Abstract
For a few years, mineral oils and their potential adverse health effects have been a constant issue of concern in many regulatory areas such as food, cosmetics, other consumer products, and industrial chemicals. Analytically, two fractions can be distinguished: mineral oil saturated hydrocarbons (MOSH) and mineral oil aromatic hydrocarbons (MOAH). This paper aims at assessing the bioaccumulative potential and associated histopathological effects of MOSH as well as the carcinogenic potential of MOAH for consumer-relevant mineral oils. It also covers the absorption, distribution, metabolism, and excretion of MOSH and MOAH upon oral and dermal exposures. The use and occurrence of consumer-relevant, highly refined mineral oils in food, cosmetics and medicinal products are summarized, and estimates for the exposure of consumers are provided. Also addressed are the challenges in characterizing the substance identity of mineral oil products under REACH. Evidence from more recent autopsy and biopsy studies, along with information on decreasing food contamination levels, indicates a low risk for adverse hepatic lesions that may arise from the retention of MOSH in the liver. With respect to MOAH, at present there is no indication of any carcinogenic effects in animals dermally or orally exposed to highly refined mineral oils and waxes. Such products are used not only in cosmetics but also in medicinal products and as additives in food contact materials. The safety of these mineral oil-containing products is thus indirectly documented by their prevalent and long-term use, with a simultaneous lack of clinical and epidemiological evidence for adverse health effects.
Introduction
Mineral oils are derived from crude petroleum oil by a number of processing steps that start with atmospheric distillation followed by vacuum distillation of the atmospheric residue () (IARC Citation1984; Mackerer et al. Citation2003; EFSA Citation2012; IARC Citation2012). The vacuum-distillate fractions, which contain hydrocarbons with boiling points (at atmospheric pressure) in the range of about 300–600 °C, and the deasphalted vacuum residue are fed into refinery processes such as solvent extraction, hydrotreatment, and dewaxing (i.e. removal of n-alkanes, also called deparaffinization) (IARC Citation1984). These refinery streams (also known as base oils or lubricant base oils) can be further purified, blended, and formulated to yield the finished mineral oil products. The by-product of the dewaxing step (called slack wax) is deoiled to yield microcrystalline waxes and (hard) paraffin waxes which can be further refined by, e.g. hydrotreatment and blending. A well-known blend of mineral oils and waxes is petrolatum, which is also called petroleum jelly or Vaseline. shows the physical properties of highly refined mineral oils, paraffin waxes, and microcrystalline waxes.
Table 1. Physical properties of highly refined mineral hydrocarbons comprising white mineral oils, paraffin waxes and microcrystalline waxes.
Mineral oil products can be classified into lubricants and products intended for non-lubricant purposes (IARC Citation1984, Citation2012). Lubricant products include, for example, automotive and industrial engine oils and greases, hydraulic oils, metalworking fluids, but also food machinery lubricants and textile oils. On the other hand, “non-lubricant” products are used, for example, as extender oils for rubber, carriers for printing inks, processing aids in the manufacturing of rubber, plastics, and textiles, and as batching oils for the softening of natural fibers such as jute. Highly refined mineral oils and waxes, and blends thereof, are used as food additives, additives for food contact materials, cosmetic ingredients, and excipients for pharmaceutical formulations ().
Table 2. Consumer-relevant product groups of mineral oils and waxes and their use in the EU as food additives (Annex II of Regulation (EC) No 1333/2008), additives for plastics in food contact (Annex I of Regulation (EU) No 10/2011), cosmetic ingredients, and excipients in medicinal products (Monographs of the European Pharmacopeia). Cosmetic ingredient names follow the international nomenclature of cosmetic ingredients (INCI).
Mineral oils are complex substances of saturated and aromatic hydrocarbons predominantly with carbon numbers ranging from C15 to C50 () (IARC Citation1984). By using certain analytical techniques, two fractions can be distinguished: mineral oil saturated hydrocarbons (MOSH) and mineral oil aromatic hydrocarbons (MOAH). MOSH are composed of straight and branched open-chain alkanes (paraffins) and largely alkylated cycloalkanes (naphthenes) (Biedermann et al. Citation2009; EFSA Citation2012). The proportion of straight-chain alkanes (n-alkanes, also called paraffin waxes) among the MOSH is low because a dewaxing step is included in the manufacturing of mineral oils. MOAH include mono- and/or polyaromatic hydrocarbons that are highly alkylated and also often partially hydrogenated (EFSA Citation2012).
The term “MOSH” was introduced by Biedermann et al. (Citation2009) for petroleum-derived (i.e. petrogenic) saturated hydrocarbons to make a distinction from those of plant origin. The term “MOAH” was originally coined by the same authors (Biedermann et al. Citation2009) referring to contaminated food in order to distinguish the aromatic hydrocarbons of petrogenic origin from the polycyclic aromatic hydrocarbons (PAHs) that are typically formed by pyrogenic processes (i.e. incomplete combustion of organic compounds at high temperatures) (Moret and Conte Citation2000). PAHs in the narrower sense of the chemical nomenclature are unsubstituted fused ring systems containing at least two benzene rings. These are often called parent PAHs to distinguish them from alkylated (e.g. methylated) derivatives.
Mineral oils can occur in consumer products and foodstuff for different reasons. They are intentionally used as ingredients in cosmetic products (Petry et al. Citation2017), as extender oils in elastomeric and polymeric materials (e.g. tool handles) (Bartsch et al. Citation2016), and as food additives (e.g. glazing agents, preservatives, anti-foaming agents, release agents, anti-dusting agents) (EFSA Citation2009, Citation2013a, Citation2013b). Contamination can result from mineral oils used in printing inks for printing of food packaging which can evaporate and migrate via the gas phase into the packaged food (EFSA Citation2012). Food contamination may also result from the migration of mineral oil from paper and cardboard made of recycled fibers (Biedermann and Grob Citation2010; EFSA Citation2012). In addition, food and consumer products can become contaminated by mineral oils and other petroleum-derived substances (e.g. diesel fuel) via the environment and by technological processes (e.g. by contact with food machinery lubricants) (EFSA Citation2012).
Mineral oil hydrocarbons (MOH) and their potential adverse health effects are a recurring issue of concern in many regulatory areas such as food, food contact materials, cosmetics, and industrial chemicals. The aim of this paper is to review the toxicology of consumer-relevant mineral oils, specifically the bioaccumulative potential and associated histopathological effects of MOSH as well as the carcinogenic potential of MOAH. The absorption, distribution, metabolism, and excretion (ADME) of MOSH and MOAH are briefly summarized. The paper describes the use and occurrence of mineral oils in food, cosmetics and pharmaceuticals. A chapter on analytics is included to describe the state-of-the-art technology for the monitoring of mineral oils in food and consumer products. This review also covers the exposure of consumers to mineral oils, the potential health risks associated with this exposure, and the regulatory measures taken to limit and to reduce these risks. Also addressed are the challenges in characterizing the substance identity of mineral oil products under the European chemicals legislation. Finally, the paper briefly summarizes the activities at the European Chemicals Agency (ECHA) with respect to the identification and regulation of hazardous mineral oil substances. Consumers may also be exposed directly to mineral oil hydrocarbons by use of technical lubricants. This topic, however, is outside the scope of this review.
Mineral oil analytics
The structural diversity of hydrocarbons in mineral oil is immense (EFSA Citation2012). The composition of mineral oils is therefore extremely complex. A widely used method for quantitative mineral oil analysis is on-line coupled liquid chromatography coupled to gas chromatography and flame ionization detection (LC–GC–FID) (Biedermann and Grob Citation2012a). This method has been successfully applied to quantify MOSH and MOAH fractions as a sum parameter in food and cardboard packaging (Biedermann and Grob Citation2010, Citation2012b; EFSA Citation2012), and in cosmetic products (Niederer et al. Citation2015). A structural characterization of selected mineral oil constituents is, however, not possible with this technique. Instead, comprehensive two-dimensional gas chromatography (GC × GC) (Biedermann and Grob Citation2010, Citation2012a) in combination with mass spectrometry (GC × GC–MS) (Biedermann and Grob Citation2015; Bartsch et al. Citation2016) enables the characterization of structural elements and to determine the degree of substitution of aromatic hydrocarbons in mineral oil. Analysis at the individual substance level, however, cannot be achieved with the currently existing analytical techniques.
To quantify MOSH and MOAH in cosmetic products and other matrices, a chromatographic pre-separation of both fractions by LC is required (Biedermann and Grob Citation2012a). The different mineral oil components elute from a normal phase column in the following order: high and low molecular weight paraffins, naphthenes as last eluting MOSH, highly alkylated benzenes as first eluting MOAH, and alkylated polyaromatics as finally eluting MOAH. Quantitative analysis is then carried out by GC–FID. On-line coupled LC–GC–FID combines the pre-separation of MOSH and MOAH by high performance LC, involving simultaneous up-concentration by solvent trapping and re-condensation, with GC analysis and quantification via the universal FID detector.
On-line coupled LC–GC–FID provides separate chromatograms for MOSH and MOAH which show unresolved humps, sometimes with discrete peaks on top representing individual compounds such as n-alkanes for the MOSH fraction and alkylated benzenes for the MOAH fraction. The volatility range and shape of the hump reveals information on the source of the mineral oil. An identification of individual peaks is possible by analyzing the samples further, for example, via GC–MS or GC × GC–MS. For the correct evaluation and interpretation of MOSH and MOAH chromatograms, well-trained and experienced analytical staff and knowledge about the sample and its history are mandatory.
Individual constituents of the cosmetic or food matrix may interfere with the on-line coupled LC–GC–FID analysis if they co-elute with MOSH or MOAH fractions. Since MOSH and MOAH are relatively non-polar, it is unlikely that more polar substances interfere with their analysis. Individual substances such as certain fragrances with terpene-like structures may appear as single peaks on top of the MOAH hump. In addition, natural polyolefins and unsaturated poly-α-olefins (PAOs) have to be considered as possible interferences for MOAH humps. For the quantitative analysis of MOAH chromatograms, single peaks sitting on the top of the hump are subtracted from the total area of the MOAH hump. If necessary for the correct integration of the MOAH hump, olefinic and polyolefinic interferences can be removed by chemical modification such as epoxidation. Interferences with MOSH humps may result from n-alkanes of plant origin and saturated synthetic hydrocarbons such as polyolefin oligomeric saturated hydrocarbons (POSH). A separate quantification of MOSH and synthetic hydrocarbons is not always possible, so that only the total amounts of both can be quantified. n-Alkanes can be removed from the MOSH fraction by chromatography on activated aluminum oxide prior to on-line LC–GC–FID analysis.
This removal procedure eliminates n-alkanes of biogenic origin, but also n-alkanes of mineral origin. It is applied when n-alkanes in plant samples or oil samples are present in very high concentration so that their peaks would overlay and mask a MOSH hump of minor concentration (Fiselier et al. Citation2009; Fiselier and Grob Citation2009). When natural n-alkanes (in e.g. food samples) are present in lower concentrations, it is not necessary to remove them from the sample in order to be able to achieve a proper quantitation of MOSH. The removal step was not applied for the analysis of MOSH in biological tissues and breast milk (Noti et al. Citation2003; Concin et al. Citation2008; Barp et al. Citation2014; Biedermann et al. Citation2015; Barp et al. Citation2017a, Citation2017b).
The coupling of two orthogonal GC columns (GC × GC) allows for the characterization of ring systems and their degree of alkylation in the mineral oil mixture (). This information can be estimated from the position in the two-dimensional chromatogram. Combination of GC × GC with time-of-flight mass spectrometry (GC × GC–ToF–MS) allows for the identification of partially hydrogenated ring systems. However, an exact quantification of the total MOAH fractions or individual ring systems by GC × GC–ToF–MS is not possible. For substances with a medium or high degree of alkylation, a resolution down to the level of single constituents cannot be achieved technically. This is because of the immense number of potential isomers.
Several classical methods exist for the analysis of PAHs in mineral oil products such as proton nuclear magnetic resonance (1H-NMR) spectroscopy or the measurement of the UV absorbance of a dimethyl sulfoxide (DMSO) extract. 1H-NMR is correlated to the content of aromatic protons, whereas all methods that use DMSO extracts focus on the most polar and therefore DMSO-extractable compounds in the MOAH fraction: aromatic ring systems with low alkylation degree. These kind of assays are used, inter alia, for impurity testing according to the European Pharmacopeia (Ph. Eur.). Consistency of the results of these classical methods with the state-of-the-art analytical methods remains to be demonstrated, as they provide a different readout compared to the MOAH results obtained with online LC–GC–FID, which refer to the total content of aromatic compounds.
Toxicology of mineral oils
The bioaccumulative potential of MOSH and the carcinogenic potential of MOAH represent two of the main toxicological concerns associated with mineral oils. This chapter focuses on these two aspects but additionally addresses the ADME (absorption, distribution, metabolism, excretion) properties of these substances and the histopathological changes associated with the accumulation of MOSH in tissues. The relevance of effects observed in animal experiments for human health is also being discussed.
Literature search
The starting point for the literature search was in most cases the comprehensive scientific opinion of the European Food Safety Authority (EFSA) on mineral oil hydrocarbons in food (EFSA Citation2012). Relevant references cited by EFSA on the bioaccumulative potential and associated histopathological effects of MOSH (in animals and humans) as well as on carcinogenic potential of MOAH and PAHs were included in the initial list of references. Additionally included from the EFSA opinion were references on the ADME of MOSH and MOAH upon dermal and oral exposure. Systematic literature searches were subsequently conducted in the Science Citation Index Expanded database of Web of Science to find additional, relevant references. The details of the literature search in Web of Science (e.g. search options, set of queries, number of returned records, number of included and excluded references) as well as the lists of included/excluded studies and of additionally included references are provided in the Appendices 1 to 5.
MOSH
Dermal absorption of MOSH
Different techniques were employed to determine the extent of skin penetration and percutaneous absorption of individual model compounds and complex mixtures in in vitro and in vivo studies using animal and human skin. This section considers studies with dermal application of neat substances and of substances present in cosmetic formulations (Nash et al. Citation1996; Petry et al. Citation2017). Studies with n-alkane-containing jet fuels (Singh et al. Citation2002; Jakasa et al. Citation2015) were excluded since these contain n-alkanes and aromatics (carbon range: C6–C17) with skin-irritating and penetration-enhancing properties. In the studies reviewed below, the amount of the applied neat substance and formulation is indicated as dermal load (mg/cm2) to enable comparison with the real-life use of cosmetic products. For a face cream, for example, the typical daily value is 2.72 mg/cm2 (SCCS Citation2016; Petry et al. Citation2017).
Excised porcine back skin (3–4 mm thick) was used to study the dermal absorption of 14C-radiolabelled n-hexadecane (n-C16) and 3H-labeled n-docosane (n-C22) in different cosmetic vehicles in Franz-type diffusion cells (Brown et al. Citation1995). The vehicles comprised petrolatum, polydecene, white mineral oil, soya oil, and a water-in-oil (w/o) cream. Test formulations were applied at a dermal load of 3–5 mg/cm2. Analysis of the radioisotope distribution some 24 h later indicated absorption of 4–42%, 0.4–1.2% and 0.3–1.0% for stratum corneum, the viable epidermis and the dermis, respectively. No radioactivity was detected in the receptor fluid.
Split-thickness cadaver skin was used to study the dermal absorption of 14C-radiolabelled dotriacontane (C32) in petrolatum in Franz-type diffusion cells (Intarakumhaeng et al. Citation2018). The petrolatum was applied to the skin with solvent deposition using hexane or hexane/pentane mixtures under finite-dose conditions. A dose range of 0.05–0.2 mg/cm2 was chosen to mimic the amounts of cosmetic ingredients deposited on the skin surface after personal care products rinsing. The amounts of the model permeant dotriacontane measured in the receptor chamber 1 h, 24 h, and 72 h were below the detection limit.
Three microscopy studies (Ghadially et al. Citation1992; Brown et al. Citation1995; Mao-Qiang et al. Citation1995) determined the penetration depth of petrolatum into intact and acetone-treated skin of hairless mice. Skin wiping with acetone was used to disrupt the skin barrier by selectively removing the stratum corneum lipids. Petrolatum was applied once for 2–6 h, or repeatedly twice daily for three days. Skin biopsies were taken and processed for electron microscopy and fluorescence microscopy. The latter required lipid-soluble dyes, either as a tracer or as histochemical stain. In both acetone-compromised and intact skin, under conditions of single and repeated exposure, petrolatum remained restricted to the stratum corneum, inducing lacunae formation in the lamellar lipid layers.
Radioisotope studies complemented the microscopy studies in hairless mice. One study (Brown et al. Citation1995) analyzed the absorption of 3H-radiolabelled n-docosane (n-C22) into acetone-treated skin. Petrolatum and 1% petrolatum in propylene glycol:ethanol (7:3 v/v) served as vehicles. Test formulations were applied to the flanks at a dermal load of 5.4–6 mg/cm2. The animals were sacrificed after 2.5 h, and excess test substance was removed from the skin surface. Excess surface material, dissected layers of the skin, subcutaneous fat, and blood were analyzed. In the case of petrolatum, 7.8% of the applied label was found in the stratum corneum, 1.4% in the viable epidermis, 0.2% in the dermis, and 0.1% in subcutaneous fat. For petrolatum in the propylene glycol:ethanol mixture, a higher proportion (45%) of the radioactivity partitioned into the stratum corneum. The proportion in the viable epidermis remained essentially unchanged (1.2%), whereas that in the dermis and subcutaneous fat increased to 1.2% and 0.4%, respectively. Blood did not contain any detectable levels of radioactivity above background.
Whole-body autoradiography was used to study the percutaneous absorption of 14C-labeled n-octadecane (n-C18) in hairless mice (Suzuki et al. Citation1978). Octadecane was applied to the back, either neat (1.25 mg/cm2) or in a 5% hydrophilic ointment (8 mg formulation per cm2). After 48 h incubation under occlusive conditions, the animals were anesthesized, shock-frozen, and sagittal sections were then prepared. The radioactivity was localized on the applied regions, no radioactivity was detected in the systemic compartments. The substance was also applied neat to the shaved backs of guinea pigs (dermal load: 1.95 mg/cm2). In skin biopsies, the distribution was analyzed by microautoradiography. After 24 h of treatment under occlusive conditions, the radioactivity concentrated in the sebaceous glands of the hair follicles with some spreading into the surrounding dermis.
In another radioisotope study, neat 14C-radiolabelled n-hexadecane (n-C16) was applied to the backs of guinea pigs at a dermal load of 3.85 mg/cm2 (Rossmiller and Hoekstra Citation1966). The animals were sacrificed after 48 h, and the residual test material was removed from the skin surface. The treated skin was excised and the stratum corneum plus viable epidermis was separated from the dermis by mechanical stretching. 15.2% of the radioactivity was found in the stratum corneum plus viable epidermis, 0.1% in the underlying dermis, and a combined total of 0.1% in the liver and kidneys. Blood did not contain measurable amounts of 14C. Applying hexadecane in a 1:1 dilution with white mineral oil markedly reduced the dermal penetration, yielding 4.4% radioactivity in the stratum corneum plus viable epidermis.
Human skin from surgery was used to study the penetration of 14C-labeled n-octadecane (n-C18) from various ointments, including w/o and o/w creams, and petrolatum (Zesch and Bauer Citation1985). Test formulations were applied at a dermal load of 1–3 mg/cm2. The skin samples were mounted in a penetration chamber. After application times of 0.5 to 16.7 h, the excess substance was removed from the skin, and each specimen was separated into individual layers by tape stripping of the stratum corneum and by horizontal slicing of the viable epidermis and dermis using a freeze-microtome. The distribution and concentration of octadecane in the stratum corneum were virtually identical for all vehicles. The concentration profiles showed a continuous decrease towards deeper layers of the stratum corneum (). The viable epidermis and the dermis did not contain detectable levels.
The dermal penetration of petrolatum was studied in human subjects using a lipid-soluble tracer dye (Strakosch Citation1943). Petrolatum was applied to the anterior region of the thigh, forearms, back or abdomen at a dermal load of 17 mg/cm2. Histological sections were prepared from skin biopsies taken 24 h after application. The distribution of the dye-labeled petrolatum was analyzed microscopically and checked by counter-staining. Petrolatum was localized in the upper horny layer, and in the upper half of the hair shafts and sebaceous glands, which anatomically belong to the dermis. No penetration through the stratum corneum was observed in this study from 1943.
Noninvasive optical methods were employed to analyze the skin penetration of petrolatum and white mineral oil in human subjects (Stamatas et al. Citation2008; Patzelt et al. Citation2012; Choe et al. Citation2014; Choe et al. Citation2015; Choe et al. Citation2017). Test substances were applied to the inner forearm, mainly at dermal loads of 2 mg/cm2. Fluorescence laser scanning microscopy was used to analyze the distribution of dye-labeled MOSH (Patzelt et al. Citation2012). After a short application time of 30 min, excess amounts of the test substances were wiped off the skin surface. In case of mineral oil, only a very weak fluorescence signal was obtained from the skin surface and the lipid layers around the uppermost layers of corneocytes. Petrolatum was exclusively detected on the skin surface. With confocal Raman microscopy, the penetration process can be monitored and the thickness of the horny layer can be determined without the use of a tracer dye (Stamatas et al. Citation2008; Choe et al. Citation2014; Choe et al. Citation2015; Choe et al. Citation2017). The stratum corneum of untreated forearm skin was measured to be 19.2 µm thick (Choe et al. Citation2014; Choe et al. Citation2015). 60 min after application of the test substances, the concentration profiles showed an exponential decrease towards deeper layers (), with a penetration depth of 6–7 µm (Choe et al. Citation2014; Choe et al. Citation2015). Petrolatum had a pronounced swelling effect, increasing the stratum corneum thickness by 32% due to the absorption of water by corneocytes.
Overall, percutaneous absorption of MOSH is low, if not, extremely low. If MOSH are enabled to pass the stratum corneum barrier, as in the case of acetone-compromised skin tissue in hairless mice, they are able to reach the subcutaneous fat layer within 2.5 h (Brown et al. Citation1995). The extremely high octanol-water partition coefficient (e.g. estimated log PO/W for n-docosane: 11.15) favors partitioning into lipophilic compartments. Easy mobilization from adipose tissue is unlikely given the findings from animal experiments with oral exposure (see Section “MOSH levels in animal tissue”). Subcutaneous injection of a water-in-oil emulsion (Freund's incomplete adjuvant) containing light mineral oil (with 14C-radiolabelled n-hexadecane) and a surfactant (mannide monooleate) in rats and monkeys revealed the radioactivity to be very slowly cleared from the site of injection (Bollinger Citation1970). Intradermal injection of Freund's incomplete adjuvant in guinea pigs showed that some of the injected MOSH traveled along lymphatic vessels and appeared as oils droplets in the regional lymph nodes draining the site of intradermal injection. Local persistence of MOSH, the possible transport to regional lymph nodes, and a mechanism to load the plasma lipoproteins and albumin with MOSH (see Section “Distribution, metabolism, and excretion of MOSH”) should be taken into consideration when addressing the systemic availability of dermally applied MOSH.
In summary, MOSH readily partition into the uppermost cell layers of the stratum corneum. Although many studies seemingly fail to indicate a transport of MOSH through the stratum corneum one should note that this can also be attributed to the experimental design, particularly in studies with short application times in conjunction with rather low analytical sensitivity. Radioisotope studies in hairless mice with acetone-compromised skin, in guinea pigs, and with excised pork skin demonstrated a weak permeation of the horny layer by experimental MOSH surrogates, that is, radiolabelled shorter-chain n-alkanes (C16–C22). It should be noted, however, that the skin of hairless mice and guinea pig is more permeable than human skin (Jung and Maibach Citation2015) and that a radioisotope study with human skin using labeled n-C18 did not show any permeation of the stratum corneum, even after 16.7 h, whereas longer time periods were not addressed. Given the slow penetration into the horny layer, the unfavorable partitioning into aqueous epidermal and dermal tissues, and the potential impact of removal processes (contact with other surfaces, skin washing, desquamation and regeneration), it therefore seems unlikely that toxicologically relevant amounts of MOSH from, e.g. skin creams become bioavailable in humans.
Intestinal absorption of MOSH
Upon ingestion, MOSH follow the intestinal absorption pathway of dietary lipids. Experiments in rats with gastrointestinal administration of n-hexadecane (n-C16), n-octadecane (n-C18), and phytane (branched C20), which were used as model compounds for MOSH, showed these to be absorbed from the small intestine into the lymphatic system (Savary and Constantin Citation1967; Albro and Fishbein Citation1970a; Albro and Thomas Citation1974; Tulliez and Bories Citation1975a; Vost and Maclean Citation1984). This is in line with the log PO/W of these compounds being well above 5 (Trevaskis et al. Citation2008; Trevaskis et al. Citation2015). There is also experimental evidence in rats that n-C16 or, perhaps more likely, its oxidation product(s) can be absorbed to some extent into the hepatic portal vein (Savary and Constantin Citation1967; Albro and Fishbein Citation1970a). Gastrointestinal emulsification and bile salt-mediated micellar solubilization are required for the transport across the intestinal mucosa (Savary and Constantin Citation1967). Co-administration of dietary lipids such as triglycerides and fatty acids promotes the micellar solubilization of n-C16 and enhances its uptake by the lymphatic system (Savary and Constantin Citation1967).
The extent of intestinal absorption was determined in rats by subtracting the amount excreted in feces from the administered dose. This approach is assumed to be valid since there is no evidence either for a (relevant) biliary excretion of unmetabolized hydrocarbons (Albro and Fishbein Citation1970a, Citation1970b; Albro and Thomas Citation1974; Le Bon et al. Citation1988; Halladay et al. Citation2002) or for hydrocarbon oxidation by the gut flora (Mitchell and Hübscher Citation1968; Albro and Fishbein Citation1970a; Albro and Thomas Citation1974). Experiments with dietary and gavage administration of increasing doses of shorter-chain n-alkanes (n-C17, n-C18) (Albro and Fishbein Citation1970a; Popovic et al. Citation1973; Tulliez and Bories Citation1978, Citation1979) revealed a relatively constant maximum uptake in the lower dose range and a decrease at higher doses (), indicating a saturation of solubilization and absorption processes. The co-administration of absorption-enhancing agents (dietary lipids, synthetic emulsifier) very likely contributed to the study-specific differences in the dose-absorption curves, whose maximum levels ranged from 45% (Albro and Fishbein Citation1970a) up to almost 100% (Popovic et al. Citation1973; Tulliez and Bories Citation1978). Application of fixed doses of differently sized n-alkanes (n-C14 to n-C32) showed the absorption to be negatively correlated with carbon number (Albro and Fishbein Citation1970a; Popovic et al. Citation1973; Tulliez and Bories Citation1975a) (). Discrepancies between studies in the carbon number-dependent absorption curves are very likely caused by differences in the experimental procedures (dose, method of administration, use of absorption-enhancing agents). Constant dosing of linear, branched, and cyclic alkanes of similar carbon numbers did not indicate a marked difference in bioavailability (Albro and Fishbein Citation1970a; Tulliez and Bories Citation1975a) ().
Not only individual compounds were tested but also mineral oils. In a toxicokinetic study (Halladay et al. Citation2002), F-344 and SD rats received two doses (34 and 340 mg/kg bw) by gavage of a low-viscosity white mineral oil (presumably P15H, see ) in olive oil containing radiolabelled 1-eicosanylcyclohexane (cyclic C6 with C20 side chain) as surrogate marker for MOSH. The fecal excretion data indicated an absorption of 24 (F-344) and 30% (SD) at the low dose, and values of 8 (F-344) and 12% (SD) at the high dose. Further data comes from studies involving dietary exposure to mineral oil. In a study with rats and pigs (Tulliez Citation1986), the animals were fed two types of white mineral oil, denoted A and B, with average carbon numbers of 20 and 28. The diet containing 0.1% mineral oil of type A or B led to an absorption rate in rats of 52% and 35%, respectively, whereas in pigs, the percentages were 38% and 20%, respectively. The rates decreased in both species when the mineral oil content in the diet was increased to 1%, indicating an absorption limitation. Pigs receiving a diet containing 0.65% of a third type of white mineral oil with an average carbon number of 16 over a period of ten days showed an absorption of 88% (Tulliez Citation1986).
Table 3. Classification of highly refined mineral hydrocarbons (intended for use in food).
Further insights into the intestinal absorption of mineral oils comes from the measurement of MOSH in tissue. In a recent study, Barp et al. (Citation2017a) exposed female F-344 rats to a diet containing 0.004% of a broad MOSH mixture with a carbon number range from C14 to C50. The test item was dissolved in olive oil before incorporating it into the diet. The retention characteristics of liver and spleen suggested that intestinal absorption of MOSH decreased above C32 and approached zero at around C40. After 30 days of exposure, 10.9% of ingested MOSH were recovered from the rats’ body (excluding the gastrointestinal tract). This retained percentage refers to the total of the administered MOSH mixture, including the constituents of lowest and highest carbon number which did not show up in the tissues. For the most efficiently retained constituents, the percentage was even higher (17.2%). These figures are lower bound estimates for the extent of intestinal absorption. The true absorption is higher due to elimination of hydrocarbons that are more amenable to metabolism. Higher dietary concentrations of MOSH decreased the percentage of MOSH retained in the body, again indicating an absorption limitation.
To summarize, ingested MOSH follow the absorption pathway of dietary lipids. The fraction of absorbed dose of MOSH is negatively correlated with carbon number and dose. Co-administration of dietary lipids enhances the absorption. Exposure of rats to a diet containing a MOSH mixture of low concentration (0.004% or 40 ppm) results in absorption rates well above 20% for constituents that are most efficiently retained in the tissues. It is noted that this low concentration is comparable to the highest average background concentration in certain food categories such as vegetable oils and canned fish (see Chapter “Evaluation of mineral oil hydrocarbons in food by the EFSA CONTAM Panel”).
Distribution, metabolism, and excretion of MOSH
Ingested alkanes may already be metabolized whilst traversing the intestinal mucosa. Metabolic studies demonstrated that the intestinal mucosa of rats (McWeeny (Citation1957), cited in Mitchell and Hübscher (Citation1968)), guinea pigs (Mitchell and Hübscher Citation1968) and rabbits (Ichihara et al. Citation1981), but also the rumen tissue of goats (McCarthy Citation1964), has the capacity to oxidize n-C16 and n-C18 into the corresponding fatty acids.
Following intestinal lymphatic uptake, alkanes are transported as solutes in the lipid moieties of lymph chylomicrons towards systemic circulation. In rats, duodenal co-administration of n-alkanes (n-C16, n-C18) and triglycerides showed these hydrocarbons to be associated with the nonpolar triglyceride core of lymphatic chylomicrons (Vost and Maclean Citation1984). Intravenous injection of these chylomicrons in rats revealed a rapid clearance of hydrocarbons from plasma chylomicrons caused, inter alia, by the uptake into the liver and the transfer to other plasma lipoprotein classes (Vost and Maclean Citation1984). Remarkably, hydrocarbon clearance was closely correlated with chylomicron triglyceride clearance. A study on the lipid composition of human serum detected MOSH in low- and high-density lipoprotein classes (LDL, HDL) and in the albumin-containing fraction (Skipski et al. Citation1967); the MOSH in the albumin-containing fraction had a carbon number range of C17–C35 with n-alkanes being most prominent. Removal of n-alkanes yielded a residual hydrocarbon fraction distributed between C26 and C33. Since human serum albumin exhibits six binding sites for long-chain fatty acids (Peters Citation1995) it may contribute to the distribution of n-alkanes throughout the body in a similar way as the usage of LDL and HDL as possible MOSH transporters.
Information on the plasma half-life of intestinally absorbed MOSH comes from toxicokinetic studies in rats with single-dose administration of white mineral oils and individual model compounds. Two studies, in female F-344 and SD rats, with gavage administration of low-viscosity white mineral oils (e.g. P15H, see ) in olive oil were performed to establish blood concentration-time profiles for MOSH in the carbon number range of C19–C24 (Boogaard et al. Citation2012) and for radiolabelled 1-eicosanylcyclohexane (cyclic C6 with C20 side chain), which was added to the mineral oil as a surrogate marker for MOSH (Halladay et al. Citation2002). In the blood the hydrocarbons reached a peak concentration essentially within 3–6 h post-dosing. The terminal phase half-life derived from these blood concentration-time profiles was relatively long (43–86 h) (Halladay et al. Citation2002; Boogaard et al. Citation2012), reflecting a relatively slow elimination from the blood compartment. Based on blood concentration-time profiles and hepatic MOSH concentrations, F-344 rats exhibited a higher systemic exposure than SD rats. This difference presumably resulted from a higher metabolic activity in the latter, since fecal excretion data (Halladay et al. Citation2002) indicated a slightly higher absorption in SD rats compared to F-344 rats (see Section “Intestinal absorption of MOSH”). In female human volunteers, the oral administration of a gelatin capsule containing 71 mg of a low-viscosity white mineral oil failed to give detectable blood levels of MOSH (Boogaard et al. Citation2012), presumably because the dose was too low. A toxicokinetic study of the blood of patients undergoing colonoscopy after taking liquid paraffin as laxative (for dosage, see Chapter “Mineral oils in medicinal products”) might be a more suitable approach to obtain plasma half-life readings for intestinally absorbed MOSH.
