Abstract
An Acute Reference Dose (ARfD) of 1 µg of delta-9-tetrahydrocannabinol (THC) per kilogram (kg) of body weight (bw) per day was recommended by the European Food Safety Authority (EFSA) for its assessment of possible acute health risks from the intake of industrial hemp food products. The scientific basis for this opinion, such as their choice of a Point of Departure for identification of the Lowest Observed Adverse Effect Level (LOAEL) for THC on the central nervous system, and the seeming absence of an experimental No Observed Adverse Effect Level (NOAEL), is critically reviewed. Moreover, the risk assessment for an ARfD derivation for THC is then reconsidered. In contrast to the EFSA Scientific Opinion of 2015, a higher LOAEL is presently identified from pharmacokinetic and pharmacodynamic studies, and forensic data, in representative cohorts of healthy humans after oral administrations of low THC doses. A NOAEL for THC is derived through this combination of results, demonstrating a threshold for impairment of psychomotor function only after intake of an oral THC bolus beyond 2.5 mg for the average healthy adult. This 2.5 mg dose produces mean THC blood serum levels of <2 ng/mL, as well as do two doses when taken daily within a time interval of ≥6 h. The forensic threshold of THC that is correlated with the impairment of psychomotor function is known to be between 2 and 5 ng/mL in blood serum for adults. For an appropriately spaced intake of 2 × 2.5 mg THC per day, an adult can therefore be regarded as being at the NOAEL. Applying a default uncertainty factor of 10 for intraspecies variability to a NOAEL of 2 × 2.5 mg (over ≥6 hours) for THC, yields a “daily dose of no concern” or a “tolerable upper intake level” of 0.50 mg, corresponding to 7 µg/kg bw. Starting with a NOAEL of only 2.5 mg, consumed as a single bolus, the lowest possible daily ARfD of THC would therefore be 0.25 mg, or 3.5 µg/kg bw for healthy adults, as the absolutely most conservative estimate. Other justifiable estimates have ranged up to 14 µg/kg bw per day.
1. Introduction
Modern industrial hemp (Cannabis sativa L.) varieties with a total (−)-trans-delta-9-tetrahydrocannabinol (THC) content not exceeding 0.3% (Williams Citation2020) are legal as a crop plant in the European Union, as listed in the common catalogue of agricultural plants (EU Commission Citation2020). This useful plant genus has been a traditional Eurasian resource for centuries to millennia (Abel Citation1980; Russo Citation2007), and aerial parts of the plant, except for the stalk and stems, but predominantly the seed, have been consumed as food. In the Western pre-industrial era, hempseed oil was one of the most frequently consumed vegetable oils (Callaway and Pate Citation2009), and in late 1920s Russia, this chlorophyllous dark green oil comprised 12.5% of the all edible oil production (Kaufmann and Juschkewitsch Citation1930), often used by those who could not afford butter. In the modern era, European producers have been selling hempseed and its oil since the early 1990s. The 1996 record of overall industrial hemp food use was measured in a survey (Nova-Institut Citation1997) requested by the European Commission in 1997, which confirmed that multiple tons of industrial hemp foods were already present on the market at that time.Footnote1
Industrial hempseed is particularly rich in high-quality, easily digestible proteins (Callaway Citation2004) and its nutritionally beneficial oil is composed primarily of essential fatty acids (Callaway and Pate Citation2009; Galasso et al. Citation2016). A shift in consumer trends towards healthy diets has led to a strong increase of industrial hemp food product demand, which has resulted in the significant development of production and processing supply chains in Europe and North America.
Phytocannabinoids are natural components of the aerial plant parts (i.e. stems, leaves, flowers, and seed) and can be regarded as contaminants only if their levels in food exceed the maximum daily intake recommended by the European Food Safety Authority (EFSA Citation2015) as a “Health Based Guidance Value” (HBGV), or the statutory limit set for food or feed. Non-psychoactive (−)-trans-cannabidiol (CBD; CAS-Reg. No. 13956-29-1) is the primary phytocannabinoid of industrial hemp (), present in amounts not exceeding a few percent by dry weight, about 20-fold that of THC (), the much more (and qualitatively different) psychoactive compound. Therefore, derived foods also contain traces of THC (also abbreviated as delta-9-THC, Δ9-THC or Δ9-THC; CAS Reg. No. 1972-08-3) and other phytocannabinoids, these remaining as faint constituents even after the most careful of cleaning processes have been applied.
Figure 1. (a) Molecular structure of CBD and (b) dibenzopyran numbering system usually employed with THC.
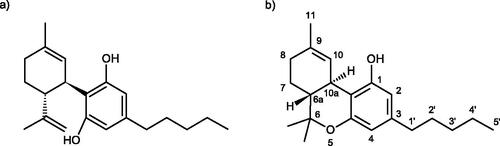
For analytical purposes, a “total-THC” concept has usually been applied to both the plant and its derived foods or feed, which is the sum of both THC and tetrahydrocannabinol-2-carboxylic acid (THCA)Footnote2. In order to obtain correct results and to differentiate between THC and THCA, each of these must be measured by liquid chromatographic methods, because gas chromatography (without prior derivatization of the phytocannabinoid carboxylic acids) will convert THCA to THC as an analytical artefact ().
This is an important point because, like the analogous cannabidiol-2-carboxylic acid (CBDA), THCA is the only phytocannabinoid actually produced biogenetically by the Cannabis plant, and these acidic compounds comprise the vast majority (>95%) of phytocannabinoids found within any analysis of fresh plant specimens (Abd-Elsalam et al. Citation2019). THCA is metabolized in the body to various hydroxylated derivatives, without being significantly decarboxylated to THC (Wohlfarth Citation2012). Unlike THC, THCA does not show any cannabinoid receptor binding affinity (Roth Citation2014) and is, therefore, not psychoactive. For this reason, it would not be reasonable to establish legally binding THC limits on a combined THC and THCA content in industrial hemp foods that are not intended to be baked. Guidance has been proposed (EIHA Citation2017) on “total-THC” values, for practical reasons, but these could be acceptable by the industry only on the grounds of comparability with historical values, easier analytical measurement, and possible decarboxylation of the corresponding acid form upon prolonged exposure to elevated temperatures. However, that conversion does not achieve nearly a molar mass balance, as much of the THCA and THC is lost due to polymerization and oxidation, with minor amounts of cannabinol (CBN) and dihydrocannabinol being produced (see e.g. Dussy et al. Citation2005). In addition, THC (but not THCA) evaporates rapidly, most noticeably at a threshold beyond approximately 150 °Celsius (at atmospheric pressure), and complete elimination of THCA may not be possible, short of unrealistic temperature conditions (EIHA Citation2016).
At the EU level, the HBGV for THC intake from food, recommended by the EFSA, is based on an overly narrow consideration of selected studies, and is unnecessarily strict. Indeed, the guidance value for oral THC intake recommended by the EFSA, upon which the European Council will most probably base its decision on THC limits in food, deserves reconsideration and revision.
2. Materials and methods
Potential studies were systematically searched and identified by the first author (B.B.). The data were collected by the first author (B.B.) and reviewed by the co-author (D.W.P.). The study selections were then jointly conducted by both authors. A review of the published literature was conducted using the PubMed database and a systematic internet search, complete to April 2021. In particular, the websites of various public health authorities (i.e. European Union, European member states, United Kingdom, Canada, USA, Australia and New Zealand, and Switzerland) were searched for the keywords THC and/or delta-9-THC and/or tetrahydrocannabinol and/or dronabinol in conjunction with the following attributes: oral, psychotropic, psychomotor, CNS, effect(s), dose–response, pharmacokinetics, pharmacodynamics, blood (level), plasma, serum, DRiving Under the Influence of Drugs (DRUID), Point of Departure (POD), NOAEL, LOAEL, uncertainty factor, safety margin, (Acute) Reference Dose, and risk assessment. To be included, studies had to either describe pharmacokinetic (PK) measurements, disclosing the main PK parameters, or to report adverse effects, in particular central nervous system (CNS) effects in humans, and to examine oral administration, although they could also include other routes of administration. Single-dose and multi-dose studies were considered. Only article abstracts which met the criteria were selected. Animal studies were usually excluded because of abundant human study data. Studies on the effects of oral administration of THC-containing substances combined with CBD were included only if either PK data or CNS effects were described.
3. Critical reviews of the European Food Safety Authority (EFSA) and German Federal Institute for Risk Assessment (BfR) derivations of lowest observed adverse effect levels (LOAEL) and no observed adverse effect levels (NOAEL) for THC as the basis for a health-based guidance value (HBGV)
3.1. Reference doses
“Reference doses” (RfDs) or HBGVs are compound-specific (i.e. a unique RfD is determined for each substance evaluated). Often, separate acute and chronic RfDs are determined for the same substance. RfDs are specific to dietary exposure.
The “Acute Reference Dose” (ARfD) is defined by the FAO/WHO Joint Expert Committee on Food Additives (JECFA) as follows: ‘‘The ARfD of a chemical is an estimate of the amount a substance in food and/or drinking water, normally expressed on a body weight basis, that can be ingested in a period of 24 h or less without appreciable health risk to the consumer on the basis of all known facts at the time of the evaluation’’ (WHO Citation2017, p. 22). In consideration of the ARfD for THC in foods, it will be important to determine what may be an “appreciable health risk”, and what is not. The EFSA ARfD for THC is presently set at the extraordinarily low level of 0.001 mg/kg of body weight (bw). This benchmark is particularly striking if compared to the HBGVs of other countries, such as those of Switzerland, at 0.007 mg/kg bw (Zoller et al. Citation2000) or Australia and New Zealand, at 0.006 mg/kg bw (FSANZ Citation2012).
Initial RfDs are usually derived from animal studies. In such studies, animals are dosed with varying amounts of the substance in question, and the largest dose is identified at which no effects are observed. This dose level is called the “No Observable Effect Level” (NOEL). To account for the fact that humans may be more or less sensitive than a test animal, a 10-fold uncertainty factor is usually applied to the NOEL. This uncertainty factor is called the "Interspecies Uncertainty Factor" (UFInter). An additional 10-fold uncertainty factor, the "Intraspecies Uncertainty Factor" (UFIntra), is usually applied to account for the fact that some humans may be substantially more sensitive to the effects of a substance than others. Additional uncertainty factors may also be applied.
The often used term “adverse effect” is defined by the OECD (Citation2003) as follows: “Change in the morphology, physiology, growth, development, reproduction or life span of an organism, system, or (sub) population that results in an impairment of functional capacity, an impairment of the capacity to compensate for additional stress, or an increase in susceptibility to other influences.”
Frequently, a “No Observed Adverse Effect Level” (NOAEL) value is used in place of a NOEL. If adverse effects are observed at all dose levels tested, then the smallest dose tested, i.e. the "Lowest Observed Adverse Effect Level" (LOAEL), is used to calculate the RfD. An additional uncertainty factor is usually applied in these cases, because the NOAEL, by definition, would be lower than the LOAEL, had it been observed. If human studies are used to determine the RfD, then the UFInter can be reduced to 1, but generally the 10-fold UFIntra is retained as the default factor, should there not exist substance-specific data on human toxicokinetics and toxicodynamics. However, if such data already exist on adverse effects, in particular from the clinical trial phases required within the pharmaceutical approval process, then these data should be used for replacing the uncertainty default factor by a factor based on measured kinetic and dynamic parameters of the studied substance (Dorne and Renwick Citation2005).
An EPA review (EPA Citation2002, p. 4–43) on the RfD process states: “…the 10-fold intraspecies factor appears to be sufficient in most cases, and chemical-specific factors often indicate a requirement for less than a 10-fold factor.”
Additionally, a Point of Departure (POD), as shown in , is very important in the process of derivation for an RfD. This is defined as the point on a pharmacological or toxicological dose-response curve established from experimental data, or observational data, generally corresponding to the LOAEL or the NOAEL, or both (Little Pro Citation2017). It marks the beginning of an extrapolation to the toxicological RfD. Some uncertainty for this value may exist for THC relative to the influence of CBD/CBDA and other phytocannabinoids within a whole plant extract matrix.
Figure 3. Model dose–response curve (linear ordinate, nonlinear abscissa) and points of departure (POD).
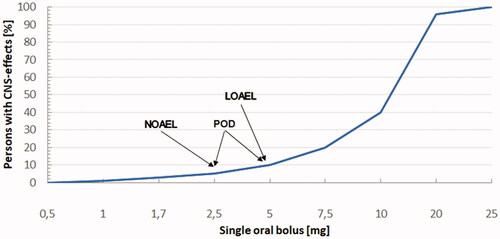
Besides basing the RfD on the LOAEL or NOAEL, there also exists the possibility of using the so-called “Benchmark Dose” (BMD) approach. This approach should use enough measuring points to demonstrate a certain effect, in order to see a correlation from the dose–response data (EFSA Sci Com Citation2009, Citation2017), where a certain point on this curve is taken as the POD. Because the POD is a point on the dose–response correlation, within a set of measurements under consistent conditions, there is more certainty about this value than for a single LOAEL or NOAEL. Therefore, the BMD approach is recommended for the competent authorities to consider, if the available data allow for it.
In the case of THC, the EFSA Scientific Committee used the LOAEL approach, obviously because consistent dose–response correlations on the most critical effects (i.e. psychoactive, psychomotor) in the range of very low THC doses (lower than therapeutic) were regarded as insufficient or missing.
3.2. LOAEL and acute reference dose for THC
3.2.1. Derivation of LOAEL for THC by the BfR
Discussion in publications on scientific and legal aspects for the consumption of “adverse” THC doses in food reveals a strong discrepancy between the oral doses of THC recognized in many studies as psychoactive and the LOAEL of 2.5 mg/d for THC used today by some authorities to derive an RfD for the substance. The minimal psychoactive dose for THC is at least a 5 mg oral bolus (see Sections 3.2.2 and 3.2.3), but a normal dose is usually considered to be 10 to 15 mg, or sometimes even 20 mg for adults as reported, for example, by Grotenhermen and Leson (Citation2002, p. 38).
The LOAEL for THC at which a CNS effect has been observed in animal or human studies, as used by the German “Federal Institute for Risk Assessment” (Bundesinstitut für Risikobewertung, or BfR) and the EFSA for the derivation of an HBGV (EFSA CONTAM Panel Citation2015), is assumed to be 2.5 mg of THC per day for an adult. This is an extremely low value which, however, cannot be derived from most relevant scientific studies with healthy people, for example, those performed for the “New Drug Applications” (NDAs) of the Syndros® and Marinol® THC products (see Section 4.5.1). Thus, it must be determined if, in the EFSA Scientific Opinion, the POD for the derivation of the ARfD of THC has been carefully considered on the basis of all known facts at the time of the evaluation.
The BfR guidance valueFootnote3 (as a recommendation) for a 1–2 µg maximum daily intake of “total-THC” of per kg bw was based on only one observational study (not double-blind, no control group) by the “German Research Foundation” (Deutsche Forschungsgemeinschaft, or DFG) involving 31 AIDS patients (DFG Citation1997–2004), 10 of whom reportedly exhibited “undesired CNS effects” at a dose of 2.5 mg/d. These effects were attributed solely to their treatment with THC. In a report on THC levels in food in 2018 (BfR Citation2018, p. 14), and without consideration of any new evidence, the BfR simply confirmed that it recognized the ARfD of 1 µg THC/kg bw recommended by the EFSA CONTAM Panel (Citation2015) as a precautionary value. However, the LOAEL of 2.5 mg THC/d and the subsequent ARfD, which were derived by the EFSA from a small number of studies, are worthy of discussion in depth.
3.2.2. Main (and related) studies for deriving a point of departure (POD) from the EFSA scientific opinion
The EFSA CONTAM Panel (Citation2015) relied mainly upon three large clinical trials in HIV patients (Struwe et al. Citation1993; Beal et al. Citation1995, Citation1997) to determine a POD for the LOAEL, and one additional very small study on 11 subjects (Ballard and de Wit Citation2011). Of these, only the last study specifically and systematically investigated the psychoactive effects of THC, whereas in the clinical trials, any adverse CNS side-effects were documented within the usual system for recording all kinds of adverse effects. This opens the question as to whether or not a mildly pleasant and transient feeling of psychoactivity should actually be considered to be an “adverse effect.”
The EFSA CONTAM Panel (Citation2015, p. 64) summarizes the outcome of its investigation as follows: "In these studies adverse effects associated with oral Δ9-THC doses of 2.5 mg twice a day (5 mg Δ9-THC/day) or a single dose of 2.5 mg Δ9-THC/day were reported (see Section 7.5.1.3). Therefore, 2.5 mg Δ9-THC/person per day may be regarded as a lowest observed adverse effect level (LOAEL). Indications in fact sheets for medical uses are in accordance with this.”
The EFSA CONTAM Panel claims in its formal “Scientific Opinion” (EFSA Citation2015, p. 64) that adverse THC effects occurred not only at 5 mg/d, but also at 2.5 mg/d (Struwe et al. Citation1993; Beal et al. Citation1995, Citation1997). However, a careful examination of the original publications does not allow for such a conclusion. provides an overview of the relevant parameters of these studies. In the trials, adverse effects are only documented for the entire patient collective, and were not attributed to a patient collective at the reduced dosage of 2.5 mg/d. Significant side-effects were not detected for most patients. Higher doses of dronabinolFootnote4 (5–10 mg/d) were actually administered to most patients to achieve the desired effect (Beal et al. Citation1995): "The main increase in appetite to end point for evaluable patients receiving dronabinol was 38% over baseline, compared with 8% for those receiving placebo (p = 0.015). Interestingly, in the 11 patients [of 72 evaluable patients] who, due to side effects, decreased their dose of dronabinol to 2.5 mg once daily, the appetite increase was the same as for those taking medication twice daily.” From this data, one could easily conclude that a mild and transient form of pleasant “adverse effect” might have actually provided some measure of a therapeutic benefit.
Table 1. Parameters of clinical trials with dronabinol (Struwe et al. Citation1993; Beal et al. Citation1995, Citation1997).
The same study showed that a single dose of 2.5 mg THC was well tolerated by all patients without side-effects (as opposed to a single dose of 5 mg): "Dronabinol was well tolerated. Most side effects reported were central nervous system disturbances that are commonly associated with cannabinoids. In most cases, they were not severe enough to warrant intervention. There was no significant difference between both treatment groups in the patient dropout rates due to adverse reactions. Six Dronabinol versus three placebo recipients discontinued therapy due to any adverse effect thought to be possibly or probably related to treatment. These numbers are small and attest to the safety and tolerance of treatment. Most patients who required dose reduction were able to tolerate the half-dose (one 2.5-mg capsule in the evening). Of 17 patients who received a reduced dose, 11 were evaluable for efficacy and showed a similar appetite increase…"
Moreover, the reliability of this study is restricted because "commercially available or investigational antiretrovirals were allowed" meaning that the assignment of any observed side-effects to any specific drug (here THC) becomes questionable.
