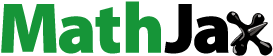
Abstract
Alpha-diketones, notably diacetyl, have been used as flavoring agents. When airborne in occupational settings, exposures to diacetyl have been associated with serious respiratory disease. Other α-diketones, such as 2,3-pentanedione, and analogues such as acetoin (a reduced form of diacetyl), require evaluation, particularly, in light of recently available toxicological studies. The current work reviewed mechanistic, metabolic, and toxicology data available for α-diketones. Data were most available for diacetyl and 2,3-pentanedione, and a comparative assessment of their pulmonary effects was performed, and an occupational exposure limit (OEL) was proposed for 2,3-pentanedione. Previous OELs were reviewed and an updated literature search was performed. Respiratory system histopathology data from 3-month toxicology studies were evaluated with benchmark dose (BMD) modelling of sensitive endpoints. This demonstrated comparable responses at concentrations up to 100 ppm, with no consistent overall pattern of greater sensitivity to either diacetyl or 2,3-pentanedione. In contrast, based on draft raw data, no adverse respiratory effects were observed in comparable 3-month toxicology studies that evaluated exposure to acetoin at up to 800 ppm (highest tested concentration), indicating that acetoin does not present the same inhalation hazard as diacetyl or 2,3-pentanedione. To derive an OEL for 2,3-pentanedione, BMD modelling was conducted for the most sensitive endpoint from 90-day inhalation toxicity studies, namely, hyperplasia of nasal respiratory epithelium. On the basis of this modelling, an 8-hour time-weighted average OEL of 0.07 ppm is proposed to be protective against respiratory effects that may be associated with chronic workplace exposure to 2,3-pentanedione.
1. Introduction
Diacetyl is a flavoring agent with a strong buttery taste and odor. Diacetyl has been widely used in flavoring mixtures, for example, in the production of popcorn. In epidemiological studies, it has been reported that chronic workplace exposure to chemicals that include diacetyl is associated with increased risks for pulmonary obstruction-related symptoms and bronchiolitis obliterans (Kreiss et al. Citation2002; Kanwal et al. Citation2006; van Rooy et al. Citation2007, Citation2009; Lockey et al. Citation2009); however, as epidemiology studies of workplace exposures typically involve concurrent exposures to multiple chemicals, it complicates the assessment of effects attributable to any individual chemical. Therefore, it is unknown the degree to which occupational co-exposures to other chemicals in conjunction with diacetyl may be relevant to the observed effects. A strong relationship was reported by Kreiss et al. (Citation2002) between the higher quartiles of estimated cumulative exposure to diacetyl and the frequency and magnitude of pulmonary obstruction (spirometric parameters including 1-s forced expiry volume [FEV1], forced vital capacity [FVC], and FEV1:FVC, as well as clinical symptoms) relative to those observed in the general population. Affected workers reported symptoms of progressive shortness of breath on exertion, chronic cough without sputum production, and wheezing (Kreiss et al. Citation2002; Harber et al. Citation2006; Kanwal et al. Citation2006; NTP Citation2018). Akpinar-Elci et al. (Citation2004) investigated bronchiolitis obliterans in 9 cases of former microwave popcorn plant workers, 5 of which had exposures to mixed flavorings, for which the onset of symptoms occurred from 5 months to 9 years after starting to work at the plant. In addition to FEV1 which was 14.0 to 66.8% of the predicted value, high-resolution computed tomography (HRCT) scans on 8 of the patients revealed marked bronchial wall thickening and mosaic attenuation with air trapping on expiratory imaging.
2,3-Pentanedione is a 5-carbon alpha-diketone used as a flavor ingredient. It is structurally similar to diacetyl (2,3-butanedione; Chemical Abstracts Service [CAS] No. 431-03-8), a 4-carbon α-diketone. While no epidemiology studies have been identified for 2,3-pentanedione to suggest it may alone be associated with obliterative bronchiolitis, there are some studies where workers were exposed to mixtures of diacetyl and 2,3-pentanedione, including associated with coffee roasting and not only with popcorn (Bailey et al. Citation2015; Harvey et al. Citation2020, Citation2021; LeBouf et al. Citation2020; Virji et al. Citation2021). Furthermore, given the similarity between the two structures, a toxicological evaluation of the potential inhalation effects of these substances was undertaken.
The chemical structures of 2,3-pentanedione and diacetyl are shown in . Occupational exposure limits for 2,3 pentanedione and diacetyl have been established by various regulatory and authoritative bodies; however, the existing limits are based on different endpoints and are variable. Eight-hour time-weighted average (TWA) occupational exposure limits (OELs) for diacetyl range from 0.005 ppm (NIOSH Citation2016) to 0.02 ppm (EU Citation2017; DFG Citation2020) while TWA OELs for 2,3-pentanedione range from 0.0093 ppm (NIOSH Citation2016) to 0.02 ppm (based on diacetyl) (DFG Citation2020). It is noted that the NIOSH (Citation2016) OEL predated toxicology studies conducted by the National Toxicology Program (NTP) on 2,3-pentanedione, the raw data from which is now available. Thus, a benchmark dose (BMD) analysis to derive an OEL that is specifically based on toxicological data for 2,3-pentanedione was undertaken as part of this research.
2. Materials and methods
2.1. Literature searches and toxicological assessment
Searches of electronic scientific literature databases including Medline, Toxfile, the National Technical Information Service (NTIS), and Embase were conducted in March 2022 to identify peer-reviewed journal articles relevant to the pulmonary effects of α-diketones. The search terms were as follows: (2,3-pentanedione OR acetyl propionyl OR acetylpropionyl OR 600-14-6 OR alpha-diketones OR α-diketones OR alpha-dicarbonyl OR α-dicarbonyl OR flavor ingredient OR flavor ingredient) AND (lung* OR airway* OR pulmonary OR respiratory OR bronch* OR breath* OR nasal OR occupation* OR exposure limit). As a first step, the titles that were identified were reviewed for potential relevance to pulmonary effects induced by 2,3-pentanedione, with particular focus on identifying articles that contain comparative information on 2,3-pentanedione, diacetyl, and other α-diketones. At the title review stage, a conservative (i.e. inclusive) approach was taken such that a title was flagged if it appeared to represent an article which may be relevant.
Abstracts were retrieved and reviewed in detail for relevance to the project, and all relevant articles were retrieved for evaluation. A total of 390 titles were identified, of which 43 abstracts were considered likely to yield relevant information, and of these, 19 articles were retrieved and reviewed for the purpose of this publication.
In addition to information identified in the published literature for the assessment of pulmonary effects, searches of government and authoritative agency websites including the NTP were performed to identify relevant research. The toxicological data were critically evaluated to deduce potential mechanisms of pulmonary toxicity and potency differences. The specific toxicological findings at the cellular level were evaluated from the published reports and peer-reviewed journals to the fullest extent possible based on the information that was reported.
Searches also were conducted of various occupational safety resources to identify information on OELs that have previously been established for 2,3-pentanedione, diacetyl, and acetoin (EU Citation2017; DFG Citation2020; HSE 2020; Ontario Ministry of Labour Citation2020; The Japan Society for Occupational Health Citation2020; ACGIH Citation2021; DGUV IFA Citation2021; OARS/TERA Citation2021; OSHA Citation2021).
2.2. Benchmark dose modeling
To assess and compare the sensitivities of mice and rats to diacetyl and 2,3-pentanedione, respiratory system histopathology data from the NTP 3-month inhalation toxicity studies in both species were reviewed with a focus on identifying endpoints for which responses to diacetyl or 2,3-pentanedione were observed beginning at or before the mid-concentration of 25 ppm and for which an adequate concentration-response relationship exists (i.e. continuing in a concentration-dependent pattern) in one or both sexes of either species. In this regard, effects that were observed only beginning at the 2 higher concentrations of 50 and 100 ppm were not considered. Endpoints fulfilling these criteria were considered the most sensitive endpoints and therefore suitable as points of departure (estimates of the dose boundaries for the onset of the adverse effects) which were calculated using BMD modeling. The United States Environmental Protection Agency (U.S. EPA) benchmark dose software (BMDS) program (version 3.2) was employed for BMD modeling in accordance with the World Health Organization (WHO) guidance for dose-response assessment (WHO Citation2020) and the U.S. EPA BMD Technical Guidance (U.S. EPA Citation2012). The quantal histopathology data were modeled using maximum likelihood estimates (i.e. frequentist methodologies) for restricted dichotomous hill, gamma, log-logistic, linear multistage, and Weibull models as well as unrestricted logistic, log-probit, and probit models (the default suite of models for quantal data in U.S. EPA BMDS). BMD values were calculated for each of the models using a benchmark response of 10% extra risk (the dose that would be associated with a 10% increase in response) with estimated background incidence (rather than zero background risk). Models were assessed for model fit using a goodness-of-fit p-value cutoff of 0.1, and the Akaike’s Information Criterion (AIC) was used to compare individual models that adequately fit the data. For each endpoint, the model with the lowest AIC was selected as the optimal model and the corresponding BMDL10 value (the 95% lower boundary estimate of the BMD) was used as input to derive candidate OELs.
3. Results and discussion
3.1. Hazard assessment
3.1.1. Metabolic considerations
As summarized by Anders (Citation2017), reduction of α-diketones to less electrophilic acyloins and keto alcohols and diols (which are less reactive and readily eliminated) represents a detoxification pathway, and in this regard the biotransformation of diacetyl and related α-diketones including 2,3-pentanedione is similar. Both diacetyl and 2,3-pentanedione may undergo hydration in aqueous environments and exist as a mixture of the α-diketone and a monohydrate (Anders Citation2017). In addition, diacetyl, 2,3-pentanedione, and 2,3-hexanedione (a 6-carbon α-diketone) are all substrates for enzymes in the short-chain dehydrogenase/reductase (SDR) and aldo-keto reductase (AKR) superfamilies, and some of these enzymes are expressed in mice, rats, and humans. The enzymes that are common across these species are L-xylulose reductase and aldose reductase, while another enzyme (dehydrogenase/reductase SDR family member 11) that metabolizes diacetyl, 2,3-pentanedione, and 2,3-hexanedione is expressed in mice and humans (Anders Citation2017). Diacetyl appears to be a substrate for some additional AKR enzymes, although it is unclear whether or not these additional AKR enzymes demonstrate activity on 2,3-pentanedione or have not been evaluated for such activity. Thus, detoxification of diacetyl, 2,3-pentanedione, and likely other α-diketones, is similar to each other and may be expected to be similar across species.