The fate of intestinally absorbed MOSH (incl. model compounds and n-alkanes) in the systemic circulation is determined by the uptake and metabolism in the liver, and the distribution to other tissues, preferentially to adipose tissue (Pokrovskii et al. Citation1969) (for MOSH levels in selective tissues, see Sections “MOSH levels in animal tissues” and “MOSH levels in human tissues”). Metabolism is the dominant elimination process as there is no evidence for the excretion of non-metabolized alkanes via the urinary tract (Tulliez and Bories Citation1975a, Citation1978, Citation1979; Le Bon et al. Citation1988) and virtually none for the biliary excretion of the unchanged form (Albro and Fishbein Citation1970a, Citation1970b; Albro and Thomas Citation1974; Le Bon et al. Citation1988; Halladay et al. Citation2002). Also, an in vitro study found no evidence for a relevant extrahepatic metabolism of n-hexadecane in lung and kidney microsomes of mice (Kusunose et al. Citation1969). Exhalation of smaller, more volatile hydrocarbons could be an additionally route of elimination, given the detection of n-alkanes (up to n-C20) in human breath and indoor air (Phillips et al. Citation1999; Phillips et al. Citation2000). However, the alkane concentrations and the alveolar gradient (concentration in breath minus concentration in ambient room air) were in the picomolar range which renders elimination via exhalation less relevant than metabolic elimination.
Depending on the type of alkane, different metabolic pathways are involved in the biotransformation (Tulliez Citation1986; EFSA Citation2012). Linear alkanes undergo terminal (ω) oxidation via alkyl/fatty alcohols to their corresponding fatty acids (), which then enter the β-oxidation pathway or are incorporated into lipids (McCarthy Citation1964; Kolattukudy and Hankin Citation1966; Savary and Constantin Citation1967; Kusunose et al. Citation1969; Popovic Citation1970; Ichihara et al. Citation1981; Popovic et al. Citation1982; Perdu-Durand and Tulliez Citation1985; Cravedi et al. Citation2011; Cravedi and Perdu Citation2012). The metabolism of cycloalkanes was studied with mono- and bicyclic model compounds. The n-alkyl side chain of cyclohexanes (cyclic C6 with C12 or C20 n-alkyl side chains) is subjected to ω-oxidation followed by β-oxidation to yield cyclohexyl alkanoic acids (), which may eventually become the target of further transformations prior to urinary excretion (Tulliez and Peleran Citation1977a, Citation1977b; Tulliez and Bories Citation1979; Tulliez et al. Citation1981; Halladay et al. Citation2002). The unsubstituted bicyclic compound decalin (C10) is hydroxylated to different decalols which are conjugated and excreted via urine (Elliott et al. Citation1966; Olson et al. Citation1986; Dill et al. Citation2003). The fate of branched alkanes was elucidated in studies with pristane (C19) and phytane (C20), which undergo terminal (ω) or subterminal (ω–1) oxidation ( (Albro and Thomas Citation1974; Le Bon et al. Citation1988). Whereas the former leads to fatty acids entering the β-oxidation route, the latter yields tertiary alcohols, which are considered as a metabolic dead end precluding any further oxidation (Albro and Thomas Citation1974; Le Bon et al. Citation1988). Depending on branching patterns, α-oxidation could step in if β-oxidation of fatty acid derivatives is prevented by a tertiary carbon center at the β-position (Hansen Citation1968).
Information on the metabolism of complex MOSH mixtures can be derived from GC × GC analysis of hydrocarbons that are retained in tissue. In a recent study, Barp et al. (Citation2017a) exposed female F-344 rats to a diet containing a broad MOSH mixture, ranging from about C14 to C50 and being largely devoid of n-alkanes above C21, over a period of 90 days, followed by a 30-day post-exposure period. Hydrocarbons retained in liver ranged from C16 to C40. Subtle differences between the MOSH composition of the administered mixture and the hydrocarbons retained in tissue provided evidence of some degree of selective elimination from the liver (via metabolism and also possibly by exchange processes between liver and blood). These differences were revealed by MS analysis of characteristic fragment ions being selective for (i) multibranched paraffins, (ii) monoalkylated cyclopentanes and cyclohexanes, (iii) C26 naphthenes with 1–4 rings, and (iv) alkylated C24 monocyclic naphthenes, respectively. Among multi-branched paraffins retained in liver, smaller constituents, including pristane and phytane, were largely absent (Barp et al. Citation2017a), suggesting an effective elimination. In the GC × GC–MS plot related to monoalkylated cyclopentanes and cyclohexanes, the series of distinct signals for n-alkyl monocyclics was virtually absent, leaving behind a cloud of unresolved, highly isomerized material. Obviously, the high degree of isomerization due to possible branching of the alkyl group seemed to prevent a fast/efficient metabolic break down. Further analysis focused on hydrocarbons of the same carbon number. The composition of retained C26 naphthenes, which mainly comprised 1–4 ring systems, indicated a facilitated elimination of compounds with a major straight alkyl chain. The analysis of C24 monocyclic naphthenes revealed an efficient metabolism of n-alkylated compounds with elimination efficiency decreasing with increasing alkyl chain branching or multi-substitution.
To summarize, the fate of intestinally absorbed MOSH is determined by the uptake and metabolism in the liver, and the partitioning to other tissues, preferentially to adipose tissue. Biotransformation is the dominant elimination process. Studies with individual model compounds showed that different oxidative pathways could be involved in biotransformation, depending on the type of alkanes. For complex MOSH mixtures that are retained in F-344 rat liver, there is evidence of some degree of selective facilitated elimination of simple-structured hydrocarbons such as small multibranched paraffins and n-alkylated naphthenes.
MOSH levels in animal tissues
MOSH levels in animal tissues were analyzed in studies with single and repeated oral administration of single model compounds and petroleum products, including white mineral oils, paraffin waxes, and microcrystalline waxes ().
Single dietary administration of linear, branched, and cyclic alkanes (C16 to C32) to Wistar rats showed a differential retention pattern in tissues, depending on alkane type, carbon number, and tissue type (Tulliez and Bories Citation1975a, Citation1978, Citation1979; Le Bon et al. Citation1988). Repeated administration of a diet containing 0.1% shorter-chain cyclic and linear alkanes (cyclic C6 with C12 n-alkyl side chain, n-C20) to Wistar rats over 3 months resulted in low concentrations (10.7–29.6 ppm) in liver and high levels (1029–1134 ppm) in adipose tissue (Tulliez and Bories Citation1975b). A similar picture was observed in a 10-day feeding study with pigs receiving a diet containing 0.65% white mineral oil composed of shorter-chain MOSH (average carbon number: 16, 60% linear alkanes and 40% branched-plus-cyclic hydrocarbons) (Tulliez Citation1986). The levels of linear and branched-plus-cyclic alkanes were low in liver (6 and 15 ppm) and high in adipose tissue (1640 and 570 ppm). The low liver levels suggest efficient metabolism of shorter-chain compounds.
Conversely, when the mineral oil constituents had higher carbon numbers and a higher proportion of branched and cyclic hydrocarbons a different picture emerged. Pigs receiving a diet containing 3.23% white mineral oil (average carbon number: ∼20) over 3 months had high MOSH concentrations in liver (1353 ± 377 ppm, N = 5 animals) and low levels in subcutaneous (293 ± 47 ppm) and back fat (256 ± 53 ppm) (Tulliez, Bories, and Peleran Citation1975). Among the other tissues, the spleen had very low levels (65 ± 15 ppm) whereas the mesenteric lymph nodes (MLNs) showed the highest value (1286 ppm, N = 1). Chromatographic analyses revealed that liver accumulated MOSH with higher and adipose tissue those with lower carbon numbers (Tulliez, Bories, and Peleran Citation1975).
It should be noted that analytical techniques have rapidly evolved during the past decades widening the scope and increasing resolution as well as sensitivity. Therefore, from the today’s perspective, the mid-1970s study results on MOSH content, composition, and carbon number range are associated with a certain degree of uncertainty.
In the literature, several studies exist in which rats were exposed subchronically or chronically to white mineral oils and to paraffin and microcrystalline waxes via feed (Baldwin et al. Citation1992; Firriolo et al. Citation1995; Smith et al. Citation1996; Scotter et al. Citation2003; Trimmer et al. Citation2004; Griffis et al. Citation2010; Barp et al. Citation2017a, Citation2017b). They provide information on the MOSH levels in liver and other tissues in dependence on the special kinds of mineral oils and waxes, dietary concentrations, strains and sexes, and exposure times. In addition, they provide information on the reversibility of accumulation effects and on the time to reach steady-state levels of MOSH.
In a 90-day study (Smith et al. Citation1996) F-344 rats were fed with diets containing 2% mineral oil or wax of various types. The feeding of low-viscosity (e.g. N15H, P15H, N70H) and medium-viscosity (P70H) oils caused elevated MOSH levels (1000–4300 ppm) in female livers (). Male livers had 4–5 times lower levels. Females exposed to a high-viscosity oil (P100H) had MOSH concentrations (600 ppm) being only slightly elevated above the background levels found in the liver of controls (∼200 ppm). Administration of paraffin waxes with low and intermediate melting points (LMPW, IMPW) induced the highest MOSH levels (7600–16900 ppm) in female livers (). Levels in male livers were about 1.5–2 times lower. For microcrystalline wax (HMPW: high melting point wax), MOSH levels in liver were not significantly different from control values. It is important to note that paraffin waxes are defined by a high content of n-alkanes, a feature that distinguishes them from mineral oils. This has important toxicokinetic consequences, best exemplified by the difference in accumulation between LMPW and P100H. This example illustrates that merely comparing the level of MOSH in tissue can be misleading, especially when varying types of petroleum products are being used, because the MOSH composition can be totally different (see below).
Test items causing high MOSH concentrations in liver also caused similar levels in the MLNs; in contrast, MOSH levels in adipose tissue were about an order of magnitude lower than those in liver (Smith et al. Citation1996). The accumulation of MOSH in liver was reversible. F-344 rats receiving a control diet for 28 days after a 90-day treatment with low-viscosity oils showed a reduction of liver MOSH contents by 10–30% (females, ) and 35–50% (males). For paraffin waxes, a reduction by 80–90% was observed after an 85-day post-exposure period (). These reductions can be directly related to elimination half-lives if the underlying process can be assumed to follow a mono-exponential decay. For example, a reduction by 80% (corresponding to a relative decrease from 1 to 0.2) in 85 days would then translate into an elimination half-life of 37 days (= −85/log2 0.2).
Several studies exist in which female F-344 rats were exposed subchronically to diets of different concentrations ranging from 0.004% to 2% () (Firriolo et al. Citation1995; Griffis et al. Citation2010; McKee et al. Citation2012; Barp et al. Citation2017a, Citation2017b). Here, the MOSH levels in liver increased less than proportional with dietary concentration. For example, a 10-fold increase in the dietary concentration of P7H (McKee et al. Citation2012), P15H (Firriolo et al. Citation1995), and LMPW (Griffis et al. Citation2010) from 0.2% to 2% caused an increase of liver MOSH levels by 1.3–1.5-fold only (). At lower dietary levels, this sub-linearity was still noticeable: A 2.5-fold increase in the concentration of two pharmaceutical-grade mineral oils (S-C25 and L-C25, Ph. Eur. Paraffinum perliquidum and P. liquidum, something close to P15 and P70 oils according to viscosity at 40 °C of 18 and 80 mm2/s, respectively) from 0.04% to 0.1% (Barp et al. Citation2017b) raised the MOSH concentration in liver by only 1.9-fold (. The under-proportional increase suggests saturation of the transport system due to a limitation in digestive solubilization and intestinal absorption of MOSH.
Comparative studies in rats revealed pronounced sex and strain differences. Female F-344 rats appear to have a predisposition to retain high MOSH levels in liver in response to the dietary exposure to low-viscosity and medium-viscosity oils, and low and intermediate melting point waxes. Much lower levels occurred in male F-344 rats () (Baldwin et al. Citation1992) and female SD rats () (Firriolo et al. Citation1995) when being exposed to low-viscosity and medium-viscosity oils. Remarkably, female SD rats ingesting the (n-alkane-rich) low melting point wax showed hepatic MOSH levels below the limit of quantification () (Griffis et al. Citation2010).
In female F-344 rats, the strong retention in liver following exposure to paraffin waxes (e.g. LMPW) indicates the inability to efficiently metabolize absorbed n-alkanes consisting of a certain carbon number range (Smith et al. Citation1996; Griffis et al. Citation2010; Barp et al. Citation2017b; Cravedi et al. Citation2017). Even very low concentrations (0.02–0.12 ppm) of natural n-alkanes in the range of C29–C33, that were present in control feed, were found to be enriched in liver of untreated control animals (Cravedi et al. Citation2017). It was hypothesized by the authors that these wax components crystallized in the liver tissue, which would be an additional factor contributing to the slow biotransformation (Barp et al. Citation2017b; Cravedi et al. Citation2017).
The time-course of MOSH retention and the elimination of MOSH during a recovery period was monitored in a chronic study with female F-344 rats (Trimmer et al. Citation2004). Animals received a diet containing P70H or P100H in a concentration of ∼2.5%, corresponding to a dose of 1200 mg/kg bw/day. After 3 months, the MOSH levels in liver increased to approximately 900 and 1600 ppm for P70H and P100H, respectively (). Further increase was slow, reaching 1400 and 2270 ppm, respectively, after two years. This suggests that the MOSH levels reach a steady state within two years. Reversibility was assessed by a twelve-month recovery phase following twelve months of exposure. During this post-exposure period, MOSH levels in liver decreased towards the levels in the control group in a mono-exponential manner () with half-lives of 91 and 122 days for P70H and P100H, respectively. Based on these elimination kinetics, the time for MOSH to reach a steady state level can be calculated. As a rule of thumb, a quasi-steady state (97% of the final level) is reached after 5 half-lives. Accordingly, it would take 1.2–1.7 years to reach the steady state when feeding these types of mineral oils.
MOSH accumulation was also studied by Barp et al. (Citation2017a) in a subchronic study in female F-344 rats which received a diet containing a broad MOSH mixture, ranging from about C14 to C50 and being largely devoid of n-alkanes above C21, at concentrations of 0.004, 0.04 and 0.4%. The test item was prepared by combining two pharmaceutical-grade white oils (S-C25 and L-C25, something close to P15 and P70 oils), a high-quality process oil (P100H-type), and the vacuum distillate of a pharmaceutical-grade light white oil (Ph. Eur. P. perliquidum). The resulting MOSH mixture displayed a chromatographic profile covering a wide carbon-number range. The test item was dissolved in soybean oil before incorporating it into the diet. Amounts and composition of the MOSH were analyzed in liver, spleen, adipose tissue, and the carcass after 30, 60, 90 and 120 days of exposure, and after 90-day exposure plus 30-day recovery period.
For all three dietary concentrations, the MOSH levels in liver increased rapidly until day 30 and then slower until it reached a plateau between day 90 and 120 (Barp et al. Citation2017a) (). During the 30-day recovery period, hepatic MOSH levels decreased by 60, 54, and 34% (corrected for hepatic MOSH levels in the control group) in the low, intermediate, and high-exposure groups, respectively. These reductions correspond to half-lives of 23, 26, and 50 days. Accordingly, the time to reach a quasi-steady-state level was 115–250 days, which agrees well with the observation of a plateau after 90 days of exposure. Compared to the MOSH levels in liver, those in spleen and adipose tissue were approximately one order of magnitude lower and did not reach a steady state during the 120 days of exposure. The post-exposure reduction of splenic MOSH levels at low food concentration was 72%. At intermediate and high food concentrations, however, splenic MOSH levels decreased by only 11% during the post-exposure period. In adipose tissue, the MOSH concentration still increased during the recovery period, suggesting an accumulation of MOSH and a post-exposure transfer from other tissues (e.g. from liver via lipoproteins), including – possibly – the gastrointestinal tract. Overall, the 30-day recovery period led to the elimination of 40–50% of the retained MOSH from the body.
To determine the most strongly retained hydrocarbons in terms of carbon numbers, Barp et al. (Citation2017a) compared the LC–GC–FID chromatograms of the dietary MOSH mixture with those of liver, spleen, and adipose tissue. The carbon number distribution of hydrocarbons was calibrated using n-alkanes as markers. The authors selected the tissue chromatograms of the recovery group (90-day exposure plus 30-day recovery) as these singled out the retained hydrocarbons at best. The data for the lowest dietary concentration (0.004%) were chosen since the liver chromatograms indicated a limiting absorption of hydrocarbons above C24 at higher dietary MOSH levels. For data analysis purposes, the x-axes of the chromatograms were binned using a segment width of one carbon number unit in terms of the n-alkane calibration. The processed profiles were finally used to calculate a so-called retention function (or transfer function) which translates the dietary MOSH distribution (“input signal”) into a given tissue MOSH distribution (“output signal”) (). The maximum retention in liver and spleen was at C29, and at C15/16 in adipose tissue (). Both the preferential sequestration of shorter-chain compounds in fat, and the more efficient metabolism of these compounds in liver, very likely contributed to the different profiles in these tissues.
Apart from the characteristic shapes of the retention profiles, the chromatogram of the MOSH in liver of female F-344 rats revealed an additional, subtle but toxicologically significant, feature. The top and the downslope of the MOSH hump above C22 showed distinct peaks which were largely invisible in the broad MOSH mixture in the animals’ diet (Barp et al. Citation2017a). The same phenomenon was observed in female F-344 rats exposed to the white oil L-C25 (Barp et al. Citation2017b). Further characterization by GC × GC analysis showed that the main peaks represented branched alkanes, whereas the small peaks were identified as n-alkanes (Barp et al. Citation2017b). These results indicate that n-alkanes and certain branched alkanes from C27 to C35 are selectively enriched in the liver of female F-344 rats (Barp et al. Citation2017b).
To summarize the results, rats and pigs show a differential retention pattern of MOSH in tissues. Shorter-chain compounds around n-C16 partition into and accumulate in adipose tissue. The liver retains MOSH with higher carbon numbers, suggesting a more efficient oxidation of shorter-chain compounds. Female F-344 rats showed a maximum retention in liver and spleen at C29. They retained high MOSH levels in liver, and in the MLNs, upon dietary exposure to low-viscosity and medium-viscosity oils, and low and intermediate melting point waxes. The highest MOSH levels in liver were caused by dietary exposure to low melting point waxes, which – together with findings in ‘untreated’ animals exposed to natural n-alkanes in control feed – indicate the inability to efficiently metabolize long n-alkanes (above C22) from both petrogenic and natural origin. Compared to female F-344 rats, much lower MOSH levels in liver and MLNs occurred in male F-344 rats and female SD rats. The latter had hepatic MOSH levels below the limit of quantification upon dietary exposure to (n-alkane-rich) low melting point wax. In female F-344 rats exposed to moderate dietary concentrations of ≤ 0.4% of a broad MOSH mixture, steady-state MOSH levels in liver were reached after 115–250 days. Conversely, adipose tissue seems to slowly accumulate MOSH.
MOSH levels in human tissues
Information on the concentration and composition of MOSH in human tissue became first available from autopsy studies performed in the mid-1960s (Boitnott and Margolis Citation1966b; Rose and Liber Citation1966; Liber and Rose Citation1967; Boitnott and Margolis Citation1970). It was not until this century that new data were obtained from autopsy and biopsy specimens using advanced analytical methods (Noti et al. Citation2003; Concin et al. Citation2008; Barp et al. Citation2014; Biedermann et al. Citation2015).
The mid-1960s studies analyzed lymph nodes, spleen, and liver tissues from autopsies performed in the US (Boitnott and Margolis Citation1966b; Rose and Liber Citation1966; Liber and Rose Citation1967; Boitnott and Margolis Citation1970). MS of saturated hydrocarbons, which were isolated from all three tissues types, identified complex mixtures of predominantly branched and cyclic compounds, a composition typical of MOSH (Boitnott and Margolis Citation1966b, Citation1970). GC–FID analysis of splenic tissue revealed a largely unresolved hump ranging from C21 to C28 (Rose and Liber Citation1966; Liber and Rose Citation1967). Using column or thin-layer chromatography, the MOSH concentrations in spleen and liver were determined gravimetrically (Rose and Liber Citation1966; Boitnott and Margolis Citation1970). MOSH levels in spleen samples were in the range of 120–4500 ppm (N = 26) (Boitnott and Margolis Citation1970) and 60–2660 ppm (N = 13) (Rose and Liber Citation1966; Liber and Rose Citation1967). Liver samples contained MOSH concentrations ranging from <100 ppm to 4100 ppm (N = 42) (Boitnott and Margolis Citation1970). It should be noted that analytical techniques have been rapidly evolved during the past decades widening the scope and increasing resolution as well as sensitivity. Therefore, from the today’s perspective, the mid-1960s study results on MOSH content and carbon number range are associated with a significant degree of uncertainty.
In samples from 37 patients (25–91 years old) autopsied in Austria in 2013, Barp et al. (Citation2014) determined the MOSH concentration in MLNs, subcutaneous abdominal adipose tissue, liver, spleen, and lung by on-line coupled LC–GC–FID. The data were approximately log-normally distributed with a median of 166 ppm in the MLNs (range: 21–1390 ppm), 87 ppm in adipose tissue (range: 17–493 ppm), 71 ppm in liver (range: 14–901 ppm), 28 ppm in spleen (range: 6–1400 ppm), and 7 ppm in lung (range: < 2–91 ppm) (). As in rats (Smith et al. Citation1996), the MLNs as the first site of contact following intestinal lymphatic uptake showed the highest MOSH concentrations, suggesting an exposure via the oral route.
The MOSH composition in MLNs and adipose tissue displayed high similarity and varied little between subjects (Barp et al. Citation2014). The bulk of hydrocarbons (highly branched and cyclic MOSH isomers) remained unseparated and formed a hump, ranging from C16 to C35, centered at C23/24 (). The tall skinny peaks on the downslope of the humps above C24 indicated the presence of even- and odd-numbered n-alkanes. The peaks of the odd-numbered n-alkanes were more prominent than those of the even-numbered ones. A strong predominance of the former points to a biogenic origin (e.g. from plant waxes), whereas a balanced occurrence of even- and odd-numbered n-alkanes is considered to be of petrogenic origin (Noti et al. Citation2003; Biedermann et al. Citation2015). MOSH in liver and spleen had similar mass distributions, which were shifted to higher values, ranging from C18 to beyond C45, centered on C25–27 () (Barp et al. Citation2014). The humps were completely devoid of skinny peaks indicative of n-alkanes, suggesting efficient metabolism of these hydrocarbons in the human liver. A similar pattern, with mass distributions being different for adipose tissue and liver/spleen, was also observed in a subchronic study in rats: Female F-344 rats were exposed to a diet containing a low concentration (0.004%) of a broad MOSH mixture relevant to human dietary exposures (Barp et al. Citation2017a). In rat liver and spleen, the maximum retention in terms of molecular mass was at C29 () which is close to the peak of the mass distributions in human liver and spleen.
Multiple linear regression (RP et al., unpublished data) was applied to analyze the age and sex dependence of the MOSH concentration in the autopsy tissues. Women had, on average, a 2.2–2.5-fold higher MOSH concentration in liver, spleen, and adipose tissue compared to men. A slower MOSH metabolism and/or a higher MOSH exposure of women relative to men could account for these differences. There was no evidence of an age dependence for the MOSH concentrations in liver and spleen, suggesting that a steady state had been reached. The MOSH levels in adipose tissue and MLNs exhibited a 1.2–1.4-fold increase per decade pointing to a lifelong accumulation in both tissues. A constant fold increase might seem to be implausible since, under conditions of constant exposure, it would imply that older subjects would experience a higher absolute increase compared to younger subjects. However, this finding could still be compatible with a plausible linear accumulation process when taking into account that a given tissue level of MOSH is the result of cumulative past exposure and that, historically, exposure to MOSH via the diet was higher in the past as it is today (see Section “Histopathological lesions associated with the deposition of MOSH in human tissues”).
Tissue-specific retention kinetics with MOSH levels reaching a steady state in human liver but not in adipose tissue is consistent with the F-344 rat model (see Section “MOSH levels in animal tissues”). Since elevated MOSH levels in liver can result in histopathological lesions (see Sections “Effects associated with the accumulation of MOSH in animal tissues” and “Histopathological lesions associated with the deposition of MOSH in human tissues”), the crucial question is whether the dose-concentration relationship for the F-344 rat can be plausibly extrapolated to humans. A MOSH concentration of 220 ppm was measured in the liver of female F-344 rats following the subchronic dietary exposure to ∼2 mg/kg bw per day of a broad MOSH mixture (0.004% in diet) (Barp et al. Citation2017a). Human autopsy livers contained a median concentration of 71 ppm. Interspecies extrapolation can be applied to derive a human equivalent oral dose from the rat data. By assuming a linear dose-concentration relationship at low doses (i.e. linear kinetics) and by using the default adjustment factor of 4 for interspecies toxicokinetic differences, the human equivalent oral dose for a liver concentration of 71 ppm would be 0.16 mg/kg bw per day (=2 × 71/220/4). The EFSA CONTAM Panel (EFSA Citation2012) estimated a background dietary exposure of 0.03–0.32 mg/kg bw per day (see Chapter “Evaluation of mineral oil hydrocarbons in food by the EFSA CONTAM Panel”). For MOSH in lip care products, an oral exposure of 0.08–0.7 mg/kg bw per day was estimated in a worst-case exposure scenario (see Chapter “Mineral oil hydrocarbons in cosmetic products” and BfR (Citation2018)”). These exposure estimates are in line with the derived human equivalent oral dose.
In a biopsy study performed in Austria in 2005–2006, Concin et al. (Citation2008) measured the MOSH concentrations in subcutaneous fat and in the fat phase of breast milk sampled from 144 women (19–47 years old). Subcutaneous fat was removed from the abdominal wall during cesarean sections. Milk samples were collected on day 4 and day 20 after birth, at the end of breast-feeding sessions. MOSH chromatograms of day-4 milk and tissue fat were virtually identical. Occasional deviations from the typical MOSH composition were observed, suggesting a local contamination most likely caused by a mineral oil-containing nipple ointment (e.g. Vaseline). The data were approximately log-normally distributed. Subcutaneous fat had a median concentration of 52.5 ppm (range: 10–360 ppm, N = 142 subjects) (). The median concentration in milk fat was 30 ppm in day-4 milk (range: 10–355 ppm) and 10 ppm in day-20 milk (range: <5–285 ppm) (). A decrease over the breast-feeding period was also observed in another study performed in Switzerland around 2002 (Noti et al. Citation2003): the median concentration in milk fat decreased from 54 ppm on day 3–5 (range: 25–190 ppm, N = 7 subjects) to 6 ppm (range: <3–66 ppm, N = 14 subjects) during month 1–13. This study (Noti et al. Citation2003) also noted a decreasing trend between the start and the end of a breast-feeding session.
The MOSH concentrations shown in refer to the fat phase of tissue and breast milk. Day-4 and day-20 milk samples had a mean fat content of 2.4% (w/v; range: 0.5–5.9%) and 3.7% (range: 0.5–8.5%) (Concin et al. Citation2008). The MOSH content in human milk was therefore considerably lower, with median levels of 0.72 ppm for day-4 milk and 0.37 ppm for day-20 milk. Human adipose tissue has an average fat content of 84% (Thomas Citation1962), so that the median MOSH content in adipose tissue would be 44 ppm (compared to the 52.5 ppm in tissue fat).
Multiple linear regression was used to identify independent variables associated with the MOSH concentration in abdominal subcutaneous fat at the time of cesarean delivery (Concin et al. Citation2011). Age, body mass index (BMI) prior to pregnancy, and cosmetics use were significantly correlated with MOSH content, showing higher levels with increasing age, lower BMI, and the use of certain cosmetic products (Concin et al. Citation2011). The observed negative correlation between MOSH concentration in tissue fat and BMI (which is highly correlated with body fat mass) might be explained by the fact that a given absolute amount of MOSH is distributed (or diluted) in a large volume of tissue fat. Three predictive categories of cosmetics use were identified: hand cream and lipstick use in daily life, and sun cream use in the present pregnancy (Concin et al. Citation2011).
A re-analysis of the data was carried out to estimate the effect sizes for age and cosmetics use (RP et al., unpublished data). The MOSH levels in tissue fat of the pregnant women exhibited a 1.5-fold increase per decade. A similar effect size (1.2-fold increase per decade) was obtained for the MOSH content in adipose tissue of the autopsied subjects. The same increase (1.2-fold per decade) can be calculated from the mean age and median MOSH level in adipose tissue of pregnant women (31 years, 44 ppm) and autopsy patients (67 years, 87 ppm).
To determine the effect size for cosmetics, the three previously identified categories of use were included in the re-analysis (RP et al., unpublished data). Women, who indicated the use of items from all three product categories (i.e. hand cream, lipstick, and sunscreen), on average had a 2.1-fold higher MOSH concentration in abdominal subcutaneous fat than non-users. This finding might suggest a dermal uptake of MOSH from cosmetic products. However, given the poor dermal absorption of MOSH (see Section “Dermal absorption of MOSH”), the good intestinal absorption of low MOSH doses via the absorption pathway for dietary lipids (see Section “Intestinal absorption of MOSH”), and the high MOSH levels in human MLNs (see also Section “Histopathological lesions associated with the deposition of MOSH in human tissues”), a relevant dermal uptake is not plausible. Instead, an oral uptake is more likely, owing to direct ingestion (lipstick material), hand-to-mouth contact, and the transfer of MOSH from hands to food (see Chapter “Mineral oil hydrocarbons in cosmetic products”).
To summarize, median MOSH levels in autopsy samples (from 2013) were 166 ppm in MLNs, 87 ppm in adipose tissue, 71 ppm in liver, and 28 ppm in spleen. The high MOSH levels in MLNs as the first site of contact following intestinal lymphatic uptake suggest an uptake via the oral route. The MLNs and subcutaneous adipose tissue showed a similar MOSH composition and mass distribution, being different from the profiles obtained for liver and spleen. MOSH in these organs were characterized by a higher mass distribution and the virtual absence of n-alkanes, indicating that only a very distinct and consistent fraction is retained from the total bioavailable material. A statistically significant, age-dependent increase in MOSH concentration was only found for the MLNs and adipose tissue, pointing to a lifelong accumulation in these tissues. Women had, on average, a 2.2–2.5-fold higher MOSH concentration in liver, spleen, and adipose tissue compared to men.
Effects associated with the accumulation of MOSH in animal tissues
The accumulation of MOSH in specific tissues of orally exposed F-344 rats was associated with increased organ weights, microgranuloma formation in MLNs, granulomatous inflammatory changes in liver, haematological changes, and clinical chemistry changes.
Light white oil (P7H) and certain low-viscosity mineral oils (N10A, N15H, P15H, P26A) caused pronounced dose-dependent increases in organ weight. Female F-344 rats subchronically exposed to a diet containing 2% of one of these materials showed increases up to 120–140% for liver, 110–200% for spleen, and 330–600% for the MLNs (Baldwin et al. Citation1992; Firriolo et al. Citation1995; Smith et al. Citation1996; Scotter et al. Citation2003; McKee et al. Citation2012). The biggest weight gain occurred in the MLNs, which represent the first site of contact following intestinal lymphatic uptake. LMPW induced even stronger increases in organ weight: up to 110–180% for liver, 140–230% for spleen, and 380–770% for the MLNs (Smith et al. Citation1996; Scotter et al. Citation2003; Griffis et al. Citation2010). Compared to female F-344 rats, male F-344 rats (Baldwin et al. Citation1992), and females SD rats (Firriolo et al. Citation1995; Griffis et al. Citation2010) were much less susceptible. There was also no evidence of treatment-related increases in liver weight of L-E rats and beagle dogs receiving a lower-dose diet containing 0.1% of different white mineral oils (Smith et al. Citation1995).
In MLNs of F-344 rats, oral exposure to mineral oils and waxes increased the incidence and severity of microgranulomas (Baldwin et al. Citation1992; Firriolo et al. Citation1995; Smith et al. Citation1996; Scotter et al. Citation2003; Griffis et al. Citation2010; McKee et al. Citation2012). These are described as “discrete collections of macrophages with medium-sized, vesicular nuclei and abundant eosinophilic, or sometimes faintly pigmented, granular to finely foamy cytoplasm”; “cell boundaries were indistinct” (Carlton et al. Citation2001). Such lipid-laden macrophages are also called foamy histiocytes (Bowdler Citation1990). In F-344 rats, microgranulomas “most commonly occurred in cortical and paracortical zones, in or adjacent to subcapsular or cortical sinuses” (Carlton et al. Citation2001) of MLNs. Histology indicates a microgranuloma associated histiocytosis (Smith et al. Citation1996; Scotter et al. Citation2003) which can be distinguished from a pathology with a more diffuse and loose accumulation of macrophages, located mostly in the medullary sinuses, which is called sinus histiocytosis (Carlton et al. Citation2001; Willard-Mack Citation2006).