A review by Plasse et al. (Citation1991) on clinical experiences with dronabinol states "the lowest rates of termination for side effects were in the 2.5 mg … groups, only 1 patient in each group" [8–9 patients per group, i.e. a maximum of 12% of patients] had side-effects and further states that: "Many of the side effects reported may have been related to underlying disease or concomitant medications rather than to dronabinol.", adding that "Drowsiness and sedation are often related to other concomitant medications and the stress of disease and therapy together." Left unmentioned was the fact that many antiretrovirals (e.g. protease inhibitors) are metabolized by the same P450 enzymes as THC, so interactions cannot be excluded.
In addition, the long-term study by Beal et al. (Citation1997) does not show that these undesirable side-effects would have occurred at a dose of 2.5 mg/d (again, patients received between 2.5 and 20 mg THC/d). It should be noted that 90% of the patients received 5 mg/d of THC (i.e. dronabinol), while only 10% received 2.5 mg/d, and 19% of the patients even increased their dose to 7.5 mg/d: "Ninety percent of the patients enrolled in the study received an initial daily dronabinol dose of 2.5 mg orally twice daily, and the remaining 10% received 2.5 mg orally once daily in the evening. Thirty-eight percent of patients modified their dronabinol dose during the study. One-half of these patients increased their dose, most commonly to 2.5 mg during the day and 5 mg with supper or at bedtime (7.5 mg daily total). Two patients increased their dronabinol dose to 5 mg twice daily and another from 5 mg twice daily to 5 mg twice daily plus 10 mg at night (20 mg daily total). The other one-half decreased their dose from 2.5 mg twice daily to 2.5 mg at bedtime."
As to the effects of these doses, it is reported: "As expected, adverse events [AEs] were primarily related to the central nervous system … [series of symptoms] …and occurred in 35 of 93 patients (38%) enrolled in the study." In the publication, nothing more specific is reported on side-effects, and these have not been broken down into individual dosing groups.
In Gorter et al. (Citation1992), the THC dose was reduced from 7.5 mg/d to 5 mg/d as a minimum dose (not 2.5 mg) to minimize side-effects: "Patients were treated with dronabinol (Marinol®, Roxane Laboratories, Columbus, Ohio, USA) at a starting dose of 2.5 mg orally three times daily. Doses, which were adjusted to minimize side-effects while stimulating appetite, ranged from 2.5 mg twice daily to 5 mg four times daily. Most patients were continuing treatment at the time of this analysis; the median duration of treatment was ≥12 weeks (range, >4 to >20 weeks). All patients tolerated therapy well. They were able to adjust the medication dose to avoid unwanted THC side-effects, such as sedation and persistent euphoria. No patient discontinued therapy because of side-effects.”
The placebo-controlled study by Struwe et al. (Citation1993), with only 12 patients, does not show CNS side-effects at a dose of 2.5 mg THC/d for all patients, or even for most. In this study, only one patient had to reduce dosing to 2.5 mg of THC twice daily (i.e. 5 mg/d), and for one other patient it was reduced to 2.5 mg once per day only. These two patients “did not tolerate Dronabinol, even following dosage reduction, and withdrew during the first period [5 weeks] because of mood altering effects and sedation.” All the other patients tolerated 5 mg twice daily (i.e. 10 mg/d) in order to achieve the desired effect. However, one must be cautious in generalizing these side-effects from only two HIV patients, as explained above.
It is generally recognized, from the toxicological literature, that it is not advisable to base RfDs on data from sick individuals. For example, Lehmann and Fitzhugh (Citation1954) had stated: “… a sick individual may be as much as 10 times more susceptible to toxic substances than an individual in good health …”. The attribution of any observed side-effects on the CNS to THC becomes even more questionable, nowadays, because it is known that HIV-associated neurocognitive disorder (HAND) affects nearly half of all HIV-infected individuals. “Synaptodendritic damage correlates with neurocognitive decline in HAND, and many studies have demonstrated that HIV-induced neuronal injury results from excitotoxic and inflammatory mechanisms" (Wu et al. Citation2019). For a decade, it has been known that HIV-related co-morbidities, including symptoms of brain dysfunction, remain common even in treated HIV-positive individuals (Heaton et al. Citation2010; Nightingale et al. Citation2014; Saloner and Cysique Citation2017). Thus, HIV-symptoms (e.g. cognitive impairment) of the patients in the cited dronabinol trials could have been misinterpreted as THC side-effects or may have been disproportionately exacerbated by the THC dose.
The study by Ballard and de Wit (Citation2011) has the disadvantage of being conducted on a very small trial group of 11 participants in a cross-over design, which has not yet been reproduced or verified by other studies. An experimental flaw here is that the time-dependence of effects is not shown in detail, including the fact that ethanol dosages were administered 60 min after the THC dose, which may have induced synergistic behavioral and psychomotor effects (Hartman et al. Citation2015), affecting their Digital Symbol Substitution Test results, as recorded at 100 min post-THC dosing. This small trial is the only one with healthy adults used by the EFSA to derive an HBGV that describes very mild effects from THC on subjective well-being and responsiveness at a dose of 2.5mg/day (mg/d) "When given alone, 2.5 mg THC produced modest effects on subjective ratings, measures of cognitive performance, and physiological measures. Although participants did not report feeling any drug effects, THC significantly reduced POMS 'vigor' scale scores and increased sedation …” It may be reasonably questioned if effects on "mood state" or slight sedation are relevant endpoints for deriving an HBGV for THC. Summarizing the result, the authors state: “… these very-low doses of ethanol and THC had only moderate effects on isolated measures…"
As to the EFSA comment on the dose of 2.5 mg/d being the lowest dose for medical uses, this only applies to treatment of the weight loss associated with AIDS-related anorexia and then, only for the more sensitive or elderly patients ingesting a 2.5 mg bid (twice daily) dose, not for the anti-emesis therapy indicated for the nausea and vomiting associated with cancer chemotherapy. In that latter case, a recommended minimum dose is 2.5 mg/m2 of body surface, which corresponds to approximately 4.8 mg/d (FDA Citation2017).Footnote5 The 2.5 mg Marinol® dosage form is useful mainly for intermediate titration of the full 5 or 10 mg dose levels slightly upwards, circumventing the need to administer another whole 5 or 10 mg increment. A moderate effect on appetite stimulation from a dose of 2.5 mg THC/d may not be regarded as an adverse effect, and most probably (see the NDA studies for Marinol® and Syndros® in Section 4.5.1), this marginal effect will disappear at any lower dose, so it may be concluded that a 2.5 mg/day (mg/d) dose is not justified as the POD for a LOAEL.
3.2.3. Other studies (clinical and observational) and reviews to be considered for deriving a POD
By contrast, another study (Gray et al. Citation2008) did not come to the same conclusion as the above work for a dose of 2.5 mg/d. In fact, no effect for THC was found at a dose of 2.5 mg/d, and only at elevated doses of 5 or 10 mg/d were changes in subjective well-being self-reported.
A recent Israeli study by Bar-Sela et al. (Citation2019) found no significant side-effects from a combination of 4.75 mg of THC (including 0.25 mg of CBD), consumed daily for periods ranging from 2 weeks to 6 months for 10 patients in cancer therapy.
Results of many other human studies on THC also do not indicate adverse effects at a dose of 2.5 mg/d, or the studies have been done only at doses of at least 5 mg/d in order to observe at least some significant measurable effects (Petro and Ellenberger Citation1981; Chesher et al. Citation1990; Leson et al. Citation2001; Strasser et al. 2006; Zuurman et al. Citation2009).
For example, Petro and Ellenberger (Citation1981) report: “Side effects of the 5- or 10-mg oral dosage were minimal. One patient reported feeling "high" after 10 mg, and another reported a "high" after placebo. No other patients reported side effects at the relatively low doses we used.”
In the study by Strasser et al. (Citation2006), it is reported in their summary of results that: “Intent-to-treat analysis showed no significant differences between the three arms [cannabis extract, THC, or placebo] for appetite, QOL [quality of life], or cannabinoid-related toxicity.” In this study, the experimental cohort had received 2.5 mg of THC twice daily, and only in the case of adverse effects was this dose reduced for some patients to a single 2.5 mg/d. The rate of AEs with a likely relationship to THC medication was only 3.6%.
The review by Zuurman et al. (Citation2009) includes studies on oral administration of THC, and while not differentiating the tabulated effects by administration route, this study mentioned adverse effects for THC at doses lower than 7 mg/d, but only for pulmonary administration, rather than for oral administration. Obviously, only oral administration is relevant for a toxicological assessment of THC in food, and it should be noted that pulmonary administration produces more immediate and higher (if more transient) THC blood levels.
Grotenhermen et al. (Citation2001) had set up a comprehensive assessment to estimate risks of THC intake to human health. They differentiated between psychomotor and psychoactive effects and conclude the following on the LOAEL and NOAEL for THC. “Acute effects: The lowest observed adverse effect level (LOAEL) for the ingestion of THC, representing a slight impairment in psychomotor functions, is represented by a single dose of 5 mg of oral THC. The NOAEL for psychotropic effects caused by the oral ingestion of THC has been established at 5 mg/day.” The authors determined an Acceptable Daily Intake (ADI) of THC to be 500 µg/d for an average adult with an overall uncertainty factor of 20 because, even at 10 mg of THC per day, there was no cumulative effect recognized.
A “meta-study” from the US by Kruger and Lodder (Citation2018) is a very short communication that does not present any data at all for the derivation of a Total Daily Intake (TDI). The TDI of 1.5 µg THC/kg bw proposed by the authors is neither substantiated nor comprehensible. Furthermore, a meta-analysis cannot logically generate more experimental data on the toxicology of THC than those data generated within the original studies. The parameter "change in heart rate," which is mentioned as important, is not specific for THC, and the study on the sublingual (i.e. enhanced dose delivery) Namisol® THC formulation by Klumpers et al. (Citation2012), cited in this analysis, reports data on minimal dosages of 5 mg (as an acute, single dose) and 6.5 or 8 mg/d THC orally, but not the 2.5 mg/d used as the EFSA POD. Moreover, the Klumpers group commented “All adverse events were of mild to moderate intensity and transitory in nature.” No significant AEs were mentioned for the treatment arm with a dose of 5 mg/d of Namisol®, and AEs were only mentioned for the higher doses.
In addition, a recent study on gender differences in the subjective perception of oral THC in cannabis users (Fogel et al. Citation2017) was also able to identify adverse effects (i.e. mild psychoactivity) only at a dose of 5 mg/d.
The above studies, including larger human clinical trials using THC for therapeutic purposes in patients, have observed adverse effects on the CNS only at a single dose of 5 mg THC, thereby allowing a LOAEL identification of 5 mg THC/d, which corresponds to 71 µg/kg bw (assuming a 70 kg subject, on average).
In general, caution should be exercised when citing details of dose-response relationships from reviews or meta-studies, as certain effects are often not correctly reported for a specific dose. This may be demonstrated with a concrete example. In a review (Badowski Citation2017) of the Nadulski et al. (Citation2005) study, a dose of 2.5 mg THC was reported, but an oral dose of 10 mg/d had actually been used. If one relies only on secondary literature, then one might be tempted to draw erroneous conclusions.
Within an extreme interpretation, the effects for 2.5 mg of pure THC on the CNS could conceivably involve a slight effect on the psyche (i.e. mood alteration) in the very most sensitive individuals. However, these effects are transient and rapidly reversible, and considerably less than the acute effects of drinking a small amount of alcohol, similar to the slight mood alteration derived from consuming a 0.33 L bottle of beer with 5% alcohol content by volume. However, no adverse CNS effects (let alone health impairments) have yet been found to occur reliably below a daily intake of 2.5 mg THC per adult.
The lesson is clear: Supportive evidence for adverse effects caused by THC at an oral dose of only 2.5 mg/d is lacking. In fact, most of the studies cited point to a single THC dose LOAEL of 5 mg per day.
4. A closer look at the absorption, distribution, metabolism, excretion, “toxicity” (ADMET) and pharmacokinetics of low-dose oral THC
The effects of THC, even “low-dose effects,” have been studied in detail with healthy adults in reviews within the NDA process for Marinol® (i.e., dronabinol) as oral soft gelatin capsules which contain pure THC (2.5 mg, 5.0 mg, and 10.0 mg) dissolved in sesame oil, and for the oral Syndros® alcohol-glycol solution of dronabinol (FDA Citation2016). The original NDA for Marinol® had already been submitted to the US Food and Drug Administration (FDA) in 1981 and was approved in 1985 (FDA Citation1985). The recommended initial dose at that time was 5 mg/m2, corresponding to 0.135 mg/kg bw, and assuming a European adult standard body weight of 70 kg, which translates to 9.45 mg. The NDA presents 8 studies, wherein the minimum applied dose of THC to cancer patients was 7.5 mg/day; all other doses were higher. For this reason, none of these studies are suitable for the derivation of an RfD for industrial hemp food consumption, as the lowest study dose exceeds the LOAEL of THC. However, the product monograph for Marinol® (Abbott Citation2011), presents PK data for THC after single doses (2.5 mg, 5 mg, and 10 mg) and multiple doses (2.5, 5, and 10 mg given twice a day) studied in healthy subjects (see Section 4.6).
4.1. Absorption
After oral ingestion of THC, systemic absorption is relatively slow, resulting in maximum blood level concentrations of both the parent drug and its major psychoactive metabolite 11-hydroxy-THC (11-OH-THC) at approximately Hours 0.5–4, subsequently declining over several hours. THC is almost completely absorbed (90–95%) after single oral doses, but due to the combined effects of first-pass hepatic metabolism and high lipid solubility, only 10–20% of the administered dose reaches the systemic circulation (Abbott Citation2011).
Because of this high lipophilicity, a pronounced effect from co-ingestion of fatty foods has been observed in the time required (Tmax) to achieve a maximum plasma concentration (Cmax) of THC, and of 11-OH-THC. Up to a 4 h delay in mean Tmax and a 2.9-fold increase in total exposure, measured as the concentration/time-curve (AUC) ad infinitum, is observed, but the Cmax was not significantly changed (FDA Citation2017). On the contrary, it was lowered by 22% or more. Therefore, the strength of the response (i.e. CNS effects) is expected to be similar or lower, but extended over time, because effects are associated mainly with actual plasma concentrations (i.e. Cmax).
4.2. Distribution
THC is distributed within the organism in three phases. In the first phase, orally administered THC is absorbed into the blood from the digestive tract via the liver and is then rapidly distributed by systemic circulation to all organs and the CNS, with the concentration of THC in the blood constantly falling. In the second phase, THC accumulates in the fatty tissues with poor blood circulation (i.e. depot formation). In the third phase, THC diffuses back from the fatty tissues into the blood, so that the terminal drop in blood THC levels, monitored as the non-psychoactive 11-nor-9-carboxy-THC (THC-9-COOH) metabolite of 11-OH-THC, is slow. THC has a large apparent volume of distribution at approximately 10 L/kg (McGilveray Pharmacon Inc and the University of Ottawa, Ontario Citation2005) because of its lipid solubility. Other studies (Grotenhermen Citation2003, p. 16), based on more advanced analytics, have calculated a smaller steady state volume of distribution at 3.4 L/kg (assuming a 70 kg body weight). The plasma protein binding of dronabinol and its metabolites is approximately 97% (Widman et al. Citation1973).
4.3. Metabolism
After absorption via the gastro-intestinal tract, THC undergoes extensive first-pass hepatic metabolism, primarily by hydroxylation, forming psychoactive 11-OH-THC. Both are present in approximately equal concentrations in plasma after oral administration, illustrating the rapid metabolism of THC, but a diverse array of other metabolites are also generated (see Section 6.1).
4.4. Excretion
The elimination of THC can be described using a two-compartment model (Abbott Citation2011, p. 16), having an initial half-life of about 4 h and a terminal half-life of 25–36 h (for doses of ≤10 mg/d). This is due to the combined aforementioned effects of first-pass hepatic metabolism and the high lipid solubility of THC and its metabolites. After the last oral intake within an average of five days, 80–90% of THC and (more so) its metabolites will have been excreted, more than 65% via feces and 20–25% via urine (Sharma et al. Citation2012).
Possible effects of THC accumulation have to be considered, and the fate of this compound and its main metabolites have been examined in more detail. For example, in a study (Beal et al. Citation1995; Abbott Citation2011, p. 17) of Marinol® involving AIDS patients (doses between 2.5 mg/d and 5 mg/d), urinary cannabinoid/creatinine concentration ratios were studied bi-weekly over a six week period. The urinary cannabinoid/creatinine ratio was closely correlated with dose. No increase in the cannabinoid/creatinine ratio was observed after the first two weeks of treatment, indicating that steady-state cannabinoid levels had been reached. This conclusion is consistent with predictions based on the observed terminal half-life of dronabinol.
Grotenhermen (Citation2001) has also commented on the possible accumulation of THC:
“The accumulation of THC in body tissue represents a source of THC to the plasma even after cessation of THC uptake. The establishment of a dynamic equilibrium between accumulation and remobilization and the slow rediffusion process indicate that corresponding THC levels in plasma will be insufficient to supply THC at rates which could result in or contribute to adverse effects.”
4.5. Toxicity: Dose-dependent effects of THC
4.5.1. Psychoactivity as “toxicity”
THC has suffered a conflation of its inherent CNS effects, which are quite real, with its somatic “toxicity,” which is largely imaginary. In NDA documents (FDA Citation2017), the effects of THC are summarized as follows: “Dronabinol also demonstrates reversible effects on appetite, mood, cognition, memory, and perception. These phenomena appear to be dose-related, increasing in frequency with higher dosages, and subject to great inter-patient variability. After oral administration, dronabinol has an onset of action of approximately 0.5–1 h and peak effect at 2–4 h. Duration of action for psychoactive effects is 4–6 h.” THC concentration drops rapidly within the first 30 min after the maximum blood level is reached, and then drops more slowly towards almost zero over the next 18–24 h. In contrast, the euphoric effect decreases significantly after 1–2 h and is essentially gone after 5–6 h (see also Grotenhermen Citation2003).
The adverse CNS effects of THC have been studied (FDA Citation2016) for the NDA of the Syndros® (i.e. enhanced dose delivery) oral solution of dronabinol and compared with those of Marinol®. Potential treatment-emergent adverse effects were categorized and subjectively evaluated as euphoric mood, abnormal thinking, and hypervigilance, but only the first of these symptoms manifested (). The incidence of euphoric mood was 3.8% after a single dose of 4.25 mg of Syndros® in the healthy and fed trial subjects, and nil for 5 mg of Marinol®. The effect frequency for this symptom was 0.6% for a dose of 5 mg of Syndros® and 1 to 1.9% for 5 mg of Marinol® in fasted subjects. Thus, the recorded psychoactive effects cannot be regarded as significant, as the incidence of adverse effects is extremely low, and the trial group was large enough to give a representative result.
Table 2. Treatment-emergent adverse events frequency (i.e. euphoric mood, abnormal thinking and hypervigilance) from pharmacokinetic studies (FDA Citation2016).
In evaluating these results, it should be noted that the contrasting Syndros® dosage form evoked a slightly greater incidence of euphoria than Marinol® in one trial. Although this may be an anomaly, it could also possibly be that the solvent system of the Syndros® oral solution facilitated more rapid and higher systemic THC levels via a degree of trans-mucosal absorption, in a manner resembling that of the similarly formulated Sativex® oro-mucosal spray product. In contrast, Marinol® is an encapsulated solution of dronabinol in sesame oil, designed for absorption exclusively via the gastrointestinal tract.
