Abstract
Bisphenol A (BPA) is a ubiquitous chemical compound constantly being released into the environment, making it one of the most persistent endocrine-disrupting chemical (EDC) in nature. This EDC has already been associated with developing various pathologies, such as diabetes, obesity, and cardiovascular, renal, and behavioral complications, among others. Therefore, over the years, BPA has been replaced, gradually, by its analog compounds. However, these compounds are structurally similar to BPA, so, in recent years, questions have been raised concerning their safety for human health. Numerous investigations have been performed to determine the effects BPA substitutes may cause, particularly during pregnancy and prenatal life. On the other hand, studies investigating the association of these compounds with the development of cardiovascular diseases (CVD) have been developed. In this sense, this review summarizes the existing literature on the transgenerational transfer of BPA substitutes and the consequent effects on maternal and offspring health following prenatal exposure. In addition, these compounds’ effects on the cardiovascular system and the susceptibility to develop CVD will be presented. Therefore, this review aims to highlight the need to investigate further the safety and benefits, or hazards, associated with replacing BPA with its analogs.
1. Introduction
Endocrine-disrupting chemicals (EDCs) are natural or synthetic exogenous chemical compounds (Ghassabian and Trasande Citation2018). They can interact with the various constituents of the endocrine system and, consequently affect several biological processes and the homeostasis of organisms. Furthermore, EDCs are ubiquitously found in the environment, so humans are constantly being exposed to them by various exposure pathways, such as ingestion, dermal absorption, inhalation, or through parenteral, sublingual, and maternal–fetal routes. Therefore, human health can be impaired by the action of EDCs at different levels, such as cardiovascular, reproductive, neurological, immune, and developmental (Diamanti-Kandarakis et al. Citation2009; Vandenberg et al. Citation2014; Rahman et al. Citation2016; Ghassabian and Trasande Citation2018; Kelley et al. Citation2019; Gingrich et al. Citation2020; Malaisé et al. Citation2020; Bakoyiannis et al. Citation2021; Banker et al. Citation2021; Kiess et al. Citation2021; Lorigo and Cairrao Citation2022; O'Shaughnessy et al. Citation2021).
Bisphenol A (4-[2-(4-hydroxyphenyl)propan-2-yl]phenol; BPA) is a synthetic monomer that is a constituent of many polycarbonate plastics, epoxy resins, and consumer products, such as food containers, water pipes, toys, bottles, medical, and electronic equipment (Chen et al. Citation2016; Eckardt and Simat Citation2017; Pelch et al. Citation2019; Zhang, Shan, et al. Citation2020; Lorigo and Cairrao Citation2022). This compound is a pseudo-persistent chemical. Although BPA has a short half-life, it is considered one of the most common EDCs in the environment, because it is constantly being released into it (Flint et al. Citation2012; Rochester and Bolden Citation2015; Zhang, Shan, et al. Citation2020). Indeed, this EDC has already been detected in various human and animal biological samples and has been associated with the development of various pathologies, such as diabetes, obesity and cardiovascular, renal and behavioral complications, among others (Flint et al. Citation2012; Eladak et al. Citation2015; Rochester and Bolden Citation2015; Chen et al. Citation2016; Gramec Skledar and Peterlin Mašič Citation2016; Pelch et al. Citation2019; Zhang, Shan, et al. Citation2020; Lorigo and Cairrao Citation2022). Therefore, over the years, this compound has gradually been replaced by its analogs, such as bisphenol S (4-(4-hydroxyphenyl)sulfonylphenol; BPS), bisphenol F (4-[(4-hydroxyphenyl)methyl]phenol; BPF), bisphenol AF (4-[1,1,1,3,3,3-hexafluoro-2-(4-hydroxyphenyl)propan-2-yl]phenol; BPAF), bisphenol E (4-[1-(4-hydroxyphenyl)ethyl]phenol; BPE), bisphenol B (4-[2-(4-hydroxyphenyl)butan-2-yl]phenol; BPB), bisphenol AP (4-[1-(4-hydroxyphenyl)-1-phenylethyl]phenol; BPAP), bisphenol Z (4-[1-(4-hydroxyphenyl)cyclohexyl]phenol; BPZ), bisphenol P (4-[2-[4-[2-(4-hydroxyphenyl)propan-2-yl]phenyl]propan-2-yl]phenol; BPP), bisphenol HPF (4-[9-(4-[9-(4-hydroxyphenyl)fluoren-9-yl]phenol; BHPF) (Liao, Liu, Guo, et al. Citation2012; Rosenmai et al. Citation2014; Chen et al. Citation2016; Gramec Skledar and Peterlin Mašič Citation2016; Pelch et al. Citation2019; Zhang, Zhang, et al. Citation2019; Mi et al. Citation2020; ). Indeed, these compounds have been used, on a large scale, in numerous everyday objects and have been detected in different environmental substrates, including sediments (Liao, Liu, Moon, et al. Citation2012), water (Yamazaki et al. Citation2015; Zhang, Zhang, et al. Citation2019), sewage (Song, Song, et al. Citation2014; Česen et al. Citation2018), and dust (Liao, Liu, Guo, et al. Citation2012), which means that humans are continuously and persistently exposed to them (Liao, Liu, Kannan Citation2012; Liao and Kannan Citation2013; Rosenmai et al. Citation2014; Chen et al. Citation2016; Gramec Skledar and Peterlin Mašič Citation2016; Björnsdotter et al. Citation2017; Eckardt and Simat Citation2017; Wu et al. Citation2018; Pelch et al. Citation2019). However, since BPA substitutes are structurally similar to BPA, doubts have been raised in recent years as to their safety for human health (Rochester and Bolden Citation2015; Chen et al. Citation2016; Wu et al. Citation2018; Pelch et al. Citation2019).
Hence, over the last few years, several studies have been carried out in animal models and in humans to assess the effects that BPA substitutes may have in periods of greater vulnerability in life, such as pregnancy and prenatal development (Catanese and Vandenberg Citation2017; Kolatorova et al. Citation2017; Grandin et al. Citation2018; Wan et al. Citation2018; Huang et al. Citation2019; Li et al. Citation2019; Pan et al. Citation2020). Furthermore, since the association of BPA with the development of some pathologies – such as those related to the cardiovascular system – is already documented (Melzer et al. Citation2010; Gore et al. Citation2015; Zhang, Shan, et al. Citation2020; Lorigo and Cairrao Citation2022; Moon et al. Citation2021), some investigations have been developed to assess the impact of BPA analogs on this system (Gao et al. Citation2015; Ferguson M et al. Citation2019; Zhang, Shan, et al. Citation2020).
In this sense, this review summarizes the existing literature on the transgenerational transfer of different BPA substitutes and consequent maternal and offspring health effects associated with prenatal exposure to these EDCs. Furthermore, the effects of exposure to these compounds on the cardiovascular system and the propensity to develop cardiovascular diseases (CVDs) are summarized. Therefore, this review aims to highlight the need to continue to conduct studies to assess safety more rigorously, and the benefits or hazards that exist in replacing BPA with its analogs.
2. Approach to the review
In this review, experimental studies that have evaluated the effects of prenatal exposure to BPA substitutes on maternal and fetal health in animal and human models were presented. Furthermore, the studies regarding the cardiovascular effects of these substitutes are summarized. Overall, the topics discuss the effects of BPA substitutes on animals (Topic 3) and humans (Topic 4) during the prenatal phase and the effects of exposure on the cardiovascular system (Topic 5). The subtopics for the studies with animals during the prenatal phase were reproductive changes; metabolism alterations; alterations in thyroid and neurological functions; alterations in behavior; morphological and survival alterations and immune system alterations. For the studies with humans, prenatal exposure was discussed and a topic describing the effects of BPA substitutes in the prenatal phase was included. Concerning the Topic of effects of exposure on the cardiovascular system, three subtopics addressed in vivo, in vitro, and epidemiological data.
A literature review was performed based on articles available in Scopus, Web of Science, PubMed, and ScienceDirect (Elsevier) databases. The search strategy was carried out using Boolean operators “AND”, “OR”, and “NOT” and a combination of terms relating to BPA substitutes (“bisphenol A substitutes”, “bisphenol A analogs”, “endocrine disruptor compound”, “bisphenol F”, and “bisphenol S”) with:
prenatal exposure (“prenatal exposure”, “maternal exposure”, “fetal exposure”, “maternal health”, “fetal health”, “pregnancy”, “pregnant women”, “progenitors”, and “offspring”) for Topics 3 and 4;
cardiovascular system (“cardiovascular system”, “arteries”, “heart”, “vascular”, “smooth muscle”, “cardiac”, “smooth muscle cells”) and cardiovascular outcomes (“cardiovascular diseases”, “hypertension”, “arrhythmia”, “heart rate variability”, “cardiovascular dysfunction”, “heart failure”, “blood pressure”) regarding Topic 5.
In addition to these terms, search-relevant citations of the articles retrieved were also included.
Regarding the prenatal effects of BPA substitutes on animals (Topic 3), the inclusion criteria were: 1) the article was an original article; 2) the study was performed using animal model of prenatal exposure; 3) the concentrations of exposure to BPA substitutes was well defined; 4) the study also evaluated the effects of prenatal exposure to BPA and other EDCs; 5) the investigation had a control group of animals not exposed to BPA substitutes; 6) the study assessed the transgenerational transfer of BPA substitutes; 7) the study outcomes included the effects for maternal and/or fetal health; 8) the article was written in English. For this topic, the exclusion criteria were: 1) the article was not an original article (e.g. review, editorial, and commentary); 2) the study was performed on humans; 3) the study only used animals that presented previous diseases or susceptible conditions; 4) the concentrations of exposure to BPA substitutes was not well established; 5) the exposure to BPA substitutes did not occur during pregnancy/prenatal phase (e.g. post-natal, young, or adult exposure); 6) the study did not determine the effects of prenatal exposure to BPA substitutes; 7) articles were duplicates, unrelated, inaccessible, or not written in English.
Concerning the prenatal effects of BPA substitutes on humans (Topic 4), the inclusion criteria were: 1) the article was an original article; 2) the study was performed on pregnant women and their fetuses; 3) the study was performed on human placental models; 4) the exposure to BPA substitutes was evaluated in maternal and/or fetal biological samples; 5) the exposure to BPA substitutes was determined over the different trimesters; 6) the study also assessed the prenatal exposure to BPA and other EDCs; 7) the study outcomes included the effects for maternal and/or fetal health; 8) the article was written in English. For this topic, the exclusion criteria were: 1) the article was not an original article (e.g. review, editorial, and commentary); 2) the study was performed on animals, non-pregnant women, or men; 3) the study population was not well characterized; 4) the exposure to BPA substitutes was not measured during and/or after pregnancy; 5) articles were duplicates, unrelated, inaccessible, or not written in English.
Finally, regarding the cardiovascular effects of exposure to BPA substitutes (Topic 5), the inclusion criteria were: 1) the article was an original article; 2) the study was performed on in vivo animal models, in vitro models, or using epidemiological data; 3) the concentrations of exposure to BPA substitutes was well defined; 4) the studies had a control group; 5) the study determined the cardiovascular outcomes associated with exposure to BPA substitutes; 6) the study also assessed the effects associated with exposure to BPA and other EDCs; 7) the study evaluated the effects of BPA substitutes on several organ systems and characteristics; 8) the article was written in English. For this topic, the exclusion criteria were: 1) the article was not an original article (e.g. review, editorial, and commentary); 2) studies including previous diseases or susceptible conditions without a control group; 3) the concentrations of exposure to BPA substitutes was not well established; 4) the study did not determine the effects for cardiovascular health associated with exposure to BPA substitutes; 5) articles were duplicates, unrelated, inaccessible, or not written in English.
These inclusion and exclusion criteria were applied when the title, abstract, and materials and methods of each article retrieved were analyzed.
3. Exposure to BPA substitutes in animals during the prenatal phase
Analyzing the effects caused by various EDCs, such as BPA-substitutes, during pregnancy and lactation periods is essential (Catanese and Vandenberg Citation2017). This is because these are both stages of development with greater vulnerability and sensitivity to endocrine disruption, which can consequently cause serious harm to mothers and their offspring (Catanese and Vandenberg Citation2017). However, the study of the effects of EDCs on humans is not always an easy task, especially in such periods of life. Indeed, in prenatal life, access to biological samples, such as placenta, amniotic fluid, and fetal blood, among others, is not simple. In this sense, model organisms (e.g. rats, mice, zebrafish, ewes, and pigs) are often used as predictive models of toxicity to discover the effects that these compounds cause and subsequently extrapolate the conclusions obtained to humans (Gore et al. Citation2015; Patisaul et al. Citation2018; Rolfo et al. Citation2020; Yang, Song, et al. Citation2020). However, care and attention must be taken when extrapolating these results, as the complexity and functioning of biological systems across species are not always the same (Gore et al. Citation2015; Grandin et al. Citation2018; Patisaul et al. Citation2018; Mao et al. Citation2020).
Therefore, over the years, several studies have been performed in animal models to determine toxicokinetic parameters of BPA substitutes and the existence of transgenerational transfer of them (Cabaton et al. Citation2006; Yabusaki et al. Citation2015; Gingrich et al. Citation2018; Grandin et al. Citation2018; Mao et al. Citation2020).
In 2006, Cabaton et al. performed, for the first time, a study in which they administered doses of BPF (low dose − 7 mg/kg BW; high dose − 100 mg/kg BW) to pregnant (administered on day 17th of pregnancy) and nonpregnant Sprague–Dawley rats to monitor various parameters over 96 h (Cabaton et al. Citation2006). Overall, the authors demonstrated that regardless of the gestational state, in pregnant and nonpregnant rats, the main pathway of BPF excretion is urinary, although some fecal excretion can also be observed. The results also showed that, in this animal model, BPF and its metabolites undergo enterohepatic circulation since they can be detected in the bile content. However, this process slows down the elimination of these EDCs and increases the exposure time. On the other hand, the authors also reported that, in pregnant and nonpregnant rats, the accumulation of these compounds in tissues was less than 1% of which around half were detected in the liver. However, the major difference between the two groups of animals is related to the higher accumulation of BPF residues in the uterus of pregnant rats (nonpregnant rats – <0.01%; pregnant rats − 0.07% and 0.18% for the low and high dose, respectively). This accumulation in the uterus, together with the higher detection of residues of this EDC in the placenta, fetus, and amniotic fluid than in the maternal blood, suggests a maternal–fetal transfer of this compound. In addition, the researchers also discovered that, after 96 h, 1.2% of the administered dose could be detected in the fetus, namely in their developing brain and liver (Cabaton et al. Citation2006). Therefore, although some caution is needed in transposing conclusions to humans (as the constitution and anatomy of placentas are different), BPF can be transferred through the rat placenta and potentially cause problems to fetus’ health, mainly in the liver and neurological levels (Cabaton et al. Citation2006). Later, in 2015, Yabusaki et al. performed a study on pregnant C57BL/6 mice and their fetuses and found that in both, the hepatic metabolization of BPAF (7.5 μM) by the enzyme UDP-glucuronosyltransferase (UGT) is reduced (Yabusaki et al. Citation2015). Therefore, this compound will tend to bioaccumulate in both the mother and the fetus during the perinatal period. In addition, the authors observed similar results for BPF (7.5 μM), although to a lesser extent. Thus, it has been shown that in addition to BPF (Cabaton et al. Citation2006), BPAF can also be transferred to the next generation, which led the authors to question whether these compounds are safe alternatives to BPA (Yabusaki et al. Citation2015). More recently, in 2018, Grandin et al. also evaluated the placental transfer of BPS and its main metabolite (BPSG) in ewes after intravenous exposure to mothers (2.7 mg/kg BPSG and 5 mg/kg BPS after 3 d) and their fetuses (5 mg BPS, and 17.5 mg BPSG after 3 d) (Grandin et al. Citation2018). These researchers found that only 0.40% of the dose of BPS present in the mothers was transferred to the fetus. However, once present in the fetal compartment, 46% of BPS is slowly eliminated in the form of BPSG, which increases its persistence and accumulation in fetal circulation. Furthermore, the researchers observed that after maternal and fetal administrations, BPS concentrations in amniotic fluid and plasma were similar, in contrast to BPSG, which was 6 times more present in plasma. Therefore, the authors concluded that both compounds could be excreted into the amniotic fluid, exposing the fetus to these EDCs, either by amniotic fluid ingestion, or dermal absorption. The results of this study are of particular concern since, in humans, fewer placental barriers are separating the maternal and fetal compartments, which may imply higher human fetal exposure to BPS, although further studies are needed to prove this assumption (Grandin et al. Citation2018). Furthermore, in 2018, Gingrich et al. also performed a study on ewes, where mothers were exposed to BPS through daily subcutaneous injections (0.5 mg/kg/d) from days 30th to 100th of pregnancy (Gingrich et al. Citation2018). In this work, it was again demonstrated that this EDC could cross the placental barrier and promote placental endocrine disruption, directly and indirectly affecting the fetus’s development by reducing circulating levels of glycoproteins associated with pregnancy. In ewes, this is of concern as these glycoproteins are important for maintaining pregnancy, so a lack of them can have profound consequences. Furthermore, the results revealed that exposure to this EDC decrease the expression of fusogenic genes and placental e-cadherin proteins (responsible for forming adhesion junctions). These events lead to a reduction in the fusion process of placental trophoblasts and, consequently, to a decrease in the number of binucleated trophoblasts cells, which are essential for the normal endocrine functioning of this organ in ewes. Interestingly, it was discovered that the effects caused by BPS were more pronounced in the placentas of male fetuses than female fetuses, which shows that gender can influence the action of these compounds. However, the authors did not propose any explanation for this relationship with offspring’ gender (Gingrich et al. Citation2018). In 2020, Mao et al. evaluated the influence of BPS on C57BL6J mice’s placenta following mothers’ exposure via ingestion (200 µg/kg BW) (Mao et al. Citation2020). This EDC was found to cause genetic and morphological changes in the placental junctional region (Mao et al. Citation2020). This placental zone, in mice, serves as a nutritional and energy store for the fetus and placenta and is considered the main endocrine component of this organ (Woods et al. Citation2018). In addition, significant variations in the content of the neurotransmitters serotonin (5-HT), dopamine (DA), and their metabolites have been observed in the placenta (Mao et al. Citation2020). According to the authors, these changes may be related to the morphological alterations observed, which may influence offspring’s neurodevelopment (Mao et al. Citation2020). Furthermore, it is important to note that alterations in the concentration of neurotransmitters in the placenta, mainly 5-HT, seem to be related to the development of cardiovascular pathologies (e.g. preeclampsia) in pregnant women (Gumusoglu et al. Citation2021).
3.1. Reproductive changes
Over the years, several research works have also been performed to examine the effects of BPA substitutes on various health levels. Between 2013 and 2021, some studies were performed in zebrafish (Danio rerio) to evaluate the effects of BPS (Ji et al. Citation2013; Qin et al. Citation2021), BPF (Yang et al. Citation2017a, Citation2017b), BPAF (Shi et al. Citation2015), and BPB (Yang et al. Citation2017a, Citation2017b) on the hypothalamic-pituitary-gonadal axis (HPG) and in several factors related to reproduction and fertility of F0 generations, such as hormonal changes and alterations in gene expression (Ji et al. Citation2013; Shi et al. Citation2015; Yang et al. Citation2017a, Citation2017b), egg production and gonadosomatic index (GSI = gonad weight × 100/body weight) (Ji et al. Citation2013; Yang et al. Citation2017a, Citation2017b; Qin et al. Citation2021).