3.1.2. Considerations for reactions with nucleophilic cellular targets
α-Diketones such as diacetyl and 2,3-pentanedione are electrophiles that react non-enzymatically with nucleophilic groups that are present in cellular macromolecules. Based on computation of hardness, softness, electrophilic, and nucleophilic parameters, Anders (Citation2017) calculated that diacetyl, 2,3-pentanedione, and 2,3-hexanedione have the same nucleophilic indices for reaction with model cellular nucleophiles including Nα-acetylarginine, Nα-acetyllysine, and guanine. Moreover, as summarized by Anders (Citation2017) and Hubbs et al. (Citation2019), the selectivity of diacetyl and other α-diketones for arginine is well established, and mass spectrometry analysis has demonstrated that similar products are formed from the reaction of Nα-acetyl-L-arginine with diacetyl, 2,3-pentanedione, and other α-diketones. Collectively, this information suggests that downstream effects resulting from the interaction of diacetyl with arginine also may be expected due to interaction of 2,3-pentanedione or other α-diketones that similarly react with arginine.
In this regard, in vitro observations made by Epperly and Dekker (Citation1989) support that 2,3-pentanedione was as reactive as diacetyl, if not more so, toward arginine groups as demonstrated by complete inhibition of the activity of Escherichia coli L-threonine dehydrogenase (an enzyme with a catalytically essential arginine residue) after 20 min of incubation with 20 mM 2,3-pentanedione, whereas the same inhibition by 20 mM diacetyl required 30 min of incubation. More recently, Morgan et al. (Citation2016) examined the in vitro reactivity of diacetyl, 2,3-pentanedione, and 2,3-hexanedione against equine liver glutathione-S-transferase (GST), another enzyme with an active site arginine. All 3 substances decreased the enzymatic activity of GST when tested at an apparent concentration of 10 mM (this concentration was not stated; it is assumed given that the enzyme solution was prepared and “incubated with equal volumes of the diketone solutions” which were prepared as 20 mM stock solutions). Based on graphical data, diacetyl and 2,3-pentanedione decreased enzyme activity in a similar manner, to approximately 50% of the initial level by 30 min and to less than 25% of the initial level by 90 min. Inhibition by 2,3-hexanedione was slower, with approximately 50% inhibition occurring at 90 min. Notably, the authors stated that 2,3-heptanedione (a 7-carbon α-diketone) was even less reactive although supporting data were not presented. The authors postulated that the longer α-diketones may be less reactive due to increased stabilization of the electrophilic carbonyl groups by the increased carbon length chain, or due to restriction of access to the active site arginine residues as a result of steric hindrance by the increased carbon length chain.
The cumulative information on reactivity suggests that 2,3-pentanedione and diacetyl are comparable in terms of their interactions with arginine residues in proteins.
3.1.3. In vitro toxicology considerations
Several studies have evaluated and compared the in vitro toxicity profiles of diacetyl and 2,3-pentanedione in various experimental systems (Fedan et al. Citation2011; Beasley et al. Citation2013; Zaccone et al. Citation2013, Citation2015; Gerloff et al. Citation2017; Park et al. Citation2019; Rim and Kim Citation2020). The following summarizes the findings from these studies.
3.1.3.1. Cell culture models
In a published conference abstract, Beasley et al. (Citation2013) demonstrated similar inflammatory responses of NCI-H292 human airway epithelial cells following incubation for 30 min with diacetyl or 2,3-pentanedione at concentrations ranging from 5 to 20 mM. Specifically, levels of the growth factor amphiregulin (AREG) and the inflammatory cytokine interleukin-8 (IL-8) produced by NCI-H292 cells were increased by comparable amounts 6 and 24 h following exposure to diacetyl or 2,3-pentanedione at the same concentrations.
Due to the involvement of sodium absorption and chloride secretion in regulating airway surface liquid and mucociliary clearance, Zaccone et al. (Citation2015) evaluated the effects on cell ion transport following exposure of cultured human bronchial/epithelial cells (NHBE cells) to diacetyl or 2,3-pentanedione. Cells were exposed for 6 h to vapors of diacetyl or 2,3-pentanedione at a concentration of 25 ppm followed by measurement of epithelial ion transport and cell morphology at 0 and 18 h post-exposure. No effects were observed on cell morphology, apical chloride conductance, or sodium-potassium pump activity with either substance, however, both substances caused an inhibition of amiloride-sensitive sodium transport immediately after exposure, which recovered to control levels at 18 h post-exposure. The authors concluded that the results allow the discrimination of functional changes from structural changes due to exposure to these substances, and that reductions in sodium transport may be a component of lung disease triggered by the flavorings.
The effects of various flavoring substances on viability and IL-8 release were evaluated by Gerloff et al. (Citation2017) in human bronchial epithelial cells (Beas2B cells), human mucoepidermoid carcinoma epithelial cells (H292 cells), and human lung fibroblasts (HFL-1 cells). No effects on cell viability were observed following incubation for 24 h with diacetyl, 2,3-pentanedione (reported only as “pentanedione” but assumed to be 2,3-pendanetione), or acetoin at concentrations ranging from 10 µM to 1 mM. Levels of IL-8 were not altered by any of the substances in H292 cells but were increased in a concentration-dependent manner in Beas2B cells by diacetyl and acetoin, and in HFL-1 cells by 2,3-pentanedione and acetoin.
Two more recent studies evaluated gene expression changes in mouse and human lung cells exposed in vitro to diacetyl or 2,3-pentanedione. The study by Rim and Kim (Citation2020) is difficult to interpret and is considered of limited utility because exposure times were not reported and results were not clearly explained. Nonetheless, the authors reported similar gene clustering following exposure of MLg mouse lung fibroblasts to each substance at concentrations of 150 or 250 µg/mL and provided a list of 7 target genes for which expression was altered by both substances. Specifically, the authors stated that “Fosl1, Rb1, Aspn, Dusp1, Rnf19b, Jun, and Hbegf were over-regulated by targeting down-regulated miRNA mutated by two chemicals.” Further interpretation was not provided and these data are considered of limited value.
Park et al. (Citation2019) cultured primary NHBE cells for 14 days during which time they differentiated into a mixture of ciliated cells, goblet cells, and some remaining basal cells, which closely mimics human airway epithelium. The authors then evaluated gene expression changes following exposure of the cells to aqueous solutions of diacetyl (25 ppm) or 2,3-pentanedione (100 ppm) for 24 h. A total of 163 and 568 differentially regulated genes were observed after exposure to diacetyl and 2,3-pentanedione, respectively, with 142 of these genes common to both substances. The authors reported that multiple genes involved in cilia biogenesis were significantly down-regulated by both substances, consistent with immunostaining results that showed the number of ciliated cells was decreased following the exposures whereas the number of goblet cells was not. Overall, the authors concluded that similar transcriptional changes were observed in these cells following exposure to diacetyl or 2,3-pentanedione.
3.1.3.2. Isolated airway models
In a published conference abstract, Fedan et al. (Citation2011) evaluated the effects of diacetyl and 2,3-pentanedione on isolated guinea pig tracheas. Diacetyl applied to the luminal (mucosal) surface at concentrations of 1 to 3 mM caused contractions at concentrations of 10 to 30 mM which were followed by relaxation. The same pattern of responses was observed for 2,3-pentanedione when applied at the same concentration ranges, leading the authors to conclude that diacetyl and 2,3-pentanedione were nearly identical in their activities in this in vitro model and that their direct effects on airway smooth muscle in workers may be comparable.
A later study by Zaccone et al. (Citation2013) investigated in vitro responses of isolated rat tracheal preparations to diacetyl and 2,3-pentanedione at concentrations ranging from 1 to 30 mM and reported similar findings to those described above for guinea pig tracheas (Fedan et al. Citation2011). The authors suggested that responses to diacetyl and 2,3-pentanedione involve a direct effect in airway smooth muscle and are independent of and not mediated by the epithelium.
3.1.3.3. In vitro summary
The cumulative information from in vitro studies in various lung cell types and isolated airway models suggest that 2,3-pentanedione and diacetyl have comparable profiles and potencies in terms of their effects on gene expression changes, growth factor and cytokine production, ion transport, cytotoxicity, and airway contraction/relaxation.
3.1.4. In vivo toxicology considerations
Obliterative bronchiolitis is caused by fibrosis of the bronchioles leading to reduced lumen size and obstructed airflow (Hubbs et al. Citation2019). Similar lesions have been observed in mice and rats exposed to diacetyl, albeit not always in the bronchioles. From a pathogenesis perspective, the mechanism of airway damage from diacetyl and related compounds is direct injury to airway epithelium that, when repeated and/or severe, can result in aberrant repair with airway fibrosis (Hubbs et al. Citation2019). As summarized by Hubbs et al. (Citation2019), species differences in sites of diacetyl toxicity within the respiratory tract can be explained by dosimetry. Rats (and mice) breathe only through their nose which may scrub much of the inhaled diacetyl. In contrast, humans often breathe through their mouth and usually switch to at least some mouth breathing while exercising which eliminates nasal scrubbing of the airstream. Thus, the predicted dose of diacetyl in human bronchioles is greatly increased relative to that of rat bronchioles, and this is accentuated during exercise. For example, Cichocki and Morris (Citation2017) calculated that a lightly exercising worker breathing 12 ppm diacetyl is estimated to receive a bronchiolar exposure comparable to that of a rat breathing 200 ppm diacetyl, which is consistent with the estimate by Gloede et al. (Citation2011) that bronchiolar tissue concentrations in a mouth-breathing, lightly exercising human exposed to 1 ppm diacetyl are likely to exceed those in a nose-breathing rat by 20- to 40-fold. Moreover, Gloede et al. (Citation2011) estimated that the tissue concentration in the bronchiolar epithelium of a mouth-breathing, lightly exercising human is comparable to that in the main stem bronchi of a nose-breathing rat. Thus, interspecies differences in sites of damage to airway epithelium are largely explainable by interspecies differences in the site of dose delivery. While the preceding discussion focused on diacetyl, there is no reason to assume the same would not also apply to 2,3-pentandione.