The occurrence of microgranulomas in MLNs of female F-344 rats was analyzed in six subchronic dietary studies (Baldwin et al. Citation1992; Firriolo et al. Citation1995; Smith et al. Citation1996; Scotter et al. Citation2003; Griffis et al. Citation2010; McKee et al. Citation2012). To assess the effect size in the treatment groups, three different measures were used (). Baldwin et al. (Citation1992) applied an incidence-severity rating scale with three levels. Firriolo et al. (Citation1995) determined the incidence (%) of microgranulomas. Griffis et al. (Citation2010) and others used an incidence-severity score which is an aggregated measure calculated from incidences and numerical severity grades ().
Across the different subchronic studies, the incidence and severity of microgranoluma formation in MLNs of female F-344 rats showed a clear dependence on dietary concentration and on the type of the test material (). Incidence and severity increased for lower molecular weight materials, including light white oil (P7H), certain low-viscosity mineral oils (N10A, N15H, P15H, N26A) and LMPW. Compared to female F-344 rats, male F-344 rats (Baldwin et al. Citation1992; Smith et al. Citation1996), other rat strains (Firriolo et al. Citation1995; Smith et al. Citation1995; Griffis et al. Citation2010), and beagle dogs (Smith et al. Citation1995) showed much less pronounced or no granulomatous alterations ().
There is no evidence from these subchronic studies, and from chronic studies with medium- and high-viscosity white mineral oils (P70A/H, P70H, P100H) (Shoda et al. Citation1997; Trimmer et al. Citation2004), that the MLN microgranulomas in F-344 rats progress to more a severe pathology such as inflammatory, necrotic or fibrotic lesions (Carlton et al. Citation2001; EFSA Citation2012; JECFA Citation2012). This is supported by findings of histiocytosis in MLNs of rats orally exposed to other high molecular weight, poorly soluble materials such as calcium lignosulfonate (Thiel et al. Citation2013), pentosan polysulphate (Abdo et al. Citation2003), and copovidone (Mellert et al. Citation2004). The presence of foamy histiocytes in MLNs indicate concentration-dependent (overloaded) MOSH-lipid-laden, metabolically activated macrophages which, according to the Joint FAO/WHO Expert Committee on Food Additives (JECFA Citation2012), do not represent an adverse effect as such. The EFSA Panel on Contaminants in the Food Chain (CONTAM Panel) considered that the presence of microgranulomas in MLNs is a nonspecific, adaptive change of low toxicological concern.
Granulomatous lesions of variable size and morphology occurred in the liver of F-344 rats due to the exposure to certain mineral oils and waxes. Some studies subclassified these lesions by size as granulomas and microgranulomas (Miller et al. Citation1996; Smith et al. Citation1996). The latter were defined as small collections of macrophages/histiocytes (three to five) with a few number of lymphocytes in the periphery (Miller et al. Citation1996; Smith et al. Citation1996). Granulomas predominantly consist of aggregations of epithelioid macrophages, with or without the participation of other cell types (Baldwin et al. Citation1992; Firriolo et al. Citation1995; Fleming et al. Citation1998; Griffis et al. Citation2010; Fleming and Carrillo Citation2018). Epithelioid macrophages are “enlarged macrophages with eosinophilic cytoplasm, indistinct cell borders, and vesicular eccentric nuclei, resembling epithelial cells” (Fleming et al. Citation1998). Another description characterizes them as “macrophages with abundant cytoplasm and ill-defined cytoplasmic borders and generally round to oval vesicular nuclei which were more elongated in some cells” (Carlton et al. Citation2001). Depending on the severity of the lesion, these aggregates are variably enclosed by an infiltrate of mononuclear inflammatory cells, mainly lymphocytes, may contain multinucleated Langhans-type giant cells, and occasionally exhibit necrosis and fibrosis (Baldwin et al. Citation1992; Firriolo et al. Citation1995; Smith et al. Citation1996; Fleming et al. Citation1998; Scotter et al. Citation2003; Griffis et al. Citation2010). These aggregates are commonly called “epithelioid (cell) granulomas”.
The occurrence of granulomatous changes in the liver of female F-344 rats was analyzed in seven subchronic dietary studies (Baldwin et al. Citation1992; Firriolo et al. Citation1995; Smith et al. Citation1996; Scotter et al. Citation2003; Griffis et al. Citation2010; McKee et al. Citation2012; Cravedi et al. Citation2017). Granulomas were generally confined to the highest dietary concentrations and mostly seen with low-viscosity mineral oils (N10A, N15H, P15H, N26A, N69H, N70A, N70H) and paraffin waxes (LMPW, IMPW) (). There was no evidence for treatment-related granulomatous changes from subchronic and chronic studies (Smith et al. Citation1996; Shoda et al. Citation1997; Scotter et al. Citation2003; Trimmer et al. Citation2004) with medium- and high-viscosity white mineral oils (P70A/H, P70H, P100H) and microcrystalline waxes (HMPW). Compared to female F-344 rats, male F-344 rats exhibited less severe granulomatous lesions (Baldwin et al. Citation1992; Smith et al. Citation1996). Such treatment-related changes were not observed in other rat strains (Shubik et al. Citation1962; Firriolo et al. Citation1995; Smith et al. Citation1995; Griffis et al. Citation2010) and beagle dogs at all (Smith et al. Citation1995).
Histological examination under polarized light of fresh-frozen liver sections from female F-344 rats being exposed to (n-alkane-rich) LMPW revealed the presence of birefringent material within hepatocytes, Kupffer cells (resident macrophages), and some granulomas, being indicative of crystalloid structures (Smith et al. Citation1996; Scotter et al. Citation2003). Further, electron microscopy showed Kupffer cells to contain crystalloid structures (Griffis et al. Citation2010) and large, irregularly shaped vacuoles, being suggestive of the presence of phagocytozed foreign material (Hoglen et al. Citation1998). These observations would be consistent with the hypothesized crystallization of n-alkanes above C25 in liver of female F-344 rats (Barp et al. Citation2017b). An increased density of lymphoid cell clusters in livers of female F-344 rats exposed to light-liquid paraffin oil (S-C25), paraffin wax-containing liquid paraffin oil (L-C25W), and LMPW was observed as an additional change (Hoglen et al. Citation1998; Cravedi et al. Citation2017).
The specific mechanism of the development of these epithelioid cell granulomas in the liver of F-344 rats is not well understood (Fleming et al. Citation1998; Hoglen et al. Citation1998; Carlton et al. Citation2001; Griffis et al. Citation2010; Adenuga et al. Citation2017). In principle, granuloma formation is regarded as a cellular attempt to engulf an offending agent that is difficult to eradicate, such as a persistent microbe (e.g. M. tuberculosis) or a self-antigen in immune granulomas (Kumar et al. Citation2018). Immunologically, there are two main types of granulomas: immune granulomas caused by immunogenic agents or particles via T cell-mediated immune responses, and foreign body granulomas caused by non-immunogenic foreign material in the absence of T cell-mediated immune responses (de Mello and Ferreira Alves Citation2018; Kumar et al. Citation2018). According to Adenuga et al. (Citation2017), the lesions appear to occur as a result of the elicitation of a “foreign body”-type immune response to MOSH in the liver of F-344 rats.
Alterations of haematological and clinical parameters associated with the histopathological lesions seen in F-344 rats were treatment-related increases in white blood cell and neutrophil counts, and in serum levels of the liver enzymes ALT (alanine aminotransferase), AST (aspartate aminotransferase), and GGT (gamma glutamyl transferase) (Baldwin et al. Citation1992; Firriolo et al. Citation1995; Smith et al. Citation1996; Hoglen et al. Citation1998; Griffis et al. Citation2010; McKee et al. Citation2012). This phenotype was judged by JECFA as indicative of a mild, chronic inflammatory reaction and a mild hepatocyte injury (JECFA Citation2012).
The induction of inflammatory granuloma in the liver of F-344 rats by low-viscosity mineral oils and (n-alkane-rich) paraffin waxes is considered an adverse effect. Yet, the relevance to humans has been repeatedly questioned because of the difference in morphology between the epithelioid cell granulomas seen in F-344 rats and the lipogranulomas observed in humans in association with the ingestion of MOSH (Miller et al. Citation1996; Smith et al. Citation1996; Fleming et al. Citation1998; Carlton et al. Citation2001; Trimmer et al. Citation2004; Griffis et al. Citation2010; Adenuga et al. Citation2017; Fleming and Carrillo Citation2018). In its opinion on low- and medium-viscosity mineral oils in 2012, JECFA noted that the available data were not sufficient to conclude on the relevance for humans of the effects seen in F-344 rats (JECFA Citation2012). Also, the CONTAM Panel in its 2012 opinion on mineral oil hydrocarbons in food stated that information was insufficient to consider the liver (micro)granulomas in the F-344 rat as specific to this strain of rat (EFSA Citation2012). A recent analysis in terms of a mode of action/human relevance framework (MoA/HRF) came to the conclusion that the MoA underlying the formation of MOSH-induced epithelioid granulomas in the F-344 rat model is not relevant for humans (Adenuga et al. Citation2017).
Histopathological lesions associated with the deposition of MOSH in human tissues
MOSH-associated lesions in human tissues occur as oil droplets. In microscopic images of paraffin-embedded tissue sections, they appear as clear spaces, demarcating previous sites of putative MOSH deposits that had been dissolved out during histological processing (Carlton et al. Citation2001). Mainly affected are the abdominal lymph nodes, spleen, and liver. The deposits appear as focal collections of extra- and intracellular vacuoles of markedly varying size (Stryker Citation1941; Boitnott and Margolis Citation1966b; Rose and Liber Citation1966; Liber and Rose Citation1967; Kelsall and Blackwell Citation1969; Boitnott and Margolis Citation1970; Nochomovitz et al. Citation1975; Blewitt et al. Citation1977; Klatskin Citation1977; Dincsoy et al. Citation1982; Cruickshank Citation1984; Cruickshank and Thomas Citation1984; Wanless and Geddie Citation1985; Fleming et al. Citation1998; Carlton et al. Citation2001; Fleming and Carrillo Citation2018). The intracellular vacuoles occur in lipophages (vacuolated macrophages) and in multinucleated giant cells, which are occasionally present (Stryker Citation1941; Boitnott and Margolis Citation1966b; Kelsall and Blackwell Citation1969; Boitnott and Margolis Citation1970; Fleming et al. Citation1998; Carlton et al. Citation2001; Fleming and Carrillo Citation2018). Other associated inflammatory cells, such as lymphocytes and plasma cells, are present in varying amounts (Stryker Citation1941; Rose and Liber Citation1966; Kelsall and Blackwell Citation1969; Nochomovitz et al. Citation1975; Blewitt et al. Citation1977; Klatskin Citation1977; Dincsoy et al. Citation1982; Cruickshank and Thomas Citation1984; Wanless and Geddie Citation1985; Fleming et al. Citation1998; Fleming and Carrillo Citation2018). Associated scarring fibrosis is rare and, if present, usually of mild degree (Blewitt et al. Citation1977; Cruickshank and Thomas Citation1984; Fleming et al. Citation1998; Fleming and Carrillo Citation2018). All the above-mentioned components form an aggregate, which is commonly called “lipogranuloma” (Klatskin Citation1977; Dincsoy et al. Citation1982; Cruickshank Citation1984; Cruickshank and Thomas Citation1984; Wanless and Geddie Citation1985; Fleming et al. Citation1998; Bellamy and Burt Citation2018; Fleming and Carrillo Citation2018).
The characteristic morphology and its correlation with deposits of mineral oil was first discovered in postmortem tissues by Stryker (Citation1941). Studies published later used specimens from autopsies and biopsies performed between as early as 1880 and 1980. Lipid histochemistry involving differential staining of fresh-frozen tissue sections was applied in an attempt to distinguish MOSH droplets from deposits of biological lipids (Stryker Citation1941; Boitnott and Margolis Citation1966a, Citation1966b; Liber and Rose Citation1967; Boitnott and Margolis Citation1970; Nochomovitz et al. Citation1975; Blewitt et al. Citation1977; Dincsoy et al. Citation1982). Chemical methods comprised the extraction of tissue lipids followed by identification and, sometimes, quantification of saturated hydrocarbons using, e.g. molecular sieves, column and thin-layer chromatography, gravimetry, infrared spectroscopy, GC–FID, and MS (Boitnott and Margolis Citation1966a, Citation1966b; Rose and Liber Citation1966; Liber and Rose Citation1967; Boitnott and Margolis Citation1970; Nochomovitz et al. Citation1975; Blewitt et al. Citation1977; Dincsoy et al. Citation1982). MS identified complex mixtures of predominantly branched and cyclic compounds, a composition typical of mineral oils (Boitnott and Margolis Citation1966b, Citation1970; Barp et al. Citation2014). GC–FID analysis of saturated hydrocarbons isolated from histologically positive spleens revealed a largely unresolved hump ranging from C21 to C28 (Rose and Liber Citation1966; Liber and Rose Citation1967). A clear positive correlation was observed between the incidence and severity of histological lesions and the MOSH concentration in the respective tissues () (Boitnott and Margolis Citation1966b; Liber and Rose Citation1967; Boitnott and Margolis Citation1970; Cruickshank Citation1984).
Affected lymph nodes show lesions restricted to the cortex and medullary cords (Stryker Citation1941; Kelsall and Blackwell Citation1969), but also a scattered distribution of lesions throughout the node has been described (Carlton et al. Citation2001). The lymph nodes mainly affected are those associated with the gastrointestinal tract and the liver (Boitnott and Margolis Citation1966b; Kelsall and Blackwell Citation1969; Nochomovitz et al. Citation1975; Cruickshank and Thomas Citation1984). Hepatic lymph nodes receive lymph fluid formed in the perisinusoidal spaces of Disse and drained into the lymphatic vessels surrounding the portal triads (Ohtani and Ohtani Citation2008; Moore et al. Citation2014). Lymph nodes from non-abdominal sites (e.g. neck and axilla) were found to show only an infrequent and scanty involvement (Kelsall and Blackwell Citation1969; Cruickshank Citation1984). In hepatic lymph nodes obtained from 49 autopsies, oil droplets were virtually absent at estimated MOSH concentrations of less than 100 ppm but invariably present at levels greater than 250 ppm () (Boitnott and Margolis Citation1966b). A high incidence of hepatic lymph node lesions of 55–88% was noted in autopsies (N = 61–300) performed in North America and Australia in the 1960s and early 1970s (Boitnott and Margolis Citation1966b; Kelsall and Blackwell Citation1969; Cruickshank and Thomas Citation1984). For the mesenteric lymph nodes, somewhat lower incidences were reported (Stryker Citation1941; Kelsall and Blackwell Citation1969; Cruickshank and Thomas Citation1984).
Splenic lesions are generally located in or close to lymphoid follicles and in the periarteriolar lymphoid sheaths (Stryker Citation1941; Hudson and Robertson Citation1966; Liber and Rose Citation1967; Boitnott and Margolis Citation1970; Nochomovitz et al. Citation1975; Cruickshank Citation1984; Carlton et al. Citation2001). The pathology is also described as “follicular lipidosis” (Rose and Liber Citation1966; Liber and Rose Citation1967; Cruickshank Citation1984; Cruickshank and Thomas Citation1984). In 26 autopsy spleens, oil droplets were absent at MOSH concentrations less than 200 ppm but invariably present at levels greater than 500 ppm () (Boitnott and Margolis Citation1970). A similar correlation was obtained in another autopsy study: the six histologically negative spleens had MOSH concentrations of 60–590 ppm whereas seven positives had levels of 270 ppm and 1120–2660 ppm, respectively (Rose and Liber Citation1966; Liber and Rose Citation1967). In North America, a high incidence of 46–76% was reported for autopsy material (N = 100–465) from the 1960s and 1970s (Liber and Rose Citation1967; Cruickshank and Thomas Citation1984; Wanless and Geddie Citation1985); three-fold lower incidences (18–24%) were observed for the 1940s (Stryker Citation1941; Cruickshank Citation1984). This historical trend can be traced back further: the incidence was zero in 1882–1937 (N = 115) compared to 33% in 1938–1956 (N = 70) (Liber and Rose Citation1967). Epidemiological analyses of autopsy material from the 1970s identified a higher incidence and severity of splenic lesions in men than in women (Cruickshank Citation1984; Wanless and Geddie Citation1985). A histologic study of spleens from autopsies (N = 50 per center) performed in 74 centers of 41 countries showed a marked geographic variation: The incidences were 50% in North America and Australasia, 30% in Great Britain, and 0–11% in continental Europe countries (Cruickshank Citation1984). The study authors explained this variation by the ingestion of MOSH from sources related to the packaging and protective coating of foodstuffs in the more affluent societies (Cruickshank Citation1984).
MOSH-related hepatic lesions tend to occur in the portal fields rather than in the parenchyma. They are usually found in the pericentral region, i.e. located around the central vein of the liver lobule (Stryker Citation1941; Boitnott and Margolis Citation1970; Nochomovitz et al. Citation1975; Blewitt et al. Citation1977; Dincsoy et al. Citation1982; Cruickshank and Thomas Citation1984; Wanless and Geddie Citation1985; Trivalle et al. Citation1991; Fleming et al. Citation1998; Carlton et al. Citation2001; Bellamy and Burt Citation2018; Fleming and Carrillo Citation2018). This differential zonal involvement is, however, not consistent across studies (Dincsoy et al. Citation1982; Wanless and Geddie Citation1985). Reversibility of MOSH retention as well as a different persistence potential between severe and mild lesions (e.g. regression of smaller oil droplets) following reduction in exposure might be additional explanatory factors to those already discussed elsewhere (Wanless and Geddie Citation1985). What complicates the identification of MOSH-related lesions is that morphologically similar changes may result from the accumulation of biological lipids, mainly triglycerides, in steatotic livers of patients with, e.g. fatty liver disease or hepatitis C infection (Delladetsima et al. Citation1987; Denk et al. Citation1994; Zhu et al. Citation2010; Coash et al. Citation2012; Bellamy and Burt Citation2018; de Mello and Ferreira Alves Citation2018). Some fat-related lipogranulomas might even persist in subjects with resolved fatty liver disease (Zhu et al. Citation2010). Lipid histochemistry with differential staining and chemical analysis of tissue lipid extracts are therefore crucial, but have not always been used to support the identification of MOSH-related lesions.
In 42 autopsy livers, oil droplets were absent in the portal tracts at MOSH concentrations less than 380 ppm but invariably present at levels greater than 800 ppm () (Boitnott and Margolis Citation1970). MOSH concentration in liver was positively correlated with that in spleen (r = 0.76, N = 23) (Boitnott and Margolis Citation1970). In North America, a high incidence (45 and 48%) of hepatic lesions was reported for autopsy material (N = 100 and 465) from the early to mid-1970s (Cruickshank and Thomas Citation1984; Wanless and Geddie Citation1985); a three-fold lower incidence (16%) was reported for autopsy material (N = 25) from the 1940s (Stryker Citation1941), which is consistent with the historical trend observed for the splenic lesions. A strong association was found between the presence of lipogranulomas in liver and spleen of the same cases (Wanless and Geddie Citation1985). In 824 consecutive liver biopsies performed in North America in 1978–1980, a considerably lower incidence (4.6%) of lipogranulomas in non-fatty livers was reported (Dincsoy et al. Citation1982). A review of 240 biopsies carried out at the same center in the early 1950s showed an incidence of 1.7% (Dincsoy et al. Citation1982), again indicating a historical trend.
The only recent study is that of Barp et al. (Citation2014). Liver and other tissues were collected from 37 subjects during autopsy performed in Austria in 2013. MOSH concentrations were in the range of 14–901 ppm for liver and 6–1400 ppm for spleen (Barp et al. Citation2014). The LC–GC–FID chromatograms for both tissues showed MOSH humps that were completely devoid of skinny peaks indicative of n-alkanes (Barp et al. Citation2014). In a later publication Barp and others indicated that “virtually no granulomas were observed” in both tissues (Barp et al. Citation2017b). The absence of MOSH-associable lesions in these autopsy patients could result from a considerably lower dietary exposure in post-2000 Europe compared to pre-2000 North America. In the U.S., the average exposure to mineral oils and waxes from food-use applications was estimated to be approximately 2 mg/kg bw per day in the 1960s (Boitnott and Margolis Citation1966b) and 0.875 mg/kg bw per day in 2002 (Heimbach et al. Citation2002). These intakes are 1–2 orders of magnitude higher than the mean background exposure to MOSH which was estimated by EFSA using European food contamination data, most of which were collected in 1997–2010 (EFSA Citation2012).
In conclusion, MOSH-related lipogranulomas in human livers are morphologically quite different from inflammatory epithelioid granulomas seen in F-344 rats following the oral exposure to low-viscosity mineral oils and (n-alkane-rich) paraffin waxes (Miller et al. Citation1996; Smith et al. Citation1996; Fleming et al. Citation1998; Carlton et al. Citation2001; Fleming and Carrillo Citation2018). The lesions in human livers and other affected tissues are generally regarded as incidental findings with no clinical significance (Stryker Citation1941; Klatskin Citation1977; Dincsoy et al. Citation1982; Cruickshank and Thomas Citation1984; Wanless and Geddie Citation1985; Fleming et al. Citation1998; Carlton et al. Citation2001; Bellamy and Burt Citation2018; Fleming and Carrillo Citation2018). The EFSA CONTAM Panel in its 2012 opinion noted that “the clinical significance of the lipogranulomas is not known, but their presence had not been associated with inflammatory responses, and not reported to be associated with clinical abnormalities” (EFSA Citation2012). The Panel concluded that “whilst dietary exposure to mineral oil hydrocarbons could result in the formation of lipogranulomas in the liver and other tissues of humans, the current incidence is very low and that these do not appear to have any adverse consequences”.
While a general agreement has been reached on the non-adversity of MOSH-related lipogranulomas in humans, this is not the case for the human relevance of the epithelioid cell granulomas seen in the F-344 rat model (EFSA Citation2012; JECFA Citation2012; Adenuga et al. Citation2017). To solve this issue, the question has been raised whether there could be an atypical response to MOSH in humans resulting in granulomas of similar morphology to those in F-344 rats (Fleming et al. Citation1998; Fleming and Carrillo Citation2018). This led to the question whether some/all of the idiopathic (unexplained) granulomas in human biopsies could be atypical reactions to MOSH. There is no evidence that this is the case. But even if it were, a worst-case scenario suggests that the implied numbers of cases across the EU are low and a progression to significant liver disease is unlikely (Fleming and Carrillo Citation2018).
MOAH
Gastrointestinal and dermal absorption of MOAH and PAHs
Data on the gastrointestinal and dermal absorption of complex mixtures of highly alkylated MOAH are virtually non-existent. Given that MOAH are structurally and physico-chemically related to PAHs and MOSH, and given that MOAH occur associated with MOSH in petroleum products, it is likely that they are absorbed to comparable degrees. Upon absorption MOAH are likely being metabolized (oxidized) as they could not be detected in human tissues that are burdened by high levels of MOSH (Barp et al. Citation2014). The absorption of MOSH has already been reviewed in the previous sections. This section complements the picture with information on PAHs.
Following oral ingestion, PAHs (including simple methylated compounds) are absorbed in the gastrointestinal tract via the lymphatic system and portal vein, then metabolized, and finally excreted (Laher et al. Citation1984; Laher and Barrowman Citation1987; Lindstrom et al. Citation1987; Laher and Barrowman Citation1988; Laurent et al. Citation2002; Cavret et al. Citation2003; Ramesh et al. Citation2004; Harris et al. Citation2013; Harris et al. Citation2016). PAHs with two or three aromatic rings can be absorbed more rapidly and extensively than those with five or six rings (Rahman et al. Citation1986; Laurent et al. Citation2002; Cavret et al. Citation2003). The transfer into circulation occurs concurrently with dietary lipids (Stavric and Klassen Citation1994; Harris et al. Citation2016). Co-administration of long-chain dietary lipids and bile salts significantly facilitates the absorption of the larger, more lipophilic PAHs (Laher et al. Citation1983; Rahman et al. Citation1986; Barrowman et al. Citation1989). Bioavailability data exist for some simple substituted compounds such as mono- and di-methylated naphthalene and di-methylated benzanthracene (Laher et al. Citation1983; Rahman et al. Citation1986; Laher and Barrowman Citation1987; Barrowman et al. Citation1989), but also for di-isopropylated naphthalene (Kojima et al. Citation1978).
Upon dermal exposure, PAHs are well absorbed and become systemically available. When reaching the viable epidermis, PAHs can already be converted into a variety of metabolites such as phenols (hydroxy derivatives), dihydrodiols (diols), quinones (diones), and catechols (Pannatier et al. Citation1978; Ng et al. Citation1991, Citation1992; Jacques et al. Citation2010; Brinkmann et al. Citation2013). These derivatives can be further converted into glucuronide and sulfate conjugates (Pannatier et al. Citation1978; Ng et al. Citation1992; Jacques et al. Citation2010). The extent of percutaneous absorption of PAHs is negatively related to their molecular size and hydrophobicity (Vanrooij et al. Citation1995; Roy et al. Citation1998; Gute et al. Citation1999; Sartorelli et al. Citation1999; Moss et al. Citation2002; Beriro et al. Citation2016). Dermal absorption is also influenced by extrinsic factors such as PAH concentration in the vehicle, the amount of formulation applied per skin area, the viscosity of the vehicle, the stratum corneum/vehicle partition coefficient (Dankovic et al. Citation1989; Sartorelli et al. Citation1999), and the anatomical site of application (Vanrooij et al. Citation1993). In vitro and in vivo experiments with different mineral oil products spiked with radiolabelled benzo[a]pyrene (B[a]P) showed a reduced percutaneous absorption of B[a]P with increasing viscosity of the mineral oil product (Roy et al. Citation1996; Potter et al. Citation1999).
Carcinogenicity of PAHs and MOAH
Several PAHs with four to six aromatic rings, including methylated derivatives, are carcinogenic in animal studies (JECFA Citation2006; EFSA Citation2008; IARC Citation2010). Mono- and polymethylations can convert non-carcinogenic PAHs (e.g. pyrene, anthracene) into genotoxic carcinogens, depending on the methylation position (LaVoie et al. Citation1985; Rice et al. Citation1987; Surh et al. Citation1990). Conversely, a higher degree of alkylation abrogates carcinogenicity of certain PAHs if the alkylated derivatives become directed to non-toxifying metabolic pathways (→ side-chain oxidation instead of ring oxidation). Isopropylation of naphthalene is an example (Honda et al. Citation1990; Höke and Zellerhoff Citation1998). Bulky ring substitutions can also eliminate carcinogenicity by preventing the intercalation of activated derivatives into DNA. Apart from alkylation, partial hydrogenation can also modulate the tumorigenic activity of PAHs (Lijinsky et al. Citation1965; Lijinsky et al. Citation1970; Lijinsky and Garcia Citation1972). In general, however, it is not predictable how alkylation and partial hydrogenation affect the carcinogenic potential of parent PAHs.
For mineral oils, products with a high concentration of polycyclic aromatic compounds (PAC) with 3–7 rings (parent PAHs, short-alkylated MOAH, compounds containing heteroatoms), such as unrefined and poorly refined base oils, were carcinogenic in mouse skin painting assays (Roy et al. Citation1988; McKee, Daughtrey, et al. Citation1989; Blackburn et al. Citation1996; Mackerer et al. Citation2003). The aromatics concentration was determined by target analytics for specific parent PAHs (McKee, Daughtrey, et al. Citation1989; Concawe Citation1994) and by solvent extraction with dimethyl sulfoxide (DMSO) followed by GC separation according to the ring number using two to seven ring unsubstituted PAHs as internal standards (Roy et al. Citation1988; Mackerer et al. Citation2003; Gray et al. Citation2013). The latter technique is used by the Mobil PAC Method for predicting dermal carcinogenicity (see below).
The mouse skin painting bioassay is used as an indicator assay for carcinogenicity. It is performed in two different experimental designs, as a chronic or as an initiation/promotion (I/P) bioassay. Mineral oils (C15–C50) were mostly tested in chronic bioassays (see below), with the exception of one highly solvent-refined mineral oil stream, which was tested in both kinds of protocols (Skisak et al. Citation1994). Substances of other petroleum-derived categories with lower carbon number ranges were tested as well, including kerosenes (C9–C16) such as jet fuel, gas oils (C9–C30) such as diesel fuel, and hydrocarbon solvents (C5–C20) (Clark et al. Citation2013; McKee and White Citation2014; McKee et al. Citation2015). These substance mixtures represent, together with mineral oils, relevant sources of food contamination (see Chapter “Evaluation of mineral oil hydrocarbons in food by the EFSA CONTAM Panel”) so that they are briefly discussed below with respect to their carcinogenic properties.
Some gas oils contain aromatic constituents with more than three rings in toxicologically relevant concentrations which induce skin tumors in mice via genotoxicity (Clark et al. Citation2013; McKee and White Citation2014). In addition, kerosenes, gas oils, and hydrocarbon solvents can produce skin tumors. These tumors appear to be caused by a promotional process secondary to repeated dermal irritation as they are not mutagenic and thus unable to act as cancer initiating agents (McKee, Plutnick, et al. Citation1989; Nessel et al. Citation1998; Nessel et al. Citation1999). This skin irritation-based promotion effect seems to apply to alkylbenzene mixtures such as C10–C15 (predominantly C12) branched-chain alkyl benzene (i.e. dodecylbenzene) as well (Iversen Citation1989; Assogne Citation1990; Ingram and Grasso Citation1991; European Chemicals Bureau Citation1999). It also seems to apply to a jute batching oil variety (JBO-P) (Mehrotra et al. Citation1988), a straight-run unrefined broad cut from the atmospheric crude distillation unit that includes constituents of both kerosene and base oil (Agarwal et al. Citation1985; Agarwal et al. Citation1986; Chaudhuri Citation2011). This JBO-P additionally carries cancer initiating activity due to the presence of a fraction of PAHs with more than three rings (Agarwal et al. Citation1988; Mehrotra et al. Citation1988).
The following paragraphs are focused on mineral oils and waxes. The carcinogenic potential of mineral oils and waxes has been assessed in the food safety area for the oral exposure route and in the area of industrial chemicals for the dermal exposure route.
The Panel on Food Additives and Nutrient Sources added to Food (ANS Panel) of the European Food Safety Authority (EFSA) evaluated the safety of certain mineral hydrocarbon products for their use as food additives, including certain medium viscosity white mineral oils (MVMO) (EFSA Citation2013b) and high viscosity white mineral oils (HVMO) (EFSA Citation2009), as well as a microcrystalline wax (E 905) (EFSA Citation2013a). The physical specifications of these products are given in . Purity specifications were set in order to limit the residual amounts of PAHs in these products. For MVMO and HVMO, the purity specifications stipulate that the UV absorbance of a DMSO extract of the white mineral oil is not higher than that of a reference. For microcrystalline wax, a limit level of 50 μg/kg is specified for B[a]P. MOAH (total aromatics) are not addressed in these purity specifications but are still present in these products. Based on available data, the ANS Panel concluded that there is no concern for genotoxicity of these products. In addition, no carcinogenic effect was observed in chronic dietary studies with HVMO and MVMO in F-344 rats (Shoda et al. Citation1997; Trimmer et al. Citation2004), and with paraffin and microcrystalline waxes in SD rats (Shubik et al. Citation1962).
There is sufficient evidence from occupational epidemiological studies that dermal exposure to untreated and mildly treated mineral oils causes skin cancer in humans (IARC Citation2012). The current gold standard for determining the dermal carcinogenicity of base oils is the chronic mouse skin-painting bioassay (Chasey and McKee Citation1993; Concawe Citation1994; Blackburn et al. Citation1996; Mackerer et al. Citation2003), which was designed to mimic the adverse effects seen in humans. For routine use, the petroleum industry has developed quicker alternatives based on in vitro bioassays and chemical procedures for predicting dermal carcinogenicity. These are the modified Ames test (Mackerer et al. Citation2003), the Mobil PAC Method (Roy et al. Citation1988; Roy et al. Citation1995; Mackerer et al. Citation2003), and the IP 346 method (Institute of Petroleum Citation2004). Of regulatory relevance in the EU is the IP 346, which is a gravimetric method that determines the weight percentage of DMSO extractables (e.g. PAC) in the oil (Chasey and McKee Citation1993; Mackerer et al. Citation2003). The two-step solvent extraction protocol enables a selective enrichment of fused aromatic ring systems with three to seven rings. This is due to the affinity of the sulfur atom of DMSO to the π-electron-rich aromatic ring systems (Natusch and Tomkins Citation1978). The extraction efficiency decreases with decreasing size and increasing extent of alkylation of the aromatic ring systems, respectively.
According to Concawe, a division of the European Petroleum Refiners Association, the IP 346 method specifically applies to unused lubricating base oils with at least 95% of components with a boiling point beyond 300 °C (Concawe Citation2016). The method has been used in the EU for the classification of base oils as carcinogens. Under the CLP Regulation (EC) No 1272/2008, the members of three categories of petroleum substances (i.e. other lubricant base oils, foots oils, treated distillate aromatic extracts) (Concawe Citation2001) are considered carcinogenic (category 1B) unless it can be shown that the substance contains less than 3% (w/w) DMSO extractable material.