Overall, the F0 generation’s exposure to the four BPA substitutes mentioned above has been observed to disturb the hormonal balance in these animals (Ji et al. Citation2013; Shi et al. Citation2015; Yang et al. Citation2017a, Citation2017b). In other words, these EDCs were found to promote increased concentrations of 17β-estradiol (E2) present in maternal plasma (Ji et al. Citation2013; Shi et al. Citation2015) and in the homogenates of combined anterior and posterior parts of male and female F0 fish (Yang et al. Citation2017a, Citation2017b). As for testosterone (T) concentrations, the results are not so concordant. In two studies, the presence of BPF or BPB was inversely related to T concentrations (Yang et al. Citation2017a, Citation2017b), and in the studies where plasma samples were evaluated, no significant relationships were established between the concentrations of BPS and BPAF and this parameter (Ji et al. Citation2013; Shi et al. Citation2015). Concerning the progesterone concentrations, in the study performed by Yang et al. no significant concentrations changes were observed (Yang et al. Citation2017a, Citation2017b). The ability of these EDCs to alter the gene expression of various gene sets – such as those related to the HPG axis – was also assessed (Ji et al. Citation2013; Shi et al. Citation2015; Yang et al. Citation2017a, Citation2017b). The exposure of F0 generations to BPA substitutes can promote downregulation of gene expression of genes present in the brain (e.g. gnrh3, fshβ, cyp19a1b, erα, and gnrhr2) and ovary samples (e.g. hmgra, hmgrb, fshr, star, cyp11a, and lhr) (Ji et al. Citation2013; Shi et al. Citation2015; Yang Citation2017a, Citation2017b). Therefore, these results are suggestive that, in zebrafish, one of the mechanisms of disruption of these compounds is related to these genomic alterations that, subsequently, end up impairing several cellular processes, such as steroidogenesis, gametogenesis, and oocyte maturation (Ji et al. Citation2013; Shi et al. Citation2015; Yang et al. Citation2017a, Citation2017b). On the other hand, it is also relevant to determine parameters, such as egg production or GSI, since these, in addition to the factors mentioned above, may be indicators of possible sexual dysfunctions associated with the action of BPA substitutes (Ji et al. Citation2013; Yang et al. Citation2017a, Citation2017b). In this sense, some investigations reported exposure of F0 generations to BPS (Ji et al. Citation2013; Qin et al. Citation2021), BPF (Yang et al. Citation2017a, Citation2017b), and BPB (Yang et al. Citation2017a, Citation2017b) related to lower egg production. Regarding GSI, the same relationship was observed (Ji et al. Citation2013; Yang et al. Citation2017a, Citation2017b), except in the study performed by Qin et al. where higher concentrations of BPS were found to be directly related to GSI. However, in this investigation for lower concentrations of BPS, no significant changes in GSI were observed compared to control (Qin et al. Citation2021). The variations detected for these two parameters, according to the authors, seem to be explained by the endocrine-disrupting action that these compounds exert on hormone homeostasis, gonadal structure, and growth, and on the decrease in the number of viable gametes (Ji et al. Citation2013; Yang et al. Citation2017a, Citation2017b; Qin et al. Citation2021). Therefore, it is understood that mothers’ fertility is affected by these EDCs, which inevitably affects offspring’s survival (Shi et al. Citation2015; Qin et al. Citation2021). However, according to Yang et al. and Qin et al. BPF and BPS can make oocytes unviable by other mechanisms than disrupting the HPG axis (Yang et al. Citation2017a, Citation2017b; Qin et al. Citation2021). The results seem to reveal that BPF inhibits follicular growth and ovarian maturation, reducing the number of mature oocytes (Yang et al. Citation2017a, Citation2017b). On the other hand, the disruption of lipid metabolism promoted by BPS seems responsible for the premature maturation of oocytes, making them less viable (Qin et al. Citation2021). In other words, the excessive use of lipids as an energy source for oocyte maturation leads to an insufficient lipid stock necessary for normal egg development (Qin et al. Citation2021).
In addition, between 2016 and 2019, several studies in rodents have been developed with the aim of assessing the influence that BPA substitutes, such as BPS (Hill et al. Citation2017; LaPlante et al. Citation2017; Shi et al. Citation2018; Tucker et al. Citation2018; da Silva et al. Citation2019; Shi, Sekulovski, et al. Citation2019; Shi, Whorton, et al. Citation2019a, Citation2019b; Ullah et al. Citation2019), BPF (Ohtani et al. Citation2017; Ullah et al. Citation2019), BPB (Ullah et al. Citation2019), BPE (Shi et al. Citation2018; Shi, Sekulovski, et al. Citation2019; Shi, Whorton, et al. Citation2019a, Citation2019b), and BPAF (Li et al. Citation2016; Tucker et al. Citation2018) have on the reproduction and fertility of dams and their offspring. LaPlante et al. observed that dams’ exposure to BPS can cause hormonal changes in them, although this is not one of the most commonly assessed parameters (LaPlante et al. Citation2017; da Silva et al. Citation2019). Moreover, the authors found that higher BPS concentration tended to decrease E2 concentrations in maternal serum (LaPlante et al. Citation2017). In the study performed by da Silva et al., the same association was found for maternal plasma samples, although statistical significance was not obtained (da Silva et al. Citation2019). However, in this work, it was possible to observe an inverse relationship between the concentration of this EDC and the concentration of T in maternal plasma (da Silva et al. Citation2019). Furthermore, variations in dams’ body weight throughout pregnancy can be assessed; however, it was found that exposure to BPS, BPF, and BPB does not seem to be associated with this physiological parameter (da Silva et al. Citation2019; Ullah et al. Citation2019; Brulport et al. Citation2021). Nonetheless, according to Li et al. daily consumption of BPAF by dams causes their body weight to decrease during pregnancy compared to control dams (Li et al. Citation2016).
Regarding the effects on the reproductive system and HPG axis of rodent offspring, studies are more extensive and wide-ranging (Li et al. Citation2016; Hill et al. Citation2017; Ohtani et al. Citation2017; Shi et al. Citation2018; Tucker et al. Citation2018; da Silva et al. Citation2019; Shi, Sekulovski, et al. Citation2019; Shi, Whorton, et al. Citation2019a, Citation2019b; Ullah et al. Citation2019). Overall, studies have monitored characteristics and parameters such as hormone concentrations, gene expression, tissue morphology, tissue, and organ organization, the onset of puberty, and fertility, after prenatal exposure to BPA substitutes (Li et al. Citation2016; Shi et al. Citation2018; Tucker et al. Citation2018; da Silva et al. Citation2019; Shi, Sekulovski, et al. Citation2019; Shi, Whorton, et al. Citation2019a, Citation2019b; Ullah et al. Citation2019). Thus, although the results are not always concordant, it has been found that dams’ exposure to BPA substitutes, namely BPS (Shi et al. Citation2018; Tucker et al. Citation2018; da Silva et al. Citation2019; Shi, Sekulovski, et al. Citation2019; Shi, Whorton, et al. Citation2019a, Citation2019b; Ullah et al. Citation2019), BPE (Shi et al. Citation2018; Shi, Sekulovski, et al. Citation2019; Shi, Whorton, et al. Citation2019a, Citation2019b), BPF (Ullah et al. Citation2019), BPB (Ullah et al. Citation2019), and BPAF (Li et al. Citation2016; Tucker et al. Citation2018) can impair offspring’s hormonal homeostasis at various periods of their development (). The administration of these EDCs to the F0 generation appears several times associated with increased E2 levels in plasma or serum of F1 (Shi et al. Citation2018; Tucker et al. Citation2018; Ullah et al. Citation2019) and F3 generations (Shi, Whorton, et al. Citation2019a, Citation2019b), although sometimes statistically significant relationships could not be established for F1 (Li et al. Citation2016; Shi et al. Citation2018; Tucker et al. Citation2018; da Silva et al. Citation2019; Shi, Sekulovski, et al. Citation2019) and F3 offspring (Shi, Whorton, et al. Citation2019a, Citation2019b). Regarding offspring’ plasma and serum concentrations of progesterone and dehydroepiandrosterone (DHEA), similar relationships were observed to those obtained for E2 (Tucker et al. Citation2018; da Silva et al. Citation2019), except in the study by da Silva et al. where decreased plasma concentrations of progesterone was associated with increased levels of vitamin D later in offspring’ life, after prenatal BPS exposure (da Silva et al. Citation2019). As for T concentrations in plasma or serum, the results are even more varied throughout life, especially in the case of female offspring: for females, their mothers’ exposure to these compounds may increase (Shi, Sekulovski, et al. Citation2019), decrease (Tucker et al. Citation2018) or not significantly influence T levels (Tucker et al. Citation2018; da Silva et al. Citation2019; Shi, Whorton, et al. Citation2019a). Whereas in male offspring this exposure appears to reduce (Tucker et al. Citation2018; Shi, Whorton, et al. Citation2019b; Ullah et al. Citation2019) or not interfere with the concentrations of this hormone (Li et al. Citation2016; Shi et al. Citation2018; Tucker et al. Citation2018; da Silva et al. Citation2019). Two studies have further assessed whether changes in pituitary hormones – luteinizing hormone (LH), and follicle-stimulating hormone (FSH) – concentrations occur in F1 offspring following prenatal exposure to BPA substitutes (Li et al. Citation2016; Ullah et al. Citation2019). They discovered that there are not always significant differences compared to controls (Li et al. Citation2016), but when these exist, the relationships established are inverse (Ullah et al. Citation2019). Finally, Li et al. discovered that prenatal exposure to BPAF decreases the concentrations of inhibin B in the serum and testes of young offspring, which may be of concern since this hormone, in rodents, is associated with the normal functioning of Sertoli cells and, consequently, with spermatogenesis (Li et al. Citation2016). In this sense, these alterations may be of concern since the hormones indicated regulate the development and functionality of various organs and systems and may even influence processes, such as the onset of puberty, and the morphology of reproductive organs and mammary glands in rodents (as will be described in greater detail below) (Tucker et al. Citation2018; Shi, Sekulovski, et al. Citation2019; Ullah et al. Citation2019; Kaimal et al. Citation2021).
Table 1. Disruption of offspring’s hormonal homeostasis at various periods of their development, following prenatal exposure to various BPA substitutes.
Furthermore, in these works, it was determined what influence BPA substitutes may have on the gene expression of gene sets associated with the HPG axis and the regulation of growth and development of offspring’s mammary gland and reproductive organs (Li et al. Citation2016; Hill et al. Citation2017; Shi et al. Citation2018; Tucker et al. Citation2018; Shi, Sekulovski, et al. Citation2019; Shi, Whorton, et al. Citation2019a, Citation2019b). The evidences showed that exposure of F0 generation to these EDCs can alter the expression of F1 generation’ genes present in samples from uterus (Igf-1) (Hill et al. Citation2017), ovary (Igf-1, Egf-r, Star, Cyp11a1, Hsd3b1, Cyp19a1, and Cyp17a1) (Hill et al. Citation2017; Shi, Sekulovski, et al. Citation2019), testes (Cd177, Star, Cyp11a1, Hsd3b1, Cyp19a1, Apaf1, Bad, Bax, Cycs, Atg3, Atg5, Atg7, Atg12, Atg16l1, Cat, Gpx4, Gsr, Sod1, Sod2, Dnmt1, Dnmt3a, Dnmt3b, Setd1a, Setd1b, Kmt2d, Kmt2e, Ezh2, Suz12, and Dot1l) (Li et al. Citation2016; Shi et al. Citation2018) and mammary gland (Esr1 and Pgr) (Tucker et al. Citation2018). In addition, exposure of the F0 generation may alter the expression of F3 generation genes present in ovarian (Cyp11a1 and Cyp17a1) (Shi, Whorton, et al. Citation2019a), testicular (Star, Cyp17a1, Hsd3b1,Cyp19a1, Dnmt1, Dnmt3a, Dnmt3b, Dnmt3l, Setd1b, Kmt2a, Kmt2b, Kmt2c, Kmt2d, Kmt2e, Dot1l, Eed, and Suz12) (Shi, Whorton, et al. Citation2019b) and mammary gland samples (Esr1 and Pgr) (Tucker et al. Citation2018). Thus, it can be seen, once again, that these compounds can affect multiple biological processes. For example, BPAF, by influencing the expression of certain genes, can interfere with steroidogenesis and spermatogenesis since it has been proven that this compound can cause various alterations in the meiotic process (Li et al. Citation2016). Furthermore, BPAF and BPS, by altering, albeit very slightly, gene expression of steroidogenic receptors located in the offspring’s mammary gland, seem to be implicated in the phenotypic alterations observed in this organ (Tucker et al. Citation2018). On the other hand, BPS, by disrupting gene expression, can interfere with folliculogenesis, increasing the number of mature follicles (Hill et al. Citation2017). In one of the studies performed by Shi et al., it was evidenced that in the F1 males, BPS and BPE, by affecting gene expression in neonatal testis cells, may promote the occurrence of meiotic errors; affect the balance of the intrinsic apoptotic pathway, causing mitochondrial dysfunction; inhibit autophagy, causing disruptions in cell growth and development; alter the activity of antioxidant enzymes, increasing oxidative stress; and modify epigenetic regulation (Shi et al. Citation2018). Later, these researchers evaluated the F3 generation to understand if the effects caused by these EDCs were transmitted transgenerationally (Shi, Whorton, et al. Citation2019b). Thus, they discovered that not all genetic changes and consequent effects, previously described for the F1 generation, could be detected in the F3 generation, although modifications in epigenetic regulation could be observed. Therefore, it is assumed that one of the mechanisms involved with the transmission between generations of the effects caused by these compounds in neonatal testis is related to variations in epigenetic inheritance, as can be seen by the modification of gene expression of DNA methyltransferases, histone methyltransferases and their associated factors, responsible for this process (Shi et al. Citation2018; Shi, Whorton, et al. Citation2019b). Shi et al. further studied the consequences for the F1 and F3 female generation after F0 animals were exposed to BPS and BPE. Even though it was possible to detect changes in the expression of steroidogenic enzymes in the ovaries of F1 females, the authors could not state with certainty if these alterations were responsible for damaging the HPG axis and, consequently, impairing the functions performed by these organs (Shi, Sekulovski, et al. Citation2019). For the F3 generation, it was found that the changes observed in the gene expression of steroidogenic enzymes are not sufficient to justify the transgenerationally transmitted consequences (Shi, Whorton, et al. Citation2019a). However, it is thought that this transmission may be associated with epigenetic changes in germ cells, although further confirmatory studies are needed (Shi, Whorton, et al. Citation2019a). Therefore, BPA substitutes, by interfering with these processes, can cause serious consequences for offspring’s cellular and organological functionality. For example, these compounds impair spermatogenesis (Li et al. Citation2016; Shi et al. Citation2018; Shi, Whorton, et al. Citation2019b; Ullah et al. Citation2019), as can be seen by their detrimental effect on the number, quality, and viability of spermatozoa (Shi et al. Citation2018; Shi, Whorton, et al. Citation2019b; Ullah et al. Citation2019) and ability to promote the occurrence of oxidative stress in the male sexual organs (Ullah et al. Citation2019). In the female offspring, these EDCs stimulate premature ovarian development, although they decrease the response of the ovary at later stages of life (Hill et al. Citation2017); affect the uterine tissue organization. However, these changes only appear to be visible after puberty (Hill et al. Citation2017); inhibit germ cell nest breakdown in the developing ovaries, which is an essential process for primordial follicle pool formation (Shi, Sekulovski, et al. Citation2019; Shi, Whorton, et al. Citation2019a); anticipate the onset of puberty (Shi, Sekulovski, et al. Citation2019); disrupt the estrous cycle (Shi, Sekulovski, et al. Citation2019; Shi, Whorton, et al. Citation2019a); accelerate mammary gland development and the occurrence of inflammation and non-neoplastic lesions in this organ (Tucker et al. Citation2018). Consequently, reproductive functions and fertility of male and female offspring may be compromised following prenatal exposure to these compounds (Shi et al. Citation2018; Shi, Sekulovski, et al. Citation2019; Shi, Whorton, et al. Citation2019a, Citation2019b; Ullah et al. Citation2019).
3.2. Metabolism alterations
To assess the influence of BPA substitutes on offspring’ lipid, glycolytic, and amino acidic metabolisms, after prenatal exposure, several studies in zebrafish (Wang W et al. Citation2019; Qin et al. Citation2021), rodents (Ivry Del Moral et al. Citation2016; Meng et al. Citation2018; Meng, Tian, et al. Citation2019; Meng, Wang, et al. Citation2019; Meng, Zhu, et al. Citation2019; Ahn et al. Citation2020; Brulport et al. Citation2021), and ewes (Pu et al. Citation2017) have been performed between 2016 and 2021.
Concerning zebrafish, egg yolk lipids are maternally supplied, metabolized, and transported into the body of the developing zebrafish larvae, where, in their gut, these molecules can be processed into lipoproteins and subsequently released and used in organ development (Wang W et al. Citation2019). Then, in 2019, Wang et al. assessed how the exposure of F0 generations to BPS disrupted this metabolic process (Wang W et al. Citation2019). After assessing the expression of several genes (e.g. apoa1a, apobb.1, apoea, mtp, lpl, pparγ, fabp1b, fabp2, and fabp11a) involved in these metabolic processes, the researchers found that although the transport of lipids from the yolk to the larvae and their processing into lipoproteins was being stimulated, the subsequent hydrolysis of these molecules and lipids’ release was diminished. Thus, they concluded that there was an accumulation of lipids in the yolk, which affected the development of these organisms (Wang et al. Citation2019). In 2021, Qin et al. also observed that prenatal exposure to BPS promoted lipid accumulation in zebrafish embryos and in larvae viscera, which consequently affected offspring development (Qin et al. Citation2021). Thus, the authors concluded that lipid metabolism was directed toward the maturation of the maternal oocytes, which consequently decreased the availability of lipids present in the eggs necessary for the offspring’s normal development.
In rodents, metabolic effects of prenatal exposure to BPA substitutes at different stages of offspring’s development and after administration of a standard (Meng et al. Citation2018; Meng, Tian, et al. Citation2019; Meng, Wang, et al. Citation2019; Meng, Zhu, et al. Citation2019; Brulport et al. Citation2021), or high-fat diet (Ivry Del Moral et al. Citation2016; Meng, Wang, et al. Citation2019; Ahn et al. Citation2020) have been assessed.