In an FDA review (Unimed Pharmaceuticals Inc Citation2004), a summary on the treatment of chemotherapy-induced emesis with Marinol® was evaluated in 454 patients with cancer and demonstrated () that escalating the dose above 7 mg/m2 (corresponding to 12 mg THC/d) increased the frequency (i.e. from 12% to 28%) of “dysphoric” adverse experiences, with no additional antiemetic benefit. However, these results must be viewed with some reservation, because they are derived from patients receiving various (and often multiple) anticancer therapeutics having severe side-effects which may affect the bioavailability of THC, in addition to any effects possibly provided by the illness itself.
Table 3. Dose-dependent response/adverse events frequencies in the treatment of 454 patients with Marinol® for chemotherapy-induced emesis (Unimed Citation2004).
4.5.2. Somatic toxicity
Instead of relying on a few studies for an HBGV derivation, it is necessary for a somatic toxicological evaluation to take into account the entire ensemble of scientific studies on the effects of pure THC. Since the complete molecular structure elucidation of THC by Gaoni and Mechoulam (Citation1964), an intense effort has been made to investigate its properties and effects as the isolated compound within a wide range of human and other animal studies, as well as its interactions with other substances (Mechoulam Citation2005). As a result of these comprehensive studies, THC certainly would have been identified as a substance of significant toxicity had it possessed that trait, but in fact, the antithesis is true (WHO Citation2018).
An ARfD for THC (ingested with food or food supplements) of 1 µg/kg bw was proposed by the EFSA CONTAM Panel in 2015. The derivation of an RfD for chronic intake of THC will not be discussed here, because the EFSA decided that the establishment of a TDI was not necessary. Based on the evaluation of the dose-response relationship in sub-chronic and chronic oral exposure studies with rats, a BMD corresponding to a Benchmark Dose Response of 10% (BMDL10) was found to be 700 times higher than the ARfD. This implies that ensuring an exposure below the ARfD would also protect against possible effects of THC after repeated human exposure. Chronic toxic effects in humans are not often observed clinically with THC ingestion (discounting occasional idiopathic reactions) at normal doses and usual frequencies, and certainly not with micro-doses, let alone incidental trace ingestion. Moreover, such a dramatic difference between ARfD and BMDL10 also suggests a very broad therapeutic index for THC, which is actually greater than 50,000, the acute lethal dose in humans being roughly estimated at amounts greater than 9000 mg/kg bw, extrapolating from toxicity tests with monkeys (Thompson et al. Citation1973). No such fatality has ever been observed, for obvious reasons. Somatic toxicity in rodents can be elicited only upon the administration of a chronic regimen of massive overdose (NIH Citation1996), but evidence for carcinogenicity is non-existent or ambiguous, even under those extreme conditions.
4.6. Pharmacokinetics
The available data on dose-dependent toxicokinetics for THC are quite detailed, including for a low single dose of 2.5 mg/d, and even lower dosages.
A recent comprehensive review (Poyatos et al. Citation2020) provides plenty of dose-dependent PK data (mainly as the Tmax and Cmax for THC and its metabolites) and their correlation with various THC formulations. However, possible dose-related effects from low, and very low, doses of THC do not represent the main focus of the review, and data therein on low-dose (i.e. sub-clinical) PK parameters is sparse.
Administration of a single 2.5 mg dose of dronabinol () in healthy volunteers (Abbott Citation2011) was reported to yield a mean plasma Tmax at 2 h (range: 30 min–4 h) with a mean plasma Cmax of 0.7 ng/mL (range: 0.3–1 ng/mL). A single 5 mg dose resulted in a mean plasma Cmax of 1.8 ng/mL (range: 0.4–3.3 ng/mL), whereas a single 10 mg dose gave a mean plasma THC Cmax of 6.2 ng/mL (range: 3.5–8.9 ng/mL).
Table 4. Summary of Marinol® pharmacokinetic parameters in healthy volunteers (Abbott Citation2011).
Because PK data are available from the Marinol® NDA for single doses of 2.5 mg, 5 mg, and 10 mg of THC/d, and for each of these doses twice per day, these data could be used to determine the uncertainty sub-factor for the toxicokinetic part of UFintra and serve as a check to see if the observed standard deviations (SDs) are covered by the default uncertainty sub-factor for this dosing regimen. Furthermore, a correlation could be established between the dose-dependent Cmax and psychoactive effects from other “DRiving Under the Influence of Drugs” (DRUID) studies on the association between THC blood levels and psychomotor performance. These dosages and blood levels are very much higher than the amounts that could be expected from the daily consumption of foods derived from industrial hemp, though trace detection in “zero tolerance” countries is highly problematic.
The doses within these Marinol® studies have not been normalized to the body mass, or to the body mass index (BMI), of the trial subjects. Such variables are a main factor for intraspecies differences in dose–response correlations. Therefore, the evident “noise” in results probably reflects this unaccounted factor. This is an important point because it has been observed (e.g. Goodwin et al. Citation2006) that the PK parameters of THC, in particular the Cmax and AUC, are highly correlated with body weight. This correlation, however, could be observed only with higher therapeutic doses (>7.5 mg THC/d). It should also be noted that correlating THC-blood levels with effects is notoriously challenging. THC is quickly distributed from blood to other tissues (e.g. fat, brain). Slow back-diffusion leads to overall long detectability of THC and its metabolites. Especially frequent consumption can lead to prolonged detectability long after any effects have ceased.
A single dose of 2.5 mg THC/d is certainly below the NOAEL because no psychoactive effects are reported for this low dose, while the Cmax remained under 0.65 (± 0.3) ng/mL in the blood plasma, which corresponds to 0.42 ng/mL in whole blood or 0.84–0.95 ng/mL in blood serum.Footnote6 Thus, these results are under even the overly stringent 1.0 ng/mL German legal THC blood serum limit (corresponding to around 0.5 ng/mL in whole blood) which is lower than in most other countries for vehicle driving, and is generally recognized to produce absolutely no relevant cannabis effects (Tönnes et al. Citation2016). Even dosages of 4.25 and 5 mg THC/d produced hardly any adverse effect (see the Syndros® and Marinol® NDA studies previously described in Section 4.5.1).
Of course, the Cmax from a 2.5 mg single dose is, therefore, also well under the lower impairment threshold for THC in serum determined by Ramaekers et al. (Citation2006) from performance tests measuring skills related to driving. In this study on smoked cannabis, it was concluded that the lowest threshold for impairment of skill performance is associated with a serum THC concentration of 2 ng/mL: “The proportion of observations showing impairment progressively increased as a function of serum THC in every task. Binomial tests showed an initial and significant shift towards impairment in the critical tracking task for serum THC concentrations between 2 and 5 ng/ml … It is concluded that serum THC concentrations between 2 and 5 ng/ml establish the lower and upper range of a THC limit for impairment”. This threshold should be considered as being independent of the route of administration, as the term “cannabis” is not limited to products intended for smoking. The effects on performance were all correlated to the measured THC content in serum and oral fluid, as well. The level of 11-OH-THC within the serum samples always ran in parallel within the concentration versus time measurements. Also, the sum of THC and 11-OH-THC (thus, the psychoactive substances together) did not provide higher correlations with performance change than THC alone.
This finding can be compared to the results of the studies used for the dronabinol NDA (Abbott Citation2011), wherein the mean Cmax for THC (in plasma) was 1.32 (±0.62) ng/mL after a dose of 5 mg/d administered as 2.5 mg bid, corresponding to approximately 1.8 (±0.9) ng/mL in serum. This suggests that an oral dose higher than 2 × 2.5 mg/d would be required to achieve the lower range of impairment and is consistent with a “lowest LOAEL” of 2 × 2.5 mg of THC, or 5 mg THC/d, which is very near to the NOAEL. It should be noted that the variability of measuring points around the mean value is to be considered later (see below and Section 6), only in the next step of risk assessment (i.e. derivation of the PK part of UFintra).
Furthermore, a 10-year study of 3398 driving fatalities (of which 58 cases had only THC present) in three Australian states found that there was no increased risk of car accidents at whole blood THC concentrations of ≤5 ng/mL (Drummer et al. Citation2004), as reviewed by Grotenhermen et al. (Citation2007), who presented a graph for the correlation between the Drummer Odds Ratio and blood THC level, developed by a polynomial regression. A whole blood THC concentration of 5 ng/mL would be equivalent to a plasma concentration of about 7.5 ng/mL and to a serum concentration of about 11 ng/mL, applying the distribution rates for THC described by Giroud et al. (Citation2001).
The caveat for such comparisons lies in the following. The Ramaekers et al. (Citation2006) smoking study correlated only the THC levels in serum and oral fluid with performance tasks, without taking into account the 11-OH-THC levels, because that parameter did not give a better correlation. Actually, the main reason was that 11-OH-THC comprises only a minor proportion (ca. 10%) of total measured THC in serum after smoking cannabis, compared to the oral ingestion, the latter route of administration yielding a Cmax of 11-OH-THC equal to, or higher than, that of THC (Wall and Perez-Reyes Citation1981). However, if different oral doses correlate well with the degree of observed CNS effects, and these oral doses are clearly correlated with PK parameters for THC only, then a comparison is possible between different studies with different administration routes of THC. Of course, the later onset of effect observed with oral administration (30 min after ingestion, at the earliest) should be considered, as well as the fact that oral bioavailability is only approximately one third that of smoking (Huestis Citation2007).
A more recent review (BASt Citation2012, p. 31) summarized 21 studies with 482 effects, in total, as a meta-analysis on the effects of oral THC administration (dose range 7.5–39 mg). In this analysis, it was shown that the impairment caused by 3.7 ng/mL THC (range 3.1–4.5 ng/mL) in blood serum corresponds to that caused by a blood alcohol concentration (BAC) of 0.05%, the German legal blood alcohol driving limit. In the same study, an additional meta-analysis on the effects of smoking THC on performance led to a comparable result. Here, a total of 78 studies were included within the scope of the meta-analysis, with a total of 888 effects (for doses between 1 and 52 mg) resulting in their very similar determination of 3.8 ng THC/mL being the threshold of impairment, with an added value for measurement error and confidence interval (Hargutt et al. Citation2011).
In contrast to the DRUID studies above, a recent Swiss review concludes that only higher THC levels cause driving impairment, similar to the established Canadian levels. The Swiss “Federal Office of Public Health” (Bundesamt für Gesundheitswesen, or BAG) commissioned a report from the Institute for Forensic Medicine of the University of Basel (Bucher et al. Citation2020) on the correlation of THC blood level thresholds with driving impairment. After a review of the scientific literature on driving under the influence of cannabis, they concluded that THC levels between 3.0 and 4.1 ng/mL in whole blood (6–8 ng/mL in serum) cause an impairment comparable to that of a BAC of 0.05%, and that the risk for car accidents is not significantly increased at THC whole blood levels not exceeding 5 ng/mL.
Notably, in an earlier meta-analysis (Grotenhermen et al. Citation2007), a legal limit for the concentration of THC in serum was proposed that produces the same level of impairment, and possibly accident risk, as a BAC of 0.05% and would be somewhere above 4–5 ng/mL of serum. The correlation between THC serum concentrations and impairment did not depend on the route of administration of cannabis (inhalation vs. oral ingestion). Considering intra- and interspecies variability and analytical errors, the authors conclude on a legally enforceable threshold of 7–8 ng/mL THC in serum for a DRUID offence.
In Canada, as another example, the current regulations have fixed per se limits of 2 ng/mL and 5 ng/mL of THC (in whole blood) for DRUID offences. The 5 ng/mL THC per se limitFootnote7 (presenting an indictable offence) is based upon impairment considerations, while the 2 ng/mL THC per se limit (resulting in a finable offence) is based upon a wide margin for conceivable public safety considerations. Their “Drugs and Driving Committee” recommended the use of distinct, but corresponding, per se limits for blood plasma (Government of Canada Citation2019). Using the distribution factors described by Giroud (Citation2001), the Canadian lower and upper per se limits would correspond to 3 ng/mL and 7.5 ng/mL THC in plasma (Coefficient of Variation [CV] 11%), respectively, or to 4.4 ng/mL and 11 ng/mL THC in serum (CV 22%), respectively.
Serum concentrations after intake of 2.5 mg THC (single dose) and of 2 × 2.5 mg/d are under the Dutch critical limit of 3.0 ng/mL for an influence on driving ability, according to a study from The Netherlands (Mütze Citation2017). At these THC dosages, blood levels also remained under a recent recommendation from the German “Expert Panel on Drink and Drug Driving Limits” (Grenzwertkommission) of 3.0 ng/mL of THC in blood serum (Auwärter et al. Citation2015). This level established their benchmark for discriminating occasional cannabis use from current use while driving, a level which was also construed to differentiate between regular use and recent consumption.
A THC dose of 2.5 mg given twice daily may be regarded as the NOAEL if these single-dose administrations are separated by a time interval of at least 6 h, because each Cmax will have subsided by then. For this total dose (2 × 2.5 mg), plasma Cmax was 1.32 ng/mL with a SD of 0.62 ng/mL, and the time to reach this concentration was found to be 1.00 h as the median (Abbott Laboratories Ltd, Quebec, Canada Citation2011). These Tmax values appeared between Hours 0.50 to 4.00 h (the latter obviously not representing a normal distribution) for both THC and its metabolite 11-OH-THC. The uneven distribution is probably due to repeat phase hepatic recirculation of THC, which varies considerably between individuals and also depends on fed versus fasted status.
The most important parameters that assess the strength of the effects are the Cmax and the area under the plasma AUC. The median AUC found for a 12 h time interval was 2.88 ng*h/mL with a SD of 1.57 ng*h/mL. Obviously, at such low levels and with such a small sample size, the SD is large relative to the AUC. Therefore, for Cmax, the variation is 47% of the mean, which results in an uncertainty sub-factor of about 2.5 for toxicokinetics using the correlations described by Renwick and Lazarus (Citation1998).Footnote8 For the AUC(0–12) the CV is 55% of the mean, resulting in an uncertainty sub-factor of about 2.7 for the toxicokinetics.
Comparing the Cmax plasma THC values for 2 × 2.5 mg/d dosing with serum levels, and applying the factors determined by Giroud et al. (Citation2001), yields a Cmax of approx. 1.9 ng/mL in serum (mean value), which is also under the lower threshold of 2 ng/mL for any impairment as shown by Ramaekers et al. (Citation2006). For 5 mg/d (as a single bolus), the mean serum level would be expected at approximately 2.6 ng/mL, which is still under the 3 ng/ml serum THC limit regarded as critical by some forensic experts and government authorities (as explained previously).
A Phase I study (Ahmed et al. Citation2014) evaluated the PKs of three oral single doses of THC (3 mg, 5 mg, and 6.5 mg, as Namisol®) in 12 healthy older subjects (mean age: 72; range: 65–80 years). This study revealed wide inter-individual variation in the plasma concentrations of THC and 11-OH-THC. For those subjects who reached a Cmax within 2 h, the mean THC concentration was 1.42 ng/mL (range: 0.53–3.48 ng/mL) for the 3 mg dose, 3.15 ng/mL (range: 1.54–6.95 ng/mL) for the 5 mg dose, and 4.57 ng/mL (range: 2.11–8.65 ng/mL) for the 6.5 mg dose. Drowsiness was the main observed AE, but no correlation of incidences to the doses administered could be established. All AEs were mild to moderate in degree.
A subsequent randomized, double-blind, placebo-controlled, cross-over trial (Ahmed et al. Citation2015), which evaluated the PKs of oral THC (as Namisol®) over a 12-week period in 10 older patients with dementia (mean age 77 years), reported the median Tmax was between one and two hours, with the PKs increasing linearly with increasing dose, but again, with wide inter-individual variation. Patients received 0.75 mg THC orally, twice daily (6 hour time interval between these doses), on three consecutive days of the week, followed by 4 days of wash-out, then placebo (same regimen) over the next 6 weeks, and then 1.5 mg THC twice daily (same schedule) over the second 6-week period. The mean Cmax after the first 0.75 mg THC dose was 0.41 ng/mL, and after the first 1.5 mg THC dose it was 1.01 ng/mL (in blood plasma). After the second dose of 0.75 mg THC or 1.5 mg THC, the Cmax was 0.50 and 0.98 ng/mL, respectively. “Psychedelic effects” were evaluated using a “Visual Analog Scale” (VAS). Only 6 of the 98 reported AEs in the study were related to THC. The VAS of feeling “high,” external perception, body-sway with eyes open, and diastolic blood pressure, were not significantly different than control.
As the data from both of these studies are from elderly populations, a group not often considered separately in studies for deriving an RfD, these data should be considered within the UFIntra context. These data show that, even for the elderly, THC doses of 0.75 mg, once or twice/d, give rise to serum levels which remain under 1 ng/mL, doses of 1.5 mg, once or twice per day, remain under 2 ng/mL, as calculated with distribution factors from Giroud et al. (Citation2001), and a single dose of 3 mg just reaches 2 ng/mL. Thus, no adverse psychomotor or psychoactive effects could be expected for these doses.
The results of Cmax measurements for various studies are shown in , together with corresponding SDs from the mean of the ranges, their CVs, and their calculated “Pharmacokinetic Uncertainty Factors” (UFPK) of the UFIntra.
Table 5. Pharmacokinetic parameters of THC, expressed as plasma and equivalent calculated serum levels, their coefficients of variation (CVs) and resulting uncertainty sub-factors for the PK part of overall uncertainty.
Psychoactivity and psychomotor performance versus PK parameters are generally regarded as dose-dependent. However, it is difficult to show a very consistent correlation among many experimental variables, including: pure compound versus natural product mixture; variable dosage form and matrix; sample preparation and applied analysis of whole blood versus plasma versus serum; and various experimental methodologies, including route of administration. In addition, the published data are relatively scarce, in particular, those of the Cmax for THC and 11-OH-THC and their consequent physiological influences. Thus, it is difficult to draw firm conclusions from measured Cmax values on their subjective effects and compare them between studies, if these effects have not been measured within the same study, as the following examples illustrate.
A closed clinical study (randomized, double-blind, placebo-controlled) by Goodwin et al. (Citation2006) on sub-chronic oral administration of various THC doses to experienced cannabis users gives some indications on the fate of low and high THC doses. The doses administered orally in a five day session were: 0.39, 0.47, 7.5, or 14.8 mg THC/d. Plasma concentrations of THC, 11-OH-THC, and (non-psychoactive) THC-9-COOH were determined by gas chromatography-mass spectrometry (GC-MS) with a limit of quantification (LOQ) for the three compounds being 0.5, 0.5, and 1.0 ng/mL, respectively, for 14 samples over time, the last 4 of them in the wash-out period.
Effects of the full dose range administered daily to subjects who self-evaluated were measured via a VAS that included such subjective experience evaluation items as “high”, “stoned”, “impaired/tired” or “confused/clear headed”, but little change in the scores was noted. Likewise, blood pressure, heart rate and respiratory rate remained relatively unchanged during and after the various dosing conditions.
