen at 50,000 ppm. In a more recent study, a sensitization response was induced with exposure to 80,000 ppm, but no response was seen at 40,000 ppm (Hardy et al. Citation1991).
Subchronic and chronic toxicity
The chronic toxicity/oncogenicity of HFC 134a was evaluated in Alderly Park rats by daily administration of gavage doses of 300 mg/kg in corn oil for 1 year, followed by an additional 1 year observation period. No signs of carcinogenicity or toxicity were observed (Longstaff et al. Citation1984). A chronic inhalation toxicity/oncogenicity study has also been conducted. In this study, groups of rats were exposed to levels of ≤50,000 ppm 6 h/day, 5 days/week for 2 years. Again, no signs of toxicity were seen (Collins et al. Citation1995a). Likewise, no signs of toxicity were seen when rats were exposed to ≤50,000 ppm 1 h/day, 7 days/week for 108 weeks or when mice were exposed to levels of ≤1 h/day, 7 days/week for 104 weeks (Alexander and Libretto Citation1995).
No adverse effects were seen when rats were exposed to 100,000 ppm 6 h/day, 5 days/week for 2 weeks (WEEL, 1997) or to ≤50,000 ppm 6 h/day, 5 days/week for 13 weeks (ECETOC Citation1995b). Although slight changes were reported in liver, kidney, and testes weights in a study in which rats were exposed to levels of ≤50,000 ppm 6 h/day, 5 days/week for 4 weeks, given the absence of similar findings in the more rigorous 90-day and chronic studies, this report may be coincidental.
Pharmacokinetics, metabolism, and mechanisms
The metabolism of HFC 134a was evaluated in male and female rats (Ellis et al. Citation1993). When the rats were given a 1 h exposure to 10,000 ppm, only 1% was retained. Of this, two-thirds was eliminated unchanged within 1 h after exposure. The remainder was excreted as CO2 in the exhaled air or trifluoroacetic acid in the urine; some label was eliminated as unidentified metabolites in the feces.
In vivo oxidative defluorination was demonstrated in rat, rabbit, and human liver microsomes, resulting in inorganic fluoride and trifluoroacetic acid. This metabolism appeared to proceed using cytochrome P450 11E1 (Olson et al. Citation1990).
Reproductive and developmental effects
When male mice were exposed 6 h/day for 5 days to HFC 134a at ≤50,000 ppm and bred with unexposed female mice, no effects on fertility were seen (ECETOC Citation1995b).
When both male and female rats were exposed 1 h/day to levels of ≤50,000 ppm for one generation, no effects on reproduction were seen (Alexander et al. Citation1995).
Two teratology studies were conducted. In the first, groups of rats were exposed to ≤300,000 ppm of HFC 134a 6 h/day on gestation days 6–15. Oxygen was added to maintain oxygen levels near 20%. No teratogenic effects were seen; however, there was some growth retardation at 300,000 ppm in the pups, but not at 100,000 ppm (Lu Citation1981). In the second study, groups of 25 rabbits were exposed to HFC 134a 6 h/day at levels of ≤40,000 ppm on gestation days 7–19. No treatment-related developmental or teratogenic effects were seen (Collins et al. Citation1995).
Groups of rats were exposed nose-only to levels of 2500, 10,000 and 50,000 ppm of HFC 134a 1 h/day in a one generation reproduction study. The only effect noted was a slight decrease in body weight gain in the males exposed to 50,000 ppm. There were no adverse effects on reproduction. In a peri- and post-natal study, rats were exposed to levels of 1800, 9900 and 64,400 ppm from days 17 to 20 of pregnancy and days 1 to 21 postpartum. No effects were seen in any treatment group (Alexander et al. Citation1996).
Carcinogenesis
The results from three lifetime inhalation carcinogenesis studies with HFC 134a have been published. The first study involved exposure of groups of 80 male and 80 female rats to levels of ≤50,000 ppm 6 h/day, 5 days/week for 2 years. An increase in Leydig cell tumors was seen in the male rats at 50,000 ppm (30%) compared to the air-exposed controls (12%). Likewise, there was an increase in Leydig cell hyperplasia. No effects were seen at 10,000 ppm (Collins et al. Citation1995). The second study with rats involved snout-only inhalation exposures to levels of ≤50,000 ppm 1 h/day, 7 days/week for 108 weeks. The same investigators conducted a lifetime study with mice. In this study, groups of mice were exposed to snout-only levels of ≤75,000 ppm 1 h/day, 7 days/week for 104 weeks. No adverse effects were seen in either rats or mice (Alexander et al. Citation1995). Since the total dose received by the rats in the high exposure level of this study was lower than in the Collins’ study, this report supports the observation that 10,000 ppm, 6 h/day, 5 days/week for 2 years was a NOEL.
Rats were given 300 mg of HFC 134a in corn oil 5 days/week for 52 weeks and held for a total of 125 weeks (Longstaff et al. Citation1984). There was no evidence for carcinogenicity. Leydig cell tumors are common in aged male rats but rare in Humans. Since they were only seen in the high exposure group in one study,an extensive, but unpublished review of the significance of Leydig call tumors was conducted. It was concluded that they were not a risk factor for human exposure.
Genetic and related cellular effects studies
In Vitro
The mutagenic potential of HFC 134a was evaluated in several reverse-mutation assays with S. typhimurium strains TA98, TA100, TA1535, TA1537, and TA1538 and E. coli WP2 UvrA both with and without metabolic activation. HFC 134a was inactive in all of these assays (Brusick Citation1976; Longstaff et al. Citation1984; Callander and Priestley Citation1990; Araki Citation1991). It was also inactive in chromosome aberration studies with human lymphocytes (Collins et al. Citation1995) and Chinese hamster lung cells (Asakura Citation1991).
In Vivo
HFC 134a was not active in the mouse micronucleus assay with 6 h exposure levels of ≤500,000 ppm (Muller and Hofmann Citation1989). It did not cause chromosome aberrations in rats exposed to ≤50,000 ppm 6 h/day for 5 days (Anderson and Richardson Citation1979). It did not induce unscheduled DNA synthesis in rats exposed to levels ≤100,000 ppm for 6 h (Trueman Citation1990).
Human experience
Volunteer studies
A few volunteer studies involving inhalation of HFC 134a have been reported. One (Harrison et al. Citation1996) described trials in healthy subjects using HFC 134a as the propellant in an aerosol inhaler. The second study (Pike et al. Citation1995) investigated the deposition and retention of HFC 134a in healthy subjects and patients with chronic airflow limitations. No adverse effects were seen in either study. Both demonstrated rapid elimination of the HFC 134a. Following these studies was a limited study designed to provide information on blood levels associated with a 30 min exposure to a level of 4000 ppm. The purpose of this study was to validate a pharmacokinetic model for short-term exposures to HFC 134a. This study was terminated after the first subject fainted, having a low blood pressure and pulse, and the second developed a rapid pulse and elevated blood pressure. According to both the existing clinical data and animal toxicology data, these responses were not anticipated and did not appear to represent a direct response to the exposure. The cause for these responses was not determined (Vinegar et al. Citation1998). A more recent study was conducted using a more robust design. In this study, a group of four male and four female volunteers were exposed sequentially for 1 h to 1000–8000 ppm. Each subject received one exposure per week. No changes were seen in pulse, blood pressure, or cardiac rhythm. There were no adverse clinical signs (Emmens Citation1999a).
A study was conducted to determine the uptake and disposition of inhaled HFC 134a in humans while exercising. Ten male volunteers were exposed to 500 ppm HFC 134a for 2 h while exercising (50 W exercise). The concentration in blood increased rapidly, reaching a plateau of 9.4 ± 1.9 μM (mean ± SD) within 30 min. Elimination was equally rapid. The post-exposure urinary excretion was 0.002% of the inhaled amount, and the half-life was 58 min. A physiologically based toxicokinetic (PBTK) model was developed for further analysis. The simulated relative uptake during exposure was 3.7 ± 0.5%. No remarkable findings were observed in the electrocardiographic recordings (Gunnare et al. Citation2006).
Pharmacokinetics, metabolism, and mechanisms
In humans, as with animals, most HFC 134a is exhaled unchanged. Some undergoes oxidative metabolism to yield trifluoroacetic acid, which is eliminated in the urine (Monté et al. Citation1994).
In a 28 day double blind study, the safety of HFC 134a was compared to the chlorofluorocarbon propellant previously used in MDIs (metered dose asthma inhalants). No significant differences were seen between the two propellants (Harrison et al. Citation1996). A volunteer study was conducted where 10 healthy workers were exposed to 500 ppm of HFC 134a. Blood samples were collected 22 h later and examined for anti-cytochrome P450 2E1 titers. This study was conducted to determine if exposure to fluorocarbons could induce an autoimmune response. No increases in anti-CYP2E1 was seen (Gunnare et al. Citation2005).
Standards, regulations, or guidelines of exposure
The American Industrial Hygiene Association’s WEEL Committee recommends 1000 ppm as an 8 h TWA for HFC 134a (WEEL, 134a). Also, the German MAK Commission and English Health and Safety Executive have adopted 1000 ppm as an 8 h TWA exposure limit (MAK Citation1996; Standing et al. Citation1995).
The National Advisory Committee for Acute Exposure Guidance Levels has developed the following proposed guidance levels for single, rare incidents, for exposures lasting ≤8 h (AEGL 2000).
1,1,1-Trifluoroethane CAS # 420–46-2; Mol. Wt. 84.05, C2H3F3)
Common synonyms include HFC-143a, Fluorocarbon 143a and refrigerant 143a. Its vapor density is 3.1; solubility in water is 0.761 g/L and log Pow is 1.74. It is flammable and odorless with poor warning properties (ECETOC Citation2006).
Production and use
HFC 143a is used as a refrigerant and as a component in some refrigerant blends. (ECETOC Citation2006).
Exposure assessment
When released to the environment, it is expected to partition almost exclusively into the air. The atmospheric degradation occurs via indirect photolysis by hydroxyl radicals with the formation of trifluoroacetaldehyde as a stable intermediate. This will subsequently be degraded to HF or fluoride and carbon dioxide (ECETOC Citation2006)
Toxic effects
Acute Toxicity
When rats were exposed snout-only to levels up to 540,000 ppm for 4 h, with oxygen added to maintain oxygen levels at 20%, there was no mortality. Reversible body weight loss was noted on the days following the exposures (Brock et al. Citation1996).
In a second study, Sprague–Dawley rats were exposed nose-only to levels up to 591,000 ppm for 4 h, again oxygen was added. There was no mortality. A slight, reversible body weight loss was reported (ECETOC Citation2006).
Cardiac Sensitization
The potential of HFC 143a to sensitize the heart to epinephrine (adrenaline) was investigated using beagle dogs. Exposures were conducted for 5 min at which time the dogs were given an injection of adrenaline just below the threshold required to produce a spontaneous arrhythmia. Exposures were continued for another 5 min. The NOEL was 250,000 ppm, whereas 300,000 ppm was the threshold for this effect (Brock et al. Citation1996).
Subchronic and chronic toxicity
Exposure of 10 male and 10 female rats nose-only to 40,000 ppm of HFC 143a 6 h/day, 5 days/week for 4 weeks resulted in effects in the sperm in all groups but no other signs of toxicity. It was considered that the testicular effect may have been related to heat stress from the nose-only exposure system. This study was repeated using male rats in a whole-body exposure system. No effects were seen and specifically the testes were normal in all groups. Groups of 20 male and 20 female rats were exposed to 40,000 ppm of HFC 143a 6 h/day 5 days/week for 3 months, no adverse clinical signs were seen. Histopathological examination did not reveal any adverse effects (Brock et al. Citation1996).
Groups of 36 male and 36 female rats were given daily oral doses of 300 mg/kg body weight of HFC 143a in corn oil for 52 weeks and held for an additional 73 weeks. There were no compound related neoplastic or non-neoplastic lesions. Despite the methodological limitations, the study did show a clear positive response for two other materials included in this screening evaluation (HCFC 31 and HCFC 133a) and the absence of an effect with HFC 143a is a reasonable indication of a lack of both chronic toxicity and carcinogenic potential (Longstaff et al. Citation1984).
Pharmacokinetics, metabolism, and mechanisms
The metabolism of HFC 143a was studied in groups of three male Crl:CD BR rats. Exposures were conducted at levels of 100, 390, 1040, 2050, and 4800 ppm for from 4 to 5 h. This data was used to develop a PB/PK model for uptake and distribution of HFC 143a. Additionally a 4 h exposure was conducted at 40,000 ppm to identify metabolites in the urine. The study showed that HFC 143a is poorly adsorbed into the body and rapidly eliminated. The major metabolite was trifluoroethanol. Minor amounts of trifluoroacetic acid and trifluoroacetaldehyde were also detected (Keller Citation1994).
Reproductive and developmental effects
No reproductive toxicity studies have been conducted with HFC 143a. However, no effects were seen in a two-generation reproductive study with 1,1,1,2-tetrafluoroethane (Alexander et al. Citation1995). While effects were seen in the sperm in the first 28 day study with HFC 143a, this effect was neither seen in the second 28 day study or in the 90 day study. Therefore the weight of evidence demonstrates that HFC-143a does not impart adverse effects on male spermatogenesis and these effects are not considered to be related to the exposure to HFC 143a.
Developmental toxicity studies were conducted with rats (25/group) and rabbits (24/group). Exposure levels in both studies were 2000, 10,000 and 40,000 ppm. Exposures were 6 h/day, 7 days/week on gestation days 7–16 (rats) or 6–18 (rabbits). No indication of developmental toxicity was seen in either study (Brock et al. Citation1996).
Carcinogenesis
Rats were given 300 mg/kg body weight of HFC 143a in corn oil 5 days/week for 52 weeks and held for a total of 125 weeks (Longstaff et al. Citation1984). There was no evidence for carcinogenicity.
Genetic and related cellular effects studies
Although HFC 143a gave a positive response in an early Ames assay (Longstaff et al. Citation1984), this response was not replicated in either of two subsequent studies (WEEL Citation1994). It was also not active in two E. coli assays, a chromosome aberration assay with human lymphocytes and a mouse micronucleus assay (Brock et al. Citation1996).
Human experience
Clinical cases
Nine male human volunteers were exposed to 500 ppm of HFC 143a for 2 h during light exercise. Blood, urine, and exhaled air were collected before, during, and up to 2 days post-exposure. The study results showed a low metabolic rate, low solubility in blood, rapid uptake and rapid elimination (two-compartment half-life 4 and 300 min) (Gunnare et al. Citation2003).
A clinical study was conducted where nine healthy workers were exposed to 500 ppm of HFC 143a. Blood samples were collected 22 h later and examined for anti-cytochrome P450 2E1 titers. This study was conducted to determine if exposure to fluorocarbons could induce an autoimmune response. No increases in anti-CYP2E1 were seen (Gunnare et al. Citation2005).
Standards, Regulations, or Guidelines of Exposure
The American Industrial Hygiene Association’s WEEL Committee recommends 1000 ppm (2700 mg/m3) as an 8 h TWA exposure limit for HFC 143a (WEEL Citation1996a).
Studies on environmental impact
Atmospheric Lifetime
The atmospheric degradation of HFC 143a will occur initially in the troposphere, initiated by attack by naturally occurring hydroxyl radicals. The overall lifetime is estimated to be 47.1 years (Myhre et al. 2013).
Global Warming Potential
On the basis of an atmospheric lifetime of 47.1 years, the GWP of HFC 143a is estimated to be 4800 (Myhre et al. 2013; WMO Citation1991a; Schmoltner et al. Citation1993).
1,1-Difluoroethane (CAS# 75–37-6; mol wt 66.05; C2H4F2)
Common synonyms include HFC 152a, R-152a, ethylidene difluoride, difluoroethane, Fluorocarbon 152a. Its vapor density is 2.4; solubility in water is 2.8 g/L; and logPow is 0.75. Its limits of flammability are 3.9–16.9% by volume and it is odorless, with poor warning properties. (WEEL Citation2005; WMO Citation2007). It is important to remember that the review below describes HFC-152a NOT HFC-152. As noted in the metabolism section HFC-152a is metabolized to acetate and therefore is not very toxic. In contrast, HFC-152 is metabolized to monofluoroacetate, a Krebs Cycle inhibitor and highly toxic (Keller et al. Citation1996).
Production and use
HFC 152a is a specialty liquified hydrofluorocarbon gas. It is used as an intermediate in the production of fluorinated polymers, as an aerosol propellant in some specialty applications, and as a component in some refrigerant blends (WEEL Citation2018).
Acute toxicity
In rats, the 4 h inhalation approximate lethal concentration for HFC 152a is 383,000 ppm (v/v) (Moore Citation1975) whereas the 3 min ALC is >500,000 ppm (Lester et al. 1950). In mice the 2 h LC50 is 369,000 ppm (Izmerov et al. Citation1982). When HFC 152a was administered orally, in corn oil, the lethal dose was >1500 mg/kg body weight. There is no published information on dermal or occular toxicity.
Cardiac sensitization
The potential of HFC 152a to sensitize the heart to epinephrine (adrenaline) was investigated using beagle dogs (Mullin et al. Citation1972). Exposures were conducted for 5 min at which time the dogs were given an injection of adrenaline just below the threshold required to produce a spontaneous arrhythmia. Exposures were continued for another 5 min. The NOEL was 50,000 ppm, whereas 150,000 ppm produced an effect in 3 of 12 dogs.
Subchronic and chronic toxicity
Exposure of 10 rats to 100,000 ppm of HFC 152a 6 h/day, 5 days/week for 2 weeks did not produce any signs of toxicity. During the exposure, a slight CNS depression may have been observed. When a group of eight rats were exposed to 100,000 ppm of HFC 152a 16 h/day for 2 months, no adverse clinical signs were seen. Histopathological examination of the lungs revealed mild, diffuse infiltration of small and large round cells in the lung, suggesting mild irritation (Lester et al. 1950).
Groups of rats were exposed by inhalation to 0 (control), 2000, 5000, and 25,000 ppm of HFC 152a 6 h/day, 5 days/week for 2 years. Overall, there were no significant signs of toxicity (McAlack and Schneider Citation1982).
Pharmacokinetics, metabolism, and mechanisms
No specific studies on these endpoints were found. However, in the chronic study, an increase in urinary fluoride levels was seen in the groups exposed to 5000 and 25,000 ppm, suggesting that some metabolism does occur (McAlack and Schneider Citation1982). Based on its structure, HFC-152a will be metabolized to acetate and would not be expected to be very toxic (Keller et al. Citation1996).
Reproductive and developmental effects
Groups of 27 pregnant rats were exposed to levels of ≤50,000 ppm of HFC 152a 6 h/day on gestation days 6–15. There were no effects on fetal development. There were no teratogenic effects and there was no evidence of maternal toxicity. No reports or publications on reproductive toxicity were found. However, the absence of any effects on reproductive organs in the chronic study and the lack of effects in the developmental study described above, indicate that it is unlikely that exposure to HFC 152a would cause reproductive effects (Culik and Kelly Citation1980).
Carcinogenesis
There was no evidence of carcinogenic response in the 2 year inhalation study described above (McAlack and Schneider Citation1982).
Genetic and related cellular effects studies
HFC 152a was not active in a reverse-mutation assay with S. typhimurium either with or without metabolic activation (Longstaff et al. Citation1984). Although a positive response was seen in an early sex-linked recessive lethal study in Drosophila melanogaster, this study appears flawed in that all chemicals including oxygen gave a positive response (Foltz and Fuerst Citation1974; Garrett and Fuerst Citation1974).
Neurological, pulmonary, skin sensitization
It has been reported that HFC 152a has good anesthetic properties when administered at a level of 50% (Van Poznak and Artusio Citation1960).
Human experience
Several volunteers were exposed to 500,000 ppm of HFC 152a for several minutes. Good analgesia and an impending loss of consciousness were reported (Van Poznak and Artusio Citation1960). There have been several reports of injury (Kuspis and Krenzelok Citation1999; Moreno and Beierle Citation2007; Kumar et al. Citation2016) and death (Broussard et al. Citation1997; Xiong et al. Citation2004; Avella et al. Citation2006; Hahn et al. Citation2006) associated with intentional abusive inhalation of HFC 152a. While uncommon, there was a case report by Kumar et al. described an individual who had been abusively inhaling HFC-152 regularly for two years and then almost continuously for two days and developed cardiomyopathy and several other signs of systemic toxicity. While these findings are noteworthy, there are no descriptions of his past history of medical problems and no discussion of other abusive drugs he may have used. Therefore a direct causal relationship has not been established. More common, individuals briefly inhale high levels of the propellant to induce a feeling of being high and, in a few situations, develop a cardiac arrhythmia which can be fatal. Typically, tissue injury results from frost bite and associated tissue destruction. Occasionally, the burns have been described as resulting directly from exposure to the chemical or a proposed breakdown product such as hydrogen fluoride. However, given the stability of this class of chemicals, direct irritation or chemical breakdown under normal conditions is extremely unlikely.
Standards, regulations, or guidelines of exposure
The American Industrial Hygiene Association’s WEEL Committee recommends 1000 ppm (2700 mg/m3) as an 8 h TWA exposure limit for HFC 152a (WEEL Citation1994a). The AIHA’s ERP committee has developed emergency guideline levels for exposures lasting up to 1 h (ERPG Citation2018).
1,1,1,2,3,3,3-Heptafluoropropane (CAS# 431–89-0; mol wt 170.03; C3HF7)
Common synonyms include HFC 227ea, R-227ea, HFA 227ea, and 2H-heptafluoropropane. It has a vapor density of 4.2; its solubility in water is 0.108 gm/L and its log Pow is 2.51. It is nonflammable and has a light ethereal odor with poor warning properties.
Production and use
HFC 227ea was developed as a CFC replacement in refrigerants, high temperature heat pumps, air-conditioning systems; as a fire suppressant; and for pharmaceutical aerosols and metered-dose inhalers.
Air
There is no natural source for HFC 227ea. On the basis of its physical properties, when released into the environment, it is expected to partition almost exclusively into the air.