The in vivo pulmonary effects of 2,3-pentanedione have been evaluated in numerous studies, several of which have directly compared the effects of diacetyl and 2,3-pentanedione (Kelly et al. Citation2010; Hubbs et al. Citation2012; Morgan et al. Citation2012, Citation2015, Citation2016; Zaccone et al. Citation2013; Flake and Morgan Citation2017). In addition, diacetyl and 2,3-pentanedione have both been evaluated in mouse and rat inhalation toxicity studies conducted by the NTP; specifically, both substances have been evaluated in 3 month studies while diacetyl also has been evaluated in 2-year toxicity and carcinogenicity studies. Notably, in the review article by Brass and Palmer (Citation2017), the authors consider mice to be a less-than-ideal model of occupational diacetyl exposure because, although they develop fibrotic like changes, they do not develop obliterative bronchiolitis-like lesions, whereas rats do develop such lesions (see page 8 of the article, and page 10 Discussion). Nonetheless, information from studies in mice and rats is summarized here for the sake of completeness.
3.1.4.1. Single-dose toxicity
Kelly et al. (Citation2010) administered a single intratracheal dose of 2,3-pentanedione to male Sprague-Dawley rats at a dose level of 140 mg/kg body weight and 7 days later observed intraluminal polypoid and concentric fibrotic lesions characteristic of bronchiolitis obliterans in humans. A pattern of early injury to Clara cells was reported and corresponded with significant increases in cell proliferation and pro-mitotic transcripts 1, 3, and 7 days post-dosing. The same research group subsequently observed similar results when diacetyl was administered to male Sprague–Dawley rats at a dose level of 125 mg/kg body weight (Palmer et al. Citation2011). Thus, although the dose levels and method of administration are of uncertain relevance to occupational exposure scenarios, the results of these studies demonstrated that a single intratracheal exposure to 2,3-pentanedione or diacetyl in rats can cause pulmonary injury with features reminiscent of those observed in human bronchiolitis obliterans. A scientific meeting abstract was identified that reported on the results of an intratracheal instillation study in rats of diacetyl, acetoin, and acetyl propionyl (Flake et al. Citation2010). Based on the limited description provided in the abstract, groups of male Sprague-Dawley rats received a single intratracheal instillation of diacetyl (125 mg/kg), acetoin (125 mg/kg), acetyl propionyl (140 mg/kg) or the sterile water vehicle. Bronchoalveolar lavage fluid was obtained on Days 1, 3, or 7 following treatment, and the lungs from some rats from each group were examined microscopically on Days 1, 3, 7, and 14 following treatment. The authors concluded that the findings indicated a general progression of histological changes consistent with bronchiolitis obliterans for all 3 substances, including inflammation, necrosis and denudation of the epithelium (Day 1); epithelial regeneration, hyperplasia, early type endobronchial polyps (Day 3); endobronchial polypoid and intramural fibrosis (Day 7); and regeneration of bronchial/bronchiolar epithelium and, for some animals, decreased incidences of endobronchial fibrosis. As the results are from a study that would have been completed in 2010 or earlier, a literature search was conducted to identify a peer-reviewed publication of the full study results. A detailed publication of the study by the same authors was identified; however, only the results for diacetyl were included (Palmer et al. Citation2011). The findings presented for diacetyl were in keeping with the information included in the brief abstract, however, no results could be confirmed for acetoin.
Hubbs et al. (Citation2012) evaluated respiratory toxicity in male Sprague-Dawley rats exposed by whole-body inhalation to vapors of 2,3-pentanedione (target concentrations of 120, 240, 320, or 360 ppm) or diacetyl (240 ppm) for 6 h. Histopathological evidence of necrotizing rhinitis, tracheitis, and bronchitis was observed in rats exposed to 2,3-pentanedione or diacetyl; the severity of the effects was comparable for 2,3-pentanedione and diacetyl at the common concentration of 240 ppm, and increased at higher concentrations of 2,3-pentanedione. The histopathological findings of injury were most apparent in the airway respiratory and transitional epithelium, supportive of comparable respiratory epithelial cytotoxicity for both substances.
Functional effects of single exposures to 2,3-pentanedione and diacetyl also have been investigated, along with evaluation of functional effects of acetoin. Zaccone et al. (Citation2013) observed no biologically significant effects on basal lung resistance or dynamic compliance in male Sprague-Dawley rats 18 h after a 6-h whole-body inhalation exposure to 2,3-pentanedione or diacetyl (each evaluated at up to 360 ppm) or a 6-h whole-body inhalation exposure to acetoin (150 ppm). Moreover, airway reactivity to inhaled methacholine was not increased but rather was decreased by 2,3-pentanedione (at 120, 240, and 320 ppm) and diacetyl (at 360 ppm), suggesting the former has a more prominent albeit unexpected dampening effect on airway reactivity. No effects of acetoin (150 ppm) were observed on reactivity to inhaled methacholine.
3.1.4.2. Repeat-dose toxicity
Several studies by Morgan and colleagues have characterized the pulmonary effects of repeated inhalation exposure to 2,3-pentanedione in mice and rats, including one study that evaluated the comparative effects of 2,3-pentanedione, diacetyl, and 2,3-hexanedione in rats (Morgan et al. Citation2012, Citation2015, Citation2016). Brief summaries of these studies are provided here.
In the Morgan et al. (Citation2012) study, B6C3F1 mice and Wistar–Han rats of both sexes were exposed by whole-body inhalation to vapors of 2,3-pentanedione at concentrations of 0 (control), 50, 100, or 200 ppm for 6 h/day, 5 days/week for a total of 12 days of exposure. Histopathological changes were observed in the nose (at all concentrations in both species), larynx (primarily at 100 and 200 ppm in mice and 200 ppm in rats), and lung (primarily at 100 and 200 ppm in rats and all concentrations in mice). Notably, the epithelium lining the respiratory tract was the site of toxicity in all high-concentration mice and rats, and high-concentration rats (but not mice) developed fibrotic lesions in the bronchi and bronchioles that the authors considered similar to those observed in human bronchiolitis obliterans. The same authors subsequently evaluated gene expression changes in the bronchi of male rats exposed by whole-body inhalation to 200 ppm 2,3-pentanedione on the same schedule and reported predominant down-regulation of many inflammatory cytokine and chemokine genes in non-fibrotic bronchi and up-regulation in fibrotic bronchi (Morgan et al. Citation2015).
In the Morgan et al. (Citation2016) study, male Wistar–Han rats were exposed by whole-body inhalation to diacetyl, 2,3-pentanedione, or 2,3-hexanedione for 6 h/day, 5 days/week for a total of 12 days of exposure. Each substance was evaluated at concentrations of 0 (control), 100, 150, and 200 ppm. Half of the animals in each group were evaluated 1 day after the last exposure and the other half were evaluated after a 2-week recovery period. Slightly more severe lesions were observed in the upper respiratory tract (nose, larynx, and trachea) in animals exposed to diacetyl than in those exposed to 2,3-pentanedione, a finding attributed by the authors as potentially reflective of its higher water solubility (200 mg/mL for diacetyl versus 60 mg/mL for 2,3-pentanedione) and resulting ability to more easily penetrate the aqueous fluids that protect the epithelium in the upper respiratory tract. Upper respiratory tract lesions were less frequent and less severe in the 2,3-hexanedione group. Bronchial fibrosis was observed in animals exposed to diacetyl or 2,3-pentanedione at ≥150 ppm. The severity of the fibrosis at 200 ppm was greater in the 2,3-pentanedione group after the 12 days of exposure, and this continued through to the end of the recovery period where the average fibrosis severity grade remained at the highest value (4 out of 4) for 2,3-pentanedione and increased from 1.6 to 2.2 for diacetyl. No fibrosis and little bronchial pathology were observed with 2,3-hexanedione at any test concentration. Notably, decreased airway compliance was observed in subsets of animals exposed to 150 ppm diacetyl or 2,3-pentanedione that underwent invasive lung function assessment after the 2-week recovery period; this finding coincided with the observed airway fibrosis in both groups, and no such fibrosis or change in airway compliance were observed in the 2,3-hexanedione group. The authors concluded that the chemical reactivity and respiratory toxicity of diacetyl and 2,3-pentanedione were greater than those of 2,3-hexanedione. Moreover, they speculated that the slightly higher toxicity of diacetyl than 2,3-pentanedione in the upper respiratory tract and the apparent reverse scenario in the lung could relate to the higher water solubility of diacetyl, resulting in greater absorption of diacetyl than 2,3-pentanedione in the upper respiratory tract and decreasing the concentration of diacetyl that reached the lung.