The applicability of the IP 346 for predicting the dermal carcinogenicity of certain base oils is based on the positive correlation between the DMSO extractable material and the skin cancer incidence observed in more than 100 mouse skin-painting studies performed by the petroleum industry in the 1970s and 1980s (Concawe Citation1994). The data base describing this relationship includes samples of substances from five petroleum substance categories (Concawe Citation2016): highly refined base oils (HRBO, the white oils), other lubricant base oils (OLBO), unrefined/acid-treated oils, treated distillate aromatic extracts, and untreated distillate aromatic extracts (). The data base also includes blends thereof. HRBO and OLBO are commonly known as “mineral oils”. Test materials were applied undiluted in a defined volume to the shaved backs of the animals 1–3 times per week (Doak et al. Citation1983; Blackburn et al. Citation1984; Doak et al. Citation1985; Blackburn et al. Citation1986; Roy et al. Citation1988; McKee, Daughtrey, et al. Citation1989; McKee, Nicolich, et al. Citation1990; Chasey and McKee Citation1993; Concawe Citation1994). In some cases, test materials were diluted in highly refined mineral oil or toluene for reasons of high toxicity or high viscosity (Blackburn et al. Citation1986; McKee, Daughtrey, et al. Citation1989; McKee, Nicolich, et al. Citation1990). Only some studies reported the size of the dermal treatment area (McKee, Daughtrey, et al. Citation1989; Chasey and McKee Citation1993). White mineral oil and toluene were reported to be used as negative/vehicle controls, and B[a]P (in toluene or acetone) and catalytically cracked clarified oil as positive controls (Doak et al. Citation1985; Blackburn et al. Citation1986; McKee, Daughtrey, et al. Citation1989; McKee, Nicolich, et al. Citation1990; McKee, Scala, et al. Citation1990). Shaved but untreated animals were reported to be used as additional negative controls (Doak et al. Citation1985; Blackburn et al. Citation1986; McKee, Scala, et al. Citation1990).
The dermal carcinogenicity studies were conducted with two mouse strains of different sex, female CF1 mice and male C3H mice. Dose-response profiles of these mice demonstrate a somewhat different responsiveness (). The data for C3H mice showed a pronounced scattering around the steep part of the fitted dose response curve (). This suggests that additional factors (e.g. PAH profile, different treatment regimens) influence the tumorigenic response of samples with elevated levels of DMSO extractable material. Unfortunately, incomplete information on test conditions and on physical and chemical data prevents a statistically based analysis of additional predictors.
In the respective studies an oil sample was judged (potentially) carcinogenic if at least two out of 50 test animals (≥4%) developed skin tumors of benign or malignant nature (Blackburn et al. Citation1986; Concawe Citation1994). The background tumor rate in negative controls treated with highly refined mineral oil was well below 1%. An analysis of negative controls of three carcinogenesis programs revealed that following treatment with highly refined mineral oil two out of 610 C3H mice (=0.3%) developed benign papillomas and none developed carcinomas (McKee, Nicolich, et al. Citation1990). The same order of magnitude was found when the relationship between skin cancer incidence and DMSO extractable content was modeled. For CF1 and C3H mice this results in comparable background tumor rates of 0.19 and 0.12%, respectively (). Similarly, rates of 0.2% and <0.5% (none out of 200) were reported for shaved and untreated controls (Doak et al. Citation1983; Blackburn et al. Citation1986). Toluene-treated vehicle controls showed tumor rates of 0.6% (2 out of 350 animals) (Blackburn et al. Citation1986).
Compared to these background rates the selected carcinogenic threshold of 4% might appear high. However, given the typical sample size of 50 animals per group this value is statistically well justified as the following example illustrates. If 5 out of 50 animals (i.e. 10%) were to develop a tumor in the treatment group and none of the animals in the control group, the one-sided Fisher’s exact test results in a p-value of 0.028 and thus well below the common significance level of 0.05. Hence a threshold of 4% (i.e. 2 of 50 animals), instead of 10%, can be deemed conservative. Alternatively one could refer to the tumor rate of the historical controls as a set background. With a set background of 0.2% and a sampling size of 50 animals, 4% is then the minimum tumor rate which can be statistically resolved by a one-sided exact binomial test and a significance level of 0.05. Discussions of this value by Concawe with the EU Technical Progress Committee Classification and Labeling working group and the IARC, respectively, found this 4% classification threshold “to be valid, although possibly severe” (Concawe Citation1994).
To determine the threshold for the DMSO extractable content that gives the most accurate discrimination between potentially carcinogenic and non-carcinogenic base oils, Concawe applied a binary classification analysis to a data set of 104 samples using decision thresholds (or cutoffs) of 1, 2, and 3% (w/w) of DMSO extract (Concawe Citation1994). The data set included samples of substances from five petroleum substance categories and blends thereof (). The cutoff of 3% (w/w) DMSO extractables yielded the highest accuracy (=94%). There were three false negative predictions and three false positives which resulted in a sensitivity of 92% and a specificity of 96%. In 2016, Concawe reviewed the data and included 29 new study results on substances within the chemical scope of the IP 346 (Concawe Citation2016). The re-analysis of the enlarged data set of 133 samples yielded a virtually identical sensitivity of 93% and a slightly lower specificity of 91%.
For mineral oil, it is particularly the performance characteristics of the 92 tested base oils in the HRBO and OLBO categories which are relevant (Concawe Citation2016). There were three false negative predictions and seven false positive ones. The false negatives had a tumor incidence of 5–10% and 1.77–2.82% (w/w) of DMSO extractable content (). The sensitivity was 86% and the specificity 90%. The sensitivity describes the ability of the IP 346 to positively identify base oils that are also tested carcinogenic in the mouse skin test. The alignment of the two tests is crucial for the applicability of the IP 346 for safety testing. Of equivalent importance, at least for consumer safety, is the negative predictive value (NPV). The NPV describes the probability that a base oil being predicted to be non-carcinogenic by the IP 346 also is non-carcinogenic in the mouse skin test. The NPV can be calculated from sensitivity, specificity, and prevalence, the latter being the proportion of base oils that are (or would be) carcinogenic in mice. The prevalence among the 92 base oils in the aforementioned data set was 21%, which translates into a NPV of 95% (Concawe Citation2016). It should be noted that for commercial products the NPV would rather expected to be higher, as the prevalence amongst these oils is likely to be lower than 21%. The reason is that a large part of the Concawe database originates from one company’s samples, most of which were prepared with laboratory pilot units with the tumorigenic oils being specifically refined by experimental conditions that are, according to Chasey and McKee (Citation1993), not representative of those used in commercial refineries.
In addition to the assessment of carcinogenicity under the CLP regulation, the IP 346 is used as a qualifier for refining efficiency. In case of mineral white oils and waxes used in food, pharmaceutical or cosmetic applications, the IP 346 is applied to the solvent-extracted vacuum distillate (also called waxy raffinate) from which these substances originate. Only if the feedstock passes the IP 346 test, it is subjected to further processing such as dewaxing, de-oiling, hydro-treatment, acid and clay treatment (). According to industry representatives, these processing steps are quite efficient and result in a pronounced reduction of PAC and MOAH concentrations, DMSO extractable contents, and mutagenicity indexes (Jouanneau Citation2017; Sothmann and Südkamp Citation2017). The achievable reduction of MOAH depends on whether it is a white oil, a hard paraffin wax, or a microcrystalline wax. Representative data for commercial products from different oil companies are not available.
In summary, mineral oil products with a high proportion of three to seven aromatic ring constituents, such as unrefined and poorly refined base oils, are carcinogenic in the mouse skin painting bioassay. The IP 346 is used as a quasi-analytical tool to predict the carcinogenicity of mineral oils. Since its introduction, it developed into a key indicator method applied in carcinogenicity classification of certain petroleum stream categories under the CLP regulation. It is also used as a qualifier for refining efficiency in the manufacturing of mineral oils and waxes. For highly refined white mineral oils and waxes, there are no indications from animal studies for and no epidemiological evidence of carcinogenicity.
Evaluation of the inhalation toxicity of pharmaceutical white oil by the german MAK commission
The Permanent Senate Commission for the Investigation of Health Hazards of Chemical Compounds in the Work Area (MAK Commission) proposes, inter alia, maximum workplace concentrations (MAK values) for volatile chemicals and dusts in the air. These proposals are published in the annual list of MAK values. In 2015, the MAK Commission published its evaluation of pharmaceutical white oil and proposed a MAK value for the inhalation exposure of workers to aerosols of such oils (MAK Citation2015).
White mineral oil (CAS no. 8042-47-5) is defined as a “highly refined petroleum mineral oil consisting of a complex combination of saturated hydrocarbons (carbon numbers predominantly in the range of C15–C50) obtained from the intensive treatment of a petroleum fraction with sulfuric acid and oleum, or by hydrogenation, or by a combination of hydrogenation and acid treatment” (ECHA Citation2017c). Additional washing and treating steps may be included in the processing operation. Compared to technical white oil, which may contain traces of potentially carcinogenic aromatic hydrocarbons, pharmaceutical white (mineral) oil is more thoroughly refined and has to comply with the European Pharmacopeia (see Chapter “Mineral oils in medicinal products”). Pharmaceutical white oil is for example used as a laxative, as a base for ointments, creams, hair care products, and baby oils. Furthermore, it serves as lubricant for machines used in the pharmaceutical, cosmetics, and food packaging industry.
Inhaled mineral oil deposited in the lungs is taken up by macrophages and, presumably because of incomplete phagocytosis and/or biotransformation, can cause inflammation and microgranulomas through to fibrotic responses. This pathology is called exogenous lipid pneumonia. For humans, there are reports on exogenous lipid pneumonia after inhalation or accidental aspiration of mineral oil products (Cullen et al. Citation1981; Spickard and Hirschmann Citation1994; Bandla et al. Citation1999). Quantitative exposure data are lacking though. For the derivation of a MAK value, the MAK Commission followed the same approach as the Scientific Committee on Occupational Exposure Limits (SCOEL Citation2011). Since inhalation studies with pharmaceutical white oil were not available, four animal inhalation studies with highly refined mineral oils as well as with white oils of not exactly known composition and PAH content were used for deriving the MAK value.
The MAK Commission evaluated a 4-week study with hydro-treated and acid-washed white oil (60% paraffinic, “no aromatics”) (Dalbey et al. Citation1991) and a 13-week study with three highly refined mineral oils (cutting oil, gear oil, engine oil) (Dalbey Citation2001). Both studies were conducted with SD rats. Two chronic (6–26 months) studies with a white mineral oil (25–30 carbon atoms, 95% naphthenic with 1–6 rings) (Wagner et al. Citation1964) and with a fiber-yarn preparation (70% paraffinic mineral oil, 30% synthetic fiber adjuvants) plus 1000 ppm acetone (fiber solvent) in the test atmosphere were also evaluated (Stula and Kwon Citation1978). These studies used SD rats, mongrel dogs, CF and CAF/JAX mice, rabbits, Syrian hamsters, and gerbils. The animals were exposed to a respirable mineral oil aerosol with a mass median aerodynamic diameter (MMAD) of approximately 1–2 µm.
Rats and dogs responded most sensitively in these studies. In the chronic studies at an aerosol concentration of 5 mg/m³, these species showed oil-filled macrophages in the lung which, in the absence of any other toxicity, was considered as a physiological reaction rather than an adverse effect, so that this concentration was regarded as the chronic NOAEC (No Observed Adverse Effect Concentration). Subacute and subchronic exposures of rats to higher aerosol concentrations resulted in the following effects: occurrence of oil-filled macrophages (foam cells) in the alveoli, macrophage accumulation in the alveoli and tracheobronchial lymph nodes, neutrophil infiltration in the alveoli, thickening of alveolar walls, epithelial hyperplasia, increase of pulmonary hydroxyproline (collagen marker for fibrotic changes), and increased lung wet weight. The chronic exposure of rats and dogs to 100 mg/m3 resulted in an increase in alkaline phosphatase (indication of inflammatory processes in the lung) and in the occurrence of microgranulomas and lipogranulomas in the lung. These granulomatous reactions were considered as adverse and therefore established a LOAEC (Lowest Observed Adverse Effect Concentration). At the same aerosol concentration, hamsters, mice, and gerbils only showed some oil-filled macrophages in the lung. The most sensitive mouse strain (CAF/JAX mice) gave no indications of tumor promotion in the lung.
Based on these results, the MAK Commission derived a MAK value of 5 mg/m3 for the respirable fraction (i.e. alveolar fraction) of aerosols of pharmaceutical white oil. Given the large margin between the chronic NOAEC (5 mg/m3) and the chronic LOAEC (100 mg/m3) as well as the NOAEC of 50 mg/m3 in a subchronic study, and further considering that the effect was attributed to a local overload of the lung, for which the inter-individual differences were judged to be low, and that there was no evidence for tumor promotion in the lung, no further safety factors were applied.
Evaluation of mineral oil hydrocarbons in food by the EFSA CONTAM panel
In 2012 the EFSA Panel on Contaminants in the Food Chain (CONTAM) published a scientific opinion on mineral oil hydrocarbons (MOH) in food (EFSA Citation2012). The initiation of risk assessment was triggered by the detection of highly contaminated batches of sunflower oil being imported into the EU. The term “mineral oil hydrocarbons” was used by the CONTAM Panel in a broader sense, as it comprised not only the entry into and contamination of food from mineral oil products (e.g. white oils, lubricants) but also the contamination from products belonging to other categories of petroleum-derived substances such as diesel fuel and hydrocarbon solvents. The focus was particularly on hydrocarbons within the carbon number range of C10 to C50. Hydrocarbons with carbon numbers outside this range were not considered relevant because of the high volatility of the smaller and the low absorption of the larger compounds.
MOH occur in food as a result of intended uses of food-grade products (e.g. as food additives, additives used in food-contact materials; see ) and due to contamination. Food becomes contaminated via the environment, food processing, and migration from food packaging materials such as paperboard (see Chapter “Activities of surveiillance and regulatory authorities on MOH in food and food contact materials”) and jute bags. Depending on the source, the composition of the contaminating MOH mixtures varies.
The CONTAM Panel based its exposure assessment on a set of 1455 data on MOH in food, which were provided by four European countries. Most of the data were from 1997–2000 and from 2008–2010. Sufficient data were available only for the MOSH fraction. The MOAH fraction was specifically determined in few samples only, and it was estimated to be approximately in the range of 15–35% of the total MOH in the different food groups. The highest average background concentrations for MOSH were identified in confectionery (46 ppm), vegetable oils (41–45 ppm) and canned fish (40 ppm).
Bread and rice were cases for which the MOSH concentration values followed a bimodal distribution. This was explained by a low background exposure (mean: ≤4 ppm) and a high extra exposure with mean values of 532 ppm (bread) and 997 ppm (rice). These high values were likely caused by the use of food additives (E 905, E 905a; see ) as release agents in bakeries and as spraying agents in rice. These specific uses are not authorized in the EU today.
In dry food such as breakfast cereals, packaging material based on recycled paper can form a major source of MOSH and MOAH. This is due to the transfer of volatile compounds via the gas phase (evaporation and re-condensation). Data from a German survey with samples collected in 2010 (Vollmer et al. Citation2011) revealed that among the dry food items packaged in recycled paperboard without barrier the highest average MOSH concentrations were detected in cream pudding mix (32.4 ppm) and semolina (23.9 ppm).
Based on the data for MOSH in food and on food consumption data (from 32 different dietary surveys carried out in 22 European countries), average and high (95th percentile, P95) consumptions were estimated for different age groups of the general population. Background levels of MOSH in food resulted in chronic exposures between 0.03 (mean) and 0.1 mg/kg bw per day (P95) for adults and the elderly. Children (3–10 years) had higher exposures of up to 0.17 (mean) and 0.32 mg/kg bw per day (P95). Specific scenarios for the consumption of bread and rice with high MOSH levels resulted in a chronic exposure of up to 6.4 mg/kg bw per day for bread and 1.3 mg/kg bw per day for rice. In addition, MOSH from dry food packed in recycled paperboard without barrier forms a relevant contribution to total dietary exposure.
Information on the toxicity of MOSH derives mainly from rodent studies. A subchronic study in SD rats with gavage administration of de-aromatized hydrocarbon solvents (carbon number range: C10–C13) resulted in moderate liver cell hypertrophy (Adenuga et al. Citation2014) which, in the absence of any pathological alterations, was considered by the CONTAM Panel to be an adaptive response and not an adverse effect. Subchronic studies with dietary exposure of F-344 rats to highly refined mineral oils and waxes showed that MOSH in the carbon number range C16–C40 can accumulate in tissue and may cause microgranulomas in the MLNs and the liver of this rat strain, in particular in females (see Sections “MOSH levels in animal tissues” and “Effects associated with the accumulation of MOSH in animal tissues”). MOSH-related lipogranulomas had been observed in human liver, spleen and lymph nodes, without clear association with any adverse effect (see Section “Histopathological lesions associated with the deposition of MOSH in human tissues”).
The CONTAM Panel’s hazard characterization of MOSH identified inflammatory liver microgranulomas in F-344 rats as the critical adverse effect. Since none of the highly refined mineral oils and waxes tested in animal studies were regarded as representative of the human dietary exposure in terms of carbon number distribution, the CONTAM Panel selected NOAEL values for the different tested grades as reference points and applied a margin of exposure (MOE) approach. For the background exposure scenario, the lowest NOAEL of 19 mg/kg bw per day for the most potent grades (i.e. low and intermediate melting point waxes) (Smith et al. Citation1996) was selected. For the chronic high exposure scenarios reflecting the consumption of highly contaminated bread and rice, the CONTAM Panel selected the NOAEL of 45 mg/kg bw per day for a hydro-treated white oil (HTWO, similar to P70H) (Baldwin et al. Citation1992), because white oils, but not waxes, were used as release and spraying agents in these cases.
Risk characterization for MOSH under the background exposure scenario yielded MOEs of 100–680 for average consumers. For high consumers under the same scenario, the MOEs were lowest in toddlers and other children (MOE range: 59–110). For the chronic high exposure scenario involving the exposure to high MOSH levels in bread, the MOEs were 16–65 for average consumers and 7–32 for high consumers. For highly contaminated rice, the MOEs were 35–1900 for average consumers and 12–200 for high consumers. The MOEs obtained in the high exposure scenarios (especially for bread high in release agents) were in most/many cases below 100, in some cases below 10, which were considered to be of particular concern.
Concerning the carcinogenicity of MOAH, information was derived from animal studies with PAHs (including methylated derivatives), C10–C15 (predominantly C12) branched-chain alkyl benzene (i.e. dodecylbenzene), a partially hydrogenated aromatic hydrocarbon (tetralin), mineral oils, and other petroleum-derived substances. Several food-grade mineral oils and waxes, which very likely contained (but had not been tested for) MOAH, were not carcinogenic in chronic dietary studies (see Section “Carcinogenicity of PAHs and MOAH”). No data are available for the dietary exposure to non-food grade mineral oils. Dermal and inhalation studies were therefore used instead. The carcinogenicity of petroleum-derived materials appeared to be in general due to the presence of PAC containing three or more aromatic rings. The CONTAM Panel concluded that many MOAH with three or more aromatic rings and little or no alkylation can be bioactivated to genotoxic carcinogens. C10–C15 branched-chain alkyl benzene, which was taken as a representative of highly alkylated MOAH, has a skin irritation-related promotion effect (see Section “Carcinogenicity of PAHs and MOAH”). Some simple MOAH such as naphthalene (C10) are carcinogenic by a non-genotoxic mode of action, involving cytotoxicity and a proliferative response.
The data situation for MOAH did not permit a hazard characterization for carcinogenicity. Given that MOAH in food originate from different petroleum-derived substances and sources, and given the lack of information on the carcinogenicity of non-food grade mineral oils by the oral route, the CONTAM Panel considered the MOAH fraction as potentially genotoxic and carcinogenic. Given further that the MOAH fraction can be up to 30–35% of the MOH present in food, the CONTAM Panel considered the dietary exposure to MOAH of potential concern.
In summary, the CONTAM Panel considered the dietary exposure to MOSH (background exposure and exposures associated with the use of white oils as release agents in bread and for spraying of grains) and MOAH to be of potential concern.
The CONTAM Panel recommended that future monitoring should distinguish between MOSH and MOAH, and between subclasses of MOSH based on carbon numbers and chemical structures. Moreover, migration from recycled paperboard used for food packaging should be prevented by the inclusion of a functional barrier into the packaging assembly. Regarding toxicology, the relevance to humans of the liver microgranulomas seen in F-344 rats should be investigated further.
In order to fill data gaps in risk assessment, EFSA funded a project on bioaccumulation and toxicity of mineral oil hydrocarbons in female F-344 rats. The results of this project were published recently by Cravedi et al. (Citation2017) and others (Barp et al. Citation2017a, Citation2017b) (see Sections “Distribution, metabolism, and excretion of MOSH” and “MOSH in animal tissues”). This project focused, inter alia, on the accumulation of the different MOSH substructures and in particular on the impact of n-alkanes on granuloma formation. It included a study arm with subchronic exposure to a diet containing a MOSH mixture representative of the whole MOSH range to which humans are exposed via the diet. This is important to mention since none of the petroleum products tested in previous animal studies were regarded by the CONTAM Panel as representative of the human background exposure via food. To nevertheless enable risk characterization under the background exposure scenario, the CONTAM Panel in its 2012 opinion had chosen a pragmatic conservative approach by using the lowest NOAEL of those studies as reference point for the MOE calculation. The results of EFSA-funded project offer an opportunity for revisiting the reference point selection. An evaluation of the study results by the CONTAM Panel is pending.
Activities of surveillance and regulatory authorities on MOH in food and food contact materials
In 2009, the Official Food Control Authority of the Swiss Canton of Zürich reported on high contents of MOH in paperboard food packaging (BfR Citation2010a; Lorenzini et al. Citation2010). Their presence was attributed to MOH from offset printing inks and recycled fibers. It was found that hydrocarbons up to about C24 are sufficiently volatile for relevant migration into dry food. To minimize the transfer of MOH from recycled carton-board packaging into food, the German Federal Institute for Risk Assessment (BfR) in 2010 proposed the use of fresh fiber cartons and internal bags with barrier effect as well as a reduction of MOH entering the paper recycling process (BfR Citation2010c). From today’s view, the former management option has come into effect.
To improve the data situation for the evaluation of MOH mixtures, the German Federal Ministry of Food, Agriculture and Consumer Protection funded a project entitled “Degree of migration of undesirable substances from packaging materials made of recycled paper into food” (Vollmer et al. Citation2011). 119 samples of dry foods intended for long-term storage at ambient temperature were collected from the German market in 2010 (Vollmer et al. Citation2011; Harling et al. Citation2012). The samples were analyzed for MOH in the packaging materials (paperboard, internal bag) and the food at three time points in 2010 and 2011 (Harling et al. Citation2012). The maximum concentration in food was 100 mg/kg for MOSH and 16 mg/kg for MOAH. Concentrations in the packed dry foods increased with increasing storage duration.
The above-mentioned project had a special focus on the volatile MOH fraction from C16 to C24 since transfer via the gas phase is regarded as main route for the contamination of dry food by recycled paper and board. This range covers the lower part of the carbon-number range of low-viscosity mineral oils of the JECFA class III (). For this class of oils, JECFA had specified a temporary acceptable daily intake (ADI) of 0–0.01 mg/kg bw per day (JECFA Citation2002), which was later withdrawn (JECFA Citation2012). In the project this reference value was used to derive a specific migration limit (SML) of 0.6 mg/kg food for packaging materials (Vollmer et al. Citation2011). In 58% of the samples (excluding those packed in impermeable internal bags of polyethylene terephthalate (PET) or aluminium), the transfer of MOSH exceeded the SML at the respective best-before date or after 16 months of storage (Harling et al. Citation2012). The MOH transferred into food commonly contained 10–20% MOAH. In 58% of the samples the transfer of MOAH exceeded 1 mg/kg food. In products packaged in recycled cardboard without an internal bag acting as a functional barrier, the extent of migration after prolonged storage was 70–80% of the MOH originally contained in the packaging. Internal bags made of PET provided a good barrier function, whereas bags made of polyolefins turned out to be less effective for long-term storage.
On March 15, 2017, the German Federal Ministry of Food and Agriculture published a new draft version of the 22nd ordinance amending the Consumer Goods Ordinance (the “Mineral oil Ordinance”). Since for MOAH a carcinogenic potential cannot be excluded, the transfer into food should be reduced as far as possible by the introduction of functional barriers if transfer is not prevented by other conditions. According to the draft ordinance the functionality of the barrier is defined by a specific detection limit for the transfer of MOAH into food of 0.5 mg/kg food (BMEL Citation2017).
In 2017, the Commission Recommendation (EU) 2017/84 on the monitoring of mineral oil hydrocarbons in food and in materials and articles intended to come into contact with food was issued. The monitoring is aimed to a better understanding of the relative presence of MOSH and MOAH in food commodities. It should focus on the food categories which have been addressed in the EFSA Opinion (EFSA Citation2012) as main contributors to dietary exposure and should also cover food contact materials used for those products. It was originally planned to conduct the monitoring in 2017 and 2018, but this was hampered by the fact that the guidance developed by the EU Reference Laboratory for Food Contact Materials was not available until February 2019 (JRC Citation2019). It is to be expected that the results of the monitoring are used by the European Commission for a decision on regulatory measures on MOH under the food contaminants law.
Mineral oil hydrocarbons in cosmetic products
Highly refined mineral oils and waxes (e.g. Paraffinum liquidum and petrolatums) are found as ingredients in many products available to consumers including cosmetics (). Annex II of the Regulation (EC) No 1223/2009 on cosmetic products prohibits substances of other petroleum categories (Concawe Citation2001) including other lubricant base oils (OLBO), foots oils, slack waxes, petrolatums, treated distillate aromatic extracts, and other gas oils. The ban does not apply to e.g. petrolatums and slack waxes if the full refining history is known and if it can be shown that the substance from which it is produced is not a carcinogen. The ban does also not apply to e.g. OLBO if they contain less than 3% (w/w) DMSO extractable material. Although mineral oils and waxes as ingredients of cosmetics in the European market place are subject to regulation (Regulation [EC] No 1223/2009) requiring industry to demonstrate lack of carcinogenicity, recent findings of MOAH in products have caused general concern about potential risk from PAC that might remain entrained within refined mineral oils.
Mineral oils, waxes and petrolatum as currently used in cosmetic products are complex combinations of hydrocarbons obtained through chemical refinement including distillation, extraction, crystallization and hydrogenation from petroleum fractions (). The carbon chain length in these mixtures ranges from C18 to > C90 (Petry et al. Citation2017). Mineral oils serve diverse functions in cosmetics e.g. as vehicles, viscosity regulators and moisturizers, and are consequently found in various product groups (e.g. skin care products, sunscreen, lipsticks, Vaseline and baby oil). Depending on the product type, the mineral oil content in cosmetics may vary from low to nearly 100%. An example for the latter is petrolatum that was patented under the brand name Vaseline by Robert Chesebrough in 1872 and has been marketed ever since. Petrolatums are blended from high quality white oils and petroleum waxes (Health Canada Citation2016).
Compositional testing of dermally applied cosmetic products that contain mineral oil indicated MOAH concentrations of up to 4.5%. Vaseline appeared to contain 1.7–5.0% MOAH; although only four samples have been analyzed (BfR Citation2018; references therein). Elevated MOAH levels in cosmetics appear to be correlated with the presence of petrolatum in a formulation (BfR, unpublished data). As part of petrolatum, refined microcrystalline waxes are characterized by a seemingly high MOAH content. This is due to residual one or two aromatic and partially hydrogenated ring systems bearing long alkyl chains. These high molecular weight structures contribute significantly to the weight percentage of MOAH. According to industry, food-grade mineral microcrystalline waxes may contain up to 7.0% MOAH but nevertheless comply with medicinal quality standards in terms of PAC content (Jouanneau Citation2017; Sothmann and Südkamp Citation2017). Medicinal white oils (e.g. Paraffinum liquidum) typically retain less than 0.1% of MOAH (Jouanneau Citation2017; Sothmann and Südkamp Citation2017).
Testing of a subset of MOAH-containing samples of cosmetic products indicated compliance with international purity standards (Ph. Eur.). This finding is in line with a statement published recently by industry representatives to only use mineral oils and waxes of pharmaceutical grade in European cosmetic products (Petry et al. Citation2017). It should be noted that this negotiated agreement has not been officially endorsed yet by Cosmetics Europe (the European trade association for the cosmetics and personal care industry) and in any case is not legally binding.
There are multiple lines of evidence that the dermal absorption of highly refined mineral oil and wax is restricted to the stratum corneum (i.e. the outmost layer of the epidermis) indicating a negligible risk of bioavailability (see Section “Dermal absorption of MOSH” for details). Long-term dermal exposure studies with highly refined mineral oils and waxes failed to produce any local or systemic toxicity including tumor formation (Shubik et al. Citation1962; Nash et al. Citation1996; Concawe Citation2016).
Mineral oil-containing lip care may lead to an oral exposure of consumers. To ensure a high level of safety for this product group, Cosmetics Europe has issued recommendations with regard to the quality standard of the respective oils and waxes (Cosmetics Europe Citation2004, Citation2018). To comply with those recommendations, mineral oils must be of food-grade purity and fulfill the physico-chemical properties of medium and high viscosity oils and microcrystalline waxes for which ADI levels by EFSA (EFSA Citation2009, Citation2013b, Citation2013a) and JECFA (JECFA Citation2002, Citation2012) have been established. It should be stressed that in chronic feeding studies in female F-344 rats these highly refined mineral oils did not cause the formation of inflammatory lesions or granulomas in the liver or any other adverse effects.
Market analysis of mineral oil qualities in lipstick has shown that consumers may be exposed to non-compliant qualities. A more detailed analysis of MOSH revealed that a high percentage of the products contained more than 5% of hydrocarbons below C25 indicating that mineral oils of a lower molecular mass range have been used. Female F-344 rats exposed to low viscosity oils in their daily diet for 90 days exhibited inflammatory liver granulomas which are regarded as adverse by EFSA and JECFA (see Section “Effects associated with the accumulation of MOSH in animal tissues” for details). Mineral oil-induced histological changes can also occur in the human liver, noteworthily, with a different morphology designated as lipogranuloma. The induced effect has been considered clinically unimportant in humans because there is no evidence of a concurrent inflammatory response (for details see Section “Histopathological lesions associated with the deposition of MOSH in human tissues”). The amount of MOSH per day (up to 0.7 mg/kg bw per day) that a consumer accidentally ingests using a lipstick contributes roughly 10% to the ADI (BfR Citation2018).
In summary, mineral oil-containing cosmetic products do not pose a health risk to the consumer when the ingredients are of food and medicinal grade quality. Regarding MOAH occurring in cosmetic products it should also be kept in mind that currently this fraction cannot be specifically correlated either with biological activity nor adverse health effects due to a lack of appropriate assays.
Mineral oils in dermatology
From the dermatological point of view, the pharmacological effect of mineral oil-based products such as petrolatum (see Chapter “Mineral oils in medicinal products”) is of primary importance for the health assessment of mineral oils in cosmetic products. In the US, petrolatum is approved for infection prophylaxis after dermatosurgery and for the maintenance therapy of atopic dermatitis (AD). There is mounting evidence that PAHs play a critical role in mediating the beneficial effect (see below). PAHs occur not only in mineral oil-based products, they are highly enriched in coal tar also. Coal tar-based products are also used to treat AD and other chronic skin diseases such as psoriasis. The following summary of selected studies on petrolatum and coal tar considers the dermatological viewpoint.
Molecular responses induced by white petrolatum USP (United States Pharmacopeia) in the skin were studied in patients with moderate AD and healthy subjects by using different methods performed on control skin, petrolatum-occluded skin (48-h occlusion using a Finn chamber), and skin occluded by the Finn chamber only (Czarnowicki et al. Citation2016). Petrolatum-occluded skin showed an enhanced expression of antimicrobial peptides (AMPs), which are regulated by the interleukin IL-17/IL-22 signaling pathway. Control experiments showed that the induction of AMPs was not attributable to the occlusive effect itself. The application of petrolatum also induced the expression of key barrier differentiation markers (filaggrin and loricrin), increased stratum corneum thickness, and significantly reduced T-cell infiltrates in the setting of ‘‘normal-appearing’’ or non-lesional AD skin, which is known to harbor barrier and immune defects. Among the non-occluded controls, non-lesional AD skin compared with non-AD skin displayed a disturbed keratinization (parakeratosis) and a reduced expression of key barrier differentiation markers (filaggrin and loricrin). Petrolatum-occluded AD skin demonstrated a restoration of normal keratinization (orthokeratosis) and an enhanced expression of loricin und filaggrin. It is unclear how petrolatum induces the molecular and structural changes in the epidermis. A possible mechanism could involve the activation of the arylhydrocarbon receptor (AhR) in Th17 (T-helper 17) cells by PAHs, which activates the interleukin IL-17/IL-22 signaling pathway and thereby induces the AMPs in the epidermis.