Acute toxicity
A 4 h exposure of rats to levels of 110,000 and 800,000 ppm of HFC 227ea did not cause mortality (Skaggs et al. Citation1995).
Cardiac sensitization
The potential of HFC 227ea to sensitize the heart to epinephrine (adrenaline) was investigated using beagle dogs (Skaggs et al. Citation1995). Exposures were conducted for 5 min at which time the dogs were given an injection of adrenaline just below the threshold required to produce a spontaneous arrhythmia. Exposures were continued for another 5 min. The NOEL was 90,000 ppm, whereas 105,000 ppm produced an effect while the median effect level was 140,000 ppm.
Subchronic and chronic toxicity
Exposure of Wistar rats to levels of ≤50,000 ppm of HFC 227ea, for 6 h/day, 5 days/week for 4 weeks did not result in any observable signs of toxicity. It was also reported that exposure of rats to a level of ≤105,000 ppm did not cause adverse effects (Skaggs et al. Citation1995).
Pharmacokinetics, metabolism, and mechanisms
The biotransformation of HFC 227ea was investigated in vivo and in vitro in rat and human liver microsomes. In the urine of rats exposed to 5000 ppm, very small amounts of hexafluoroacetone trihydrate were identified, while total urinary fluoride levels were not significantly different from those of controls. In the in vitro studies, only very low levels of hexafluoroacetone and fluoride were detected. No covalent binding of HFC 227ea adducts to liver proteins was seen (Koster et al. Citation1996).
Reproductive and developmental effects
A developmental study was conducted in which pregnant rats were exposed to levels of ≤105,000 ppm 6 h/day on gestation days 6–15. (Oxygen supplementation to maintain oxygen levels at 20% was used for the 105,000 ppm exposure level.) There was no evidence for systemic effects on the dams or for embryotoxic, developmental, or teratogenic effects on the pups (Nemec Citation1994).
Genetic and related cellular effects studies
In Vitro Studies
The mutagenic potential of HFC 227ea was evaluated in a reverse-mutation assay using S. typhimurium strains TA98, TA100, TA1535, TA1537, and TA1538. HFC 227ea was inactive in both the presence and absence of S9 metabolic activation. It was also inactive in a forward mutation assay using mouse lymphoma cells (Dodd et al. Citation1997).
In Vivo Studies
It was inactive in an in vivo mouse bone marrow micronucleus assay that utilized exposure levels of ≤105,000 ppm (Dodd et al. Citation1997).
Human experience
General Information
In addition to the unavailable clinical toxicity data developed to support the use of HFC 227ea as a propellant in metered-dose inhalers, two additional volunteer studies have been conducted. The first study was designed to provide information on blood levels of HFC 227ea found during a 30 min exposure to a level of 6000 ppm. This study was not completed because of observations of increased pulse rate during the exposure (Vinegar et al. Citation2000). In the second study, a group of four male and four female volunteers were exposed sequentially for 1 h to levels of 1000–8000 ppm. No changes were seen in pulse, blood pressure, or cardiac rhythm. There were no adverse clinical signs (Emmens Citation1999b).
Standards, Regulations, or Guidelines of Exposure
There are no legislated occupational or environmental exposure level guidelines regarding HFC 227ea. The WEEL committee of the American Industrial Hygiene Association has established a recommended exposure level for HFC-227ea of 1000 ppm as an 8-h TWA (WEEL, 2000)
1,1,1,3,3,3-Hexafluoropropane (CAS# 690–39-1; mol wt 152.04; C3H2F6)
Common synonyms include HFC 236fa, R-236fa. Vapor density is 6.430; water solubility is 0.32 gm/L. The log Pow is 2.65. It is nonflammable and odorless, with poor warning properties (WEEL Citation1998).
Production and use
HFC 236fa is a CFC replacement for use as a refrigerant and fire-extinguishing agent (WEEL Citation1998).
Acute toxicity
It was reported that the approximate lethal concentration for a 4 h exposure with the rat was >189,000 ppm, a level that produced slight CNS depression. No effects were seen in this study at 134,000 ppm (Valentine et al. Citation1996). In a second study, the 4 h LC50 in the rat was >457,000 ppm. Hyperactivity followed by prostration was noted during the exposure. On necropsy, the lungs had a dark red color (WIL Citation1996). There were no data available on dermal or ocular toxicity.
Cardiac sensitization
Six dogs were exposed for 10 min to HFC 236fa at exposure levels of 50,000, 100,000, 150,000, 200,000, or 250,000 ppm. Five minutes after initiation of the exposure, an injection of adrenaline (epinephrine) was given, along with an additional 5 min. of exposure. A positive response was the observation of multiple ectopic beats or ventricular fibrillation following the adrenaline injection. No response was seen at 50,000 or 100,000 ppm. At 150,000 ppm two of six dogs showed a positive response and at 200,000 and 250,000 ppm, three of six dogs showed a positive response. The threshold for a cardiac arrhythmia was therefore 150,000 ppm (Brock Citation2002).
Subchronic and chronic toxicity
In a 2 week inhalation toxicity study, rats were exposed 6 h/day, 5 days/week to levels of ≤50,000 ppm. With the exception of a reversible reduced startle reflex observed during exposure at 20,000 and 50,000 ppm, but not at 5000 ppm, no adverse effects were seen (Brock Citation2002).
In a 90 day inhalation study, groups of male and female rats were exposed to the same levels for the same time periods as above. Again, the only effect seen was a reduced startle reflex at 50,000 ppm but not at 20,000 ppm or lower. The animals’ degree of alertness returned to normal almost immediately after each exposure. There are no data available on the chronic toxicity (Brock Citation2002).
Pharmacokinetics, metabolism, and mechanisms
When rats were exposed to levels of ≤20,000 ppm of HFC 236fa, fluorine NMR (nuclear magnetic resonance) of the exhaled air indicated that it was readily absorbed. Analysis of blood, urine, and feces failed to detect any metabolites. The HFC 236fa was eliminated unchanged (Vinegar and Jepson Citation1995).
Reproductive and developmental effects
Both rat and rabbit developmental toxicity studies have been conducted with HFC 236fa and HFC 236ea using daily 6 h exposure levels of ≤50,000 ppm. In the study with rats, the exposures were conducted on gestation days 6–15. Although maternal body weight gain was lower at both 20,000 and 50,000 ppm, but not at 5000 ppm, there were no developmental effects. In the study with rabbits, exposures were conducted on gestation days 7–19. There were no maternal or developmental effects at any level (DuPont 1995, 1996; Brock Citation2002).
Genetic and related cellular effects
HFC 236fa was not active in a reverse-mutation assay using S. typhimurium strains TA100, TA97, and TA1535 as well as E. coli strain WP2 uvrA either with or without metabolic activation. It was also inactive in an in vitro chromosome aberration assay with human lymphocytes with vapor exposure levels of ≤100%. It was inactive in an in vivo mouse micronucleus assay with exposure levels of ≤50,000 ppm, 6 h/day for 2 days (Brock Citation2002).
Human experience
There are no reports of human exposure to HFC 236fa.
Standards, regulations, or guidelines of exposure
The American Industrial Hygiene Association’s WEEL Committee recommends 1000 ppm (6300 mg/m3) as an 8 h TWA for HFC 236fa (WEEL Citation1998).
Studies on environmental impact
HFC 236fa was developed as a CFC replacement since it is a non-ozone-depleting substance.
1,1,2,3,3-Pentafluoropropane (CAS# 24270–66-4; mol wt 134.05; C3H3F5)
The only common synonym is HFC-245ea. The vapor density, water solubility and logPow were not available. It is nonflammable and has poor warning properties.
Production and use
HFC 245ea is being produced in low levels as a foam-blowing agent.
Exposure assessment
There are no reports of human exposure.
Acute toxicity
Inhalation exposure of mice to vapors of HFC 245ea for 4 h did not cause any lethality. No adverse reactions were seen when 2.0 ml/kg of the liquid was applied to the backs of New Zealand white rabbits (AlliedSignal Citation1997a).
Cardiac sensitization
As with many other fluorocarbons, inhalation of HFC 245ea coupled with an intravenous injection of adrenaline to simulate stress can induce a cardiac sensitization response in dogs. The threshold concentration for this effect was 20,000 ppm, and the no-observed-effect concentration (NOEC) was 10,000 ppm (AlliedSignal Citation1997b).
Genetic and related cellular effects studies
In vitro
HFC 245ea was not active in a reverse-mutation assay with S. typhimurium strains TA98, TA100, TA1535, TA1537, and TA1538 and E. coli strain WP2 uvrA (392). It did, however, show limited activity in a chromosome aberration study with human lymphocytes at gas-phase exposure levels of 50,000–100,000 ppm without metabolic activation and 60,000 ppm with metabolic activation (AlliedSignal Citation1997c, Citation1997d).
In vivo
HFC 245ea was not active in a mouse bone marrow micronucleus assay where the mice were exposed to a level of 31,800 ppm for 4 h (AlliedSignal Citation1997e).
1,1,1,3,3-Pentafluoropropane (CAS# 460–73-1; mol wt 134.05; C3H3F6)
Common synonyms are HFC 245fa, R-245fa. Vapor density is 4.6; the solubility in water is 7.18 g/L and the log Pow is 1.35. It is nonflammable and has a light ethereal odor, with poor warning properties (Rusch et al. Citation1999).
Production and use
HFC 245fa was developed as a replacement for the fully halogenated chlorofluorocarbons as a foam-blowing agent and refrigerant.
Exposure assessment
There are no reports of human accidental exposures to HFC-245fa and there have been only minimal environmental releases (Rusch et al. Citation1999).
Air
As it is a low boiling liquid (boiling point 15.3 °C) with a relatively low water solubility (7.18 g/L at 25 °C), it will partition almost entirely into the air (Rusch et al. Citation1999).
Acute toxicity
There was no mortality when rats and mice were given 4 h exposures to levels of 203,000 ppm or 101,000 ppm, respectively. There was also no significant sign of irritation when 2 ml of HFC 245fa was applied to the backs of albino rabbits, and it did not cause dermal sensitization when applied to the skin of guinea pigs (Rusch et al. Citation1999).
Cardiac sensitization
The potential of HFC 245fa to sensitize the heart to adrenaline was investigated in a group of six beagle dogs (Rusch et al. Citation1999). Each dog was exposed at the target level to HFC 245fa for 5 min, then given an injection of adrenaline and observed with continuing exposure for an additional 5 min. An exposure of 73,000 ppm caused a fatal ventricular fibrillation. At 44,000 ppm a second dog responded, but no dogs responded at 34,100 and 54,100. These results indicate that, the threshold for a response was 44,000 ppm and the NOEL was 34,100 ppm for the development of a cardiac arrhythmia in the presence of an injected adrenaline.
Subchronic and chronic toxicity
A series of three inhalation toxicity studies were conducted in the rat. All involved daily 6 h exposures of ≤50,000 ppm. The first study involved 14 consecutive snout-only exposures. There were no treatment-related effects on body weight, survival, or histological parameters. Blood urea nitrogen (BUN), glutamic pyruvic transaminase (GPT), and glutamic oxaloacetic transaminase (GOT) levels frequently were elevated compared to controls; however, cholesterol levels tended to be lower. The second study involved 28 consecutive whole-body exposures. Again there were no treatment-related effects on body weight, survival, or histological parameters. Urine volume was increased. Increases were seen in BUN levels and alkaline phosphatase (AP), GPT, GOT, and CPK activities, primarily in rats exposed at 10,000 and 50,000 ppm. Urinary fluoride levels were also elevated in an exposure-related pattern. In the third study, whole-body exposures were conducted 6 h/day 5 days/week for 13 weeks. There were no treatment-related effects on survival, clinical observations, body weight gain, or food consumption. Urine volumes were increased, urinary fluoride levels were elevated, and increases were seen in AP, GOT, GPT, and CPK activities. These effects were seen in the 10,000 and 50,000 ppm exposure level groups. Histopathological examination did not show any effects on the kidney, liver, or lungs. There was an increased incidence of myocarditis in all animals exposed at 50,000 ppm and the majority exposed at 10,000 ppm. It was described as mild. On the basis of these findings, 2000 ppm appears to be the no-observed-adverse-effect level (NOAEL) (Rusch et al. Citation1999). This finding had not been previously reported, but a similar observation was seen with some hydrofluoro propenes. Even at high exposure levels (50,000 ppm) it did not induce systemic effects.
Following these studies, a limited, snout-only exposure study was conducted in the dog. Groups of two dogs were exposed to levels of 0 (control), 1000, or 10,000 ppm of HFC 245fa for 6 h/day, 5 days/week for 2 weeks. On the day following the final exposure, each dog was evaluated in a cardiac sensitization study involving exposure to 35,000 ppm of HFC 245fa and a challenge injection of adrenaline. There were no adverse responses nor histopathological changes in the heart (AlliedSignal Citation1998).
Pharmacokinetics, metabolism, and mechanisms
The metabolism of HFC 245fa was studied in the rat, rat liver microsomes and human liver microsomes. Biotransformation was by cytochrome P450 2E1. The major metabolites were trifluoroacetic acid and inorganic fluoride, minor metabolites were 3,3,3-trifluoropropanoic acid and 1,1,1,3,3-trifluoropropane-2-ol (Bayer et al. Citation2002).
Five male and five female healthy volunteers were exposed to air (control), 100 and 300 ppm of HFC 245fa, in random order. Equilibrium between the blood and air was reached within minutes. At the end of the exposure, clearance was also complete within a few minutes. An analysis of the urine for the proposed metabolites, ionic fluoride, 3,3,3-trifluoropropanoic acid (TFPA) and trifluoroacetic acid (TFA) was conducted. None of these metabolites were identified in the urine at levels above background, suggesting that metabolism of HFC 245fa, in humans, following exposure at 100 and 300 ppm was minimal. PBPK simulations suggested that a maximum of 2.1% of the inhaled HFC 245fa was retained, however, only 0.7% was exhaled unchanged. No clinical or physiological effects were attributed to the exposures (Ernstgård et al. Citation2010).
Reproductive and developmental effects
In an inhalation developmental toxicity study, rats were exposed to levels of 0 (control), 500, 2000, 10,000, and 50,000 ppm of HFC 245fa 6 h/day on gestation days 6–15. A slight reduction in pup weight was seen at 50,000 ppm, but not at 10,000 ppm. There were no developmental effects at any level (Rusch et al. Citation1999).
A two-generation reproduction toxicity study was conducted in rats. The exposure levels were 0, 500, 2000, 10,000 and 50,000 ppm, based on the results of the 13 week study. All typical reproductive parameters were judged to be normal. However, there was an unexpected mortality of lactating dams in the medium and high dose group beginning with day 10 of lactation. Histopathology revealed that the brain, particularly the cerebellum, and to a minor degree, the heart, turned out to be the toxicological target tissues of the substance. Statistically significant histopathological alterations were observed in the cerebellum of a total of 9/30 females of the high dose group of the F0-generation and in 10/27 females of the high dose group of the F1-generation. There were no changes in the brain found in males or non-pregnant females of all dose groups.
The increased incidence of myocardial fibrosis and mononuclear cell infiltration in the males—indicating a cardiomyopathy—was only seen in the F0-generation. Only the females of the F1-generation showed a statistically significant increase of minimal myocardial fibrosis. Serum enzyme measurements provided no evidence for acute myocardial damage.
The reason for the observed maternal mortality is possibly an indirect interaction of the compound and the stress associated with lactation. The authors based this comment on the observations: 1. During the latter part of the gestation period, the dams are under stress to produce adequate milk; 2. There was no evidence of this type of systemic toxicity in males or non-lactating females; and histopathological examination of these does did not reveal direct evidence for a cause of death (Buschmann et al. Citation2013).
Genetic and related cellular effects studies
In vitro
HCF 245fa was not active in a reverse-mutation assay using S. typhimurium tester strains TA1535, TA1537, TA98, and TA100 and E. coli WP2P uvrA. The cultures were exposed to atmospheric levels of ≤100% with and without S9 metabolic activation. The clastogenic activity of HFC 245fa was evaluated with human lymphocytes. Some activity was seen when the cultures were exposed to atmospheric levels of ≤30% for 24 h without metabolic activation. No activity was seen with either shorter contact times or lower exposure levels. No activity was seen in the presence of metabolic activation even with 6 h exposure of 70% (Rusch et al. Citation1999).
In vivo
HFC 245fa was inactive in a mouse micronucleus assay that involved a 4 h exposure to a level of 101,000 ppm (Rusch et al. Citation1999).
Human experience
No adverse effects were seen in a group of human volunteers exposed to 300 ppm for 2 h. Also analysis of blood and serum collected following the exposures did not show any measurable levels of metabolites (Ernstgård et al. Citation2010).
Standards, regulations, or guidelines of exposure
The American Industrial Hygiene Association’s WEEL Committee recommends 300 ppm as an 8 h TWA (WEEL Citation2009).
Studies on environmental impact
Since HFC 245fa does not contain bromine or chlorine, it has a 0 ODP. Its atmospheric lifetime is 8.4 years. Its GWP is 790 relative to a value of 1 for carbon dioxide with an integrated time horizon of 100 years.
Hydrofluoroolefins (HFOs) and hydrochlorofluoroolfins (HCFOs)
Following development of the hydrofluorocarbons, there was regulatory concern that the global warming potentials of these materials were still an environmental issue. The candidates such as carbon dioxide recommended by some regulatory authorities would have created more problems than they would have solved. In mobile air conditioning, replacement of current refrigerants, due to concerns regarding leakages from the system, with carbon dioxide, would have resulted in using a substance with an even higher pressure and greater potential for leakage. In other cases, such as domestic refrigeration, replacement of current refrigerants and foam blowing agents would result in using substances with lower efficiencies and higher total global warming (UNEP Citation2014). Industry identified new materials, the HFOs and HCFOs as more efficient substitutes with very low global warming potentials. 2,3,3,3-tetrafluoropropene (HFO-1234yf), trans-1,3,3,3-tetrafluoropropene (HFO-1234ze(E) and trans-1-chloro-3,3,3-trifluoropropene (HCFO-1233zd(E) are the only three chemicals for which there is published toxicology information.
2,3,3,3-tetrafluoropropene (HFO-1234yf) (CAS# 754–12-1; Mol. Wt. 114; C3F4H2)
Common synonyms include HFO-1234yf and R1234yf. It has a vapor density of 4, its solubility in water is 0.1982 g/L and its log Pow is 2.15. It is flammable and has poor warning properties (ERPG 2012).
Production and use
HFO-1234yf is a low GWP refrigerant (<1) for use in automobile air conditioners. It is also used in blends in stationary air conditioners (Hodneborog et al. 2013).
Air exposure assessment
It has a tropospheric life time of only 11 days and therefore is not transported to the stratosphere. Environmental breakdown occurs via attack on the double bond by atmospheric hydroxyl radicles (Nielsen et al. Citation2007; Hodnebrog et al. Citation2013).
Acute toxicity
A 4-h acute inhalation screening study was conducted with 2 male and 2 female CD-1 mice at an exposure level of 99,830 ppm. Post-exposure observation time was 7 days. There was no mortality and no clinical signs of toxicity. Gross necropsy observations did not show any abnormal findings (ERPG Citation2012a).
In a second study, two groups of 5 male and 5 female Sprague Dawley rats were exposed nose only to target levels of either 201,600 ppm or 405,800 ppm for 4 h (oxygen added). The animals were held for a 14-day observation period. There were no clinical signs of toxicity except for decreased breathing rates reported for 2 males and 2 females at 201,600 ppm and all animals exposed to 405,800 ppm. Body weight gain was normal. There was no mortality and gross necropsy observations were normal (Tveit et al. Citation2013a).
In a subsequent study designed to look for possible toxicological effects, groups of 5 male, 5 female and 6 pregnant New Zealand White rabbits were exposed to HFO-1234yf at levels of 0 (control), 50,000 and 100,000 ppm for 1 h. The rabbits were subsequently held for a 14-day observation period. There were no deaths or clinical signs of toxicity. A histopathological analysis was performed on the heart, liver, lungs and kidneys from the control and high exposure level rabbits. Body weight gain was normal and no gross or microscopic treatment related abnormalities were seen. Exposure of non-pregnant females at 50,000 ppm was not included in this study as data exists for them at this level (Schmidt et al. Citation2012).
In a study to evaluate cardiac sensitization potential, a group of 6 beagle dogs were exposed to levels of 20,000, 40,000 or 120,000 ppm of HFO-1234yf. A total of three exposures were conducted, with at least a 2-day recovery period between exposures. Initially a determination was made in each dog for the maximum level of epinephrine that would not cause a cardiac arrhythmia (each dog served as its own control). On the test material exposure day, animals were administered a pre-exposure dose of epinephrine as a bolus injection approximately 5 min prior to exposure to the test material. The dogs were then exposed to the test compound for a total of 10 min. After the first five minutes of exposure, each dog received a challenge-dose injection of epinephrine at the pre-determined maximum sub-arrhythmia dose. During the next five minutes of exposure, the dogs were monitored for the development of a cardiac arrhythmia. There were no arrhythmias, nor other signs of toxicity seen in any of these dogs. Thus it was concluded that the No-Effect-Level (NOEL) for HFO 1234yf was 120,000 ppm. HFO-1234yf did not cause cardiac sensitization to epinephrine under the conditions of this study (Tveit et al. Citation2013a).
Subchronic and chronic toxicity
Groups of 5 male and 5 female Sprague Dawley rats were exposed, nose only to vapors of HFO-1234yf at levels of 0 (control), 5000, 20,000 or 50,000 ppm 6-h/day, 5 days/week for 2 weeks. The No-Observed-Adverse-Effect-Level (NOAEL) for a 2-week exposure to HFO-1234yf was at least 50,000 ppm. Groups of 10 male and 10 female Sprague Dawley rats were exposed, nose only to vapors of HFO-1234yf at levels of 0 (control), 5000, 20,000 or 50,000 ppm 6-h/day, 5 days/week for 4 weeks.