No trial subject samples exhibited THC or 11-OH-THC plasma levels greater than the LOQ during or after the lowest 0.39 mg/d or 0.47 mg/d dosing regimens. The non-psychoactive THC-9-COOH metabolite could be detected at concentrations very near to the LOQ for these two low THC doses, but dropped below the limit of detection (LOD) 2 days after the last dose, on Day 7 of the study period. It is important to note that these very low dose results are representative of possible trace THC exposure from hemp foods, and are very near to the RfD of 7 µg/kg bw tolerance proposed by the hemp industry (EIHA Citation2017).
A more recent study (Pellesi et al. Citation2018) on subjective effects and PKs for low doses of cannabis extracts may shed light on the variability of measured parameters as a function of sample matrix and potential substance interactions. Patients (N = 9) received a single dose of either a decoction or an oil formulation, each containing a suite of measured phytocannabinoids. In the decoction dose, the absolute amounts were THC (1.85 mg), THCA (2.22 mg), CBD (1.93 mg) and CBDA (8.82 mg), and those for the oil formulation dose were THC (2.2 mg), THCA (2.3 mg), CBD (2.4 mg) and CBDA (4.4 mg). The decoction dose led to a Cmax of 1.38 ± 0.75 ng/mL for THC, whereas consumption of the oil formulation dose resulted in a Cmax of 3.29 ± 1.39 ng/mL, both measured in whole blood.
However, these results have to be interpreted with care because the SDs of the analytical measurements were high. For the decoction, the CV for the Cmax was ±54%, and for the oil formulation it was ±42%. No serious AEs were reported after the administration of either oral cannabis preparation. No clinically relevant changes in blood pressure and heart rate were found. Compared to other studies, in particular to those with isolated THC, the observed physiological THC-levels were rather high and should provoke more detailed PK studies on orally consumed cannabis extracts versus pure THC.
These results are in obvious discrepancy to the Cmax levels measured by Vandrey et al. (Citation2017) who reported a mean Cmax of only 1, 3.5, and 3.3 ng/mL in the whole blood of subjects who had consumed cannabis brownies containing 10, 25, or 50 mg of THC, respectively. This corresponds to an ingestion of THC doses between 5- and 25-fold those given in the study cited above.
Further, a recent pilot study (Pichini et al. Citation2020) reported data on one healthy individual treated with two suites of phytocannabinoids, the first dose being in a decoction, followed by a subsequent oil dose trial. The decoction dose contained a combination of THC (0.36 mg), THCA (1.6 mg), CBD (0.42 mg) and CBDA (4.0 mg), with the resulting Cmax for THC in blood serum being 1.0 ng/mL. After a washout period, the subject then received an oil dose containing a mixture of THC (0.95 mg), THCA (1.5 mg), CBD (0.86 mg), and CBDA (2.8 mg). The Cmax for THC in blood serum following this larger oil-based THC was 0.5 ng/mL. In both cases, SDs were given as ±15%. The serum THC level was within an expected range for the oil dose, but that for the decoction seemed disproportionately high, and did not echo the decoction results of the above Pellesi et al. (Citation2018) study.
In a study by Leson et al. (Citation2001), 15 THC-naïve adults were given four different and increasing amounts of THC in a mixture of industrial hempseed and rapeseed oils over four successive 10-day “dosing periods”, with a 3 day washout phase at the end (Days 40–43), thus evaluating THC ingestion in increasing amounts over 40 consecutive days. The increasing “total-THC” (THC + THCA) doses administered were 90, 190, 290, 450 or 600 µg/d (the highest dose given to only three subjects within the fourth period), corresponding to an actual THC (without THCA) range of 74–492 µg/d. No psychoactive side-effects were observed in the volunteers at any dose, a fact which was confirmed by the author (2020 personal communication by Leson; unreferenced, see “Notes”).Footnote9 Evaluations were made on the impact of extended daily ingestion of THC in the hempseed-rapeseed solutions on urine concentrations of its non-psychoactive metabolite THC-9-COOH for each of the four distinct daily doses. Urine samples (taken on Days 1 and 3 after each last ingestion, and on Days 9 and 10 of each 10-day dosing period) were screened by radioimmunoassay (RIA) for total-cannabinoids, and analyzed by GC-MS for THC-9-COOH specifically. For “total-THC” doses up to the 450 µg/d maximum, the total cannabinoid levels in the RIA were all below 20 ng/mL, except for the highest dose on Day 39, which was only 21 ng/mL. Quantification by GC-MS for THC-9-COOH gave levels under the 2.5 ng/mL LOQ until Day 28. Starting a third period thereafter, when the dose was increased to 290 µg/d, levels of 3.6 and 4.4 ng/mL were found for the two last samples within that period. At the end of the 450 µg/kg fourth period, THC-9-COOH values in urine maintained in a similar range, with only one sample showing a level of around 5 ng/mL. On washout Day 3, all samples had cannabinoid levels below the LOQ.
Unfortunately, the levels of THC and 11-OH-THC (versus their major THC-9-COOH metabolite) were not measured separately. This would have been useful because conclusions on acute and recent cannabis (or other THC) consumption can be drawn only with this differentiation. Therefore, a correlation of the analytical data in this trial with potential psychoactive effects is not possible. Another detail of this study deserves attention. The THC doses administered are expressed as “total-THC” (i.e. THC + THCA), with the content of THC in the industrial hempseed-rapeseed oils comprising 82% of the two phytocannabinoids. Therefore, the highest dose of 600 μg/d “total-THC” corresponds to only 492 µg/d of actual THC, as previously mentioned. Overall, the authors of the study conclude: “At daily THC intake rates of up to 0.6 mg, the highest measured THC-COOH concentration in urine of 5.2 ng/mL is well below the 15 ng/mL confirmation cut-off [by GC-MS] used by [US] federal and many private employers.”
Results from the study above are also in general agreement with a very small study by Bosy and Cole (Citation2000), in which 6 human male adults were exposed to single THC doses in industrial hempseed oil over 7 days, ranging from 0.1 to 1.77 mg THC/d. In one trial, 546 µg of THC ingestion daily produced a single positive urine cannabinoid screening result at the US administrative initial screening cut-off level (via RIA) of 50 ng/mL, over 14 days, but caused no positives within another trial. Likewise, GC-MS test results for the former series only once exceeded the THC-9-COOH confirmatory cut-off value of 15 ng/mL, and then only by 1 ng/mL, whereas all results for the latter series were below this threshold, although quantitation varied quite substantially. Possible psychoactive side-effects for this dose range were not mentioned, but are highly unlikely. Any presumed accumulation of THC, as measured by the observed (GC-MS) THC-9-COOH levels steadily increasing over time, was not observed for any dose up to 546 µg THC (corresponding to 9.9 µg/kg bw), but was observed for the high THC dose of 1.77 mg (21.6 µg/kg bw) within the first 6 days.
From the two studies above (Bosy and Cole Citation2000; Leson et al. Citation2001), it may be concluded that an oral daily THC intake between 0.25 mg and 0.50 mg is without substantial accumulation, as analyzed by THC-9-COOH in blood plasma or urine. Within this context, an oral THC maximum dose of approximately 0.49 mg/d ingested via industrial hemp food was recommended (EIHA Citation2017) to provide an ample margin of safety from presumed adverse health effects and to represent the ARfD for THC (see Section 7).
Because THC in industrial hemp food is only a minor or trace constituent and CBD (as CBDA) is the dominant phytocannabinoid, potential CBD/THC-interactions are relevant, in particular those affecting changes of THC PKs and pharmacodynamics. Agurell et al. (Citation1981) found that co-administration of 40 mg of oral CBD did not notably change the PKs of oral THC (20 mg) as indicated by average plasma THC levels. Their single dose PK study of – inter alia – a combination of 10.8 mg of THC with 10 mg of CBD in healthy volunteers reported this formulation to be safe and tolerable. No adverse event was considered serious, and no clinically significant abnormalities in vital signs, ECG recordings, physical findings or safety lab tests were noted.
Interestingly, PK data on the interaction of large CBD doses with co-administered very low oral doses of THC () may be found in the Human Abuse Potential (HAP) study for Epidiolex® (US Public Health Service, Dept of Health & Human Services (HHS) 2018, p. 12). This pharmaceutical CBD product, which contains traces of THC (<0.1%), is administered in relatively high doses (approx. 10–20 mg/kg bw per day) to achieve the desired antiepileptic effect as an adjunctive therapy. The measured respective Cmax plasma concentrations of those THC traces were within the expected range, upon administration of the two lower therapeutic doses of 750 mg CBD (0.3–0.45 mg THC), and 1500 mg CBD (0.45–0.9 mg THC), when compared to plasma THC levels after ingestion of those corresponding dronabinol amounts alone. However, the supratherapeutic dose of 4500 mg CBD (1.35–2.70 mg THC), gave a maximum THC plasma level of only 0.48 ng/mL, approximately half the expected level, which suggests nonlinear PKs. The FDA concludes: “Thus, it is unlikely that THC contributed to the slight positive responses on some of the subjective measures or contributed to the euphoric AE responses reported following the higher doses of CBD.” From these results, it may be concluded that high CBD/THC ratios might decrease THC plasma levels, although the opposite could have been expected (see Section 6.1) due to the inhibitory effect of CBD on the major THC metabolizing enzyme CYP2C9 (Qian et al. Citation2019). Such enzyme inhibition would attenuate the metabolic conversion of THC to 11-OH-THC, the latter of which is equally as psychoactive as THC (Grotenhermen Citation2003), if also being an enzymatic step closer to inactivation.
5. Implied human effects of THC micro-dosing
It remains to be determined whether or not sub-psychoactive doses of THC have beneficial effects in humans. Sarne et al. (Citation2018) described a study designed to test whether ultra-low THC doses could reverse age-dependent cognitive impairments in old mice. They reported the brain being protected from neuro-inflammation-induced damage “suggest[ing] that extremely low doses of THC that are devoid of any psychotropic effect and do not induce desensitization may provide a safe and effective treatment for cognitive decline in ageing humans.”
A previous report by Bilkei-Gorzo et al. (Citation2017) had revealed that very low doses of THC in older mice restored learning and memory, and social recognition abilities, to levels seen in healthy young mice. The THC micro-dosing treatment also enhanced molecular processes involved in cell plasticity, synapse formation, and cell signaling, and positively affected genes involved in extending lifespan and improving cognition, particularly a gene thought to be protective against Alzheimer’s disease. Some improvements lasted several weeks after treatment. “Cannabis preparations and THC … have an excellent safety record and do not produce adverse side effects when administered at a low dose to older individuals,” the authors conclude, adding that “chronic low-dose treatment with THC or cannabis extracts could be a potential strategy to slow down or even to reverse cognitive decline in the elderly.”
6. Application of uncertainty factors in deriving an HBGV from the LOAEL/NOAEL for trace THC limits in food versus presently legal recreational drugs
Currently, in Europe, including most of the EU member states, there are no legally binding daily maximum levels for THC traces in foods or food supplements. So far, only non-binding recommendations exist. However, an EU Commission stakeholder consultation on these limits began in 2020 and is expected to conclude with an agreement amongst the EU member states in 2022.
In 1997, Germany was the first country to recommend a maximum daily intake of 1–2 µg of “total-THC” per kg bw from industrial hemp foods as part of a precautionary consumer health protection effort. In their risk analysis, an overall safety factor of 20–40 was applied to a LOAEL of 2.5 mg/d, which is highly questionable because it was based on only a small clinical study (not double-blind, no control group) involving severely ill HIV patients, and the pertinent publication was not even named (DFG Citation1997–Citation2004).
On the basis of this maximum daily intake, recommendations on guideline values for THC in food were issued, but only for Germany. Although noted as preliminary, this maximum daily intake recommendation has never been revised. Despite a commission of the “German Research Foundation” (Deutsche Forschungsgemeinschaft, or DFG) stating in 1999 that it urgently requested the determination of the NOEL for the psychomotor effects of orally administered THC in humans (DFG Citation1997–Citation2004), none was soon forthcoming. Eventually, the BfR (Citation2021) published a notice in which it recommended basing the toxicological assessment of industrial hemp-containing food on the EFSA ARfD for THC only, thus throwing into disarray the previous German guideline values for this compound in foods.
Since 1997, numerous studies have published reliable data on the effects of THC. However, in 2015, the EFSA (CONTAM Panel) still concluded in its risk assessment: "The identified LOAEL of 0.036 mg delta-9-THC/kg bw per day is considered to be relevant for sensitive individuals, since it is the lowest daily dose administered in clinical studies for the therapeutical use of delta-9-THC." However, in the derivation of an HBGV, it is not justified to apply the standard uncertainty factor of 10 for intraspecies variability because this factor should be applied only if an adverse effect was observed as common for most of the healthy participants in a representative human study, and if it was of statistical significance, neither of which was the case in the cited studies.
The EFSA line of argument for justification of uncertainty factors is the following: “The CONTAM Panel concluded that an UF of 3 is sufficient to allow for extrapolation from the LOAEL to a NOAEL considering that the LOAEL is based on effects of low or moderate severity. An additional UF of 10 is required for interindividual differences because although the data on adverse effects are partly derived from studies in patients with severe diseases, data on adverse effects in infants and children are not available and there are interindividual differences in metabolism (CYP2C polymorphism).”
Indeed, lower uncertainty factors for extrapolation to the NOAEL are to be applied if the observed effects (LOAEL) are mild and transient. Grotenhermen et al. (Citation2001) state: “When using a LOAEL for determination of the sub-threshold dose, the severity of the effect at the LOAEL level is to be considered. Mild effects that may represent an adverse impact will require lower UFs.” This statement is also supported by Dourson et al. (Citation1996). Research by Lewis et al. (Citation1990) showed that the most likely value of the true risk in the extrapolation of LOAEL to NOAEL is an uncertainty factor of 2, meaning that for THC the estimated NOAEL would be 2.5 mg/d. Although this does not differ much from the extrapolation factor of 3 applied by the EFSA, it strongly influences the resulting RfD because of its multiplication with the uncertainty factor for intraspecies variability (for which the EFSA had used the default value of 10).
It must be emphasized that the purpose of the 10-fold default factor is the “extrapolation of an average human NOAEL to a sensitive human NOAEL” (Dourson et al. Citation1996). In other words, the POD for a NOAEL should be a dataset obtained from average, healthy individuals, and is extrapolated by an uncertainty factor to obtain an RfD that also considers the sensitive part of the population. The inter-human (i.e. intraspecies) uncertainty factor “is intended to account for the variation in sensitivity among humans and is thought to be composed of toxicokinetic and toxicodynamic uncertainties.” This also implies that “if sufficient data on sensitive individuals exist, the subthreshold dose [or RfD] can be estimated directly, i.e. without the need of an uncertainty factor.”
Dourson et al. (Citation1996) state that a default value of 10 for inter-human variability appears to be adequate and protective when starting from a median response, or by inference, from a NOAEL obtained from an average group of humans. “However, when NOAELs are available in a known sensitive human subpopulation, or if human toxicokinetics or toxicodynamics are known with some certainty, this default value of 10 should be adjusted or replaced accordingly.” These statements clearly allow the conclusion that a default factor of 10 for intraspecies variability is too high if the NOAEL or LOAEL is already derived from results on the most sensitive individuals.
It has been proposed that one may regard the default factor of 10 for intraspecies variability to be composed of two sub-factors, consisting of 3.16 each (equivalent to 2√10) for the toxicokinetic and the toxicodynamic aspects (Renwick and Lazarus Citation1998). Compared to other substances (e.g. caffeine, alcohol, morphine), the content of which must be limited within food or beverages, the approach by governing authorities who have fixated on these extremely high safety factors for THC is justifiably very questionable (Iffland et al. Citation2016; EIHA Citation2017).
Coffee and alcohol, for example, are both variably addictive drugs that are known to have significant effects on the CNS in socially tolerated quantities. With coffee, which contains caffeine, daily consumption would have to be strictly regulated (see Section 6.1.2) if an analogous approach to the EFSA assumptions on THC were to be used. In the case of alcohol, an analogous limitation would consequently lead to a restriction on the marketability of dairy products such as yoghurt, and of fruit juices and the like, because alcohol is unavoidably produced as a minor by-product of fermentation. A thorough risk assessment of alcohol would also lead to a ban on the marketing of all larger containers, and more concentrated forms, of alcoholic beverages. However, in contrast to the properties of alcohol as a cellular poison, THC has distinct neuroprotective qualities and both THC and CBD have been shown to prevent hydroperoxide-induced oxidative damage not only in a chemical system (i.e. the Fenton reaction), but also in neuronal cultures (Hampson et al. Citation1998).
Of course, one would also need to ban acetaldehyde in food, which is also the primary human metabolite of ethanol and a known carcinogen (DFG Citation2020).Footnote10 Acetaldehyde occurs naturally in many products, and it is even used as a flavouring substance (European Parliament and the Council Citation2008) in numerous products that are marketed to children, because of its fruity flavour.
To date, no scientific evidence exists to support the assumption that, for example, THC consumption of 7 µg/kg bw is no longer "safe" or that it can be assumed to pose a health risk or induce unacceptable damage to health. When comparing the safety assessment of THC with that of demonstrably more dangerous substances, there appear to be substantial and peculiar differences in the methodology and exaggerated standards of risk assessment for THC; for example, when compared to the case of morphine and its physiological precursor, codeine (see Section 6.1.1).
6.1. Genetic polymorphisms as a potential THC risk in intraspecies variability
During Phase I detoxification in the liver (), THC is converted mainly to 11-OH-THC by the cytochrome P450 isozymes CYP2C9 and CYP2C19, the latter having only minor catalytic activity (Watanabe et al. Citation2007). This psychoactive metabolite is then further oxidized to the inactive THC-9-COOH metabolite by a microsomal aldehyde oxygenase (MALDO), which is affiliated with the CYP2C sub-family (Gasse et al. Citation2020). In addition, both this metabolite and the THC parent molecule, and also other metabolites, can be glucuronidated () via Phase II detoxification (Hassenberg et al. Citation2020) by uridine 5′-diphospho-glucuronosyltransferases (Mazur et al. Citation2009).
Alternative minor THC metabolic routes include allylic hydroxylation to 8β-OH-THC and 8β,11-(OH)2-THC, which has been demonstrated in vivo, as well as epoxidation of the 9,10-double bond to 9,10-epoxyhexahydrocannabinol by CYP3A4 (Maurer et al. Citation2006; Gasse et al. Citation2018) eventually yielding the 9,10-dihydroxy-derivative or glutathione conjugate. So far, more than one hundred THC metabolites have been identified, including di- and tri-hydroxy compounds, aldehydes, ketones and carboxylic acids (Huestis Citation2007). These data are reflective of the intense level of scientific scrutiny that has been focused on THC and its metabolism in recent years.