The pharmacological effect is presumably linked to the presence of PAHs which are AhR ligands. Certain PAHs, which bind to or activate the AhR, are regarded as model carcinogens in coal tar, which has a dermato-pharmacologically similar effect to petrolatum. The role of the AhR in epidermal effects induced by coal tar treatment was studied in vitro in human keratinocytes (from AD patients and healthy volunteers) (van den Bogaard et al. Citation2013). Immunofluorescence microscopy showed a nuclear translocation of the AhR following treatment with 2% medicinal coal tar (pix lithanthracis). The expression of AhR-responsive genes encoding for proteins such as CYP1A1 and CYP1B1 was increased. The expression of genes encoding for the epidermal differentiation proteins filaggrin, hornerin, and involucrin, which are all pivotal for skin barrier function (and all are functionally related to AD), was also increased. AhR knockdown by siRNA completely abrogated this effect. These data support a regulatory role of the AhR signaling pathway in the coal tar-mediated induction of epidermal differentiation genes. This hypothesis was confirmed in a follow-up study with AhR-deficient and AhR agonist-treated keratinocytes (van den Bogaard et al. Citation2015). Histological and immunohistochemical investigations in human organotypic skin models revealed an accelerated epidermal differentiation following coal tar treatment (van den Bogaard et al. Citation2013). It was also shown that the AhR activated the Nrf2 antioxidative stress pathway in keratinocytes. Coal tar induced the nuclear translocation of Nrf2 and the subsequent induction of its target gene, the NAD(P)H quinone oxidoreductase 1 (NQO1). Upon AhR knockdown, induction of NQO1 expression was inhibited. AhR-mediated Nrf2 activation due to coal tar treatment could thus inhibit (Th2-induced) oxidative stress and inflammatory processes resulting in a favorable effect on the treatment of psoriasis and AD.
The dermal metabolism and effects of the model carcinogen B[a]P was studied in human hair follicles (Merk et al. Citation1987). Human hair was plucked from the scalp of healthy volunteers before and after shampooing their hair daily for 4 days with crude coal tar-containing shampoo. Hair follicles were shown to convert B[a]P to several metabolic products including 7,8-dihydroxy-B[a]P (B[a]P-7,8-diol), a major precursor of the ultimate carcinogenic metabolite of B[a]P. The repeated exposure of the scalp to coal tar-containing shampoo resulted in the metabolic activation of hair follicle enzymes, which manifested themselves in vitro in DNA-binding experiments by an enhanced B[a]P-DNA adduct formation.
A US retrospective study on the incidence rates of skin cancer was conducted in patients with psoriasis that had received an initial Goeckerman therapy (combination of crude coal tar and artificial ultraviolet radiation) and of which many continued to use coal tar regularly on an outpatient basis (Pittelkow et al. Citation1981). The 25-year follow-up analysis of the initial patient population revealed that 19 out of 260 patients reported the incident of non-melanoma skin cancer (NMSC), and one patient reported the development of a malignant melanoma. Expected incidence rates of NMSC for the study population were calculated from annual age-specific incidence rates for four US areas, which were available from the 3rd National Cancer Survey. In a study group of 260 patients the number of subjects expected to develop skin cancer was 49 for Dallas, 16 for Iowa, 19 for Minneapolis and 23 for San Francisco. This suggests that the incidence of skin cancer is not appreciably increased above the expected incidence.
The possible percutaneous absorption of mutagens or pro-mutagens (i.e. substances metabolically converted to mutagens in the body or by the UV radiation applied) from crude coal tar in subjects receiving a Goeckerman therapy was investigated in volunteers and psoriatic patients by measuring the mutagenicity of urine using the Ames test (Salmonella typhimurium strain TA98 plus S9 mix) (Wheeler et al. Citation1981). Two healthy volunteers and a psoriatic patient (all non-smokers) received a single application of 5% crude coal tar (USP) in petrolatum (30 g) to the trunk skin for 6 h. This was followed by ultraviolet light treatment. The mutagenic activity in urine samples was measured in a time window from two hours before to 30 h after the start of the experiment. As a negative control, the two healthy volunteers had been treated with petrolatum and ultraviolet light at an earlier time point, using the same experimental regimen. The mutagenic activity in the urine of coal-tar-treated subjects increased after the start of treatment, reached its maximum after 6–8 h, then declined and approached the individual background level after a total of 30 h. No mutagenicity was detected in the urine after petrolatum treatment. Given the small number of patients, however, only very limited conclusions can be drawn from this study.
Whether the dermatological exposure to coal tar ointments, which leads to a urinary excretion of (carcinogenic) PAH metabolites, is associated with an increased risk of bladder cancer was addressed in a population-based case-control study performed in the Netherlands (Roelofzen et al. Citation2015). About 1387 cases diagnosed with bladder cancer between 1995 and 2006 and 5182 population controls were included. Information on the use of coal tar and the history of skin disease was obtained through questionnaires. Logistic regression analyses were performed to estimate the risk of bladder cancer after coal tar treatment, adjusted for age, gender, smoking status, duration of smoking, and intensity of smoking. The use of coal tar ointments was approximately equal among cases and controls (3.8% vs. 3.0%, respectively). Dermatological application of coal tar was not significantly associated with bladder cancer (adjusted odds ratio: 1.37, 95% CI: 0.93–2.01). An inverse association between skin disease and bladder cancer was observed (adjusted odds ratio: 0.74, 95% CI: 0.61–0.90). The results of this study provide no evidence of bladder cancer due to dermatological coal tar treatment. Limitations of the study are the low number of patients and the relatively short duration of dermatological coal tar exposure in comparison to occupational exposures.
In the context of dermal mineral oil exposure, AhR hence is a janus-faced protein: On the one hand it induces enzymes which are involved in the formation of mutagenic/carcinogenic PAH metabolites, while on the other hand it mediates protective effects involved in the treatment of skin diseases. The composition of coal tar with respect to PAHs deviates from that of petrolatum and mineral oils in cosmetic products, which is due to the different source/origin and differences in the manufacturing process. In addition, medicinal products, as opposed to consumer products, are subject to a balancing of the therapeutic benefits and the risk of adverse effects.
Mineral oils in medicinal products
Mineral oils and waxes have been used in medicinal products for decades. The European Pharmacopeia (Ph. Eur.) 8.0 distinguishes between liquid, semi-liquid, and solid paraffins ().
Liquid paraffin (Paraffinum liquidum) and light liquid paraffin (P. perliquidum) are defined in the respective Ph. Eur. Monographs as a purified mixture of liquid saturated hydrocarbons obtained from petroleum. In the United States Pharmacopeia (USP) and the National Formulary (NF), the corresponding qualities are termed mineral oil (USP) and light mineral oil (NF).
Semi-liquid paraffin is a blend of solid and liquid qualities, comprising paraffin wax, microcrystalline wax, and a white oil (Barry and Grace Citation1971; van Heugten et al. Citation2017). Semi-liquid paraffin is also called petrolatum, Vaseline, or petroleum jelly. The Ph. Eur. categorizes semi-liquid paraffin further into white soft paraffin (Vaselinum album) and yellow soft paraffin (V. flavum). In contrast to liquid paraffin, which is based on medicinal white oil, semi-liquid paraffin can be blended from technical white oil. This explains why the attribute “saturated” is missing in the definition in the respective Monographs and why the limit values for impurities of PAHs are higher (see below). Yellow soft paraffin derives its color from base materials that are not as thoroughly purified as those used for white soft paraffin. In the USP, the corresponding qualities are termed white petrolatum and petrolatum.
The solid paraffins are differentiated in the Ph. Eur. into hard paraffin (P. solidum), also called paraffin wax, and microcrystalline wax, for which currently only a draft Monograph exists. Both products are defined as saturated hydrocarbons. Hard paraffin mainly contains straight-chain alkanes. Microcrystalline wax has a high content of branched and cyclic alkanes, which is important for the manufacturing of petrolatum, as it imparts a low bleeding tendency (i.e. the tendency for the oil component to separate from the wax component). In the USP and the NF, the corresponding qualities are termed paraffin and microcrystalline wax.
Paraffins are characterized in the Ph. Eur. by physical parameters, including viscosity, drop point, and melting point, depending on what is applicable to the respective product. The test for impurities includes a proof of absence of PAHs by measuring the UV absorbance (260–420 nm) of a DMSO extract of a paraffin sample dissolved in hexane, using a naphthalene solution as reference. The limit values (in naphthalene equivalents) are ≤0.5 ppm for liquid and light liquid paraffins, <70 ppm for hard paraffin, <300 ppm for white soft paraffin, and <450 ppm for yellow soft paraffin.
Mineral oils are used predominantly as excipients in topical pharmaceutical formulations such as ointment bases (emollients, solvents), emulsions (microencapsulation of many drugs), ophthalmic ointments, and otic preparations. They are rarely used as excipient in capsules and tablets (solvents and lubricants). In Germany, the use of liquid paraffin as an active substance in mild laxatives is currently under reevaluation by the German Federal Institute for Drugs and Medical Devices (BfArM).
The legal framework for medicinal products for human use in Europe is set by the Directive 2001/83/EC. Annex I contains the analytical, pharmacotoxicological and clinical standards and protocols with respect to the testing of medicinal products. The standard requirements for an authorization dossier include quality and nonclinical assessment. The nonclinical assessment follows the requirements described in Module 2 and Module 4 of Annex I. The toxicity testing is focused on active substance(s) but is often performed with final medicinal products (e.g. dermal and ophthalmic products). For excipients such as medicinal paraffins, no pharmacokinetic data are required for the authorization of medicinal products. Limited “indirect” toxicity data on excipients can potentially be derived from studies with the final formulation (active pharmaceutical ingredient and excipients), in particular from the comparison of the vehicle control with the untreated control group (e.g. irritant potential, skin sensitization). The quality assessment of substances follows the requirements specified in Module 3. For excipients such as medicinal paraffins, the Ph. Eur. Monographs are applicable. The applicant’s confirmation of compliance with the Monograph is mostly acceptable for excipients.
The Ph. Eur. provides substance identification criteria and impurity requirements, which are key elements for the quality and safety of the overall drug product. For example, the Monograph on liquid paraffin (P. liquidum) specifies an identification based on infrared absorption spectrophotometry (comparison with a Ph. Eur. reference spectrum of liquid paraffin), a viscosity measurement, and a phenolphthalein test. The test on impurities includes, inter alia, a proof of absence of PAHs by measuring the UV absorbance of a DMSO extract using a naphthalene solution as reference. An identification of individual aromatic hydrocarbons is not requested and no specific limit has been implemented.
Finished medicinal products containing light liquid paraffin for oral use include soft capsules, where the active ingredients are dissolved or suspended in the oil, and hard capsules, where the oil is used as plasticizer in film coatings. The mass of light liquid paraffin per dosage form is in the range of 0.35–73.59 mg for products on the German market. Medicinal products containing liquid paraffin for oral use include, for example, laxatives where the oil is the active substance. The amount of liquid paraffin per dosage for products can be up to 10 g for a laxative. It should be noted that such medical preparations are to be used only in the short term. Medicinal products containing white soft paraffin are widely applied for topical treatments including therapeutic treatments (see Chapter “Mineral oils in dermatology”). They are not approved for oral use. Yellow soft paraffin is rarely used for dermatological preparations. Finally, hard paraffins serve as embedding or encapsulating material for active substances in oral dosage forms with retarded release. The amount of hard paraffin per dosage form is in the range of 0.1–100 mg for products on the German market.
Mineral-oil based substances under REACH and CLP
Regulation (EC) 1907/2006 (REACH) provides the legal basis for the registration, evaluation, authorization, and restriction of chemicals in the EU. It is interconnected in multiple ways with Regulation (EC) 1272/2008 (CLP) on the classification, labeling, and packaging of hazardous chemical substances and mixtures, which translates the UN GHS, the so-called Globally Harmonized System which has been developed for these purposes at the UN level, into European Law.
Identification of mineral oil-based substances
Under the REACH and CLP regulations mineral oil-based substances (as a subgroup of substances derived from petroleum or coal streams, so-called “PetCo substances”) belong to the group of UVCBs, i.e. “substances of unknown or variable composition, complex reaction products, or biological materials”. More specifically, often both the “U” and the “V” apply to mineral oil-based substances, as it is impossible to specify the exact content of each constituent over the whole carbon number and substance class range covered by a given stream and because refineries will use crude oil from varying sources for production, resulting in a potentially significant variation of composition between batches.
Requirements for the identification and naming of UVCB substances under REACH and CLP are detailed in the corresponding guidance provided by the European Chemicals Agency (ECHA). While acknowledging that “[…] UVCB substances either cannot be uniquely specified with the IUPAC name of the constituents, as not all the constituents can be identified; or they may be generically specified but with a lack of specificity due to variability of the exact composition”, the ECHA guidance nevertheless requests registrants under REACH to give the chemical composition and the identity of the constituents as far as known, e.g. at least in a more generic way, on the basis of well-known reference samples or standards, indexes and existing codes, and/or analytical fingerprints. More specifically, the guidance requires that for a UVCB substance, “[…] all known constituents and all constituents present at concentrations ≥10% should be specified by at least an English-language IUPAC name and preferably a CAS number; the typical concentrations and concentrations ranges of the known constituents should be given as well. […] Unknown constituents should be identified as far as possible by a generic description of their chemical nature. Additives should be completely specified in a similar way to that described for well-defined substances”. Furthermore, and of great importance in the case of mineral oil-based substances, “[…] constituents that are relevant for the classification […] of the substance shall always be identified by the same identifiers, independently from their concentration”. However, the ECHA guidance does not specify the nature or extent of analytical investigations which registrants need to perform in order to ascertain or exclude the presence of constituents in amounts relevant for classification (ECHA Citation2017b).
In addition to the ECHA guidance, other sector-specific guidance exists, such as the OECD guidance documents for characterizing oleochemical substances or hydrocarbon solvents for assessment purposes, which are less strict regarding the specification of relevant individual constituents, but more specific with respect to naming conventions and analytical verification of composition (OECD Citation2014, Citation2016). With respect to the latter, it is of particular importance for hazard assessment that specified compositions not only cover all potentially relevant constituents (as far as known) but also the inter-batch variability in PetCo substances from the same refinery and process. To this end both OECD guidance documents endorse the concept of “five-batch analysis”: “The typical, minimum and maximum concentration value for a given constituent should be included in the substance specification. […]. This information can be established by analyzing a number of separate batches (typically five) over a period of time. The average value of this multiple batch analysis should be used to set the mean (typical value). The minimum and maximum values should be no more than 3 standard deviations from the mean/typical value” (OECD Citation2014).
As a basis for their approach to the environmental risk assessment of petroleum-based substances (PETRORISK approach), Concawe has introduced the so-called “hydrocarbon block method” (HCBM) (King et al. Citation1996; Comber et al. Citation2014). Briefly, a matrix of 3-carbon number ranges (e.g. C15–C17) vs. substance class (e.g. n-P = n-paraffins or DiAr = di-aromatics) is constructed. Composition is then specified by giving the content of each individual fraction characterized by a specific combination of 3-carbon number ranges and substance class (e.g. C12–C14 DiAr) as determined by GC × GC analysis. Rorije and coworkers (Rorije et al. Citation2012) from the Dutch National Institute for Public Health and the Environment (RIVM) in reply to a service request by ECHA have identified shortcomings of the HCBM as practically implemented (and even more so, of the PETRORISK approach itself, for which they saw “[…] a serious possibility that the tool PETRORISK […] will lead to an underestimation of the (environmental) risk related to the production and use of petroleum products”) and recommended improvements. However, while certain practical aspects may need further discussion, at least the theoretical concept of the HCBM matrix as such can be a helpful starting point for characterizing the composition of mineral oil-based UVCB substances in a transparent and comparable way, as a pre-requisite for risk assessment based on the presence/absence of certain hydrocarbon fractions or constituents (cf. Section “SVHC Roadmap 2020 and PetCo group”).
Classification for hazardous properties
While both the CLP (through hazard classes and categories) and REACH (through chemical safety assessment) regulations essentially address all relevant toxicological endpoints in the area of human health, they place particular focus on carcinogenic, mutagenic, and reprotoxic (CMR) properties. According to Article 36 (1) of the CLP Regulation, substances with these properties require “harmonized classification” (CLH), i.e. these classifications are officially identified by the European Commission based on an evaluation by the Risk Assessment Committee (RAC) of the European Chemicals Agency (ECHA). By subsequent publication in Annex VI of the CLP regulation such classifications then become legally binding in the EU.
Classification not only applies to the main constituent(s) of well-defined substances. CLP Art. 11 states that “[…] where a substance contains another substance, itself classified as hazardous, whether in the form of an identified impurity, additive or individual constituent, this shall be taken into account for the purposes of classification, if the concentration of the identified impurity, additive or individual constituent is equal to, or greater than, the applicable cutoff value […]”. For CMR properties these cutoffs are given in the form of endpoint-specific “Generic Concentration Limits” (GCLs, e.g. 0.1% (w/w) in the case of carcinogens of CLP category 1) or as “Specific Concentration Limits” based on individual substance data (SCLs, e.g. 0.01% (w/w) for the carcinogenic PAH benzo[a]pyrene).
In line with the CLP guidance issued by ECHA, CLH of UVCB mineral oil-based substances for CMR properties should be done preferably based on the presence of one or more relevant constituents/additives/impurities (e.g. B[a]P) above the applicable concentration limits whereas for other human health endpoints, CLH is in general obtained based on data for the substance as a whole. It is self-evident that for constituent-based classification an adequate and comprehensive specification of composition is a key pre-requisite.
The official identification of CMR properties by CLH in itself already constitutes a regulatory measure which may have severe consequences in sector-specific downstream legislation. It also constitutes a fundamental pre-requisite for any other regulatory risk management measures under REACH, such as identification of “Substances of Very High Concern” (SVHC), authorization, or restriction.
Registration and evaluation under REACH
Under REACH, substances must be registered at a market volume per registrant of 1 ton or more per year. Depending on the total tonnage level registrants must fulfill a set of standard information requirements (mostly in vitro or in vivo tests in animals, cf. REACH Annexes VII-X) which can be adapted under certain circumstances (REACH Annex XI). In addition, if certain conditions are fulfilled, they must submit a so-called “Chemical Safety Assessment (CSA)” in order to demonstrate that potential risks due to hazardous properties of the registered substance are adequately controlled. For the registration of most, if not all petroleum-derived substances, the REACH standard information requirements have been adapted in favor of category/grouping approaches, i.e. individually registered substances are allocated to groups/categories (e.g. based on comparable production processes) and their risk assessment is based on a matrix of physico-chemical and (eco)toxicological properties, and biological fate data which are read across between group members in order to fill data gaps. While this approach is sensible as such, in particular from an animal welfare and resource perspective, it is clear that it can only produce reliable results if sufficient mutual similarity in composition between all materials registered under each individual registration, between category members, and between the substances used for testing can be established with confidence.
Under Title VI, REACH also foresees processes by means of which registrants, ECHA or EU Member State Competent Authorities (MSCAs) can scrutinize registration dossiers for potential data gaps and propose (registrants) or request (authorities) the generation of further data. Again, a sufficient specification of the composition is key, in particular to establish the representativeness of test materials used in the studies submitted for the registered composition.
Restriction
MSCAs or ECHA (on request by the European Commission) can initiate the restriction of one or more uses of a substance under REACH Title VIII, if they are able to demonstrate that this use/these uses pose an unacceptable risk to human health. This process normally involves scrutiny by RAC and SEAC (ECHA’s Committee for socio-economic analysis) and – aside from the burden of proof – places a heavy workload on the dossier submitter. In the case of a substance with CMR properties which may be used by consumers (on its own, in mixtures, or articles), a simplified route according to REACH Art. 68 (2) is available, which e.g. has been used for the restriction of PAHs in consumer products proposed by the German MSCA in 2010 (for details of the risk assessment for consumers performed by BfR in this context, cf. BfR (Citation2010b)). While in general restriction is a powerful and versatile regulatory tool, in particular for the regulation of substances in articles, it is often difficult to prove unacceptable risk, most of all for consumers, where reliable data on exposure (including migration of hazardous substances from articles) are often not available. An advantage is given by the fact that for petroleum-derived substances, restriction can focus on relevant hazardous constituents, since the origin of e.g. carcinogenic polycyclic aromatic compounds (PAC, i.e. including also those polycyclic aromatic substances containing heteroatoms) in articles cannot be traced back to the use of a specific petroleum-based substance in the production process.
SVHC identification and authorization
In 2006, as a follow-up to the 2002 Johannesburg sustainability summit, the policy goal “to achieve, by 2020, that chemicals are used and produced in ways that lead to the minimization of significant adverse effects on human health and the environment, using transparent science-based risk assessment procedures and science-based risk management procedures, taking into account the precautionary approach” was expressed at UN level in the form of the Strategic Approach to International Chemicals Management (SAICM, cf. www.saicm.org, UN (Citation2003)). This has been translated under REACH into the concept of so-called “Substances of Very High Concern” (SVHCs). SVHCs are officially identified and regulated under REACH Title VII (authorization) with the aim “[…] to ensure the good functioning of the internal market while assuring that the risks from substances of very high concern are properly controlled and that these substances are progressively replaced by suitable alternative substances or technologies where these are economically and technically viable” (REACH Art. 55).
Inter alia, CMR properties of CLP category 1 can trigger official identification of a substance as SVHC (by the European Commission based on a vote of the MSC, ECHA’s Member State Committee), in which case it is put first on a so-called Candidate List (CL) for authorization. In a second step, based on certain prioritization criteria as well as on available capacity of the authorities, it is transferred to REACH Annex XIV, the list of substances requiring authorization. CL entries refer to single substances or to closed lists of substances. As a consequence, one of the problems encountered in practice is presented by the fact that petroleum-based UVCB substances can either be put on the CL one by one (which is not efficient, given the large number of these substances) or as groups defined in such a way that a definitive (closed) list of affected substances can be identified (with the chance of missing relevant similar substances). It is not possible to include open (insufficiently determined) lists of substances, e.g. “all substances containing >0.01% benzo[a]pyrene” (substance-in-substance approach), both because there could be insufficient certainty for registrants about whether their substance is affected and because the total number of affected substances would not be known. As a result of the latter, the authorities’ capacities for e.g. further processing later applications for authorization (AfAs) could be overtaxed, blocking other important regulatory activities in the field of chemical safety. A further disadvantage of the authorization procedure is the fact that it can regulate the use of substances in the production of articles, but not the articles themselves, hence the import of articles manufactured outside the EU cannot be regulated via an Annex XIV entry directly. However, after the so-called “sunset date”, i.e. the date from which a substance in Annex XIV may no longer be placed on the market unless authorized, REACH Art. 69(2) obliges ECHA to consider “whether the use of that substance in articles poses an unacceptable risk to human health or the environment that is not adequately controlled”. In cases where such a risk is established, ECHA then must prepare a restriction dossier (cf. previous section).
SVHC roadmap 2020 and PetCo group
To address the SAICM 2020 goal, the European Commission in 2012 devised the so-called “SVHC roadmap 2020” with the aim to have all “relevant currently known SVHCs” included in the Candidate List by 2020 (European Commission Citation2013). This goal not only included the aim to determine the relevance of SVHCs that are known today, but also to identify new potential SVHCs that will come out of REACH registration and evaluation. Since 2013, comprehensive screening activities by ECHA and the MSCAs have taken place and several working groups on EU level have been working on the implementation of this roadmap. The progress of this work is tracked on the website of the European Chemicals Agency (https://echa.europa.eu/de/svhc-roadmap-to-2020-implementation, last accessed 2019-06-20).
Depending on the source material and the production process, petroleum- and coal-derived (UVCB) substances may contain constituents with SVHC properties. Since they form a large group of substances with a number of specific problems, the roadmap activities related to regulatory risk management of petroleum and coal stream (PetCo) substances are coordinated since 2015 by the so-called PetCo Working Group (WG), which consists of representatives of ECHA, the European Commission, EU Member States, and industry stakeholders; for more details on the composition and remit of this group, cf. https://echa.europa.eu/de/petco-working-group (last accessed 2019-06-20). In August 2017, the PetCo WG has published a general approach for how to further handle the roadmap work on these substances (ECHA Citation2017a). Briefly, this approach foresees a first prioritization for further work of substances with consumer or professional uses (widespread uses, highest priority) over those with uses only at industrial sites (medium priority), or used only as fuel or intermediates (lowest priority). Next the prioritized substances will be further categorized as substances of known hazard (regulatory action possible, if needed), of no known hazard (no regulatory action needed for the time being), and of potential hazard (further investigation of hazardous properties needed).
On-going discussions in the PetCo WG inter alia circle around the question which type of composition information is needed to reliably identify constituents relevant for classification and labeling. For human health hazard assessment, the HCBM approach might serve as a basis, if solutions for certain problems (e.g. insufficient granularity of the blocks, partial ambiguity of the blocks, lack of a standardized GC × GC method) can be found. As part of the PetCo work, ECHA has compiled a library of potential PetCo constituents. Constituents characterized as potentially hazardous based on experimental data or using in silico modeling could then be mapped to specific hydrocarbon blocks. In the future, this might provide registrants with a means of easily identifying which “constituents relevant for classification and labeling” they should address (e.g. by further targeted chemical analysis and/or by risk assessment) based on a reliable GC × GC analysis of their registered material. For the latter a 5-batch-analysis of representative samples could increase the reliability of the assessment.
Practical experience from screening projects has shown that many PetCo registration dossiers suffer from insufficient substance identification and, as a result, insufficient justification of the substance categories used for risk assessment. On top of compliance issues observed also in REACH registrations of mono- or multi-constituent substances (UBA Citation2015), insufficient or absent specification of composition, insufficient justification of category formation, huge variation of compositional parameters – where specified – that are relevant for classification and labeling (e.g. under the same registration ID, substances with DMSO extractables ranging from <1 to >8% are found), absence of key data for CMR properties with missing/insufficient justification, and unclear identity of test material used in toxicological studies, are all deficiencies frequently encountered in PetCo registration dossiers.
The need to improve the justification of the categories formed so far for the PetCo substances has already been acknowledged in general by industry. To that end, Concawe inter alia has initiated the so-called Cat-App program. According to Concawe, “the concept is to develop a framework based on chemical-biological read-across, a novel direction in regulatory decision making. The approach is to integrate innovations in (i) in vitro testing, (ii) high-throughput genomics, and (iii) integrative data analyses and visualization into a workflow for read-across assessment of UVCBs in regulatory programs“. First results were published on the Concawe website in September 2018 (https://www.concawe.eu/publication/cat-app-project-2018-results-brochure, last accessed 2019-06-20) and in the peer-reviewed literature (Kamelia et al. Citation2017; Kamelia et al. Citation2018; Kamelia et al. Citation2019a; Kamelia et al. Citation2019b). The project is interesting from a scientific perspective while the regulatory acceptability of its results will need further discussion. Anyway, it is noted that – provided the project will be successful – the subsequent revision of PetCo categories as a result cannot be expected to be completed by the end of this decade, i.e. in time for the 2020 goal.
Conclusions
The objective of this review was to evaluate the potential health risks arising from oral and dermal exposures of consumers to mineral oil in food and non-food products such as cosmetics. Mineral oils are complex substances. Analytically, two fractions can be distinguished: mineral oil saturated hydrocarbons (MOSH) and mineral oil aromatic hydrocarbons (MOAH). The carcinogenic potential of individual constituents of the MOAH fraction and the bioaccumulation potential of MOSH represent two of the main toxicological concerns.
Autopsy and biopsy studies showed the presence of MOSH in human tissue. Highest MOSH levels occurred in the mesenteric lymph nodes (MLNs), which suggests an exposure route that is primarily oral. Intestinally absorbed MOSH follow the route of transport of lipids and are primarily taken up by the liver. Biotransformation of MOSH in liver is slow. Human (and animal) data indicate a lifelong accumulation in adipose tissue and MLNs but not in liver and spleen. Although contaminated food is the main culprit, mineral oil-containing cosmetics are also contributing to the body burden of ingested MOSH.
MOSH in human liver are complex mixtures consisting predominantly of highly branched and cyclic compounds. Autopsy studies from the mid-1960s showed livers to contain oil droplets, so-called lipogranuloma; their occurrence and extent correlated with the MOSH content in human liver tissue. In F-344 rats, dietary exposure to certain highly refined oils and waxes caused inflammatory epithelioid cell granulomas in liver. These adverse lesions are morphologically distinct from the MOSH-induced non-inflammatory lipogranuloma in human livers. The incidence and severity of rat liver granulomas correlated with the tissue MOSH content. In histologically positive rat livers, n-alkanes made up a prominent part of the MOSH fraction. The MOSH content in rat liver correlated with the viscosity and n-alkane content of the administered substances. Paraffin waxes (n-alkane-rich) induced the strongest response, followed by low-viscosity mineral oils. To assume that the causative agents for granuloma formation in F-344 rat liver by low-viscosity mineral oils are the n-alkanes is conceivable, but this remains speculative without further supportive data.
Since the mid-1960s, exposure to contaminated food has continuously decreased and, consequently, so has the MOSH level in human liver. Virtually no granulomas were found in autopsy patients in a more recent study. The relevance of hepatic lesions in F-344 rats for humans is still unclear, but doubts regarding the suitability of this rat model are warranted. Present-day human data, ideally derived from subjects with a higher exposure to contamined food due to eating habits, are needed to clarify this issue. Since MOSH have the intrinsic property to induce tissue lesions, to minimize their contamination level in food is mandatory. For the intended use of highly refined mineral oils and waxes in lip care products, the recommendations of Cosmetics Europe should be followed.
Recent analytical findings of MOAH in cosmetic products have caused concern about potential dermal cancer risk arising from MOAH constituents. For highly refined mineral oils and waxes, which are used inter alia as cosmetic ingredients, animal studies support the safe use of these substances. Additionally, the safety of these cosmetic products is documented by their prevalent and long-term use, with a simultaneous lack of clinical and epidemiological evidence of adverse health effects. Regarding consumer exposure to less refined and characterized mineral oil, however, data on safety is incomplete. This applies, for example, to contamination of food by unrefined mineral oils. Given that MOAH in food originate from different sources, and given the lack of information on the carcinogenic potential of non-food grade mineral oils, the MOAH impurities in food should be minimized.
Declaration of interest
All authors are or have been employed by the German Federal Institute for Risk Assessment (BfR). Two coauthors (Bärbel Vieth and Karla Pfaff) have retired recently, but were working for the BfR for the entire drafting period. The manuscript resulted from the authors' risk assessment activities at the BfR as part of their official duties. The authors affirm that there are no conflicts of interest associated with this academic publication.