Five males and 5 females were used for the basic 4-week study, an additional 5 males and 5 females were used in the control and high-level exposure groups for the 2-week recovery period and additional male rats were used at all exposure levels for a unscheduled DNA synthesis study. The NOAEL for a 4-week exposure to HFO-1234yf was at least 50,000 ppm (Tveit et al. Citation2013a).
In another study, groups of ten male and female Sprague Dawley rats were exposed, nose-only, to HFO-1234yf at levels of 0 (control), 5000, 15,000 or 50,000 ppm for 6 h/day, 5 days/week during a 14-week period. The NOAEL for a 14-week exposure to HFO-1234yf was at least 50,000 ppm (Tveit et al. Citation2013a).
A 28-day whole body inhalation toxicity study was conducted with New Zealand white rabbits . The study was divided into two Phases. In the first Phase, 5/sex/group were exposed to levels of 0 (control), 500, 1000, and 5500/4500 ppm, 6-h/day, for 6 or 13 days. In Phase 1,five rabbits/sex were scheduled to be euthanized at the end of both the 6 and 13 day exposure periods. One male and 1 female rabbit in the 5500 ppm exposure level group died prior to sacrifice. In Phase 2, 15 rabbits/sex/group were exposed to levels of 0(control), 500, 1000, or 4500 ppm, 6 h/day for 28 consecutive days. One female in the 4500 exposure level group died prior to sacrifice. Five/sex were held for a 28 day post exposure observation period, the remainder were sacrificed on the day following the final exposure. Other than the death of the 3 rabbits, no gross abnormalities were observed. Microscopic examination revealed myocardial inflammation at all exposure levels with males being more effected than females and with the number of rabbits effected increasing with increasing exposure level. The lesions were minimal to mild and did not progress over time. Complete recovery was seen in the 28 day recovery group. Also, an increased incidence of skeletal muscle necrosis was seen. However, the acute nature of the change was inconsistent with the duration of exposure suggesting that the change was not a direct consequence of the exposure to HCFO-1234yf. The direct cause of death was not established. Based on mortality, exposures in the rabbit were tolerated up to 1500 ppm. The NOEL based in histopathology was 500 ppm in males and 1000 ppm in females. (ASHRAE Citation2017).
The minipig is known by cardiologists to be a much better model for cardiac effects than the rabbit. This is because rabbits have a high prevailing sympathetic tone, whereas humans and pigs have a high prevailing vagal tone. The higher sympathetic tone of rabbits predisposes them to atril and ventricular arrhythmias and limits extrapolation of findings in rabbits to humans. Therefore a whole body inhalation study using Gottingen minipigs was conducted with daily 6 h exposures at levels of 0 (control), 5500 or 10,000 ppm for 14 days (phase 1) or 28 days (phase 2). At the end of both Phase 1 and 2 treatment periods, the minipigs were sacrificed. Microscopic examination was conducted on multiple samples of the heart and skeletal muscle. The no-observed-effect-level in these studies was 10,000 ppm (ASHRAE Citation2017).
Metabolism pharmacokinetics
The blood/air partition coefficient for human, rat and rabbit blood was measured using published methodology. It was determined that the concentration of HFO-1234yf in human blood would be only 50% of the concentration in either rat or rabbit blood under the same exposure conditions. Therefore at equivalent exposure levels the dose delivered to a person would be half of that delivered to a rabbit. Thus, an exposure of 4000 ppm in the rabbit would be equivalent to an exposure of 8,000 ppm in the human. The rabbit and human differences in HFO-1234yf partitioning into blood provide further conservatism (safety) in the use of the rabbit toxicity data (Schuster et al. Citation2010).
Reproductive and developmental effects
Groups of 25 pregnant Sprague Dawley rats were exposed, nose-only, to vapors of HFO-1234yf at levels of 0 (control), 5000, 20,000 or 50,000 ppm for 6-h/day on Days 6–19 of gestation. The authors concluded “no adverse maternal and developmental effects were observed in rats after inhalation for 6 h with HFO-1234yf during Gestation Day 6–19 at a concentration up to 50,000 ppm” (Tveit et al. Citation2013a).
In a pilot rabbit developmental toxicity study, groups of 12 pregnant rabbits were scheduled to be exposed to levels of 0 (control) 2500, 10,000 and 50,000 ppm 6-h/day, daily from Day 6–28 of gestation. By Day 14 of gestation, 9/12 rabbits had died in the 50,000 ppm exposure level group and subsequent exposures at this level were discontinued. By Day 21 of gestation, 5/12 rabbits had died in the 10,000 ppm exposure level group and subsequent exposures at this level were discontinued.
There were no deaths or apparent effects at 2500 ppm. Evaluation of the pups from the surviving does in all exposure groups did not reveal any evidence of developmental toxicity or effects on survival (Tveit et al. Citation2013).
Following completion of the pilot study, a study was conducted using groups of 24 pregnant rabbits exposed to levels of 0 (control), 2500, 4000, 5500 and 7500 ppm. Again the exposures were 6-h/day, from Days 6–28 of gestation. The full series of exposures were conducted on all groups. Six of 24 rabbits died or were sacrificed in extremis in the 7500 ppm exposure level group and 2/24 died in the 5500 ppm exposure level group. There were no deaths in the 4000 or 2500 ppm groups nor in the controls. There were no significant differences in survival of pups or litter size in any treatment group. Skeletal evaluation of all pups from the 4000 ppm exposure level group and ½ of the pups from the control, 2500 ppm and 7500 ppm exposure level groups did not reveal any significant developmental effects in the pups from any exposure group. As the complete evaluation was conducted on the does and pups at 4000 ppm and there were no effects on any parameter in either adults or pups, it can be concluded that the no-observed-effect-level for maternal and pup toxicity is at least 4000 ppm (Tveit et al. Citation2013a). The possible uniqueness of the rabbit model is discussed above in the subchronic section.
A two-generation GLP inhalation reproduction study was also conducted with HFC-1234yf. The study used exposure levels of 0 (control), 5,000, 15,000, and 50,000.ppm 6 h/day 5 days per were prior to mating and 7 days per week during mating, gestation and lactation. No effects on bone ossification or rib development were seen. There was a delay in sexual maturation of a few days in the female rats but not the males and there was an increase in the number of female pups with a corresponding decrease in the number of male pups at 15,000 and 50,000 ppm. A clear NOEL was seen at 5000 ppm (TNO Citation2008).
Carcinogenesis
Gene expression changes were used to assess the carcinogenic potential of HFO-1234yf following exposure of female B6C3F1 mice and male F344 rats to levels of 10,000 or 50,000 ppm for 6 h/day, 5 days/wk for 13 weeks. The assessment was based on a comparison of the responses seen with HFO-1234yf to results from both positive (tetrafluoroethylene, 1-amino-2,4-dibromoanthraquinone, and Tris(2,3-dibromopropyl)phosphate) and negative (trichlorofluoromethane, iodoform, tetrafluoroethane and N-(1-naphthyl)ethylenediamine dihydrochloride) controls. Vehicle controls were also included. In addition, histopathological examination of selected tissues was conducted. No treatment-related histopathological lesions were observed, and statistical classification analysis predicted HFO-1234yf to be non-carcinogenic in both female mouse liver and male rat kidney. In addition lung tissue from the mouse was evaluated for gene expression, however the number of altered genes was to small to permit a meaningful analysis (Hamner Institute for Health Sciences Citation2007).
Genetic studies
An Ames assay was conducted which involved exposure of Salmonella typhimurium (TA 1535, TA1537, TA 98, and TA 100) and E coli (WP2 uvrA) both in the presence and absence of S-9 metabolic activation. Exposure levels of up to 76% (plus 19% O2 and 5% CO2) were used. At exposure levels of 20% and above a two-fold increase in mutations was seen with TA 100 and WP2 uvr2 in the presence of S-9. All other cell lines were inactive (Tveit et al. Citation2013a). Cultured human lymphocytes were exposed to levels up to 76%, both in the presence and absence of S-9 metabolic activation. Under the conditions of this test, HFO-1234yf was not active (not clastogenic) in this assay (Tveit et al. Citation2013a). In a micronucleus study, mice were exposed to levels of up to 200,000 ppm of HFO-1234yf. Bone marrow cells were collected and analyzed for the presence of micronuclei. Under the conditions of this assay HFO 1234yf did not produce chromosomal damage or damage to the mitotic spindle apparatus in bone marrow cells (Tveit et al. Citation2013a).
As an additional component in the 4-week study described earlier where groups of male and female Sprague Dawley rats were exposed, nose-only, to HFO-1234yf at levels of 0 (control), 5000, 20,000 or 50,000 ppm for 6-h/day, 5 days/week for 4 weeks, a micronucleus assay was conducted on these rats and additional male rats were included at all exposure levels for an unscheduled DNA synthesis study. There was no evidence of genotoxicity in either the rat micronucleus or unscheduled DNA synthesis assays (Tveit et al. Citation2013a).
Based on the preceding in vitro and in vivo studies the overall weight-of-evidence suggests that HFO-1234yf is not mutagenic or genotoxic.
Human experience
HFO-1234yf has only recently been introduced into commerce and there is no data on human experience.
Standards, regulations or guidelines of exposure
The TERA WEEL Committee (WEEL Citation2009) has developed a workplace exposure level guideline for HFO-1234yf of 500 ppm as an 8-h TWA. The AIHA ERP Committee (2012) has recommended emergency response exposure guidelines of:
ERPG-1 (annoyance) – NA
ERPG-2 (serious toxicity) – 24,000 ppm
ERPG-3 (potential lethality) – NA
NA indicates that this level is not appropriate. For ERPG-1, it is not an irritant nor does it have an annoying odor. For ERPG-3, single exposures of rats, dogs or rabbits to levels as high as 100,000 ppm have not caused lethality.
Trans-1,3,3,3-tetrafluoropropene (HFO-1234ze(E); CAS#29118–24-9; mol wt 114; C3F4H2)
Common synonyms include HFO-1234ze and R-1234ze(E). It has a vapor density of 4, its solubility in water is 0.373 g/L and its log Pow is 1.6. It is nonflammable and has poor warning properties (Honeywell Citation2011).
Production and use
It was developed as a foam blowing agent and is also used in refrigeration blends.
Air exposure assessment
HFO-1234ze is a low GWP products. It has a GWP of <1.0 and an atmospheric lifetime of less than 10 days. With such a short atmospheric life time, it will not migrate into the stratosphere (Hodnebrog et al. Citation2013).
Acute toxicity
An acute 4-h inhalation toxicity study was conducted with three groups of 5 male and 5 female Sprague-Dawley CD rats that were exposed nose only to HFO-1234ze at levels of 0 (control), 100,000 or 207,000 ppm for 4 h with oxygen added to maintain the levels at 21%. The animals were held for a 14-day observation period. There was no mortality or signs of toxicity. The 4-h LC50 for HFO-1234ze in the rat is greater than 207,000 ppm. Based on these results, HFO-1234ze is considered practically nontoxic by the inhalation route of exposure (Rusch et al. Citation2013).
It is well known that dermal exposure to large amounts of refrigerant gases can cause frost bite from evaporative cooling. Since the substance is a gas, it normally would not be tested for dermal irritation; however certain applications required this testing.
Animals were scored at 1, 24, 48, and 72 h after treatment. There was no evidence of erythema, edema or frost bite. It was concluded that HFO-1234ze is not a dermal irritant (Rusch et al. Citation2013).
Following the rabbit dermal screen, a Human Repeat Insult Patch test was conducted using a panel of 100 subjects. This panel of 100 human subjects was exposed to HFO-1234ze. Due to the low boiling point of this material, special procedures were used to minimize volatilization. HFO-1234ze (0.3 ml) was injected into Hill-Top chambers which were immediately placed on the skin of subjects. Subjects were evaluated for skin irritation at 24 and 48 h following Hill Top chamber removal. No irritation was observed following HFO-1234ze exposure. Following challenge, no adverse responses were observed in subjects exposed to HFO-1234ze. Under the conditions employed in this study, there was no evidence of skin sensitization to HFO-1234ze (TKL Research 2010).
In an acute cardiac sensitization study, a group of 6 beagle dogs were exposed to vapors of HFO-1234ze at concentrations of 2%, 6% or 12% (20,000, 60,000 or 120,000 ppm). Exposures were conducted on different days; with at least a 2-day separation between the exposures. Initially a determination was made for each dog of the maximum level of epinephrine that would not cause a cardiac arrhythmia. The dogs were then exposed to the test substance for a total of 10 min. After the first five minutes of exposure, each dog received an injection of epinephrine at the pre-determined maximum sub-arrhythmia dose. During the next five minutes of exposure, the dogs were monitored for the development of a cardiac arrhythmia. No arrhythmias or other signs of toxicity were induced in any of the dogs. Thus, it was concluded that the No-Observed-Effect-Level (NOEL) for HFO-1234ze was 120,000 ppm. HFO-1234ze did not cause cardiac sensitization to epinephrine under the conditions of this study (Rusch et al. Citation2013).
Subchronic and chronic toxicity
A 2-week inhalation toxicity study was conducted with four groups of 5 male and 5 female Sprague Dawley rats that were exposed nose only to vapors of HFO-1234ze at levels of 0 (control), 5000, 20,000 or 50,000 ppm for 6 h/day, 5 days/week during a 2 week period, with a total of 10 exposure days. Hematologic analysis, clinical chemistry analysis, organ weight measurements, and macroscopic and microscopic examination of the heart, liver and nasal passages revealed treatment-related effects in animals exposed to 20,000 and 50,000 ppm. The main effects were concentration-related and occurred in the heart (muscle fiber vacuolation and mononuclear cell infiltrates) and liver (hepatocellular vacuolation and mononuclear cell infiltrates) of animals exposed to 20,000 and 50,000 ppm and in the nasal passages (decreased goblet cell expression) of animals exposed to 50,000 ppm. Thus 5,000 ppm was considered to be the NOEL for HFO-1234ze in this study (Rusch et al. Citation2013).
In a 4-week inhalation toxicity study, five groups of male and female Sprague Dawley rats were exposed nose only to vapors of HFO-1234ze at levels of 0 (control), 1000, 5000, 10,000 and 15,000 ppm for 6 h/day, 5 days/week during a 4 week period, with a total of 20–21 exposure days. As an additional component of this study, at necropsy, liver cells from male rats in the control, 5000 and 15,000 ppm groups were evaluated in an unscheduled DNA synthesis test and bone marrow from male rats in the control, 5000, 10,000 or 15,000 ppm groups was assessed in a micronucleus test. Results of these two mutagenicity tests are presented below under the heading Mutagenicity. No treatment-related changes were observed when clinical observations, body weight gain, food consumption and food conversion efficiency were evaluated. Although clinical chemistry analysis showed some variations, they did not appear in a concentration-related pattern and thus, they were not considered treatment-related. At necropsy, no treatment-related changes were found during macroscopic examination or in organ weights. Microscopic examination revealed very slight to moderate inflammation of the heart of male rats exposed to 15,000 ppm; two of which also showed muscle fiber vacuolation. Based on these results, 10,000 ppm was considered the No-Observed-Adverse-Effect Level (NOAEL) for a 4-week exposure to HFO-1234ze. While this finding is not common in current fluorocarbon products, it has been seen in other fluorinated propanes and propenes (Rusch et al. Citation2013).
A 13-week inhalation toxicity study was conducted with four groups of 10 male and 10 female Sprague Dawley rats that were exposed to HFO-1234ze at levels of 0 (control), 1,500, 5,000, or 15,000 ppm for 6 h/day, 5 days/week during a 13-week period, with a total number of 63–64 exposure days. No treatment related changes were observed when clinical observations, body weight gain, food consumption and food conversion efficiency were evaluated. Analysis of hematology parameters and clinical chemistry showed some slight variations at 15,000 ppm which may have been treatment-related. At necropsy, no treatment related gross changes were observed during macroscopic examination and no treatment-related organ weight changes were measured. However, microscopic examination revealed multifocal mononuclear cell infiltrates in the heart of both sexes at 15,000 ppm. Fibrosis was not observed. Based on these results, 15,000 ppm was considered a Low-Observed-Adverse-Effect Level (LOAEL) and 5000 ppm was considered. the No-Observed-Effect Level (NOEL) for a 13-week exposure to HFO-1234ze (Rusch et al. Citation2013).
A chronic study was not conducted with HFO-1234ze, but a genomic evaluation following a 13 week exposure was run. The results are described below.
Metabolism and pharmacokinetics
HFO-1234ze underwent minimal metabolism when rats were exposed to 50,000 ppm HFO-1234ze for a single 4-h period. No metabolism was seen with exposures at lower levels (Schuster et al. Citation2009).
Reproductive and developmental effects
Groups of 24 mated female rats were exposed nose only, to levels of 0 (control), 1500, 5000 or 15,000 ppm of HFO-1234ze for 6 h/day on Days 6–19 of gestation.
There was no mortality. It was concluded that the no-observed effect level was 15,000 ppm, the highest level tested (Rusch et al. Citation2013).
An inhalation range-finding prenatal developmental toxicity study of HFO-1234ze in rabbits was conducted for the purpose of determining appropriate exposure concentrations for a definitive prenatal developmental toxicity study. Four groups of time-mated female New Zealand rabbits (6/group) were exposed by whole-body inhalation to 0 (control), 1500, 5000 or 15,000 ppm HFO-1234ze for 6 h/day during Gestation Days 6–28. All animals survived to the scheduled necropsy on Gestation Day 29. No signs of maternal toxicity were observed. Intrauterine growth and survival were unaffected by maternal exposure to all exposure levels. No external fetal malformations or developmental variations were found. It was concluded that inhalation exposures up to 15,000 ppm HFO-1234ze to pregnant rabbits did not cause maternal or developmental toxicity in this pilot study (Rusch et al. Citation2013).
An inhalation prenatal developmental toxicity study of HFO-1234ze in rabbits was conducted. Four groups of time-mated female New Zealand rabbits (22/group) were exposed by whole-body inhalation to 0 (control), 1500, 10,000 or 15,000 ppm HFO-1234ze for 6 h/day during Gestation Days 6–28. All animals survived to the scheduled necropsy on Gestation Day 29. No signs of maternal toxicity were observed.
Intrauterine growth and survival were unaffected by maternal exposure to all exposure levels. It was concluded that inhalation exposures up to 15,000 ppm HFO-1234ze to pregnant rabbits did not cause maternal toxicity in this study. Also, there were no effects on pup live birth, sex ratio nor malformations. The NOEL for exposure of pregnant rabbits to vapors of HFO-1234ze for both does and pups was 15,000 ppm (Rusch et al. Citation2013).
Carcinogenesis
The carcinogenic potential of HFO-1234ze was evaluated using a genomic assay (Thomas Citation2007). Statistical analysis of rodent gene expression changes were conducted as part of the toxicological assessment of HFO-1234ze. Potential gene expression changesin liver, kidney, and lung tissue were assessed following exposure of female B6C3F1 mice and male F344 rats to levels of 5000 and 15,000 ppm HFO-1234ze 6 h/day, 5 days/wk for 13 weeks. The assessment was based on the results from a comparison of the responses seen with HFO-1234ze to both positive [tetrafluoroethylene, 1-amino-2,4-dibromoanthraquinone, and Tris(2,3-dibromopropyl)phosphate] and negative [trichlorofluoromethane, iodoform, tetrafluoroethane and N-(1-naphthyl)ethylenediamine dihydrochloride] controls. Vehicle controls were also included. In addition histopathological examination of selected tissues was conducted.
Statistical classification analysis predicted HFO-1234ze to be non-carcinogenic in both female mouse liver and male rat kidney. These findings had a statistical probability for selecting true negatives of 100% for kidney and 99.2% for liver. The probability for a true positive being identified was 90% for the kidney and 97.2% for the liver. Mouse lung was also initially included in the analysis. While a positive response was seen with the female mouse lung its predictability was only 71.3%. There was no dose-response relationship with respect to changes in gene expression. Furthermore, as only a limited number of genes were altered, it was concluded that for the female mouse lung, the number of genes altered was too small to perform a meaningful gene ontology enrichment analysis (Thomas Citation2007).
No treatment related histopathological lesions were observed in the liver or kidney and only 1/10 mice in both exposure groups showed mild irritation in the lung (ERPG Citation2012b). The weight of evidence suggests that HFC-1234ze is not likely to be carcinogenic. This conclusion is supported by the lack of mutagenic activity in all mammalian cell studies, the lack of significant metabolic activity, the lack of systemic toxicity and the lack of significant lesions in the livers, kidneys and lungs in any of the studies.
Genotoxicity/mutagenicity
In vitro
An Ames assay screen was conducted with the bacteria Salmonella typhimurium (strains TA1535, TA1537, TA98, and TA100) and Escherichia coli (strain WP2 uvrA) both with and without metabolic activation from a rat liver preparation. Each strain was exposed to a single exposure level of 5% (50,000 ppm) HFO 1234ze. Under the conditions of this assay, HFO-1234ze did not induce any evidence of mutagenic activity (Rusch et al. Citation2013).
An Ames assay was conducted with HFO-1234ze which involved exposure of bacterial cells TA 1535, TA1537, TA 98, TA 100 and WP2 uvrA both with and without S-9 metabolic activation. Exposure levels of up to 76% (plus 19% O2 and 5% CO2) were used. HFO-1234ze did not induce a response in any strain tested either without or in the presence of metabolic activation (Rusch et al. Citation2013).
A chromosome aberration test was conducted with cultured human lymphocytes that were exposed to vapor levels of HFO-1234ze up to 76%, both with and without S-9 metabolic activation. Under the conditions of this test, HFO-1234ze was not actively mutagenic (not clastogenic) (Rusch et al. Citation2013).
In Vivo
A mouse micronucleus assay was conducted following a single 4-h exposure to 29,208 ppm HFO-1234ze. At 48 and 72 h after exposure, peripheral blood smear samples were obtained from 5 male and 5 female exposed mice, as well as from negative and positive control animals. It was concluded that a 4-h exposure to 29,208 ppm HFO-1234ze did not cause chromosome damage in the peripheral blood of exposed mice (Rusch et al. Citation2013).