Some investigators state that other phytocannabinoids can influence the metabolism of THC, in particular CBD (Niesink and van Laar Citation2013), which is able to modulate some of the psychotropic effects of THC (Zuardi et al. Citation1982). CBD is an inhibitor of CYP3A isoforms (Yamaori Citation2011) and this effect could theoretically inhibit THC-9,10-epoxidation and allylic hydroxylation. However, the interaction of CBD with THC is complex (Wikipedia Citation2021), and the effects of this interaction on humans also depend on the quantities and the sequence of ingestion of THC and CBD (Zuardi et al. Citation2012). For example, it was found that CBD inhibits THC-elicited paranoid symptoms and hippocampal-dependent memory impairment (Englund et al. Citation2013). Recently, Hudson et al. (Citation2019) identified a molecular mechanism that may account for how CBD functionally mitigates the neuropsychiatric side-effects of THC. However, it should be considered if physiological concentrations of phytocannabinoids from medical/recreational cannabis (let alone, hemp foods) consumption will be high enough to have any significant effects on CYP-enzymes (Silva et al. Citation2020), and thus, on interactions with each other and/or other exogenous substances. Stout and Cimino (Citation2014) concluded that “Inhibitory constants and/or half maximal inhibitory concentration values of THC, CBD, and CBN at studied CYP-450 isoforms are generally well below the expected systemic concentrations of these cannabinoids with most use”. At present, this issue has not been conclusively determined. It also remains unclear if the interactions are psychodynamic or metabolic in nature.
In a human study (N = 43, healthy volunteers), designed to measure the impact of CYP2C9 polymorphism on the PKs of orally administered THC (a significant single dose of 15 mg), it was found that plasma THC PKs did not differ due to CYP2C9*2 allele status (Sachse-Seeboth et al. Citation2009). However, the median area under the curve (AUC0→∞) for THC in the CYP2C9*3/*3 genotype was threefold that of the CYP2C9*1/*1 genotype, and the AUC for THC-9-COOH was 69% lower. Maximum THC plasma concentration after oral administration of the 15 mg THC dose was a median of 6.3 ng/mL in carriers of CYP2C9*3/*3, compared to 2.7 ng/mL in carriers of CYP2C9*1/*1. CYP2C9*3/*3 carriers also showed a trend towards increased sedation following administration of THC. Therefore, the CYP2C9*3/*3 variant may influence both the therapeutic and adverse effects of THC.
Polymorphisms in the relevant CYP enzymes would have an influence on the degradation of THC, if these enzymes would be the only, or major, enzymes for the metabolism of THC. However, this is not the case. For example, CYP2C9 polymorphism is not relevant for the application of an additional uncertainty in the risk assessment of THC because: (1.) Additional enzymes are involved (e.g. CYP2C19 and CYP3A4) in hydroxylation and epoxidation of THC. (2.) Only 13.4% of the Caucasian population are low-metabolizing carriers of a CYP2C9*3 allele (heterozygotes thereof being intermediate metabolizers), and a minuscule 0–1.3% (average 0.4%) of Caucasians are carriers of the homozygotic genotype CYP2C9*3/*3, which metabolizes THC at a markedly reduced rate (Lee Citation2002; Sachse-Seeboth et al. Citation2009).
Concerning the possible influence of genetic polymorphism, Renwick and Lazarus (Citation1998) write: “Genetically determined differences are of greatest relevance to risk assessment when the polymorphic pathway represents the major route of elimination. Poor metaboliser (PM) subjects would be at greater risk if the polymorphic pathway resulted in detoxification but at less risk than the extensive metaboliser (EM) group in cases where the pathway is involved in a bioactivation process leading to toxicity.” They further add: “For many compounds the presence of alternative or multiple pathways of elimination means that PM subjects would show little or no increase in plasma concentrations of the parent compound compared to EMs. Therefore, knowledge that a chemical is a substrate for a metabolic pathway which shows polymorphic expression raises questions about the validity of the 3.16-fold default uncertainty factor for kinetics (and therefore the combined 10-fold factor for human variability), but does not automatically invalidate the default or specifically adjusted values.”
Indeed, for THC, there are various enzymatic pathways for detoxification, not only by other cytochrome P450 enzymes producing the same metabolites (such as the inactive oxidation product THC-9-COOH), but also for alternative metabolites. For example, metabolites exist that are hydroxylated on other allylic positions of the terpenoid portion of the ring system, or on the pentyl side chain (). Therefore, there is no reason to account for an additional uncertainty (sub)factor for genetic polymorphism, because CYP2C9 is not the only enzyme employed for detoxification of THC.
6.1.1. Comparison with the risk assessment for morphine
As to the potential relevance of polymorphisms to intraspecies variability, the EFSA CONTAM Panel (Citation2018) states “Codeine metabolism to morphine is dependent of the CYP2D6 activity. Individuals can be classified into poor metaboliser, intermediate metaboliser, extensive metaboliser or ultra-rapid metaboliser. The extensive metaboliser represents the majority of the Caucasian population. The phenotypes generated by different CYP2D6 alleles affect the sensitivity of humans to adverse effects of codeine.”
“• There is a sharp increase in both morphine AUC and Cmax following codeine exposure in extensive/ultra-rapid metabolisers.”
“• No new data were identified that provide a basis to change the previous conclusion from 2011 that the maximal metabolic conversion of codeine into morphine does not exceed 20%.”
From an analysis of CYP2D6 allele-frequency data, a considerable variation was seen across major ethnic groups, and the prevalence of poor metabolizers was found to be in the range of 0.4–5.4% of the world population (Gaedigk et al. Citation2017). Concerning the genetic polymorphism of CYP2D6, which is responsible for the metabolism of codeine (i.e. the direct metabolic precursor to morphine), this obviously did not play any role in the decision on the ARfD for morphine.
The BfR (Citation2006) has given morphine a safety factor of only 4.3, based on the lowest therapeutic single oral dose of 1.9 mg of morphine per adult, which led to a recommended maximum daily intake of 6.3 µg/kg bw, whereas the EFSA CONTAM Panel (Citation2011, Citation2018) assumed a safety factor of only 3, from which a morphine ARfD of 10 µg (equivalent)/kg bw was derived. [Note: In studies with this dose, the placebo group also exhibited some effects. It should also be noted that an effective dose of opium alkaloids leads to pain relief and sedation, in a manner somewhat resembling THC.] On the other hand, if the analogous procedure with an uncertainty factor of 5 was applied to the derivation of an ARfD for THC, a value of approximately 7 µg THC/kg bw would be obtained (from the lowest therapeutic single dose of 2.5 mg, divided by factor 5, relative to an average 70 kg body weight). This corresponds exactly to the value proposed by the European hemp industry for THC (EIHA Citation2017).
The EFSA CONTAM Panel (Citation2018) concluded: “The data that have become available since the 2011 CONTAM opinion do not provide a basis for revising the ARfD for morphine of 10 µg/kg bw, which is derived from the lowest therapeutic dose of morphine. In order to characterise the risks of combined exposure to morphine and codeine, the ARfD is a group ARfD for morphine and codeine, expressed in morphine equivalents using an equivalence factor of 0.2 for codeine (The lowest single oral therapeutic dose reported for morphine is 1.9 mg, corresponding to 27 µg/kg bw for an adult weighing 70 kg).”
If these rules were applied to THC, which may exert a few adverse (but not potentially lethal) acute CNS effects somewhat similar to that of morphine, the resulting calculation should include the lowest adult therapeutic dose of THC: 2.5 mg/d and apply a factor of about 2.7–3 to derive a logical ARfD which is somewhere between 0.833 and 0.925 mg THC/d, or around 12–13 µg/kg bw (which coincides with the recommendations of Geiwitz et al. Citation2001). This is over an order of magnitude above the unrealistically conservative ARfD that has been suggested for THC by the EFSA.
6.1.2. Comparison with the risk assessment for caffeine
In the EFSA Scientific Opinion on the safety of caffeine (EFSA NDA Panel Citation2015), it is notable that certain types of risks, which have been included in the risk assessment for THC, have been excluded a priori in the derivation of a “(tolerable) upper intake level” for caffeine: “It is outside the scope of the present opinion to address possible adverse health effects of caffeine … in subgroups of the population selected on the basis of a disease condition or in sub-populations with extreme and distinct vulnerabilities due to genetic predisposition or other conditions which may require individual advice; or in combination with medicines and/or drugs of abuse, or in combination with alcohol doses which, by themselves, pose a risk to health (e.g., during pregnancy, binge drinking).” From a scientific viewpoint, it is incomprehensible that the scope of risk assessment should be so different for THC and caffeine, considering that both substances are naturally occurring constituents of plant parts (i.e. fruits, leaves, flowers) and are consumed as food ingredients, plant extracts, additives, flavourings, and food supplements.
As an aside, there similarly exists no systematic assessment by the EFSA of the amount of risk to health posed by specific alcohol doses, although it is obviously substantial (Public Health England Citation2016). Actually, the EFSA (as well as the BfR), did evaluate alcohol, but only in relation to its potential interactions with THC, in their risk assessments of the latter drug. This again exposes an illogical bias against cannabis, a cultural blindness to alcohol, or possible negligence.
Concerning genetic predisposition or polymorphism, it is known that cytochrome P450 (i.e. CYP1A2) is the main enzyme responsible for the metabolism of caffeine. In four publications, the combined prevalence of the “slow” CC and AC genotypes for the expression of CYP1A2 was reported to be between 52 and 60%, whereas the “fast” AA genotype prevalence was reported to be between 48 and 40% (EFSA NDA Panel Citation2015). Therefore, a high probability exists that a large part of the population metabolizes caffeine much more slowly than others, and there is substantial intraspecies variability in the clearance of caffeine from the body. In practice, this may explain why some people enjoy/tolerate large amounts of daily caffeine, and others consume only limited amounts, while a few refrain entirely from all methylated xanthines (i.e. theophylline from tea or theobromine from chocolate).
For example, data from a study (Palatini et al. Citation2009) on 553 individuals with a follow-up after 8.2 years (median) showed that the risk of hypertension associated with coffee intake varies according to CYP1A2 genotype. Carriers of the slow *1 F allele are at increased risk, and should therefore abstain from coffee, whereas individuals with the *1 A/*1A genotype can safely drink coffee. Another study (Palatini et al. Citation2015) on the risk of impaired fasting glucose through coffee consumption demonstrated that among the subjects, stratified by CYP1A2 genotype, heavy coffee drinkers being carriers of the slow *1 F allele (59%) had a higher adjusted risk of impaired fasting glucose (HR 2.8, 95% CI 1.3–5.9) compared to abstainers, whereas this association was of borderline statistical significance among those homozygous for the A allele (HR 1.7, 95% CI 0.8–3.8). Taken together, these data show that coffee consumption increases the risk of impaired fasting glucose in hypertension, particularly among carriers of the slow CYP1A2*1F allele. This effect may influence daily dietary choices, such as increased intake of refined carbohydrates, which can lead to chronic disease over time.
Consequently, the conclusions on caffeine by the EFSA NDA Panel (Citation2015) read as follows: “The Panel notes that several genetic and non-genetic factors have been reported to significantly affect caffeine metabolism by CYP1A2 for various population groups. Considering the reduced maternal clearance and prolonged half-life during pregnancy, and the fetus’s exposure to maternal caffeine plasma levels, the Panel considers unborn children to be the most vulnerable group for adverse effects of caffeine among the general population.” However, these acknowledgments are partly excluded from the scope of the risk assessment and the subsequent derivation of a safe intake level. A consideration of uncertainty factors was missing in the analysis, or missing a safety margin between the LOAEL and the recommended maximum caffeine intake for adults. A LOAEL of 1.0–1.3 mg/kg bw for caffeine was established for the effect of tolerance development on withdrawal symptoms, and a LOAEL of 2.5 mg/kg bw for the onset of acute anxiety and jitteriness (EFSA NDA Panel Citation2015), obviously one of the more sensitive of possible adverse effect end points. The EFSA risk assessment does not apply any uncertainty factors for extrapolation to the NOAEL and RfD. Note that the maximum recommended single dose of caffeine for healthy adults is set at about 3 mg/kg bw, or about 200 mg of caffeine per adult (more than the LOAEL for an adverse effect) and for the maximum caffeine intake over the whole day, to about 5.7 mg/kg (more than twice the LOAEL for an adverse effect!).
A recent study (Beyer and Hixon Citation2018) found that the “Human Equivalent Dose” for the NOAEL concerning cardiovascular effects of caffeine was 260 mg (2–3 cups of coffee) as a single dose for a 70 kg adult, while the LOAEL was 770 mg (7–8 cups). Application of the usual uncertainty factors in risk assessment to the LOAEL of caffeine (3 for extrapolation to NOAEL, and a default of 10 for extrapolation to the safe RfD), would result in an allowance of approximately 26 mg of caffeine (one quarter-cup of coffee) per adult per day as being the safe upper limit, which is roughly only one tenth of that currently recommended as safe. Thus, the methodology of risk assessment for caffeine is substantially different from that for THC, possibly due to an attachment bias of the investigators, caused by their habitual use of the former drug.
6.1.3. Comparison with the risk assessment for cyanide
Industrial hemp (Cannabis sativa L., fam. Cannabinaceae, ord. Rosales) and flax (Linum usitatissimum L., fam. Linaceae, ord. Malpighiales) represent two quite unrelated plant taxa, yet are traditionally thought to be “agronomic twins” due to their shared production of both a useful bast fibre and a nutritious seed. Interestingly, industrial hempseed possesses a 1:3 ratio of omega-3 to omega-6 fatty acids and flaxseed has the exact opposite 3:1 ratio. Like many plants, industrial hempseed possesses small traces of compounds thought to act as natural defensive compounds against various biotic and abiotic environmental threats (Pate Citation1994), mostly in the form of phytocannabinoids, predominantly THCA and CBDA.
Flax also possesses various secondary compounds in its seed, presumably for the same purpose, and one of them is the infamous deadly poison, cyanide, embedded within certain glycosidic phytochemicals, such as linamarin or amygdalin. Ironically, this is not a very common source of public concern, but should provoke the question of what concentrations of this compound in the seed may be harmful to consume. This has been experimentally addressed (Abraham et al. Citation2016) and it seems that the answer is not entirely assuring, at least within a worst case scenario.
Flaxseed may contain mean total cyanide levels (Abraham et al. Citation2016, p. 563) of 154 mg/kg (range 80–300 mg/kg). Their data reveal that the daily consumption of a maximum 60 g of raw flaxseed (containing 220 mg cyanide per kg of flaxseed) might be considered only marginally below concern, on an acute basis, leading to a peak concentration of 20 µM blood cyanide. The World Health Organization’s “Provisional Maximum Tolerable Daily Intake” recommendation of 20 µg/kg bw total cyanide (WHO Citation2012, p. 308) allows only 6.4 g of flaxseed daily, accounting for a hundred-fold chronic intake margin, including an approximate 10-fold margin to avoid the lower threshold for acute cyanide toxicity. Yet, unlike industrial hempseed, no serious consumer concern is usually expressed over this common item of agricultural commerce, and its cyanide levels are still unregulated in the European Union.
The health risk from an intake of other cyanide or cyanogenic glycoside containing foods was evaluated by the EFSA CONTAM Panel (Citation2016) for apricot kernel (products) and for other foods (EFSA CONTAM Panel Citation2019). The derivation of the ARfD for cyanide, which again in this case, seems to be governed by rules other than those applied for the ARfD for THC, deserves to be mentioned. In their risk assessment on cyanide, the POD is the lowest toxic threshold value for cyanide in human blood (20 µM), resulting from a cyanide intake of 0.105 mg/kg bw. Applying an uncertainty factor of 1.5 to account for toxicokinetic (and of 3.16 to account for toxicodynamic) inter‐individual differences (giving an overall UF of nearly 5) results in an ARfD of 20 µg/kg bw. Because the lowest toxic threshold value for cyanide is equivalent to a LOAEL, it is obvious that any UF for extrapolation from the LOAEL to the NOAEL was omitted, which is against the normal rules for risk assessment. Moreover, the default UF of 10 for intraspecies variability was not used, although there are not enough reliable human data for reducing the toxicokinetic part of the UFIntra to 1.5 only.
7. Risk assessments on THC by various countries, and shifting policy trends
In 1996, the Swiss BAG had derived an HBGV for THC on the basis of various studies (BAG Citation1996), defined as the “Preliminary Tolerable Daily Intake” (PTDI) and using a LOAEL of 5 mg/d per adult person. This careful analysis included calculations of plasma and urine concentrations to detect intentional cannabis use and to distinguish that from the harmless ingestion of THC traces in food. This PTDI for THC was derived by the Swiss competent authority in two ways. The first was by a single 5 mg oral THC adult dose (70 µg/kg bw) which gave rise to typical CNS symptoms in 1 of 5 adults, corresponding to a LOAEL for sensitive individuals. “Application of an UF of 10 in regard of accumulation in the body gives a provisional tolerable daily intake of 7 µg/kg body mass”. The second was by correlation of the THC concentration in blood plasma with psychomotor effects. “THC-concentrations of >1 µg/L (1 ng/mL) in plasma often seem to be correlated with difficulties in driving of motor vehicles. A stationary THC-concentration in plasma of 1 µg/L may be achieved theoretically by a chronic oral supply of approx. 85 µg THC/h or 2040 µg THC/d (absorption 20%, half-life in plasma 24 h, plasma clearance 17 L/h). Using an UF of 5 results in a provisional tolerable daily intake of 7 µg/kg bm [body mass].”
In Canada, there is no HBGV defined for THC. Over twenty years ago, a tolerable daily dose of 14 µg/kg bw of THC had been proposed in Canada by Geiwitz et al. (Citation2001), within the context of a comprehensive risk assessment on industrial hemp foods and THC, but their recommendations were ignored by Health Canada.
Food Standards Australia and New Zealand (FSANZ) derived a dose of 5 mg THC per day as the LOAEL in a careful and comprehensive 2011 risk assessment re-examination of THC in food (FSANZ Citation2011), from which was confirmed a level of 6 µg THC/kg bw as the TDI, based – inter alia – on the comprehensive work by Chesher et al. (Citation1990).
The competent authority of Croatia (HAPIH) issued an ADI as an HBGV of 0.5 mg/d or 7 µg/kg bw (HAH Citation2015), which was based on the rationale by Grotenhermen et al. (Citation2007).
Uruguay was the first country to officially legalize both recreational and medical cannabis in 2013 (Wikipedia Citation2021a). This development was followed by Canada in 2018, and on 14 June 2019, the Government of Canada announced new regulations for edible cannabis, cannabis extracts, and cannabis topicals. These regulations were published in the Canada Gazette, Part II, on 26 June 2019, and came into force on October 17, 2019. Edible cannabis, for example, has a recommended THC limit of 10 mg per discrete consumable unit (Government of Canada Citation2021). In the US, the federal capital and a clear majority the 50 states, plus some territories, currently allow legal access to either non-prescription medical cannabis or recreational cannabis (or both) under a very wide variety of regulations, which has greatly contributed to a newly developing multi-billion dollar North American industry (Wikipedia Citation2021b).
The situation is also changing in Europe, if more slowly. The modern re-establishment of cannabis-derived prescription medicines began in The Netherlands and England during the 1990s with the founding of (and subsequent collaboration between) HortaPharm BV and then GW Pharmaceuticals plc, respectively, the latter of which was recently acquired by an Irish corporation, Jazz Pharmaceuticals plc, for USD 7.2 billion.