Abbreviations | ||
AD | = | Atopic dermatitis |
ADI | = | Acceptable daily intake |
ADME | = | Absorption, Distribution, Metabolism, and Excretion |
AfA | = | Application for Authorization |
AhR | = | Arylhydrocarbon Receptor |
ALT | = | Alanine aminotransferase |
AMP | = | Antimicrobial peptide |
ANS | = | EFSA Panel on Food Additives and Nutrient Sources added to Food |
AST | = | Aspartate aminotransferase |
B[a]P | = | Benzo[a]pyrene |
BfR | = | German Federal Institute for Risk Assessment |
CL | = | Candidate List (under REACH) |
CLH | = | harmonized classification and labeling |
CLP | = | European regulation on Classification, Labeling and Packaging of substances and mixtures |
CMR | = | Carcinogenic, Mutagenic, toxic for Reproduction |
Concawe | = | A division of the European Petroleum Refiners Association |
CONTAM | = | EFSA Panel on Contaminants in the Food Chain |
Cosmetics Europe | = | European trade association for the cosmetics and personal care industry |
DMSO | = | Dimethyl sulfoxide |
ECHA | = | European CHemicals Agency |
EFSA | = | European Food Safety Authority |
EPA | = | U.S. Environmental Protection Agency |
FAO | = | Food and Agriculture Organization of the United Nations |
FCM | = | food contact material |
FID | = | Flame ionization detection |
GC | = | Gas chromatography |
GC–FID | = | Gas chromatography coupled to flame ionization detection |
GC × GC | = | Comprehensive (two-dimensional) gas chromatography |
GC × GC−MS | = | Comprehensive gas chromatography coupled to mass spectrometry |
GC × GC–ToF–MS | = | Comprehensive gas chromatography coupled to time-of-flight mass spectrometry |
GC–MS | = | Gas chromatography coupled to mass spectrometry |
GHS | = | Globally harmonized system of classification and labeling of chemicals |
GGT | = | Gamma glutamyl transferase |
HCBM | = | hydrocarbon block method |
HDL | = | High density lipoprotein |
HMPW | = | High melting point wax |
NMR | = | Nuclear magnetic resonance (spectroscopy) |
HRBO | = | Highly refined base oils |
HRF | = | Human relevance framework |
HTWO | = | Hydro-treated white oil |
HVMO | = | High viscosity (white) mineral oils |
IARC | = | International Agency for Research on Cancer |
IL | = | Interleukin |
IMPW | = | Intermediate melting point wax |
INCI | = | International Nomenclature of Cosmetic Ingredients |
I/P | = | Initiation/Promotion |
JECFA | = | Joint FAO/WHO Expert Committee on Food Additives |
L-E | = | Long-Evans (rat strain) |
LC | = | Liquid chromatography |
LC–GC–FID | = | Liquid chromatography coupled to gas chromatography and flame ionization detection |
LDL | = | Low density lipoprotein |
LOAEC | = | Lowest observed adverse effect concentration |
LMPW | = | Low melting point wax |
MAK | = | German Senate Commission for the investigation of health hazards of chemical compounds in the work area |
MLN | = | Mesenteric lymph node |
MMAD | = | Mass median aerodynamic diameter |
MoA | = | Mode of action |
MOAH | = | Mineral oil aromatic hydrocarbons |
MOE | = | Margin of exposure |
MOH | = | Mineral oil hydrocarbons |
MOSH | = | Mineral oil saturated hydrocarbons |
MS | = | Mass spectrometry |
MSCA | = | Member States Competent Authority |
MVMO | = | Medium viscosity (white) mineral oils |
NPV | = | Negative predictive value |
NF | = | National formulary |
NMSC | = | Non-melanoma skin cancer |
NOAEC | = | No observed adverse effect concentration |
NQO1 | = | NAD(P)H quinone oxidoreductase |
Nrf2 | = | Nuclear factor erythroid 2-related factor 2 |
OECD | = | Organization for Economic Co-operation and Development |
OLBO | = | Other lubricant base oils |
OTWO | = | oleum-treated white oil |
PAC | = | Polycyclic aromatic compounds |
o/w | = | oil-in-water (emulsion) |
PAH | = | Polycyclic aromatic hydrocarbon |
PAO | = | Poly-α-olefin |
PET | = | polyethylene terephthalate |
PetCo | = | Attribute related to petroleum or coal streams |
Ph. Eur. | = | European Pharmacopeia |
POSH | = | Polyolefin oligomeric saturated hydrocarbons |
RAC | = | Risk Assessment Committee (at ECHA) |
REACH | = | Registration, Evaluation, Authorization, and restriction of CHemicals |
SAICM | = | Strategic approach to international chemicals management |
SD | = | Sprague Dawley (rat strain) |
SCOEL | = | Scientific Committee on Occupational Exposure Limits |
SEAC | = | Committee for Socio-Economic Analysis (at ECHA) |
SML | = | Specific migration limit |
SVHC | = | Substance of very high concern |
Th | = | T-helper (cells) |
USP | = | United States Pharmacopeia |
UVCB | = | Attribute related to substances of unknown or variable composition, complex reaction products or biological materials |
WHO | = | World Health Organization |
w/o | = | water-in-oil (emulsion) |
Acknowledgement
This review emanated from two expert meetings and a consumer protection forum held in Berlin at the BfR in between 2015 and 2017. The meetings brought together experts from German federal and state authorities, from European authorities as well as from academia and from industry. The authors would like to thank the following experts/speakers for their valuable contributions/presentations: Rüdiger Bartsch, Jan van Benthem, Maurus Biedermann, Marco Binaglia, Juan Carlos Carillo, Eddo Hoekstra, Ute Galle-Hoffmann, Jürgen Lademann, Hans Merk, Jochen Norwig, Kristof Seubert, Michal Skowron, Dierk Sothmann, Klaus Südkamp, Chrystele Tissier. The authors would like to thank Koni Grob and Nicole Concin for providing the raw data on MOSH levels in human tissues.
References
- Abdo KM, Johnson JD, Nyska A. 2003. Toxicity and carcinogenicity of Elmiron in F344/N rats and B6C3F1 mice following 2 years of gavage administration. Arch Toxicol. 77(12):702–711.
- Adenuga D, Carrillo JC, McKee RH. 2014. The sub-chronic oral toxicity of dearomatized hydrocarbon solvents in Sprague–Dawley rats. Regul Toxicol Pharmacol. 70(3):659–672.
- Adenuga D, Goyak K, Lewis RJ. 2017. Evaluating the MoA/human relevance framework for F-344 rat liver epithelioid granulomas with mineral oil hydrocarbons. Crit Rev Toxicol. 47(9):750–766.
- Agarwal R, Kumar S, Mehrotra NK. 1986. Polyaromatic hydrocarbon profile of a mineral oil (JBO-P) by gas chromatography. J Chromatogr Sci. 24(7):289–292.
- Agarwal R, Kumar S, Shukla Y, Antony M, Mehrotra NK. 1985. Quantification of tumour initiating effect of jute batching oil and its distillates over mouse skin. Cancer Lett. 28(3):281–290.
- Agarwal R, Shukla Y, Kumar S, Mehrotra NK. 1988. Evaluation of carcinogenic effect of jute batching oil (JBO-P) fractions following topical application to mouse skin. Arch Toxicol. 62(6):406–410.
- Albro PW, Fishbein L. 1970a. Absorption of aliphatic hydrocarbons by rats. Biochim Biophys Acta. 219(2):437–446.
- Albro PW, Fishbein L. 1970b. Short-term effects of piperonyl butoxide on the deposition of dietary hydrocarbon in rat tissues. Life Sci II. 9(13):729–739.
- Albro PW, Thomas RO. 1974. Metabolism of phytane in rats. Biochim Biophys Acta. 372(1):1–14.
- Amin S, Desai D, Hecht SS. 1992. Comparative tumorigenicity of dimethylchrysenes in mouse skin. Chem Res Toxicol. 5(2):237–241.
- Amin S, Desai D, Hecht SS. 1993. Tumor-initiating activity on mouse skin of bay region diol-epoxides of 5,6-dimethylchrysene and benzo[c]phenanthrene. Carcinogenesis. 14(10):2033–2037.
- Amoruso MA, Forgash RC, Gilmour SK, Freeman JJ. 2003. Determination of the skin cancer potential of benzo(a)pyrene (BaP) and a petroleum refinery stream in K6/ODC mice. Toxicol Sci. 72:86–86.
- Assogne CB. 1990. Studies of the carcinogenesis and tumorigenesis of skin applications of dodecylbenzene on hairless mice. Br J Ind Med. 47(5):356–358.
- Baldwin MK, Berry PH, Esdaile DJ, Linnett SL, Martin JG, Peristianis GC, Priston RA, Simpson BJ, Smith JD. 1992. Feeding studies in rats with mineral hydrocarbon food grade white oils. Toxicol Pathol. 20(3-1):426–435.
- Bakker GCM. 2011. Absorption and kinetics of P15H oil after single oral administration of P15H oil in human volunteers. TNO Quality of Life Report V9501.
- Bandla HP, Davis SH, Hopkins NE. 1999. Lipoid pneumonia: a silent complication of mineral oil aspiration. Pediatrics. 103(2):E19.
- Barp L, Biedermann M, Grob K, Blas YEF, Nygaard UC, Alexander J, Cravedi JP. 2017a. Accumulation of mineral oil saturated hydrocarbons (MOSH) in female Fischer 344 rats: comparison with human data and consequences for risk assessment. Sci Total Environ. 575:1263–1278.
- Barp L, Biedermann M, Grob K, Blas YEF, Nygaard UC, Alexander J, Cravedi JP. 2017b. Mineral oil saturated hydrocarbons (MOSH) in female Fischer 344 rats; accumulation of wax components; implications for risk assessment. Sci Total Environ. 583:319–333.
- Barp L, Kornauth C, Wuerger T, Rudas M, Biedermann M, Reiner A, Concin N, Grob K. 2014. Mineral oil in human tissues, Part I: concentrations and molecular mass distributions. Food Chem Toxicol. 72:312–321.
- Barrowman JA, Rahman A, Lindstrom MB, Borgstrom B. 1989. Intestinal absorption and metabolism of hydrocarbons. Prog Lipid Res. 28(3):189–203.
- Barry BW, Grace AJ. 1971. Structural, rheological and textural properties of soft paraffins. J Text Stud. 2(3):259–279.
- Bartsch N, Heidler J, Vieth B, Hutzler C, Luch A. 2016. Skin permeation of polycyclic aromatic hydrocarbons: a solvent-based in vitro approach to assess dermal exposures against benzo[a]pyrene and dibenzopyrenes. J Occup Environ Hyg. 13(12):969–979.
- Baxter JH, Steinberg D, Mize CE, Avigan J. 1967. Absorption and metabolism of uniformly 14C-labeled phytol and phytanic acid by the intestine of the rat studied with thoracic duct cannulation. Biochim Biophys Acta. 137(2):277–290.
- Bellamy C, Burt AD. 2018. The liver in systemic disease. In: Burk AD, Ferrel LD, Hubscher SG, editors. Macsween's pathology of the liver (Seventh Edition). Philadelphia, PA: Elsevier; p. 966–1018.
- Beriro DJ, Cave MR, Wragg J, Thomas R, Wills G, Evans F. 2016. A review of the current state of the art of physiologically-based tests for measuring human dermal in vitro bioavailability of polycyclic aromatic hydrocarbons (PAH) in soil. J Hazard Mater. 305:240–259.
- BfR. 2010a. 4. Sitzung der BfR-Kommission für Bedarfsgegenstände. Protokoll vom 17. November 2009 [Minutes of the 4th meeting of the BfR Committee for Consumer Products. 17 November 2009]. German. [accessed 2019 Dec 1]. https://www.bfr.bund.de/cm/343/4_sitzung_der_bfr_kommission_fuer_bedarfsgegenstaende.pdf
- BfR. 2010b. Carcinogenic polycyclic aromatic hydrocarbons (PAHs) in consumer products to be regulated by the EU – risk assessment by BfR in the context of a restriction proposal under REACH. BfR Opinion Nr. 032/2010, 26 July 2010. [accessed 2019 Dec 1]. https://mobil.bfr.bund.de/cm/349/carcinogenic_polycyclic_aromatic_hydrocarbons_pahs_in_consumer_products_to_be_regulated_by_the_eu.pdf
- BfR. 2010c. Übergänge von Mineralöl aus Verpackungsmaterialien auf Lebensmittel [Migration of mineral oil from packaging materials to foodstuffs. BfR Opinion No. 008/2010, 09 December 2009]. German. [accessed 2019 Dec 1]. https://mobil.bfr.bund.de/cm/343/uebergaenge_von_mineraloel_aus_verpackungsmaterialien_auf_lebensmittel.pdf
- BfR 2018. Hochraffinierte Mineralöle in Kosmetika: Gesundheitliche Risiken sind nach derzeitigem Kenntnisstand nicht zu erwarten [Highly refined mineral oils in cosmetics: Health risks are not to be expected according to current knowledge. Updated BfR Opinion No. 008/2018 of 27 February 2018]. German. [Accessed 2019 Dec 1]. https://www.bfr.bund.de/cm/349/highly-refined-mineral-oils-in-cosmetics-health-risks-are-not-to-be-expected-according-to-current-knowledge.pdf
- Biedermann M, Barp L, Kornauth C, Wurger T, Rudas M, Reiner A, Concin N, Grob K. 2015. Mineral oil in human tissues, part II: characterization of the accumulated hydrocarbons by comprehensive two-dimensional gas chromatography. Sci Total Environ. 506-507:644–655.
- Biedermann M, Fiselier K, Grob K. 2009. Aromatic hydrocarbons of mineral oil origin in foods: method for determining the total concentration and first results. J Agric Food Chem. 57(19):8711–8721.
- Biedermann M, Grob K. 2010. Is recycled newspaper suitable for food contact materials? Technical grade mineral oils from printing inks. Eur Food Res Technol. 230(5):785–796.
- Biedermann M, Grob K. 2012a. On-line coupled high performance liquid chromatography-gas chromatography for the analysis of contamination by mineral oil. Part 1: method of analysis. J Chromatogr A. 1255:56–75.
- Biedermann M, Grob K. 2012b. On-line coupled high performance liquid chromatography-gas chromatography for the analysis of contamination by mineral oil. Part 2: migration from paperboard into dry foods: interpretation of chromatograms. J Chromatogr A. 1255:76–99.
- Biedermann M, Grob K. 2015. Comprehensive two-dimensional gas chromatography for characterizing mineral oils in foods and distinguishing them from synthetic hydrocarbons. J Chromatogr A. 1375:146–153.
- Biedermann M, Munoz C, Grob K. 2017. Update of on-line coupled liquid chromatography - gas chromatography for the analysis of mineral oil hydrocarbons in foods and cosmetics. J Chromatogr A. 1521:140–149.
- Bingham E, Barkley W. 1979. Bioassay of complex mixtures derived from fossil fuels. Environ Health Perspect. 30:157–163.
- Bingham E. 1988. Carcinogenicity of mineral oils. Ann NY Acad Sci. 534:452–458.
- Bird MG, Lewis SC, Freeman JJ, et al. 1990. Subchronic feeding study of white mineral oils in rats and dogs. Toxicologist. 10:143.
- Blackburn GR, Deitch RA, Schreiner CA, Mackerer CR. 1986. Predicting carcinogenicity of petroleum distillation fractions using a modified Salmonella mutagenicity assay. Cell Biol Toxicol. 2(1):63–84.
- Blackburn GR, Deitch RA, Schreiner CA, Mehlman MA, Mackerer CR. 1984. Estimation of the dermal carcinogenic activity of petroleum fractions using a modified Ames assay. Cell Biol Toxicol. 1(1):67–80.
- Blackburn GR, Roy TA, Bleicher WT, Reddy MV, Mackerer CR. 1996. Comparison of biological and chemical predictors of dermal carcinogenicity of petroleum oils. Polycyc Aromat Comp. 11(1-4):201–210.
- Blanding FH, King WH, Priestley W, et al. 1951. Properties of high-boiling petroleum products: quantitative analysis of tumor-response data obtained from the application of refinery products to the skin of mice. AMA Arch Ind Hyg Occup Med. 4(4):335–345.
- Blewitt RW, Bradbury K, Greenall MJ, Burrow H. 1977. Hepatic damage associated with mineral oil deposits. Gut. 18(6):476–479.
- BMEL. 2017. Entwurf des Bundesministeriums für Ernährung und Landwirtschaft: Zweiundzwanzigste Verordnung zur Änderung der Bedarfsgegenständeverordnung [Draft of the German Federal Ministry of Food and Agriculture: 22nd ordinance amending the Consumer Goods Ordinance]. German. [accessed 2019 Dec 1]. https://www.bmel.de/SharedDocs/Downloads/Ernaehrung/Rueckstaende/MineraloelVO_Entwurf.pdf?__blob=publicationFile
- Boitnott JK, Margolis S. 1966a. Mineral oil in human tissues. I. Detection of saturated hydrocarbons using thin-layer chromatography. Bull Johns Hopkins Hospit. 118(5):402–413.
- Boitnott JK, Margolis S. 1966b. Mineral oil in human tissues. II. Oil droplets in lymph nodes of the Porta Hepatis. Bull Johns Hopkins Hospit. 118:414–421.
- Boitnott JK, Margolis S. 1966c. The increasing incidence of mineral oil in human tissues. Fed Proc. 25:200.
- Boitnott JK, Margolis S. 1970. Saturated hydrocarbons in human tissues. III. Oil droplets in the liver and spleen. Johns Hopkins Med J. 127(2):65–78.
- Bollinger JN. 1970. Metabolic fate of mineral oil adjuvants using 14C-labeled tracers. I. Mineral oil. J Pharm Sci. 59(8):1084–1088.
- Boogaard PJ, Goyak KO, Biles RW, van Stee LL, Miller MS, Miller MJ. 2012. Comparative toxicokinetics of low-viscosity mineral oil in Fischer 344 rats, Sprague–Dawley rats, and humans – Implications for an Acceptable Daily Intake (ADI). Regul Toxicol Pharmacol. 63(1):69–77.
- Bowdler AJ. 1990. The spleen: structure, function and clinical significance. London: Chapman & Hall Mecical.
- Brinkmann J, Stolpmann K, Trappe S, Otter T, Genkinger D, Bock U, Liebsch M, Henkler F, Hutzler C, Luch A. 2013. Metabolically competent human skin models: activation and genotoxicity of benzo[a]pyrene. Toxicol Sci. 131(2):351–359.
- Broddle WD, Dennis MW, Kitchen DN, Vernot EH. 1996. Chronic dermal studies of petroleum streams in mice. Fundam Appl Toxicol. 30(1):47–54.
- Brown BE, Diembeck W, Hoppe U, Elias PM. 1995. Fate of topical hydrocarbons in the skin. J Soc Cosmet Chem. 46(1):1–9.
- Carlton WW, Boitnott JK, Dungworth DL, Ernst H, Hayashi Y, Mohr U, Parodi AL, Pattengale PK, Rittinghausen S, Ward JM. 2001. Assessment of the morphology and significance of the lymph nodal and hepatic lesions produced in rats by the feeding of certain mineral oils and waxes. Proceedings of a pathology workshop held at the Fraunhofer Institute of Toxicology and Aerosol Research Hannover, Germany, May 7–9, 2001. Exp Toxicol Pathol. 53(4):247–255.
- Cavalieri EL, Higginbotham S, RamaKrishna NV, et al. 1991. Comparative dose-response tumorigenicity studies of dibenzo[alpha,l]pyrene versus 7,12-dimethylbenz[alpha]anthracene, benzo[alpha]pyrene and two dibenzo[alpha,l]pyrene dihydrodiols in mouse skin and rat mammary gland. Carcinogenesis. 12(10):1939–1944.
- Cavret S, Laurent C, Feidt C, Laurent F, Rychen G. 2003. Intestinal absorption of 14C from 14C-phenanthrene, 14C-benzo[a]pyrene and 14C-tetrachlorodibenzo-para-dioxin: approaches with the Caco-2 cell line and with portal absorption measurements in growing pigs. Reprod Nutr Dev. 43(2):145–154.
- Chasey KL, McKee RH. 1993. Evaluation of the dermal carcinogenicity of lubricant base oils by the mouse skin painting bioassay and other proposed methods. J Appl Toxicol. 13(1):57–65.
- Chaudhuri UR. 2011. Fundamentals of petroleum and petrochemical engineering. Boca Raton: CRC Press. (Chemical industries; 130).
- Choe C, Lademann J, Darvin ME. 2015a. Analysis of human and porcine skin in vivo/ex vivo for penetration of selected oils by confocal Raman microscopy. Skin Pharmacol Physiol. 28(6):318–330.
- Choe C, Lademann J, Darvin ME. 2015b. Confocal Raman microscopy for investigating the penetration of various oils into the human skin in vivo. J Dermatol Sci. 79(2):176–178.
- Choe C, Schleusener J, Lademann J, Darvin ME. 2017. In vivo confocal Raman microscopic determination of depth profiles of the stratum corneum lipid organization influenced by application of various oils. J Dermatol Sci. 87(2):183–191.
- Choe C-S, Lademann J, Darvin ME. 2014. Gaussian-function-based deconvolution method to determine the penetration ability of petrolatum oil into in vivo human skin using confocal Raman microscopy. Laser Phys. 24(10):105601.
- Clark CR, McKee RH, Freeman JJ, Swick D, Mahagaokar S, Pigram G, Roberts LG, Smulders CJ, Beatty PW. 2013. A GHS-consistent approach to health hazard classification of petroleum substances, a class of UVCB substances. Regul Toxicol Pharmacol. 67(3):409–420.
- Cnubben NPH, van Stee LLP. 2011. Single dose pharmacokinetic study of P15 white oil in two rat strains. TNO Quality of Life Report V8503.
- Coash M, Forouhar F, Wu CH, Wu GY. 2012. Granulomatous liver diseases: a review. J Formos Med Assoc. 111(1):3–13.
- Comber M, Paumen ML, Redman A, Parkerton T, Eadsforth C, Linington S, den Haan K. 2014. Development of the Hydrocarbon Block Method for environmental risk assessment of petroleum substances. Poster TU282. Poster Presented at: SETAC Europe 24th Annual Meeting, Basel, Switzerland; May 11–15. [Accessed 2019 Dec 1]. https://www.concawe.eu/wp-content/uploads/2017/01/ccw_setac_2014_tu_282_p1_-_hydrocarbon_block_method-2014-02136-01-e.pdf
- Concawe. 1994. The use of the dimethyl sulphoxide (DMSO) extract by the IP 346 method as an indicator of the carcinogenicity of lubricant base oils and distillate aromatic extracts. Brussels: Concawe. Report no. 94/51. [Accessed 2019 Dec 1]. https://www.concawe.eu/wp-content/uploads/2017/01/rpt9451ocr-2005-00417-01-e.pdf
- Concawe. 2001. Classification and labelling of petroleum substances according to the EU dangerous substances directive (CONCAWE recommendations - August 2001). Brussels: Concawe. Report no. 01/53.
- Concawe. 2010. Review of dermal effects and uptake of petroleum hydrocarbons. Report prepared for Concawe’s health management group. Concawe report No. 5/10. Brussels, December 2010 [accessed 2019 Dec 1]. https://www.concawe.eu/wp-content/uploads/2017/01/rpt_10-5-2010-05153-01-e.pdf
- Concawe. 2016. Critical review of the relationship between IP346 and dermal carcinogenicity studies. Brussels: Concawe. Report no. 6/16. [Accessed 2019 Dec 1]. https://www.concawe.eu/wp-content/uploads/2017/01/report-6-16-final-version-3.pdf
- Concawe. 2017. Mineral oils are safe for human health? Retrieved from Brussels: Brochure prepared by the Concawe mineral hydrocarbons task force special MOCRINIS (STF-33). Brussels, October 2017 [accessed 2019 Dec 1]. https://www.concawe.eu/wp-content/uploads/2017/10/DEF_C_MM_digital.pdf
- Concin N, Hofstetter G, Plattner B, Tomovski C, Fiselier K, Gerritzen K, Fessler S, Windbichler G, Zeimet A, Ulmer H. 2008. Mineral oil paraffins in human body fat and milk. Food Chem Toxicol. 46(2):544–552.
- Concin N, Hofstetter G, Plattner B, Tomovski C, Fiselier K, Gerritzen K, Semsroth S, Zeimet AG, Marth C, Siegl H. 2011. Evidence for cosmetics as a source of mineral oil contamination in women. J Women's Health. 20(11):1713–1719.
- Cosmetics Europe. 2004. Recommendation N 15: Petrolatum. [accessed 2019 Dec 1]. https://www.cosmeticseurope.eu/download/Qk0zZGpsbGl6OFBLTGs3R1M2ZmVXQT09
- Cosmetics Europe. 2018. Recommendation N 14: Mineral hydrocarbons in cosmetic lip care products. [accessed 2019 Dec 1]. https://www.cosmeticseurope.eu/download/N08vNnB0TUhMbWpwQmlqVk9UZzdwZz09
- Cravedi J, Thibaut R, Tulliez J, Perdu E. 2011. Comparative in vitro study of the biotransformation of n-alkanes by liver and small intestine microsomes from different rat strains. Toxicol Lett. 205:S188.
- Cravedi J-P, Grob K, Nygaard UC, Alexander J. 2017. Bioaccumulation and toxicity of mineral oil hydrocarbons in rats – specificity of different subclasses of a broad mixture relevant for human dietary exposures. EFSA Supporting Publications. 14(2):EN-1090. 1–98.
- Cravedi J-P, Perdu E. 2012. In vitro metabolic study on alkanes in hepatic microsomes from humans and rats. EFSA Supporting Publications. 9(4):EN-263. 1–64.
- Cruickshank B, Thomas MJ. 1984. Mineral oil (follicular) lipidosis: II. Histologic studies of spleen, liver, lymph nodes, and bone marrow. Hum Pathol. 15(8):731–737.
- Cruickshank B. 1984. Follicular (mineral oil) lipidosis: I. Epidemiologic studies of involvement of the spleen. Hum Pathol. 15(8):724–730.
- Cullen MR, Balmes JR, Robins JM, Smith GJ. 1981. Lipoid pneumonia caused by oil mist exposure from a steel rolling tandem mill. Am J Ind Med. 2(1):51–58.
- Czarnowicki T, Malajian D, Khattri S, Correa da Rosa J, Dutt R, Finney R, Dhingra N, Xiangyu P, Xu H, Estrada YD. 2016. Petrolatum: barrier repair and antimicrobial responses underlying this “inert” moisturizer. J Allergy Clin Immunol. 137(4):1091–1102.e7.
- Dalbey W, Osimitz T, Kommineni C, Roy T, Feuston M, Yang J. 1991. Four-week inhalation exposures of rats to aerosols of three lubricant base oils. J Appl Toxicol. 11(4):297–302.
- Dalbey WE. 2001. Subchronic inhalation exposures to aerosols of three petroleum lubricants. AIHAJ. 62(1):49–56.
- Dankovic DA, Wright CW, Zangar RC, Springer DL. 1989. Complex mixture effects on the dermal absorption of benzo[a]pyrene and other polycyclic aromatic hydrocarbons from mouse skin. J Appl Toxicol. 9(4):239–244.
- de Mello ES, Ferreira Alves VA. 2018. Chapter 19 - Hepatic granulomas: differential diagnosis. In: Saxena R, editor. Practical hepatic pathology: a diagnostic approach. 2nd edition. Philadelphia: Elsevier; p. 289–300.
- Delladetsima JK, Horn T, Poulsen H. 1987. Portal tract lipogranulomas in liver biopsies. Liver. 7(1):9–17.
- Denk H, Scheuer PJ, Baptista A, Bianchi L, Callea F, De Groote J, Desmet VJ, Gudat F, Ishak KG, Korb G. 1994. Guidelines for the diagnosis and interpretation of hepatic granulomas. Histopathology. 25(3):209–218.
- Devillers J. 2000. Nonlinear modeling of the percutaneous absorption of polycyclic aromatic hydrocarbons. Polycyclic Aromatic Compounds. 18(2):231–241.
- Dill JA, Fuciarelli AF, Lee KM, Mellinger KM, Chan PC, Burka LT, Roycroft JH. 2003. Single administration toxicokinetic studies of decalin (decahydronaphthalene) in rats and mice. Toxicol Sci. 72(2):210–222.
- Dincsoy HP, Weesner R. 1981. Lipogranuloma in non-fatty human livers – a mineral oil-induced environmental disease. Lab Investigat. 44(1):A15.
- Dincsoy HP, Weesner RE, MacGee J. 1982. Lipogranulomas in non-fatty human livers. A mineral oil induced environmental disease. Am J Clin Pathol. 78(1):35–41.
- Doak SM, Brown VK, Hunt PF, Smith JD, Roe FJ. 1983. The carcinogenic potential of twelve refined mineral oils following long-term topical application. Br J Cancer. 48(3):429–436.
- Doak SM, Hend RW, van der Wiel A, Hunt PF. 1985. Carcinogenic potential of hydrotreated petroleum aromatic extracts. Br J Ind Med. 42(6):380–388.
- ECHA. 2017a. Approach on how to prioritise and address petroleum and coal stream UVCB substances for further work under the Roadmap for SVHC identification and implementation of REACH Risk Management Measures. Helsinki, Finland: European Chemicals Agency.
- ECHA. 2017b. Guidance for identification and naming of substances under REACH and CLP. Version 2.1. Helsinki, Finland: European Chemicals Agency.
- ECHA. 2017c. Information on chemicals. Dataset on white mineral oil (petroleum) (CAS Number 8042-47-5). [Accessed 2019 Dec 1]. https://echa.europa.eu/de/substance-information/-/substanceinfo/100.029.500
- EFSA. 2008. Polycyclic aromatic hydrocarbons in food – Scientific opinion of the Panel on Contaminants in the Food Chain. EFSA J. 724:1–114.
- EFSA. 2009. Scientific opinion on the use of high viscosity white mineral oils as a food additive. EFSA J. 7(11):1387.
- EFSA. 2012. Scientific opinion on mineral oil hydrocarbons in food. EFSA J. 10(6):2704.
- EFSA. 2013a. Scientific opinion on the re-evaluation of microcrystalline wax (E 905) as a food additive. EFSA J. 11(4):3146.
- EFSA. 2013b. Scientific opinion on the safety assessment of medium viscosity white mineral oils with a kinematic viscosity between 8.5 and 11 mm2/s at 100 °C for the proposed uses as a food additive. EFSA J. 11(1):3073.
- Elliott TH, Robertson JS, Williams RT. 1966. The metabolism of cis- and trans-decalin. Biochem J. 100(2):403–406.
- European Chemicals Bureau. 1999. European Union Risk Assessment Report: Benzene C10-13 alkyl derivs. Luxembourg: Office for Official Publications of the European Communities.
- European Commission. 2013. Roadmap on Substances of Very High Concern. European Commission, DG Environment/DG Enterprise and Industry 5867/13 TG/mf 4 ANNEX DGG 3A. [accessed 2019 Dec 1]. http://register.consilium.europa.eu/doc/srv?l=EN&f=ST%205867%202013%20INIT
- Firriolo JM, Morris CF, Trimmer GW, Twitty LD, Smith JH, Freeman JJ. 1995. Comparative 90-day feeding study with low-viscosity white mineral oil in Fischer-344 and Sprague–Dawley-derived CRL:CD rats. Toxicol Pathol. 23(1):26–33.
- Fischer HG, Priestley W, Jr., Eby LT, et al. 1951. Properties of high-boiling petroleum products; physical and chemical properties as related to carcinogenic activity. AMA Arch Ind Hyg Occup Med. 4(4):315–324.
- Fiselier K, Fiorini D, Grob K. 2009. Activated aluminum oxide selectively retaining long chain n-alkanes: Part II. Integration into an on-line high performance liquid chromatography-liquid chromatography-gas chromatography-flame ionization detection method to remove plant paraffins for the determination of mineral paraffins in foods and environmental samples. Anal Chim Acta. 634(1):102–109.
- Fiselier K, Grob K. 2009. Determination of mineral oil paraffins in foods by on-line HPLC-GC-FID: lowered detection limit; contamination of sunflower seeds and oils. Eur Food Res Technol. 229(4):679–688.
- Fleming K, Carrillo J-C. 2018. MOH accumulation in F344 rats. Sci Total Environ. 615:1095–1098.
- Fleming KA, Zimmerman H, Shubik P. 1998. Granulomas in the livers of humans and Fischer rats associated with the ingestion of mineral hydrocarbons: a comparison. Regul Toxicol Pharm. 27(1):75–81.
- Freeman JJ, Federici TM, Mckee RH. 1993. Evaluation of the contribution of chronic skin irritation and selected compositional parameters to the tumorigenicity of petroleum middle distillates in mouse skin. Toxicology. 81(2):103–112.
- Gazzarrini F, Nagy B. 1966. Saturated hydrocarbons in human femoral arterial tissues and plaques. Arch Biochem Biophys. 113(1):245–247.
- Gerhart JM, Hatoum NS, Halder CA, Warne TM, Schmitt SL. 1988. Tumor initiation and promotion effects of petroleum streams in mouse skin. Fundam Appl Toxicol. 11(1):76–90.
- Ghadially R, Halkier-Sorensen L, Elias PM. 1992. Effects of petrolatum on stratum corneum structure and function. J Am Acad Dermatol. 26(3):387–396.
- Gradiski D, Vinot J, Zissu D, Limasset JC, Lafontaine M. 1983. The carcinogenic effect of a series of petroleum-derived oils on the skin of mice. Environ Res. 32(2):258–268.
- Gray TM, Simpson BJ, Nicolich MJ, Murray FJ, Verstuyft AW, Roth RN, McKee RH. 2013. Assessing the mammalian toxicity of high-boiling petroleum substances under the rubric of the HPV program. Regul Toxicol Pharmacol. 67(2):S4–S9.
- Griffis LC, Twerdok LE, Francke-Carroll S, Biles RW, Schroeder RE, Bolte H, Faust H, Hall WC, Rojko J. 2010. Comparative 90-day dietary study of paraffin wax in Fischer-344 and Sprague–Dawley rats. Food Chem Toxicol. 48(1):363–372.
- Gute BD, Grunwald GD, Basak SC. 1999. Prediction of the dermal penetration of polycyclic aromatic hydrocarbons (PAHs): a hierarchical QSAR approach. SAR QSAR Environ Res. 10(1):1–15.
- Habs M, Schmahl D, Misfeld J. 1980. Local carcinogenicity of some environmentally relevant polycyclic aromatic-hydrocarbons after lifelong topical application to mouse skin. Archiv Geschwulstforsc. 50(3):266–274.
- Halladay JS, Mackerer CR, Twerdok LE, Sipes IG. 2002. Comparative pharmacokinetic and disposition studies of [1-14C]1-eicosanylcyclohexane, a surrogate mineral hydrocarbon, in female Fischer-344 and Sprague–Dawley rats. Drug Metab Dispos. 30(12):1470–1477.
- Hard GC. 2000. Short-term adverse effects in humans of ingested mineral oils, their additives and possible contaminants – a review. Hum Exp Toxicol. 19(3):158–172.
- Harrell Jr. FE; with contributions from Charles Dupont and many others. (2019). Hmisc: Harrell Miscellaneous. R package version 4.2-0. https://CRAN.R-project.org/package=Hmisc
- Hansen RP. 1968. 4,8,12-Trimethyltridecanoic acid: its isolation and identification from sheep perinephric fat. Biochim Biophys Acta. 164(3):550–557.