A second micronucleus assay was conducted with 10 male and 10 female CD-1 mice that were exposed, nose-only, to 103,300 ppm HFO-1234ze for 4 h. At 24 and 48 h after exposure, bone marrow cells from 5 mice per sex per interval were collected and analyzed for the presence of micronuclei. No signs of toxicity were observed during or after exposure. It was concluded that HFO 1234ze was non-genotoxic at a level of 103,300 ppm, as it did not cause an increase in micronuclei or evidence of bone marrow cell toxicity in mice (Rusch et al. Citation2013).
A rat micronucleus test was added to the 4-week inhalation toxicity study (described above), in which groups of male and female Sprague-Dawley rats were exposed nose only to vapors of HFO-1234ze at levels of 0 (control), 1000, 5000, 10,000 or 15,000 ppm 6-h/day, 5 days/week during a 4-week period. At necropsy, bone marrow from male rats in the control, 5000, 10,000 or 15,000 ppm groups was used in the micronucleus test. At the highest concentration tested (15,000 ppm), no damage to chromosomes or increased incidence of micronuclei was observed in the bone marrow cells of male rats (Rusch et al. Citation2013).
An unscheduled DNA synthesis test was also included in the 4-week inhalation toxicity study (described above), in which groups of male and female Sprague-Dawley rats were exposed nose only to vapors of HFO-1234ze at levels of 0 (control), 1000, 5000, 10,000 or 15,000 ppm 6-h/day, 5 days/week during a 4-week period. At necropsy, liver cells from male rats in the control, 5,000 and 15,00 ppm groups were used in the unscheduled DNA synthesis test. At the highest concentration tested (15,000 ppm), no unscheduled DNA synthesis was observed in the liver cells of male rats (Rusch et al. Citation2013).
Human experience
HFO-1234ze has only recently been introduced into commerce and there is no data on human experience.
Standards, regulations or guidelines of exposure
The TERA WEEL Committee has developed a workplace exposure level guideline for HFO-1234ze(E) of 800 ppm as an 8-h TWA (WEEL Citation2012) The AIHA ERP Committee has recommended emergency response exposure guidelines for exposures up to 1 h of:
ERPG-1 (annoyance) – NA
ERPG-2 (serious toxicity) – 15,000 ppm
ERPG-3 (potential lethality – 69,000 ppm
NA indicates that this level is not appropriate. For ERPG-1, it is not an irritant nor does it have an annoying odor (ERPGCitation2012b).
Trans-1-chloro-3,3,3-trifluoropropene (trans-HCFO-1233zd; CAS#102687–65-0; C3H2ClF3)
Common synonyms include HCFO-1233zd(E) and R-1233zd(E). It has a vapor density of 5.3, its solubility in water is 1.90 g/L and its log Pow is 2.2. It is nonflammable and has a very slight odor with poor warning properties (Schmidt et al. Citation2013).
Production and use
As HCFO-1233zd has only recently gone into production, production figures are not available. It has been developed as a solvent, foam blowing agent and refrigerant.
Air exposure assessment
HCFO-1233zd is a liquefied gas with a bp of 19 °C and a Henry ‘s law constant of 4.1 × 103 bars at 20 °C. It is rapidly degraded in the troposphere (life time = 21 days) by reaction with atmospheric hydroxyl radicals and has a GWP of 1 (Myhre et al. 2013) and an ozone depleting potential of almost 0 (0.00034) relative to CFC-11 = 1 (Hodnebrog et al. Citation2013).
Acute toxicity
As this substance is a liquefied gas, all animal studies were conducted by the inhalation route. Rats were exposed nose-only to vapors of HCFO-1233zd(E) at levels of 96,000, 120,000 or 156,000 ppm for 4 h. Following a 14 day observation period surviving rats were sacrificed. Gross necropsy observations on rats dying from the exposure revealed red discoloration of the lungs. In addition, signs suggestive of CNS depression such as hunched posture, lethargy, piloerection and restlessness were reported. The 4-h LC50 for HCFO-1233zd(E) was 118,200 ppm for males and 121,700 ppm for females. The combined 4-h LC50 for males and females is 120,000 ppm. The difference between males and females was not significant. Based on these results, HCFO-1233zd(E) is considered practically nontoxic by the inhalation route of exposure (Tveit et al. Citation2014).
Cardiac sensitization
Acute cardiac sensitization potential was evaluated according to the method described by ECETOC (Citation2009). A group of 6 beagle dogs were exposed to vapors of HCFO-1233zd(E) at concentrations of 25,000, 35,000 or 50,000 ppm. Each exposure level was conducted on a different day with at least a 2-day separation between the exposures. Initially a determination was made for the maximum level of epinephrine that would not cause a cardiac arrhythmia. The dogs were then exposed to the test substance for a total of 10 min. After the first five minutes of exposure, each dog received an injection of epinephrine at the pre-determined maximum sub-arrhythmia dose. During the next five minutes of exposure, the dogs were monitored for the development of a cardiac arrhythmia. No signs of cardiac sensitization were observed in any of the dogs. However, at 35,000 ppm dogs exhibited tremors and excessive salivation that made it impossible to conduct the electrocardiogram (EKG) monitoring. At 50,000 ppm only two dogs were exposed and no EKG measurements were obtained. One dog exhibited convulsions and one dog exhibited tremors which interfered with the EKG data. Based on these findings the threshold for general toxicity (not cardiac sensitization) was 35,000 ppm and the NOEC was 25,000 ppm (Tveit et al. Citation2014).
Subchronic and chronic toxicity
A 2-week inhalation toxicity study was conducted with four groups (5/sex) of Sprague Dawley rats exposed to HCFO-1233zd(E). Four groups of rats were exposed to vapors of HCFO-1233zd(E) 6 h/day, 5 days/wk for 2 weeks at levels of 0 (control), 2,000, 7,500 or 20,000 ppm. There was no mortality. Both males and females in the high-level group showed increases in urea levels. While histopathological examination of the kidney and liver did not show evidence for treatment-related effects, clinical chemistry observations could be suggestive of the beginning of effects on these organs. The livers in the 20,000 ppm females showed increases in hepatocellular vacuolation. Minimal histopathological changes in the heart were seen in the 20,000 ppm males and females and the 7,500 ppm males. The NOEC is 2,000 ppm. A 4-week inhalation toxicity study was conducted with five groups (5/sex) of Sprague Dawley rats. They were exposed nose only to vapors of HCFO-1233zd(E) at levels of 0 (control), 2,000, 4,500, 7,500 or 10,000 ppm for 6 h/day, 5 days/week during a 4-week period. As an additional component of this study, at necropsy liver cells from male rats in the control, 7,500 and 10,000 ppm groups were evaluated in an Unscheduled DNA Synthesis Test, and bone marrow from male rats in the control, 4,500, 7,500 and 10,000 ppm groups was assessed in a Micronucleus Test. Results of these two mutagenicity tests are discussed in the Genotoxicity and mutagenicity section below. Based on the slight increase in potassium levels at 7,500 and 10,000 ppm, the NOEC was 4,500 ppm.
A 13-week inhalation toxicity study was conducted with four groups (10/sex) of Sprague Dawley rats that were exposed nose-only to vapors of HCFO-1233zd(E) at levels of 0 (control), 4,000, 10,000 or 15,000 ppm for 6 h/day, 5 days/week during a 13-week period. Microscopic examination revealed multifocal mononuclear cell infiltrates in the hearts of all rats at 15,000 ppm, 7 of 10 males at 10,000 ppm and 1 male at 4,000 ppm. Fibrosis was not observed. The cardiac lesion in the one male at 4,000 ppm was not considered to be adverse based on its location (apex of heart) and its similarity to spontaneous lesions seen in this strain of rat. Thus the 4,000 ppm level in this study was considered to be the NOAEC (Tveit et al. Citation2014). Chronic toxicity studies were not conducted with HCFO-1233zd(E).
Pharmacokinetics, metabolism, and mechanisms
The biotransformation of HCFO-1233zd(E) and kinetics of metabolite excretion in urine were assessed in vitro and in animals after inhalation exposure. To characterize biotransformation in vitro, liver microsomes from rats, rabbits and humans were incubated with HCFO-1233zd(E). Male Sprague Dawley rats and female New Zealand White rabbits were exposed by inhalation to 2,000, 5,000 or 10,000 ppm of HCFO-1233zd(E) for 6 h and urine was collected for 48 h after the end of the exposure. Urine samples and microsomal incubation supernatants were analyzed for metabolites using 19F-NMR, LC-MS/MS and GC/MS. S-(3,3,3-trifluoro-trans-propenyl)-glutathione was identified as predominant metabolite of HCFO-1233zd(E) in rat, rabbit and human liver microsomes in the presence of glutathione. Products of the oxidative biotransformation of HCFO-1233zd(E) were only minor metabolites when glutathione was present. In rats, both 3,3,3-trifluorolactic acid and N-acetyl-(3,3,3-trifluoro-transpropenyl)-L-cysteine were observed as major urinary metabolites. 3,3,3-Trifluorolactic acid was not detected in the urine of rabbits exposed to HCFO-1233zd(E). Several minor metabolites formed by alternative reactions of S-(3,3,3-trifluoro-trans-propenyl)-glutathione were also excreted. Quantitation showed rapid excretion of both metabolites with urine T1/2 of less than 6 h in both species. Based on metabolite recovery in urine and estimated doses of HCFO-1233zd(E) received by inhalation, the extent of biotransformation of HCFO-1233zd(E) was determined as approximately 0.01% of received dose in rabbits and approximately 0.002% in rats. Based on metabolite structures, HCFO-1233zd(E) undergoes both oxidative biotransformation and glutathione conjugation at very low rates. The very low extent of biotransformation and the rapid excretion of metabolites formed are consistent with the very low potential for toxicity of HCFO-1233zd(E) in mammals. This metabolic profile is very similar to the tetrafluoro analog, HFO-1234ze (Schmidt et al. Citation2013). The blood:air partition coefficient for HFCO-1233zd(E) was determined for rats, rabbits and humans. As seen in the table below, the blood:air partition coefficient is lower for humans than for rats or rabbits. Therefore, at equivalent exposure concentrations the dose delivered to the systemic circulation of humans is less than half as much as a rat. PB/PK model was also developed using the structure and function of the physiological model jointly developed by the U.S. Environmental Protection Agency (U.S. EPA), Industry and the US Department of Defense scientists. Using this model, the rat NOAEC of 4,000 ppm for 6 h/day, 5 days/week for 28 days would be equivalent to a concentration of approximately 10,000 ppm in humans exposed for 8 h/day, 5 days/week for 28 days (Vingar et al. 2000). Thus the uptake in humans is less than half as much as in rats.
Blood air partition coefficient HCFO-1233zd(E) in humans rabbits and rats
Reproductive developmental
Groups of 24 mated female Wistar rats were exposed nose-only to levels of 0 (control), 4,000, 10,000 or 15,000 ppm of HCFO- 1233zd(E) for 6 h/day on days 6–19 of gestation. There was no mortality. There were no significant differences in fecundity index, the number of corpora lutea, the nimber of implantation sites, the number of live fetuses, post implantation loss or sex ratio. A statistically significant increases numbers of dialated urinary bladders, a visceral anomaly was observed in fetuses from the 15,000 ppm exposure level group. This effect may not be relevant as the kidneys were normal. However considering this effect, the NOEC was 10,000 ppm (Tveit et al. Citation2014).
An inhalation prenatal developmental toxicity study of HCFO- 1233zd(E) in rabbits was conducted. Four groups of time-mated female New Zealand rabbits (22/group) were exposed by wholebody inhalation to 0 (control), 2,500, 10,000 or 15,000 ppm HCFO-1233zd(E) for 6 h/day during gestation days 6 to 28. No signs of maternal toxicity were observed. Intrauterine growth and survival were normal. No external fetal developmental variations or malformations were found. It was concluded that inhalation exposures up to 15,000 ppm HCFO-1233zd(E) did not cause adverse effects (Tveit et al. Citation2014).
In a 2-generation reproductive toxicity study, groups of 19 Wistar rats were exposed to HCFO-1233zd(E) by nose-only or whole body inhalation for 6 h/day, 5 days/week during premating, and daily during mating, gestation and lactation at concentrations of 2,000, 5,000 or 15,000 ppm. No female exposures occurred between gestation Day 19 and lactation Day 4. Mortality occurred in females at the high dose (15,000 ppm) in both the F0 and F1 generations. Two females in the F0 generation were found dead on lactation Days 16 and 17. One female in the F1 generation was found dead on lactation Day 19. Histological evaluation of these animals revealed no obvious cause of death. There were no other compound related findings in either F0 or F1 generations, including microscopic examination of the heart. The NOEC for parental toxicity was considered to be 5,000 ppm and was based on the mortality observed in the high-dose females at 15,000 ppm. The NOEC for fertility and development was considered to be 15,000 ppm as no adverse effects were seen on fertility, reproduction or development (Tveit et al. Citation2014). A similar effect of mortality in the dames during the late stages of lactation was seen in the reproduction study with HFC-245fa. It was speculated that it could be due in part to the stress from nursing during the latter part of lactation (Buschmann et al. Citation2013).
Genotoxic effects
In vitro
An Ames assay was conducted with HCFO-1233zd(E) which involved exposure of bacterial cells S. typhimurium TA 1535, TA1537, TA 98, TA 100 and E. coli WP2 uvrA with and without S-9 metabolic activation. Exposure levels of up to 76% (plus 19% O2 and 5% CO2) were used. HCFO-1233zd(E) did not induce a response in any strain tested with or without metabolic activation (Tveit et al. Citation2014).
A chromosome aberration test was conducted with cultured human lymphocytes that were exposed to vapor levels of HCFO-1233zd(E) up to 50,000 ppm, with and without S-9 metabolic activation. The objective of this study was to assess the potential of HCFO-1233zd(E) to induce chromosome aberrations in human lymphocytes cultured in vitro. HCFO-1233zd(E) did not cause a positive response either in the absence or presence of S-9 metabolic activation. It was concluded that HCFO-1233zd(E) showed no evidence of increasing the frequency of structural chromosome aberrations under the conditions of this test (Tveit et al. Citation2014).
In Vivo
In a bone marrow micronucleus study a group of 10 male mice were exposed to a level of 50,000 ppm of HCFO-1233zd(E) for 4 h. Both positive and negative controls were included. At 24 and 48 h after the exposure, bone marrow was collected from the femurs of 5 mice and evaluated for the presence of micronucleated polychromatic erythrocytes. It was concluded that the test material did not induce chromosomal damage or damage to the mitotic spindle apparatus (micronuclei) in the target bone marrow cells. Thus it was concluded that HCFO-1233zd(E) was not active in this assay (Tveit et al. Citation2014).
A rat micronucleus test was an added procedure to the 4-week inhalation toxicity study (described above), in which groups (5/sex) of Sprague-Dawley rats were exposed nose-only to vapors of HCFO-1233zd(E) at levels of 0 (control), 2,000, 4,500, 7,500 or 10,000 ppm 6 h/day for 5 days/week. At the highest concentration tested (10,000 ppm), no damage to chromosomes or increased incidence of micronuclei was observed in the bone marrow cells of male rats (Tveit et al. Citation2014).
An unscheduled DNA synthesis test was also included in the 4-week inhalation toxicity study (described above) in which groups (5/sex) of Sprague-Dawley rats were exposed nose only to vapors of HCFO-1233zd(E) at levels of 0 (control), 2,000, 4,500, 7,500 or 10,000 ppm 6 h/day, 5 days/week during a 4-week period. At necropsy, liver cells from male rats in the control, 7,500 and 10,000 ppm groups were used in the unscheduled DNA synthesis test. At the highest concentration tested (10,000 ppm), no unscheduled DNA synthesis was observed in the liver cells of male rats (Tveit et al. Citation2014).
Human experience
HCFO-1233zd(E) was evaluated for skin sensitization in a human repeated insult patch test (RIPT) under semi-occlusive conditions. No evidence of skin irritation or skin sensitization was observed in any of the subject (TKL Citation2010, Citation2012).
Standards, regulations or guidelines of exposure
The OARS WEEL Committee has developed a workplace exposure level guideline for HFO-1233zd(E) of 800 ppm as an 8-h TWA (WEEL Citation2013).
Studies on environmental impact
HCFO-1233zd has a low GWP (1) and low ODP (0.00034 relative to trichlorofluoromethane). Therefore it will have a minimal effect on both greenhouse warming and ozone depletion.
Conclusions
In 1987 Governments reached agreement on the Montreal Protocol on Substances that Deplete the Ozone Layer under the auspices of the United Nations Environmental Program to control chlorofluorocarbons and halons that were contributing to the ozone depletion seen over Antarctica . The global fluorocarbon industry formed a consortium to investigate the toxicology of potential alternative products for chlorofluorocarbons and halons that were in widespread use in the aerosol, refrigeration, air-conditioning, foam insulation, solvent and fire-fighting industries. This consortium, The Program for Alternative Fluorocarbon Toxicity enabled companies to independently develop global production of alternatives in less than 5 years that had both lower GWP and ODP properties. Subsequent to the introduction of these products, the industry moved on to develop a new generation of fluorochemical products with zero, or almost zero ozone depletion potentials and greenhouse warming potentials close to or even lower than carbon dioxide. As most of the new materials in the PAFT program (hydrochlorofluorocarbons and hydrofluorocarbons) were not in production at the onset of the PAFT program, this was only possible as a result of the international cooperation between the world’s major chlorofluorocarbon producers. Many companies produced test materials from their own laboratories for the program adhering to impurity levels developed by the toxicology committee. As a class, these products have very low water solubility, most below 1 g/L and only 3 (HCFC-123, HCFC-141b and HFC-32) being above 2 g/L. With the exception of HFC-32, the log Pow values are all above 1 and most are above 2. They all have high vapor pressures and most are gasses at 25 °C. As a consequence, these materials will not accumulate in water and will partition rapidly into air. The only substance that caused lethality in a 4 h acute exposure study at 30,000 ppm (3%) was HCFC-123. All of the other chemicals had LC50s above 45,000 ppm and all HFCs and HFOs used in commercial applications had LC50s above 100,000 ppm (10%). None of these commercial substances were shown to be highly mutagenic, developmental toxins or carcinogenic. In conclusion based on available evidence, working together on toxicology and environmental issues, the Global Fluorocarbon Industry was able to develop new, safe refrigeration products and have them into commercial markets in less than 10 years.
Declaration of interest
The current professional affiliation and address of the author is shown on the cover page. Veritox, Inc. is a consulting firm providing scientific advice to private and public organizations. The author has been associated with Veritox,Inc. on a part-time basis since his retirement in 2015 from Honeywell, Inc., a producer and marketer of fluorocarbon. The review draws on the author’s extensive knowledge of halogenated hydrocarbons he gained as a Honeywell employee; however, he received no compensation from Honeywell for preparation of this review. Most of the author’s effort in preparing this review was done without compensation from the Journal or others. The paper was finalized with partial financial support from the European FluorocarbonTechnical Committee (EFCTC). Three members of the EFCTC reviewed this manuscript, Dr David Farrar, Dr Gary Jepson and Dr. Archie McCulloch. Dr. Farrar is a consultant with CCERT and consults for Mexichem. Mexichem was not a part of the PAFT research program although they currently market fluorocarbon products. Dr. Jepson works for Chemours, the successor to DuPont. At the time of the PAFT program, he was doing research on fluorocarbons for the military at Wright Patterson Air Force Base. Dr McCulloch is an independent contractor with an expertise in atmospheric chemistry. He provided valuable input on the ozone depletion and greenhouse warming potentials of these chemicals. The opinions expressed and conclusions are exclusively the professional work product of the author and do not necessarily reflect the views of his prior employer or his current consulting affiliates. The author has received consulting fees from two producers and marketers of refrigerants during the past 3 years, however, neither client was provided the opportunity to influence or review this manuscript.
Abbreviations | ||
AFEAS | = | Alternative Fluorocarbon Environmental Acceptability Study |
AL | = | Atmospheric Lifetime |
CFC | = | Chlorofluorocarbons |
ECETOC | = | European Centre for Ecotoxicology and Toxicology of Chemicals |
GWP | = | Global Warming Potential |
HCFC | = | Hydrochlorofluorocarbons |
HCFO | = | Hydrochlorofluoroolefins |
HFO | = | Hydrofluoroolefins |
HFC | = | Hydrofluorocarbons |
IPCS | = | International Program for Chemical Safety |
MRGL | = | Maximum Recommended Guidance Level |
OEL | = | Occupational Exposure Levels |
ODP | = | Ozone Depletion Potential |
PAFT | = | Program for Alternative Fluorocarbon Toxicity testing |
UNEP | = | United Nations Environmental Program |
WMO | = | World Meteorical Organization. |
Acknowledgements
It is impossible to thank all of the people who have made significant contributions to this 10 year program. I do however wish to thank the members of the PAFT toxicology committee: Pauline Bingham, William Brock, Michael Collins, Felix Debits, Christ de Rooij, Moussaka Coxall, Toshihiko. Kawano, Steven Magda, Giuseppe Malinverno, Dieter Mayer, Rene Millischer, Fumiaki Sato, Bruno Schmidt, Joel Seckar, Seiji Shin-Ya, Christine Stevens, Henry Trochimowicz, Julie Wade; the three toxicologists who made significant scientific contributions to the program: Wolfgang Dekant, Harry Emmen and Linda Malley and the current members of the European Fluorocarbon Technical Committee: David Farrar, Gary Jepson and Archie McCulloch for their helpful comments on this manuscript.
References
- Adir J, Blake DA, Mergner GM. 1975. Pharmacolinetics of fluorocarbon 11 and 12 in dogs and humans. J Clin Pharmacol. 15:760–770.