In the study by Meng et al. exposure of dams to BPS and BPAF was found to disturb the hepatic lipid metabolism of their female adolescent offspring (Meng et al. Citation2018). The authors observed that, in these animals, after prenatal exposure to BPS, triglyceride (TG) synthesis and fatty acid synthesis and transport increased, while their β-oxidation decreased. Thus, there was a lipid accumulation in the liver, as can be seen by the increased concentrations of TG, total cholesterol, and some free fatty acids in this organ. On the other hand, prenatal exposure to BPAF caused the exact opposite effects to those observed for BPS, thus reducing lipid accumulation in the liver. In this study, it was also observed that both BPS and BPAF inhibited gluconeogenesis and stimulated glycolysis and glycogen transport, which consequently decreased glucose and glycogen levels in the liver. Therefore, by interfering with these metabolic processes, these compounds can disturb the hepatic metabolism of lipids, glucose, and some amino acids. In addition, these authors evaluated the influence of BPF on metabolism; however, the changes observed were much smaller, so it is assumed that this compound has less influence on these hepatic processes (Meng et al. Citation2018). In 2019, these researchers determined the metabolic impact of these 3 EDCs in adolescent female offspring and their later life stages and demonstrated that, over the years, some metabolic disturbances are recovered (Meng, Zhu, et al. Citation2019). Although BPS, BPF, and BPAF have been shown to influence the synthesis and degradation of ketone bodies – which may consequently affect energy metabolisms, such as lipid and glycolytic metabolisms – and the metabolism of some amino acids, the effects are more pronounced in adolescence than in adulthood (Meng, Zhu, et al. Citation2019). Although the reason is unknown, this causality associated with age was discovered, so future studies are needed to clarify it. However, this situation may be associated with epigenetic and immune metabolism alterations (Meng, Zhu, et al. Citation2019). Also, in 2019, Meng et al. performed two further studies to determine the effects caused by BPS (Meng, Wang, et al. Citation2019), BPF and BPAF (Meng, Tian, et al. Citation2019) prenatal exposure on the metabolism of male mice at a later age (13 weeks of age). Regarding BPS (Meng, Wang, et al. Citation2019), similar results were obtained to those previously described for adolescent females (Meng et al. Citation2018). Therefore, it was observed that prenatal exposure to this EDC increased serum concentration of TG and alanine aminotransferase (ALT; an indicator of early liver injury), the weight of liver and epididymal white adipose tissues (epiWAT), size of epiWAT cells and expression of inflammatory factors in these tissues. Moreover, they found that in hepatocytes and epiWAT cells, the expression of genes encoding proteins involved in fatty acid (Fasn, Scd1, and Acaca) and TG synthesis (Dgat1 and Mogat1), TG uptake (Pparγ), gluconeogenesis (G6Pase), glycogenolysis (Gys2) and glucose transport (Glut2) was upregulated. Thus, the authors concluded that there was also a disruption of lipid and glucose metabolisms in these animals that may be associated with inflammation or hepatic steatosis (Meng, Wang, et al. Citation2019). However, in the study done by da Silva et al. prenatal exposure to BPS was found to be related to decreased plasma levels of the hormone tetraiodothyronine (thyroxine, T4) and, consequently, to less accumulation of lipids in brown adipocytes of adult F1 females (da Silva et al. Citation2019). In this work, it was not possible to establish any relationship between the concentrations of this EDC and the disruption of glucose metabolism (da Silva et al. Citation2019). On the other hand, prenatal exposure of adult male mouse offspring to BPAF and BPF was associated with liver glucose metabolism disruption, as evidenced by, respectively, increased and decreased liver glycogen and glucose concentrations (Meng, Tian, et al. Citation2019). Furthermore, for BPF it was possible to establish relations between lipid and antioxidant metabolisms, i.e. it was found that this EDC promoted lipid accumulation in the liver – as evidenced by increased TG concentrations – and decreased the activity of the antioxidant enzyme catalase (CAT). Thus, according to this study, BPF has more toxic consequences for metabolism than BPAF (Meng, Tian, et al. Citation2019), contrary to what was stated previously for F1 adolescent females (Meng et al. Citation2018). In 2021, Brulport et al. assessed the transgenerational effects on adult offspring (23 weeks of age) fed with a standard diet following perinatal exposure to BPS (Brulport et al. Citation2021). In general, for males, it was found that in the F1 generation, there was a decrease in blood cholesterol levels associated with a greater intestinal inflammatory response. For the male F2 generation was observed reductions in blood concentrations of TG, glucose, and insulin that were associated with intestinal inflammation. These serological lipid changes seem to be explained by the inflammatory state promoted by BPS, i.e. intestinal inflammation is thought to inhibit the absorption of fatty acids in the intestine. In addition, it is thought that the pro-inflammatory cytokines released in this inflammatory state are detected by hepatocytes, which alters the products secreted by them. In the F3 generation, they observed a lower intestinal inflammatory response associated with increased blood cholesterol and glucose levels and decreased fat mass. The activation of gluconeogenesis can explain the increase in blood glucose as a compensatory mechanism for transgenerational exposure. Thus, it is important to highlight that, since the changes were observed over several generations, the effects caused by BPS seem to be associated with germ cell epigenetic modifications that can be maintained even when there is no environmental exposure to this EDC, which ultimately potentiates its effects at biological, etiological, and evolutionary levels (Brulport et al. Citation2021). On the other hand, there have been some studies in which the offspring, after prenatal exposure to BPS, was subjected to a high-fat diet (Ivry Del Moral et al. Citation2016; Meng, Wang, et al. Citation2019; Ahn et al. Citation2020). Overall, it has been shown that this compound amplifies the negative metabolic effects associated with this type of diet, i.e. BPS can further increase the storage of lipids in the adipose tissue and the liver (Ivry Del Moral et al. Citation2016; Meng, Wang, et al. Citation2019; Ahn et al. Citation2020). Furthermore, this EDC can disturb glucose metabolism (Ivry Del Moral et al. Citation2016; Meng, Wang, et al. Citation2019; Ahn et al. Citation2020), and may even aggravate the reduction in sensitivity to insulin and leptin (Ivry Del Moral et al. Citation2016). Finally, in 2017, Pu et al. performed a study in ewes and discovered that daily subcutaneous injections of BPS to female progenitors during pregnancy affected gene expression of preadipocyte steroid receptors – ESR1, ESR2, and GR – and their terminal differentiation (Pu et al. Citation2017). Thus, the authors concluded that prenatal exposure to this BPA substitute sustained the development of a dysfunctional phenotype in these cells, which may disrupt normal adipocyte function and subsequently lead to metabolic consequences in the offspring (Pu et al. Citation2017). However, this correlation remains to be proven (Pu et al. Citation2017).
In conclusion, BPA substitutes seem to be associated with metabolic disorders in these animal models, which may have severe consequences for their development and even stimulate some pathologies’ appearance.
3.3. Alterations in thyroid and neurological functions
Concerning possible changes in neonatal development caused by exposure to these EDCs, there have been performed some studies related to thyroid (Wei et al. Citation2018; da Silva et al. Citation2019) and neurological functions (Castro et al. Citation2015; Catanese and Vandenberg Citation2017; Wei et al. Citation2018; Zhang et al. Citation2021).
Thus, in 2018, Wei et al. performed a study on zebrafish, where they found that exposure to BPS, in the F0 generation modified the expression of genes related to the hypothalamic–pituitary–thyroid axis (HPT), the plasma levels of thyroid hormones (THs) – decreased levels of T4 and increased those of triiodothyronine (T3) – and the histology of thyroid follicles (Wei et al. Citation2018). In the F1 generation, this EDC altered plasma levels of TH in eggs, affecting their development. In the F0 generation, an increased gene expression of crh and tshβ was observed, which consequently over-stimulated thyroid follicles (evidenced by hyperplasia and hypertrophy of the follicular epithelium), increasing the synthesis and secretion of THs, namely of T3, into the bloodstream. Furthermore, these animals showed increased gene expression of dio1 and dio2 (responsible for the conversion of T4 into T3), dio3 (associated with the inactivation of excessive T3), and ugt1ab (increases T4 metabolism and excretion), which seems to explain the reduction in plasma T4 concentrations in female progenitors. In the early stages of development, THs are provided by the mother. Therefore, once the concentrations of these hormones are altered in the female progenitors, it is easy to understand that the same pattern will be observed in the offspring. Thus, in the F1 generation, there is also a decrease and increase in the levels of T4 and T3, respectively. This disruption subsequently leads to serious offspring consequences, namely at the level of cognitive and morphological development. Indeed, the head-trunk angle (HTA) and otic vesicle size (OVL) are two morphological endpoints frequently used to evaluate embryonic zebrafish development. In this study, in the F1 generation, HTA and OVL were significantly decreased and increased, respectively. These alterations revealed a developmental delay that the authors hypothesized to be associated with excessive concentrations of T3 in eggs. On the other hand, the increment in T3 concentrations was associated with reduced surfactant proteins – responsible for lowering the surface tension of the swim bladder – production, and, consequently, decreased swim bladder inflation. Finally, according to the researchers, the excessive levels of this TH also contributed to the hypopigmentation of lateral body stripes, by interfering with the morphogenesis of melanocytes from the neural crest and with the melanin synthesis (Wei et al. Citation2018). In 2019, a study was also performed, in mice, where the impact of BPS on THs concentrations was assessed (da Silva et al. Citation2019). They discovered some gender and age differences for some concentrations of this EDC. In the male offspring, 21 d after birth, plasma T4 levels were increased, and 180 d after birth, T3 concentrations were reduced. As for the female offspring, 21 d after birth, no significant changes were observed, in contrast to 180 d after birth, when a decrease in plasma T4 levels was observed. Although no reason has been given to explain the differences observed between gender and age, the exposure to this compound also disrupts the homeostasis of THs in these animals, which may subsequently disturb their development and behavior (da Silva et al. Citation2019).
As for zebrafish neurological system development, Wei et al. found that exposure of female progenitors to BPS decreased and increased gene expression of syn2a and gfap genes, respectively (Wei et al. Citation2018). Therefore, processes such as synapse formation, neurotransmitter release, and glial cell integrity and functionality may be compromised, disrupting signal transmission between neurons, culminating in neurobehavioral and mobility damages. Thus, the response of these animals to various factors present in their environment may be reduced, compromising their capacity for survival (Wei et al. Citation2018). Regarding the effects of this compound at the neurological level in rodents, it was discovered that it could interfere with several processes involved in the normal functioning and development of this system in dams and their offspring (Castro et al. Citation2015; Catanese and Vandenberg Citation2017). In 2017, Catanese et al. demonstrated that exposure to BPS increased the expression of estrogen receptor alpha (ERα) in the rostral central medial preoptic area (cMPOA) of F0 animals (Catanese and Vandenberg Citation2017). In rodents, this brain area is highly associated with maternal protective behavior toward offspring (Catanese and Vandenberg Citation2017). However, the implications associated with these variations are still unclear. In this study, the expression of ERα in the F0 offspring was also evaluated; however, no significant changes were observed. Nevertheless, it is thought that some neuronal modifications must be transmitted transgenerationally since the F1 generation presents drastic behavioral changes toward its offspring (please see topic 3.4). Furthermore, Castro et al. discovered that BPS could affect serotoninergic metabolism in the prefrontal cortex (PFC) of female F1 offspring by altering the gene expression of genes encoding enzymes responsible for metabolizing the neurotransmitter 5-HT (Castro et al. Citation2015). On the other hand, the authors also reported that BPF, in addition to affecting serotoninergic metabolism, can also disturb dopaminergic metabolism in PFC, by altering the transcription of genes encoding enzymes in charge of this process. Moreover, both BPA substitutes could slightly influence the transcription of DA receptors in the PFC (e.g. Drd1) and several 5-HT receptor subtypes (e.g. Htr1a). Therefore, BPS and BPF may interfere with the regulation of cortical neurogenesis. In addition, both substitutes can increase gene expression of Cyp2d4, which is involved in the hydroxylation of allopregnanolone (AlloP) and progesterone to produce corticosteroids. Thus, the AlloP and corticosteroid levels are, respectively, decreased and increased in the offspring’s brain, affecting the development of this organ. Finally, these researchers observed that BPF downregulated the expression of genes involved in neurogenesis, neurodevelopment (e.g. App), and cortical neuronal survival (e.g. Bndf), thus impairing these processes (Castro et al. Citation2015). In 2021, a research study was performed to assess the impact of perinatal exposure to BPAF on adult mice offspring in terms of their cognitive functions (Zhang et al. Citation2021). Overall, in males, this EDC decreased the expression of ERα, synapsin-1, and protein postsynaptic density 95 (PSD-95), the two latter being involved in synaptic plasticity. In females, the authors observed a reduction in the expression of PSD-95 – only for the highest BPAF concentrations – and of estrogen receptor beta (ERβ). These gender differences may be related to the different concentrations of steroid hormones detected in males and females, namely E2. Furthermore, BPAF perinatal exposure was found to impair dendritic morphology and dendritic spine density of neurons present in hippocampal CA1 and/or DG neurons, culminating in disruption of hippocampal plasticity. Therefore, these results suggest that this EDC could impair and damage learning and memory in adult offspring. Importantly, the authors believe that the disruptions caused by low concentrations of BPAF in both genders are related to endocrine factors; and that the disorders caused by higher concentrations in females are associated with the induction of apoptosis in nervous system cells and with epigenetic dysregulation (Zhang et al. Citation2021).
In summary, both the HPT axis and the nervous system can be affected by these EDCs, which can subsequently lead to severe consequences for the offspring, namely their development, behavior, and survival.
3.4. Alterations in behavior
In recent years, studies have also been performed in zebrafish (Wei et al. Citation2018) and rodents (Kim et al. Citation2015; Catanese and Vandenberg Citation2017; Gong et al. Citation2017; LaPlante et al. Citation2017; Ohtani et al. Citation2017; da Silva et al. Citation2019; Shi, Whorton, et al. Citation2019a) to assess the impact that prenatal exposure to BPS, BPF, or BPAF has on maternal and offspring behavior.
Wei et al. discovered that exposure of female progenitors to BPS causes mobility problems in the F1 generation, namely in terms of spontaneous movements, touch-evoked escape response, average swimming speed, and swirl escape rate (Wei et al. Citation2018). Thus, several behaviors important for the survival of these animals, such as swimming, feeding, and escaping from predators, may be compromised (Wei et al. Citation2018). As for rodents, LaPlante et al. found that the F0 generation’s exposure to high concentrations of BPS increased the time they spent nursing (LaPlante et al. Citation2017). These results, together with changes observed in the mammary gland, led the authors to conclude that by changing their behavior, dams try to compensate for milk deficiencies. However, this behavioral modification does not always have the desired result, as the offspring of these animals seem to be less interested in feeding and receiving maternal care (LaPlante et al. Citation2017). On the other hand, Catanese et al. reported that the F0 generation exposed to BPS had some moderate neglect behaviors toward its offspring, showing a longer latency period to interact with and retrieve their pups (Catanese and Vandenberg Citation2017). In other words, these animals may take longer to get their pups to a safe place, for example. However, when F0 dams were exposed to higher concentrations of BPS, they tended to spend more time on the nest even when their offspring no longer required constant protection and care, which may reveal a difficulty in adapting to the changing needs of their pups. In addition, these authors evaluated the behavior of the F1 generation as the F2 generation’s progenitors and verified that they tended to spend less time in the nest during the first days of their pups’ lives, which can put their survival at risk, as at this time they need constant protection and care. On the other hand, the researchers also observed that these animals (F1) spent a lot of time building the nest, which may indicate repetitive or obsessive-compulsive disorder-like behavior. The F1 generation, in contrast to F0, showed a shorter latency period in transporting the pups to a safe place, which seems to be associated with greater anxiety and hyperactivity. Finally, surprisingly, they observed that the F1 generation exposed to lower concentrations of BPS during the perinatal phase exhibited moderate to severe neglectful behavior and murdered their offspring (Catanese and Vandenberg Citation2017). Furthermore, in 2019, a study revealed that when the F0 generation was exposed to BPS, the F3 generation exhibited neglect problems, i.e. the F3 dams did not provide proper maternal care and their offspring ultimately did not survive (Shi, Whorton, et al. Citation2019a). In addition, this behavior tended to worsen with maternal age. The conclusions obtained by this work can be considered alarming since it is observed that this EDC can disturb the rodents’ behavior, even if they have not been directly exposed to it (Shi, Whorton, et al. Citation2019a).
On the other hand, offspring’s behavior after exposure to BPA substitutes can also be assessed. In this sense, Kim et al. reported that when the F1 generation is exposed to BPS in the early stages of development, they later exhibit behavioral changes, such as increased anxiety and reduced interest in establishing social interactions, although these changes did not directly impair their social dominance (Kim et al. Citation2015). In addition, another study was performed in 2019, where the effects of BPS on adult F1 generation’s behavior were assessed (da Silva et al. Citation2019). Behavioral differences between genders were observed, which are thought to be related to the sexual dimorphism of the brain that is associated with different steroid hormone concentrations between males and females. Therefore, males showed more significant anxiety and locomotor activity, while females showed less willingness to explore new spaces. However, in both genders, a tendency to choose a high-fat diet was observed, which may favor the development of obesity. This choice seems to reveal an obsessive behavior toward food that may be associated with alterations in the dopaminergic reward system, although this relationship has yet to be proven (da Silva et al. Citation2019).
Concerning prenatal exposure of the F1 generation to BPF, this was associated with a state of anxiety in females and with depressive behavior in both genders, although in a less expressive way in males (Ohtani et al. Citation2017). These differences could be associated with the steroidogenic activity of BPF. Progesterone can regulate neuroendocrine functions by acting on its receptors present in the central nervous system. Furthermore, previous studies have demonstrated that BPF can increase progesterone concentrations, which are already higher in females (Rosenmai et al. Citation2014). Therefore, the authors suggested that this may be one of the possible reasons for the differences in behavior observed between genders. On the other hand, exposure to BPF has been associated with altered serotoninergic and dopaminergic metabolisms in female rats, due to alterations in gene expression of genes encoding enzymes responsible for metabolizing the neurotransmitters 5-HT and DA (Castro et al. Citation2015). According to this relation between 5-HT and DA changes and psychiatric disorders, Ohtani et al. (Citation2017) hypothesized that this association may be responsible for the behavioral impairment observedOn the contrary, exposure to BPAF in the early life stages of the F1 generation was associated with higher depressive and anxiety states in males (Gong et al. Citation2017). In females, exposure to low concentrations of this EDC seems to be related to a decrease in anxiety upon discovering a novel environment. Thus, this compound appears to exert an anxiogenic effect in males and an anxiolytic effect in females. In addition, the contextual fear memory was disrupted in males, and the formation of new memories disturbed both sexes. These authors also hypothesized that these behavioral changes are related to alterations in the brain’s neurotransmitters, which depend on the steroid hormone balance that can be disturbed by this compound. These changes in memory and learning promoted by BPAF have also been observed in the offspring of another study and were more impactful in males than in females (Zhang et al. Citation2021). Once again, these authors proposed that the observed gender differences may be associated with different steroid hormone concentrations (Zhang et al. Citation2021).
In summary, it seems clear that these EDCs can affect the behavior of these animal models, compromising their welfare, the well-being of their offspring, and ultimately their pups’ survival.
3.5. Morphological and survival alterations
EDCs may also be associated with the development of morphological abnormalities, both in the progenitors exposed during pregnancy and in their offspring. Thus, exposure to BPF, BPB, and BPS of zebrafish F0 generations has been associated with an increase in their hepatosomatic index (HSI = liver weight × 100/body weight) (Yang et al. Citation2017a, Citation2017b; Qin et al. Citation2021), although in the case of BPS, it is not always possible to observe this relationship (Ji et al. Citation2013). As for progenitors’ brain somatic index (BSI = brain weight × 100/body weight), body weight and length, no significant variations were observed across treatments (Ji et al. Citation2013; Yang et al. Citation2017a, Citation2017b), except in the study done by Qin et al. (Citation2021) where exposure to BPS was directly related to the first two parameters listed.
In rodents, LaPlante et al. (Citation2017) established a link between exposure of dams to BPS and less development and density of their mammary glands. The results showed that this exposure decreased the number of lobuloalveolar units, responsible for milk secretion. Regarding offspring, more significant and diversified changes were observed (Ji et al. Citation2013; Shi et al. Citation2015; Ivry Del Moral et al. Citation2016; Yang et al. Citation2017a,Citation2017b; Kolla et al. Citation2018; Meng et al. Citation2018; Tucker et al. Citation2018; Wei et al. Citation2018; da Silva et al. Citation2019; Meng, Wang, et al. Citation2019; Meng, Zhu, et al. Citation2019; Ullah et al. Citation2019; Ahn et al. Citation2020; Brulport et al. Citation2021; Kaimal et al. Citation2021; Qin et al. Citation2021; Zhang et al. Citation2021). Exposure to BPA substitutes – BPS, BPF, BPAF, and BPB – during the early stages of development, appears often reported to be related to increased occurrence of malformations in zebrafish offspring (Shi et al. Citation2015), namely at the cardiovascular (Ji et al. Citation2013; Yang et al. Citation2017a, Citation2017b; Qin et al. Citation2021) and yolk sac levels (Qin et al. Citation2021); shortened tails (Ji et al. Citation2013); reduced body length (Qin et al. Citation2021); tail deformities (Yang et al. Citation2017a,Citation2017b); severe spinal kyphosis (Ji et al. Citation2013; Yang et al. Citation2017a, Citation2017b); reduced HTA and OVL – evidencing a developmental delay – (Wei et al. Citation2018); decreased swim bladder inflation (Wei et al. Citation2018); hypopigmentation of lateral body stripes (Wei et al. Citation2018); and decreased eye size (Qin et al. Citation2021). Moreover, in these works, exposure to these compounds was proven to reduce and delay the hatching rate of eggs (Ji et al. Citation2013; Shi et al. Citation2015; Yang et al. Citation2017a,Citation2017b; Dong et al. Citation2018; Wei et al. Citation2018; Qin et al. Citation2021), increasing their vulnerability to predator attack (Wei et al. Citation2018) and decreasing offspring’ survival rate (Ji et al. Citation2013; Shi et al. Citation2015; Yang et al. Citation2017a,Citation2017b; Dong et al. Citation2018; Qin et al. Citation2021). There is only to highlight the fact that in one study, a higher concentration of BPS was related to a higher hatching rate of eggs (Qin et al. Citation2021).