Generally speaking, EU cannabis policy has presently evolved into three camps. The most liberal sector is led by Luxembourg, which had already effectively decriminalized personal possession of cannabis in 2001, and in 2018 committed to become the first EU country to officially legalize both medical and recreational cannabis by 2023 (Wikipedia Citation2021c). The Netherlands is included in this category because it has followed a policy of decriminalization for decades, and has even developed quasi-legal recreational-product licensing and medical-product prescription systems for their technically illegal products (Wikipedia Citation2021d). Spain has developed a particularly liberal informal cannabis policy (Wikipedia Citation2021e) and Portugal has decriminalized cannabis (and all other drugs) for personal use (Wikipedia Citation2021f).
Most of the other EU countries are employing more gradualist strategies. For example, in Belgium (Health Belgium Citation2020) and Austria (Wikipedia Citation2021g), smoked or vaped products must not have a THC level above the current EU 0.3% THC standard limit for industrial hemp. Other middle-path EU members employ various policies, ranging from that pattern to the policy of Germany, which has set up a strict prescription medical cannabis system funded by state insurance. EU non-member Switzerland allows industrial hemp products, not including isolated hashish resin, containing less than 1.0% THC (Wikipedia Citation2021h).
Some European states remain intransigent within the most conservative school of regulatory thought, for example France (Wikipedia Citation2021i) and Sweden (Wikipedia Citation2021j), although the former has instituted some very limited changes and the latter now allows the Sativex® cannabis extract upon prescription.
This movement toward the evolution of European cannabis laws (echoed in other countries worldwide) is motivated primarily by the social benefits of legalized cannabis via elimination of the vast social costs of criminalization and the provision of substantial new tax revenues, in light of the its relatively low public health concerns (Lachenmeier and Rehm Citation2015). The resultant impending wave of normalized cannabis use promises to re-frame attitudes and de-prioritize the industrial hemp trace-level THC concerns discussed herein.
8. Summary and conclusions
The LOAEL of 2.5 mg of THC, defined by the EFSA and BfR for effects on the CNS, is derived from only a few clinical studies or trials, respectively, the results of which are not conclusive because the adverse effects studied were predominantly affecting HIV-infected or cancer patients rather than healthy persons. Such patients could have already suffered from HIV-related neurocognitive impairments, and they were receiving concomitant medication which could have induced a synergy that exacerbated the observed adverse effects. Within these clinical studies, adverse effects have not been specifically reported for small groups of patients administered the lowest oral THC dose of 2.5 mg/d. Apart from these studies, there is only one trial with a small group of 11 persons who had been administered oral THC doses of 2.5 mg/d which produced only modest effects on subjective ratings, measures of cognitive performance, and physiological measures. However, this small study cannot be considered as representative for the derivation of a LOAEL.
Over the last 50 years, the threshold amount of THC that is required for psychoactivity has been carefully studied in humans and is now quite well known. Aside from the mild psychoactive effect in most humans when 5 mg of THC is taken orally, there are no other physiological or psychological effects that can be ascribed to lower amounts of THC. The whole ensemble of clinical trials and observational studies on THC shows that the LOAEL should be set to 5 mg, as a single adult daily dose.
The overall uncertainty factor (or safety factor) of around 36 that is applied to a dose of 2.5 mg THC per day, for deriving an ARfD, is set too high for such a substance of extremely low acute toxicity, compared to other substances of concern in food or consumer products such as morphine (from poppy seed), caffeine (from coffee), or cyanogenic glycosides (from flax seed). Hence, the ARfD of 1 µg of THC per kg bw, per day, recommended in 2015 by the EFSA for their assessment of an acute health risk from the intake of industrial hemp food products, cannot be justified. It is unnecessarily low, taking into account all the available scientific evidence. This conclusion is also in line with the risk assessments by the competent authorities of other countries.
More meaningfully, studies of effects in healthy people show that oral doses below 5 mg/d of THC are without significant adverse effects, so consequently, a single 5 mg dose is derived as the LOAEL. Furthermore, based on the various correlations reviewed herein (i.e. THC intake versus THC blood plasma levels versus CNS effects), a twice daily dose of 2.5 mg (with a time lag between doses of more than 6 hours) should be considered as the NOAEL. PK data (i.e. mean maximum blood plasma concentrations, half-life) show that this regimen, and certainly a single THC dose of 2.5 mg, provides systemic levels that are below any definition of legal driving impairment, as determined by a 2 ng/ml THC blood serum threshold. Below this benchmark level, which has been established by various forensic publications, no negative influences on performance tests (i.e. psychomotor function) have been found.
The intraspecies uncertainty factor is herein proposed as no more than 10 (the default factor according to generally accepted rules of risk assessment), which would then also include the more sensitive part of the population, including the elderly. Analogous to the EFSA risk assessment on caffeine, the total daily upper tolerable dose for THC should be based on two or more harmless single 2.5 mg doses administered at time intervals of 6 or more hours. Application of an overall uncertainty factor of 10 for intraspecies variability (which includes the elderly) to a NOAEL of 2 × 2.5 mg THC per day for an adult, results in a “daily intake of no concern” or a “tolerable upper intake” of 500 µg of THC per day, corresponding to 7 µg/kg bw per day. This intake can also be defined as the ARfD because it falls under the FAO/WHO JECFA definition (see Section 3.1). The ARfD derived from the NOAEL for a 2.5 mg single oral THC bolus is therefore defined as 250 µg per adult or 3.6 µg/kg of body weight, as the absolutely most conservative estimate. Thus, the resulting range for an HBGV of THC intake from food consumption is between 250 and 500 µg/d for an adult, or correspondingly between 3.6 and 7 µg/kg bw per day, which is about half the value of other justifiable published estimates.
In view of the excessively low 1 µg/kg bw EFSA daily HBGV and the consequently derived maximum for THC levels in food, recently repealed by the BfR (Citation2021), it is not surprising that they had previously issued a report (BfR Citation2018) in which it regarded THC content in food as being too high. That report was deficient because of its lacking a differentiation between psychoactive THC and non-psychoactive THCA, the latter being the major component of so-called “total-THC” (Skoczinski et al. Citation2019) and – inter alia – because of insufficient evidence of data from chemical analysis.
The EFSA (Citation2020) has been much more diligent and careful in its recent assessment of acute human exposure to THC because it acknowledged difficulties with the correct chemical analysis of THC content in foods, although it continues to base its risk assessment on an unrealistically low daily HBGV of 1 µg/kg bw.
Regulatory limits for substances (residues or contaminants, plant-inherent or not) must be based on solid toxicology studies (preferentially with a Klimisch score of 1, meaning reliable without restriction), good exposure assessments for the substance in question, comparison with toxicology results, and conclusive and consistent risk assessments. However, for the case of THC, this has not yet been achieved, particularly in contrast to the relatively lax treatment of some other toxic substances commonly found in food.
The 2020 exposure assessment by the EFSA used only samples in which “total-THC” (i.e. THC + THCA) was analyzed, therefore exaggerating psychoactivity exposure scenarios as much as 20-fold, which resulted in a large overestimation of the psychoactive THC component in, at least, unbaked food. Moreover, many other uncertainties remained, which the EFSA summarized, as follows. "The use of proxies for the consumption of hemp and hemp-containing products, the limited number of occurrence data and the analytical limitations in the quantification of Delta9-THC represent the most important sources of uncertainty. Overall, exposure estimates presented in this report are expected to represent an overestimation of acute exposure to Delta9-THC in the EU." However, the discrepancy between high estimated acute hemp food THC exposures (e.g. for industrial hempseed, at up to 9 µg/kg bw; for its oil, at up to 21 µg/kg bw; for derived raw pasta, at up to 6.4 µg/kg bw) and the established ARfD, is not only a consequence of the “total-THC” concept, but is even more so for the case of the ARfD level being set much too low. Reports from health care systems on acute intoxications by THC in food from industrial hemp (alone, without alcohol) are extremely rare, with those undoubtedly resulting from THC dosing via drug-type cannabis-derived products.
The ARfD was derived for THC alone (i.e. without THCA), and not for the “total-THC” (THC + THCA) in industrial hemp products, for the simple reason that all studies on which the EFSA CONTAM Panel (Citation2015) based its Scientific Opinion had been carried out with dronabinol (i.e. pure THC). Hence, no conclusions are possible on any presumed risk to the consumer, because the actual proportions of psychoactive THC versus non-psychoactive THCA were not known, and any possible THC inhibiting effects due to the predominant CBD proportions that are present in industrial hemp were ignored.
Glossary
ADI = Acceptable Daily Intake
ADMET = Absorption, distribution, metabolism, excretion, toxicity
AE = Adverse event
AIDS = Acquired Immune Deficiency Syndrome
AM = Arithmetic Mean
ARfD = Acute Reference Dose
AUC = Area under concentration/time-curve
BAC = Blood alcohol content
BAG = Bundesamt für Gesundheitswesen (Swiss “Federal Office of Public Health”)
BAC = Blood alcohol content
BfR = Bundesinstitut für Risikobewertung (German “Federal Institute for Risk Assessment”)
bid = Twice daily
BMD = Benchmark Dose
BMDL10 = Benchmark Dose Response of 10%
BMI = Body mass index
Bw = Body weight
Cmax = Maximum Drug Concentration Achieved
CBD = (-)-trans-cannabidiol
CBDA = Cannabidiol-2-carboxylic acid
CBN = Cannabinol
CI = Confidence Interval
CNS = Central nervous system
CONTAM Panel = EFSA Panel on Contaminants in the Food Chain
CV = Coefficient of Variation
d = day
DFG = Deutsche Forschungsgemeinschaft (“German Research Foundation”)
dronabinol = Generic name for synthetic (-)-trans-delta-9-tetrahydrocannabinol
DRUID = DRiving Under the Influence of Drugs
EFSA = European Food Safety Authority
EM = Extensive metaboliser
Epidiolex® = CBD oral liquid formulation from GW Pharmaceuticals
FDA = U.S. Food and Drug Administration
FSANZ = Food Standards of Australia and New Zealand
GC-MS = Gas chromatography-mass spectrometry
GM = Geometric mean
Grenzwertkommission = (German “Expert Panel on Drink and Drug Driving Limits”)
HAND = HIV-Associated Neurocognitive Disorder
HAP = Human Abuse Potential
HBGV = Health Based Guidance Value
HHS = Dept. of Health & Human Services of the U.S. Public Health Service
HIV = Human Immunodeficiency Virus
HR = Hazard Ratio
JECFA = (FAO/WHO) Joint Expert Committee on Food Additives
LOAEL = Lowest Observed Adverse Effect Levels
LOD = Limit of Detection
LOQ = Limit of Quantification
Marinol® = THC oral capsule formulation from Alkem Laboratories
Namisol® = THC oral liquid formulation from Echo Pharmaceuticals
NDA = New Drug Application
NOEL = No Observable Effect Level
NOAEL = No Observed Adverse Effect Level
P450 = Hepatic oxidative enzyme system
PK = Pharmacokinetic
PM = Poor Metaboliser
POD = Point of Departure
PTDI = Preliminary Tolerable Daily Intake
RfD = Reference dose
RIA = Radioimmunoassay
Sativex® = THC oro-mucosal liquid spray formulation from GW Pharmaceuticals
SD = Standard Deviation
Syndros® = THC oral liquid formulation from Benuvia Therapeutics
TDI = Tolerable Daily Intake
THC = (-)-trans-delta-9-tetrahydrocannabinol
THCA = tetrahydrocannabinol-2-carboxylic acid
Tmax = Time required to achieve maximum drug level
THC-9-COOH = 11-nor-9-carboxy-THC
11-OH-THC = 11-hydroxy-THC
UFInter = Interspecies Uncertainty Factor
UFIntra = Intraspecies Uncertainty Factor
UFPK = Pharmacokinetic Uncertainty Factors
VAS = Visual Analog Scale
Acknowledgments
The authors appreciate J. C. Callaway of Finola OY for proof-reading the manuscript. The comments and suggestions of Dr Callaway, and resulting discussions, greatly helped to improve our efforts. The authors also thank Dr McClellan for manuscript submission advice, and extend our gratitude to the anonymous reviewers.
Special thanks go to Josie Brown for her wonderful and patient production help.
Declaration of interest
Bernhard Beitzke is an independent consultant, and a member of the Advisory Committee to the European Industrial Hemp Association (EIHA). The EIHA is an industrial hemp processing industry association based in Brussels (Belgium), with about 250 members in more than 30 countries, representing the interests of industrial hemp producers and traders on both the pan-European and national levels. Dr Beitzke has not received financial support for this work, which is a summary of his five year engagement with industrial hemp and the study of phytocannabinoids. David W. Pate has no known competing financial interests or personal relationships which could have appeared to influence the work reported in this manuscript. Dr Pate has drawn upon only his 50-plus years of involvement with Cannabis as an organism providing a potentially unparalleled agricultural resource, and cannabis as the natural product origin for an array of medicinal and recreational products. The authors’ affiliations are shown on the cover page. They take sole responsibility for the writing and content of this manuscript, and neither of them has been involved in legal or regulatory matters related to its content.
Correction Statement
This article has been corrected with minor changes. These changes do not impact the academic content of the article.
Notes
1 Data collected from 23 EU enterprises by on behalf of Hanfgesellschaft (Berlin, Germany), 01.12.1997: Hempseed: ca 200 tonnes; Hempseed oil: ca 33,000 L; Hemp ready-made products (snacks, flour, muesli, bakery products, and pasta): ca 55 tonnes; drinks with hemp flowers/leaves: ca 115,000 L; snacks with hemp flowers ca 2 tonnes. https://eiha.org/documents/hanfgesellschaft-01-12-1997/.
2 “Total-THC” is the sum of delta-9-THC and delta-9-tetrahydrocannabinol carboxylic acid (THCA), normalized by calculation to delta-9-THC using the ratio of their molecular masses: [“total-THC”] = [THC] + [THCA*0.877]. The content of THC in the plant is still analysed in EU as “total-THC” by gas chromatography according to Commission Delegated Regulation (EU) 2017/1155 of 15 February 2017.
3 Total THC is according to the BfR definition: "The above values refer to the food ready for consumption and apply to total THC including delta-9-tetrahydrocannabinol carboxylic acid". See https://www.bfr.bund.de/de/presseinformation/2000/07/bgvv_empfiehlt_richtwerte_fuer_thc__tetrahydrocannabinol__in_hanfhaltigen_lebensmitteln-884.html (Accessed 18.09.2020).
4 Dronabinol is the INN of the pharmaceutical active ingredient (-)-trans-Δ9-Tetrahydrocannabinol (Δ9-THC) which by its chemical structure is identical to the THC naturally occurring in hemp. For avoidance of doubt, this Δ9-THC is free from Δ9-tetrahydrocannabinol carboxylic acid, and not to be confused with “total-THC” (the latter often simply called “THC”). “Total-THC” is the sum of Δ9-THC and Δ9-tetrahydrocannabinol carboxylic acid, normalized by calculation to Δ9-THC.
5 Human body surface area used here (1.88 m²) is the average of male and female body surface areas as found by: Tikuisis et al.: human body surface area: measurement and prediction using three dimensional body scans. Eur J Appl Physiol. 2001; 85(3–4):264–271.
6 Using the distribution factors determined experimentally by Giroud et al. (Citation2001).
7 THC is understood as Δ9-THC because of its definition in the Canadian Cannabis Regulations – SOR/2018-144 (Section 1), Definitions – Act and Regulations 1 (1): Cannabis Regulations (justice.gc.ca).
8 For correlation of Coefficients of Variation with the UF for pharmacokinetics see Renwick AG, Lazarus NR. 1998. Human variability and noncancer risk assessment – an analysis of the default uncertainty factor. Regul Toxicol Pharmacol. 27 (1Pt 2), 3–20, chapter on data analysis. Accordingly, a coefficient of variation of 30% correlates to an UFPK=2, of 50% to an UFPK=2.5, and of 80% to an UFPK≥3.2.
9 Personal communication by G. Leson to B. Beitzke.
10 Deutsche Forschungsgemeinschaft (DFG) – Permanent Senate Commission for the Investigation of Health Hazards of Chemical Compounds in the Work Area, List of MAK and BAT Values, 2020, https://www.dfg.de/en/dfg_profile/statutory_bodies/senate/health_hazards/. Acetaldehyde is listed there under Carcinogenic Substances classified as Carc cat 5 and Muta cat 5: “5. Substances that cause cancer in humans or animals or that are considered to be carcinogenic for humans and for which a MAK value can be derived. A genotoxic mode of action is of prime importance but is considered to contribute only very slightly to human cancer risk, provided the MAK and BAT values are observed. The classification and the MAK and BAT values are supported by information on the mode of action, dose-dependence and toxicokinetic data”. Under the Regulation (EC) No 1272/2008 (on classification, labelling and packaging of chemical substances), acetaldehyde is legally classified as Carc Cat 2.
References
- Abbott Laboratories Ltd, Quebec, Canada. 2011. Marinol® product monograph. 01.01.2011. https://pdf.hres.ca/dpd_pm/00013378.PDF
- Abd-Elsalam WH, Alsherbiny MA, Kung JY, Pate DW, Löbenberg R. 2019. LC-MS/MS quantitation of phytocannabinoids and their metabolites in biological matrices. Talanta. 204:846–867.
- Abel EL. 1980. Marijuana: the first twelve thousand years. New York, NY: Plenum Press. ISBN: 978-1-4899-2191-8.
- Abraham K, Buhrke T, Lampen A. 2016. Bioavailability of cyanide after consumption of a single meal of foods containing high levels of cyanogenic glycosides: a crossover study in humans. Arch Toxicol. 90(3):559–574.
- Agurell S, Carlsson S, Lindgren JE, Ohlsson A, Gillespie H, Hollister L. 1981. Interactions of delta 1-tetrahydrocannabinol with cannabinol and cannabidiol following oral administration in man. Assay of cannabinol and cannabidiol by mass fragmentography. Experientia. 37(10):1090–1092.
- Ahmed AIA, van den Elsen GAH, Colbers A, Kramers C, Burger DM, van der Marck MA, Rikkert MGMO. 2015. Safety, pharmacodynamics, and pharmacokinetics of multiple oral doses of delta-9-tetrahydrocannabinol in older persons with dementia. Psychopharmacology (Berl). 232(14):2587–2595..
- Ahmed AIA, van den Elsen GAH, Colbers A, van der Marck MA, Burger DM, Feuth TB, Rikkert MGMO, Kramers C. 2014. Safety and pharmacokinetics of oral delta-9-tetrahydrocannabinol in healthy older subjects: a randomized controlled trial. Eur Neuropsychopharmacol. 24(9):1475–1482.
- Auwärter V, Daldrup T, Graw M, Jachau K, Käferstein H, Knoche A, Musshoff F, Skopp G, Thierauf-Emberger A, Tönnes S. 2015. Empfehlung der Grenzwertkommission für die Konzentration von Tetrahydrocannabinol (THC) im Blutserum zur Feststellung des Trennungsvermögens von Cannabiskonsum und Fahren [Recommendation by the expert panel on drink and drug driving limits on the concentration of tetrahydrocannabinol (THC) in blood serum to determine the separability of cannabis use and driving]. Blutalkohol. 52(5):322–323.
- Badowski ME. 2017. A review of oral cannabinoids and medical marijuana for the treatment of chemotherapy-induced nausea and vomiting: a focus on pharmacokinetic variability and pharmacodynamics. Cancer Chemother Pharmacol. 80(3):441–449.