- AEGL. 2002a. Acute Exposure Guideline Levels. In: Clark K, editor. Technical Support Document 1,1,1,2-Tetrafluoroethane. Vol. 2. Washington, DC: National Advisory Committee on Acute Exposure Guideline Levels for Hazardous Substances, U.S. EPA p. 120–165.
- AEGL. 2002b. Acute Exposure Guideline Levels, In: Clark K, editor. Technical Support Document 1,1-Dichloro-1-Fluoroethane., National Advisory Committee on Acute Exposure Guideline Levels for Hazardous Substances. Vol. 2. Washington, DC: USEPA; p. 120–165.
- AER. 1992. Atmospheric and Environmental Research, Private communications to AFEAS. Cambridge, MA: AER.
- AFEAS. 1991. Alternative Fluorocarbons Environment Acceptability Study, Energy and Global Warming Impacts of CFC Alternative Technologies, Executive Summary. Washington, DC: U.S. Department of Energy, Rand Corp.
- AFEAS. 1992. Alternative Fluorocarbons Environment Acceptability Study. Proceedings of the AFEAS Workshop: Atmospheric Wet and Dry Deposition of Carbonyl and Haloacetyl Halides. Brussels. Washington (DC).: U.S. Department of Energy, Rand Corp.
- Alexander DJ, Libretto SE. 1995. An overview of the toxicology of HFA-134a (1,1,1,2-tetrafluoroethane). Hum Exp Toxicol. 14:715–720.
- Alexander DJ, Libretto SE, Adams MJ, Hughes EW, Bannerman M. 1996. HFA-134a (1,1,1,2-tetrafluoroethane): effects of inhalation exposure on reproductive performance, development and maturation of rats. Hum Exp Toxicol. 15:508–517.
- Alexander DJ, Libretto SE, Chevalier HJ, Imamura T, Pappritz G, Wilson J. 1995. HFA-134a (1,1,1,2-tetrafluoroethane); lack of oncogenicity in rodents after inhalation. Hum Exp Toxicol. 14:706–714.
- AlliedSignal. 1997a. A Four-Hour Acute Inhalation Toxicity Study with HFC 245ea in Mice (personal communication). Morristown (NJ): AlliedSignal.
- AlliedSignal. 1997b. Cardiac Sensitization Study with HFC 245ea in the Dog (personal communication). Morristown (NJ): AlliedSignal.
- AlliedSignal. 1997c. Genotoxic Evaluation of HFC 245ea with the Salmonella typhimurium Reverse Mutation Assay (personal communication). Morristown (NJ): AlliedSignal.
- AlliedSignal. 1997d. An Evaluation of HFC 245ea: The Chromosome Aberration Test Using Human Lymphocytes (personal communication). Morristown (NJ): AlliedSignal.
- AlliedSignal. 1997e. An Evaluation of HCFC 245ea in the In Vivo Mouse Micronucleus Assay (personal communication). Morristown (NJ): AlliedSignal.
- AlliedSignal. 1998. A Two Week Dose Range-Finding Inhalation Toxicity Study with HFC 245fa in Dogs (unpublished data), Huntingdon Life Sci. Rep. ALS 174/982608. Morristown (NJ): AlliedSignal.
- Amin YM, Thompson EB, Chiou WL. 1979. Fluorocarbon aerosol propellant. XIII. Correlation of blood levels of trichloromonofluoromethane to cardiovascular and respiratory responses in anesthetized dogs. J Pharm Sci. 68:160–163.
- Anderson D, Richardson CR. 1979. Arcton 134a: A Cytogenetic Study in the Rat, CTL Study No. SR0002. Cheshire, UK: Imperial Chemical Industries, Central Toxicology Laboratory, Alderley Park.
- Angerer J, Schröder B, Heinrich R. 1985. Exposure to fluorotrichloromethane (R-11). Int Arch Occup Environ Health. 56:67–72.
- Antti-Poika M, Heikkila J, Saarinen L. 1990. Cardiac arrhythmias during occupational exposure to fluorinated hydrocarbons. Br J Ind Med. 47:138–140.
- Araki A. 1991. Report on Reverse Mutation Assay in Bacteria on Tetrafluoroethane, Japan Bioassay Laboratory. Kanakawa: Japan Industrial Safety and Health Association. Study Nos. 5292 and 5312.
- Asakura M. 1993. Report on a Chromosomal Aberration Test of Difluoromethane in Cultured Mammalian Cells, Japan Bioassay Lab. Kanakawa: Japan Industrial Safety and Health Association. Rep. No. 5918.
- Asakura M. 1991. Report on a Chromosomal Aberration Test of 1,1,1,2-Tetrafluoro-Ethane in Cultured Mammalian Cells, Japan Bioassay Laboratory. Kanakawa: Japan Industrial Safety and Health Association. Rep. No. 5879.
- ASHRAE. 2017. Chemours Application to ASHRAE; Zeotropic Refrigerant Blend: R-744/R-125/R-1234yf/R-134a. May 2017 personal communication from Dr. Gary Jepson to the author.
- Atochem. 1986. Chlorodifluoromethane: Local Tolerance Tests in the Rabbit, Ocular Irritation—Cutaneous Irritation—Cutaneous Sensitizing Test in the Guinea Pig. Paris: Hazleton France for Elf Atochem, La Defense,. Hazleton IFT, Rep. No. 603344.
- Auton TR, Woollen BH. 1991. A physiologically based mathematical model for the human inhalation pharmacokinetics of 1,1,2-trichloro 1,2,2-trifluoroethane. Int Arch Occup Environ Heath. 63:133–138.
- Avella J, Wilson JC, Lehrer M. 2006. Fatal cardiac arrhythmia after repeated exposure to 1,1-difluoroethane. Am J Forensic Med Pathol. 27:58–60.
- Aviado DM. 1978. Effects of fluorocarbons, chlorinated solvents, and inosine on the cardiopulmonary system. Environ Health Perspect. 26:207–215.
- Aviado DM, Belej MA. 1974. Toxicity of aerosol propellants on the respiratory and circulatory systems. I. Cardiac arrhythmia in the mouse. Toxicology. 2:31–42.
- Aviado DM, Belej MA. 1975. Toxicity of aerosol propellants in the respiratory and circulatory systems V. ventricular function in the dog. Toxicology. 3:79–86.
- Aviado DM, Micozzi MS. 1981. Fluorine-containing organic compounds in industrial hygiene and toxicology. In G. D. Clayton, and F. E. Clayton, editors. Patty’s Industrial Hygiene and Toxicology. 3rd rev. ed. Vol. 2B. New York: Wiley; p. 3071–3115.
- Aviado DM, Smith DG. 1975. Toxicity of aerosol propellants in the respiratory and circulatory systems. VIII. Respiration and circulation in primates. Toxicology. 3:241–252.
- Azar A, Reinhardt CF, Maxfield ME, Smith PE, Mullin LS. 1972. Experimental human exposures to fluorocarbon 12 (dichlorodifluoromethane)). Am Ind Hyg Assoc J. 33:207–216.
- Azar A, Trochimowicz HJ, Terrill JB, Mullin LS. 1973. Blood levels of fluorocarbon related to cardiac sensitization. Am Ind Hyg Assoc J. 34:102–109.
- Barsky FC. 1976. In Vitro Microbial Mutagenicity Studies of Ethane, 1-Chloro-1,2,2,2-Trifluoro. Newark (DE): Haskell Laboratory, E.I. du Pont de Nemours & Co. Rep. No. 349-76.
- Barsky FC, Butterworth BE. 1976. In Vitro Microbial Mutagenicity Studies of Ethane Chlorodifluoro, Newark (DE): Haskell Laboratory, E.I. du Pont de Nemours & Co. Rep. No. 322-76.
- Bartsch H, Malaveille C, Camus A-M, Martel-Planche G, Brun G, Hautefeuille A, Sabadie N, Barbin A, Kuroki T, Drevon C, et al. 1980. Validation and comparative studies on 180 chemicals with S typhimurium strains and V79 Chinese hamster cells in the presence of various metabolizing systems. Mutat Res. 76:1–50.
- Bass M. 1970. Sudden Sniffing Death. JAMA. 212:2075–2079.
- Bayer T, Amberg A, Bertermann R, Rusch GM, Anders MW, Dekant W. 2002. Biotransformation of 1,1,1,3,3-pentafluoropropane (HFC-245fa). Chem Res Toxicol. 15:723–733.
- Belej MA, Smith DG, Aviado DM. 1974. Toxicity of aerosol propellants in the respiratory and circulatory systems. IV. Cardiotoxicity in the monkey. Toxicology. 2:381–395.
- Blake DA, Mergner GW. 1974. Inhalation studies on the biotransformation and elimination of 14C-trichlorofluoromethane and 14C-dichlororodifluoromethane in beagles. Toxicol Appl Pharmacol. 30:397–407.
- Bootman J, Hodson-Walker G, Lloyd JM. 1998. CFC141b: Investigation of Mutagenic Activity at the HGPRT Locus in a Chinese Hamster V79 Cell Mutation System. Suffolk, UK: Life Science Laboratory, Life Sciences Research, Eye.
- Bootman J, Hodson-Walker G. 1988. In Vitro Assessment of the Clastogenic Activity of CFC 141b in Cultured Hamster Ovary (CHO-K1) Cells (88/PSV007/214). Suffolk, UK: Life Science Laboratory, Life Sciences Research, Eye.
- Boucher R, Hanna C, Rusch GM, Stidham D, Swan E, Vazquez M. 2003. Hepatoxicity Associated with Overexposure to 1,1-dichloro-2,2,2-trifluoroethane (HCFC 123). J Amer Indus Hygiene Assoc. 64:68–79.
- Brock WJ. 1988a. Acute Dermal Toxicity Study of FC-141b. Newark, DE.: Haskell Laboratory, E.I. du Pont de Nemours & Co. Rep. No. 501-88.
- Brock WJ. 1988b. DuPont Haskell Laboratory for Toxicology & Industrial Medicine. Primary Dermal Irritation Study with NCFC 123 in Rabbits. Newark (DE): Haskell Laboratory, E.I. du Pont de Nemours & Co. HLR 535-88 (unpublished data).
- Brock WJ. 2002. Inhalation toxicity and genotoxicity of hydrofluorocarbon (HFC)-236fa and 236ea. Intern J Toxicol. 19:69–83.
- Brock WJ, Trochimowicz HJ, Millischer RJ, Farr C, Kawano T, Rusch GM. 1995. Acute and subchronic toxicity of 1,1-dichloro-1-fluoroethane (HCFC-141b)). Food Chem Toxicol. 33:483–490.
- Brock WJ, Rusch GM, Trochimowicz HJ. 2003. Cardiac sensitization: methodology and interpretation in risk assessment. Regul Toxicol Pharmacol. 38:78–90.
- Brock WJ, Trochimowicz HJ, Farr CH, Millischer RJ, Rusch GM. 1996. Acute, subchronic, and developmental toxicity and genotoxicity of 1,1,1-trifluoroethane (HFC-143a)). Fundam Appl Toxicol. 31:200–209.
- Brodzinsky R, Singh HB. 1982. Volatile Organic Chemicals in the Atmosphere: An Assessment of Available Data. Research Triangle Park, NC.: Environmental Sciences Research Laboratory, Office of Research and Development, U.S. Environmental Protection Agency. Final report on Contract No. 68-022-3452.
- Broussard LA, Brustowicz T, Pittman T, Atkins KD, Presley L. 1997. Two traffic fatalities related to the use of difluoroethane. J Forensic Sci. 42:1186–1187.
- Brugnone F, Perbellini L, Mangani F. 1981. Blood concentrations of solvents in industrial exposure. Collect Méd Leg Toxicol Méd. 125:165–168.
- Brusick DJ. 1976. Mutagenicity Data of Genetron 134a. Morristown (NJ): Litton Bionetics for Allied Chemical Corp. Final Report, LBI Proj. No. 2683 (unpublished data).
- Brusick DJ. 1976. Mutagenicity Evaluation of Genetron 124 (LBI No. 2547). Morristown (NJ): Litton Bionetics for Allied Chemical Corp.
- Buschmann J. 1996. Crossover Study with HCFC 123 in Lactating Sprague–Dawley Rats including Additional Studies on Milk Production and Metabolites in Offspring Urine. Hannover, Germany: Fraunhofer Institute.
- Buschmann J, Fuhst R, Tillmann T, Ernst H, Kolling A, Pohlmann G, Preiss A, Berger-Preiss E, Hansen T, Kellner R et al. 2013. Unexpected Brain Lesions in Lactating Rats in a two-generation Reproductive toxicology study. Experimental Toxicology Pathology. 65:875–882.
- Butterworth BE. 1976. In Vitro Microbial Mutagenicity Studies of Methane, Chlorodifluorocarbon. Newark (DE): Haskell Laboratory, E.I. du Pont de Nemours & Co. Rep. No. 398-76.
- Callander RD. 1989. HCFC 123—An Evaluation Using the Salmonella Mutagenicity Assay. Alderley Park, Macclesfield, Cheshire, UK: Imperial Chemical Industries, Central Toxicology Laboratory. Rep. No. CTL/P/2421.
- Callander RD, Priestley KP. 1990. HFC 134a. An Evaluation Using the Salmonella Mutagenicity Assay, CTL. Alderley Park, Cheshire, UK: Imperial Chemical Industries, Central Toxicology Laboratory. Rep. No. CTL/P2422 (unpublished data),
- Cappon GD, Keller DA, Brock WJ, Slauter RW, Hurtt ME. 2002. Effects of HCFC-123 exposure to maternal and infant rhesus monkeys on hepatic biochemistry, lactational parameters and postnatal growth. Drug Chem. Toxicol. 25:481–496.
- Carney I. 1977. Arcton 22 (Chlorodifluoromethane) Relationship between Blood Levels and Inhaled Concentrations in Anesthetised Artificially Ventilated Rats, Alderley Park, Cheshire, UK: Imperial Chemical Industries PLC, Central Toxicology Laboratory. Rep. No. CTL/R/422.
- Carpenter CP, Smyth HF, Pozzani UC. 1949. The Assay of acute vapor toxicity, and the grading and interpretation of results on 96 chemical compounds. J Ind Hyg Toxicol. 31:343–346.
- Carter VL. 1970. Effects of Inhalation of Freon 113 on Laboratory Animals. Springfield, VA: National Technical Information Service. NTIS AD Rep. No. 727523.
- Clark DG, Tinston DJ. 1972. The influence of fluorocarbon propellants on the arrhythmogenic activities of adrenaline and isoprenaline. Proc Eur Soc Study Drug Toxic. 13:212–217.
- Clark DG, Tinston DJ. 1982. Acute inhalation toxicity of some halogenated and non-halogenated hydrocarbons. Hum Toxicol. 1:239–247.
- Clayton JW. 1966. The mammalian toxicology of organic compounds containing fluorine. Handb Exp Pharmakol. 20:459–500.
- Clayton JW. 1967. Fluorocarbon toxicity and biological action. Fluorine Chem Rev. 1:97–252.
- Coate WB. 1976. LC50 of G124 in Rats. Morristown, NJ: Hazleton Laboratories America, Allied Chemical Corp. Proj. No. 165-163. Final Report.
- Collins M, Rusch G, Sato F, Hext P, Millischer R. 1995. Toxicity studies of 1,1,1,2-tetrafluoroethane, a hydrofluorocarbon alternative to chloro-fluorocarbons. Toxicol Sci. 25:271–280.
- Coombs DW. 1984. HCFC 123–13-Week Inhalation Neurotoxicity Study in the Rat. Huntingdon, Cambridgeshire, UK: Huntingdon Research Centre.
- Cox RA, Derwent RG, Eggleton AEJ, Lovelock JE. 1976. Photochemical oxidation of halocarbons in the troposphere. Atmos Environ. 10:305–308.
- Cox PJ, King LJ, Parke DV. 1972. A comparison of the interactions of trichlorofluoromethane and carbon tetrachloride with hepatic cytochrome P-450. Biochem J. 130:87P.
- Cox PJ, King LJ, Parke DV. 1972. A study of the possible metabolism of trichlorofluoromethane. Biochem J. 130:13P–14P.
- Culik R, Kelly DP. 1980. Embryotoxicity and Teratogenicity Studies in Rats with 1,1-Dichloroethane. Newark, DE.: Haskell Laboratory, E.I. du Pont de Nemours & Co. (HFC-152a) (unpublished report 437-79).
- Damske DR, Mecler FJ, Beliles RP. 1978. Teratology Study in Rats; Isotron 142b. Monochlorodifluoroethane. England: Litton Bionetics, Kensington, MD, for ICI, Macclesfield. Final Report, LBI Proj. No. 20980.
- Dance CA. 1991. In Vitro Assessment of the Clastogenic Activity of HCFC 123 in Cultured Human Lymphocytes, Final Report. Suffolk, UK: Life Sciences Research, Eye.
- Darr RW. 1981. An Acute Inhalation Toxicity Study of Fluorocarbon 123 in the Chinese Hamster, Corporate Medical Affairs Rep. No. MA-25-78-15. Morristown (NJ): Allied Corporation.
- Davies RH, Bagnall RD, Bell W, Jones WGM. 2009. The hydrogen bond proton donor properties of volatile halogenated hydrocarbons and ethers and their mode of action in anaesthesia. Int J Quantum Chem. 10:171–185.
- DEC. 1987. Dutch Expert Committee RAIS/87. Health Based Recommended Occupational Exposure Limits for Fluorocarbons. The Netherlands: Dutch Expert Committee for Occupational Standards, Directorate-General of Labor.
- Dekant W. 1984. Metabolism of 1,1-Dichloro-2,2,2-Trifluoroethane (personal communication), Wurzburg, Germany: Institute for Toxicology, University of Wurzburg.
- Dekant W. 1996. Toxicology of chlorofluorocarbon replacements. Environ Health Perspect. 104:75–83.
- Desoille H, Truffert L. 1968. Experimental study on the toxicity of trichlorotrifluoroethane (Freon 113). I Arch Mal Prof Méd Trav Sécur Soc. 29:381–388.
- Desoille H. 1973. Experimental research on the long term chronic toxicity of dichlorotetrafluoroethane. Arch Mal Prof Med Trav Secur Soc. 34:117–125.
- DFG. 1989. Deutsche Forschungsgemeinschaft, Maximale Arbeitsplatzkonzentrationen und Biologische Arbeitsstofftoleranzwerte, D-6940. Weinheim: VCH Verlag. 151. H.
- Dodd DE, Brashear WT, Vinegar A. 1993. Metabolism and pharmacokinetics of selected Halon replacement candidates. Toxicol Lett. 68:37–47.
- Dodd DE, Ledbetter AD, Mitchell AD. 1997. Genotoxicity testing of the halon replacement candidates trifluoroiodomethane and 1,1,1,2,3,3,3-heptafluoropropane (HFC 227ea) using Salmonella typhimurium and L5178Y mouse lymphoma mutation assays and the mouse micronucleus test. Inhal Toxicol. 9:111–131.
- Doherty RE, Aviado DM. 1975. Toxicity of aerosol propellants in the respiratory and circulatory systems. VI. Influence of cardiac and pulmonary vascular lesions in the rat. Toxicology. 3:213–224.
- Du Pont. 1995. Inhalation Developmental Toxicity Study of HFC-236fa in Rats, Newark (DE): Haskell Laboratory, E.I. du Pont de Nemours & Co. Rep. No. 66–95 (unpublished data).
- Du Pont. 1996. Inhalation Developmental Toxicity Study HFC-236fa in Rabbits, Newark (DE): Haskell Laboratory, E.I. du Pont de Nemours & Co. Rep. No. 417-96 (unpublished data).
- European Centre for Ecotoxicology and Toxicology of Chemicals (ECETOC). 1991. Joint Assessment of Commodity Chemicals, No. 17, 1-Chloro-1, 1-Difluoromethane. Brussels, Belgium: ECETOC.
- European Centre for Ecotoxicology and Toxicology of Chemicals (ECETOC). 1994a. Joint Assessment of Commodity Chemicals, No. 25, 1-Chloro-1,2,2,2-Tetrafluoroethane. Brussels, Belgium: ECETOC.
- European Centre for Ecotoxicology and Toxicology of Chemicals (ECETOC). 1994b. Joint Assessment of Commodity Chemicals, No. 29, 1-1-Dichloro-1-Fluoroethane. Brussels, Belgium: ECETOC.
- European Centre for Ecotoxicology and Toxicology of Chemicals (ECETOC). 1994c. Joint Assessment of Commodity Chemicals, No. 24: Pentafluoroethane (HFC 125). Brussels, Belgium: ECETOC.
- European Centre for Ecotoxicology and Toxicology of Chemicals (ECETOC). 1995a. Joint Assessment of Commodity Chemicals, No. 32: Difluoromethane. Brussels, Belgium: ECETOC.
- European Centre for Ecotoxicology and Toxicology of Chemicals (ECETOC). 1995b. Joint Assessment of Commodity Chemicals, No. 31: 1,1,1,2-Tetrafluoroethane. Brussels, Belgium: ECETOC.
- European Centre for Ecotoxicology and Toxicology of Chemicals (ECETOC) 1995a. Joint Assessment of Commodity Chemicals, No. 31: 1,1,1,2-Tetrafluoroethane. Brussels, Belgium: ECETOC.
- European Centre for Ecotoxicology and Toxicology of Chemicals (ECETOC). 1995b. Joint Assessment of Commodity Chemicals, No. 32: Difluoromethane. Brussels, Belgium: ECETOC.
- European Centre for Ecotoxicology and Toxicology of Chemicals (ECETOC). 1996. Joint Assessment of Commodity Chemicals, No. 33, 1-1-Dichloro-2,2,2-Trifluoroethane. Brussels, Belgium: ECETOC.
- European Centre for Ecotoxicology and Toxicology of Chemicals (ECETOC). 2005. Joint Assessment of Commodity Chemicals, No. 47, 1-1-Dichloro-2,2,2-Trifluoroethane. Brussels, Belgium: ECETOC.