In rodents, exposure to BPS, BPF, and BPAF has been directly related to the appearance of morphological alterations in the mammary glands and to delayed development (Kolla et al. Citation2018; Tucker et al. Citation2018). In addition, BPS has been associated with the appearance of changes in ovarian morphology (Kaimal et al. Citation2021). Regarding changes in body weight over the lifetime of these animals, it was found that exposure to BPF does not seem to have an influence on this factor (Catanese and Vandenberg Citation2017; Ohtani et al. Citation2017; Meng et al. Citation2018; Tucker et al. Citation2018; Meng, Tian, et al. Citation2019; Meng, Zhu, et al. Citation2019; Ullah et al. Citation2019; Malaisé et al. Citation2020; Kaimal et al. Citation2021; Malaisé et al. Citation2021), or when it has, it is inversely related to this parameter (Malaisé et al. Citation2020, Citation2021). Whereas submission to some concentrations of BPAF has been linked to decreased offspring body weight (Li et al. Citation2016; Meng et al. Citation2018; Tucker et al. Citation2018; Meng, Zhu, et al. Citation2019; Zhang et al. Citation2021). However, in some cases, this relationship could not be observed (Tucker et al. Citation2018; Meng, Tian, et al. Citation2019; Zhang et al. Citation2021) and in one study BPAF was associated with increased body weight (Gong et al. Citation2017). In the case of BPS, the results are also quite diverse, with relationships having been established between this EDC and increased (Meng et al. Citation2018; Meng, Wang, et al. Citation2019; Meng, Zhu, et al. Citation2019) and decreased body weight (Kim et al. Citation2015; LaPlante et al. Citation2017; Tucker et al. Citation2018; Shi, Whorton, et al. Citation2019b), although in most cases, the observed variations were not statistically significant (Kim et al. Citation2015; Ivry Del Moral et al. Citation2016; Kasneci et al. Citation2017; LaPlante et al. Citation2017; Shi et al. Citation2018; Tucker et al. Citation2018; da Silva et al. Citation2019; Shi, Sekulovski, et al. Citation2019; Shi, Whorton, et al. Citation2019a; Ullah et al. Citation2019; Ahn et al. Citation2020; Malaisé et al. Citation2020; Brulport et al. Citation2021; Kaimal et al. Citation2021; Malaisé et al. Citation2021). Furthermore, for BPB (Ullah et al. Citation2019) and BPE (Shi et al. Citation2018; Shi, Sekulovski, et al. Citation2019; Shi, Whorton, et al. Citation2019a, Citation2019b) no significant differences could be observed with regard to this morphological factor. Sometimes, it is also investigated whether there are variations in organ weights of rodent offspring, after exposure to BPA substitutes (Ivry Del Moral et al. Citation2016; Kasneci et al. Citation2017; Meng et al. Citation2018; Meng, Zhu, et al. Citation2019; Kaimal et al. Citation2021; Zhang et al. Citation2021). Overall, no significant differences were observed (Ivry Del Moral et al. Citation2016; Kasneci et al. Citation2017; Meng et al. Citation2018; Meng, Tian, et al. Citation2019; Meng, Zhu, et al. Citation2019; Kaimal et al. Citation2021; Malaisé et al. Citation2021; Zhang et al. Citation2021), except, for example, for liver’ (Meng, Wang, et al. Citation2019), abdominal adipose tissue’ (Kaimal et al. Citation2021) and spleen’ weight (Kasneci et al. Citation2017), in cases of BPS exposure; and for epididymal adipose tissue’ weight (Meng, Wang, et al. Citation2019; Kaimal et al. Citation2021), after males exposure to BPS and BPF. For head and abdominal circumferences, crown rump length, nipple retention (NR) and ano-genital distance (AGD) no significant changes were detected (Kasneci et al. Citation2017; Ullah et al. Citation2019; Kaimal et al. Citation2021), except for males exposed prenatally to BPS, where there was a decrease in AGD (Kaimal et al. Citation2021). Furthermore, exposure to these EDCs does not appear to be associated with changes in pregnancy duration (Meng et al. Citation2018; Meng, Tian, et al. Citation2019; Meng, Wang, et al. Citation2019; Meng, Zhu, et al. Citation2019; Ullah et al. Citation2019; Kaimal et al. Citation2021), nor in litter size (Kim et al. Citation2015; Li et al. Citation2016; Catanese and Vandenberg Citation2017; Meng et al. Citation2018; Meng, Tian, et al. Citation2019; Meng, Wang, et al. Citation2019; Meng, Zhu, et al. Citation2019; Ullah et al. Citation2019; Kaimal et al. Citation2021). In general, these compounds do not appear to be gender dependent (Li et al. Citation2016; Catanese and Vandenberg Citation2017; Ohtani et al. Citation2017; Shi, Whorton, et al. Citation2019a; Kaimal et al. Citation2021), although in one study prenatal exposure to BPS and BPE was found to increase the number of females in the offspring (Shi, Sekulovski, et al. Citation2019) and in another investigation exposure to BPF increased the number of male pups (Malaisé et al. Citation2021). As for survival rate, on the one hand, according to work done by Li et al. (Citation2016) BPAF does not seem to influence the survival rate of rodent offspring. On the other hand, Kaimal et al. (Citation2021) found that BPF appears to increase F0 generation’ miscarriage rate, which consequently reduces the survival rate of the F1 generation.
In summary, the exposure of the progenitors to these EDCs can cause several morphological changes for these animals and their offspring. Some of these modifications may compromise the quality of life of these animals and, in some situations, affect their survival.
3.6. Immune system alterations
Regarding the immune system, some studies have assessed the impact of exposure to these EDCs on the immune system’s normal functioning in model organisms zebrafish (Dong et al. Citation2018) and mice (Tucker et al. Citation2018; Meng, Wang, et al. Citation2019; Malaisé et al. Citation2020; Brulport et al. Citation2021; Malaisé et al. Citation2021). Hence, in a study performed on zebrafish, it was observed that exposure of the F0 generations to BPS and BPF caused alterations in the F1 generation’s immune functions, namely, the response to viral and bacterial infections, which, in turn, may impair the health of these organisms and/or compromise their survival (Dong et al. Citation2018). In other words, these compounds decreased the offspring’s immune response by delaying the induction of antiviral and antibacterial cytokines upon infection and downregulating the expression of antioxidant proteins (Cat, Cu/Zn-Sod, and Mn-Sod) and Tlr2 and Tlr3 proteins. Subsequently, a reduction in expression of the Tlr downstream signaling molecules – Myd88 and Trif – activation occurs thus, preventing the recognition of pathogen-associated molecular patterns (PAMPs). Furthermore, they discovered that BPS and BPF could disrupt the development of lymphoid tissue, which is essential for the immune system, as evidenced by inhibition of the expression of rag1 and rag2 genes. Thus, both innate and adaptive immune responses appear to be compromised, which leaves these animals vulnerable to infectious agents (Dong et al. Citation2018). In mice, different studies have shown that BPS, BPF, and BPAF administered orally or dermally to the F0 generation can promote the development of inflammatory responses in several F1 generation’s organs, such as in the gut (Malaisé et al. Citation2020; Brulport et al. Citation2021; Malaisé et al. Citation2021), mammary gland (Tucker et al. Citation2018), liver, and epiWAT cells (Meng, Tian, et al. Citation2019). Sometimes, these animals ended up developing chronic systemic inflammatory responses that could contribute to immunotoxicity in development (Meng, Wang, et al. Citation2019; Malaisé et al. Citation2020; Brulport et al. Citation2021; Malaisé et al. Citation2021). It should also be noted that some of the damage caused to the immune system can be transmitted to several generations, even when they are not directly exposed to these EDCs. Therefore, the effects of exposure to these compounds can be amplified (Brulport et al. Citation2021).
Thus, it is demonstrated that BPA substitutes can impair the normal functioning of the offspring’s immune system, lowering their defenses, and eventually promoting the development of immune pathologies, which may compromise the survival of the animals (Dong et al. Citation2018; Malaisé et al. Citation2020; Brulport et al. Citation2021; Malaisé et al. Citation2021).
In summary, BPA substitutes – BPS, BPF, BPAF, BPB, BPE – can cause a diversity of problems in various systems of model organisms, including zebrafish and mice (see ). Therefore, exposure to these compounds can compromise maternal’ and offspring’s health at various levels and ultimately affect their survival (Ji et al. Citation2013; Shi et al. Citation2015; Catanese and Vandenberg Citation2017; Yang et al. Citation2017a,Citation2017b; Dong et al. Citation2018; Kaimal et al. Citation2021; Qin et al. Citation2021). Hence, it appears that, at least in animal models, these compounds do not appear to be as safe as previously thought, so the replacement of BPA with its substitutes should be well evaluated and rethought (Hill et al. Citation2017; Grandin et al. Citation2018; Malaisé et al. Citation2020, Citation2021). So, the next step was to demonstrate that these compounds also have adverse effects on human health, particularly during critical and vulnerable periods, such as pregnancy.
Figure 2. Schematic representation of the various stages of vulnerability to endocrine disruption caused by BPA substitutes in animals. Exposure of progenitors to BPA substitutes during the prenatal phase causes adverse effects on maternal and offspring’s health since these compounds can be transferred transgenerationally. BPS: bisphenol S; BPF: bisphenol F; BPAF: bisphenol AF; BPB: bisphenol B; BPAP: bisphenol AP; BPZ: bisphenol Z; BPP: bisphenol P; BPE: bisphenol E; BHPF: bisphenol HPF; HPT axis: hypothalamic–pituitary–thyroid axis; HPG axis: hypothalamic–pituitary–gonadal axis. Figure created with PowerPoint version 2204 and using pictures from Servier Medical Art. Servier Medical Art by Servier, licensed under a Creative Commons Attribution 3.0 Unported License (https://creativecommons.org/licenses/by/3.0/).
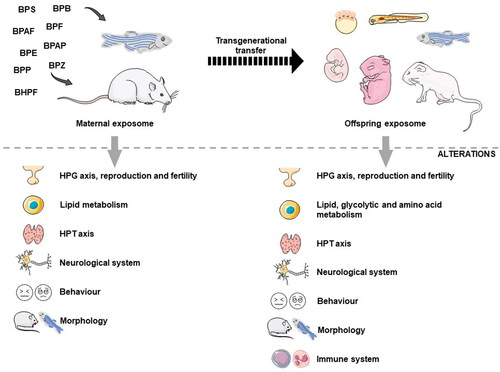
4. Prenatal exposure of humans
Exposure to EDCs is a growing concern in today’s societies, especially in the early stages of development, such as pregnancy and the prenatal period, when individuals may be more susceptible to their effects (Liu and Peterson Citation2015; Marie et al. Citation2016; Kelley et al. Citation2019; Gingrich et al. Citation2020; Rolfo et al. Citation2020; Varshavsky et al. Citation2020; Lorigo and Cairrao Citation2022; Padmanabhan et al. Citation2021). During pregnancy, women undergo a set of physiological, metabolic, endocrine, reproductive, and immune changes that leave them more sensitive to the action of these chemical compounds (Varshavsky et al. Citation2020; Lorigo and Cairrao Citation2022). However, according to the Developmental Origins of Health and Disease (DOHaD) theory, the developing fetus may also be affected since some EDCs that are in the maternal circulation can cross the placenta and bioaccumulate, possibly compromising various metabolic and physiological processes and consequently increasing the risk of developing pathologies during the postnatal period and later in adulthood (Rahman et al. Citation2016; Ghassabian and Trasande Citation2018; Kelley et al. Citation2019; Gingrich et al. Citation2020; Street and Bernasconi Citation2020; Tanner et al. Citation2020; Lorigo and Cairrao Citation2022; Lucaccioni et al. 2021; O'Shaughnessy et al. 2021). This exposure to EDCs during pregnancy is defined as “prenatal exposure” and can be subdivided into “maternal exposure” and “fetal exposure” (Rager et al. Citation2020; Lorigo and Cairrao Citation2022).
Several studies have already proven that BPA can cause serious problems for human health, which is why this compound has been replaced by its analogs/substitutes (Flint et al. Citation2012; Rochester and Bolden Citation2015; Chen et al. Citation2016; Zhang, Xia, et al. Citation2019; Pan et al. Citation2020). Therefore, it is essential to assess whether human exposure to these substitutes will be safer than exposure to BPA (Kolatorova et al. Citation2017), namely, in the periods of most significant vulnerability as is the case in the prenatal phase (Wan et al. Citation2018; Huang et al. Citation2019; Pan et al. Citation2020).
BPA substitutes – BPS, BPF, BPAF, BPB, BPAP, BPZ, BPP, and BPE – and the BPS derivative (BPSIP) have already been detected in a variety of biological samples, namely, in maternal blood (Liu et al. Citation2017; Kolatorova et al. Citation2018; Pan et al. Citation2020; Zhang, He, et al. Citation2020; Liang et al. Citation2022), cord blood (Liu et al. Citation2017; Ihde et al. Citation2018; Kolatorova et al. Citation2018; Pan et al. Citation2020; Zhang, He, et al. Citation2020), maternal urine (Ferguson KK et al. Citation2018; Ihde et al. Citation2018; Liu et al. Citation2018; Machtinger et al. Citation2018; Mustieles et al. Citation2018; Philips et al. Citation2018; Wan et al. Citation2018; Aker et al. Citation2019; Aung et al. Citation2019; Hu et al. Citation2019; Huang et al. Citation2019; Li et al. Citation2019; Zhang, Xia, et al. Citation2019; Kang et al. Citation2020; Zhang, He, et al. Citation2020), placenta (Pan et al. Citation2020), and amniotic fluid (Zhang, He, et al. Citation2020; ). Furthermore, the concentrations of these chemical compounds in different biological samples have been assessed in various countries, such as, in China (Liu J et al. Citation2017; Wan et al. Citation2018; Li et al. Citation2019; Zhang, Xia, et al. Citation2019; Pan et al. Citation2020; Zhang, He, et al. Citation2020; Liang et al. Citation2022), Czech Republic (Kolatorova et al. Citation2018), United States of America (Ihde et al. Citation2018), Canada (Liu et al. Citation2018), Israel (Machtinger et al. Citation2018), the Netherlands (Philips et al. Citation2018), and South Korea (Kang et al. Citation2020).
Table 2. Concentrations and detection rates of BPA substitutes in different prenatal exposure studies.
In 2017, Liu et al. analyzed the detection frequencies of three BPA substitutes (BPS, BPAF, and BPB) in maternal and cord blood samples from 61 Chinese pregnant women (Liu J et al. Citation2017). However, in this study, only conjugated BPS could be detected at a low frequency in both biological samples. Thus, according to the authors, these data suggest, for the first time, that there is a maternal–fetal transfer of BPS via the placenta (Liu et al. Citation2017). In 2018, Kolatorova et al. examined maternal blood samples from 27 Czech women collected in the 37th week of pregnancy and umbilical cord blood samples collected after delivery (Kolatorova et al. Citation2018). The researchers noted that unconjugated BPS and BPF could only be detected in maternal plasma samples, and BPF was identified in just one umbilical cord plasma sample. Therefore, similarly to BPS (Liu et al. Citation2017), a maternal–fetal transfer of BPF also occurs (Kolatorova et al. Citation2018). Also, in 2018, Ihde et al. assessed the presence of BPS, BPF, BPB, and BPAF in maternal urine and cord blood samples from 30 North American women (Ihde et al. Citation2018). These authors reported that BPAF, BPB, and BPS were the most prevalent substitutes in maternal urine samples, while BPAF and BPF were predominantly present in cord blood samples. Therefore, they demonstrated that BPAF and BPB – albeit to a lesser extent – are also transferred from the mother to the developing fetus during pregnancy. However, as the Kolatorova et al. study observed, BPS was not detected in cord blood samples (Ihde et al. Citation2018). In 2022, Liang et al. also observed the presence of BPB, BPF, and BPS in maternal blood samples (Liang et al. Citation2022).
In addition, in 2018 and 2019, several studies evaluated the presence of BPA substitutes throughout the various periods of pregnancy in maternal urine samples from women living in a wide variety of countries, such as Canada (Liu et al. Citation2018), Israel (Machtinger et al. Citation2018), the Netherlands (Philips et al. Citation2018), China (Wan et al. Citation2018; Hu et al. Citation2019; Huang et al. Citation2019; Li et al. Citation2019; Zhang, Xia, et al. Citation2019), USA (Ferguson KK et al. Citation2018; Mustieles et al. Citation2018; Aung et al. Citation2019), Puerto Rico (Aker et al. Citation2019), and South Korea (Kang et al. Citation2020). Overall, across these investigations, BPS (Ferguson et al. Citation2018; Liu et al. Citation2018; Machtinger et al. Citation2018; Mustieles et al. Citation2018; Philips et al. Citation2018; Wan et al. Citation2018; Aker et al. Citation2019; Aung et al. Citation2019; Hu et al. Citation2019; Huang et al. Citation2019; Li et al. Citation2019; Zhang, Xia, et al. Citation2019), BPF (Liu et al. Citation2018; Machtinger et al. Citation2018; Philips et al. Citation2018; Aker et al. Citation2019; Hu et al. Citation2019; Li et al. Citation2019; Zhang, Xia, et al. Citation2019; Kang et al. Citation2020) and BPAF (Liu et al. Citation2018; Zhang, Xia, et al. Citation2019) were detected in all trimesters of pregnancy, and BPZ, BPB, and BPAP (Philips et al. Citation2018) were found in samples from the first trimester (). Thus, maternal exposure to these EDCs during pregnancy is once again evidenced. It should be noted that Wan et al. (Citation2018) found that BPS concentrations in samples from mothers who delivered a girl were higher than from those who delivered a boy. This trend may be related to the hypothesis that EDCs, by interfering with maternal hormones during the conception period, may be able to modify the sex ratio of the fetus (James Citation2008; Wan et al. Citation2018). Furthermore, it should be noted that in the studies performed by Hu et al. and Huang et al. BPS concentrations in maternal urine slightly increased over the three trimesters, which may be associated with higher exposure and bioaccumulation, and consequently with a higher risk of endocrine disruption (Hu et al. Citation2019; Huang et al. Citation2019). On the contrary, in the study developed by Li et al., there were no significant changes in the concentrations of BPS and BPF present in maternal urine over the various trimesters (Li et al. Citation2019). However, the authors could not provide any justification for this finding and only suggested that in future studies, several measurements should be made throughout the pregnancy period to reduce the likelihood of error (Li et al. Citation2019). It is also important to mention that in the study performed by Liu et al. 3 months after delivery, it was still possible to detect BPS in maternal urine − 0.17 ng/mL; 0.20 μg/g creatinine (Liu et al. Citation2018). Thus, the effects caused by BPA substitutes may continue to be manifested in the postnatal period. To our knowledge, there is no literature proving that EDC levels return to normal after pregnancy. Therefore, the effects caused by these compounds may be even more concerning, both for maternal and fetal health, as they may persist in the following stages of life.