- [BAG] Bundesamt für Gesundheitswesen [Federal Office of Public Health] (Switzerland). 1996. Lebensmittel-Info: Verwendung von Hanf in Lebensmitteln und Gebrauchsgegenständen [Food notes: Use of hemp in foods and objects of utility]. Bulletin 24, 24.6.1996, Kreisschreiben Nr. 2, 13.3.1996.
- Ballard ME, de Wit H. 2011. Combined effects of acute, very-low-dose ethanol and delta(9)-tetrahydrocannabinol in healthy human volunteers. Pharmacol Biochem Behav. 97(4):627–631.
- Bar-Sela G, Zalman D, Semenysty V, Ballan E. 2019. The effects of dosage-controlled cannabis capsules on cancer-related cachexia and anorexia syndrome in advanced cancer patients: pilot study. Integr Cancer Ther. 18:1–8.
- [BASt] Bundesanstalt für Straßenwesen [Federal Highway Research Institute, Germany]. 2012. DRUID Final Report Deliverable 0.1.8 Rev 2.0. Authors: Schulze H, Schumacher M, Urmeew R, Auerbach K. https://www.bast.de/Druid/EN/Dissemination/downloads_and_links/Final_Report.html?nn=613800.
- Beal JE, Olson R, Laubenstein L, Morales JO, Bellman P, Yangco B, Lefkowitz L, Plasse TF, Shepard KV. 1995. Dronabinol as a treatment for anorexia associated with weight loss in patients with AIDS. J. Pain Sympt Managem. 10(2):89–97.
- Beal JE, Olson R, Lefkowitz L, Laubenstein L, Bellman P, Yangco B, Morales JO, Murphy R, Powderly W, Plasse TF, et al. 1997. Long-term efficacy and safety of dronabinol for acquired immunodeficiency syndrome-associated anorexia. J Pain Symptom Manage. 14(1):7–14. .
- Beyer LA, Hixon ML. 2018. Review of animal studies on the cardiovascular effects of caffeine. Food Chem Toxicol. 118:566–571.
- [BfR] Bundesinstitut für Risikobewertung [Federal Institute for Risk Assessment] (Germany). 2006. BfR recommends provisional daily upper intake level and a guidance value for morphine in poppy seeds. BfR Health Assessment No. 012/2006, 27 Dec 2005. https://www.bfr.bund.de/de/a-z_index/morphin-7391.html.
- [BfR] Bundesinstitut für Risikobewertung [Federal Institute for Risk Assessment] (Germany). 2018. Tetrahydrocannabinol levels are too high in many food products containing hemp – adverse health effects are possible, BfR Opinion no. 034/2018 of 8 Nov 2018.
- [BfR] Bundesinstitut für Risikobewertung [Federal Institute for Risk Assessment] (Germany). 2021. The BfR recommends acute reference dose as basis for assessing hemp-containing foodstuff. BfR Opinion No 006/2021 issued 17 Feb 2021.
- Bilkei-Gorzo A, Albayram O, Draffehn A, Michel K, Piyanova A, Oppenheimer H, Dvir-Ginzberg M, Rácz I, Ulas T, Imbeault S, et al. 2017. A chronic low dose of Δ9-tetrahydrocannabinol (THC) restores cognitive function in old mice. Nat Med. 23(6):782–787.
- Bosy TZ, Cole KA. 2000. Consumption and quantitation of delta9-tetrahydrocannabinol in commercially available hemp seed oil products. J Anal Toxicol. 24(7):562–566.
- Bucher B, Gerlach K, Frei P, Knöpfli K, Scheurer E. 2020. Bericht THC-Grenzwerte im Strassenverkehr – eine Literaturanalyse [Report THC limits in driving – a literature analysis], Institut für Rechtsmedizin der Universität Basel [Institute of Forensic Medicine], Dec 2020, Im Auftrag des Bundesamtes für Gesundheit (BAG) [Ordered by BAG]. German.
- Callaway JC. 2004. Hempseed as a nutritional resource: an overview. Euphytica. 140(1–2):65–72.
- Callaway JC, Pate DW. 2009. Hempseed oil. In: Moreau RA, Kamal-Eldin A, editors. Gourmet and health promoting specialty oils. Urbana (IL): American Oil Chemists Society Press. Chapter 5. p. 185–213. ISBN 978-1-8939-9797-4.
- Government of Canada. 2019. Dept of Justice. Legislative Background: reform to the Transportation Provisions of the Criminal Code (Bill C-46). Annex 2 – Drugs and Driving Committee report on drug per se limits. https://www.justice.gc.ca/eng/cj-jp/sidl-rlcfa/c46/p8.html. [Date modified: 2019-08-30].
- Government of Canada. 2021. Cannabis Regulations SOR/2018-144. Part 6, 96(1). https://laws-lois.justice.gc.ca/eng/regulations/SOR-2018-144/page-15.html#docCont; (accessed 30 Jun 2021).
- Chesher GB, Bird KD, Jackson DM, Perrignon A, Starmer GA. 1990. The effects of orally administered Δ9-tetrahydrocannabinol in man on mood and performance measures: a dose-response study. Pharmacol Biochem Behav. 35(4):861–864.
- [DFG] Deutsche Forschungsgemeinschaft [German Research Foundation]. 1997–2004. Δ9-Tetrahydrocannabinol (THC) in Hanfprodukten [Δ9-Tetrahydrocannabinol (THC) in hemp foods]. Lebensmittel und Gesundheit II, Sammlung der Beschlüsse und Stellungnahmen [Food and Health II, Collection of Decisions and Opinions]. Mitt. 7, Kap. 25, p. 172 ff.
- [DFG] Deutsche Forschungsgemeinschaft [German Research Foundation] Permanent Senate Commission for the Investigation of Health Hazards of Chemical Compounds in the Work Area. 2020. List of MAK and BAT Values. https://www.dfg.de/en/dfg_profile/statutory_bodies/senate/health_hazards/.
- Dorne JLCM, Renwick AG. 2005. The refinement of uncertainty/safety factors in risk assessment by the incorporation of data on toxicokinetic variability in humans. Toxicol Sci. 86(1):20–26.
- Dourson ML, Felter SP, Robinson D. 1996. Evolution of science-based uncertainty factors in noncancer risk assessment. Regul Toxicol Pharmacol. 24(2 Pt 1):108–120.
- Drummer OH, Gerostamoulos J, Batziris H, Chu M, Caplehorn J, Robertson MD, Swann P. 2004. The involvement of drugs in drivers of motor vehicles killed in Australian road traffic crashes. Accid Anal Prev. 36(2):239–248. .
- Dussy FE, Hamberg C, Luginbühl M, Schwerzmann T, Briellmann TA. 2005. Isolation of Delta9-THCA-A from hemp and analytical aspects concerning the determination of Delta9-THC in cannabis products . Forensic Sci Int. 149(1):3–10.
- EFSA CONTAM Panel (EFSA Panel on Contaminants in the Food Chain). 2011. Scientific opinion on the risks for public health related to the presence of opium alkaloids in poppy seeds. EFSA J. 9(11):2405–2150..
- EFSA CONTAM Panel (EFSA Panel on Contaminants in the Food Chain). 2015. Scientific opinion on the risks for human health related to the presence of tetrahydrocannabinol (THC) in milk and other food of animal origin. EFSA J. 13(6):1–125.
- EFSA CONTAM Panel (EFSA Panel on Contaminants in the Food Chain). 2016. Scientific opinion on the acute health risks related to the presence of cyanogenic glycosides in raw apricot kernels and products derived from raw apricot kernels. EFSA J. 14(4):4424:1–47.
- EFSA CONTAM Panel (EFSA Panel on Contaminants in the Food Chain). 2018. Update of the scientific opinion on opium alkaloids in poppy seeds. EFSA J. 16(5):5243:1–74.
- EFSA CONTAM Panel (EFSA Panel on Contaminants in the Food Chain). 2019. Scientific opinion on the evaluation of the health risks related to the presence of cyanogenic glycosides in foods other than raw apricot kernels. EFSA J. 17(4):5662:1–78.
- EFSA NDA Panel (EFSA Panel on Dietetic Products, Nutrition and Allergies). 2015. Scientific opinion on the safety of caffeine. EFSA J. 13(5):4102:1–120.
- EFSA Sci Com (EFSA Scientific Committee). 2009. Guidance of the Scientific Committee on a request from EFSA on the use of the benchmark dose approach in risk assessment. EFSA J. 7(6):1150. 72 pp.
- EFSA Sci Com (EFSA Scientific Committee). Hardy A, Benford D, Halldorsson T, Jeger MJ, Knutsen KH, More S, Mortensen A, Naegeli H, Noteborn H, Ockleford C. 2017. Update: guidance on the use of the benchmark dose approach in risk assessment. EFSA J. 15(1)4658:1–41.
- EFSA (European Food Safety Authority). Arcella D, Cascio C, Mackay K. 2020. Acute human exposure assessment to tetrahydrocannabinol (Δ9-THC). EFSA J. 18(1):5953:1–41.
- EIHA (European Industrial Hemp Association). 2016. Decarboxylation of tetrahydrocannabinolic acid (THCA) to active THC. Authors: Iffland K, Carus M, Grotenhermen F, nova-Institute GmbH. https://eiha.org/wp-content/uploads/2021/10/16-10-25-Decarboxylation-of-THCA-to-active-THC.pdf.
- EIHA (European Industrial Hemp Association). Baňas B, Beitzke B, Carus M, Iffland K, Kruse D, Sarmento L, Sfrija D. 2017. Reasonable guidance values for THC (Tetrahydrocannabinol) in food products, Position Paper. https://eiha.org/wp-content/uploads/2021/01/17-09-18-THC-Position-paper_EIHA.pdf.
- Englund A, Morrison PD, Nottage J, Hague D, Kane F, Bonaccorso S, Stone JM, Reichenberg A, Brenneisen R, Holt D, et al. 2013. Cannabidiol inhibits THC-elicited paranoid symptoms and hippocampal-dependent memory impairment. J Psychopharmacol. 27(1):19–27.
- EPA (Environmental Protection Agency (US)). Risk Assessment Forum. 2002. A Review of the Reference Dose and Reference Concentration Processes, EPA/630/P-02/002F, Final Report. https://www.epa.gov/risk/review-reference-dose-and-reference-concentration-processes-document.
- EU Commission. 2020. Common Catalogue of varieties of agricultural plant species. 37th complete ed. (2019/C13/01). OCJ 13, 01.11.2019, p. 241-3. See also: EU. Plant variety database. A-85-Hemp-cannabis sativa L. https://ec.europa.eu/food/plant/plant_propagation_material/plant_variety_catalogues_databases/search/public/index.cfm; (accessed 2021 Jul 02).
- European Parliament and the Council. 2008. Regulation (EC) No. 1334/2008 of the on flavourings and certain food ingredients with flavouring properties for use in and on foods, consolidated version 03.12.2020, Annex I, Table 1, FL no. 05.001. http://data.europa.eu/eli/reg/2008/1334/2020-12-03.
- FDA (Food and Drug Administration (US)). 1985. NDA 018651. Review, 31.05.1985; https://www.accessdata.fda.gov/scripts/cder/daf/index.cfm?event=overview.process&ApplNo=018651.
- FDA (Food and Drug Administration (US)). Center for Drug Evaluation and Research. 2016. Memorandum, April 22, 2016, Reference ID: 3921488, p. 16 ff. In: Application number: 205525Orig1s000, Other Review(s). Syndros® Dronabinol Oral Solution, New Drug Application (NDA). https://www.accessdata.fda.gov/drugsatfda_docs/nda/2016/205525Orig1s000TOC.cfm.
- FDA (Food and Drug Administration (US)). 2017. Marinol® (dronabinol) capsules, for oral use, Prescribing Information, Revised 08/2017, Reference ID: 4145204. https://www.accessdata.fda.gov/drugsatfda_docs/label/2017/018651s029lbl.pdf.
- Fogel JS, Kelly TH, Westgate PM, Lile JA. 2017. Sex differences in the subjective effects of oral Δ9-THC in cannabis users. Pharmacol Biochem Behav. 152:44–51.
- FSANZ (Food Standards Australia New Zealand). 2011. Supporting Document 1, Risk Assessment Report, Application A360. https://www.foodstandards.govt.nz/code/applications/Pages/applicationa1039lowt4708.aspx.
- FSANZ (Food Standards Australia New Zealand). 2012. Application A1039 – Low THC Hemp as Food, Supporting Document 1 -, Safety Assessment (Approval) – Application A1039. 8 Nov 2012. https://www.foodstandards.govt.nz/code/applications/Pages/applicationa1039lowt4708.aspx.
- Gaedigk A, Sangkuhl K, Whirl-Carrillo M, Klein T, Leeder JS. 2017. Prediction of CYP2D6 phenotype from genotype across world populations. Genet Med. 19(1):69–76.
- Galasso I, Russo R, Mapelli S, Ponzoni E, Brambilla IM, Battelli G, Reggiani R. 2016. Variability in seed traits in a collection of Cannabis sativa L. genotypes. Front Plant Sci. 7:688–689.
- Gaoni Y, Mechoulam R. 1964. Isolation, structure, and partial synthesis of an active constituent of hashish. J Am Chem Soc. 86(8):1646–1647.
- Gasse A, Pfeiffer H, Köhler H, Schürenkamp J. 2018. 8β-OH-THC and 8β,11-diOH-THC-minor metabolites with major informative value? Int J Legal Med. 132(1):157–164.
- Gasse A, Vennemann M, Köhler H, Schürenkamp J. 2020. Toxicogenetic analysis of Δ9-THC-metabolizing enzymes. Int J Legal Med. 134(6):2095–2103.
- Geiwitz J, the Ad Hoc Committee on Hemp Risks. 2001. THC in Hemp Foods and Cosmetics: The Appropriate Risk Assessment. www.hempreport.com/response/ResponseHCfinal2001.pdf.
- Giroud C, Ménétrey A, Augsburger M, Buclin T, Sanchez-Mazas P, Mangin P. 2001. Delta(9)-THC, 11-OH-Delta(9)-THC and Delta(9)-THCCOOH plasma or serum to whole blood concentrations distribution ratios in blood samples taken from living and dead people. Forensic Sci Int. 123(2–3):159–164.
- Goodwin RS, Gustafson RA, Barnes A, Nebro W, Moolchan ET, Huestis MA. 2006. Delta(9)-tetrahydrocannabinol, 11-hydroxy-delta(9)-tetrahydrocannabinol and 11-nor-9-carboxy-delta(9)-tetrahydrocannabinol in human plasma after controlled oral administration of cannabinoids . Ther Drug Monit. 28(4):545–551.
- Gorter R, Seefried M, Volberding P. 1992. Dronabinol effects on weight in patients with HIV infection. AIDS. 6(1):127.
- Gray KM, Hart CL, Christie DK, Upadhyaya HP. 2008. Tolerability and effects of oral Delta9-tetrahydrocannabinol in older adolescents with marijuana use disorders . Pharmacol Biochem Behav. 91(1):67–70.
- Grotenhermen F. 2003. Clinical pharmacokinetics of cannabinoids. J Cannabis Ther. 3(1):3–51.
- Grotenhermen F, Leson G, Berghaus G, Drummer OH, Krüger HP, Longo M, Moskowitz H, Perrine B, Ramaekers JG, Smiley A, et al. 2007. Developing limits for driving under cannabis. Addiction. 102(12):1910–1917.
- Grotenhermen F, Leson G, Pless P. 2001. Assessment of exposure to and human health risk from THC and Other Cannabinoids in Hemp Foods, Oct 11. Berkeley, CA: Leson Environmental Consulting.
- Grotenhermen F, Leson G. 2002. Reassessing the Drug Potential of Industrial Hemp, nova-Institut, Hürth, Germany, and Leson Environmental Consulting, Berkeley, CA, 2002, pp. 2, 23. https://www.semanticscholar.org/paper/Reassessing-the-Drug-Potential-of-Industrial-Hemp-Grotenhermen-Leson/79dbfcf834485087bfcc7b6e84e781ffeca32d3c.
- HAH (Hrvatska Agencija za Hranu. Radna grupa za donošenjeznanstvenog mišljenja) [Croatian Food Agency Working Group on Scientific Opinion]. 2015. Znanstveno Mišljenje, Znanstveno mišljenjeo Utjecaju na zdravljerazličitih vrsta hrane od sjemenki koja sadržisjemenke industrijske konoplje (Zahtjev HAH-Z-2015-1) 25. svibnja 2015 [Scientific Opinion, Impact on the health of various types of seed foods containing industrial hemp seeds (REQUEST HAH-Z-2015-1) 25 May 2015 (English summary on p. 3–4)]. https://www.hah.hr/doneseno-znanstveno-misljenje-hah-a-o-utjecaju-na-zdravlje-razlicitih-vrsta-hrane-od-sjemenki-i-koja-sadrzi-sjemenke-industrijske-konoplje/; (accessed 16 Mar 2021).
- Hampson AJ, Grimaldi M, Axelrod J, Wink D. 1998. Cannabidiol and (-)Delta9-tetrahydrocannabinol are neuroprotective antioxidants . Proc Natl Acad Sci USA. 95(14):8268–8273.
- Hargutt V, Krüger H-P, Knoche A. 2011. DRUID: driving under the influence of alcohol, illicit drugs and medicines. Risk estimations from different methodological approaches. BASt/UWURZ, 6th Framework Programme, DRUID Deliverable 1.3.1. https://www.bast.de/Druid/EN/deliverales-list/downloads/Deliverable_1_3_1.html.
- Hartman RL, Brown TL, Milavetz G, Spurgin A, Gorelick DA, Gaffney G, Huestis MA. 2015. Controlled cannabis vaporizer administration: blood and plasma cannabinoids with and without alcohol. Clin Chem. 61(6):850–869.. See also: Science Daily: Any dose of alcohol combined with cannabis significantly increases levels of THC in blood, May 27 (2015), Source: American Association for Clinical Chemistry, https://www.sciencedaily.com/releases/2015/05/150527112728.htm.
- Hassenberg C, Clausen F, Hoffmann G, Studer A, Schürenkamp J. 2020. Investigation of phase II metabolism of 11-hydroxy-Δ-9-tetrahydrocannabinol and metabolite verification by chemical synthesis of 11-hydroxy-Δ-9-tetrahydrocannabinol-glucuronide. Int J Legal Med. 134(6):2105–2119..
- Health Belgium 2020. Positive List of Herbal product for smoking in Belgium. www.health.belgium.be/sites/default/files/uploads/fields/fpshealth_theme_file/positivelist_hps_002_14.pdf; (accessed 30 Jun 2021).
- Heaton RK, Clifford DB, Franklin DR, Woods SP, Ake C, Vaida F, Ellis RJ, Letendre SL, Marcotte TD, Atkinson JH, CHARTER Group, et al. 2010. HIV-associated neurocognitive disorders persist in the era of potent antiretroviral therapy: CHARTER study. Neurology. 75(23):2087–2096.