- European Centre for Ecotoxicology and Toxicology of Chemicals (ECETOC). 2006. Joint Assessment of Commodity Chemicals, No. 52, 1,1,1-Trifluoroethane. Brussels, Belgium: ECETOC.
- European Centre for Ecotoxicology and Toxicology of Chemicals (ECETOC). 2008. Toxicity of Possible Impurities and By-products in Fluorocarbon Products, Technical Report No. 103, ECETOC. Brussels, Belgium: ECETOC.
- European Centre for Ecotoxicology and Toxicology of Chemicals (ECETOC). 2009. Evaluation of Cardiac Sensitization Test Methods, Technical Report No. 105, ECETOC. Brussels, Belgium: ECETOC.
- Eddy CW, Griffith FD. 1971. Metabolism of Dichlorodifluoromethane-C14 by Rats, presented at the American Industrial Hygiene Association Conference, Toronto, Canada, May 1971, American National Standards Institute, New York, and American Industrial Hygiene Association, Akron, OH.
- Edling C, Olson CG. 1988. Health Risks with Exposure to Freons in (Swedish). Uppsala, Sweden: Department of Occupational Medicine, University Hospital.
- Edwards CN. 1991a. HCFC 123 (Vapour Phase): In Vitro Assessment of Clastogenic Activity in Cultured Human Lymphocytes. Suffolk, UK: Life Sciences Research, Eye. Final Rep. No. 91/PFE002/0125.
- Edwards CN. 1991b. In Vitro Assessment of the Clastogenic Activity of HCFC 124 in Cultured Chinese Hamster Ovary (CHO-K1) Cells. Suffolk, UK: Life Sciences Research, Eye. Final Rep. No. 91/PAR002/0855a.
- Egeland GM, Bloom TF, Schnorr TM, Hornung RW, Suruda AJ, Wille KK. 1992. Fluorocarbon 113 exposure and cardiac dysrhythmias among aerospace workers. Am J Ind Med. 22:851–857.
- Ellis MK, Gowans LA, Green T, Tanner RJN. 1993. Metabolic fate and disposition of 1,1,1,2-tetrafluoroethane (HCFC 134a) in the rat following a single exposure by inhalation. Xenobiotica. 23:719–729.
- Ellis MK, Naylor JL, Collins MA, Green T. 1996. Metabolism and disposition of difluoromethane (HFC32) in the mouse. Hum Exp Toxicol. 15:592–596.
- Ellis MK, Trebilcock R, Naylor JL, Tseung K, Collins MA, Hext PM, Green T. 1996. The inhalation toxicology, genetic toxicology, and metabolism of difluoromethane in the rat. Fundam Appl Toxicol. 31:243–251.
- Ema M, Naya M, Yoshida K, Nagaosa R. 2010. Reproductive and developmental toxicity of hydrofluorocarbons used as refrigerants. Reprod Toxicol. 29:125–131.
- Ember LR. 1996. The changing atmosphere. Chem Eng News. 70:14–64.
- Emmens H. 1999a. Report on an Ascending Dose Safety Study Comparing HFA-134a with CFC-12 and Air-Administered by Whole-Body Exposure to Health, Volunteers (unpublished data), Zeist, The Netherlands: TNO Nutrition and Food Research Institute. TNO. Rep. V98.754.
- Emmens H. 1999b. Report on an Ascending Dose Safety Study Company HFA-227 with CFC-12 and Air, Administered by Whole-Body Exposure to Healthy Volunteers. The Netherlands: TNO Nutrition and Food Research Institute. TNO Rep. V98.755.
- Ernstgård L, Andersen M, Dekant W, Sjögren B, Johanson G. 2010. Experimental exposure to 1,1,1,3,3-pentafluoropropane (HFC-245fa): uptake and disposition in humans. Toxicol Sci. 113:326–336.
- Epstein SS, Joshi S, Andrea J, Clapp P, Flak H, Mantel N. 1967. Synergistic toxicity and carcinogenicity of “'freons' and piperonyl butoxide”. Nature (London). 214:526–528.
- Epstein SS, Arnold E, Andrea J, Bass W, Bishop Y. 1972. Detection of chemical mutagens by the dominant lethal assay in the mouse. Toxicol Appl Pharmacol. 23:288–325.
- Emergency Response Planning Guide Committee (ERPG). 2012a. Emergency Response Planning Guidelines. 2,3,3,3-Tetrafluoropropene. Falls Church (VA): AIHA Guideline Foundation.
- Emergency Response Planning Guide Committee (ERPG). 2012b. Trans-1,3,3,3-tetrafluoropropene. Emergency Response Planning Guidelines. Falls Church (VA): AIHA Guideline Foundation.
- Emergency Response Planning Guide Committee (ERPG). 2014. Emergency Response Planning Guide: 1-Chloro-1, 1-Difluoroethane. Farifax, VA: AIHA- American Industrial Hygiene Association.
- Emergency Response Planning Guide Committee (ERPG). 2017. Emergency Response Planning Guide: 1,1-Dichloro-2,2,2-Trifluoroethane. Farifax, VA: AIHA- American Industrial Hygiene Association.
- Emergency Response Planning Guide Committee (ERPG). 2018. Emergency Response Planning Guide: 1,1-Difluoroethane. Farifax, VA: AIHA- American Industrial Hygiene Association.
- Farman JC, Gardiner BG, Shanklin JD. 1985. Large losses of total ozone in Antartica; several seasonal ClOxNOx interactions. Nature. 315:207–210.
- Ferrara R. 1995. Reductive activation of 2,2-dichloro-1,1,1-trifluoroethane (HCFC-123) by rat liver microsomal cytochrome P-450. 5th International Symposium for Biological Reactions Intermediates. Munich, Germany.
- Ferrara R, Tolando R, King LJ, Manno M. 1997. Cytochrome P450 inactivation during reactive metabolism of 1,1-dichloro-2,2,2-trifluoroethane (HCFC-123) by phenobarbitol- and pyridine-induced rat liver microsomes. Toxicol Appl Pharmacol.143:420–428.
- Flowers NC, Hand RC, Horan LG. 1975. Concentrations of fluoroalkanes associated with cardiac conduction system toxicity. Arch Environ Health. 30:353–360.
- Flowers NC, Horan LH. 1972. Effects of respiratory acidosis on the cardiac response to aerosol inhalation. Clin Res. 20:619.
- Fogel S. 1976. Letter: Sudden death and fluorocarbon-containing aerosols. Can Med Assoc J. 114:671–672.
- Foll GE. 1976. Results of Toxicology Testing on Arctons® 123 and 124 at Central Toxicology Laboratory, attachment to correspondence to M. B. Berenbaum, Allied Chemical Corp. from G. E. Foll. Cheshire, UK: Imperial Chemical Industries.
- Foltz VC, Fuerst R. 1974. Mutation studies with Drosophila melanogaster exposed to four fluorinated hydrocarbon gases. Environ Res. 7:275–285.
- Franklin J. 1993. The atmospheric degradation and impact of 1,1,1,2-tetrafluoroethane (hydrofluorocarbon 134a). Chemosphere. 27:1565–1601.
- Friedman SA, Cammarato M, Aviado DM. 1973. Toxicity of aerosol propellants on the respiratory and circulatory systems. II. Respiratory and bronchopulmonary effects in the rat. Toxicology. 1:345–355.
- Furuya M. 1979. Experimental chlorofluorohydrocarbon poisoning. Tokyo Jikeikai Ika Daigaku Zasshi. 94:1201–1214.
- Gardner JR. 1988. Acute Dermal Toxicity to Rats of Dichlorofluoroethane, Huntingdon, Cambridgeshire, UK: Huntingdon Research Centre. Rep. No. 881114/PWT91/AC.
- Gargas ML, Burgess RJ, Voisard DE, Cason GH, Andersen ME. 1989. Partition coefficients of low-molecular-weight volatile chemicals in various liquids and tissues. Toxicol Appl Pharmacol. 98:87–99.
- Gargas ML, Clewell HJ, Andersen ME. 1986. Metabolism of inhaled dihalomethanes in vivo: differentiation of kinetic constants for two independent pathways. Toxicol Appl Pharmacol. 82:211–223.
- Garrett S, Fuerst R. 1974. Sex-linked mutation in Drosophila after exposure to various mixtures of gas atmosphere. Environ Res. 7:286–293.
- Godin CS, Drerup JM, Vinegar A. 1993. Conditions influencing the rat liver microsomal metabolism of 2,2,-dichloro-1,1,1-trifluoroethane (HCFC-123)). Drug Metab Dispos. 21:551–553.
- Graff-Lonnevig V. 1979. Diurnal expiratory flow after inhalation of Freons and fenoterol in childhood asthma. J Allergy Clin Immunol. 64:534–538.
- Greenberg MJ, Pines A. 1967. Pressurized aerosols in asthma. Br Med J. 1:563.
- Gunnare S, Ernstgaard L, Sjogren B, Johanson G. 2003. Uptake and disposition of 1,1,1-trifluoroethane in man. Toxicol Sci. 72:338. Poster 42nd Annual Meeting Society of Toxicology, Salt Lake City, UT.
- Gunnare S, Ernstgård L, Sjögren B, Johanson G. 2006. Toxicokinetics of 1,1,1,2-tetrafluoroethane (HFC-134a) in male volunteers after experimental exposure. Toxicol Lett. 167:54–65.
- Gunnare S, Vidali M, Albano E, Johanson G. 2005. Antibodies against CYP2E1 after exposure to 1,1,1-trifluoroethane and 1,1,1,2-tetrafluoroethane. Toxicol Sci. 84:185.
- Gunnare S, Vidali M, Lillienberg L, Ernstgård L, Sjögren B, Hagberg M, Albano E, Johanson G. 2007. Non-positive autoimmune responses against CYP2E1 in refrigeration mechanics exposed to halogenated hydrocarbons. Sci Total Environ. 383:90–97.
- Hahn T, Avella J, Lehrer M. 2006. A motor vehicle accident fatality involving the inhalation of 1,1-difluoroethane. J Anal Toxicol. 30:638–642.
- Hall GT. 1975. Acute Inhalation Toxicity of Freon 123. Newark, DE.: Haskell Laboratory, E.I. du Pont de Nemours & Co. Rep. No. 426-75.
- Hamner Institute for Health Sciences. 2007. Toxicogenomic Assessment of the carcinogenic potential. Of 2,3,3,3-tetrafluoropropene. Research Triangle Park, NC: The Hamner Institute for Health Sciences. Report no. 06014.
- Hanst PL. 1978. Noxious trace gases in the air. Part II. Halogenated pollutants. Chemistry. 51:6–12.
- Hardy CJ. 1989. HCFC 141b Acute Inhalation Toxicity Study in Rats, 4-Hour Exposure. Huntingdon, Cambridgeshire, UK: Huntingdon Research Centre. Rep. No. PWT95/881676.
- Hardy CJ, Kieran PC. 1992. Hydrofluorocarbon 32: Assessment of Cardiac Sensitisation Potential in Beagle Dogs. Huntingdon, Cambridgeshire, UK: Huntingdon Research Centre. ISN 276/920493.
- Hardy CJ, Sharman IJ, Clark GC. 1991. Assessment of Cardiac Sensitization in Dogs, Comparison of HFA 134a and A12, CTL. Huntingdon, Cambridgeshire, UK: Huntingdon Research Centre. Rep. No. CTL/C/2521, ICI, CTL.
- Harris JW, Jones JP, Martin JL, LaRosa AC, Olson MJ, Pohl LR, Anders MW. 1992. Pentahaloethane-based chlorofluorocarbons substitutes and halothane: correlation of in vivo hepatic protein trifluoroacetylation and urinary trilfuoroacetic acid excretion with calculated enthalpies of activation. Chem Res Toxicol. 5:720–725.
- Harris JW, Anders MW. 1991a. Metabolism of the hydrochlorofluorocarbon 1,2-dichloro-1,1-difluoroethane. Chem Res Toxicol. 4:180–186.
- Harris JW, Anders MW. 1991b. In vivo metabolism of the hydrochlorofluorocarbon 1,1-dichloro-1-fluoroethane (HCFC-141b). Biochem Pharmacol. 41:R13–R16.
- Harriset WS. 1971. Evidence from animals and man that Freon depresses myocardial contractility. Circulation. 44:119.
- Harrison LI, Donnell D, Simmons JL, Ekholm BP, Cooper KM, Wyld PJ. 1996. Twenty-eight-day double-blind safety study of an HFA-134a inhalation aerosol system in healthy subjects. J Pharm Pharmacol. 48:596–600.
- Hartop PJ, Adams MG. 1989. Simulated consumer exposure to propellant HCFC 22 (chlorodifluoromethane) in aerosol personal products. Int J Cosmet Sci. 11:27–34.
- Henschler D. 1987. Gesundheitsschadliche Arbeitstoffe, Toxikologisch-arbeitsmedizinische, Begrundung von MAK-Werten, No. 12, VCH Verlag.
- Hino Y, Yamasaki K, Schiraishi K. 1992. Twenty Eight-Day Repeated Inhalation Toxicity Study of HCFC 141b in Rats. OITA, Japan: Hita Research Laboratory Chemical Biotesting Center, Chemicals Inspection and Testing Institute. Rep. B 18-0005.
- Hodnebrog O, Etminan M, Fuglestvedt S, Marston G, Myhre G, Nielsen CJ. 2013. Global warming potentials and radiative efficiencies of halocarbons and related compounds: a comprehensive review. Rev Geophys. 51:300–378.
- Hodson-Walker G. 1990. In Vitro Assessment of the Clastogenic Activity of HCFC141b in Cultured Chinese Hamster Ovary (CHO-K1) Cells. Suffolk, UK: Life Sciences Research, Eye. LSR Rep. 89/PFG001/0971a.
- Hoet P, Graf MLM, Bourdi M, Pohl LR, Duray PH, Chen W, Peter RM, Nelson SD, Verlinden N, Lison D. 1997. Epidemic of liver disease caused by hydrochlorofluorocarbons used as ozone-sparing substitutes of chlorofluorocarbons. Lancet. 350:556–559.
- Honeywell. 2011. HFO-1234ze Material Safety Data Sheet, Morristown NJ.
- Horiguchi Y. 1985. Studies on cutaneous sensitization to 1,1,2-trichloro-1,2,2-trifluoroethane. Bull—Kanagawa Prefect Public Health Lab. 15:39–41.
- Huwyler J, Aeschlimann D, Christen U, Gut J. 1992. The kidney as novel target tissues for protein adduct formation associated with metabolism of halothane and the candidate chlorofluorocarbon replacement 2,2-dichloro-1,1,1-trifluoroethane. Eur J Biochem. 207:229–238.
- Huwyler J, Gut J. 1992. Exposure to the chlorofluorocarbon substitute 2,2-dichloro-1,1,1-trifluoroethane and the anesthetic agent halothane is associated with transient protein adduct formation in the heart. Biochem Biophys Res Commun. 184:1344–1349.
- Imbus HR, Adkins C. 1972. Physical examinations of workers exposed to trichlorotrifluoroethane. Arch Environ Health. 24:257–261.
- Intergovernmental Panel on Climate Change (IPCC). 1990. International Program for Chemical Safety. Fully Halogenated Chlorofluorocarbons, Environmental Health Criteria 113. Geneva: World Health Organization.
- Intergovernmental Panel on Climate Change (IPCC). 1992. International Program for Chemical Safety. Partially Halogenated Chlorofluorocarbons, Environmental Health Criteria 139, 2PCS. Geneva: World Health Organization.
- Intergovernmental Panel on Climate Change (IPCC). 1994. Radiative Forcing of Climate Change 1994. The 1994 Report of the Scientific Assessment Working Group of IPCC, Summary of Policymakers, IPCC. Geneva: World Health Organization.
- Izmerov NF, Sanotsky IV, Sidorov KK. 1982. Toxicometric Parameters of Industrial Toxic Chemicals under Single Exposure. Moscow: Russian USSR State Committee for Science and Technology.
- Jack D. (1971) Allen & Hanburys Ltd., An Investigation of Possible Cardiotoxic Effects of the Aerosol, Propellants, Arctons 11 and 12, Vol. 1, unpublished report, courtesy of D. Jack, Managing Director, Allen & Hanburys Ltd.
- Jagannath DR. 1977. Mutagenic Evaluation of Isotron 142b. Kensington, MD: Litton Bionetics, Final Report, LBI Proj. No. 20838.
- Janssen PJM, Pot TE. 1988. Acute Dermal Toxicity Study with FC 141b in Rats, Interim Doc. No. 56645/23/88. Duphar B.V., The Netherlands: Department of Toxicology, Weesp.
- Jefferson IG. 1978. Accidental death of child playing with deodorant aerosol. Lancet. 1:779.
- Jenkins LJ, Jones RA, Coon RA, Siegel J. 1970. Repeated and continuous exposures of laboratory animals to trichlorofluoromethane. Toxicol Appl Pharmacol. 16:133–142.
- Khalil MAK, Rasmussen RA. 1981. Increase of CHClF2 in the earth’s atmosphere. Nature (London). 292:823–824.
- Kaiser KLE, Comba ME, Huneault H. 1983. Volatile halocarbon contaminants in the Niagara River and in Lake Ontario. J Great Lakes Res. 9:212–223.
- Karpov BD. 1963a. The chronic toxicity of Freon 22. Tr Leningr Sanit Gig Med Inst. 75:231–240.
- Karpov BD. 1963b. The chronic toxicity of Freon 22. Tr Leningr Sanit-Gig Med Inst. 75:128–137.
- Kaufman JD, Silverstein MA, Moure-Eraso R. 1994. Atrial fibrillation and sudden death related to occupational solvent exposure. Am J Ind Med. 25:731–735.
- Kawano T, Trochimowicz HJ, Malinverno G, Rusch GM. 1995. Toxicological evaluation of 1,1,1,2,2-pentafluoroethane (HFC-125)). Fundam Appl Toxicol. 28:223–231.
- Kehoe RA. 1943. Report on Human Exposure to Dichlorodifluoromethane in Air, unpublished report. Cincinnati, OH: Kettering Laboratory.
- Keller DA. 1994. Metabolism of HFC-143a in the Rat, unpublished report HLR 3-94. Newark (DE): Haskell Laboratory for Toxicology and Industrial Medicine. DuPont de Nemours.
- Keller DA, Roe CD, Lieder PH. 1996. Fluoroacetate-mediated toxicity of fluorinated ethanes. Fundam Appl Toxicol. 30: 213–219.
- Kelly DP. 1978. Inhalation teratology studies on three fluorocarbons. Toxicol Appl Pharmacol. 45:293.
- Kelly DP. 1990. Four-Hour Inhalation Approximate Lethal Concentration (ALC) of HCFC-124 in Rats. Newark (DE): Haskell Laboratory, E.I. du Pont de Nemours Co. Rep. No. 71-90.
- Kelly DP, Trochimowicz HJ. 1976. Two-Week Inhalation Toxicity Studies FC21 and FC 123. Newark, DE: Haskell Laboratory, E.I. du Pont de Nemours & Co.. Rep. No. 149-76.
- Kennedy GL. 1979. Acute Inhalation Toxicity Study of Tetrafluoroethane (FC 134a), Newark, DE: Haskell Laboratory, E.I. du Pont de Nemours & Co. Rep. No. 422-79.
- Kennelly JC. 1993. Assessment for the Induction of Unscheduled DNA Synthesis in Rat Liver After Inhalation Exposure. Cheshire, UK: Zeneca Central Toxicology Laboratory, Alderley Park, Macclesfield.
- Ko M, Shia RL, Sze N-D, Magid H, Bray RG. 1999. Atmospheric lifetime and global warming potential of HFC-245fa. J Geophys Res.104:8173–8181.
- Koops A, Krahn DF. 1977. Mutagenic Activity of Ethane Chlorodifluoro in the Salmonella/Microsome Assay. Newark, DE.: Haskell Laboratory, E.I. du Pont de Nemours & Co. Rep. No. 578-77.
- Koster U. 1996. The biotransformation of the aerosol propellant 1,1,1,2,3,3,3-heptafluoropropane (HFA-227)—lack of protein-binding of the metabolite hexafluoroacetone. Drug Metab Dispos. 24:906–910.
- Krahn DF. 1977. Mutagenic Activity of Methane, Chlorodifluoro, in the Salmonella Microsome Assay. Newark, DE: Haskell Laboratory, E.I. du Pont de Nemours & Co. Rep. No. 577-77.
- Krahn DF, Barsky FC, McCooey KT. 1982. CHO/HGPRT mutation assay: evaluation of gases and volatile liquids. Environ Sci Res. 25:91–103.
- Kumar S, Joginpally T, Kim D, Yadava M, Norgals K, Laird-Fick H. 2016. Cardiomyopathy from 1,1-Difluoroethane inhalation. Cardiovasc Toxicol. 4:370–373.
- Kuspis DA, Krenzelok EP. 1999. Oral frostbite injury from intentional abuse of a fluorinated hydrocarbon. J Toxicol Clin Toxicol. 37:873–875.
- Lashof DA, Ahuja DR. 1990. Relative contributions of greenhouse gas emissions to global warming. Nature (London). 344:529–531.
- Lee J, Lee C, Kim CH. 2009. Uncontrolled occupational exposure to 1,1-dichloro-1-fluoroethane (HCFC-141b) is associated with acute pulmonary toxicity. Chest. 135:149–155.
- Lee KP, Suzuki K. 1981. Studies on the male reproductive toxicity of Freon 22. Fundam Appl Toxicol. 1:266–270.
- Lessard Y, Desbrousses S, Paulet G. 1977. Arythmie cardiaque chez le chien sous I’action de I’adrénaline et du difluorodichloromethane (FC-12). C. R. Seances Soc Biol Ses Fil. 171:1270–1282.
- Lessard Y, Paulet G. 1986. A proposed mechanism for cardiac sensitization: electrophysiological study of effects of difluorodichloromethane and adrenaline on different types of cardiac preparations isolated from sheep hearts. Cardiovasc Res. 20:807–815.