In 2020, Pan et al. investigated the occurrence and distribution of bisphenols (some BPA substitutes and a BPS derivative (BPSIP)) in the fetoplacental unit (Pan et al. Citation2020). For this purpose, samples of maternal blood, umbilical cord blood, and placenta – always from the same placenta area – were collected from 60 Chinese pregnant women. Overall, it was evidenced that BPS in free form, BPAF, BPE, BPAP, and BPSIP can be transferred from the mother to the developing fetus since these compounds were identified in the biological samples collected. However, BPF was only detected in maternal plasma and placental samples, which may be related to a higher rate of excretion, and consequently to a lower transfer of this compound to the fetus (Pan et al. Citation2020). In addition, placental transfer efficiency (PTE), cord − maternal concentration ratio (RCM), and placental − maternal concentration ratio (RPM) were calculated in this study for the BPA substitutes. Thus, the researchers concluded that BPAF has a greater tendency to accumulate in cord blood and consequently in the fetus (RCM 6.3; RPM 2.2; PTE > 100%). On the contrary, BPSIP (RCM 0.059; RPM 0.20; PTE < 100%) and BPE (RCM 0.82; RPM 0.89; PTE < 100%) have a greater tendency to accumulate in the maternal blood and therefore are less likely to be transferred to the fetus, although this is still a possibility (Pan et al. Citation2020). Finally, also in 2020, Zhang et al. analyzed for the first time, samples of maternal serum, maternal urine, umbilical cord serum, and amniotic fluid from 106 Chinese women living in places where electronic device recycling is abundant (Zhang, He, et al. Citation2020). In this study, it was possible to detect the presence of BPP, BPS, BPAF, and BPAP in all biological samples. Thus, it is again demonstrated that women living in these places are exposed to these EDCs, as are their children, due to the maternal–fetal transfer of these substitutes. Furthermore, when it was possible to detect the substitute in the mother–fetus pair simultaneously, the researchers determined the RCM for BPAF and BPS. Thus, as was the case in the previously described study (Pan et al. Citation2020), BPAF (RCM = 3.26 for 22 pairs) was found to have a high PTE, while BPS (RCM = 1.11 for 11 couples) showed a lower PTE (Zhang, He, et al. Citation2020).
In 2019, Grandin et al. performed a study to evaluate the placental transfer of BPS and its main metabolite, BPSG, in the maternal–fetal direction and the opposite direction (Grandin et al. Citation2019). For this purpose, the authors used perfused human placental cotyledon models. The results showed that BPS can be transferred in both directions across the placenta, although transfer in the fetal–maternal direction is less extended. In the case of BPSG, although this compound has been detected in the fetal compartments, the data demonstrated that transfer in the maternal–fetal direction is almost non-existent. This result is consistent with a study performed by these researchers on ewes (as previously described in topic 3) (Grandin et al. Citation2018). Therefore, the detection of BPSG in fetal compartments is thought to be associated with the BPS metabolization by the fetus. In the fetus–mother direction, it was possible to observe the transfer of the BPSG, although slowly. Therefore, in this placental model, BPS and its metabolite can be transferred between the two compartments exposing the fetus to the effects of this EDC (at least during late pregnancy) (Grandin et al. Citation2019).
Finally, it is also important to mention that some studies have been performed with the aim of understanding whether there are factors associated with mothers’ lifestyles that can influence the concentrations of these compounds in biological samples (Liu et al. Citation2018; Philips et al. Citation2018; Li et al. Citation2019; Pan et al. Citation2020). For example, women with a high pre-pregnancy body mass index (Philips et al. Citation2018; Pan et al. Citation2020) and/or folic acid deficiency have been found to have higher concentrations of bisphenols in their urine (Philips et al. Citation2018). Folic acid (vitamin B9) is a methyl donor molecule, so it is essential in preventing epigenetic changes. Consequently, a deficit of this vitamin during the first trimester can have very worrying impacts since, at this stage of the pregnancy, the occurrence of these alterations is common (Philips et al. Citation2018; Lorigo and Cairrao Citation2022). Furthermore, it is essential to note that, as previously discussed, in animal models; it is thought that BPA substitutes may be associated with the development of epigenetic changes. It is also believed that these modifications, in turn, are directly related to the transmission of these compounds’ effects to the following generations (Shi et al. Citation2018; Shi, Whorton, et al. Citation2019b; Malaisé et al. Citation2020). Thus, in future studies, it will be important to evaluate whether in humans exposure to these EDCs can also promote the development of these changes that when associated with nutritional deficits would represent an even greater reason for concern. Furthermore, in the study performed by Li et al. a correlation was established between excessive weight gain during pregnancy and increased concentrations of BPF and BPS. These results may be associated with the endocrine-disrupting (e.g. disruption of THs) and metabolic (e.g. lipid metabolism disturbance) effects or with the dietary exposure pathways associated with these compounds, as BPF is present mainly in meat and BPS in plant products (Li et al. Citation2019). Liu et al. also identified a relationship between mustard consumption during pregnancy and elevated concentrations of BPF in maternal urine (Liu et al. Citation2018). However, future studies are needed to clarify which lifestyle factors may affect the concentrations of these EDCs, and consequently increase the risk of exposure before and during pregnancy.
In summary, the various BPA substitutes were detected in samples of maternal blood, umbilical cord blood, maternal urine, placenta, and amniotic fluid (). Furthermore, it was possible to detect the presence of some substitutes over the various trimesters, which may indicate that there is no concrete trimester in which the presence of EDCs should be assessed. Ideally, exposure to EDCs should be analyzed in different biological samples and over the entire course of pregnancy so that consequences for the mother and fetus can be understood and identified (Lorigo and Cairrao Citation2022). On the other hand, it may also be important to identify these compounds in the postnatal phase, as this remains a period of increased sensitivity to EDCs’ exposure (Liu et al. Citation2018). Most studies, evidence a maternal–fetal transfer of these compounds, which may be related to the development of health problems for the mother, such as possible hematological changes (Kang et al. Citation2020) and glycolytic metabolism disruption (Zhang, Xia, et al. Citation2019); and for the fetus, such as alterations in development and growth (Mustieles et al. Citation2018; Hu et al. Citation2019; Pan et al. Citation2020). Therefore, bioaccumulation of BPA substitutes can disturb maternal and fetal health.
4.1. Effects of BPA substitutes in the prenatal phase
Exposure to BPA substitutes during the prenatal phase can have serious consequences for the health of both the mother and the developing fetus. These EDCs can exert various alterations in maternal and fetal systems, for example, they may trigger the onset of pregnancy-associated complications, such as gestational diabetes mellitus (Zhang, Xia, et al. Citation2019), maternal hematological changes (Kang et al. Citation2020), variations in the duration of pregnancy (longer pregnancy or preterm birth - before 37 weeks) (Wan et al. Citation2018; Aker et al. Citation2019; Aung et al. Citation2019; Huang et al. Citation2019; Pan et al. Citation2020), modifications of morphological characteristics, such as birth weight (Ferguson KK et al. Citation2018; Mustieles et al. Citation2018; Hu et al. Citation2019; Pan et al. Citation2020), body length (Aker et al. Citation2019; Hu et al. Citation2019), head (Ferguson KK et al. Citation2018; Mustieles et al. Citation2018), abdominal circumference (Ferguson et al. Citation2018), and femur size (Ferguson KK et al. Citation2018; ).
Figure 3. Schematic representation of the various stages of vulnerability to endocrine disruption caused by BPA substitutes. Exposure to BPA substitutes begins in the prenatal phase, as the placenta is not an entirely effective barrier to maternal-fetal transfer of these compounds. Consequently, the bioaccumulation of these EDCs in the fetus can be promoted. Maternal and fetal exposure can harm the health of both individuals. BPS: bisphenol S; BPF: bisphenol F; BPAF: bisphenol AF; BPB: bisphenol B; BPAP: bisphenol AP; BPZ: bisphenol Z; BPP: bisphenol P; BPE: bisphenol E. Figure created with PowerPoint v.2204 and using pictures from Servier Medical Art. Servier Medical Art by Servier, licensed under a Creative Commons Attribution 3.0 Unported License (https://creativecommons.org/licenses/by/3.0/).
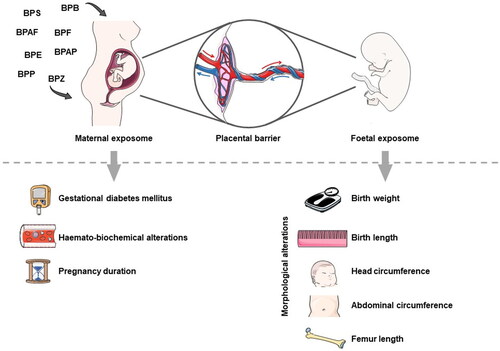
In a cohort study performed in China, Zhang et al. assessed the relationship between concentrations of BPS, BPF, and BPAF present in maternal urine samples collected during the first trimester and the disruption of glycolytic metabolism, associated with the development of gestational diabetes mellitus (Zhang, Xia, et al. Citation2019). Overall, the authors demonstrated that BPAF and BPS in maternal urine were related to higher urine glucose levels. However, the effects caused by BPS appear to be influenced by the sex of the developing fetus. Overall, these results indicate that BPS only disrupts glucose metabolism in women carrying a female fetus, which may be caused by differences in the content of hormones present in the maternal circulation. Furthermore, the authors reported that women with body weight before pregnancy within the reference values, when exposed to BPAF, appear to have a higher propensity to develop gestational diabetes mellitus, although these results should be interpreted with caution and must be confirmed in future studies. However, regarding BPF exposure, it was not possible to establish any relationship with changes in glycolytic metabolism (Zhang, Xia, et al. Citation2019).
In 2020, Kang et al. investigated the effects of BPS and BPF on hematopoiesis and maternal serological biochemical parameters (Kang et al. Citation2020). In the unadjusted model, it was possible to observe an association between the increase in BPS concentration and the decrease in erythrocytes, hemoglobin levels, and hematocrit. For BPF, on the contrary, there was a positive correlation between the parameters mentioned. However, when the models were adjusted the significance was lost. Therefore, although changes in hematological parameters could be indicative that BPS exposure could induce anemic consequences, in this study this relationship could not be established (Kang et al. Citation2020). However, in 2022, Jiang et al. performed a study in which they were able to associate prenatal exposure to a group of BPA substitutes (BPB, BPS, and BPF) with a decrease in hemoglobin levels and the consequent increased risk of anemia during pregnancy (Liang et al. Citation2022). Interestingly, these effects are thought to be essentially related to BPF exposure. According to the authors, this BPA substitute by increasing progesterone levels could impair hemoglobin homeostasis. In addition, it was observed that women carrying a male fetus had a greater susceptibility to developing these anemic consequences, which may be related to a disruption in the hormone’s levels, namely T, present in the maternal circulation. These authors hypothesized that this gender-specific effect could be linked to decreased T levels – caused by bisphenol exposure – and consequently decreased erythropoietin production (Liang et al. Citation2022). In summary, the results are still inconsistent and, consequently, the hematological effects of these compounds in humans are not very concrete, implying a need for further studies (Kang et al. Citation2020; Liang et al. Citation2022).
Over the years, several studies have been performed to assess the influence that BPA substitutes may have on the duration of pregnancy and the greater or lesser propensity for preterm birth (Wan et al. Citation2018; Aker et al. Citation2019; Aung et al. Citation2019; Huang et al. Citation2019; Pan et al. Citation2020). The results obtained in the various studies are quite diverse regarding BPS. In the study performed by Wan et al., it was concluded that higher concentrations of BPS in maternal urine are associated with a longer duration of pregnancy and a consequent lower likelihood of preterm birth. According to the literature, prolonged gestations (>42 weeks) can be associated with the development of post-term complications, such as meconium aspiration syndrome, shoulder dystocia, oligohydramnios, perinatal asphyxia, and higher risk of stillbirth (Chantry and Lopez Citation2011; Wan et al. Citation2018). However, in the study performed by Wan et al., there were no post-term deliveries since if labor had not yet occurred by 42 weeks it was induced. Therefore, in this investigation, the association between prolonged pregnancy, due to BPS exposure, and the development of post-term complications could not be analyzed (Wan et al. Citation2018). Furthermore, it was found that this positive correlation is more significant in women carrying a female fetus (Wan et al. Citation2018). According to the authors, the extension of pregnancy in cases where exposure to this EDC occurs may be related to progesterone levels increasing, which allows pregnancy maintenance to occur (Wan et al. Citation2018). However, the study performed by Aung et al. showed opposite results, as they observed that when BPS concentrations in maternal urine were higher, the probability of preterm birth during the third trimester increased (Aung et al. Citation2019). However, this second study has some limitations compared to the first, as the sample size was smaller, and sampling was performed in previous years when the use of this substitute was not yet so widespread (Aung et al. Citation2019). However, three other studies effectuated in 2019 and 2020 could not observe significant correlations between BPS concentration in maternal urine (Aker et al. Citation2019; Huang et al. Citation2019) or maternal/umbilical cord blood (Pan et al. Citation2020) and pregnancy’ duration. Regarding BPF, one study concluded that increased concentrations in maternal urine appear to be associated with an increased likelihood of preterm birth (Aker et al. Citation2019). In another study, no significant relation was observed between this EDC concentration in maternal/umbilical cord plasma and the duration of pregnancy (Pan et al. Citation2020). As for BPAF, only one study reported that high concentrations of this BPA substitute in cord blood were associated with an increased likelihood of preterm birth (Pan et al. Citation2020).
In addition, studies have been performed to determine what effects BPA substitutes have on various morphological parameters of the developing fetus (Ferguson et al. Citation2018; Mustieles et al. Citation2018; Aker et al. Citation2019; Hu et al. Citation2019; Pan et al. Citation2020). Some studies showed that when BPS was present in the maternal urine of women carrying a male fetus, the babies’ body weight at birth tended to decrease (Ferguson et al. Citation2018; Mustieles et al. Citation2018; Hu et al. Citation2019). However, in other studies, no significant correlations have been observed between the presence of these compounds in maternal urine or umbilical cord blood/plasma and this morphological characteristic (Ihde et al. Citation2018; Aker et al. Citation2019; Pan et al. Citation2020). The results were even less consistent when women were carrying a female fetus. The detection of BPS in maternal urine has already been associated with a tendency for increased – although not statistically significant (Mustieles et al. Citation2018) – and decreased birth weight – although less pronounced than in women carrying a male fetus (Hu et al. Citation2019). In other studies, no association was found between this EDC and birth body weight of newborn girls (Ferguson KK et al. Citation2018; Aker et al. Citation2019; Pan et al. Citation2020). Regarding BPF, one study associated the presence of this compound in maternal urine with decreased body weight, this being more pronounced in newborn boys (Hu et al. Citation2019), while others revealed no significant associations between this parameter and the presence of BPF in maternal urine (Ihde et al. Citation2018; Aker et al. Citation2019) and in cord blood (Ihde et al. Citation2018; Pan et al. Citation2020). Concerning BPAF, Pan et al. observed a negative correlation between cord blood BPAF concentrations and newborn body weight (Pan et al. Citation2020). Babies’ length at birth and during gestational age has also been assessed when BPS and BPF were detected in maternal urine (Aker et al. Citation2019; Hu et al. Citation2019). Therefore, these results suggest that detecting these EDCs is inversely related to body length (Hu et al. Citation2019). Regarding gestational length, the investigations demonstrated that BPS and BPF concentrations determined between the 24th and 28th weeks of pregnancy are associated with a small gestational age (SGA), i.e. the fetus is smaller than usual for the number of weeks of pregnancy (Aker et al. Citation2019). However, BPS concentrations detected between the 16th and 20th weeks of pregnancy appear to be associated with large gestational age (LGA), i.e. the fetus is larger than usual for its age (Aker et al. Citation2019). According to the authors, this discrepancy in effects caused by BPS may be related to the interaction of this EDC with the varying hormone levels associated with different periods of pregnancy. However, the results should be analyzed with caution and, ideally, further studies should be performed to understand better the dynamics associated (Aker et al. Citation2019). In addition, the head and abdominal circumferences and the size of the femur are relevant morphological parameters that can be evaluated (Ferguson et al. Citation2018; Mustieles et al. Citation2018). In the study performed by Ferguson et al., it was concluded that these three variables are inversely related to urine BPS concentrations in pregnant women carrying a male fetus. On the contrary, the authors found that in women carrying a female fetus, higher concentrations of this compound are directly related to a higher head circumference and femur size, while no statistical relationship could be established with abdominal circumference (Ferguson et al. Citation2018). Finally, in the work by Mustieles et al., no significant relationships were observed between this EDC and the head circumference (Mustieles et al. Citation2018).
In summary, BPA substitutes, namely BPS, BPF, and BPAF may cause adverse effects on the health of the mother and the developing fetus, and these effects may complicate children’s and their mothers’ future lives (Ferguson KK et al. Citation2018; Mustieles et al. Citation2018; Wan et al. Citation2018; Aker et al. Citation2019; Aung et al. Citation2019; Hu et al. Citation2019; Huang et al. Citation2019; Zhang, Xia, et al. Citation2019; Kang et al. Citation2020; Pan et al. Citation2020). However, it will be important to conduct further studies evaluating the concentrations of these compounds in human biological samples and determine their relationship with the possible development of maternal and fetal pathologies and anomalies, clarify the effects caused by these EDCs, and understand the mechanisms associated with these phenomena (Wan et al. Citation2018; Aker et al. Citation2019; Hu et al. Citation2019; Huang et al. Citation2019; Zhang, Xia, et al. Citation2019; Pan et al. Citation2020). Thus, it may be important to review the safety of replacing BPA with these analogs, as some studies seem to indicate some similarity between the adverse effects caused by them (Huang et al. Citation2019; Li et al. Citation2019; Zhang, Xia, et al. Citation2019; Pan et al. Citation2020).
5. Effects of exposure on the cardiovascular system
CVDs include a heterogeneous group of diseases related to a disturbance in the functioning and structure of the blood vessels and heart, such as atherosclerosis, myocardial infarction, hypertension, heart failure, dilated cardiomyopathy, coronary artery disease, arrhythmia, ischemia, and stroke, among others (Andersson and Vasan Citation2018; Soler-Botija et al. Citation2019; Zhang, Shan, et al. Citation2020). According to the World Health Organization (WHO), CVD are considered the leading cause of death worldwide (Soler-Botija et al. Citation2019; Zhang, Shan, et al. Citation2020; Lorigo and Cairrao Citation2022), and its incidence is expected to increase over the years (Lorigo and Cairrao Citation2022). Due to the increase in risk behaviors among young adults, who reside mainly in the Western world, there has been a higher incidence of these diseases in these age groups, which may become problematic in the future (Andersson and Vasan Citation2018). Therefore, if the effects caused by risk behaviors at these ages cannot be reversed, the number of individuals suffering from these types of pathologies at younger ages will be even higher, thus increasing the incidence of the disease (Andersson and Vasan Citation2018). Thus, the incidence of CVD may increase (Andersson and Vasan Citation2018). However, each person can make some efforts to avoid lifestyle risk factors, such as maintaining a healthy balanced diet, being active, not smoking or using substances of abuse (Van Camp Citation2014; Andersson and Vasan Citation2018; Francula-Zaninovic and Nola Citation2018). However, environmental risk factors are not easy to avoid, such as exposure to some EDCs that are ubiquitously present in the environment, such as BPA and its substitutes (Gore et al. Citation2015; Zhang, Shan, et al. Citation2020). Indeed, some epigenetic dysregulation that can be transmitted transgenerationally may predispose individuals to certain diseases later in life (Lorigo and Cairrao Citation2022). Epigenetic dysregulation such as alterations in methylation and acetylation patterns, genomic imprinting, modifications in microRNAs (miRNAs) and non-coding RNAs expressions may be transmitted to the developing fetus. Therefore, the machinery modulating methylation, chromatin organization, and gene expression may be altered, increasing the fetus’ susceptibility to endocrine disruption (Lorigo and Cairrao Citation2022). Thus, this genetic predisposition may potentially amplify the environmental risk factors associated with EDC exposure. Indeed, several studies have shown that exposure to BPA can be associated with higher prevalence of CVD (Melzer et al. Citation2010; Gore et al. Citation2015; Feiteiro et al. Citation2018; Zhang, Shan, et al. Citation2020; Lorigo and Cairrao Citation2022; Moon et al. Citation2021). For this reason, as BPA substitutes are increasingly being used to replace BPA in several everyday products, it is important to assess whether these compounds may also have a disruptive effect on the cardiovascular system (Gao et al. Citation2015; Vought and Wang Citation2018; Ferguson M et al. Citation2019; Yang, Cheng, et al. Citation2020; Zhang, Shan, et al. Citation2020).