- Harling A, Helling R, Simat T, Grob K. 2012. Analytik von 119 Lebensmitteln auf unerwünschte Substanzen aus Altpapier. Eine Ergänzung zur wissenschaftlichen Studie “Ausmaß der Migration unerwünschter Stoffe aus Verpackungsmaterialien aus Altpapier in Lebensmittel” [Analysis of 119 foods packaged in recycled paperboard cartons. Appendix to the report entitled "Degree of migration of undesirable substances from packaging materials made of recycled paper in food"]. German. [Accessed 2019 Dec 1]. http://download.ble.de/09HS012.pdf
- Harris KL, Banks LD, Harris KJ, Archibong AE, Hood DB, Ramesh A. 2016. Absorption of food toxicants: an overview on polycyclic aromatic hydrocarbons. In: Bagchi D, Swaroop A, editors. Food toxicology. CRC Press; p. 83–96.
- Harris KL, Banks LD, Mantey JA, Huderson AC, Ramesh A. 2013. Bioaccessibility of polycyclic aromatic hydrocarbons: relevance to toxicity and carcinogenesis. Expert Opin Drug Metab Toxicol. 9(11):1465–1480.
- Health Canada. 2016. Screening Assessment Petroleum Sector Stream Approach Petrolatum and Waxes [Stream 4] Chemical Abstracts Service Registry Numbers 8009-03-8, 64742-61-6, 64743-01-7. [Accessed 2019 Dec 1]. http://www.ec.gc.ca/ese-ees/E78B1855-EE77-40D6-BBC7-964AF3A8E3EB/FSAR_PSSA4-Petrolatum%20and%20Waxes_EN.pdf.
- Heimbach JT, Bodor AR, Douglass JS, Barraj LM, Cohen SC, Biles RW, Faust HR. 2002. Dietary exposures to mineral hydrocarbons from food-use applications in the United States. Food Chem Toxicol. 40(5):555–571.
- Higginbotham S, RamaKrishna NV, Johansson SL, et al. 1993. Tumor-initiating activity and carcinogenicity of dibenzo[a,l]pyrene versus 7,12-dimethylbenz[a]anthracene and benzo[a]pyrene at low doses in mouse skin. Carcinogenesis. 14(5):875–878.
- Hoglen NC, Regan SP, Hensel JL, Younis HS, Sauer JM, Steup DR, Miller MJ, Waterman SJ, Twerdok LE, Sipes IG. 1998. Alteration of Kupffer cell function and morphology by low melt point paraffin wax in female Fischer-344 but not Sprague–Dawley rats. Toxicol Sci. 46(1):176–184.
- Höke H, Zellerhoff R. 1998. Metabolism and toxicity of diisopropylnaphthalene as compared to naphthalene and monoalkyl naphthalenes: a minireview. Toxicology. 126(1):1–7.
- Honda T, Kiyozumi M, Kojima S. 1990. Alkylnaphthalene. XI. Pulmonary toxicity of naphthalene, 2-methylnaphthalene, and isopropylnaphthalenes in mice. Chem Pharm Bull. 38(11):3130–3135.
- Horton AW, Denman DT, Trosset RP. 1957. Carcinogenesis of the skin. II. The accelerating properties of aliphatic and related hydrocarbons. Cancer Res. 17(8):758–766.
- Hudson RP, Robertson GM. 1966. Demonstration of mineral oil in splenic lipid granulomas. Lab Invest. 15(6):1134–1135.
- IARC. 1984. Polynuclear aromatic hydrocarbons, Part 2, Carbon blacks, mineral oils (lubricant base oils and derived products) and some nitroarenes. IARC Monogr Eval Carcinog Risk Chem Hum. 33:1–222.
- IARC. 2010. Some non-heterocyclic polycyclic aromatic hydrocarbons and some related exposures. Lyon: IARC Press. (IARC Monographs on the evaluation of carcinogenic risk of chemicals to humans).
- IARC. 2012. Mineral oils, untreated or mildly treated (IARC Monographs on the Evaluation of Carcinogenic Risks to Humans, No. 100F). IARC Working Group on the Evaluation of Carcinogenic Risk to Humans. Chemical Agents and Related Occupations. Lyon (FR): International Agency for Research on Cancer. [Accessed 2019 Dec 1]. https://monographs.iarc.fr/wp-content/uploads/2018/06/mono100F-19.pdf
- Ichihara K, Ishihara K, Kusunose E, Kusunose M. 1981. Some properties of a hexadecane hydroxylation system in rabbit intestinal mucosa microsomes. J Biochem. 89(6):1821–1827.
- Ingram AJ, Grasso P. 1991. Evidence for and possible mechanisms of non-genotoxic carcinogenesis in mouse skin. Mutat Res. 248(2):333–340.
- Ingram AJ, Scammells DV, May K. 1994. An investigation of the main mutagenic components of a carcinogenic oil by fractionation and testing in the modified Ames assay. J Appl Toxicol. 14(3):173–179.
- Institute of Petroleum. 2004. Determination of polycyclic aromatics in unused lubricating base oils and asphaltene free petroleum fractions – Dimethyl sulphoxide extraction refractive index method. IP 346/92. London: Institute of Petroleum.
- Intarakumhaeng R, Shi Z, Wanasathop A, Stella QC, Wei KS, Styczynski PB, Li C, Smith ED, Li SK. 2018. In vitro skin penetration of petrolatum and soybean oil and effects of glyceryl monooleate. Int J Cosmet Sci. 40(4):367–376.
- Iversen OH. 1989. Studies of the carcinogenesis and tumorigenesis of skin applications of dodecylbenzene on hairless mice. Br J Ind Med. 46(9):608–616.
- Jacques C, Perdu E, Duplan H, Jamin EL, Canlet C, Debrauwer L, Cravedi JP, Mavon A, Zalko D. 2010. Disposition and biotransformation of 14C-benzo(a)pyrene in a pig ear skin model: ex vivo and in vitro approaches. Toxicol Lett. 199(1):22–33.
- Jakasa I, Kezic S, Boogaard PJ. 2015. Dermal uptake of petroleum substances. Toxicol Lett. 235(2):123–139.
- Jampolis RW, McDonald JR, Clagett OT. 1953. Mineral oil granuloma of the lungs; an evaluation of methods for identification of mineral oil in tissue. Int Abstr Surg. 97(2):105–119.
- Janz S, Shacter E. 1995. Disposition of the plasmacytomagenic alkane pristane (2,6,10,14-tetramethylpentadecane) in mice. Cancer Biochem Bioph. 15(1):25–34.
- JECFA. 2002. Evaluation of certain food additives – Fifty-ninth report of the Joint FAO/WHO Expert Committee on food Additives. Geneva: WHO.
- JECFA. 2006. Polycyclic aromatic hydrocarbons. Safety evaluation of certain contaminants in food. Geneva: WHO; p. 563–743.
- JECFA. 2012. Evaluation of certain food additives – Seventy-sixth report of the Joint FAO/WHO Expert Committee on Food Additives. Geneva: WHO.
- Jouanneau J. 2017. Manufacture of mineral oil and wax composition and specifications. Mocrinis II Workshop; 17–18 October 2017; Brussels.
- JRC. 2019. Guidance on sampling, analysis and data reporting for the monitoring of mineral oil hydrocarbons in food and food contact materials. Luxembourg: Publications Office of the European Union. [Accessed 2019 Dec 1]. https://op.europa.eu/en/publication-detail/-/publication/85bdda9a-3656-11e9-8d04-01aa75ed71a1/language-en
- Jung EC, Maibach HI. 2015. Animal models for percutaneous absorption. J Appl Toxicol. 35(1):1–10.
- Kamelia L, Brugman S, De Haan L, Ketelslegers HB, Rietjens I, Boogaard PJ. 2019. Prenatal developmental toxicity testing of petroleum substances using the zebrafish embryotoxicity test. Altex. 36(2):245–260.
- Kamelia L, de Haan L, Ketelslegers HB, Rietjens I, Boogaard PJ. 2019. In vitro prenatal developmental toxicity induced by some petroleum substances is mediated by their 3- to 7-ring PAH constituent with a potential role for the aryl hydrocarbon receptor (AhR). Toxicol Lett. 315:64–76.
- Kamelia L, Louisse J, De Haan L, Maslowska-Gornicz A, Ketelslegers HB, Brouwer A, Rietjens I, Boogaard PJ. 2018. The role of endocrine and dioxin-like activity of extracts of petroleum substances in developmental toxicity as detected in a panel of CALUX Reporter Gene Assays. Toxicol Sci. 164(2):576–591.
- Kamelia L, Louisse J, de Haan L, Rietjens I, Boogaard PJ. 2017. Prenatal developmental toxicity testing of petroleum substances: application of the mouse embryonic stem cell test (EST) to compare in vitro potencies with potencies observed in vivo. Toxicol in Vitro. 44:303–312.
- Kelsall GR, Blackwell JB. 1969. The occurrence and significance of lipophage clusters in lymph nodes and spleen. Pathology. 1(3):211–220.
- King D, Lyne RL, Girling A, Peterson Dr Stephenson R, Short D. 1996. Environmental risk assessment of petroleum substances: The hydrocarbon block method. Brussels, Belgium: Concawe.
- Klatskin G. 1977. Hepatic granulomata: problems in interpretation. Mt Sinai J Med. 44(6):798–812.
- Kojima S, Nakagawa M, Suzuki R, Horio M, Tanaka Y. 1978. Alkylnaphthalenes. I. Absorption, tissue distribution and excretion of 2,6-diisopropylnaphthalene in rats. Chem Pharm Bull. 26(10):3007–3009.
- Kolattukudy PE, Hankin L. 1966. Metabolism of a plant wax paraffin (n-nonacosane) in the rat. J Nutr. 90(2):167–174.
- Kumar V, Abbas AK, Aster JC. 2018. Robbins basic pathology. 10th edition ed. Philadelphia: Elsevier.
- Kusunose M, Ichihara K, Kusunose E. 1969. Oxidation of n-hexadecane by mouse liver microsomal fraction. Biochim Biophys Acta. 176(3):679–681.
- Laher JM, Barrowman JA. 1987. Role of the lymphatic system in the transport of absorbed 7,12-dimethylbenzanthracene in the rat. Lipids. 22(3):152–155.
- Laher JM, Barrowman JA. 1988. Intestinal absorption of carcinogenic hydrocarbons. Ann NY Acad Sci. 534(1):565–574.
- Laher JM, Chernenko GA, Barrowman JA. 1983. Studies of the absorption and enterohepatic circulation of 7,12-dimethylbenz[a]anthracene in the rat. Can J Physiol Pharmacol. 61(11):1368–1373.
- Laher JM, Rigler MW, Vetter RD, Barrowman JA, Patton JS. 1984. Similar bioavailability and lymphatic transport of benzo(a)pyrene when administered to rats in different amounts of dietary fat. J Lipid Res. 25(12):1337–1342.
- Laurent C, Feidt C, Grova N, Mpassi D, Lichtfouse E, Laurent F, Rychen G. 2002. Portal absorption of 14C after ingestion of spiked milk with 14C-phenanthrene, 14C-benzo[a]pyrene or 14C-TCDD in growing pigs. Chemosphere. 48(8):843–848.
- LaVoie EJ, Bedenko V, Tulley-Freiler L, et al. 1982. Tumor-initiating activity and metabolism of polymethylated phenanthrenes. Cancer Res. 42(10):4045–4049.
- LaVoie EJ, Cai ZW, Meegalla RL, et al. 1993. Evaluation of the tumor-initiating activity of 4-, 5-, 6-, and 7-fluorobenzo[b]fluoranthene in mouse skin. Chem Biol Interact. 89(2–3):129–139.
- LaVoie EJ, Coleman DT, Rice JE, Geddie NG, Hoffmann D. 1985. Tumor-initiating activity, mutagenicity, and metabolism of methylated anthracenes. Carcinogenesis. 6(10):1483–1488.
- LaVoie EJ, He ZM, Meegalla RL, et al. 1993. Exceptional tumor-initiating activity of 4-fluorobenzo[j]-fluoranthene on mouse skin: comparison with benzo[j]-fluoranthene, 10-fluoro-benzo[j]fluoranthene, benzo[a]pyrene, dibenzo[a,l]pyrene and 7,12-dimethylbenz[a]anthracene. Cancer Lett. 70(1–2):7–14.
- LaVoie EJ, Tulley-Freiler L, Bedenko V, et al. 1981. Mutagenicity, tumor-initiating activity, and metabolism of methylphenanthrenes. Cancer Res. 41(9 Pt 1):3441–3447.
- Le Bon AM, Cravedi JP, Tulliez JE. 1988. Disposition and metabolism of pristane in rat. Lipids. 23(5):424–429.
- Lewis SC. 1983. Crude petroleum and selected fractions. Skin cancer bioassays. Prog Exp Tumor Res. 26:68–84.
- Liber AF, Rose HG. 1967. Saturated hydrocarbons in follicular lipodosis of the spleen. Arch Pathol. 83(2):116–122.
- Lijinsky W, Garcia H, Saffiotti U. 1970. Structure-activity relationships among some polynuclear hydrocarbons and their hydrogenated derivatives. J Natl Cancer Inst. 44(3):641–649.
- Lijinsky W, Garcia H, Terracini B, Saffiotti U. 1965. Tumorigenic activity of hydrogenated derivatives of dibenz[a,h]anthracene. J Natl Cancer Inst. 34(1):1–6.
- Lijinsky W, Garcia H. 1972. Skin carcinogenesis tests of hydrogenated derivatives of anthanthrene and other polynuclear hydrocarbons. Z Krebsforsch. 77(3):226–230.
- Lindstrom MB, Barrowman JA, Borgstrom B. 1987. Fate of 7,12-dimethylbenz(a)anthracene absorbed from the rat intestine and transported in chylomicrons. Lipids. 22(4):278–281.
- Lorenzini R, Fiselier K, Biedermann M, Barbanera M, Braschi I, Grob K. 2010. Saturated and aromatic mineral oil hydrocarbons from paperboard food packaging: estimation of long-term migration from contents in the paperboard and data on boxes from the market. Food Addit Contam Part A. 27(12):1765–1774.
- Mackerer CR, Griffis LC, Grabowski JS, Jr, Reitman FA. 2003. Petroleum mineral oil refining and evaluation of cancer hazard. Appl Occup Environ Hyg. 18(11):890–901.
- MAK. 2015. Weißöl, pharmazeutisch [MAK Value Documentation in German Language, 2015]. The MAK Collection for Occupational Health and Safety: Annual Thresholds and Classifications for the Workplace. Germany: Wiley & Sons. pp. 1–13.
- Mao-Qiang M, Brown BE, Wu-Pong S, Feingold KR, Elias PM. 1995. Exogenous nonphysiologic vs physiologic lipids. Divergent mechanisms for correction of permeability barrier dysfunction. Arch Dermatol. 131(7):809–816.
- McCarthy RD. 1964. Mammalian metabolism of straight-chain saturated hydrocarbons. Biochim Biophys Acta. 84:74–79.
- McKee RH, Adenuga MD, Carrillo JC. 2015. Characterization of the toxicological hazards of hydrocarbon solvents. Crit Rev Toxicol. 45(4):273–365.
- McKee RH, Daughtrey WC, Freeman JJ, Federici TM, Phillips RD, Plutnick RT. 1989. The dermal carcinogenic potential of unrefined and hydrotreated lubricating oils. J Appl Toxicol. 9(4):265–270.
- McKee RH, Drummond JG, Freeman JJ, Letinski DJ, Miller MJ. 2012. Light white oils exhibit low tissue accumulation potential and minimal toxicity in F344 rats. Int J Toxicol. 31(2):175–183.
- McKee RH, Nicolich MJ, Scala RA, Lewis SC. 1990. Estimation of epidermal carcinogenic potency. Fundam Appl Toxicol. 15(2):320–328.
- McKee RH, Plutnick RT, Przygoda RT. 1989. The carcinogenic initiating and promoting properties of a lightly refined paraffinic oil. Fundam Appl Toxicol. 12(4):748–756.
- McKee RH, Scala RA, Chauzy C. 1990. An evaluation of the epidermal carcinogenic potential of cutting fluids. J Appl Toxicol. 10(4):251–256.
- McKee RH, White R. 2014. The mammalian toxicological hazards of petroleum-derived substances: an overview of the petroleum industry response to the high production volume challenge program. Int J Toxicol. 33(1_suppl):4S–16S.
- McWeeny DJ. 1957. Intestinal absorption of paraffins. Birmingham: University of Birmingham.
- Mehrotra NK, Kumar S, Antony M. 1988. Carcinogenic property of JBO(P) variety of jute batching oil. Drug Chem Toxicol. 11(2):181–193.
- Mehrotra NK, Saxena AK. 1979. Evaluation of carcinogenic effect of mineral oil used in the processing of jute fibres. Br J Exp Pathol. 60(5):518–525.
- Mellert W, Deckardt K, Gembardt C, Hildebrand B, Schulte S. 2004. Carcinogenicity and chronic toxicity of copovidone (Kollidon VA 64) in Wistar rats and Beagle dogs. Food Chem Toxicol. 42(10):1573–1587.
- Merk HF, Mukhtar H, Kaufmann I, Das M, Bickers DR. 1987. Human hair follicle benzo[a]pyrene and benzo[a]pyrene 7,8-diol metabolism: effect of exposure to a coal tar-containing shampoo. J Invest Dermatol. 88(1):71–76.
- Miller MJ, Lonardo EC, Greer RD, Bevan C, Edwards DA, Smith JH, Freeman JJ. 1996. Variable responses of species and strains to white mineral oils and paraffin waxes. Regul Toxicol Pharmacol. 23(1):55–68.
- Mitchell MP, Hübscher G. 1968. Oxidation of n-hexadecane by subcellular preparations of guinea pig small intestine. Eur J Biochem. 7(1):90–95.
- Moore KL, Dalley AF, Agur A. 2014. Clinically orientated anatomy. Baltimore: Lippincott Williams & Wilkins.
- Moret S, Conte LS. 2000. Polycyclic aromatic hydrocarbons in edible fats and oils: occurrence and analytical methods. J Chromatogr A. 882(1-2):245–253.
- Moss GP, Dearden JC, Patel H, Cronin MT. 2002. Quantitative structure-permeability relationships (QSPRs) for percutaneous absorption. Toxicol in Vitro. 16(3):299–317.
- Murata Y, Denda A, Maruyama H, et al. 1993. Chronic toxicity and carcinogenicity studies of 1-methylnaphthalene in B6C3F1 mice. Fund Appl Toxicol. 21(1):44–51.
- Murata Y, Denda A, Maruyama H, et al. 1997. Chronic toxicity and carcinogenicity studies of 2-methylnaphthalene in B6C3F mice. Fund Appl Toxicol. 36(1):90–93.
- Nagy B, Modzeleski VE, Scott WM. 1969. Saturated hydrocarbons in bovine liver. Biochem J. 114(3):645–648.
- Nash JF, Gettings SD, Diembeck W, Chudowski M, Kraus AL. 1996. A toxicological review of topical exposure to white mineral oils. Food Chem Toxicol. 34(2):213–225.
- Natusch DFS, Tomkins BA. 1978. Isolation of polycyclic organic compounds by solvent-extraction with dimethyl-sulfoxide. Anal Chem. 50(11):1429–1434.
- Nessel CS. 1999. A comprehensive evaluation of the carcinogenic potential of middle distillate fuels. Drug Chem Toxicol. 22(1):165–180.
- Nessel CS, Freeman JJ, Forgash RC, McKee RH. 1999. The role of dermal irritation in the skin tumor promoting activity of petroleum middle distillates. Toxicol Sci. 49(1):48–55.
- Nessel CS, Priston RA, McKee RH, Cruzan G, Riley AJ, Hagemann R, Plutnick RT, Simpson BJ. 1998. A comprehensive evaluation of the mechanism of skin tumorigenesis by straight-run and cracked petroleum middle distillates. Toxicol Sci. 44(1):22–31.
- Ng KM, Chu I, Bronaugh RL, Franklin CA, Somers DA. 1991. Percutaneous absorption/metabolism of phenanthrene in the hairless guinea pig: comparison of in vitro and in vivo results. Fundam Appl Toxicol. 16(3):517–524.
- Ng KM, Chu I, Bronaugh RL, Franklin CA, Somers DA. 1992. Percutaneous absorption and metabolism of pyrene, benzo[a]pyrene, and di(2-ethylhexyl) phthalate: comparison of in vitro and in vivo results in the hairless guinea pig. Toxicol Appl Pharmacol. 115(2):216–223.
- Niederer M, Stebler T, Grob K. 2015. Mineral oil and synthetic hydrocarbons in cosmetic lip products. Int J Cosmet Sci. 38(2):194–200.
- Nochomovitz LE, Uys CJ, Epstein S. 1975. Massive deposition of mineral oil after prolonged ingestion. S Afr Med J. 49(53):2187–2190.
- Noti A, Grob K, Biedermann M, Deiss U, Bruschweiler BJ. 2003. Exposure of babies to C15-C45 mineral paraffins from human milk and breast salves. Regul Toxicol Pharmacol. 38(3):317–325.
- OECD. 2014. OECD guidance for characterising oleochemical substances for assessment purposes. Paris, France: Organisation for Economic Co-Operation and Development.
- OECD. 2016. OECD guidance for characterising hydrocarbon solvents for assessment purposes. Paris, France: Organisation for Economic Co-Operation and Development. ENV/JM/MONO 2015. 52.
- Ohtani O, Ohtani Y. 2008. Lymph circulation in the liver. Anat Rec. 291(6):643–652.
- Olson CT, Yu KO, Serve MP. 1986. Metabolism of nephrotoxic cis- and trans-decalin in Fischer-344 rats. J Toxicol Environ Health. 18(2):285–292.
- Pannatier A, Jenner P, Testa B, Etter JC. 1978. The skin as a drug-metabolizing organ. Drug Metab Rev. 8(2):319–343.
- Patzelt A, Lademann J, Richter H, et al. 2011. Oils on skin: penetration and occlusion of mineral oil and vegetable oils. Acta Paediatr. 100:27.
- Patzelt A, Lademann J, Richter H, Darvin ME, Schanzer S, Thiede G, Sterry W, Vergou T, Hauser M. 2012. In vivo investigations on the penetration of various oils and their influence on the skin barrier. Skin Res Technol. 18(3):364–369.
- Payan JP, Lafontaine M, Simon P, et al. 2008. In vivo and in vitro percutaneous absorption of [14C]pyrene in Sprague Dawley male rats: skin reservoir effect and consequence on urinary 1-OH pyrene excretion. Arch Toxicol. 82(10):739–747.
- Perdu-Durand EF, Tulliez JE. 1985. Hydrocarbon hydroxylation system in liver microsomes from four animal species. Food Chem Toxicol. 23(3):363–366.
- Peters T. Jr 1995. Ligand binding by albumin. In: Peters T, editor. All About Albumin. Biochemistry, genetics, and medical applications. San Diego: Academic Press; p. 76–132.
- Petry T, Bury D, Fautz R, Hauser M, Huber B, Markowetz A, Mishra S, Rettinger K, Schuh W, Teichert T. 2017. Review of data on the dermal penetration of mineral oils and waxes used in cosmetic applications. Toxicol Lett. 280:70–78.
- Phillips M, Greenberg J, Cataneo RN. 2000. Effect of age on the profile of alkanes in normal human breath. Free Radic Res. 33(1):57–63.
- Phillips M, Herrera J, Krishnan S, Zain M, Greenberg J, Cataneo RN. 1999. Variation in volatile organic compounds in the breath of normal humans. J Chromatogr B Biomed Sci Appl. 729(1-2):75–88.
- Pittelkow MR, Perry HO, Muller SA, Maughan WZ, O'Brien PC. 1981. Skin cancer in patients with psoriasis treated with coal tar. A 25-year follow-up study. Arch Dermatol. 117(8):465–468.
- Pokrovskii AA, Markelova VF, Tatsievskii VA, Levachev MM. 1969. [Transformation of hydrocarbons in animals]. Biokhimiia. 34(4):791–794.
- Popovic M, Gernecevic N, Dimovski J. 1973. Absorption of n-paraffins in the rat. Acta Pharm Jugosl. 23:195–201.
- Popovic M, Toman L, Mesaric M, Ismaili M, Xhaferi M. 1982. Distribution of exogenous n-paraffin and its incorporation into glycerolipids in the rat. Acta Pharm Jugosl. 32(1):59–66.
- Popovic M. 1970. The metabolism of paraffins in rats. FEBS Lett. 12(1):49–50.
- Potter D, Booth ED, Brandt HC, Loose RW, Priston RA, Wright AS, Watson WP. 1999. Studies on the dermal and systemic bioavailability of polycyclic aromatic compounds in high viscosity oil products. Arch Toxicol. 73(3):129–140.
- Rahman A, Barrowman JA, Rahimtula A. 1986. The influence of bile on the bioavailability of polynuclear aromatic hydrocarbons from the rat intestine. Can J Physiol Pharmacol. 64(9):1214–1218.
- Ramesh A, Walker SA, Hood DB, Guillen MD, Schneider K, Weyand EH. 2004. Bioavailability and risk assessment of orally ingested polycyclic aromatic hydrocarbons. Int J Toxicol. 23(5):301–333.
- Rees ED, Mandelstam P, Lowry J. 1969. In vitro and in vivo studies of intestinal absorption of aromatic polycyclic hydrocarbon carcinogens. Fed Proc. 28(2):749.
- Rice JE, Rivenson A, Braley J, LaVoie EJ. 1987. Methylated derivatives of pyrene and fluorene: evaluation of genotoxicity in the hepatocyte/DNA repair test and tumorigenic activity in newborn mice. J Toxicol Environ Health. 21(4):525–532.
- Ritz C, Baty F, Streibig JC, Gerhard D. 2015. Dose-response analysis using R. PLOS One. 10(12):e0146021.
- Roelofzen JH, Aben KK, Van de Kerkhof PC, Van der Valk PG, Kiemeney LA. 2015. Dermatological exposure to coal tar and bladder cancer risk: a case-control study. Urol Oncol. 33(1):20–22.
- Rorije E, Verbruggen EMJ, de Knecht JA. 2012. Service request on a critical review of the environmental and physicochemical methodologies commonly employed in the environmental risk assessment of petroleum substances in the context of REACH registrations. Bilthoven, The Netherlands: Dutch National Institute for Public Health and the Environment (RIVM).
- Rose HG, Liber AF. 1966. Accumulation of saturated hydrocarbons in human spleens. J Lab Clin Med. 68(3):475–483.
- Rossmiller JD, Hoekstra WG. 1966. Hexadecane-induced hyperkeratinization of guinea pig skin. III. Cutaneous penetration of topically applied hexadecane-1-C-14. J Invest Dermatol. 47(1):39–43.
- Roy TA, Blackburn GR, Mackerer CR. 1995. Evaluation of analytical endpoints to predict carcinogenic potency of mineral oils. Polycyc Aromat Comp. 5(1-4):279–287.
- Roy TA, Blackburn GR, Mackerer CR. 1996. Evaluation of physicochemical factors affecting dermal penetration and carcinogenic potency of mineral oils containing polycyclic aromatic compounds. Polycyc Aromat Comp. 10(1-4):333–342.
- Roy TA, Johnson SW, Blackburn GR, Mackerer CR. 1988. Correlation of mutagenic and dermal carcinogenic activities of mineral oils with polycyclic aromatic compound content. Fundam Appl Toxicol. 10(3):466–476.
- Roy TA, Krueger AJ, Mackerer CR, Neil W, Arroyo AM, Yang JJ. 1998. SAR models for estimating the percutaneous absorption of polynuclear aromatic hydrocarbons. SAR QSAR Environ Res. 9(3-4):171–185.
- Salvayre R, Negre A, Rocchiccioli F, et al. 1988. A new human pathology with visceral accumulation of long-chain n-alkanes; tissue distribution of the stored compounds and pathophysiological hypotheses. Biochim Biophys Acta. 958(3):477–483.
- Sartorelli P, Cenni A, Matteucci G, Montomoli L, Novelli MT, Palmi S. 1999. Dermal exposure assessment of polycyclic aromatic hydrocarbons: in vitro percutaneous penetration from lubricating oil. Int Arch Occup Environ Health. 72(8):528–532.
- Sarkar D. 2008. Lattice: multivariate data visualization with R. New York: Springer-Verlag.
- Savary P, Constantin MJ. 1967. [On the micellar solubilization of hexadecane and its passage into thoracic duct lymph of the rat]. Biochim Biophys Acta. 137(2):264–276.
- SCCS. 2016. Scientific Committee on Consumer Safety report SCCS/1564/15. The SCCS notes of guidance for the testing of cosmetic substances and their safety evaluation. 9th revision, revised version 25 April 2016.
- SCOEL. 2011. Recommendation from the Scientific Committee on occupational exposure limits for aerosols of severely refined mineral oils. SCOEL/SUM/163. [Accessed 2017 Jan 27]. https://ec.europa.eu/social/BlobServlet?docId=6662&langId=en
- Scotter MJ, Castle L, Massey RC, Brantom PG, Cunninghame ME. 2003. A study of the toxicity of five mineral hydrocarbon waxes and oils in the F344 rat, with histological examination and tissue-specific chemical characterisation of accumulated hydrocarbon material. Food Chem Toxicol. 41(4):489–521.
- Shoda T, Toyoda K, Uneyama C, Takada K, Takahashi M. 1997. Lack of carcinogenicity of medium-viscosity liquid paraffin given in the diet to F344 rats. Food Chem Toxicol. 35(12):1181–1190.
- Shubik P, Rappaport H, Tomatis L, Raha CR, Lijinsky W, Feldman R, Toth B, Pietra G, Ramahi H, Saffiotti U. 1962. Studies on the toxicity of petroleum waxes. Toxicol Appl Pharm. 4:1–61.
- Shubik P, Saffiotti U. 1955. The carcinogenic and promoting action of low boiling catalytically cracked oils. Acta Union Int Contr. 11(6):707–711.
- Singh S, Zhao K, Singh J. 2002. In vitro permeability and binding of hydrocarbons in pig ear and human abdominal skin. Drug Chem Toxicol. 25(1):83–92.
- Skipski VP, Barclay M, Barclay RK, Fetzer VA, Good JJ, Archibald FM. 1967. Lipid composition of human serum lipoproteins. Biochem J. 104(2):340–352.
- Skisak CM, Furedi-Machacek EM, Schmitt SS, Swanson MS, Vernot EH. 1994. Chronic and initiation/promotion skin bioassays of petroleum refinery streams. Environ Health Perspect. 102(1):82–87.
- Smith JH, Bird MG, Lewis SC, Freeman JJ, Hogan GK, Scala RA. 1995. Subchronic feeding study of four white mineral oils in dogs and rats. Drug Chem Toxicol. 18(1):83–103.
- Smith JH, Mallett AK, Priston RA, Brantom PG, Worrell NR, Sexsmith C, Simpson BJ. 1996. Ninety-day feeding study in Fischer-344 rats of highly refined petroleum-derived food-grade white oils and waxes. Toxicol Pathol. 24(2):214–230.
- Smith WE, Sunderland DA, Sugiura K. 1951. Experimental analysis of the carcinogenic activity of certain petroleum products. AMA Arch Ind Hyg Occup Med. 4(4):299–314.
- Sothmann D, Südkamp K. 2017. Aufreinigung von Mineralöl für den Einsatz in kosmetischen Mitteln und Arzneimitteln. BfR-Forum Verbraucherschutz Mineralöle; 7/8. Dezember 2017; Berlin [Refining of mineral oil for use in cosmetic products and pharmaceuticals. 17th BfR consumer protection forum "Mineral oil at the focus of consumer health protection"; 7–8 December 2017; Berlin. German. [Accessed 2019 Dec 1]. https://www.bfr.bund.de/cm/343/aufreinigung-von-mineraloel-fuer-den-einsatz-in-kosmetischen-mitteln-und-arzneimitteln.pdf
- Spickard A, Hirschmann JV. 1994. Exogenous lipoid pneumonia. Arch Intern Med. 154(6):686–692.
- Stamatas GN, de Sterke J, Hauser M, von Stetten O, van der Pol A. 2008. Lipid uptake and skin occlusion following topical application of oils on adult and infant skin. J Dermatol Sci. 50(2):135–142.
- Stavric B, Klassen R. 1994. Dietary effects on the uptake of benzo[a]pyrene. Food Chem Toxicol. 32(8):727–734.
- Strakosch EA. 1943. Studies on ointments. I. Penetration of various ointment bases. J Pharmacol Exp Ther. 78(1):65–71.
- Stryker WA. 1941. Absorption of liquid petrolatum (“Mineral oil”) from the intestine. Arch Pathol. 31:670–692.
- Stula EF, Kwon BK. 1978. Pulmonary pathology from inhalation of a complex mineral oil mist in dogs, rats, mice and gerbils. Am Ind Hyg Assoc J. 39(5):393–399.
- Surh YJ, Blomquist JC, Liem A, Miller JA. 1990. Metabolic activation of 9-hydroxymethyl-10-methylanthracene and 1-hydroxymethylpyrene to electrophilic, mutagenic and tumorigenic sulfuric acid esters by rat hepatic sulfotransferase activity. Carcinogenesis. 11(9):1451–1460.