- Lester D, Greenberg LA. 1950. Acute and chronic toxicity of some halogenated derivatives of methane and ethane. Arch Ind Hyg Occup Med. 2:335–344.
- Leuschner F, Neumann BW, Hübscher F. 1983. Report on subacute toxicological studies with several fluorocarbons in rats and dogs by inhalation. Arzneimittelforschung. 33:1475–1476.
- Lillian D, Singh HB, Appleby A, Lobban L, Arnts R, Gumpert R, Hague R, Toomey J, Kazazis J. 1975. Atmospheric fates of halogenated compounds. Environ Sci Technol. 9:1042–1048.
- Linder RL, Lerner SF, Wesson DR. 1974. Solvent sniffing: a continuing problem among youth. J Drug Educ. 4:469–473.
- Litchfield MH, Longstaff E. 1984. The toxicological evaluation of chlorofluorocarbon 22 (CFC 22). Food Chem Toxicol. 22:465–475.
- Loizou GD, Urban G, Dekant W, Anders MW. 1994. Gas-uptake pharmacokinetics of 2,2-dichloro-1,1,1-trifluoroethane (HCFC-123). Drug Metab Dispos. 22:511–517.
- Loizou GD, Anders MW. 1993. Gas-uptake pharmacokinetics and biotransformation of 1,1-dichloro-1-fluoroethane (HCFC-141b)). Drug Metab Dispos. 21:634–639.
- Loizou GD, Anders MW. 1995. Gas-uptake pharmacokinetics and metabolism of 2-chloro-1,1,1,2-tetrafluoroethane (HCFC-124) in the rat, mouse, and hamster. Drug Metab Dispos. 23:875–880.
- Longstaff E, Robinson M, Bradbrook C, Styles JA, Purchase IF. 1984. Genotoxicity and carcinogenicity of fluorocarbons: assessment by short-term in vitro tests and chronic exposure in rats. Toxicol Appl Pharmacol. 72:15–31.
- Longstaff E, McGregor D. 1978. Mutagenicity of a halocarbon refrigerant monochlorodifluoromethane (R-22) in Salmonella Typhimurium. Toxicol Lett. 2:1–4.
- Lu M. 1981. 1,1,1,2-Tetrafluoroethane (FC-134a): embryo-foetal toxicity and teratogenicity study by inhalation in the rat. Newark, DE: Newark, DE.: Haskell Laboratory, E.I. du Pont de Nemours & Co. (unpublished data).
- Mackay JM. 1992. HCFC 123: An Evaluation in the In Vitro Cytogenetic Assay. Alderley Park, Cheshire, UK: Imperial Chemical Industries, Central Toxicology Laboratory.
- Maeng S-H, Kim H-Y, Chung H-W, Kim S-J, Han J-H, Lee Y-M, Kim K-J, Yu I-J. 2004. Micronuclei induction by 13 week-inhalation of 1,1-dichloro-1-fluoroethane in Sprague-Dawley rats. Toxicol Lett. 146:129–137.
- MAK. 1996. 1,1,1,2-Tetrafluoethan Toxikolgisch-Arbeits Medizinsche. Weinheim: Begrundung von MAK-Werten, VCH Verlag.
- Malinverno G, Rusch GM, Millischer RJ, Hughes EW, Schroeder RE, Coombs DW. 1996. Inhalation teratology and reproduction studies with 1,1-dichloro-2,2,2-trifluoroethane (HCFC-123). Fundam Appl Toxicol. 34:276–287.
- Malley LA, Carakostas M, Hansen JF, Rusch GM, Kelly DP, Trochimowicz HJ. 1995. Two-year inhalation toxicity study in rats with hydrochlorofluorocarbon 123 (HCFC-123). Fundam Appl Toxicol. 25:101–114.
- Malley LA, Carakostas M, Elliott GS, Alvarez L, Schroeder RE, Frame SR, Van Pelt C, Trochimowicz HJ, Rusch GM. 1996. Subchronic toxicity and teratogenicity of 2-chloro-1,1,1,2-tetrafluoroethane (HCFC-124). Fundam Appl Toxicol. 32:11–22.
- Malley LA, Frame SR, Elliott GS, Bentley KS, Brock WJ, Trochimowicz HJ, Rusch GM. 1998. Chronic toxicity, oncogenicity, and mutagenicity studies with chlorotetrafluoroethane (HCFC-124). Drug Chem Toxicol. 24. 21:417–447.
- Maltoni C, Lefemine G, Tovoli D, Perino G. 1988. Long term carcinogenicity bioassays on three chlorofluorocarbons (trichlorofluoromethane, FC-11; dichlorodifluoromethane, FC-12; chlorodifluoromethane, FC-22) administered by inhalation to Sprague–Dawley rats and Swiss mice. Ann NY Acad Sci. 534:261–282.
- Maltoni C, Ciliberti A, Carretti D. 1982. Experimental contributions in identifying brain potential carcinogens in the petrochemical industry. Ann NY Acad Sci. 381:216–249.
- Marier G, MacFarland GH, Dussault P. 1973. A study of blood fluorocarbon levels following exposure to a variety of household aerosols. Household Pers Prod Ind. 10:68, 70, 92, 99.
- Marit GB, Dodd DE, George ME, Vinegar A. 1994. Hepatotoxicity in guinea pigs following acute inhalation exposure to 1,1-dichloro-2,2,2-trifluoroethane. Toxicol Pathol. 22:404–414.
- Marshall RR. 1992. Evaluation of chromosomal aberration frequencies in peripheral blood lymphocytes from rats treated with HCFC 123. North Yorkshire, UK: Hazleton Microtest, Harrogate.
- May DC, Blotzer MJ. 1984. A report of occupational deaths attributed to fluorocarbon-113. Arch Environ Health. 39:352–354.
- McAlack JW, Schneider PW. 1982. Two-Year Inhalation Study with 1,1-Difluoroethane (FC 152a) in Rats (unpublished report 8-82). Newark, DE: Haskell Laboratory, E.I. du Pont de Nemours & Co.
- McClure DA. 1972. Failure of fluorocarbon propellants to alter the electrocardiogram of mice and dogs. Toxicol Appl Pharmacol. 22:221–230.
- McCooey KT. 1980. Methane Dichlorodifluoro, Chinese Hamster Ovary Cell Assay for Mutagenicity. Newark, DE: Haskell Laboratory, E.I. du Pont de Nemours & Co. Rep. No. 149-90.
- McKnight JE, McGraw JL. 1983. Ultrastructural study of the effects of trichlorotrifluoroethane on the liver of hairless mice. J Submicrosc Cytol. 15:447–451.
- Mecler FJ, Knapinsky PJ. 1978. Acute Inhalation Toxicity Study in Rat. Isotron 142b, LBI Proj. No. 20888. Kensington, MD: Litton Bionetics.
- Mercier O. 1990a. HFA-134a: Test to Evaluate the Ocular Irritation in the Rabbit. La Defense Paris: Hazleton, France for Elf Atochem. Rep. No. 912349.
- Mercier O. 1990b. HFA-134a: Test to Evaluate the Sensitizing Potential by Topical Applications in the Guinea Pig. The Epicutaneous Maximization Test. La Defense Paris: Hazleton, France for Elf Atochem. Rep. No. 001380.
- Mercier O. 1989. HFA-134a: Test to Determine the Index of Primary Cutaneous Irritation in the Rabbit. Paris: Hazleton, France for Elf Atochem, La Defense. Rep. No. 911422.
- Mergner GW, Blake DA, Helrich M. 1975. Biotransformation and elimination of 14C-trichlorofluoromethane (FC-11) and 14C-dichlorodifluoromethane (FC-12) in man (Laboratory Report). Anaesthesiology. 42:345–351.
- Meridian. 1991. Results of Employee Exposure Monitoring for HCFC 123 at Centrifugal Chiller Installations, Final Report 68-D90068. Washington, DC: Global Change Division, Office of Atmospheric and Indoor Air Programs, Office of Air and Radiation, USEPA.
- Millischer RJ, de Rooij CG, Rush GM, Farr CH, Ben-Dyke R, Hardy CJ, Lewis DJ, Hodson-Walker G. 1995. Evaluation of the genotoxicity potential and chronic inhalation toxicity of 1,1-dichloro-1-fluoroethane (HCFC-141b)). Food Chem Toxicol. 33:491–500.
- Millischer RJ, Jepson G, Rusch GM, Schmit B, Farrar D, Regnier JF, Binaglia M, Bingham P. 2007. Maximum recommended guidance levels (MRGLs) for organohalogenated impurities potentially present in fluorocarbons. Abstract 1521. Toxicologist. 96:315.
- Molina MJ, Rowland FS. 1974. Stratospheric sink for chlorofluoromethanes: chlorine atom catalyzed destruction of ozone. Nature (London). 249:810–812.
- Monté SY, Ismail I, Mallett DN, Matthews C, Tanner RJN. 1994. The minimal metabolism of inhaled 1,1,1,2-tetrafluoroethane to trifluoroacetic acid in man. J Pharma Biomed Anal. 12:1489–1493.
- Moore BL. 1975. Acute Inhalation Toxicity (unpublished report 699-75). Newark, DE: Haskell Laboratory, E.I. du Pont de Numours & Co.
- Moore BL, Trochimowicz HJ. 1976. Subacute (Two-Week) Inhalation Toxicity. Newark, DE.: Haskell Laboratory, E.I. du Pont de Nemours & Co. Rep. No. 145-76.
- Moreno C, Beierle EA. 2007. Hydrofluoric acid burn in a child from a compressed air duster. J Burn Care Res. 28:909–912.
- Morgan A, Black A, Walsh M, Belcher DR. 1972. The absorption and retention of inhaled fluorinated hydrocarbon vapours. Int J Appl Radiat Isot. 23:285–291.
- Morita M, Miki A, Kazama H, Sakata M. 1977. Case report of deaths caused by Freon gas. Forensic Sci. 10:253–260.
- Muller W, Hofmann T. 1988. HCFC 123—Micronucleus Test in Male and Female NMRI Mice after Inhalation. Germany: Pharma Research Toxicology and Pathology, The Hoechst Research Laboratory, Hoechst AG, Kastengrund.
- Muller W, Hofmann T. 1989. CFC 134a: Micronucleus Test in Male and Female NMRI Mice after Inhalation. Germany: Pharma Research Toxicology and Pathology, The Hoechst Research Laboratory, Hoechst AG, Kastengrund. Study No. 88. 1244.
- Mullin LS. 1969. (unpublished data). Newark, DE: Haskell Laboratory, E.I. du Pont de Nemours & Co.
- Mullin LS. 1976. Cardiac Sensitization. Newark, DE.: Haskell Laboratory, E.I. du Pont de Nemours & Co. Rep. No. 113-76.
- Mullin LS. 1977. Cardiac Sensitization (HFA 141b), Newark, DE.: Haskell Laboratory, E.I. du Pont de Nemours & Co. Rep. No. 957-77.
- Mullin LS. 1979. FC 134a: Cardiac Sensitization. Newark, DE.: Haskell Laboratory, E.I. du Pont de Nemours & Co.
- Mullin L, Azar A, Reinhardt C, Smith P, Fabryka E. 1972. Halogenated hydrocarbon-induced cardiac arrhythmias associated with release of endogenous epinephrine. Am Ind Hyg Assoc J. 33:389–396.
- Myhre G, D, Shindell F-M, Bréon W, Collins J, Fuglestvedt J, Huang D, Koch J.-F L, D L, B, Mendoza, et al. 2013. Anthropogenic and Natural Radiative Forcing. In: Stocker, T.F., D. Qin, G.-K. Plattner, M. Tignor, S.K. Allen, J. Boschung, A. Nauels, Y. Xia, V. Bex and P.M. Midgley (editors). Climate Change 2013: The Physical Science Basis. Contribution of Working Group I to the Fifth Assessment Report of the Intergovernmental Panel on Climate Change. United Kingdom and New York, NY, USA: Cambridge University Press, Cambridge.
- National Aeronautics and Space Administration NASA. 1986. Present State of Knowledge of the Upper Atmosphere: An Assessment Report. Washington, DC: NASA. Ref. Publ. No. 1162.
- NCI. 1978. National Cancer Institute, Bioassay of Trichlorofluoromethane for Possible Carcinogenicity, Case No. 75-69-4, NCI Carcinoa. Tech. Rep. Ser. No. 106, NTIS Pub. No. PB-286-187, National Technical Information Service, Springfield, VA.
- Nemec M. 1994. An assessment of the potential developmental toxicity of heptafluoropropane (FM-200.RTM) by inhalation in the rat. Teratology. 49:420.
- Niazi S, Chiou WL. 1975. Fluorocarbon aerosol propellants. IV. Pharmacokinetics of trichloromonofluoromethane following single and multiple dosing in dogs. J Pharm Sci. 64:763–769.
- Niazi S, Chiou WL. 1977. Fluorocarbon aerosol propellants XI: Pharmacokinetics of dichlorodifluoromethane in dogs following single and multiple dosing. J Pharm Sci. 66:49–53.
- Nielsen OJ, Javadi MS, Sulbaek Andersen MP, Hurley MD, Wallington TJ, Singh R. 2007. Atmospheric chemistry of CF3CF = CH2: kinetics and mechanisms of gas phase reactions with Cl atoms OH radicles and O3. Chem Phys Lett. 439:18–22.
- Nikitenko TK, Tolgskaya MS. 1965. Toxicologically induced pathomorphic changes in animals affected by freons—141, 142, 143 and intermediate products arising in the course of their production. Gig Tr Prof Zabol. 9:37–44.
- National Institute for Occupational Safety and Health (NIOSH). 1980. Health Hazard Evaluation Determination. NIOSH: Cincinnati, OH. Rep. No. 79-127-644, NTIS PB 80-169816.
- Nuckolls AH. 1993. The Comparative Life, Fire, and Explosion Hazards of Common Refrigerants, Misc. Hazard No. 2375. Chicago, IL: Underwriters Laboratories; p. 5–9, 24–26, 57–61, 106–107.
- OECD www.oecd-library.org/environment/oecd-guidelines-for-the-testing-of-chemicals-section-4-health-effects
- Olson MJ. 1990. Oxidation of 1,1,1,2-Tetrafluoroethane (R-134a) in Rat Live Microsomes is Catalyzed Primarily by Cytochrome P45011E1. Michigan: General Motors Research Laboratories.
- Olson MJ, Johnson JT, O'Gara JF, Surbrook SE. 1991. Metabolism in vivo and in vitro of the refrigerant substitute 1,1,1,2-tetrafluoro-2-chloroethane. Drug Metab Dispos. 19:1004–1011.
- Olson MJ, Reidy CA, Johnson JT. 1990. Defluorination of 1,1,1,2-tetrafluoroethane (R-134a) by rat hepatocytes. Biochem Biophys Res Commun. 166:1390–1397.
- Palmer AK. 1978a. Effect of Arcton 22 on Pregnant Rats: Relationship to Anophthalmia and Microphthalmia. Cambridgeshire, UK: Huntingdon Research Centre, Huntingdon. Rep. No. ICI 174/78208.
- Palmer AK. 1978b. Effect of Arcton 22 on Pregnancy of the New Zealand White Rabbit. Cambridgeshire, UK: Huntingdon Research Centre, Huntingdon. Rep. No. ICI 177/78505.
- Pantaleoni GC, Luzi V. 1975. Cardiotoxicity of monochlorodifluoromethane (contribution to the study on the toxicopathology of sniffing syndrome). Note 3. Rass Med Sper. 22:255–269.
- Paterson JW, Sudlow MF, Walker SR. 1971. Blood levels of fluorinated hydrocarbons in asthmatic patients after inhalation of pressurized aerosols. Lancet. 298:565–568.
- Patten KO, Wuebbles DJ. 2010. Atmospheric lifetimes and Ozone Depletion Potentials of trans-1-chloro-3,3,3-trifluoropropylene and trans-1,2-dichloroethylene in a three-dimensional model. Atmos Chem Phys. 10:10867–10874.
- Paulet G. 1969. De l’action des hydrocarbures chlorofluorés sur l’organism. Labo-Pharma Probl Tech. 180:74–78.
- Paulet G, Lanoë J, Thos A, Toulouse P, Dassonville J. 1975. Fate of fluorocarbons in the dog and rabbit after inhalation. Toxicol Appl Pharmacol. 34:204–213.
- Paulet G, Chevrier R. 1969. Modalités de l’limination par l’air expiré du fluorane 11 inhalé. Etude chez l’homme et chez l’animal. Arch Mal Prof Méd Trav Secur Soc. 30:251–256.
- Paulet G, Desbrousses S. 1969. Dichlorotetrafluoroethane. Acute and chronic toxicity. Arch Mal Prof Méd Trav Secur Soc. 30:477–492.
- Peter H, Filser JG, von Szentpály L, Wiegand HJ. 1986. Different pharmacokinetics of dichlorofluoromethane (CFC 21) and chlorodifluoromethane (CFC 22). Arch Toxicol. 58:282–283.
- Pike VW, Aigbirhio FI, Freemantle CA, Page BC, Rhodes CG, Waters SL, Jones T, Olsson P, Ventresca GP, Tanner RJ, et al. 1995. Disposition of inhaled 1,1,1,2-tetrafluoroethane (HFA134A) in healthy subjects and in patients with chronic airflow limitation. Measurement by 18F-labeling and whole-body gamma-counting. Drug Metab Dispos. 23:832–839.
- Pohl LR, Satoh H, Christ DD, Kenna JG. 1988. The immunologic and metabolic basis of drug hypersensitivities. Annu Rev Pharmacol Toxicol. 28:367–387.
- Prendergast JA, Jones RA, Jenkins LJ, Siegel J. 1967. Effects on experimental animals of long-term inhalation of trichloroethylene, carbon tetrachloride, 1,1,1-trichloroethane, dichlorodifluoromethane, and 1,1-dichloroethylene. Toxicol Appl Pharmacol. 10:270–289.
- Quevauviller A. 1965. Hygiéne et séurité des pulseurs pour aérosols médicamenteux. Prod Probl Pharm. 20:14–29.
- Quevauviller A, Schrenzel M, Vu-Ngoc H. 1964. Local tolerance to chlorofluorohydrocarbons by the animal (Tolérance local (peau, muqueuses, plaies, brûlures) chez l’animal aux hydrocarbures chlorofluorés). Thérapie. 19:247–263.
- Raffi GB, Violante FS. 1981. Is Freon 113 neurotoxic? A case report. Int Arch Occup Environ Heath. 49:125–127.
- Raventos J, Lemon PG. 1965. The impurities in Fluothane: their biological properties. Br J Anaesth. 37:716–737.
- Reinhardt CF, Azar A, Maxfield ME, Smith PE, Mullin LS. 1971a. Cardiac arrhythmias and aerosol “sniffing”. Arch Environ Health. 22:265–279.
- Reinhardt CF, McLaughlin M, Maxfield ME, Mullin LS, Smith PE. 1971b. Human exposures to fluorocarbon 113 (1,1,2-trichloro-1,2,2-trifluoroethane). Am Ind Hyg Assoc J. 32:143–152.
- Reinhardt CF, Mullin LS, Maxfield ME. 1973. Epinephrine-induced cardiac arrhythmia potential of some common industrial solvents. J Occup Med 15:953–955.
- Rickard LB. 1990. Mouse Bone Marrow Micronucleus Assay of HCFC-124. Newark (DE): Haskell Laboratory, E.I. du Pont de Nemours & Co. Rep. No. 52-90.
- Ritchie GD, Kimmel EC, Bowen LE, Reboulet JE, Rossi JIII. 2001. Acute neurobehavioral effects in rats from exposure to HFC 134a or CFC 12. Neurotoxicology. 22:233–248.
- Rowland FS. 1990. Stratospheric ozone depletion by chlorofluorocarbons. Ambio. 19:281–292.
- Rusch GM. 1990. Results from the Program for Alternative Fluorocarbon Toxicology Testing Activities: DECHEMA DCKW-Ausstieg Wohin? (CFC Phaseout Whereto?). Frankfurt Germany: DECEMA; p. 245–261.
- Rusch GM. 2002. Organic chlorofluoro hydrocarbons. In: Bingham E, Cohrssen B, editors. Patty’s toxicology. Chapter 46, Vol. 3. Hoboken (NJ): John Wiley & Sons. p. 359–427.
- Rusch GM, Coombs DW, Hardy C. 1999. The acute, genetic, developmental and inhalation toxicology of 1,1,1,3,3-pentafluoropropane. Toxicol Sci. 52:289–301.
- Rusch GM, Millischer RJ, de Rooij C, Brooker AJ, Hughes E, Coombs D. 1995. Inhalation teratology and two-generation reproduction studies with 1,1-dichloro-1-fluoroethane (HCFC-141b). Food Chem Toxicol. 33:285–300.
- Rusch GM, Trochimowicz HJ, Malley LJ, Kelly DP, Peckham J, Hansen J, Charm JB. 1994. Subchronic inhalation toxicity studies with hydrochlorofluorocarbon 123 (HCFC 123). Fundam Appl Toxicol. 23:169–178.
- Rusch GM, Tveit A, Muijser H, Tegelenbosch-Schouten M, Hoffman GM. 2013. The acute, genetic, developmental and inhalation toxicology of 1,3,3,3-tetrafluoropropene (HFO-1234ze(E)). Drug and Chemical Toxicol. 36:170–180.
- Russe BR, McCoy CB, Barton JE. 1980. Recent findings concerning inhalant use. Chem Depend Behav Biomed Issues. 4:113–126.
- Russell JF, Sippel ME, Krahn DF. 1980. Methods for the detection of mutagenic gases and volatile liquids in the Salmonella/microsome assay. Environ Mutagen. 2:307.
- Sakata M, Kazama H, Miki A, Yoshida A, Haga M, Morita M. 1981. Acute toxicity of fluorocarbon-22: toxic symptoms, lethal concentration, and its fate in rabbit and mouse. Toxicol Appl Pharmacol. 59:64–70.