Studies conducted by the NTP have evaluated respiratory toxicity in B6C3F1 mice and Wistar–Han rats of both sexes following whole-body inhalation exposure to diacetyl or 2,3-pentanedione for 3 months (6 h/day, 5 days/week for 14 weeks). The same concentrations of both test articles were evaluated in both species, namely 0 (control), 6.25, 12.5, 25, 50, and 100 ppm. The NTP also has evaluated respiratory toxicity in B6C3F1 mice and Wistar-Han rats of both sexes following 3 months of whole-body inhalation exposure to acetoin, although higher concentrations of the test article were utilized (0, 50, 100, 200, 400, and 800 ppm). The results of the 3-month studies with diacetyl are summarized in NTP Technical Report 593 (NTP Citation2018), which also summarizes the results of 2-year inhalation toxicity/carcinogenicity studies in both species. As of 1 June 2022, the results of the 3-month studies with 2,3-pentanedione and acetoin are not available in published form; rather, only raw data tables are available on the NTP website (https://tools.niehs.nih.gov/cebs3/views/?action=main.dataReview&bin_id=3168; last accessed 1 June 2022).
The results of the acetoin studies are not published, but a summary statement in NTP Technical Report 593 (NTP Citation2018) indicates that there were no exposure-related effects on survival, body weights, organ weights, clinical pathology, or histopathology in mice or rats exposed to up to 800 ppm acetoin for 3 months. Thus, based on both this statement and the draft raw data, 800 ppm could be considered a no-observed-adverse-effect concentration (NOAEC) for acetoin in these studies. In contrast, several respiratory effects were observed in the diacetyl and 2,3-pentanedione 3 month studies and are summarized below.
To allow for direct comparison between the effects of diacetyl and 2,3-pentandione in the NTP studies in mice and rats, the respiratory system histopathology data from the 3-month studies with diacetyl that are provided in NTP Technical Report 593 (NTP Citation2018) were summarized alongside the corresponding raw data for the same endpoints that were reported for the 3-month studies with 2,3-pentanedione. For the diacetyl studies, a total of 28 respiratory system histopathology endpoints were reported in the 3-month mouse study while 31 endpoints were reported in the 3-month rat study. The comparative data for diacetyl and 2,3-pentanedione are provided in Supplemental Tables 1 and 2 for mice and rats, respectively. From these data, the histopathological endpoints selected for BMD modeling are described below in and , respectively.
Table 1. Mouse respiratory system histopathology in NTP 3-month studies.
Table 2. Rat respiratory system histopathology in NTP 3-month studies.
3.2. Benchmark dose modeling
Upon review of the histopathology data for diacetyl published by NTP (Citation2018) and of the draft raw histopathology data for 2,3-pentanedione (available on the NTP website), 10 histopathology endpoints for mice and 5 histopathology endpoints for rats were identified for which a response to the test article (either diacetyl or 2,3-pentanedione) was observed at or before the mid-concentration (25 ppm) and an adequate concentration-response pattern was observed. The overall incidence for these histopathological endpoints for each dose group are summarized in and for mice and rats, respectively, and BMD modeling for these data was conducted. The BMDL10 values for the histopathology data for diacetyl and 2,3-pentanedione are summarized in and for mice and rats, respectively, for comparison. Among the 10 histopathology endpoints for mice that were subjected to BMD modeling (), the BMDL10 in both sexes was lower (more sensitive) for diacetyl for 5 endpoints and was lower for 2,3-pentanedione for 3 endpoints. One endpoint (“larynx, squamous epithelium, hyperplasia”) had a lower BMDL10 for diacetyl in male mice but essentially equivalent BMDL10 values for diacetyl and 2,3-pentanedione in female mice (11.78 and 10.69 ppm, respectively). For the tenth endpoint (“lung, bronchus, epithelium, regeneration”), the BMDL10 was lower for 2,3-pentanedione in male mice but lower for diacetyl in female mice. Based on the BMDL10 calculations for mice (), it appears as though the nose and lung were generally more sensitive to 2,3-pentanedione whereas the larynx and trachea were generally more sensitive to diacetyl.
Table 3. Benchmark dose modelling for mouse respiratory system histopathology in NTP 3-month studies.
Table 4. Benchmark dose modeling for rat respiratory system histopathology in NTP 3-month studies.
Among the 5 histopathology endpoints for rats that were subjected to BMD modeling (), the BMDL10 in both sexes was lower (more sensitive) for diacetyl for 1 endpoint and lower for 2,3-pentanedione for 3 endpoints. For the fifth endpoint (“nose, olfactory epithelium, metaplasia, respiratory”), the BMDL10 was lower for diacetyl in male rats but lower for 2,3-pentanedione in female rats. Based on the BMDL10 calculations for rats (), it appears as though the nose and larynx were generally more sensitive to 2,3-pentanedione than to diacetyl; no BMD modeling was performed for endpoints in the trachea or lung because there were no endpoints for which responses to diacetyl or 2,3-pentanedione were observed beginning at or before the mid-concentration of 25 ppm and continuing in a concentration-dependent pattern.
Histopathology effects in the nose were expected in both species given it is the site of first contact for obligate nose-breathing rodents. Notably, while these effects might be expected to be more sensitive to diacetyl due to its higher water solubility and resulting ability to more easily penetrate the aqueous fluids that protect the epithelium in the upper respiratory tract, they were arguably at least as sensitive, if not more so, to 2,3-pentanedione. Indeed, while nasal respiratory epithelium necrosis in mice was observed more frequently with diacetyl (with correspondingly lower BMDL10 values in males and females), other endpoints that represent downstream events in the epithelial injury and repair continuum were observed more frequently with 2,3-pentanedione (respiratory epithelium regeneration and squamous metaplasia) with correspondingly lower calculated BMDL10 values. Thus, it is possible that 2,3-pentanedione caused earlier and more extensive nasal injury than was observed with diacetyl, with the reparative process correspondingly further along in 2,3-pentanedione-treated groups at the time of tissue evaluation.
Collectively, the respiratory system histopathology data and the results of the BMD modeling that was performed for the most sensitive endpoints in both species demonstrate no consistent overall pattern of greater sensitivity to diacetyl or 2,3-pentanedione. As an example, the only histopathology endpoint that was subjected to BMD modeling for both species (“nose, respiratory epithelium, metaplasia, squamous”) was found to be more sensitive to 2,3-pentanedione than to diacetyl in mice (lower BMDL10 values in males and females) while the opposite was observed in rats.
3.3. OEL for 2,3-pentanedione derivation based on toxicology data
A candidate OEL for 2,3-pentanedione was derived based on the results of the 90-day inhalation toxicity studies conducted in mice and rats. The lowest BMDL10 value among those presented in (for the 90-day mouse study) and (for the 90-day rat study) was used as the point of departure for calculation of the toxicology-based OEL. In both species, the lowest BMDL10 was for effects in the nose, which is perhaps not surprising as it was the site of first contact and therefore the site at which exposure to the highest concentration of 2,3-pentanedione occurred in both species. A BMDL10 of 2.51 ppm was calculated for regeneration of nasal respiratory epithelium in male mice and a BMDL10 of 1.42 ppm was calculated for hyperplasia of nasal respiratory epithelium in male rats.
The lower of these values, 1.42 ppm, was used as the point of departure for the OEL calculation as it provides for the lowest OEL (notably for both 2,3-pentanedione and diacetyl) and therefore offers the best possibility of being protective against adverse effects of 2,3-pentanedione that might occur in all regions of the respiratory system. Although there is no consensus on the methodology that should be used for toxicology-derived OELs, the generally accepted approach is to adjust the point of departure to account for potential exposure differences in the toxicology species and humans (route of administration, duration, etc.) and apply uncertainty factors to account for species extrapolation and other considerations (Dankovic et al. Citation2015).
The point of departure of 1.42 ppm was first adjusted by a factor of 6/8 to account for the 6 h/day exposure scenario in the 90-day toxicology study versus the typical 8 h/day that is assumed for workplace exposure. Further adjustment related to the exposure scenarios was not required since the 90-day study utilized a dosing schedule of 5 days/week which is reflective of the typical work week.
The following 5 adjustment factors were then applied:
UFA (animal-to-human uncertainty factor): A factor of 1 to account for animal-to-human extrapolation. This is considered appropriate given that the point of departure is derived from inhalation exposure data and, as such, allometric scaling across species is not required for effects that occur in the nose via direct contact. Rodent nasal tissue is not known to be underpredictive of such effects, and available rodent and human data for alpha-diketones do not indicate that humans are more sensitive. Moreover, Flake and Morgan (Citation2017) note that lesions produced by diacetyl and 2,3-pentanedione in the larger airways of rodents serve as an indication of the end stage obliterative lesions that might occur in human obliterative bronchiolitis.
UFH (interindividual variability factor): A factor of 5 to account for interindividual variability among workers. This is considered adequate versus the typical factor of 10 that is used to account for variability in the general population given that workers are expected to be less heterogeneous than the general population (because they do not include the very old or the very young) and they are not exposed for an entire lifetime (Dankovic et al. Citation2015), resulting in less opportunity for variability to develop among individuals due to age-related inhomogeneity in toxicodynamics. Moreover, as indicated by Dankovic et al. (Citation2015), a factor of 5 is consistent with the default uncertainty factor used by the European Chemicals Agency (ECHA) for establishing derived no-effect levels in workers, and is more conservative than the factor of 3 that is used by other agencies including the European Center for Ecotoxicology and Toxicology of Chemicals (ECETOC) and Dutch National Institute of Public Health and the Environment (in cooperation with TNO Nutrition and Food Research) (TNO/RIVM).
UFL (lowest-observed-adverse-effect level [LOAEL]-to-no-observed-adverse-effect level [NOAEL] factor): A factor of 1 to account for a BMDL10 as the point of departure, which is essentially equivalent to a NOAEL for standard study designs (Dankovic et al. Citation2015).