5.1. In vivo animal studies
Over the years, there have been several studies in animal models, such as zebrafish (Ji et al. Citation2013; Song, Liang, et al. Citation2014; Moreman et al. Citation2017; Yang et al. Citation2017a, Citation2017b; Mu et al. Citation2018; Gu et al. Citation2020; Mi et al. Citation2020; Qiu et al. Citation2020; Qin et al. Citation2021), rodents (Gao et al. Citation2015; Kasneci et al. Citation2017; Pal et al. Citation2017), worms (Vought and Wang Citation2018), and planktonic crustaceans (Liu J et al. Citation2020) with the aim of assessing whether there is any relationship between exposure to these compounds and the development of anomalies in the cardiovascular system ().
Table 3. Effects of BPA substitutes on the cardiovascular system of various organisms..
In 2020, three studies were performed on zebrafish where the relationship between exposure to BPAF (Gu et al. Citation2020), BHPF (Mi et al. Citation2020), and BPS (Qiu et al. Citation2020) and the development of cardiotoxicity were observed. Overall, Gu et al. found that BPAF inhibited the activity of some antioxidant enzymes (e.g. Sod, Cat, Cu/Zn-SOD), increasing oxidative stress, which in turn increased lipid peroxidation – as evidenced by increased malondialdehyde (Mda) levels (Gu et al. Citation2020). Furthermore, in this study, exposure to this compound promoted the development of mitochondrial dysfunctions, culminating in activation of the apoptotic p53/caspases pathway in the cardiac region (Gu et al. Citation2020). Moreover, in this research, the authors observed that exposure to this EDC disrupted the expression of genes involved in the heart’ development and normal functioning. BPAF altered gene expression of nkx2.5, gata5, ta, noto, and tbx6 that participate in cardiac tube loop formation; cardiomyocyte differentiation, migration, and proliferation; and heart’ morphogenesis (Gu et al. Citation2020). Similar results were obtained in the study performed by Mi et al. (Citation2020). In this study, BHPF exposure was related to the disruption of the cardiac loop formation that is required to individualize the atrium from the ventricle in these animals’ hearts. This disturbance can be seen by the increased distance from the sinus venosus to the bulbus arteriosus (SV-BA distance). In addition, decreased expression of nkx2.5, tbx5a, and gata4 genes was also observed in this study. Interestingly, for the lower concentrations of BHPF, was observed an increase in the gene expression of myl7, which is involved in the proliferation of cardiac muscle cells, contraction processes of the heart, and myofibril assembly. This result reflects the increased proliferation of cardiomyocytes in the atrium and ventricle, as a compensatory mechanism for the cardiac abnormalities caused by this compound (Mi et al. Citation2020). On the other hand, in work by Qiu et al. BPS exposure was found to alter some immune parameters (e.g. levels of cytokines, IL-12, and NO synthetase), which disturbed the heart’ development and function (Qiu et al. Citation2020).
One of the most frequently observed consequences, in zebrafish at embryonic and larval stages, after exposure to BPAF (Song, Liang, et al. Citation2014; Moreman et al. Citation2017; Mu et al. Citation2018), BPF (Moreman et al. Citation2017; Yang et al. Citation2017a, Citation2017b; Mu et al. Citation2018), BPS (Ji et al. Citation2013; Moreman et al. Citation2017), and BHPF (Mi et al. Citation2020) is the development of cardiac malformations such as, cardiac (Ji et al. Citation2013; Moreman et al. Citation2017) and pericardiac edemas (Song, Liang, et al. Citation2014; Yang et al. Citation2017a, Citation2017b; Mu et al. Citation2018; Mi et al. Citation2020). In zebrafish, BHPF exposure was also associated with increased pericardium area (Mi et al. Citation2020). Regarding the heart morphology of these animals, exposure to BPAF decreased the number of cardiomyocytes and endocardial cells, decreasing the size of this organ (Gu et al. Citation2020). On the other hand, exposure to BHPF treatments was also associated with reducing the number of cardiomyocytes in the largest section plane of the ventricle and atrium (Mi et al. Citation2020). However, in the study by Qiu et al. exposure of zebrafish to BPS was related to increased atrial size and atrial to ventricle area, i.e. according to these authors, this compound promoted hypertrophy of the zebrafish heart (Qiu et al. Citation2020). Thus, the authors concluded that these EDCs can trigger the onset of cardiovascular lesions (Song, Liang, et al. Citation2014; Gu et al. Citation2020). In the study performed by Moreman et al., the exposure of zebrafish embryos to BPAF and BPF in the first days of life promoted the occurrence of cranial hemorrhages, probably due to vascular damage (Moreman et al. Citation2017). This may be related to the weakening of the local blood vessel network due to the disruption of the signaling pathways associated with thyroid and E2 caused by these compounds (Moreman et al. Citation2017).
On the other hand, to analyze the effects that EDCs cause on cardiovascular functions, the contractile function of the heart (Gao et al. Citation2015) and its related parameters, such as heart rate (Gao et al. Citation2015; Vought and Wang Citation2018; Gu et al. Citation2020; Liu et al. Citation2020; Mi et al. Citation2020; Qin et al. Citation2021), systolic volume (Mi et al. Citation2020), cardiac output (Mi et al. Citation2020) and longitudinal shortening of the ventricle (Mi et al. Citation2020) are often evaluated. Gao et al. demonstrated that exposure of female rats to BPS promoted an increase in the phosphorylation of PLN and ryanodine receptors (RyR) – which are involved in cardiac contractility processes (Gao et al. Citation2015). Thus, this compound, by interfering with the Ca2+ uptake and release from the sarcoplasmic reticulum, may increase the incidence of spontaneous and aberrant excitation events at the myocyte level. Therefore, at the cellular level, this EDC can increase the occurrence of arrhythmogenic events that in certain pathophysiological conditions can propagate in the myocardium, increasing the risk of developing arrhythmias. However, in this study, at the whole-heart level, no arrhythmia events were observed under normal conditions, although a moderate increase in heart rate was noted. It is also important to mention that also in this study, the events caused in female cardiomyocytes seem to be related to the activation of ERβ and, consequently, to its signaling pathway. However, once again, in this study, it was not possible to observe significant changes in males, which according to the authors, may be explained by the simultaneous activation of ERα and ERβ, which ends up nullifying the stimulatory effects associated with ERβ activation (Gao et al. Citation2015).
Regarding to heart rate, between 2018 and 2021, studies were performed in zebrafish (Gu et al. Citation2020; Mi et al. Citation2020; Qiu et al. Citation2020; Qin et al. Citation2021), Lumbriculus variegatus worms (Vought and Wang Citation2018) and female Daphnia magna planktonic crustaceans (Liu et al. Citation2020). In worms, the dorsal blood vessel is the phylogenetic homolog of higher organisms’ hearts (Vought and Wang Citation2018). Vought et al. reveal that exposure of these animals to BPS decreased the interval of the dorsal blood vessel pulsations, thereby increasing the pulse rate (Vought and Wang Citation2018). On the contrary, exposure of D. magna females to BPF caused a decrease in heart rate (Liu et al. Citation2020). In zebrafish, it was generally observed that the compounds BPAF (Gu et al. Citation2020), BHPF (Mi et al. Citation2020) and BPS (Qiu et al. Citation2020; Qin et al. Citation2021) tended to reduce heart rate, although in the study performed by Qin et al. it was found that, 48 h after fertilization, the F1 embryos exhibited an increase in heart rate (Qin et al. Citation2021). On the other hand, in the study where zebrafish embryos were exposed to BHPF, there was a decrease in heart rate, reduction in diastolic volume and cardiac output, and fractional shortening of the ventricle, which again reinforces the idea that this EDC can directly disrupt cardiac functions (Mi et al. Citation2020).
To assess the potential risks of these compounds to the cardiovascular system, it is also possible to determine various hematological and biochemical parameters in the serum (Pal et al. Citation2017). Thus, in 2017, a study was performed in adult male rats, where it was possible to establish a relationship between exposure to BPS and the disturbance of these serological parameters, which, in turn, may trigger specific processes in the body associated with a higher risk of developing CVD (Pal et al. Citation2017). This EDC was found to decrease the number of erythrocytes present in the circulation, probably due to interference with erythropoiesis. Furthermore, a reduction in hemoglobin concentration was observed, which may be explained by inhibition of erythropoiesis and hemoglobin synthesis and/or by the occurrence of hemolysis. Indeed, hemolysis was found to occur, as evidenced by increased bilirubin concentrations. On the other hand, this compound was associated with decreased coagulation time and increased serum Ca2+ levels. Since Ca2+ plays an essential role in cardiac rhythm, changes in serological levels of this ion may be of concern and could indicate, once again, a possible relationship between BPS and the development of cardiac arrhythmias in these animal models. A decrease in the number of circulating leucocytes has also been observed so this EDC may suppress some immune system functions. As previously described for the prenatal BPS exposure situations (see topic 3.2.), glucose and lipid metabolisms were disturbed here too. Hence, in this case, there was an increase in serological concentrations of glucose, TG, free fatty acids, cholesterol, low-density lipoprotein (LDL), and very LDL (VLDL). On the contrary, a decrease in high-density lipoprotein (HDL) levels has been observed. In this sense, this EDC being associated with these alterations in the lipid profile may be linked to the promotion of atherosclerotic plaque formation, which is one of the significant risk factors for the development of CVD. Moreover, the authors also found that rats exposed to BPS treatments showed hyperproteinemia that is possibly related to liver tissue damage, which in turn, may be correlated with cardiac problems. These results can be evidenced by the increased activity of the enzymes a lanine aminotransferase (ALT), aspartate aminotransferase (AST), and alkaline phosphatase (ALP). Finally, they observed an increase in urea concentrations, an indicator of heart failure. Thus, BPS might be considered a cardiovascular risk-inducing compound (Pal et al. Citation2017). In addition, another study done in 2017, found this compound to be a risk factor for the cardiovascular system in mice, after being induced a myocardial infarction (Kasneci et al. Citation2017). Continuous exposure to BPS after myocardial infarction increases the pro-inflammatory state in the injured area, which culminates in the development of complications such as cardiac rupture and left ventricular dysfunction, reducing these animals’ recovery. Thus, mice exposed to treatments with this EDC end up dying prematurely due to cardiac rupture. However, when they survive, the left ventricle is not functioning correctly, as evidenced by its dilation and the reduction in fractional area change ([left ventricular area in diastole – left ventricular area in systole]/left ventricular area in systole) (Kasneci et al. Citation2017).
5.2. In vitro animal studies
Since 2019, several in vitro studies were also performed to assess the contractility modulations (Ferguson M et al. Citation2019; Prudencio et al. Citation2021) and cardiotoxicity of BPS, BPF (Yin et al. Citation2019; Prudencio et al. Citation2021), and BPAF (Yang, Cheng, et al. Citation2020; Zhou et al. Citation2021). Ferguson et al. performed a study in which they exposed isolated perfused mouse hearts to BPS to determine what the consequences were for the contractile function of this organ (Ferguson M et al. Citation2019). Thus, the authors demonstrated that BPS, by interacting with ERβ, exerts an immediate depressant effect on these hearts, and this, in turn, appears to be related to a decrease in systolic pressure and rates of contraction and relaxation. In addition, they evaluated the phosphorylation profiles of the myofilaments that constitute the contractile apparatus and of the protein phospholamban (PLN) that functions as a regulator of contractile function. When phosphorylated, PLN dissociates from sarcoplasmic reticulum Ca2+-ATPase (SERCA), activating the Ca2+ pump – present in the sarcoplasmic reticulum membrane – which consequently increases Ca2+ removal from the cytoplasm to the sarcoplasmic reticulum. This process culminates in an increased relaxation rate and, subsequently, an enhanced myocardial contraction (MacLennan and Kranias Citation2003; Ferguson et al. Citation2019). Thus, these researchers found that exposure to this compound promoted the development of changes in the phosphorylation pattern of myofilaments that differ between genders and could directly affect myocardial contractile function. However, these variations probably end up canceling each other out without changing the myofilament function. Furthermore, they found that exposure of female hearts to BPS increased PLN phosphorylation at serine 16 and decreased threonine 17 phosphorylation. However, in males, no significant changes were observed in the phosphorylation profiles of PLN. Thus, the myocardial response after exposure to this compound shows differences between genders that, according to the authors, may be related to different phosphorylation patterns of ERβ, although further studies are needed to prove this (Ferguson M et al. Citation2019). Therefore, by decreasing myocardial contractility, particularly in the left ventricle, this compound can reduce the heart’s ability to function correctly, which in turn can trigger the occurrence of coronary hypoperfusion, increasing the risk of developing ischemic heart disease (Ferguson et al. Citation2019). In the same year, Yin et al. have established a relationship between exposure of mouse embryoid bodies (obtained from differentiated mouse embryonic stem cells via 3D structures) to BPF and BPS and the development of CVD (Yin et al. Citation2019). The authors associated these compounds with increased frequency of right ventricular cardiomyopathies (dilation, hypertrophy, and arrhythmia), cardiac and vascular smooth muscle contraction, and a greater propensity to develop myocarditis. In recent years, investigations have been performed on cardiomyocytes derived from differentiated embryonic stem cell line R1 (ESC R1), where they were exposed to various concentrations of BPAF (Yang, Cheng et al. Citation2020; Zhou et al. Citation2021). Yang et al. found that increased concentrations of this EDC were directly related to the number of cardiomyocytes undergoing apoptosis. In addition, they discovered that exposure to this BPA substitute promoted cardiomyocyte hypertrophy (Yang, Cheng, et al. Citation2020). On the other hand, in the work performed by Zhou et al. (Citation2021) concordant results were obtained. It is thought that this cell size increase may be explained by the interference of this EDC in the p38-MAPK pathway, which consequently increases the expression of collagen and promotes the development of cardiomyocyte hypertrophy, affecting the normal development of the heart (Zhou et al. Citation2021). In 2021, a study was performed where the direct nongenomic effects of BPF and BPS on cardiac electrophysiology were assessed (Prudencio et al. Citation2021). For this purpose, human-induced pluripotent stem cell-derived cardiomyocytes (hiPSC-CM) were used. Overall, these researchers found that BPF exposure caused an inhibitory effect on these cells’ ion channels, inhibiting Na+, Ca2+, and K+ currents. These alterations, namely at the Ca2+ current level, may be of concern, since Ca2+ channels, in cardiac tissue, are involved in important processes, such as nodal cell depolarization, atrioventricular conduction, the plateau phase, conduction of cardiac action potential, and contractility (Prudencio et al. Citation2021). However, it was impossible to observe these alterations for the BPS exposure cases. Moreover, in the same study, the effects associated with exposure of isolated and perfused rat hearts to BPS and BPF were analyzed (Prudencio et al. Citation2021). The exposure to BPF was associated with decreased heart rate and atrioventricular conduction and increased atrioventricular nodal refractoriness. However, in the BPS exposure cases, it was impossible to observe these alterations (Prudencio et al. Citation2021).
Thus, it is evident that in these in vitro models it is also possible to observe a decrease in cardiac responsivity, decreasing the heart rate and blood pressure, promoting the cardiotoxic and hypertrophic effects associated with BPA substitutes (Yin et al. Citation2019; Yang, Cheng, et al. Citation2020; Prudencio et al. Citation2021).
5.3. Epidemiological studies
Although studies assessing the effects of BPA substitutes at the human cardiovascular level are relatively scarce, in 2021, Jiang et al. found that the concentrations of BPS and BPF in the urine of hypertensive patients admitted to a hospital in Wuhan were statistically higher than those of patients without hypertension (Jiang et al. Citation2021). Considering these results, the authors concluded that these compounds might exert some hypertensive effects in humans, although further studies are needed to confirm it (Jiang et al. Citation2021). In 2022, Wang et al. discovered that exposure to BPS was associated with the development of CVD in older ages (50–80 years) (Wang R et al. Citation2022). Furthermore, these authors related this exposure to a higher risk of developing coronary heart disease. However, these authors did not find an association between exposure to BPF and the development of CVD (Wang R et al. Citation2022).
In summary, in animal models, BPA substitutes, such as BPS (Ji et al. Citation2013; Gao et al. Citation2015; Kasneci et al. Citation2017; Moreman et al. Citation2017; Pal et al. Citation2017; Vought and Wang Citation2018; Ferguson M et al. Citation2019; Qiu et al. Citation2020; Qin et al. Citation2021), BPF (Moreman et al. Citation2017; Yang et al. Citation2017a, Citation2017b; Mu et al. Citation2018; Liu et al. Citation2020), BPAF (Song, Liang, et al. Citation2014; Moreman et al. Citation2017; Mu et al. Citation2018; Gu et al. Citation2020), and BHPF (Mi et al. Citation2020) can modify the morphology and function of cardiovascular system components, promoting greater cardiotoxicity that can then culminate in the development of CVD. Furthermore, these consequences have also been observed in in vitro models (Yin et al. Citation2019; Yang, Cheng, et al. Citation2020; Zhou et al. Citation2021). These findings suggest that mentioned models can be beneficial in understanding the effects and mechanisms associated with these processes and could, therefore, be a good area of study in the future. However, it should be noted that studies directly evaluating the effects of the BPA substitutes on humans’ cardiovascular system are still very scarce (Jiang et al. Citation2021). However, the current epidemiological studies have been associated exposure to EDCs with the development of CVD. In this sense, some of the most observed CDV associated with EDCs exposure are hypertensive disorders, atherosclerosis, and coronary heart disease (Gore et al. Citation2015; Mariana et al. Citation2016; Fu et al. Citation2020; Lorigo and Cairrao Citation2022; Migliaccio et al. Citation2021; Vuong et al. Citation2021; Dias et al. Citation2022; Fonseca et al. Citation2022). Therefore, it will be important to conduct further studies to understand better the relationships between ubiquitous human exposure to these compounds and the development of CVD. It is therefore essential to determine whether it is indeed safe to replace BPA with its analogs, as several studies have already proven that, for example, concerning the cardiovascular system, these compounds present health risks (Gao et al. Citation2015; Vought and Wang Citation2018; Ferguson et al. Citation2019; Yang, Cheng, et al. Citation2020).
6. Conclusion
In conclusion, BPA substitutes can affect maternal and fetal health in several animal models and humans. These compounds have been detected in several biological samples and can be transferred via the placenta from the mother to the fetus. Furthermore, some studies have already established relationships between exposure to these EDCs and the development of CVD, especially in animal models, where research is more extensive. Overall, the studies seem to indicate that BPA substitutes may induce the same type of health problems as BPA. However, the mechanisms by which these effects are stimulated are not always the same as those for BPA. Therefore, in the future, epidemiological studies and studies in animal models should be continued so that it will be possible to understand better and establish more precisely what effects BPA substitutes may have on human and animal health. It will also be essential to determine which mechanisms are associated with the pathophysiology of the diseases and which characteristics are promoted after exposure to these compounds, namely at the CVD level. Further investigations should be performed to understand how these pathologies can be prevented and assess whether BPA’s replacement by its analogs is beneficial or leads to more adverse health effects.
Authors statement
Fatima Abrantes-Soares was responsible for the investigation; writing the original draft; writing, reviewing, and editing the manuscript. Margarida Lorigo was responsible for supervision; and writing, reviewing, and editing the manuscript. Elisa Cairrao was responsible for conceptualization; funding acquisition; project administration; supervision; visualization; and writing, reviewing, and editing the manuscript.