- Suzuki M, Asaba K, Komatsu H, Mochizuka M. 1978. Autoradiographic study on percutaneous absorption of several oils useful for cosmetics. J Soc Cosmet Chem. 29(5):265–282.
- Thiel A, Braun W, Cary MG, Engelhardt JA, Goodman DG, Hall WC, Romeike A, Ward JM. 2013. Calcium lignosulphonate: re-evaluation of relevant endpoints to re-confirm validity and NOAEL of a 90-day feeding study in rats. Regul Toxicol Pharmacol. 66(3):286–299.
- Thomas LW. 1962. The chemical composition of adipose tissue of man and mice. Exp Physiol. 47:179–188.
- Trevaskis NL, Charman WN, Porter CJ. 2008. Lipid-based delivery systems and intestinal lymphatic drug transport: a mechanistic update. Adv Drug Deliv Rev. 60(6):702–716.
- Trevaskis NL, Kaminskas LM, Porter CJ. 2015. From sewer to saviour – Targeting the lymphatic system to promote drug exposure and activity. Nat Rev Drug Discov. 14(11):781–803.
- Trimmer GW, Freeman JJ, Priston RA, Urbanus J. 2004. Results of chronic dietary toxicity studies of high viscosity (P70H and P100H) white mineral oils in Fischer 344 rats. Toxicol Pathol. 32(4):439–447.
- Trivalle C, Profit P, Bonnet B, Manchon ND, Ducastelle T, Hemet J, Bercoff E, Bourreille J. 1991. [Liver lipogranulomas with long-term fever caused by paraffin oil]. Gastroenterol Clin Biol. 15(6-7):551–553. French.
- Tulliez J, Bories G, Boudene C, Fevrier C. 1975. [Hydrocarbons of the spiruline algae: nature, metabolism of heptadecane by rats and swine]. Ann Nutr Aliment. 29(6):563–572.
- Tulliez J, Bories G, Peleran JC. 1975. [Effect of prolonged ingestion of paraffin oil in the pig: Selective retention, interference with cholesterol metabolism]. Comptes Rendus de L'Académie Des Sciences Paris. 280:2261–2264. French.
- Tulliez J, Bories G. 1975a. [Metabolism of paraffinic and naphthalenic hydrocarbons in higher animals. I. Retention of paraffins (normal, cyclo and branched) in rats]. Ann Nutr Aliment. 29(3):201–211.
- Tulliez JE, Bories GF. 1975b. [Metabolism of paraffinic and naphthalenic hydrocarbons in higher animals. II. Accumulation and mobilization in rats]. Ann Nutr Aliment. 29(3):213–221.
- Tulliez J, Peleran JC. 1977a. Glycine conjugation: a metabolic pathway of n-alkyl substituted mono-cycloparaffins. FEBS Lett. 78(2):300–302.
- Tulliez J, Peleran JC. 1977b. [Oxidation of a naphthenic hydrocarbon, dodecylcyclohexane, in the rat]. FEBS Lett. 75(1-2):120–122. French.
- Tulliez JE, Bories GF. 1978. Metabolism of a n-paraffin, heptadecane, in rats. Lipids. 13(2):110–115.
- Tulliez JE, Bories GF. 1979. Metabolism of naphthenic hydrocarbons. Utilization of a monocyclic paraffin, dodecylcyclohexane, by rat. Lipids. 14(3):292–297.
- Tulliez JE, Durand EF, Peleran JC. 1981. Mitochondrial hydroxylation of the cyclohexane ring as a result of beta-oxidation blockade of a cyclohexyl substituted fatty acid. Lipids. 16(12):888–892.
- Tulliez J. 1986. [New approaches to the fate and effects of dietary and medicinal mineral oils]. Méd Nutr. 22:302–309. French.
- UBA. 2015. REACH compliance: data availability of REACH registration. Part 1: screening of chemicals >1000 tpa. Dessau-Roßlau: Federal Environment Agency.
- UN. 2003. Plan of implementation of the world summit on sustainable development. [Accessed 2019 Dec 1]. http://www.un.org/esa/sustdev/documents/WSSD_POI_PD/English/WSSD_PlanImpl.pdf.
- Vachha SM, Kummerow FA, Perkins EG. 1967. Effect of mineral oil ingestion on growth and liver lipid composition in the rat. Proc Soc Exp Biol Med. 126(1):258–263.
- van den Bogaard EH, Bergboer JG, Vonk-Bergers M, van Vlijmen-Willems IM, Hato SV, van der Valk PG, Schroder JM, Joosten I, Zeeuwen PL, Schalkwijk J. 2013. Coal tar induces AHR-dependent skin barrier repair in atopic dermatitis. J Clin Invest. 123(2):917–927.
- van den Bogaard EH, Podolsky MA, Smits JP, Cui X, John C, Gowda K, Desai D, Amin SG, Schalkwijk J, Perdew GH, Glick AB. 2015. Genetic and pharmacological analysis identifies a physiological role for the AHR in epidermal differentiation. J Invest Dermatol. 135(5):1320–1328.
- van Heugten AJ, Versluijs-Helder M, Vromans H. 2017. Elucidation of the variability in consistency of pharmacopoeia quality petrolatum. Drug Dev Ind Pharm. 43(4):595–599.
- VanRooij JGM, De Roos JHC, Bodelier‐Bade MM, Jongeneelen FJ. 1993. Absorption of polycyclic aromatic hydrocarbons through human skin: differences between anatomical sites and individuals. J Toxicol Env Health. 38(4):355–368.
- Vanrooij JGM, Vinke E, De Lange J, Bruijnzeel PLB, Bodelier-Bade MM, Noordhoek J, Jongeneelen FJ. 1995. Dermal absorption of polycyclic aromatic hydrocarbons in the blood-perfused pig ear. J Appl Toxicol. 15(3):193–200.
- Vollmer A, Biedermann M, Grundbock F, Ingenhoff JE, Biedermann-Brem S, Altkofer W, Grob K. 2011. Migration of mineral oil from printed paperboard into dry foods: survey of the German market. Eur Food Res Technol. 232(1):175–182.
- Vost A, Maclean N. 1984. Hydrocarbon transport in chylomicrons and high-density lipoproteins in rat. Lipids. 19(6):423–435.
- Wagner WD, Wright PG, Stokinger HE. 1964. Inhalation toxicology of oil mists. I. Chronic effects of white mineral oil. Am Ind Hyg Assoc J. 25(2):158–168.
- Walborg EF, Jr., DiGiovanni J, Conti CJ, et al. 1998. Short-term biomarkers of tumor promotion in mouse skin treated with petroleum middle distillates. Toxicol Sci. 45(2):137–145.
- Wanless IR, Geddie WR. 1985. Mineral oil lipogranulomata in liver and spleen. A study of 465 autopsies. Arch Pathol Lab Med. 109(3):283–286.
- Wang T, Si HZ, Chen PP, Zhang KJ, Yao XJ. 2008. QSAR models for the dermal penetration of polycyclic aromatic hydrocarbons based on Gene Expression Programming. QSAR Combinat Sci. 27(7):913–921.
- Wislocki PG, Fiorentini KM, Fu PP, et al. 1982. Tumor-initiating ability of the twelve monomethylbenz[a]anthracenes. Carcinogenesis. 3(2):215–217.
- Wheeler LA, Saperstein MD, Lowe NJ. 1981. Mutagenicity of urine from psoriatic patients undergoing treatment with coal tar and ultraviolet light. J Invest Dermatol. 77(2):181–185.
- Willard-Mack CL. 2006. Normal structure, function, and histology of lymph nodes. Toxicol Pathol. 34(5):409–424.
- Withey JR, Law FC, Endrenyi L. 1993. Percutaneous uptake, distribution, and excretion of pyrene in rats. J Toxicol Environ Health. 40(4):601–612.
- Zesch A, Bauer E. 1985. Quantitative Aspekte zur perkutanen Aufnahme von Wollwachsalkoholen (Cetylalkohol) und Paraffinen (Octadecan) aus verschiedenen Salbengrundlagen [Quantitative aspects of the percutaneous uptake of wool wax alcohols (cetyl alcohol) and paraffins (octadecane) from different ointment bases]. Dermatosen. 33(1):15–20. German.
- Zhu H, Bodenheimer HC, Jr., Clain DJ, Min AD, Theise ND. 2010. Hepatic lipogranulomas in patients with chronic liver disease: association with hepatitis C and fatty liver disease. World J Gastroenterol. 16(40):5065–5069.
Appendix 1: Literature search for dermal absorption of MOSH
The starting point for the literature search was the review of Petry et al. (Citation2017) on the dermal penetration of mineral oils and waxes used in cosmetic applications. 14 references on the dermal absorption of MOSH, including individual saturated hydrocarbons as model compounds for MOSH, were identified from this review. Of the 14 references that were screened for inclusion, three were excluded because the test substances (e.g. jet fuel) were not representative for MOSH. The remaining 11 references were found suitable for inclusion in the chapter on dermal absorption of MOSH.
A systematic literature search was subsequently performed in the Science Citation Index Expanded database of Web of Science for the time span from 1945 to 2018. The search was conducted with the advanced search option using the following set of queries:
This search in Web of Science returned 355 records, of which 343 were excluded after examination of the title and/or abstract. Of the remaining 12 full-text records, three references were excluded due to the lack of quantitative information on dermal absorption. The remaining nine hits comprised the review of Petry et al. (Citation2017) and six references, which were already identified for inclusion from Petry et al. (Citation2017), and two new references for inclusion. An additional article (in German) was identified for inclusion from the reference list of a retrieved article. To sum up, 13 unique articles (11 experimental papers, 2 review papers) on dermal absorption of MOSH were finally included.
Appendix Table 1. References retrieved from the review of Petry et al. (Citation2017).
Appendix Table 2. References retrieved from literature search in Web of Science.
Appendix Table 3. Additionally included reference, identified from the reference list of a retrieved article.
Appendix 2: Literature search for ADME, tissue levels, and histopathological effects of MOSH upon oral exposure
The starting point for the literature search was the comprehensive scientific opinion of EFSA (Citation2012) on mineral oil hydrocarbons in food. 53 references on the absorption (upon oral exposure), distribution, metabolism, and excretion (ADME) of MOSH, including individual saturated hydrocarbons as model compounds for MOSH, as well as on the tissue levels and associated histopathological effects of MOSH (in animals and humans) were identified from this opinion. Of the 53 references that were screened for inclusion, seven were excluded because of inaccessible study information, low information content (abstract), unnatural (intraperitoneal) route of administration, or ingestion of vegetable food containing hydrocarbons of plant origin. The remaining 46 references were found suitable for inclusion.
A systematic literature search was subsequently performed in the Science Citation Index Expanded database of Web of Science for the time span from 1945 to 2018. The search was conducted with the advanced search option using the following set of queries:
This search in Web of Science returned 425 records, of which 382 were excluded after examination of the title and/or abstract. Of the remaining 43 records, seven references were excluded because of being precedent abstract publications of later published (and already included) full-papers, or non-usable information (single-case study with no information on the target tissues in focus, non-target species with presence in tissue of saturated hydrocarbons of mainly plant origin, different study focus, pulmonary effects caused mineral oil aspiration, topic related to the “toxic oil syndrome”). The remaining 36 hits comprised 18 references, which were already identified for inclusion from the EFSA (Citation2012) opinion. The systematic literature search identified 18 new references, among which the paper of Boogaard et al. (Citation2012) containing the results of the two inaccessible study reports cited by EFSA, and the paper of Smith et al. (Citation1995) relating to the precedent abstract publication cited by EFSA. 11 additional articles related to the ADME of MOSH were identified for inclusion from reference lists of retrieved papers, from the search for authors constantly contributing to the field (“Tulliez J*”, “Popovic M”), and among the EFSA Supporting Publications. To sum up, 75 unique articles on the ADME, tissue levels, and histopathological effects of MOSH upon oral exposure were finally included.
Appendix Table 4. References retrieved from the EFSA (Citation2012) opinion.
Appendix Table 5. References retrieved from literature search in Web of Science.
Appendix Table 6. Additionally included references which were identified from the reference list of retrieved articles, from the search for authors constantly contributing to the field (“Tulliez J*”, “Popovic M”), and among the EFSA Supporting Publications.
Appendix 3: Literature search for gastrointestinal absorption of MOAH and PAHs
The starting point for the literature search was the comprehensive scientific opinion of EFSA (Citation2012) on mineral oil hydrocarbons in food. Six references on the gastrointestinal absorption of PAHs (including simple methylated compounds) were identified from this opinion. All six references turned out to be suitable for inclusion.
A systematic literature search was subsequently performed in the Science Citation Index Expanded database of Web of Science for the time span from 1945 to 2018. The search was conducted with the advanced search option using the following set of queries:
This search in Web of Science returned 42 records, of which 36 were excluded after examination of the title and/or abstract. Of the remaining 6 records, five were included, and all of these represented newly identified references. Two additional articles were identified from the reference list of a retrieved paper. Also additionally included was a relevant book chapter.
Appendix Table 7. References retrieved from the EFSA (Citation2012) opinion.
Appendix Table 8. References retrieved from literature search in Web of Science.
Appendix Table 9. Additionally included references which were identified from the reference list of a retrieved article. Additionally included was a relevant book chapter.
Appendix 4: Literature search for dermal absorption of MOAH and PAHs
A systematic literature search was performed in the Science Citation Index Expanded database of Web of Science for the time span from 1945 to 2018. The search was conducted with the advanced search option using the following set of queries:
This search in Web of Science returned 316 records, of which 302 were excluded after examination of the title and/or abstract. Of the remaining 14 records, 10 references with relevant information on the dermal absorption of PAHs were included. The remaining four references were not included for the sake of conciseness. Five additional references with extra information on the skin metabolism of PAHs were included.
Appendix Table 10. References retrieved from literature search in Web of Science.
Appendix Table 11. Additionally included references with extra information on skin metabolism.
Appendix 5: Literature search for (dermal) carcinogenicity of PAHs and MOAH
The starting point for the literature search was the comprehensive scientific opinion of EFSA (Citation2012) on mineral oil hydrocarbons in food. 21 references on the carcinogenicity (with focus on dermal carcinogenicity) of PAHs (including alkylated compounds) and mineral oils were identified from this opinion. 12 references were not considered for the sake of conciseness.
A systematic literature search was subsequently performed in the Science Citation Index Expanded database of Web of Science for the time span from 1945 to 2018. The search was conducted with the advanced search option using the following set of queries:
This search in Web of Science returned 638 records, of which 614 were excluded after examination of the title and/or abstract. Of the remaining 24 records, six were included, and all of these represented newly identified references. The remaining 18 references were not included for the sake of conciseness. Additional references with extra information on structure-carcinogenicity relationships for alkylated and partially hydrogenated PAHs as well as with relevant special information on the mouse skin painting assay and related assays (Mobil PAC Method), and on major categories of petroleum-derived substances were included. Also included were relevent publications from CONCAWE, EFSA, European Union, Institute of Petroleum, IARC, and JECFA as well as two references to relevant expert workshop presentations.
Figure 1. Simplified refinery diagram showing the principal processing steps leading to mineral oils and waxes. Foodgrade and pharmaceutical grade qualities are shown bold. The IP 346 method as a gatekeeper and qualifier for refining efficiency is indicated. *Dewaxing not necessary for naphthenic base oils and white oils manufacture. Adapted from Concawe (Citation2017).
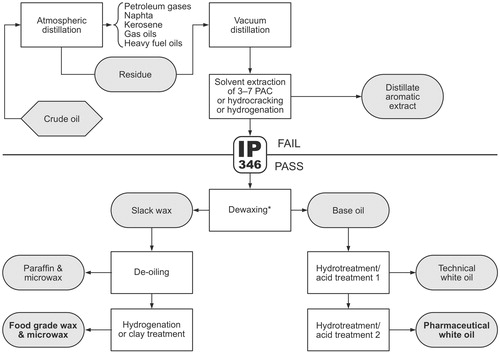
Figure 2. GC × GC–FID chromatogram of a complex mixture of mineral oils to which internal standards and the 16 polycyclic aromatic hydrocarbons selected by the U.S. Environmental Protection Agency (EPA-PAHs) were added. In the first dimension of the GC × GC, a moderately polar stationary phase was used, and a non-polar phase in the second dimension. Both MOSH and MOAH are visible. The solid line interconnects the centers of the signals representing the n-alkanes (for some, the carbon numbers are indicated); between them are little branched iso-alkanes. Numerous multibranched hydrocarbons (incl. pristane and phytane) and cycloalkanes (incl. n-alkyl cyclopentanes and -hexanes, four-ring stearanes and five-ring hopanes) are prominent. The grayish background is formed by a cloud of unresolved, highly isomerized components, most of which being naphthenes. MOAH appear as parallel bands of given number of aromatic rings. The (non-alkylated) PAH (mainly EPA) are located at the bottom of the chromatogram (dotted line). Internal standards in italics: Cycy: cyclohexyl cyclohexane; Cho: cholestane; MNs: 1- and 2-methylnaphthalene; Tbb: tri-tertbutylbenzene; Per: perylene; DEHB: di(2-ethylhexyl)benzene; 18B: n-octadecylbenzene. (Figure taken from Biedermann et al. (Citation2017), © 4694221061510).
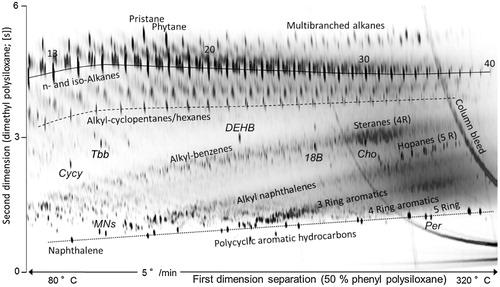
Figure 3. Penetration profiles of MOSH into the horny layer of the skin. (A) Distribution of radioactivity in stratum corneum, as determined using tape strips at 30 and 100 min after dermal application of 14C-labeled n-octadecane in petrolatum to human skin. (B) MOSH concentration in relation to stratum corneum depth, as measured by confocal Raman microscopy at 60 min after dermal application of petrolatum or paraffin oil to the skin of the inner forearm of human volunteers. Data were compiled from Zesch and Bauer (Citation1985) (A) and Choe et al. (Citation2015) (B).
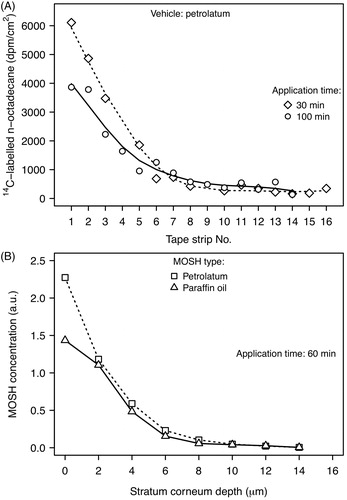
Figure 4. Intestinal absorption of model compounds of MOSH in rats in relation to dose and carbon number. Distinct colors are used to distinguish the data from different studies. (A) Dose dependency of absorption of shorter-chain n-alkanes (n-C17, n-C18) and dodecylcyclohexane (C18). The graph labels have an index reference number (in bold) linking to study details described below and provide information on carbon number, administration procedure (d: diet, g: gavage), and the use co-administered absorption-enhancing agents (e: synthetic emulsifier, p: peanut oil). (B) Absorption of linear, branched, and cyclic alkanes in relation to the number of carbon atoms. Branched alkanes comprised 2,2,4,4,6,8,8-heptamethylnonane (C16), pristane (C19), and phytane (C20). The graph labels contain the index reference number (in bold) and provide information on the applied dose (mg/kg bw per day), administration procedure, and the use of absorption-enhancing agents such as triolein (t). Experimental information for A and B: 1 – single dose of n-octadecane (A) or of an equal-weight mixture of 3–4 unsaturated and saturated aliphatic hydrocarbons, mixed with a branched C30 alkane (squalene) given to male CD rats (B) (Albro and Fishbein Citation1970a); 2 – single dose given to male albino rats (Popovic et al. Citation1973); 3 – single dose given to Wistar rats (Tulliez and Bories Citation1975a, Citation1978); 4 – single dose given to male Wistar rats (Tulliez and Bories Citation1979); 5 – single dose given to rats (Savary and Constantin Citation1967); 6 – single dose given to male Wistar rats (Le Bon et al. Citation1988); 7 – administration of 10 mg/kg bw per day of plant wax paraffins containing ∼90% n-C29 given to male Osborne-Mendel rats over a 5-day period.
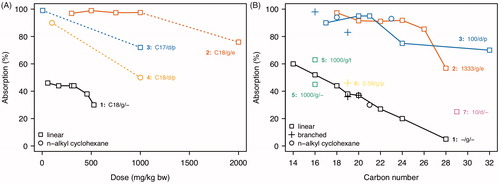
Figure 5. Metabolic pathways of n-alkanes (A), cycloalkanes (B), and branched alkanes (C) in mammals.
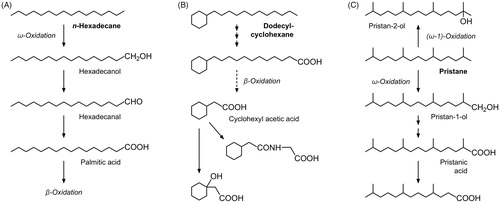
Figure 6. MOSH concentration in the liver of female F-344 rats in relation to administered mineral oil/paraffin wax type and dietary concentration. Data were compiled from Smith et al. (Citation1996) (A), McKee et al. (Citation2012) (B), and Barp et al. (Citation2017b) (C). The mineral oil/paraffin wax types and average carbon number ranges are indicated. Data in (A) show the concentrations after a 90-day treatment (white filled symbols) as means + standard deviation as well as the corresponding concentrations following a post-exposure period of 28 days (gray-filled circles) and 85 days (gray-filled rectangles). Mineral oil nomenclature: N: naphthenic (crude oil source); P: paraffinic; A: acid-treated; H: hydro-treated (catalytic hydrogenation); number (7, 10, 15, 25, 70, 100): approximate viscosity (mm2/s) at 40 °C. Paraffin wax nomenclature: LMPW: low melting point wax; IMPW: intermediate melting point wax; HMPW: high melting point wax. Abbreviations adopted from Barp et al. (Citation2017b): S-C25: presumably a light liquid paraffin oil (Ph. Eur. Paraffinum perliquidum), L-C25: presumably a liquid paraffin oil (Ph. Eur. Paraffinum liquidum), L-C25W: a 1:1 (w/w) mixture of L-C25 and a paraffin wax (W) (see ).
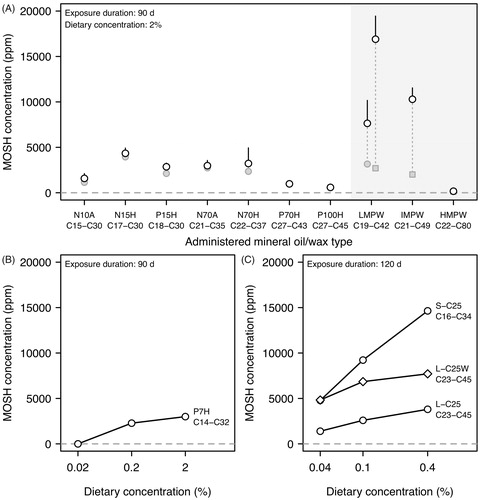
Figure 7. MOSH concentration in the liver of rats in relation to administered mineral oil type, dietary concentration, sex, and strain. Data were compiled from Firriolo et al. (Citation1995) (A), Baldwin et al. (Citation1992) (B), and Griffis et al. (Citation2010) (C). Data are given as means ± standard deviation. Mineral oil nomenclature: OTWO: oleumtreated white oil; HTWO: hydro-treated white oil. For other abbreviations, see legend of .
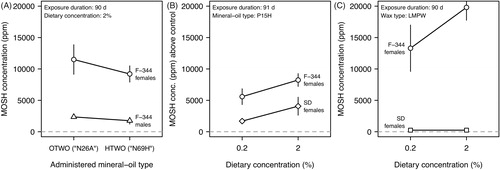
Figure 8. MOSH concentration in the liver of female F-344 rats in relation to exposure and recovery duration. (A) Data (means ± standard deviation) were compiled from a 2-year study with dietary exposure to P70H or P100H (Trimmer et al. Citation2004). Included are data from control animals, and from the recovery group which received the treated diet for 12 months and then a control diet for the following 12 months. For mineral oil type abbreviation, see legend of . (B) Data of a 120-day feeding study in which animals received different doses of a mineral oil mixture (Barp et al. Citation2017a). MOSH concentration is on a log10-transformed scale. Data for the recovery groups and the control animals are included.
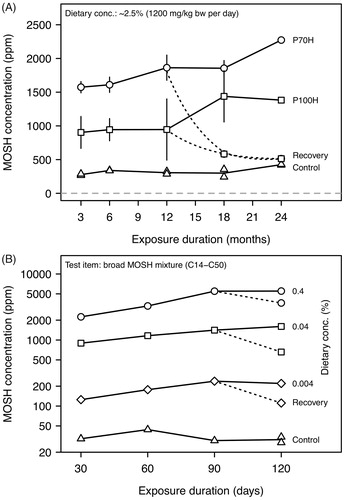
Figure 9. Retention of MOSH in tissues of female F-344 rats in relation to carbon number. (A) Illustration of the retention function (= transfer function) which translates the molecular mass distribution of MOSH in food (“input signal”) into a given tissue distribution (“output signal”). The symbol k is a normalization constant, the index i refers to the carbon number of n-alkanes. (B) Normalized retention functions for the MOSH distribution in liver, spleen, and adipose tissue. The retention functions were calculated from tissue chromatograms of a recovery group (90-day exposure plus 30-day recovery) receiving to diet containing 0.004% of a broad MOSH mixture. (Figure adapted from Barp et al. (Citation2017a), © 4622010703947).
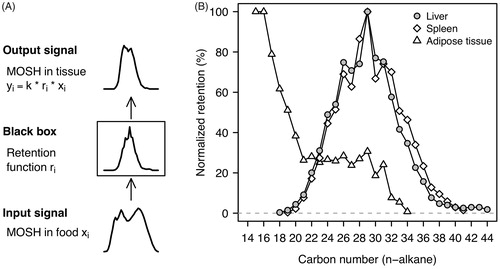
Figure 10. Concentration and molecular mass distribution of MOSH in MLN, fat tissue, liver, and spleen of autopsy samples. (A) Box-percentile plots (Harrell Citation2019) of the MOSH concentrations in the different tissues. Data were extracted from Barp et al. (Citation2014). Shown are the distributional characteristics comprising the 5th, 12.5th, 25th, 37.5th, 50th, 87.5th, and 95th percentiles. Filled circles represent the geometric means. Individual values are shown by gray-coloured tick marks. In addition, the number of subjects (n) is given. (B–E) LC-GC-FID chromatograms showing the approximate molecular mass distribution of MOSH from MLN, fat tissue, liver, and spleen, collected from the same subject. Numbers indicate the carbon number of n-alkanes. In addition, the MOSH concentration (ppm) is given. (Chromatograms adapted from Barp et al. (Citation2014), © 4622010018723).
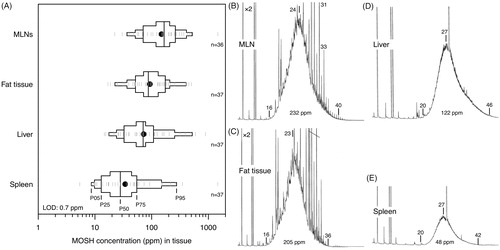
Figure 11. Box-percentile plots of the MOSH concentration in subcutaneous fat and milk fat of breast-feeding women. Data were extracted from Concin et al. (Citation2008). For further details, see legend to .
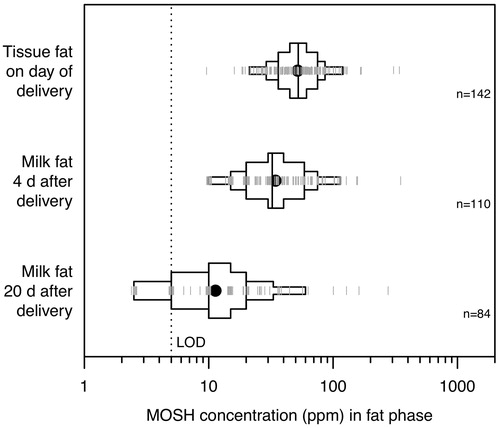
Figure 12. Summary plot for the incidence and severity of granulomatous changes in the mesenteric lymph nodes (MLNs) and liver of rats and dogs after subchronic exposure to highly refined mineral oils and waxes. A Cleveland dot plot (Sarkar Citation2008) was used to visualize the dietary concentrations of the tested mineral oil/wax materials. The data of the control groups (C) are included. The different symbols relate to females (circles), males (diamonds), and both sexes (rectangles). The coloring of the symbols according to the color key on top visualizes the incidence and severity of granulomatous changes. Depending on the study (see first-author names and year at the right margin), different scales were used to classify or quantify effect magnitude: Three-level Incidence Severity Rating (ISR), Incidence (%), Incidence Severity Score (ISS), and granulomas density. The ISS represents a mean score which, for example, was calculated in Griffis et al. (Citation2010) as 100 × ∑(relative incidence × severity rating), where severity was rated as none, minimal, moderate or moderately-severe (score of 0, 1, 2, 3 or 4, respectively) (Griffis et al. Citation2010). Depicted granuloma densities represent the medians of the treatment groups; individual density values ranged up to ∼800 granulomas per cm2. Data were compiled from Baldwin et al. (Citation1992), Firriolo et al. (Citation1995), Smith et al. (Citation1995, Citation1996), Scotter et al. (Citation2003), Griffis et al. (Citation2010), McKee et al. (Citation2012), and Cravedi et al. (Citation2017). For the nomenclature of mineral oils and waxes, see legend of . The exposure duration was ∼90 days in all studies except in Cravedi et al. (Citation2017) where animals were exposed for 120 days.
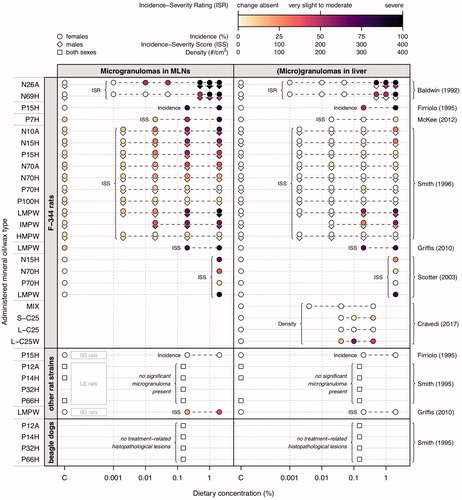
Figure 13. Histological grading of oil droplet lesions in human autopsy material of lymph nodes, liver, and spleen. Left: Stacked bar chart showing the proportion of histological grades in relation to the estimated MOSH content in hepatic lymph nodes. The MOSH content, originally reported as percent of the total lipid content (Boitnott and Margolis Citation1966b), was estimated by assuming the total lipid content to be 5% of the tissue wet weight (Boitnott and Margolis Citation1970). Values within the bars indicate the number of cases. Right: Violin and density scatter plot showing the distribution of MOSH concentration values in tissue conditional on the histological grading of the lesions in spleen and hepatic portal triads. Sample sizes (n) are indicated. Data were compiled from Boitnott and Margolis (Citation1966b, Citation1970).
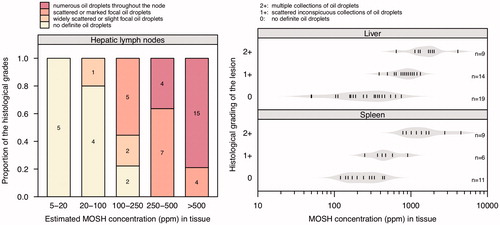
Figure 14. Correlation between skin cancer incidence (%) in CF1 (A) and C3H (B) mice and the DMSO extractable content (%) of dermally applied mineral oil samples. Symbol types indicate the different petroleum substance categories and blends thereof. Data were taken from Concawe (Citation1994) and were fitted by a three-parameter log-logistic model with the upper asymptote fixed to 100% (Ritz et al. Citation2015) (solid curves). The dashed horizontal lines and associated numbers indicate the lower asymptotes of the fits, providing estimates of the background tumor level in CF1 and C3H mice. The threshold for carcinogenicity classification at 3% (w/w) DMSO extractable material is indicated by a dotted line.
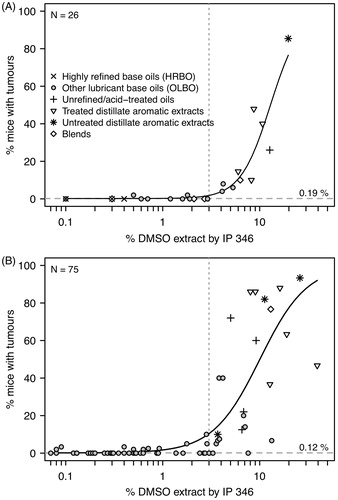
Appendix Table 12. References retrieved from the EFSA (Citation2012) opinion.
Appendix Table 13. References retrieved from literature search in Web of Science.
Appendix Table 14. Additionally included references.