UFS (shorter-term-to-longer-term factor): A factor of 3 to account for the point of departure being based on data from a 90-day (3-month) rat study whereas exposure in workers could be for 40 or more years. As summarized by Dankovic et al. (Citation2015), reviews of many high quality toxicology studies support that a factor of 3 typically appears sufficient to account for the possibility that a lower point of departure might have resulted if longer-term studies were conducted. This is supported by the lack of fibrosis in rats exposed to diacetyl for 2 years at a concentration of 12.5 ppm (NTP Citation2018) and the structure-activity relationship between diacetyl and 2,3-pentanedione that would suggest similar findings for the latter if tested in a similar study.
UFD (database inadequacy factor): A factor of 1 to account for the robust database that is available for 2,3-pentanedione.
Based on the preceding, the toxicology-based OEL was derived as follows:
Therefore, an OEL of 0.071 ppm (rounded to 0.07 ppm, or 70 ppb) can be proposed based on the available toxicology data.
3.4. OELs established by regulatory agencies and authoritative bodies for 2,3-pentanedione
Three OELs were identified for 2,3-pentanedione (NIOSH Citation2016; DFG Citation2020; DGUV IFA Citation2021). According to DGUV IFA (Citation2021), an OEL of 0.02 ppm is established in Switzerland although the source documentation that supports this value was not identified.
The source document for the derivation of the German MAK value (“maximale Arbeitsplatz‐Konzentration,” or maximum workplace concentration) of 0.02 ppm is written in German. Based on translation of the text using a web-based translation service, it appears as though the MAK value of 0.02 ppm for 2,3-pentanedione was established based on its similarity to diacetyl in terms of toxic effects in the lung in humans, and the derivation of a MAK value for 0.02 ppm for diacetyl that was based on human occupational exposure data.
As summarized below, NIOSH (Citation2016) recommended an exposure limit for diacetyl of 5 ppb (0.005 ppm) based on longitudinal analysis of pulmonary function impairment at a workplace producing microwave popcorn in which workers were exposed to diacetyl. Comparable longitudinal analyses for pulmonary function in relation to 2,3-pentanedione exposure do not appear to have been published. The NIOSH (Citation2016) recommended exposure limit for 2,3-pentanedione is 9.3 ppb (0.0093 ppm), which is based on analytical limitations for measuring 2,3-pentanedione. Indeed, the recommendation in NIOSH (Citation2016) is to keep “[…] occupational exposure to 2,3-pentanedione below a level comparable to the level recommended for diacetyl,” and it is stated on page 173 of NIOSH (Citation2016) that the exposure limit for 2,3-pentanedione “[…] would be identical to that for diacetyl but is slightly higher based upon the limitations of the analytical method.” Thus, it appears that, if it were allowed by analytical capabilities, the value of 5 ppb (0.005 ppm) for diacetyl that was established by NIOSH (Citation2016) also would have been established for 2,3-pentanedione. Strengths and weaknesses of the NIOSH (Citation2016) recommended exposure limit of 5 ppb (0.005 ppm) for diacetyl are outlined below.
3.5. OELs established by regulatory agencies and authoritative bodies for diacetyl
Established OELs ranging from 0.01 to 0.02 ppm were identified for diacetyl in the DGUV IFA (Citation2021) database, and additional OELs ranging from 0.005 to 0.02 ppm were identified in other sources (NIOSH Citation2016; EU Citation2017; DFG Citation2020; HSE 2020; Ontario Ministry of Labour Citation2020; ACGIH Citation2021). Source documents outlining the derivation of the OELs were identified and obtained only for NIOSH (Citation2016), EU (Citation2017), and DFG (2020) as these represent national/multinational regulatory or authoritative bodies as opposed to regional regulatory bodies or scientific organizations.
3.5.1. NIOSH
NIOSH (Citation2016) considered nonclinical and human exposure data in deriving the recommended exposure limit for diacetyl. Based on the modeling of 13-week mouse and rat inhalation toxicity data, and extrapolating the calculated benchmark concentrations to human-equivalent concentrations, NIOSH (Citation2016) reported candidate recommended exposure limit values for diacetyl ranging from 0.04 to 4.0 ppm. The lowest candidate recommended exposure limit of 0.04 ppm was based on chronic lung inflammation in female mice while the highest candidate recommended exposure limit of 4.0 ppm was based on nasal respiratory epithelium necrosis in male mice. The lowest candidate recommended exposure limit based on rat data was 0.06 ppm, which was based on eosinophilic lung inflammation in males.
In evaluating human exposure data, NIOSH ultimately derived a recommended exposure limit of 5 ppb (0.005 ppm) based on longitudinal analysis of pulmonary function impairment at a workplace producing microwave popcorn in which workers were exposed to diacetyl and several other volatile organic compounds (Kreiss et al. Citation2002). The workplace is referred to in NIOSH (Citation2016) as “Company G” or “Plant G.” NIOSH selected the risk estimates from this data set as the basis for a recommended exposure limit because it was the most extensive and representative set of diacetyl exposure data and the largest body of respiratory outcomes data (NIOSH Citation2016). According to NIOSH (Citation2016), employees exposed to diacetyl at this level for 8 h a day, 40 h a week for a 45-year working lifetime should have no more than a 1/1000 excess risk of lung function falling below the lower limit of normal due to diacetyl exposure.
A significant strength of the data set that was used by NIOSH (Citation2016) was that a total of 9 evaluations were conducted at Company G that involved the collection and analysis of 262 personal samples and 346 area samples over a period of approximately 2.7 years. Moreover, although problems due to humidity and elapsed time to sample extraction were uncovered with the diacetyl sample determination method that was used at the time of sampling (NIOSH Method 2557), these were acknowledged, and corrections/adjustments to the exposure data were made by NIOSH (Citation2016) during the data review and assessment; the corrections/adjustments were made according to the method of Cox-Ganser et al. (Citation2011). However, for diacetyl concentrations reported to be below the limit of detection, the sample value was set equal to one half the limit of detection. This was the case for 40% (105 of 262) of the personal samples and 42% (146 of 346) of the area samples. As summarized by EC (Citation2014), this is problematic because Ashley et al. (Citation2008) performed a direct comparison of National Institute for Occupational Safety and Health (NIOSH) Method 2557 with more recent Occupational Safety and Health Administration (OSHA)-based methods and demonstrated that the average underestimation of exposure by NIOSH Method 2557 for concentrations below the limit of detection appears to be by a factor of approximately 20 (with a range from 4.2 to 295). This means that the 105 personal samples and 146 area samples that were assigned values of one half the limit of detection for statistical purposes may in fact have had higher values that should have been used for evaluation purposes. The lack of these higher values in the overall evaluation by NIOSH (Citation2016) may have skewed the data set toward a lower recommended exposure limit than otherwise would have been derived, and this represents a weakness of this approach.
3.5.2. EU
The basis for the EU (Citation2017) exposure limit of 0.02 ppm for diacetyl is summarized in EC (Citation2014). Upon review of available animal and human data, the exposure limit was established based on decreased lung function associated with exposure to diacetyl in the same workplace (“Company G”) that formed the basis for the NIOSH (Citation2016) recommended exposure limit. The difference is that the EC (Citation2014), EU (Citation2017) limit of 0.02 ppm was derived based on the data in the article published by Kreiss et al. (Citation2002) in which the authors reported a correlation between the quartile of estimated cumulative exposure to diacetyl and the frequency and extent of airway obstruction. The exposure quartiles were derived for 117 employees with a median time of employment of 3.4 years by multiplying the time each employee spent at their job by the mean exposure in that job area as measured using NIOSH Method 2557.
The prevalence of airway obstruction and proportion of workers with abnormal spirometry results increased with increasing cumulative diacetyl exposure, and a corresponding decrease in average FEV1 was observed. More specifically, the FEV1 value in the first exposure quartile was approximately 97% of predicted, and this decreased with each successive quartile; in the second, third, and fourth quartiles the average FEV1 was 4.5, 8.9, and 12.5% lower, respectively, than in the first quartile. The cutoff points for the quartiles were reported to be 0.65, 4.5, and 11 ppm-years, meaning that exposures in the first quartile ranged from 0 to 0.65 ppm-years. Based on these data, 0.65 ppm-years was considered by EC (Citation2014) to represent a NOAEC for the observed decrement in lung function.
The NOAEC of 0.65 ppm-years was based on uncorrected air measurements made using NIOSH Method 2557, which has recognized limitations as noted earlier. As such, this value was multiplied by a “correction factor” of 3 for analytical bias, divided by 40 years (assumed working lifetime), and divided by a factor of 2 for possible sensitive subgroups to derive a recommended 8-h exposure limit of 0.02 ppm (EC Citation2014; EU Citation2017). The “correction factor” of 3 for analytical bias was based on an assessment by Ashley et al. (Citation2008) in which an average ratio of 3.64 was calculated for diacetyl concentrations measured using more recent OSHA methods to those measured using the older NIOSH Method 2557 with its associated limitations related to humidity and sample storage time, particularly at lower diacetyl concentrations.
The EC (Citation2014), EU (Citation2017) exposure limit of 0.02 ppm was based on an assumed NOAEC for diacetyl regarding lung function decline (Kreiss et al. Citation2002), thus providing a degree of confidence in its derivation, although the study sample size of 117 subjects was somewhat smaller than what was used in the NIOSH (Citation2016) evaluation. Nonetheless, an adjustment to the assumed NOAEC was made to account for the deficiencies in the analytical method that was used in the study, and an additional adjustment was applied to account for potential sensitive subgroups that may be exposed to diacetyl. Both these adjustment factors are considered appropriate and strengthen the confidence in the derived exposure limit.