Abbreviations | ||
5-HT | = | Serotonin |
AGD | = | Ano-genital distance |
AlloP | = | Allopregnanolone |
ALP | = | Alkaline phosphatase |
ALT | = | Alanine aminotransferase |
AST | = | Aspartate aminotransferase |
BHPF | = | Bisphenol HPF (4-[9-(4-[9-(4-hydroxyphenyl)fluoren-9-yl]phenol) |
BPA | = | Bisphenol A (4-[2-(4-hydroxyphenyl)propan-2-yl]phenol) |
BPAF | = | Bisphenol AF (4-[1,1,1,3,3,3-hexafluoro-2-(4-hydroxyphenyl)propan-2-yl]phenol) |
BPAP | = | Bisphenol AP (4-[1-(4-hydroxyphenyl)-1-phenylethyl]phenol) |
BPB | = | Bisphenol B (4-[2-(4-hydroxyphenyl)butan-2-yl]phenol) |
BPE | = | Bisphenol E (4-[1-(4-hydroxyphenyl)ethyl]phenol |
BPF | = | Bisphenol F (4-[(4-hydroxyphenyl)methyl]phenol) |
BPP | = | Bisphenol P (4-[2-[4-[2-(4-hydroxyphenyl)propan-2-yl]phenyl]propan-2-yl]phenol) |
BPS | = | Bisphenol S (4-(4-hydroxyphenyl)sulfonylphenol) |
BPZ | = | Bisphenol Z (4-[1-(4-hydroxyphenyl)cyclohexyl]phenol |
BSI | = | Brain somatic index (BSI = brain weight × 100/body weight) |
BW | = | Body weight |
Ca2+ | = | Calcium |
CAT | = | Catalase |
cMPOA | = | Central medial preoptic area |
CVD | = | Cardiovascular diseases |
DA | = | Dopamine |
DHEA | = | Dehydroepiandrosterone |
DOHaD | = | Developmental Origins of Health and Disease |
E2 | = | 17β-estradiol |
EDC | = | Endocrine-disrupting chemicals |
epiWAT | = | Epididymal white adipose tissues |
ERα | = | Estrogen receptor alpha |
ERβ | = | Estrogen receptor beta |
ESC R1 | = | Embryonic stem cell line R1 |
FSH | = | Follicle-stimulating hormone |
GSI | = | Gonadosomatic index (GSI = gonad weight × 100/body weight) |
HDL | = | High-density lipoprotein |
hiPSC-CM | = | Human induced pluripotent stem cell-derived cardiomyocytes |
HPG axis | = | Hypothalamic-pituitary-gonadal axis |
HPT axis | = | Hypothalamic-pituitary-thyroid axis |
HSI | = | Hepatosomatic index (HSI = liver weight × 100/body weight) |
HTA | = | Head-trunk angle |
K+ | = | Potassium |
LDL | = | Low-density lipoprotein |
LGA | = | Large gestational age |
LH | = | Luteinizing hormone |
Mda | = | Malondialdehyde |
miRNAs | = | MicroRNAs |
Na+ | = | Sodium |
NR | = | Nipple retention |
OVL | = | Otic vesicle length |
PAMPs | = | Pathogen-associated molecular patterns |
PFC | = | Prefrontal cortex |
PLN | = | Phospholamban |
PND | = | Postnatal day |
PSD-95 | = | Protein postsynaptic density 95 |
PTE | = | Placental transfer efficiency |
RCM | = | Cord − maternal concentration ratio |
RPM | = | Placental − maternal concentration ratio |
RyR | = | Ryanodine receptors |
SERCA | = | Sarcoplasmic reticulum Ca2+-ATPase |
SGA | = | Small gestational age |
SV-BA distance | = | Distance from the sinus venosus to the bulbus arteriosus |
T | = | Testosterone |
T3 | = | Triiodothyronine |
T4 | = | Tetraiodothyronine; thyroxine |
TG | = | Triglyceride |
TH | = | Thyroid hormones |
UGT | = | UDP-glucuronosyltransferase |
VLDL | = | Very low-density lipoprotein |
WHO | = | World Health Organization |
Acknowledgements
The authors have no further acknowledgements to declare. This article was prepared only by the authors, whose contributions are declared below. The authors would like to acknowledge the Editor-in-Chief, Dr. Roger O. McClellan (Independent Advisor, Toxicology and Human Health Risk Analysis, Albuquerque, New Mexico, USA) and the two anonymous reviewers selected by the Editor for their suggestions and significant input which has improved the manuscript to its current form.
Declaration of interest
The authors’ affiliations are shown on the cover page. All experts that provided input or review are coauthors or are included in the acknowledgments section. The authors have sole responsibility for the writing and content of the article. The authors report no conflict of interest. At the time of writing all authors are affiliated to the University of Beira Interior. None of the authors have participated in any legal, regulatory, or advocacy proceedings related to the contents of the article. This work was supported by the Foundation for Science and Technology (Fundação para a Ciência e a Tecnologia [FCT]), through funds from the State Budget, and by the European Regional Development Fund (ERDF), under the Portugal 2020 Program, through the Regional Operational Program of the Center (Centro2020), through the Projects with the references (UIDB/00709/2020 + UIDP/00709/2020). M.L. acknowledges the Ph.D. fellowship from FCT (Reference: 2020.06616.BD).
References
- Ahn YA, Baek H, Choi M, Park J, Son SJ, Seo HJ, Jung J, Seong JK, Lee J, Kim S. 2020. Adipogenic effects of prenatal exposure to bisphenol S (BPS) in adult F1 male mice. Sci Total Environ. 728:138759.
- Aker AM, Ferguson KK, Rosario ZY, Mukherjee B, Alshawabkeh AN, Cordero JF, Meeker JD. 2019. The associations between prenatal exposure to triclocarban, phenols and parabens with gestational age and birth weight in northern Puerto Rico. Environ Res. 169:41–51.
- Andersson C, Vasan RS. 2018. Epidemiology of cardiovascular disease in young individuals. Nat Rev Cardiol. 15(4):230–240.
- Aung MT, Ferguson KK, Cantonwine DE, McElrath TF, Meeker JD. 2019. Preterm birth in relation to the bisphenol A replacement, bisphenol S, and other phenols and parabens. Environ Res. 169:131–138.
- Bakoyiannis I, Kitraki E, Stamatakis A. 2021. Endocrine-disrupting chemicals and behaviour: a high risk to take? Best Pract Res Clin Endocrinol Metab. 35(5):101517.
- Banker M, Puttabyatappa M, O'Day P, Goodrich JM, Kelley AS, Domino SE, Smith YR, Dolinoy DC, Song PXK, Auchus RJ, et al. 2021. Association of maternal-neonatal steroids with early pregnancy endocrine disrupting chemicals and pregnancy outcomes. J Clin Endocrinol Metab. 106(3):665–687.
- Björnsdotter MK, Jonker W, Legradi J, Kool J, Ballesteros-Gómez A. 2017. Bisphenol A alternatives in thermal paper from the Netherlands, Spain, Sweden and Norway. Screening and potential toxicity. Sci Total Environ. 601–602:210–221.
- Brulport A, Lencina C, Chagnon MC, Le Corre L, Guzylack-Piriou L. 2021. Transgenerational effects on intestinal inflammation status in mice perinatally exposed to bisphenol S. Chemosphere. 262:128009.
- Cabaton N, Chagnon MC, Lhuguenot JC, Cravedi JP, Zalko D. 2006. Disposition and metabolic profiling of bisphenol F in pregnant and nonpregnant rats. J Agric Food Chem. 54(26):10307–10314.
- Castro B, Sánchez P, Torres JM, Ortega E. 2015. Bisphenol A, bisphenol F and bisphenol S affect differently 5α-reductase expression and dopamine-serotonin systems in the prefrontal cortex of juvenile female rats. Environ Res. 142:281–287.
- Catanese MC, Vandenberg LN. 2017. Bisphenol S (BPS) alters maternal behavior and brain in mice exposed during pregnancy/lactation and their daughters. Endocrinology. 158(3):516–530.
- Česen M, Lenarčič K, Mislej V, Levstek M, Kovačič A, Cimrmančič B, Uranjek N, Kosjek T, Heath D, Dolenc MS, et al. 2018. The occurrence and source identification of bisphenol compounds in wastewaters. Sci Total Environ. 616-617:744–752.
- Chantry AA, Lopez E. 2011. Fetal and neonatal complications related to prolonged pregnancy. J Gynecol Obstet Biol Reprod (Paris). 40(8):717–725.
- Chen D, Kannan K, Tan H, Zheng Z, Feng YL, Wu Y, Widelka M. 2016. Bisphenol analogues other than BPA: environmental occurrence, human exposure, and toxicity-A review. Environ Sci Technol. 50(11):5438–5453.
- da Silva BS, Pietrobon CB, Bertasso IM, Lopes BP, Carvalho JC, Peixoto-Silva N, Santos TR, Claudio-Neto S, Manhães AC, Oliveira E, et al. 2019. Short and long-term effects of bisphenol S (BPS) exposure during pregnancy and lactation on plasma lipids, hormones, and behavior in rats. Environ Pollut. 250:312–322.
- Diamanti-Kandarakis E, Bourguignon JP, Giudice LC, Hauser R, Prins GS, Soto AM, Zoeller RT, Gore AC. 2009. Endocrine-disrupting chemicals: an endocrine society scientific statement. Endocr Rev. 30(4):293–342.
- Dias P, Tvrdý V, Jirkovský E, Dolenc MS, Peterlin Mašič L, Mladěnka P. 2022. The effects of bisphenols on the cardiovascular system. Crit Rev Toxicol. 52(1):66–87.
- Dong X, Zhang Z, Meng S, Pan C, Yang M, Wu X, Yang L, Xu H. 2018. Parental exposure to bisphenol A and its analogs influences zebrafish offspring immunity. Sci Total Environ. 610–611:291–297.
- Eckardt M, Simat TJ. 2017. Bisphenol A and alternatives in thermal paper receipts - a German market analysis from 2015 to 2017. Chemosphere. 186:1016–1025.
- Eladak S, Grisin T, Moison D, Guerquin MJ, N'Tumba-Byn T, Pozzi-Gaudin S, Benachi A, Livera G, Rouiller-Fabre V, Habert R. 2015. A new chapter in the bisphenol A story: bisphenol S and bisphenol F are not safe alternatives to this compound. Fertil Steril. 103(1):11–21.
- Feiteiro J, Mariana M, Glória S, Cairrao E. 2018. Inhibition of L-type calcium channels by Bisphenol A in rat aorta smooth muscle. J Toxicol Sci. 43(10):579–586.
- Ferguson KK, Meeker JD, Cantonwine DE, Mukherjee B, Pace GG, Weller D, McElrath TF. 2018. Environmental phenol associations with ultrasound and delivery measures of fetal growth. Environ Int. 112:243–250.
- Ferguson M, Lorenzen-Schmidt I, Pyle WG. 2019. Bisphenol S rapidly depresses heart function through estrogen receptor-beta and decreases phospholamban phosphorylation in a sex-dependent manner. Sci Rep. 9(1):15948.
- Flint S, Markle T, Thompson S, Wallace E. 2012. Bisphenol A exposure, effects, and policy: a wildlife perspective. J Environ Manage. 104:19–34.
- Fonseca MI, Lorigo M, Cairrao E. 2022. Endocrine-disrupting effects of bisphenol A on the cardiovascular system: a review. J Xenobiot. 12(3):181–213.
- Francula-Zaninovic S, Nola IA. 2018. Management of measurable variable cardiovascular disease’ risk factors. Curr Cardiol Rev. 14(3):153–163.
- Fu X, Xu J, Zhang R, Yu J. 2020. The association between environmental endocrine disruptors and cardiovascular diseases: a systematic review and meta-analysis. Environ Res. 187:109464.
- Gao X, Ma J, Chen Y, Wang HS. 2015. Rapid responses and mechanism of action for low-dose bisphenol S on ex vivo rat hearts and isolated myocytes: evidence of female-specific proarrhythmic effects. Environ Health Perspect. 123(6):571–578.
- Ghassabian A, Trasande L. 2018. Disruption in thyroid signaling pathway: a mechanism for the effect of endocrine-disrupting chemicals on child neurodevelopment. Front Endocrinol (Lausanne). 9:204.
- Gingrich J, Pu Y, Roberts J, Karthikraj R, Kannan K, Ehrhardt R, Veiga-Lopez A. 2018. Gestational bisphenol S impairs placental endocrine function and the fusogenic trophoblast signaling pathway. Arch Toxicol. 92(5):1861–1876.
- Gingrich J, Ticiani E, Veiga-Lopez A. 2020. Placenta disrupted: endocrine disrupting chemicals and pregnancy. Trends Endocrinol Metab. 31(7):508–524.
- Gong M, Huai Z, Song H, Cui L, Guo Q, Shao J, Gao Y, Shi H. 2017. Effects of maternal exposure to bisphenol AF on emotional behaviors in adolescent mice offspring. Chemosphere. 187:140–146.
- Gore AC, Chappell VA, Fenton SE, Flaws JA, Nadal A, Prins GS, Toppari J, Zoeller RT. 2015. EDC-2: the Endocrine Society’s Second Scientific Statement on Endocrine-Disrupting Chemicals. Endocr Rev. 36(6):E1–e150. eng.
- Gramec Skledar D, Peterlin Mašič L. 2016. Bisphenol A and its analogs: do their metabolites have endocrine activity? Environ Toxicol Pharmacol. 47:182–199.
- Grandin FC, Lacroix MZ, Gayrard V, Gauderat G, Mila H, Toutain PL, Picard-Hagen N. 2018. Bisphenol S instead of bisphenol A: toxicokinetic investigations in the ovine materno-feto-placental unit. Environ Int. 120:584–592.
- Grandin FC, Lacroix MZ, Gayrard V, Viguié C, Mila H, de Place A, Vayssière C, Morin M, Corbett J, Gayrard C, et al. 2019. Is bisphenol S a safer alternative to bisphenol A in terms of potential fetal exposure? Placental transfer across the perfused human placenta. Chemosphere. 221:471–478.
- Gu J, Wang H, Zhou L, Fan D, Shi L, Ji G, Gu A. 2020. Oxidative stress in bisphenol AF-induced cardiotoxicity in zebrafish and the protective role of N-acetyl N-cysteine. Sci Total Environ. 731:139190.
- Gumusoglu S, Scroggins S, Vignato J, Santillan D, Santillan M. 2021. The serotonin-immune axis in preeclampsia. Curr Hypertens Rep. 23(7):37.
- Hill CE, Sapouckey SA, Suvorov A, Vandenberg LN. 2017. Developmental exposures to bisphenol S, a BPA replacement, alter estrogen-responsiveness of the female reproductive tract: a pilot study. Cogent Medicine. 4(1):1317690.
- Hu J, Zhao H, Braun JM, Zheng T, Zhang B, Xia W, Zhang W, Li J, Zhou Y, Li H, et al. 2019. Associations of trimester-specific exposure to bisphenols with size at birth: a Chinese prenatal cohort study. Environ Health Perspect. 127(10):107001.
- Huang S, Li J, Xu S, Zhao H, Li Y, Zhou Y, Fang J, Liao J, Cai Z, Xia W. 2019. Bisphenol A and bisphenol S exposures during pregnancy and gestational age - A longitudinal study in China. Chemosphere. 237:124426.
- Ihde ES, Zamudio S, Loh JM, Zhu Y, Woytanowski J, Rosen L, Liu M, Buckley B. 2018. Application of a novel mass spectrometric (MS) method to examine exposure to Bisphenol-A and common substitutes in a maternal fetal cohort. Hum Ecol Risk Assess. 24(2):331–346.
- Ivry Del Moral L, Le Corre L, Poirier H, Niot I, Truntzer T, Merlin JF, Rouimi P, Besnard P, Rahmani R, Chagnon MC. 2016. Obesogen effects after perinatal exposure of 4,4'-sulfonyldiphenol (Bisphenol S) in C57BL/6 mice. Toxicology. 357–358:11–20.
- James WH. 2008. The variations of human sex ratio at birth with time of conception within the cycle, coital rate around the time of conception, duration of time taken to achieve conception, and duration of gestation: a synthesis. J Theor Biol. 255(2):199–204.
- Ji K, Hong S, Kho Y, Choi K. 2013. Effects of bisphenol s exposure on endocrine functions and reproduction of zebrafish. Environ Sci Technol. 47(15):8793–8800.
- Jiang S, Yang G, Zhou S, Zhang X, Peng C, Lu Q. 2021. Bisphenol A, S, and F exposure, ESR1/2, CAT, and eNOS genetic polymorphisms, and the risk of hypertension. Ecotoxicol Environ Saf. 224:112684.
- Kaimal A, Al Mansi MH, Dagher JB, Pope C, Varghese MG, Rudi TB, Almond AE, Cagle LA, Beyene HK, Bradford WT, et al. 2021. Prenatal exposure to bisphenols affects pregnancy outcomes and offspring development in rats. Chemosphere. 276:130118.
- Kang S, Shin BH, Kwon JA, Lee CW, Park EK, Park EY, Kim B. 2020. Urinary bisphenol A and its analogues and haemato-biochemical alterations of pregnant women in Korea. Environ Res. 182:109104.
- Kasneci A, Lee JS, Yun TJ, Shang J, Lampen S, Gomolin T, Cheong CC, Chalifour LE. 2017. From the cover: lifelong exposure of C57bl/6n male mice to bisphenol A or bisphenol S reduces recovery from a myocardial infarction. Toxicol Sci. 159(1):189–202.
- Kelley AS, Banker M, Goodrich JM, Dolinoy DC, Burant C, Domino SE, Smith YR, Song PXK, Padmanabhan V. 2019. Early pregnancy exposure to endocrine disrupting chemical mixtures are associated with inflammatory changes in maternal and neonatal circulation. Sci Rep. 9(1):5422.
- Kiess W, Häussler G, Vogel M. 2021. Endocrine-disrupting chemicals and child health. Best Pract Res Clin Endocrinol Metab. 35(5):101516.
- Kim B, Colon E, Chawla S, Vandenberg LN, Suvorov A. 2015. Endocrine disruptors alter social behaviors and indirectly influence social hierarchies via changes in body weight. Environ Health. 14:64.
- Kolatorova L, Duskova M, Vitku J, Starka L. 2017. Prenatal exposure to bisphenols and parabens and impacts on human physiology. Physiol Res. 66(3):S305–s315.
- Kolatorova L, Vitku J, Hampl R, Adamcova K, Skodova T, Simkova M, Parizek A, Starka L, Duskova M. 2018. Exposure to bisphenols and parabens during pregnancy and relations to steroid changes. Environ Res. 163:115–122.
- Kolla S, Morcos M, Martin B, Vandenberg LN. 2018. Low dose bisphenol S or ethinyl estradiol exposures during the perinatal period alter female mouse mammary gland development. Reprod Toxicol. 78:50–59.
- LaPlante CD, Catanese MC, Bansal R, Vandenberg LN. 2017. Bisphenol S alters the lactating mammary gland and nursing behaviors in mice exposed during pregnancy and lactation. Endocrinology. 158(10):3448–3461.
- Li J, Sheng N, Cui R, Feng Y, Shao B, Guo X, Zhang H, Dai J. 2016. Gestational and lactational exposure to bisphenol AF in maternal rats increases testosterone levels in 23-day-old male offspring. Chemosphere. 163:552–561.
- Li J, Wu C, Zhao H, Zhou Y, Cao G, Yang Z, Hong Y, Xu S, Xia W, Cai Z. 2019. Exposure assessment of bisphenols in Chinese women during pregnancy: a longitudinal study. Environ Sci Technol. 53(13):7812–7820.
- Liang J, Yang C, Liu T, Tang P, Huang H, Wei H, Liao Q, Long J, Zeng X, Liu S, et al. 2022. Single and mixed effects of prenatal exposure to multiple bisphenols on hemoglobin levels and the risk of anemia in pregnant women. Environ Res. 207:112625.
- Liao C, Kannan K. 2013. Concentrations and profiles of bisphenol A and other bisphenol analogues in foodstuffs from the United States and their implications for human exposure. J Agric Food Chem. 61(19):4655–4662.
- Liao C, Liu F, Guo Y, Moon HB, Nakata H, Wu Q, Kannan K. 2012. Occurrence of eight bisphenol analogues in indoor dust from the United States and several Asian countries: implications for human exposure. Environ Sci Technol. 46(16):9138–9145.
- Liao C, Liu F, Kannan K. 2012. Bisphenol s, a new bisphenol analogue, in paper products and currency bills and its association with bisphenol a residues. Environ Sci Technol. 46(12):6515–6522.