- Salmon AG, et al. 1979. Arcton 22: Metabolism Study In Vivo in Rats and In Vitro. Alderley Park, Cheshire, UK: Imperial Chemical Industries, Central Toxicology Laboratory. Rep. No. CTL/P/438.
- Salmon AG, Nash JA, Walklin CM, Freedman RB. 1985. Dechlorination of halocarbons by microsomes and vesicular reconstituted cytochrome P-450 systems under reductive conditions. Br J Ind Med. 42:305–311.
- Sandow J. 1996. Effect of Fluorocarbons on Pituitary-Gonadal Function in a 14-Day Inhalation Toxicity Study—HCFC 123. Hoechst Study No. 95.0089 (unpublished data), Hoechst A. G. Kastengrund, Germany.
- Sarver JW. 1988. Acute Oral Toxicity Study with FC-141b Male Rats. Newark, DE.: Haskell Laboratory, E.I. du Pont de Nemours & Co. Rep. No. HRL 363-88, E.I.
- Sayers RR, Yant WP, Chornyak J, Shoaf HW 1930. Toxicity of Dichlorofluoromethane: A New Refrigerant, Rep. Invest. No. 3013. Washington, DC: Bureau of Mines, Publications Distribution Section.
- Schmidt T, Bertermann R, Rusch GM, Hoffman GM, Dekant W. 2012. Biotransformation of 2,3,3,3-tetrafluoropropene (HFO-1234yf) in male, pregnant and non-pregnant female rabbits after single high dose inhalation exposure. Toxicol Appl Pharmacol. 263:32–38.
- Schmidt T, Bertermann R, Rusch GM, Tveit A, Dekant W. 2013. Biotransformation of trans-1-chloro-3,3,3-trifluoropropene (trans-HCFO-1233zd). Toxicol Appl Pharmacol. 268:343–351.
- Schmoltner AM, Talukdar RK, Warren RF, Mellouki A, Goldfarb L, Gierczak T, McKeen SA, Ravishankara AR. 1993. Rate coefficients for reactions of several hydrofluorocarbons with OH and O(1D) and their atmospheric lifetimes. J Phys Chem. 97:8976–8982.
- Scholz J. 1962. New toxicological investigations on certain types of freon used as propellants. Fortschr Biol Aerosol Forsch Jahren 1957–1961. 4:420–429.
- Schuster P, Bertermann R, Rusch GM, Dekant W. 2009. Biotransformation of trans-1,1,1,3-tetrafluoropropene (HFO-1234ze). Toxicol Appl Pharmacol. 239: 215–223.
- Schuster P, Bertermann R, Rusch GM, Dekant W. 2010. Biotransformation of 2,3,3,3-tetrafluoropropene (HFO-1234yf) in rabbits. Toxicol. App Pharm. 244: 247–253.
- Seckar JA, Trochimowicz HJ, Hogan GK. 1986. Toxicological evaluation of hydrochlorofluorocarbon 142b. Food Chem Toxicol. 24:237–240.
- Sherman H. 1974. Long-Term Feeding Studies in Rats and Dogs with Dichlorodifluoromethane (Freon 12 Food Freezant). Newark (DE): Haskell Laboratory, E.I. du Pont de Nemours & Co. Med. Res. Proj. No. 1388. Rep. No. 24-74, unpublished.
- Shulman M, Sadove MS. 1967. 1,1,1,2-tetrafluoroethane: an inhalation anesthetic agent of intermediate potency. Anesth Analg. 46:629–633.
- Singh HB, Salas LJ, Shigeishi H, Scribner E. 1979. Atmospheric halocarbons hydrocarbons, and sulfur hexafluoride: global distributions, sources, and sinks. Science. 203:899–903.
- Singh HB, Salas LJ, Stiles RE. 1983. Selected man-made halogenated chemicals in the air and oceanic environment. J Geophys Res. 88:3675–3368.
- Skaggs SR, Moore TA, Tapscott RE. 1995. Toxicological properties of halon substitutes, In A. J. Miziolerk and W. Tsang (editors). Halon Properties, ACS Symp. Ser. 611. Washington (DC): American Chemical Society; p. 99–109.
- Skuric Z, Zuskin E, Valic F. 1975. Effects of aerosols in common use on the ventilatory capacity of the lung. Int Arch Arbeitsmed. 34:137–149.
- Slater TE. 1965. A note on the relative toxic activities of tetrachloromethane and Trichloro-fluro-methane on the rat. Biochem Pharmacol. 14:178–181.
- Smith LW, Gardner RJ, Kennedy GL. Jr. 1982. Short-term inhalation toxicity of perfluoroisobutylene. Drug Chem Toxicol. 5:295–303.
- SORG. 1987. Stratospheric Ozone Review Group, Stratospheric Ozone 1987. London: HMSO Publications Centre.
- SORG. 1993. Stratospheric Ozone Review Group, Stratospheric Ozone 1993. London: HMSO Publications Centre.
- SPARC. 2013. Report on the Lifetimes of Stratospheric Ozone-Depleting Substances, Their Replacements, and Related Species. In: M. Ko, P. Newman, S. Reimann, S. Strahan, editors. German Aerospace Center, Institut Fur Physik der Atmosphare, Muchener Strasse 20, D-82234 Oberpfaffenhofen, Germany. SPARC Report No. 6, WCRP-15/2013.
- Standing P, et al. 1995. 1,1,1,2-Tetrafluoroethane HFC 134a Criteria Doc. for an Occupational Exposure Limit. Sudbury, Suffolk, UK: HSE Books.
- Stewart RD, Newton PE, Baretta ED, Herrmann AA, Forster HV, Soto RJ. 1978. Physiological response to aerosol propellants. Environ Health Perspect. 26:275–285.
- Stopps GJ, McLaughlin M. 1967. Psychophysiological testing of human subjects exposed to solvent vapors. Am Ind Hyg Assoc J. 28:43–50.
- Szmidt M, Axelson O, Edling C. 1981. Cohort study of Freon-exposed workers. Acta Soc Med. Svec Hyg. 90:77–79.
- Takebayashi T, Kabe I, Endo Y, Tanaka S, Miyauchi H, Nozi K, Takahashi K, Omae K. 1998. Acute liver dysfunction among workers exposed to 2,2-dichloro-1,1,1-trifluoroethane (HCFC 123). J Occup Health. 40:169–170.
- Taylor GJ, Drew RT. 1974. Cardiovascular effects of acute and chronic inhalations of fluorocarbon 12 in rabbits. J Pharmacol Exp Ther. 192:129–135.
- Taylor GJ, Harris WS. 1970. Cardiac toxicity of aerosol propellants. JAMA. 214:81–85.
- Taylor GJ, Harris WS, Bogdonoff MD. 1971. Ventricular arrhythmias induced in monkeys by the inhalation of aerosol propellants. J Clin Invest. 50:1546–1550.
- The Trane Company. 1992. Report of Worker Exposure to HCFC 123 During Servicing of Hermetic Centrifugal Chillers. La Crosse, WI.: The Trane Company.
- Thomas G. 1965. Narcotic effects of acute exposure to trichlorofluoromethane (Freon 11). Trans Assoc Ind Med off. 15:105–106.
- Thomas R. 2007. Toxicogenomic Assessment of the Carcinogenic Potential of HFO-1234ze. Report 06014. Study conducted by Honeywell International by The Hamner Institute, Research Triangle Park, NC. Ref. MA-RR-07-3453.
- Tinston DJ, et al. 1981a. Chlorodifluoromethane (CFC 22): Long-Term Inhalation Study in the Mouse. Alderley Park, Cheshire, UK: Imperial Chemical Industries, Central Toxicology Laboratory. Rep. No. CTL/P/547.
- Tinston DJ, et al. 1981b. Chlorodifluoromethane (CFC 22): Long-term Inhalation Study in the Rat. Alderley Park, Cheshire, UK: Imperial Chemical Industries, Central Toxicology Laboratory. Rep. No. CTL/P/548.
- TKL. 2010. Repeat Insult Patch Study. TKL Research Study Number DS 100410 MA-RR-10-4300.
- TKL. 2012. Repeat Insult Patch Study. TKL Research Study Number 103122, [HCFO-1233zd (E)] MA-RR-12-4343.
- TLV. 1991. Documentation of the Threshold Limit Values and Biological Exposure Indices: Trichlorofluoromethane. 6th ed. Cincinnati, OH.: American Conference of Governmental and Industrial Hygienists.
- TNO. 2008. A 2-Generation inhalation, reproduction study in Wistar rats with HFO-1234yf. Interim report TNO Quality of Life (conducted for Honeywell International) Zeist, The Netherlands.
- Tong Z, Utell MJ, Morrow PE, Rusch GM, Anders MW. 1998. Metabolism of 1,1-dichloro-1-fluoroethane (HCFC-141b) in human volunteers. Drug Metab Dispos. 26:711–713.
- Trochimowicz HJ. 1978. Du Pont (personal communication) Newark, DE.: Haskell Laboratory, E.I. du Pont de Nemours & Co.
- Trochimowicz HJ. 1984. The Toxicology of Fluorocarbon 113, presented to the Swedish Board of Occupational Safety and Health, Stockholm: Haskell Laboratory for Toxicology and Industrial Medicine for E.J. du Pont de Nemours & Co.
- Trochimowicz HJ. 1988. Chronic inhalation toxicity/carcinogenicity study in rats exposed to fluorocarbon 113 (FC-113). Fundam Appl Toxicol. 11:68–75.
- Trochimowicz HJ. 1989. The Toxicology of HCFC-123 (2,2-Dichloro-1,1,1-Trifluoro-Ethane), presented at the 1989 European Meeting of the Toxicology Forum, Toulouse, France, September 19–22.
- Trochimowicz HJ. 1993. Industrial research on alternative fluorocarbons. Toxicol Lett. 68:25–30.
- Trochimowicz HJ, Reinhardt CF, Mullin LS, Azar A, Karrh BW 1976. The effect of myocardial infarction on the cardiac sensitization potential of certain halocarbons. J Occup Med 18:26–30.
- Trochimowicz HJ, Azar A, Terrill JB, Mullin LS. 1974. Blood levels of fluorocarbon related to cardiac sensitization: II. Am Ind Hyg Assoc J. 35:632–639.
- Trochimowicz HJ, Moore BL, Chiu T. 1977. Subacute inhalation toxicity studies on eight fluorocarbons. Toxicol Appl Pharmacol. 41:198–199.
- Trochimowicz HJ, Mullin LS. 1973. Cardiac Sensitization of Fluorocarbon 123. Newark (DE): Haskell Laboratory, E.I. du Pont de Nemours & Co. Rep. No. 132-73, E.I.
- Trueman RW. 1990. Fluorocarbon 134a: Assessment for the Induction of Unscheduled DNA Synthesis in Rat Hepatocytes In Vivo. Cheshire, UK.: Imperial Chemical Industries, Central Toxicology Laboratory, Alderley Park. CTL Study No. SR0337.
- Tveit A, Rusch GM, Muijser H, Tegelenbosch-Schouten M. 2013. The Acute, Genetic, Developmental and Inhalation Toxicology of 2,3,3,3-tetrafluoropropene (HFO-1234yf). Drug and Chemical Toxicol. 36:412–420.
- Tveit A, Rusch GM, Muijser H, van den Hoven M, Hoffman GM. 2014. The acute, genetic, developmental and inhalation toxicology of trans-1-chloro,3,3,3-trifluoropropene (HCFO-1233zd(E))). Drug Chem Toxicol. 37:83–92.
- Uehleke H, Werner T, Greim H, Krämer M. 1977. Metabolic activation of haloalkanes and tests in vitro for mutagenicity. Xenobiotica. 7:393–400.
- UNEP. 2014. Report of the Refrigeration, Air Conditioning and Heat Pumps Technical Options Committee, Lambert Kuijpers and Robert Peixoto (Co-chairs) Montreal Protocol Secretariat. Nairobi, Kenya: UNEP. 2015. ISBN: 978-9966-076-09-0.
- UNEP/WMO. 1989. Scientific Assessment of Stratospheric Ozone: 1989, Sect. III, Ch. 4. Geneva: United Environment Programme, World Meteorological Organization. Report dated August 21, 1989.
- Urban G, Speerschneider P, Dekant W. 1994. Metabolism of the chlorofluorocarbon substitute 1,1-dichloro-2,2,2-trifluoroethane by rat and human liver microsomes: the role of cytochrome P450 2E1. Chem Res Toxicol. 7:170–176.
- Valentine R, et al. 1996. Toxicological profile on the alternative refrigerant HFC-236fa. Toxicologist. 30:291.
- Valic F, Skuric Z, Bantic Z, Rudar M, Hecej M. 1977. Effects of fluorocarbon propellants on respiratory flow and ECG. Br. J. Ind Med. 34:130–136.
- Valic F, Skuric Z, Zuskin E. 1982. Experimental exposure to freons 12, 22, and 502. Red Jazu. 402:229–243.
- Van Dyke RA. 1974. Dechloriation mechanisms of chlorinated olefins. Environ Health Perspect. 21:121–124.
- Van Ketel WG. 1976. Allergic contact dermatitis from propellants in deodorant sprays in combination with ethyl chloride. Contact Dermatitis. 2:115–119.
- Van Poznak A, Artusio JF. Jr., 1960. Anesthetic properties of a series of fluorinated hydrocarbons. I. Fluorinated compounds. Toxicol Appl Pharmacol. 2:363–373.
- Vinegar A, Williams RJ, Fisher JW, McDougal JN. 1994. Dose-dependent metabolism of 2,2-dichloro-1,1,1-trifluoroethane: a physiologically based pharmacokinetic model in the male Fischer 344 rat. Toxicol Appl Pharmacol. 129:103–133.
- Vinegar A, Jepson GW, Overton JH. 1998. Inhalation of Halon 1301, HFC-134a, and HFC 227ea for Collection of Pharmacokinetic Data. Dayton, OH.: Mann. Tech Environmental Technology. Rep. NTIS/AD-A329 1994/4.
- Vinegar A, Jepson G. 1995. Gas Uptake Kinetics of 1,1,1,3,3,3-Hexafluoropropane (HFC-236fa) and Identification of Potential Metabolites. Dayten, OH.: Mann-Tech Environmental Technology. Rep. AL/OE-TR-1995-0177 (unpublished data).
- Vinegar A, Jepson G, Cisneeros M, Rubenstein T, Brock W. 2000. Setting safe acute exposure limits for halo replacement chemicals using physiologically based pharmacokinetic modeling. Inhal Toxicol. 12:751–763.
- Vlachos DA. 1989. Mouse Bone Marrow Micronucleus Assay of FC-141b. Newark, DE.: Haskell Laboratory, E.I. du Pont de Nemours & Co. Rep. No. HRL 746-88.
- Voge VM. 1997. Possible aircrew intoxication caused by accidental release of RainBoe: a case report. Aviat Space Environ Med. 68:1159–1160.
- von Weigand W. 1971. Untersuchungen eber die Inhalationstoxizitat von Fluorderivaten des Methan, Athan und Cyclobutan. Zentralbl Arbeitsmed. 21:149–156.
- Wada H. 1977. Anesthetic Activity and Fatality (F-123, 123a, 124 and 11), attachment to correspondence to M. B. Berenbaum, Allied Chemical Corporation, Morristown, NJ, from H. Wada, Daikin Kogy Co.
- Wagner B, Anger WK, Feigley C, Generosa W, Graves I, Snyder R, Wogan G, Yost G. 1996. Subcommittee to review toxicity of alternatives to chlorofluorocarbons. In: Crossgrove R, editor. Toxicity of Alternatives to Chlorofluorocarbons: HFC 134a and HCFC 123. Washington (DC): National Academy Press. p. 13–21.
- Warheit DB. 1993. Mechanistic Studies with HCFC-123. Newark, DE.: Haskell Laboratory, E.I. du Pont de Nemours & Co. Rep. HLR 828-92 (unpublished data).
- Waritz RS. 1971. Toxicology of Some Commercial Fluorocarbons. Springfield, VA: National Technical Information Service. NTIS; AD Rep. No. 751429,
- Watanabe T, Aviado DM. 1975. Toxicity of aerosol propellants in the respiratory and circulatory systems. VII. Influence of pulmonary emphysema and anesthesia in the rat. Toxicology. 3:225–236.
- Workplace Environmental Exposure Level (WEEL). 1991. Workplace Environmental Exposure Level Guide: 1,1-Dichloro-1-Fluoroethane. Fairfax (VA): American Industrial Hygiene Association.
- Workplace Environmental Exposure Level (WEEL). 1994. Workplace Environmental Exposure Level Guide: 1-Chloro-1,1-Difluoroethane. Fairfax, VA: American Industrial Hygiene Association.
- Workplace Environmental Exposure Level (WEEL). 1996. Workplace Environmental Exposure Level Guide: 1,1,1-Trifluoroethane. Fairfax, VA: American Industrial Hygiene Association.
- Workplace Environmental Exposure Level (WEEL). 1998. Workplace Environmental Exposure Level Guide: 1,1-Dichloro-2,2,2-Trifluoroethane. Fairfax, VA: American Industrial Hygiene Association.
- Workplace Environmental Exposure Level (WEEL). 1998a. Workplace Environmental Exposure Level Guide: 1,1,1,3,3,3-Hexafluoropropane. Fairfax, VA: American Industrial Hygiene Association.
- Workplace Environmental Exposure Level (WEEL). 2003. Workplace Environmental Exposure Level Guide: Tetrafluoroethane (HFC134a). Fairfax, VA: American Industrial Hygiene Association.
- Workplace Environmental Exposure Level (WEEL). 2005. WEEL Guide 1-Chloro-1,2,2,2-Tetrafluoro-Ethane (HCFC 124). Fairfax, VA: American Industrial Hygiene Association.
- Workplace Environmental Exposure Level (WEEL). 2009. Workplace Environmental Exposure Level Committee 2,3,3,3-tetrafluoropropene. Falls Church: American Industrial Hygiene Association.
- Workplace Environmental Exposure Level (WEEL). 2009. Workplace Environmental Exposure Level Guide: 1,1,1,3,3-Pentafluorocarbons. Fairfax, VA: American Industrial Hygiene Association.
- Workplace Environmental Exposure Level (WEEL). 2009. Workplace Environmental Exposure Level Guide: Pentafluoroethane. Fairfax, VA: American Industrial Hygiene Association.
- Workplace Environmental Exposure Level (WEEL). 2012. Workplace Environmental Exposure Level Committee, 1,3,3,3-Tetrafluropropylene. OARS WEEL, Workplace Environmental Exposure Level, Cincinnati, OH.
- Workplace Environmental Exposure Level (WEEL). 2012. Workplace Environmental Exposure Level Guide: Difluoroethane. Fairfax (VA): American Industrial Hygiene Association.
- Workplace Environmental Exposure Level (WEEL). 2013. Workplace Environmental Exposure Level, trans-1-Chloro-3,3,3-trifluoro propene. OARS WEEL, Workplace Environmental Exposure Level, Cincinnati, OH.
- Workplace Environmental Exposure Level (WEEL). 2018. Workplace Environmental Exposure Level Guide: 1,1-Difluoroethane. Fairfax, VA: American Industrial Hygiene Association.
- White JF, Carlson GP. 1981. Epinephrine-induced cardiac arrhythmias in rabbits exposed to trichloroethylene: role of trichloroethylene metabolites. Toxicol Appl Pharmacol. 60:458–465.
- WIL. 1996. Research Laboratory (WIL), Acute Inhalation Toxicity Study of HFC-236fa in Albino Rats. Rep. WIL-189022. WIL: Ashland, OH.
- WMO. 1991a. World Meteorological Organization, Global Ozone Research and Monitoring Project. Geneva: WMO. Rep. No. 25
- WMO. 1991b. World Meteorological Organization, Global Research and Monitoring Project. Geneva: WMO. Rep. No. 20. Vol. II, Appendix: AFEAS Report.
- WMO. 1999. World Meteorological Organization. 1998–1999 WMO Global Ozone Research and Monitoring Project. Geneva: WMO.
- WMO. 2007. World Meteorological Association, Scientific Assessment of Ozone Depletion: 2006, Global Ozone Research and Monitoring Project. Geneva, Switzerland. Report No. 50. p. 572.
- Wolf CR, Mansuy D, Nastainczyk W, Deutschmann G, Ullrich V. 1977. The reduction of polyhalogenated methanes by liver microsomal cytochrome P450. Mol Pharmacol. 13:698–705.
- Wolf CR, King LJ, Parke DV. 1978. The anaerobic dechlorination of trichlorofluoromethane by rat liver preparations in vitro. Chem Biol Interact. 21:277–288.
- Woollen BH. 1988. Arcton 22: Pharmacokinetics in the Pregnant Female Rat Following Inhalation Exposure. CTL report number CTL/L/1982 (unpublished data), ICI Alderly Part, Cheshire, England.
- Woollen BH, Guest EA, Howe W, Marsh JR, Wilson HK, Auton TR, Blain PG. 1990. Human inhalation pharmacokinetics of 1,1,2-trichloro-1,2,2-trifluoroethane (FC113)). Int Arch Occup Environ Health. 62:73–78.
- Woollen BH, Marsh JR, Mahler JD, Auton TR, Makepeace D, Cocker J, Blain PG. 1992. Human inhalation pharmacokinetics of chlorodifluoromethane (HCFC22)). Int Arch Occup Environ Health. 64:383–387.
- Xiong Z, Avella J, Wetli CV. 2004. Sudden death caused by 1,1-difluoroethane inhalation. J Forensic Sci. 49:627–629.
- Yant WP, Schrenk HH, Patty FA. 1932. Toxicity of Dichlorotetrafluoroethane. U.S. Bureau of Mines: Washington, DC. Rep. No. R.I. 3185.
- Zakhari S, Aviado DM. 1982. Cardiovascular toxicology of aerosol propellants, refrigerants, and related solvents. In E. W. Van Stee, editor. Cardiovascular Toxicology. New York: Raven Press. p. 281–314.