3.5.3. DFG
According to the source document (The MAK‐Collection for Occupational Health and Safety Citation2016), the German MAK value of 0.02 ppm was derived based on consideration of the same workplace data reported by Kreiss et al. (Citation2002) and on additional workplace data reported by Lockey et al. (Citation2009). Specifically, the value of 0.65 ml/m3 × year [reported as 0.65 ppm-years in Kreiss et al. (Citation2002)] was assumed as the NOAEC, as described earlier, and was divided by 40 years to derive an exposure limit of 0.016 ml/m3. From the study by Lockey et al. (Citation2009), a value of 0.8 ml/m3 × year (0.8 ppm-year) was assumed as the NOAEC for effects on FEV1 and vital capacity among 725 full-time employees at 4 microwave popcorn production facilities who underwent spirometry testing between 2005 and 2006. This value was divided by 40 years to derive an exposure limit of 0.02 ml/m3.
The 725 employees evaluated in the Lockey et al. (Citation2009) study represent a much larger sample size than the 117 employees evaluated by Kreiss et al. (Citation2002), and yet the assumed NOAECs and the exposure limits derived from these studies are comparable. This lends strength to the derived MAK value of 0.02 ppm (DFG Citation2020) that was derived from the results of these studies. Although NIOSH Method 2557 was used in both studies, neither of these calculations included an adjustment for analytical bias, nor was any adjustment made for sensitive subgroups. Thus, the calculated exposure values of 0.016 and 0.02 ml/m3, and the MAK value of 0.02 ml/m3 (0.02 ppm), are potentially artificially low due to the recognized bias in the diacetyl analytical method that was used in the studies by Kreiss et al. (Citation2002) and Lockey et al. (Citation2009), as described earlier. The source document acknowledges that the actual exposures in the studies were higher than reported but notes that a fixed correction factor cannot be applied to adjust for the uncertainty. Based on these data, a value of 0.02 ml/m3, or 20 ppb (0.02 ppm), was adopted as the MAK value for diacetyl (DFG Citation2020).
3.5.4. Published studies for diacetyl
Three published articles were identified in which OELs for diacetyl have been proposed (Maier et al. Citation2010; Egilman et al. Citation2011; Beckett et al. Citation2019).
Egilman et al. (Citation2011) proposed an OEL of 0.001 ppm (1 ppb) for diacetyl based on an evaluation and critique of several sources of information available at the time. These sources included the report by Maier et al. (Citation2010) described below, several epidemiological studies in the published literature, and a confidential quantitative structure activity relationship analysis that purportedly demonstrated diacetyl to have a comparable toxicity to that of isocyanates. A majority of the article is focused toward explaining and critiquing the OEL derived by Maier et al. (Citation2010) (referred to as “TERA” throughout the article, which is the company name). A primary shortcoming, according to Egilman et al. (Citation2011), was the reliance of TERA on a single study in mice for the derivation of the OEL, and dismissal of epidemiological data as insufficiently robust, with the exception of a single study in microwave popcorn plant workers (Lockey et al. Citation2009), which Egilman et al. (Citation2011) notes was not used by TERA to derive the OEL. Based on the epidemiology data reviewed, Egilman et al. (Citation2011) determined that the safe exposure level (OEL) should be much lower as evidenced by the reported 5.7-fold increased risk of obstructive lung disease among the mixers with powered air-purifying respirators from absolute exposure levels ranging from 0.015 to 0.044 ppm in the Lockey et al. (Citation2009) epidemiology study.
Maier et al. (Citation2010) proposed an OEL of 0.2 ppm (200 ppb) for diacetyl based on the results of an inhalation toxicity study in male mice (Morgan et al. Citation2008). In that study, male C57Bl/6 mice were exposed to diacetyl by inhalation for 6 or 12 weeks (6 h/day, 5 days/week) at concentrations of 0 (control), 25, 50, or 100 ppm. The critical effect was reported as peribronchial lymphocytic inflammation, which was observed after 6 weeks in 0/5, 3/5, 5/5, and 5/5 mice at 0, 25, 50, and 100 ppm, respectively, and after 12 weeks in 0/5, 2/5, 4/5, and 5/5 mice at 0, 25, 50, and 100 ppm, respectively. Maier et al. (Citation2010) combined the incidence data for the 6- and 12-week time points and calculated a BMDL10 value of 1.98 ppm for this effect. Adjustment of the BMDL10 was performed to account for the 6 h/day mouse study exposure scenario versus an 8 h/day workplace exposure scenario, and to account for dosimetry differences between species using a computational fluid dynamics model developed for rats and humans (but applied here for mice). These adjustments resulted in a human-equivalent concentration of 1.8 ppm that was rounded up to 2 ppm. The human-equivalent concentration of 2 ppm was then adjusted by a composite uncertainty factor of 10 to account for interspecies extrapolation (reported as a factor of 3) and human variability (reported as a factor of 3), resulting in a proposed OEL of 0.2 ppm. It can be argued that additional adjustments should be made to account for potential cumulative effects over a longer duration and the lower number of animals per group than recommended in Organization for Economic Co-operation and Development (OECD) guidance. More criticisms are discussed in Egilman et al. (Citation2011).
More recently, Beckett et al. (Citation2019) proposed an OEL of 0.2 ppm for diacetyl based on the results of the 2-year NTP inhalation carcinogenicity study in rats. In that study, male and female Wistar-Han rats were exposed to diacetyl by inhalation for 104 weeks (6 h/day, 5 days/week) at concentrations of 0 (control), 12.5, 25, or 50 ppm. The critical effect was reported as “bronchiole, epithelium, hyperplasia” for which a BMDL10 of 23.14 ppm was calculated for male and female rats combined (the authors noted that similar values were derived for each sex individually, such that combining the data provided a larger sample size and greater statistical power). Adjustment of the BMDL10 was performed to account for the 6 h/day rat study exposure scenario versus an 8 h/day workplace exposure scenario, and to account for dosimetry differences between species using a computational fluid dynamics model developed for rats and humans. In addition, an adjustment was made to account for an estimated 6 h/day of nose breathing and 2 h/day of mouth breathing during light exercise in the workplace. These adjustments resulted in a human-equivalent concentration of 1.24 ppm. The human-equivalent concentration of 1.24 ppm was then adjusted by a composite uncertainty factor of 8 to account for interspecies extrapolation (reported as a factor of 2.5) and human variability (reported as a factor of 3.2), resulting in a proposed OEL of 0.16 ppm which was rounded to 0.2 ppm. Like Maier et al. (Citation2010), Beckett et al. (Citation2019) dismiss the epidemiology data as the basis of an OEL, noting that many of the proposed OELs based on human data are lower than diacetyl concentrations released from brewing coffee (up to 0.016 ppm) and lower than concentrations in expelled air from humans (up to 0.19 ppm), although the latter may be due to the oral cavity serving as a reservoir for diacetyl from butter since it was not detected in the blood of healthy volunteers (Mochalski et al. (Citation2013). Furthermore, the more recent chronic NTP studies, conducted according to standard methodology, which Beckett et al. (Citation2019) used to derive an OEL, did not have the shortcomings of the 12-week study in mice that was available to Egilman et al. (Citation2011).
3.6. Comparison of proposed OEL for 2,3-pentandione to existing limits
Based on draft raw data from the 90-day inhalation toxicity studies conducted by the NTP, a candidate OEL of 70 ppb (0.07 ppm) can be proposed for 2,3-pentanedione. This OEL is based on the lowest BMDL10 value calculated from the data in mice and rats (1.42 ppm for hyperplasia of nasal respiratory epithelium in male rats) and therefore offers the best possibility of being protective of effects of 2,3-pentanedione that might occur in all regions of the respiratory system.
Cumulatively, the information summarized in this report supports the conclusion that the mechanistic and in vitro effects of 2,3-pentanedione and diacetyl are comparable and that these α-diketones have similar toxicity profiles in the respiratory system of mice and rats. As a result, in the absence of human exposure data upon which to base an OEL for 2,3-pentanedione, regulatory and authoritative bodies have treated occupational exposure to it in the same manner as for diacetyl, for which longitudinal human exposure and lung function data have been used to establish OELs. It is noted, however, that analytical bias that was present in the workplace studies upon which the existing diacetyl OELs were established have not been properly addressed. Specifically, concentrations above the limit of detection were appropriately adjusted per the method of Cox-Ganser et al. (Citation2011) to account for recognized humidity and sampling time effects on diacetyl concentrations. However, concentrations reported to be below the limit of detection were assigned a value of one half the limit of detection, which based on the work of Ashley et al. (Citation2008) may represent an average underestimation of approximately 20-fold. This has likely skewed the OELs for diacetyl (and by extension 2,3-pentanedione) to values that are lower than required. Moreover, draft raw data from 90-day inhalation toxicity studies with 2,3-pentanedione in mice and rats are recently available and can inform on a toxicology-based OEL for this substance.
A summary of the established regulatory/authoritative OELs for 2,3-pentanedione and diacetyl and the candidate OEL that was derived based on the 90-day inhalation toxicity study data is presented in .
Table 5. OELs for 2,3-pentanedione and diacetyl.
The data in demonstrate a range of OELs for 2,3-pentanedione from 0.0093 to 0.07 ppm (9.3 to 70 ppb). The lower end of this range (0.0093 ppm, or 9.3 ppb) represents the analytical limit for measurement of 2,3-pentanedione, and it is stated in NIOSH (Citation2016) that the OEL would be even lower (0.005 ppm, or 5 ppb) if analytical capabilities were available to measure at this level. Notably, therefore, the NIOSH (Citation2016) OEL of 0.0093 ppm, which is intended to be as close as possible to the diacetyl OEL of 0.005 ppm, and the DFG (2020) MAK value of 0.02 ppm (20 ppb), are both based, at least in part, on assigning a value of one half the limit of detection for concentrations reported to be below the limit of detection, which based on the work of Ashley et al. (Citation2008) may represent an average underestimation of approximately 20-fold. As such, they are considered artificially low and likely overly conservative. The proposed OEL for 2,3-pentanedione of 0.07 ppm (70 ppb) that is based on data from the NTP 90-day inhalation toxicity studies in mice and rats is 3.5- to 7.5-fold higher than the established regulatory/authoritative OELs of 0.02 ppm and 0.0093 ppm, respectively. Moreover, because it is derived from the lowest BMDL10 of those derived from the mouse and rat 90-day study data, the proposed OEL of 0.07 ppm is anticipated to be protective against adverse effects of 2,3-pentanedione that might occur in all regions of the respiratory system.