- Hudson R, Renard J, Norris C, Rushlow W, Laviolette SR. 2019. Cannabidiol counteracts the psychotropic side-effects of Δ-9-tetrahydrocannabinol in the ventral hippocampus through bidirectional control of ERK1-2 phosphorylation. J Neurosci. 39(44):8762–8777.
- Huestis MA. 2007. Human cannabinoid pharmacokinetics. Chem Biodivers. 4(8):1770–1804.
- Iffland K, Kruse D, Carus M. 2016. Comparison of EFSA’s rationale behind using uncertainty factors for plant ingredients in food. EIHA Paper, Hürth (Germany). https://eiha.org/wp-content/uploads/2021/06/Comparison-of-EFSAs-rationale-behind-UFs-for-plant-ingredient-in-food_EIHA_20161.pdf.
- Kaufmann HP, Juschkewitsch S. 1930. Quantitative Analyse des Hanföls [Quantitative Analysis of Hemp Oil] (German). Z Angew Chem. 43(4):90–91.
- Klumpers LE, Beumer TL, van Hasselt JG, Lipplaa A, Karger LB, Kleinloog HD, Freijer JI, de Kam ML, van Gerven JM. 2012. Novel Δ(9) -tetrahydrocannabinol formulation Namisol® has beneficial pharmacokinetics and promising pharmacodynamic effects. Br J Clin Pharmacol. 74(1):42–53.
- Kruger C, Lodder R. 2018. Establishing limits for THC-content in hemp-derived foods, Food Technol. 72:20–21. https://www.ift.org/news-and-publications/food-technology-magazine/issues/2018/september/columns/food-medicine-and-health-thc-content-in-hemp-derived-foods; (accessed 16 March 2021).
- Lachenmeier DW, Rehm J. 2015. Comparative risk assessment of alcohol, tobacco, cannabis and other illicit drugs using the margin of exposure approach. Sci Rep. 5(1):8126.
- Lee CR, Goldstein JA, Pieper JA. 2002. Cytochrome P450 2C9 polymorphisms: a comprehensive review of the in-vitro and human data. Pharmacogenetics. 12(3):251–263.
- Lehmann AJ, Fitzhugh OG. 1954. 100-Fold margin of safety. Assoc. Food and Drug Official US Q. Bulletin 18, 33-35. ISSN: 0004-5721. https://hero.epa.gov/hero/index.cfm/reference/details/reference_id/3195.
- Leson G, Pless P, Grotenhermen F, Kalant H, ElSohly MA. 2001. Evaluating the impact of hemp food consumption on workplace drug tests. J Anal Toxicol. 25(8):691–698. In this study “total-THC” (in contrast to delta-9-THC) is “determined by GC-MS. It includes both free (phenolic) Δ9-tetrahydrocannabinol and THC acids A and B”. Analyses were carried out according to the Health Canada Industrial Hemp Technical Manual: TPP-BDS-004 – Basic method for determination of THC in hempseed oil, 1992.
- Lewis SC, Lynch JR, Nikiforov AI. 1990. A new approach to deriving community exposure guidelines from “no-observed-adverse-effect-levels. Regul Toxicol Pharmacol. 11(3):314–330.
- Little Pro. 2017. https://www.chemsafetypro.com/Topics/CRA/What_is_Point_of_Departure_(POD)_in_Toxicology_and_How_to_Use_It_to_Calculate_Reference_Dose_RfD.html.
- Maurer HH, Sauer C, Theobald DS. 2006. Toxicokinetics of drugs of abuse: current knowledge of the isoenzymes involved in the human metabolism of tetrahydrocannabinol, cocaine, heroin, morphine, and codeine, review. Ther Drug Monit. 28(3):447–453.
- Mazur A, Lichti CF, Prather PL, Zielinska AK, Bratton SM, Gallus-Zawada A, Finel M, Miller GP, Radomińska-Pandya A, Moran JH. 2009. Characterization of human hepatic and extrahepatic UDP-glucuronosyltransferase enzymes involved in the metabolism of classic cannabinoids. Drug Metab Dispos. 37(7):1496–1504..
- McGilveray Pharmacon Inc and the University of Ottawa, Ontario. 2005. Pharmacokinetics of cannabinoids. Pain Res Managem. 10(Suppl A):15A–22A.
- Mechoulam R, editor. 2005. Cannabinoids as Therapeutics, Birkhäuser, Basel.
- Mütze F. 2017. The drug driving situation in the Netherlands: regulating drug driving to protect all road users, https://etsc.eu/17-november-2017-regulating-drug-driving-to-protect-all-road-users-vienna/.
- Nadulski T, Sporkert F, Schnelle M, Stadelmann AM, Roser P, Schefter T, Pragst F. 2005. Simultaneous and sensitive analysis of THC, 11-OH-THC, THC-COOH, CBD, and CBN by GC-MS in plasma after oral application of small doses of THC and cannabis extract. J Anal Toxicol. 29(8):782–789.
- Niesink RJ, van Laar MW. 2013. Does cannabidiol protect against adverse psychological effects of THC? Front Psychiatry. 4:130.
- Nightingale S, Winston A, Letendre S, Michael BD, McArthur JC, Khoo S, Solomon T. 2014. Controversies in HIV-associated neurocognitive disorders. Lancet Neurol. 13(11):1139–1151.
- NIH. 1996. National Toxicology Program: Technical Report on the toxicology and carcinogenesis of 1-trans-delta-9-tetrahydrocannabinol (CAS NO. 1972-08-3) in F344/N rats and B6C3F1 mice (gavage studies). National Toxicology Program Technical Report 446, NIH Publication No. 97-3362. https://pubmed.ncbi.nlm.nih.gov/12594529/.
- Nova-Institut. 1997. Survery commissioned by Hanfgesellschaft (Berlin) for information of the European Commission. Hürth (Germany). https://eiha.org/documents/hanfgesellschaft-01-12-1997/.
- OECD. 2003. Descriptions of Selected Key Generic Terms Used in Chemical Hazard/Risk Assessment, ENV/JM/MONO (2003) 15, OECD Series on Testing and Assessment, No. 44. 13 p. http://www.oecd.org/officialdocuments/displaydocumentpdf/?cote=ENV/JM/MONO(2003)15&doclanguage=en; (accessed 16 Mar 2021).
- Palatini P, Benetti E, Mos L, Garavelli G, Mazzer A, Cozzio S, Fania C, Casiglia E. 2015. Association of coffee consumption and CYP1A2 polymorphism with risk of impaired fasting glucose in hypertensive patients. Eur J Epidemiol. 30(3):209–217.
- Palatini P, Ceolotto G, Ragazzo F, Dorigatti F, Saladini F, Papparella I, Mos L, Zanata G, Santonastaso M. 2009. CYP1A2 genotype modifies the association between coffee intake and the risk of hypertension. J Hypertens. 27(8):1594–1601.
- Pate DW. 1994. Chemical ecology of Cannabis. J Intl Hemp Assoc. 1(2):29,32–37. http://www.internationalhempassociation.org/jiha/iha01201.html.
- Pellesi L, Licata M, Verri P, Vandelli D, Palazzoli F, Marchesi F, Cainazzo MM, Pini LA, Guerzoni S. 2018. Pharmacokinetics and tolerability of oral cannabis preparations in patients with medication overuse headache (MOH)-a pilot study . Eur J Clin Pharmacol. 74(11):1427–1436.
- Petro DJ, Ellenberger JC. 1981. Treatment of human spasticity with delta 9-tetrahydrocannabinol. J Clin Pharmacol. 21(S1):413S–416S.
- Pichini S, Mannocchi G, Gottardi M, Pérez-Acevedo AP, Poyatos L, Papaseit E, Pérez-Mañá C, Farré M, Pacifici R, Busardò FP. 2020. Fast and sensitive UHPLC-MS/MS analysis of cannabinoids and their acid precursors in pharmaceutical preparations of medical cannabis and their metabolites in conventional and non-conventional biological matrices of treated individual. Talanta. 209:120537.
- Plasse TF, Gorter RW, Krasnow SH, Lane M, Shepard KV, Wadleigh RG. 1991. Recent clinical experience with dronabinol. Pharmacol Biochem Behav. 40(3):695–700.
- Poyatos L, Pérez-Acevedo AP, Papaseit E, Pérez-Mañá C, Martin S, Hladun O, Siles A, Torrens M, Busardo FP, Farré M. 2020. Oral administration of cCannabis and Δ-9-tetrahydrocannabinol (THC) preparations: a systematic review. Medicina. 56(6):309.
- Public Health England. 2016. Alcohol Risk Assessment. Messages for NHS Health Check. D. Lavoie, Alcohol Programme Manager. https://www.healthcheck.nhs.uk/search-results/?search=alcohol&submit=.
- Qian Y, Gurley BJ, Markowitz JS. 2019. The potential for pharmacokinetic interactions between cannabis products and conventional medications. J Clin Psychopharmacol. 39(5):462–471.
- Ramaekers JG, Moeller MR, van Ruitenbeek P, Theunissen EL, Schneider E, Kauert G. 2006. Cognition and motor control as a function of Delta9-THC concentration in serum and oral fluid: limits of impairment. Drug Alcohol Depend. 85(2):114–122.
- Renwick AG, Lazarus NR. 1998. Human variability and noncancer risk assessment – an analysis of the default uncertainty factor. Regul Toxicol Pharmacol. 27(1 Pt 2):3–20.
- Roth NB. 2014. Δ9-Tetrahydrocannabinolsäure A – Studien und Untersuchungen zur Anwendung als Cannabis-Konsummarker in der forensischen Toxikologie [Δ9-Tetrahydrocannabinolic acid A – studies and investigations for an application as a cannabis consumption marker in forensic toxicology]. Dissertation, University Freiburg i.Br. German. Abstract English: https://freidok.uni-freiburg.de/data/9551.
- Russo EB. 2007. History of cannabis and its preparations in Saga, Science, and Sobriquet. Chem Biodivers. 4(8):1614–1648.
- Sachse-Seeboth C, Pfeil J, Sehrt D, Meineke I, Tzvetkov M, Bruns E, Poser W, Vormfelde SV, Brockmöller JC. 2009. Interindividual variation in the pharmacokinetics of Delta9-tetrahydrocannabinol as related to genetic polymorphisms in CYP2C9. Clin Pharmacol Ther. 85(3):273–276.
- Saloner R, Cysique LA. 2017. HIV-associated neurocognitive disorders: a global perspective. J Int Neuropsychol Soc. 23(9–10):860–869.
- Sarne Y, Toledano R, Rachmany L, Sasson E, Doron R. 2018. Reversal of age-related cognitive impairments in mice by an extremely low dose of tetrahydrocannabinol. Neurobiol Aging. 61:177–186.
- Sarne Y. 2019. Beneficial and deleterious effects of cannabinoids in the brain: the case of ultra-low dose THC. Am J Drug Alcohol Abuse. 45(6):551–562.
- Sharma P, Murthy P, Bharath MM. 2012. Chemistry, metabolism, and toxicology of cannabis: clinical implications. Iran J Psychiatry. 7(4):149–156.
- Silva DA, Pate DW, Clark RD, Davies NM, El-Kadi AOS, Löbenberg R. 2020. Phytocannabinoid drug-drug interactions and their clinical implications. Pharmacol Ther. 215(November):107621–107621.
- Skoczinski P, Carus M, Grotenhermen F, Beitzke B, Kruse D. 2019. Limit and guideline values for THC (tetrahydrocannabinol) in hemp foods, nova Institut für Ökologie und Innovation, Hürth, Germany. http://news.bio-based.eu/limit-and-guideline-values-for-thc-tetrahydrocannabinol-in-hemp-foods/.
- Stout SM, Cimino MN. 2014. Exogenous cannabinoids as substrates, inhibitors, and inducers of human drug metabolizing enzymes: a systematic review. Drug Metab Rev. 46(1):86–95.
- Strasser F, Luftner D, Possinger K, Ernst G, Ruhstaller T, Meissner W, Ko Y-D, Schnelle M, Reif M, Cerny T. 2006. Comparison of orally administered cannabis extract and Delta-9-tetrahydrocannabinol in treating patients with cancer-related anorexia-cachexia syndrome: a multicenter, phase III, randomized, double-blind, placebo-controlled clinical trial from the Cannabis-In-Cachexia-Study-Group. JCO. 24(21):3394–3400.
- Struwe M, Kaempfer SH, Geiger CJ, Pavia AT, Plasse TF, Shepard KV, Ries K, Evans TG. 1993. Effect of dronabinol on nutritional status in HIV infection. Ann Pharmacother. 27(7–8):827–831.
- Thompson GR, Rosenkrantz H, Schaeppi UH, Braude MC. 1973. Comparison of acute oral toxicity of cannabinoids in rats, dogs and monkeys. Toxicol Appl Pharmacol. 25(3):363–372.
- Tönnes S, Auwärter V, Knoche A, Skopp G. 2016. Stand der wissenschaftlichen Erkenntnisse zur Feststellung einer mangelhaften Trennung von Cannabiskonsum und Fahren anhand der Konzentration von Tetrahydrocannabinol [Scientific evaluation on the ability to separate driving from cannabis consumption on the basis of tetrahydrocannabinol (THC) concentrations in blood serum]. Blutalkohol [Alcohol, Drugs, Behavior and Traffic Safety]. 53:409–414. German, English summary. https://www.bads.de/wissen/fachzeitschrift-blutalkohol/archiv-2002-2016/.
- Unimed Pharmaceuticals Inc. 2004. Marinol® (Dronabinol) Capsules, NDA 18-651/S-021, 500012 Rev Sep 2004.
- US Public Health Service, Dept of Health & Human Services (HHS). 2018. Letter to the DEA. Basis for the recommendation to place cannabidiol in Schedule V of the Controlled Substances Act, Chapter “Human Potential Abuse Study (Study #GWEP1431)” p. 9–13. See also: https://hempindustrydaily.com/fda-cbd-shouldnt-be-a-controlled-substance-but-treaties-require-it/.
- Vandrey R, Herrmann ES, Mitchell JM, Bigelow GE, Flegel R, LoDico C, Cone EJ. 2017. Pharmacokinetic profile of oral cannabis in humans: blood and oral fluid disposition and relation to pharmacodynamic outcomes. J Anal Toxicol. 41(2):83–99.
- Wall ME, Perez-Reyes M. 1981. The metabolism of delta 9-tetrahydrocannabinol and related cannabinoids in man. J Clin Pharmacol. 21(S1):178S–189S.
- Watanabe K, Yamaori S, Funahashi T, Kimura T, Yamamoto I. 2007. Cytochrome P450 enzymes involved in the metabolism of tetrahydrocannabinols and cannabinol by human hepatic microsomes. Life Sci. 80(15):1415–1419.
- WHO (World Health Organization), Expert Committee on Drug Dependence (ECDD). 2018. Critical Review, Delta-9-tetrahydrocannabinol. Section 3: Toxicology. https://www.who.int/medicines/access/controlled-substances/THCv1.pdf.
- WHO (World Health Organization), Joint FAO/WHO Expert Committee on Food Additives (JECFA). 2012. Food Additive Series: 65, Safety evaluation of certain food additives and contaminants/prepared by the Seventy-fourth meeting of the JECFA, p. 308.
- WHO (World Health Organization), Joint FAO/WHO Expert Committee on Food Additives (JECFA). 2017. Guidance document for the establishment of Acute Reference Dose (ARfD) for veterinary drug residues in food. Geneva. ISBN 978-92-4-151262-6.
- Widman M, Nilsson IM, Nilsson JLG, Agurell S, Borg H, Granstrand B. 1973. Plasma protein binding of 7-hydroxy- 1-tetrahydrocannabinol: an active 1-tetrahydrocannabinol metabolite. J Pharm Pharmacol. 25(6):453–457.
- Wikipedia. 2021. https://en.wikipedia.org/wiki/Cannabidiol; (accessed 16 Mar 2021).
- Wikipedia. 2021a. https://en.wikipedia.org/wiki/Cannabis_in_Uruguay; (accessed 16 Mar 2021).
- Wikipedia. 2021b. https://en.wikipedia.org/wiki/Cannabis_in_the_United_States; (accessed 16 Mar 2021).
- Wikipedia. 2021c. https://en.wikipedia.org/wiki/Cannabis_in_Luxembourg; (accessed 16 Mar 2021).
- Wikipedia. 2021d. https://en.wikipedia.org/wiki/Cannabis_in_the_Netherlands; (accessed 16 Mar 2021).
- Wikipedia. 2021e. https://en.wikipedia.org/wiki/Cannabis_in_Spain; (accessed 16 Mar 2021).
- Wikipedia. 2021f. https://en.wikipedia.org/wiki/Cannabis_in_Portugal; (accessed 16 Mar 2021).
- Wikipedia. 2021g. https://en.wikipedia.org/wiki/CannabisinAustria. (Accessed 16 March 2021).
- Wikipedia. 2021h. https://en.wikipedia.org/wiki/Cannabis_in_Switzerland; (accessed 16 Mar 2021).
- Wikipedia. 2021i. https://en.wikipedia.org/wiki/Cannabis_in_France; (accessed 16 Mar 2021).
- Wikipedia. 2021j. https://en.wikipedia.org/wiki/Cannabis_in_Sweden; (accessed 16 Mar 2021).
- Williams P. 2020. https://www.hempgrower.com/article/european-parliament-votes-bring-hemp-thc-back-down-lower-percent/.
- Wohlfarth A. 2012. Pharmakokinetik und Metabolismus von Δ9-Tetrahydrocannabinolsäure A (THCA) im Menschen [Pharmacokinetics and metabolism of Δ9-Tetrahydrocannabinolic acid A (THCA) in Humans]. Dissertation. URN: urn:nbn:de:bsz:25-opus-85046. German. Abstract English: https://freidok.uni-freiburg.de/data/8504.
- Wu MM, Zhang X, Asher MJ, Thayer SA. 2019. Druggable targets of the endocannabinoid system: implications for the treatment of HIV-associated neurocognitive disorder. Brain Res. 1724:146467.
- Yamaori S, Ebisawa J, Okushima Y, Yamamoto I, Watanabe K. 2011. Potent inhibition of human cytochrome P450 3A isoforms by cannabidiol: role of phenolic hydroxyl groups in the resorcinol moiety. Life Sci. 88(15–16):730–736.
- Zoller O, Rhyn P, Zimmerli B. 2000. High-performance liquid chromatographic determination of Δ9-tetrahydrocannabinol and the corresponding acid in hemp containing foods with special regard to the fluorescence properties of Δ9-tetrahydrocannabinol. J Chromatogr A. 872(1–2):101–110.
- Zuardi AW, Hallak JEC, Crippa JAS. 2012. Interaction between cannabidiol (CBD) and Δ(9)-tetrahydrocannabinol (THC): influence of administration interval and dose ratio between the cannabinoids. Psychopharmacology (Berl). 219(1):247–249.
- Zuardi AW, Shirakawa I, Finkelfarb E, Karniol IG. 1982. Action of cannabidiol on the anxiety and other effects produced by delta 9-THC in normal subjects . Psychopharmacology (Berl). 76(3):245–250.
- Zuurman L, Ippel AE, Moin E, van Gerven JMA. 2009. Biomarkers for the effects of cannabis and THC in healthy volunteers. Br J Clin Pharmacol. 67(1):5–21.