- Liao C, Liu F, Moon HB, Yamashita N, Yun S, Kannan K. 2012. Bisphenol analogues in sediments from industrialized areas in the United States, Japan, and Korea: spatial and temporal distributions. Environ Sci Technol. 46(21):11558–11565.
- Liu J, Li J, Wu Y, Zhao Y, Luo F, Li S, Yang L, Moez EK, Dinu I, Martin JW. 2017. Bisphenol A metabolites and bisphenol S in paired maternal and cord serum. Environ Sci Technol. 51(4):2456–2463.
- Liu J, Shen J, Lu G, Xu X, Yang H, Yan Z, Chen W. 2020. Multilevel ecotoxicity assessment of environmentally relevant bisphenol F concentrations in Daphnia magna. Chemosphere. 240:124917.
- Liu J, Wattar N, Field CJ, Dinu I, Dewey D, Martin JW, APrON Study Team. 2018. Exposure and dietary sources of bisphenol A (BPA) and BPA-alternatives among mothers in the APrON cohort study. Environ Int. 119:319–326.
- Liu Y, Peterson KE. 2015. Maternal exposure to synthetic chemicals and obesity in the offspring: recent findings. Curr Environ Health Rep. 2(4):339–347.
- Lorigo M, Cairrao E. 2022. Fetoplacental vasculature as a model to study human cardiovascular endocrine disruption. Mol Aspects Med. 87:101054.
- LucacciLucacconi L, Trevisani V, Passini E, Righi B, Plessi C, Predieri B, Iughetti L. 2021. Perinatal exposure to phthalates: from endocrine to neurodevelopment effects. Int J Mol Sci. 22(8):4063.
- Machtinger R, Berman T, Adir M, Mansur A, Baccarelli AA, Racowsky C, Calafat AM, Hauser R, Nahum R. 2018. Urinary concentrations of phthalate metabolites, bisphenols and personal care product chemical biomarkers in pregnant women in Israel. Environ Int. 116:319–325.
- MacLennan DH, Kranias EG. 2003. Phospholamban: a crucial regulator of cardiac contractility. Nat Rev Mol Cell Biol. 4(7):566–577.
- Malaisé Y, Lencina C, Cartier C, Olier M, Ménard S, Guzylack-Piriou L. 2020. Perinatal oral exposure to low doses of bisphenol A, S or F impairs immune functions at intestinal and systemic levels in female offspring mice. Environ Health. 19(1):93.
- Malaisé Y, Lencina C, Cartier C, Olier M, Ménard S, Guzylack-Piriou L. 2021. Bisphenol A, S or F mother’s dermal impregnation impairs offspring immune responses in a dose and sex-specific manner in mice. Sci Rep. 11(1):1650.
- Mao J, Jain A, Denslow ND, Nouri M-Z, Chen S, Wang T, Zhu N, Koh J, Sarma SJ, Sumner BW, et al. 2020. Bisphenol A and bisphenol S disruptions of the mouse placenta and potential effects on the placenta-brain axis. Proc Natl Acad Sci USA. 117(9):4642–4652.
- Mariana M, Feiteiro J, Verde I, Cairrao E. 2016. The effects of phthalates in the cardiovascular and reproductive systems: a review. Environ Int. 94:758–776.
- Marie C, Cabut S, Vendittelli F, Sauvant-Rochat MP. 2016. Changes in cosmetics use during pregnancy and risk perception by women. Int J Environ Res Public Health. 13(4):383.
- Melzer D, Rice NE, Lewis C, Henley WE, Galloway TS. 2010. Association of urinary bisphenol a concentration with heart disease: evidence from NHANES 2003/06. PLoS One. 5(1):e8673.
- Meng Z, Tian S, Yan J, Jia M, Yan S, Li R, Zhang R, Zhu W, Zhou Z. 2019. Effects of perinatal exposure to BPA, BPF and BPAF on liver function in male mouse offspring involving in oxidative damage and metabolic disorder. Environ Pollut. 247:935–943.
- Meng Z, Wang D, Liu W, Li R, Yan S, Jia M, Zhang L, Zhou Z, Zhu W. 2019. Perinatal exposure to Bisphenol S (BPS) promotes obesity development by interfering with lipid and glucose metabolism in male mouse offspring. Environ Res. 173:189–198.
- Meng Z, Wang D, Yan S, Li R, Yan J, Teng M, Zhou Z, Zhu W. 2018. Effects of perinatal exposure to BPA and its alternatives (BPS, BPF and BPAF) on hepatic lipid and glucose homeostasis in female mice adolescent offspring. Chemosphere. 212:297–306.
- Meng Z, Zhu W, Wang D, Li R, Jia M, Yan S, Yan J, Zhou Z. 2019. H NMR-based serum metabolomics analysis of the age-related metabolic effects of perinatal exposure to BPA, BPS, BPF, and BPAF in female mice offspring. Environ Sci Pollut Res Int. 26(6):5804–5813.
- Mi P, Tang YQ, Feng XZ. 2020. Acute fluorene-9-bisphenol exposure damages early development and induces cardiotoxicity in zebrafish (Danio rerio). Ecotoxicol Environ Saf. 202:110922.
- Migliaccio S, Bimonte VM, Besharat ZM, Sabato C, Lenzi A, Crescioli C, Ferretti E. 2021. Environmental contaminants acting as endocrine disruptors modulate atherogenic processes: new risk factors for cardiovascular diseases in women? Biomolecules. 12(1):44.
- Moon S, Yu SH, Lee CB, Park YJ, Yoo HJ, Kim DS. 2021. Effects of bisphenol A on cardiovascular disease: an epidemiological study using National Health and Nutrition Examination Survey 2003–2016 and meta-analysis. Sci Total Environ. 763:142941.
- Moreman J, Lee O, Trznadel M, David A, Kudoh T, Tyler CR. 2017. Acute toxicity, teratogenic, and estrogenic effects of bisphenol A and its alternative replacements bisphenol S, bisphenol F, and bisphenol AF in zebrafish embryo-larvae. Environ Sci Technol. 51(21):12796–12805.
- Mu X, Huang Y, Li X, Lei Y, Teng M, Li X, Wang C, Li Y. 2018. Developmental effects and estrogenicity of bisphenol A alternatives in a zebrafish embryo model. Environ Sci Technol. 52(5):3222–3231.
- Mustieles V, Williams PL, Fernandez MF, Mínguez-Alarcón L, Ford JB, Calafat AM, Hauser R, Messerlian C, Environment and Reproductive Health (EARTH) Study Team. 2018. Maternal and paternal preconception exposure to bisphenols and size at birth. Hum Reprod. 33(8):1528–1537.
- O’Shaughnessy KL, Fischer F, Zenclussen AC. 2021. Perinatal exposure to endocrine disrupting chemicals and neurodevelopment: how articles of daily use influence the development of our children. Best Pract Res Clin Endocrinol Metab. 35(5):101568.
- Ohtani N, Iwano H, Suda K, Tsuji E, Tanemura K, Inoue H, Yokota H. 2017. Adverse effects of maternal exposure to bisphenol F on the anxiety- and depression-like behavior of offspring. J Vet Med Sci. 79(2):432–439.
- Padmanabhan V, Moeller J, Puttabyatappa M. 2021. Impact of gestational exposure to endocrine disrupting chemicals on pregnancy and birth outcomes. Adv Pharmacol. 92:279–346.
- Pal S, Sarkar K, Nath PP, Mondal M, Khatun A, Paul G. 2017. Bisphenol S impairs blood functions and induces cardiovascular risks in rats. Toxicol Rep. 4:560–565.
- Pan Y, Deng M, Li J, Du B, Lan S, Liang X, Zeng L. 2020. Occurrence and maternal transfer of multiple bisphenols, including an emerging derivative with unexpectedly high concentrations, in the human maternal-fetal-placental unit. Environ Sci Technol. 54(6):3476–3486.
- Patisaul HB, Fenton SE, Aylor D. 2018. Animal models of endocrine disruption. Best Pract Res Clin Endocrinol Metab. 32(3):283–297.
- Pelch K, Wignall JA, Goldstone AE, Ross PK, Blain RB, Shapiro AJ, Holmgren SD, Hsieh JH, Svoboda D, Auerbach SS, et al. 2019. A scoping review of the health and toxicological activity of bisphenol A (BPA) structural analogues and functional alternatives. Toxicology. 424:152235.
- Philips EM, Jaddoe VWV, Asimakopoulos AG, Kannan K, Steegers EAP, Santos S, Trasande L. 2018. Bisphenol and phthalate concentrations and its determinants among pregnant women in a population-based cohort in the Netherlands, 2004–5. Environ Res. 161:562–572.
- Prudencio TM, Swift LM, Guerrelli D, Cooper B, Reilly M, Ciccarelli N, Sheng J, Jaimes R, Posnack NG. 2021. Bisphenol S and bisphenol F are less disruptive to cardiac electrophysiology, as compared with bisphenol A. Toxicol Sci. 183(1):214–226.
- Pu Y, Gingrich JD, Steibel JP, Veiga-Lopez A. 2017. Sex-specific modulation of fetal adipogenesis by gestational bisphenol A and bisphenol S exposure. Endocrinology. 158(11):3844–3858.
- Qin JY, Ru S, Wang W, Hao L, Wei S, Zhang J, Xiong JQ, Wang J, Zhang X. 2021. Unraveling the mechanism of long-term bisphenol S exposure disrupted ovarian lipids metabolism, oocytes maturation, and offspring development of zebrafish. Chemosphere. 277:130304.
- Qiu W, Chen B, Greer JB, Magnuson JT, Xiong Y, Zhong H, Andrzejczyk NE, Zheng C, Schlenk D. 2020. Transcriptomic responses of bisphenol S predict involvement of immune function in the cardiotoxicity of early life-stage zebrafish (Danio rerio). Environ Sci Technol. 54(5):2869–2877.
- Rager JE, Bangma J, Carberry C, Chao A, Grossman J, Lu K, Manuck TA, Sobus JR, Szilagyi J, Fry RC. 2020. Review of the environmental prenatal exposome and its relationship to maternal and fetal health. Reprod Toxicol. 98:1–12.
- Rahman A, Kumarathasan P, Gomes J. 2016. Infant and mother related outcomes from exposure to metals with endocrine disrupting properties during pregnancy. Sci Total Environ. 569–570:1022–1031.
- Rochester JR, Bolden AL. 2015. Bisphenol S and F: a systematic review and comparison of the hormonal activity of bisphenol A substitutes. Environ Health Perspect. 123(7):643–650.
- Rolfo A, Nuzzo AM, De Amicis R, Moretti L, Bertoli S, Leone A. 2020. Fetal-maternal exposure to endocrine disruptors: correlation with diet intake and pregnancy outcomes. Nutrients. 12(6):1744.
- Rosenmai AK, Dybdahl M, Pedersen M, Alice van Vugt-Lussenburg BM, Wedebye EB, Taxvig C, Vinggaard AM. 2014. Are structural analogues to bisphenol a safe alternatives? Toxicol Sci. 139(1):35–47.
- Shi J, Jiao Z, Zheng S, Li M, Zhang J, Feng Y, Yin J, Shao B. 2015. Long-term effects of bisphenol AF (BPAF) on hormonal balance and genes of hypothalamus-pituitary-gonad axis and liver of zebrafish (Danio rerio), and the impact on offspring. Chemosphere. 128:252–257.
- Shi M, Sekulovski N, MacLean JA, Hayashi K. 2018. Prenatal exposure to bisphenol A analogues on male reproductive functions in mice. Toxicol Sci. 163(2):620–631.
- Shi M, Sekulovski N, MacLean JA, Whorton A, Hayashi K. 2019. Prenatal exposure to bisphenol A analogues on female reproductive functions in mice. Toxicol Sci. 168(2):561–571.
- Shi M, Whorton AE, Sekulovski N, MacLean JA, Hayashi K. 2019a. Prenatal exposure to bisphenol A, E, and S induces transgenerational effects on female reproductive functions in mice. Toxicol Sci. 170(2):320–329.
- Shi M, Whorton AE, Sekulovski N, MacLean JA, Hayashi K. 2019b. Prenatal exposure to bisphenol A, E, and S induces transgenerational effects on male reproductive functions in mice. Toxicol Sci. 172(2):303–315.
- Soler-Botija C, Gálvez-Montón C, Bayés-Genís A. 2019. Epigenetic biomarkers in cardiovascular diseases. Front Genet. 10:950.
- Song M, Liang D, Liang Y, Chen M, Wang F, Wang H, Jiang G. 2014. Assessing developmental toxicity and estrogenic activity of halogenated bisphenol A on zebrafish (Danio rerio). Chemosphere. 112:275–281.
- Song S, Song M, Zeng L, Wang T, Liu R, Ruan T, Jiang G. 2014. Occurrence and profiles of bisphenol analogues in municipal sewage sludge in China. Environ Pollut. 186:14–19.
- Street ME, Bernasconi S. 2020. Endocrine-disrupting chemicals in human fetal growth. Int J Mol Sci. 21(4):1430.
- Tanner EM, Hallerbäck MU, Wikström S, Lindh C, Kiviranta H, Gennings C, Bornehag CG. 2020. Early prenatal exposure to suspected endocrine disruptor mixtures is associated with lower IQ at age seven. Environ Int. 134:105185.
- Tucker DK, Hayes Bouknight S, Brar SS, Kissling GE, Fenton SE. 2018. Evaluation of prenatal exposure to bisphenol analogues on development and long-term health of the mammary gland in female mice. Environ Health Perspect. 126(8):087003.
- Ullah A, Pirzada M, Jahan S, Ullah H, Razak S, Rauf N, Khan MJ, Mahboob SZ. 2019. Prenatal BPA and its analogs BPB, BPF, and BPS exposure and reproductive axis function in the male offspring of Sprague Dawley rats. Hum Exp Toxicol. 38(12):1344–1365.
- Van Camp G. 2014. Cardiovascular disease prevention. Acta Clin Belg. 69(6):407–411.
- Vandenberg LN, Welshons WV, Vom Saal FS, Toutain PL, Myers JP. 2014. Should oral gavage be abandoned in toxicity testing of endocrine disruptors? Environ Health. 13(1):46.
- Varshavsky J, Smith A, Wang A, Hom E, Izano M, Huang H, Padula A, Woodruff TJ. 2020. Heightened susceptibility: a review of how pregnancy and chemical exposures influence maternal health. Reprod Toxicol. 92:14–56.
- Vought V, Wang HS. 2018. Impact of common environmental chemicals bisphenol A and bisphenol S on the physiology of Lumbriculus variegatus. Environ Toxicol Pharmacol. 60:225–229.
- Vuong AM, Braun JM, Sjödin A, Calafat AM, Yolton K, Lanphear BP, Chen A. 2021. Exposure to endocrine disrupting chemicals (EDCs) and cardiometabolic indices during pregnancy: the HOME Study. Environ Int. 156:106747.
- Wan Y, Huo W, Xu S, Zheng T, Zhang B, Li Y, Zhou A, Zhang Y, Hu J, Zhu Y, et al. 2018. Relationship between maternal exposure to bisphenol S and pregnancy duration. Environ Pollut. 238:717–724.
- Wang R, Fei Q, Liu S, Weng X, Liang H, Wu Y, Wen L, Hao G, Cao G, Jing C. 2022. The bisphenol F and bisphenol S and cardiovascular disease: results from NHANES 2013–2016. Environ Sci Eur. 34(1):4.
- Wang W, Zhang X, Qin J, Wei P, Jia Y, Wang J, Ru S. 2019. Long-term bisphenol S exposure induces fat accumulation in liver of adult male zebrafish (Danio rerio) and slows yolk lipid consumption in F1 offspring. Chemosphere. 221:500–510.
- Wei P, Zhao F, Zhang X, Liu W, Jiang G, Wang H, Ru S. 2018. Transgenerational thyroid endocrine disruption induced by bisphenol S affects the early development of zebrafish offspring. Environ Pollut. 243(Pt B):800–808.
- Woods L, Perez-Garcia V, Hemberger M. 2018. Regulation of placental development and its impact on fetal growth-new insights from mouse models. Front Endocrinol (Lausanne). 9:570.
- Wu LH, Zhang XM, Wang F, Gao CJ, Chen D, Palumbo JR, Guo Y, Zeng EY. 2018. Occurrence of bisphenol S in the environment and implications for human exposure: a short review. Sci Total Environ. 615:87–98.
- Yabusaki R, Iwano H, Tsushima S, Koike N, Ohtani N, Tanemura K, Inoue H, Yokota H. 2015. Weak activity of UDP-glucuronosyltransferase toward Bisphenol analogs in mouse perinatal development. J Vet Med Sci. 77(11):1479–1484.
- Yamazaki E, Yamashita N, Taniyasu S, Lam J, Lam PK, Moon HB, Jeong Y, Kannan P, Achyuthan H, Munuswamy N, et al. 2015. Bisphenol A and other bisphenol analogues including BPS and BPF in surface water samples from Japan, China, Korea and India. Ecotoxicol Environ Saf. 122:565–572.
- Yang C, Song G, Lim W. 2020. Effects of endocrine disrupting chemicals in pigs. Environ Pollut. 263(Pt B):114505.
- Yang Q, Yang X, Liu J, Ren W, Chen Y, Shen S. 2017a. Effects of BPF on steroid hormone homeostasis and gene expression in the hypothalamic-pituitary-gonadal axis of zebrafish. Environ Sci Pollut Res Int. 24(26):21311–21322.
- Yang Q, Yang X, Liu J, Ren W, Chen Y, Shen S. 2017b. Exposure to bisphenol B disrupts steroid hormone homeostasis and gene expression in the hypothalamic–pituitary–gonadal axis of zebrafish. Water Air Soil Pollut. 228(3):112.
- Yang S, Cheng W, Li X, Liang F, Zhou R, Wang H, Feng Y, Wang Y. 2020. Use of embryonic stem cell-derived cardiomyocytes to study cardiotoxicity of bisphenol AF via the GPER/CAM/eNOS pathway. Toxicology. 432:152380.
- Yin N, Liang X, Liang S, Liang S, Yang R, Hu B, Cheng Z, Liu S, Dong H, Liu S, et al. 2019. Embryonic stem cell- and transcriptomics-based in vitro analyses reveal that bisphenols A, F and S have similar and very complex potential developmental toxicities. Ecotoxicol Environ Saf. 176:330–338.
- Zhang B, He Y, Zhu H, Huang X, Bai X, Kannan K, Zhang T. 2020. Concentrations of bisphenol A and its alternatives in paired maternal-fetal urine, serum and amniotic fluid from an e-waste dismantling area in China. Environ Int. 136:105407.
- Zhang C, Wu XC, Li S, Dou LJ, Zhou L, Wang FH, Ma K, Huang D, Pan Y, Gu JJ, et al. 2021. Perinatal low-dose bisphenol AF exposure impairs synaptic plasticity and cognitive function of adult offspring in a sex-dependent manner. Sci Total Environ. 788:147918.
- Zhang H, Zhang Y, Li J, Yang M. 2019. Occurrence and exposure assessment of bisphenol analogues in source water and drinking water in China. Sci Total Environ. 655:607–613.
- Zhang W, Xia W, Liu W, Li X, Hu J, Zhang B, Xu S, Zhou Y, Li J, Cai Z, et al. 2019. Exposure to bisphenol a substitutes and gestational diabetes mellitus: a prospective cohort study in China. Front Endocrinol (Lausanne). 10:262.
- Zhang YF, Shan C, Wang Y, Qian LL, Jia DD, Zhang YF, Hao XD, Xu HM. 2020. Cardiovascular toxicity and mechanism of bisphenol A and emerging risk of bisphenol S. Sci Total Environ. 723:137952.
- Zhou R, Xia M, Zhang L, Cheng W, Yan J, Sun Y, Wang Y, Jiang H. 2021. Individual and combined effects of BPA, BPS and BPAF on the cardiomyocyte differentiation of embryonic stem cells. Ecotoxicol Environ Saf. 220:112366.