The cumulative information presented in this report suggests that there is support for a higher OEL for 2,3-pentanedione than has been established by regulatory/authoritative bodies to date, and that a value of 0.07 ppm (70 ppb) can be proposed based on the results of 90-day inhalation toxicity studies in mice and rats.
4. Conclusion
Several studies have evaluated the repeat-dose toxicity of diacetyl and 2,3-pentanedione in mice and rats following inhalation exposures for varying durations, and therefore allow for direct comparison (while carcinogenicity studies are available for diacetyl, there are no 2,3-pentanedione chronic/carcinogenicity studies to allow for a direct comparison). The most thorough of these studies were the 3-month studies conducted by the NTP, and the respiratory system histopathology findings from these studies were tabulated and summarized to allow for direct comparison of the effects of diacetyl and 2,3-pentanedione following exposure for the same duration in both species. Based on calculation of BMDL10 values for sensitive endpoints (those for which responses to diacetyl or 2,3-pentanedione were observed beginning at or before the mid-concentration of 25 ppm and continuing in a concentration-dependent pattern) in mice, it appears as though the nose and lung were generally more sensitive to 2,3-pentanedione whereas the larynx and trachea were generally more sensitive to diacetyl. In rats, the nose and larynx were generally more sensitive to 2,3-pentanedione than to diacetyl, although no BMD modeling was performed for endpoints in the rat trachea or lung. Notably, the only histopathology endpoint that was modeled as a sensitive endpoint for both species (“nose, respiratory epithelium, metaplasia, squamous”) was found to be more sensitive to 2,3-pentanedione than to diacetyl in mice (lower BMDL10 values in males and females) while the opposite was observed in rats.
Collectively, along with information from short-term studies summarized in the published literature, the respiratory system histopathology data from the 3-month NTP studies in mice and rats and the results of the BMD modeling that was performed for the most sensitive endpoints demonstrate comparable responses to both test articles, with no consistent overall pattern of greater sensitivity to diacetyl or 2,3-pentanedione.
Based on draft raw data from the 90-day inhalation toxicity studies conducted by the NTP, a candidate TWA OEL of 70 ppb (0.07 ppm) can be proposed for 2,3-pentanedione. This OEL is based on the lowest BMDL10 value calculated from the data in mice and rats (1.42 ppm for hyperplasia of nasal respiratory epithelium in male rats) and therefore offers the best possibility of being protective against adverse effects of 2,3-pentanedione that might occur in all regions of the respiratory system. Notable is that the selected BMDL10 value for 2,3-pentanedione was lower than any modeled value for diacetyl, and given the body of evidence that the potency of effects of both are comparable, the proposed OEL could be viewed as protective for both compounds.
On the basis of the modeling, the proposed OEL for 2,3-pentanedione is expected to be protective of employees who are working in the industry in combination with other good manufacturing practices for flavoring and food production.
A limitation of this work is that the data upon which the candidate OEL for 2,3-pentanedione of 70 ppb (0.07 ppm) are based are draft raw data that had not been formally published at the time this work was performed. In addition, the data are from 90-day studies whereas longer (2-year) studies of inhalation exposure to diacetyl in mice and rats have recently been performed and reported (NTP Citation2018). Thus, the work described in this manuscript may be revisited and updated when additional data for 2,3-pentanedione become available.
It is noted that although the toxicology data for acetoin are limited, in the available 3-month studies, in contrast to the data for diacetyl and 2,3-pentanedione, no adverse respiratory effects were observed following exposure to acetoin up to the highest concentration tested (800 ppm). The available data therefore indicates that acetoin does not share the same hazard as the α-diketones diacetyl and 2,3-pentanedione.
Supplemental Material
Download MS Word (62.1 KB)Acknowledgments
The authors would like to acknowledge the contributions of Heidi Kuang and Barry Lynch.
Declaration of interest
Jeffrey W. Card, Kevin M. Scaife and Lois A. Haighton are employees of Intertek Health Sciences Inc. Intertek Health Sciences Inc. has provided consulting services to General Mills. This research is important as a contribution to the safety of workers given that past exposures to diacetyl have been associated with serious respiratory disease. The authors have not participated in and do not anticipate participation in any legal, regulatory, or advocacy proceedings related to the contents of the paper. The authors had sole responsibility for the manuscript and the opinions and conclusions expressed in the article are those of the authors and are independent of entities providing financial support. General Mills provided funding for this study.
Data availability statement
The authors confirm that the data supporting the findings of this study are available within the article and/or its supplementary materials.
References
- ACGIH. 2021. Diacetyl. In: 2021 TLVs® and BEIs® based on the documentation of the threshold limit values (TLVs) for chemical substances and physical agents & biological exposure indices. Cincinnati (OH): American Conference of Governmental Industrial Hygienists (ACGIH). p. 25, 67, 267.
- Akpinar-Elci M, Travis WD, Lynch DA, Kreiss K. 2004. Bronchiolitis obliterans syndrome in popcorn production plant workers. Eur Respir J. 24(2):298–302.
- Anders MW. 2017. Diacetyl and related flavorant α-diketones: biotransformation, cellular interactions, and respiratory-tract toxicity. Toxicology. 388:21–29.
- Ashley K, McKernan LT, Burroughs E, Deddens J, Pendergrass S, Streicher RP. 2008. Analytical performance criteria. Field evaluation of diacetyl sampling and analytical methods. J Occup Environ Hyg. 5(11):D111–D116.
- Bailey RL, Cox-Ganser JM, Duling MG, LeBouf RF, Martin SB, Jr.Bledsoe TA, Green BJ, Kreiss K. 2015. Respiratory morbidity in a coffee processing workplace with sentinel obliterative bronchiolitis cases. Am J Ind Med. 58(12):1235–1245.
- Beasley RF, Sun J, Kelly FL, Martinu T, Morgan DL, Palmer SM. 2013. New artificial butter flavoring, 2,3-pentanedione, induces similar amphiregulin and inflammatory cytokine interleukin 8 production by pulmonary epithelial cells as diacetyl. Am J Respir Crit Care Med. 187: a4729.
- Beckett EM, Cyrs WD, Abelmann A, Monnot AD, Gaffney SH, Finley BL. 2019. Derivation of an occupational exposure limit for diacetyl using dose-response data from a chronic animal inhalation exposure study. J Appl Toxicol. 39(5):688–701.
- Brass DM, Palmer SM. 2017. Models of toxicity of diacetyl and alternative diones. Toxicology. 388:15–20.
- Cichocki JA, Morris JB. 2017. Inhalation dosimetry modelling provides insights into regional respiratory tract toxicity of inhaled diacetyl. Toxicology. 388:30–39.
- Cox-Ganser J, Ganser G, Saito R, Hobbs G, Boylstein R, Hendricks W, Simmons M, Eide M, Kullman G, Piacitelli C. 2011. Correcting diacetyl concentrations from air samples collected with NIOSH method 2557. J Occup Environ Hyg. 8(2):59–70.
- Dankovic DA, Naumann BD, Maier A, Dourson ML, Levy LS. 2015. The scientific basis of uncertainty factors used in setting occupational exposure limits. J Occup Environ Hyg. 12(sup1):S55–S68.
- DFG. 2020. 2,3-Pentanedione; diacetyl. In: List of MAK and BAT values 2020: maximum concentrations and biological tolerance values at the workplace (Report 56). Weinhiem, Germany: Wiley-VCH Verlag GmbH & Co. KGaA/Bonn; Germany: Deutsche Forschungsgemeinschaft, Permanent Senate Commission for the Investigation of Health Hazards of Chemical Compounds in the Work Area.
- DGUV IFA. 2021. 2,3-Pentanedione; diacetyl. In: GESTIS – International limit values for chemical agents (Occupational Exposure Limits, OELs). Berlin, Germany: institut für Arbeitsschutz der Deutschen Gesetzlichen Unfallversicherung (DGUV IFA).
- EC. 2014. Recommendation from the Scientific Committee on Occupational Exposure Limits for diacetyl. Brussels, Belgium: European Commission, Scientific Committee on Occupational Exposure Limits (SCOEL) (SCOEL/SUM/149).
- Egilman DS, Schilling JH, Menendez L. 2011. A proposal for a safe exposure level for diacetyl. Int J Occup Environ Health. 17(2):122–134.
- Epperly BR, Dekker EE. 1989. Inactivation of Escherichia coli L-threonine dehydrogenase by 2,3-butanedione. Evidence for a catalytically essential arginine residue. J Biol Chem. 264(31):18296–18301.
- EU. 2017. Commission Directive (EU) 2017/164 of 31 January 2017 establishing a fourth list of indicative occupational exposure limit values pursuant to Council Directive 98/24/EC, and amending Commission Directives 91/322/EEC, 2000/39/EC and 2009/161/EU. Off J Eur Union. 60(L27):115–120.
- Fedan JS, Thompson JA, Zaccone EJ, Hubbs AF. 2011. Complex profile of mechanical responses of guinea-pig isolated airways to the popcorn butter flavorings, diacetyl and 2,3-pentanedione. Am J Respir Crit Care Med. 183: a 3250–A3250.
- Flake GP, Morgan DL. 2017. Pathology of diacetyl and 2,3-pentanedione airway lesions in a rat model of obliterative bronchiolitis. Toxicology. 388:40–47.
- Flake GP, Kirby PJ, Price HC, Gage PS, Kelly FL, Palmer SM, Foley JF, Morgan DL. 2010. Bronchiolitis obliterans-like lesions in rats treated with diacetyl, acetoin, or acetyl propionyl by intratracheal instillation. Toxicol Sci. 114(Toxicologist suppl 1):316.
- Gerloff J, Sundar IK, Freter R, Sekera ER, Friedman AE, Robinson R, Pagano T, Rahman I. 2017. Inflammatory response and barrier dysfunction by different e-cigarette flavoring chemicals identified by gas chromatography-mass spectrometry in e-liquids and e-vapors on human lung epithelial cells and fibroblasts. Appl in Vitro Toxicol. 3(1):28–40.
- Gloede E, Cichocki JA, Baldino JB, Morris JB. 2011. A validated hybrid computational fluid dynamics-physiologically based pharmacokinetic model for respiratory tract vapor absorption in the human and rat and its application to inhalation dosimetry of diacetyl. Toxicol Sci. 123(1):231–246.
- Harvey RR, Blackley BH, Korbach EJ, Rawal AX, Roggli VL, Bailey RL, Cox-Ganser JM, Cummings KJ. 2021. Case report: flavoring-related lung disease in a coffee roasting and packaging facility worker with unique lung histopathology compared with previously described cases of obliterative bronchiolitis. Front Public Health. 9:657987.
- Harvey RR, Fechter-Leggett ED, Bailey RL, Edwards NT, Fedan KB, Virji MA, Nett RJ, Cox-Ganser JM, Cummings KJ. 2020. The burden of respiratory abnormalities among workers at coffee roasting and packaging facilities. Front Public Health. 8:5–9.
- Harber P, Saechao K, Boomus C. 2006. Diacetyl-induced lung disease. Toxicol Rev. 25(4):261–272.
- HSE. 2020. Diacetyl. In: EH40/2005 workplace exposure limits: containing the list of workplace exposure limits for use with the control of substances hazardous to health regulations 2002 (as amended), 4th edition. Bootle, Merseyside, UK: Health and Safety Executive (HSE)/Norwich, Norfolk, UK: The Stationery Office.
- Hubbs AF, Cumpston AM, Goldsmith WT, Battelli LA, Kashon ML, Jackson MC, Frazer DG, Fedan JS, Goravanahally MP, Castranova V, et al. 2012. Respiratory and olfactory cytotoxicity of inhaled 2,3-pentanedione in Sprague-Dawley rats. Am J Pathol. 181(3):829–844.
- Hubbs AF, Kreiss K, Cummings KJ, Fluharty KL, O'Connell R, Cole A, Dodd TM, Clingerman SM, Flesher JR, Lee R, et al. 2019. Flavorings-related lung disease: a brief review and new mechanistic data. Toxicol Pathol. 47(8):1012–1026.
- Kanwal R, Kullman G, Piacitelli C, Boylstein R, Sahakian N, Martin S, Fedan K, Kreiss K. 2006. Evaluation of flavorings-related lung disease risk at six microwave popcorn plants. J Occup Environ Med. 48(2):149–157.
- Kelly FL, Kirby PJ, Price HC, Gage PS, Flake GP, Morgan DL, Palmer SM. 2010. A rodent model of toxin induced bronchiolitis obliterans. Am J Respir Crit Care Med. 181: a 1750–A1750.
- Kreiss K, Gomaa A, Kullman G, Fedan K, Simoes EJ, Enright PL. 2002. Clinical bronchiolitis obliterans in workers at a microwave-popcorn plant. N Engl J Med. 347(5):330–338.
- LeBouf RF, Blackley BH, Fortner AR, Stanton M, Martin SB, Groth CP, McClelland TL, Duling MG, Burns DA, Ranpara A, et al. 2020. Exposures and emissions in coffee roasting facilities and cafés: diacetyl, 2, 3-pentanedione, and other volatile organic compounds. Front Public Health. 8:561740.
- Lockey JE, Hilbert TJ, Levin LP, Ryan PH, White KL, Borton EK, Rice CH, McKay RT, LeMasters GK. 2009. Airway obstruction related to diacetyl exposure at microwave popcorn production facilities. Eur Respir J. 34(1):63–71.
- Maier A, Kohrman-Vincent M, Parker A, Haber LT. 2010. Evaluation of concentration-response options for diacetyl in support of occupational risk assessment. Regul Toxicol Pharmacol. 58(2):285–296.
- Mochalski P, King J, Klieber M, Unterkofler K, Hinterhuber H, Baumann M, Amann A. 2013. Blood and breath levels of selected volatile organic compounds in healthy volunteers. Analyst. 138(7):2134–2145.
- Morgan DL, Flake GP, Kirby PJ, Palmer SM. 2008. Respiratory toxicity of diacetyl in C57BL/6 mice. Toxicol Sci. 103(1):169–180.
- Morgan DL, Jokinen MP, Price HC, Gwinn WM, Palmer SM, Flake GP. 2012. Bronchial and bronchiolar fibrosis in rats exposed to 2,3-pentanedione vapors: implications for bronchiolitis obliterans in humans. Toxicol Pathol. 40(3):448–465.
- Morgan DL, Merrick BA, Gerrish KE, Stockton PS, Wang Y, Foley JF, Gwinn WM, Kelly FL, Palmer SM, Ton TV, et al. 2015. Gene expression in obliterative bronchiolitis-like lesions in 2,3-pentanedione-exposed rats. PLOS One. 10(2):e0118459.
- Morgan DL, Jokinen MP, Johnson CL, Price HC, Gwinn WM, Bousquet RW, Flake GP. 2016. Chemical reactivity and respiratory toxicity of the α-diketone flavoring agents: 2,3-butanedione, 2,3-pentanedione, and 2,3-hexanedione. Toxicol Pathol. 44(5):763–783.
- NIOSH. 2016. Criteria for a recommended standard: occupational exposure to diacetyl and 2,3-pentanedione. Cincinnati (OH): National Institute for Occupational Safety and Health (NIOSH)/Atlanta (GA): centers for Disease Control (CDC) (Publication No. 2016-111).
- NTP. 2018. NTP Technical report on the toxicology and carcinogenesis studies of 2,3-butanedione in Wistar Han [Crl: WI (Han)] rats and B6C3F1/N mice (inhalation studies). Research Triangle Park (NC): National Toxicology Program (NTP) (NTP Technical Report, No. 593).
- OARS/TERA. 2021. OARS/WEELTM table. Cincinnati (OH): Occupational Alliance for Risk Science (OARS)/Toxicology Excellence for Risk Assessment (TERA™).
- Ontario Ministry of Labour. 2020. Diacetyl. In: Current occupational exposure limits for Ontario workplaces required under Regulation 833. Toronto (ON): Ontario Ministry of Labour.
- OSHA. 2021. OSHA annotated table Z-1. In: Permissible exposure limits – annotated tables. Washington (DC): Occupational Safety and Health Administration (OSHA).
- Palmer SM, Flake GP, Kelly FL, Zhang HL, Nugent JL, Kirby PJ, Foley JF, Gwinn WM, Morgan DL. 2011. Severe airway epithelial injury, aberrant repair and bronchiolitis obliterans develops after diacetyl instillation in rats. PLOS One. 6(3):e17644.
- Park H-R, O'Sullivan M, Vallarino J, Shumyatcher M, Himes BE, Park J-A, Christiani DC, Allen J, Lu Q. 2019. Transcriptomic response of primary human airway epithelial cells to flavoring chemicals in electronic cigarettes. Sci Rep. 9(1):1400.
- Rim KT, Kim SJ. 2020. A toxicogenomics study of two chemicals in coffee roasting process. Mol Cell Toxicol. 16(1):25–38.
- The Japan Society for Occupational Health. 2020. Recommendation of occupational exposure limits (2020-2021). Environ Occup Health Pract. 2(1); 34 pp. https://www.sanei.or.jp/english/files/topics/oels/OEL.pdf.
- The MAK‐Collection for Occupational Health and Safety. 2016. Diacetyl. https://doi.org/10.1002/3527600418.mb43103e5816.
- U.S. EPA. 2012. Benchmark dose technical guidance. Washington (DC): U.S. Environmental Protection Agency, Risk Assessment Forum.
- van Rooy FG, Smit LA, Houba R, Zaat VA, Rooyackers JM, Heederik DJ. 2009. A cross-sectional study of lung function and respiratory symptoms among chemical workers producing diacetyl for food flavourings. Occup Environ Med. 66(2):105–110.
- van Rooy FG, Rooyackers JM, Prokop M, Houba R, Smit LA, Heederik DJ. 2007. Bronchiolitis obliterans syndrome in chemical workers producing diacetyl for food flavorings. Am J Respir Crit Care Med. 176(5):498–504.
- Virji MA, Cummings KJ, Cox-Ganser JM. 2021. A strategy for field evaluations of exposures and respiratory health of workers at small-to medium-sized coffee facilities. Front Public Health. 9:705225.
- WHO. 2020. Chapter 5: dietary exposure assessment of chemicals in food. In: principles and methods for the risk assessment of chemicals in food. 2nd edition. Rome, Italy: Food and Agriculture Organization of the United Nations (FAO)/Geneva, Switzerland: World Health Organization (WHO), International Programme on Chemical Safety (IPCS) (Environmental Health Criteria, No. 240).
- Zaccone EJ, Thompson JA, Ponnoth DS, Cumpston AM, Goldsmith WT, Jackson MC, Kashon ML, Frazer DG, Hubbs AF, Shimko MJ, et al. 2013. Popcorn flavoring effects on reactivity of rat airways in vivo and in vitro. J Toxicol Environ Health A. 76(11):669–689.
- Zaccone EJ, Goldsmith WT, Shimko MJ, Wells JR, Schwegler-Berry D, Willard PA, Case SL, Thompson JA, Fedan JS. 2015. Diacetyl and 2,3-pentanedione exposure of human cultured airway epithelial cells: ion transport effects and metabolism of butter flavoring agents. Toxicol Appl Pharmacol. 289(3):542–549.